- Laboratory of Malaria Research, Instituto Oswaldo Cruz, Fundação Oswaldo Cruz, Rio de Janeiro, Brazil
In the last decade it has become clear that, similarly to nucleated cells, enucleated red blood cells (RBCs) are susceptible to programmed apoptotic cell death. Erythrocytic apoptosis seems to play a role in physiological clearance of aged RBCs, but it may also be implicated in anemia of different etiological sources including drug therapy and infectious diseases. In malaria, severe anemia is a common complication leading to death of children and pregnant women living in malaria-endemic regions of Africa. The pathogenesis of malarial anemia is multifactorial and involves both ineffective production of RBCs by the bone marrow and premature elimination of non-parasitized RBCs, phenomena potentially associated with apoptosis. In the present overview, we discuss evidences associating erythrocytic apoptosis with the pathogenesis of severe malarial anemia, as well as with regulation of parasite clearance in malaria. Efforts to understand the role of erythrocytic apoptosis in malarial anemia can help to identify potential targets for therapeutic intervention based on apoptotic pathways and consequently, mitigate the harmful impact of malaria in global public health.
Introduction
Kerr and colleagues introduced the term apoptosis in 1972 to designate a physiologic process of programmed cell death implicated in normal maintenance of tissue cell population as well as in development of pathologies such as cancer. Since then, an enormous number of studies focusing on apoptosis have been published. These studies have focused on apoptosis of nucleated cells, whose dramatic changes occurring in the nucleus, i.e., chromatin condensation and DNA fragmentation (Kerr et al., 1972; Wyllie, 1980; Totino et al., 2006), labeled apoptosis as a nucleus-dependent stereotyped process. However, pioneer studies with experimentally enucleated cells demonstrated that typical cytoplasmic and cell membrane features of apoptosis, such as membrane blebbing, cell shrinkage, loss of mitochondrial transmembrane potential, and exposure of phosphatidylserine can be induced in the absence of a nucleus (Jacobson et al., 1994; Schulze-Osthoff et al., 1994; Castedo et al., 1996), raising the possibility that apoptosis could also determine the life span of physiologically enucleated cells, i.e., the red blood cells (RBC).
Indeed, even the lack of organelles in RBCs, including those directly implicated in apoptosis induction (i.e., mitochondria and endoplasmic reticulum), does not impair activation of the cytoplasmic machinery present in the cell that coordinates the apoptotic process (Bratosin et al., 2001; Lang et al., 2012). Although the pathways leading to apoptosis in RBC are not well known, they have been shown to be initiated by an increase in cytosolic Ca2+ due to activation of Ca2+ permeable non-selective cation channels in the cell membrane, which can be triggered by a variety of xenobiotics and endogenous substances (Lang et al., 2012). In turn, influx of Ca2+ leads to (1) activation of caspases and calpains, which participate in cell disassembly and formation of cell membrane blebbing; (2) cell shrinkage through exit of K+ via Ca2+-sensitive K+ channel; and (3) stimulation of cell membrane scrambling, resulting in phosphatidylserine (PS) exposure at the cell surface (Gao et al., 2012; Lang et al., 2012). This last event is critical for elimination of apoptotic cells, before cell disintegration, and subsequent release of cytoplasmic proinflammatory mediators, because PS works as an “eat me” signal for phagocytic cells that operate in an anti-inflammatory manner (Wu et al., 2006).
Similarly to apoptosis of nucleated cells, apoptosis of erythrocytes participates in the body homeostasis through physiological clearance of aged cells. It is estimated that 360 billion of senescent erythrocytes undergo apoptotic processes and are removed every day by the spleen, avoiding systemic complications related to intravascular hemolysis (Bratosin et al., 1998, 2009). On the other hand, apoptosis is also believed to contribute to anemia and vascular disorders associated with clinical conditions in which excessive rates of apoptotic RBCs are documented, including diabetes, renal insufficiency, sickle-cell anemia, chronic lead exposure (Lang et al., 2002; Myssina et al., 2003; Calderón-Salinas et al., 2011; Aguilar-Dorado et al., 2014) as well as infectious diseases such as sepsis and mycoplasmosis (Kempe et al., 2007; Felder et al., 2011).
In view of the pathophysiological significance of erythrocytic apoptosis, our group has attempted to study this erythrocytic process in malaria, a vector-borne infection caused by protozoa of the genus Plasmodium that infect RBCs and induce strong hematologic and vascular disturbances (Anstey et al., 2009; Grau and Craig, 2012; Carvalho et al., 2014). Our studies have shown that induction of apoptosis is not limited to parasitized RBCs (pRBCs) but also occurs in non-parasitized RBCs (nRBCs), pointing to an involvement of both pRBC and nRBC in the pathogenesis of malaria through deflagration of suicide processes (Totino et al., 2009, 2011, 2013, 2014). Thus, the present review covers evidence implicating erythrocytic apoptosis in the pathogenesis of severe anemia, a common complication of malaria that represents an important public health concern strongly related to mortality in children and pregnant women living in malaria-hyperendemic regions of sub-Saharan Africa (Schantz-Dunn and Nour, 2009; Muoneke et al., 2012).
Malarial Anemia and RBC Removal
It should not be a surprise that anemia is one of the most common disorders associated with malaria, because Plasmodium parasites develop an intraerythrocytic schizogony process culminating in lysis of RBCs. In non-immune children and adults infected with P. falciparum, the degree of anemia may correlate with parasitemia (Phillips and Pasvol, 1992; Biemba et al., 2000; Menendez et al., 2000) and in most experimental rodent malaria, acute anemia is a consequence of the rupture of high percentages of pRBCs (Lamikanra et al., 2007). Nevertheless, it is known that destruction of pRBCs alone cannot account for anemia severity in malaria patients (Ekvall, 2003; Akinosoglou et al., 2012). Acute anemia can be observed in both experimental and human infections presenting low parasitemia and even after antimalarial treatment and clearance of parasites, anemia can persist or worsen (Phillips et al., 1986; Camacho et al., 1998; Carvalho et al., 2003; Helleberg et al., 2005; Evans et al., 2006). In view of these facts, both ineffective production of RBCs by the bone marrow and premature elimination of nRBC have been postulated as important determinants of malaria anemia pathogenesis (Jakeman et al., 1999; Casals-Pascual et al., 2006; Evans et al., 2006; Thawani et al., 2014).
The extent of RBC elimination in malaria was reported in studies estimating that, for every pRBC, up to 32 nRBC are removed from circulation (Jakeman et al., 1999; Collins et al., 2003; Evans et al., 2006). In addition, there is evidence supporting that nRBC removal is mediated by phagocytic activity rather than intravascular hemolysis (Phillips et al., 1986; Abdalla, 1988; Goka et al., 2001; La Raja, 2002; Satpathy et al., 2004; Evans et al., 2006), highlighting the mononuclear phagocytic reticuloendothelial system as an important mechanism of malarial anemia pathogenesis. Indeed, besides directly participate in malarial anemia by uptake of both pRBCs and nRBCs, macrophages may also interfere with the erythropoietic process by limiting systemic bioavailability of recycled iron, the main source of which is erythrophagocytosis occurring in spleen and bone marrow (Beaumont and Delaby, 2009; Spottiswoode et al., 2014). However, the pathways underlying clearance of RBCs in malarial anemia remain elusive and therefore, apoptosis as a well-known mechanism of cell depletion participating in cytopenia of several pathologies could mediate elimination of nRBCs.
Malaria and Erythrocytic Apoptosis Inducers
Studies conducted by Dondorp et al. (1999) with P. falciparum-infected patients demonstrated that similarly to pRBCs, in which parasites induces drastic changes on the host cell membrane, nRBCs also present reduced deformability correlating with anemia severity. This phenomenon, also recorded in nRBC from P. falciparum in vitro culture and P. yoelii-infected mice (Paul et al., 2013; Huang et al., 2014), has been related to adsorption of malaria parasite antigens on the cell surface (Layez et al., 2005; Omodeo-Salè et al., 2005) and interestingly, more recently identified as a hallmark of erythrocytic apoptosis (Mindukshev et al., 2013), raising the possibility that antigens of malaria parasites have proapoptotic proprieties on RBCs.
Microbes in general could affect proapoptotic pathways in host cells. Gram-positive and gram-negative bacteria, as well as protozoan parasites, have been shown to manipulate apoptotic processes of both infected and non-infected host nucleated cells via pathogen antigens that act as apoptosis regulatory molecules through multifarious pathways, impacting on the outcome of disease (Ulett and Adderson, 2006; Rodrigues et al., 2012). A diversity of antigens produced by pathogenic bacteria such as enterotoxins, hemolysins, lethal toxins, lipotechoic acid, and lipopolysaccharide, have the ability to promote apoptosis in several types of host cells including T and B lymphocytes, dendritic cells, monocytes, macrophages, neutrophils, endothelial cells, epithelial cells (Ulett and Adderson, 2006; Carrero and Unanue, 2012). Similarly, malaria toxins glycosylphosphatidylinositol and hemozoin purified from P. falciparum showed proapoptotic activity in cardiomyocytes and erythroblasts, respectively (Wennicke et al., 2008; Lamikanra et al., 2009). Moreover, such apoptogenic effect of parasite factors was recorded by incubating brain vascular endothelial and neuroglia cells with P. falciparum-pRBC conditioned medium (Wilson et al., 2008), and induction of apoptosis in lung endothelial cells can additionally take place by physical contact due to pRBC adhesion phenomenon (Pino et al., 2003).
There are no studies further exploring the proapoptotic potential of plasmodial antigens in erythrocytic apoptosis. However, it was already reported that in P. falciparum in vitro cultures nRBCs are marked by PS externalization (Koka et al., 2007; Pattanapanyasat et al., 2010), and in our previous study with BALB/c mice infected by the lethal P. yoelii 17XL parasites, increased levels of nRBC apoptosis correlated with hiperparasitemia (Totino et al., 2013), indirectly suggesting that as do bacterial toxins (listeriolysin from Listeria monocytogenes and hemolysin from Vibrio parahaemolyticus), peptidoglycan, and Schistosoma mansoni egg antigens (Lang et al., 2004b; Föller et al., 2007; Kasinathan and Greenberg, 2010; Jilani et al., 2012; Abed et al., 2013), plasmodial antigens can play a role in induction of erythrocytic apoptosis.
Additionally, several host factors have the potential to modulate erythrocytic apoptosis during malaria (Lang and Lang, 2015). They may comprise: (1) proapoptotic factors whose increased levels are associated with malarial severity, such as soluble Fas ligand (sFasL), anti-erythrocyte antibodies, hematin, granzyme B, and oxidative stress (Das et al., 1993; Waitumbi et al., 2000; Hermsen et al., 2003; Issifou et al., 2003; Dalko et al., 2015) and; (2) antiapoptotic factors, i.e., erythropoietin, vitamin E, and nitric oxide, that show either reduced levels or low bioavability in malaria, and consequently, could contribute to amplify erythrocytic apoptosis induction (Burgmann et al., 1996; Kulkarni et al., 2003; Sobolewski et al., 2005).
It is also noteworthy that several routine therapeutic drugs present proapoptotic properties on erythrocytes, which has been linked to drug-associated anemia (Lang and Lang, 2015). In vitro studies performed by Alzoubi et al. (2014a,b) showed that the antimalarial drugs artesunate and lumefantrine have proapoptotic effect on erythrocytes, possibly through generation of oxidative stress, a feature already reported for both drugs (Xie et al., 2005; Abolaji et al., 2013). Thus, the proapoptotic effect of artesunate and lumefantrine could explain the reduction of erythrocytic blood parameters reported in healthy rats and humans (Xie et al., 2005; Kongpatanakul et al., 2009) as well as in occurrence of acute anemia in malarial patients following antimalarial drug treatment (De Nardo et al., 2013; Raffray et al., 2014; Sowunmi et al., 2015).
Removing nRBCs in Malaria by Apoptosis
Although it is known that sensitization of nRBCs by antibodies and complement system contributes to malarial anemia pathogenesis ((Daniel-Ribeiro et al., 1986); (Daniel-Ribeiro and Zanini, 2000; Waitumbi et al., 2000), there is evidence indicating that the elimination of nRBCs could also occur in an opsonin-independent manner. For instance, a lack of correlation between RBC sensitization and anemia degree has been reported in some studies with malarial patients (Abdalla, 1986; Merry et al., 1986) and a shortened lifespan of nRBCs was observed during P. yoelii induced-anemia in a B cell-deficient environment provided by severe combined immunodeficient mice (Salmon et al., 1997). In addition, in a recently described novel model of severe malarial anemia presenting low parasitemia, C3−/− mice still developed acute anemia following P. berghei infection (Harris et al., 2012).
The participation of a nonopsonic phagocytosis in nRBC elimination and its relation to apoptosis can be sustained by data concerning the scavenger receptor CD36 and PS. CD36 is a well-known phagocytic receptor for apoptotic cells that acts via recognition of PS on the apoptotic cell surface (Greenberg et al., 2006). Experiments with both monoclonal anti-CD36 antibodies and CD36-deficient rodent macrophages have shown that CD36 plays also a role in phagocytic uptake of RBC in malaria (McGilvray et al., 2000; Patel et al., 2004; Ayi et al., 2005). In these experiments, the contribution of parasite antigens with affinity to CD36 (e.g., P. falciparum erythrocyte membrane protein 1—PfEMP-1) on the pRBC surface cannot be ruled out, since they were performed with pRBC. Nevertheless, intraerythrocytic plasmodia development progressively induces PS exteriorization on pRBC as a result of non-selective cation channel activation in the host RBC membrane (Eda and Sherman, 2002; Lang et al., 2004a), indicating that pRBC could be removed via PS.
Importantly, PS has been recognized as a key molecule mediating erythrophagocytosis, as in vitro treatment with annexin V or PS-containing liposomes is able to inhibit phagocytosis of senescent or oxidatively stressed RBC, an effect also achieved with the use of anti-CD36 antibodies (Bratosin et al., 1997; Tait and Smith, 1999; Mandal et al., 2002). In a similar way, phagocytosis of RBC from uremic patients, with increased levels of PS-exposing RBC with great propensity for phagocytosis, was also inhibited after preincubation of macrophages with glycerophosphorylserine, a structural derivate of PS (Bonomini et al., 2001), indicating that PS can mediate shortened life span of RBC in pathological conditions in which anemia is a common feature. In this context, anemia has been associated with the rates of PS-exposing RBC in peritoneal dialysis and sepsis patients (Bi et al., 2006; Shi et al., 2013) as well as with erythrophagocytosis mediated by PS in rats treated with lead or naphthoquinone—two inducers of erythrocytic apoptosis (Noh et al., 2010; Jang et al., 2011).
Hence, PS exposure on nRBCs induced to apoptosis during malaria could also be involved in anemia development, as suggested initially by our studies on lethal P. yoelii experimental infection, in which the levels of PS-exposing nRBCs were increased during the stage of infection marked by an acute anemia (Totino et al., 2010). Both erythrophagocytosis and PS-exposing RBCs are increased in P. falciparum infected-children presenting severe anemia as compared to those with uncomplicated malaria (Fendel et al., 2010). Apoptosis can also be induced by incubating RBCs from healthy individuals with plasma from P. falciparum patients, an effect that is not observed with plasma from P. vivax infections, which, comparatively to P. falciparum, are less prone to lead to severe disease (Totino et al., 2014). Furthermore, since CD36 is a key molecule in the RBC clearance process, resistance to severe malarial anemia reported in children with a non-sense CD36 mutation can be explained, in part, by a deficiency in phagocytosis of apoptotic nRBCs mediated by CD36-PS interaction (Pain et al., 2001; Chilongola et al., 2009).
Recently, additional evidence for the contribution of PS to malarial anemia was reported in a study of mice infected with the nonlethal (17XNL) P. yoelii (Fernandez-Arias et al., 2016). In this model, as observed by our group with the lethal (17XL) strain P. yoelii (Totino et al., 2010), there was a significant increase in the levels of PS-exposing nRBCs. In addition, it was shown that P. yoelii infection induces autoantibodies against PS exposed on nRBCs and that these antibodies enhance anemia when transferred to infected mice, an effect related to in vitro nRBC phagocytosis. Importantly, anti-PS antibodies were also detected in sera from P. falciparum-infected patients and the highest levels were associated with late postmalarial anemia in nonimmune travelers. Therefore, besides participating in the pathogenesis of malarial anemia by directly promoting RBC elimination via the innate immune receptor CD36, PS may also contributes antigenically as a target of acquired immune response, a novel described autoimmune phenomenon in malaria whose source needs further investigation.
Another cell event that links erythrocytic apoptosis and anemia is a change in CD47 expression. CD47 is a ubiquitous glycoprotein expressed on the cell surface that was identified as a “marker of self” by suppressing phagocytosis of normal viable cells through interaction with signal-regulatory protein alpha (SIRPα), which operates as a inhibitory receptor on phagocytes (Sosale et al., 2015). Initially identified in studies demonstrating a rapid in vivo clearance of CD47-deficient RBCs and an increased in vitro phagocytosis of normal RBCs by macrophages treated with SIRPα-blocking antibodies, the CD47/SIRPα system of cell clearance was suggested to play role in anemia of Rhnull individuals, given that expression of CD47 on normal RBCs is coupled to Rh antigen complex (Oldenborg et al., 2000). Indeed, the involvement of CD47 in anemia was also observed in mild autoimmune hemolytic anemia typical of experimental nonobese diabetes, which was worsened and became lethal in CD47-deficient mice (Oldenborg et al., 2002) and in patients with myelodysplastic syndrome, the degree of anemia was correlated to the levels of CD47 expressed on erythroblasts (Jiang et al., 2013). Furthermore, down-regulation of surface CD47 occurring specifically on platelets was connected to thrombocytopenia of Escherichia coli infected-mice (Guo et al., 2009), supporting data suggesting that CD47 participates in cytopenia of different etiological sources through cell depletion processes.
Comprehensibly CD47/SIRPα self-recognition system has been implicated in clearance of apoptotic cells. It was demonstrated that apoptosis of neutrophils and Jurkat cells is followed by decreased expression of surface CD47 (Lawrence et al., 2009; Azuma et al., 2011), while in apoptosis of several other nucleated cell types CD47, initially clustered in lipid rafts, is diffused on the cell surface, reducing its avidity for SIRPα (Lv et al., 2015). In both cases, it is believed that the inhibitory signaling triggered by CD47 via phosphorylation of the SIRPA cytoplasmic tail is discontinued and consequently, apoptotic cells are phagocytized. Therefore, elimination of RBCs contributing to the pathogenesis of malarial anemia could also be a reflex of CD47 changes during the apoptosis process.
Reduction of surface CD47 expression has been observed on RBCs maintained under conditions that induce erythrocytic apoptosis, such as blood storage and hypoxia, as detected by externalization of PS (Stewart et al., 2005; Mao et al., 2011), and there is a consensus that in vivo elimination of senescent RBCs, which are marked by deflagration of apoptotic processes (Bosman et al., 2005), is regulated by CD47 (Khandelwal et al., 2007; Burger et al., 2012). Nevertheless, with the exception of studies with CD47-deficient mice and Rhnull individuals, those addressing a relationship between RBC CD47 and anemia are limited. Two studies on warm autoimmune hemolytic anemia showed no difference in CD47 expression when comparing RBCs from patients with those from healthy individuals, suggesting that CD47 does not play a role in the development of this pathology, in which autoantibodies are well known to be involved (Ahrens et al., 2006; Barros et al., 2009). However, it has been demonstrated that CD47, as a regulator of RBC clearance, is able to overwhelm phagocytosis mediated by antibody and complement (Oldenborg et al., 2001; Olsson and Oldenborg, 2008). Therefore, the contribution of CD47 to autoimmune anemia, possibly by means of its reorganization on cell surface (not assayed in the above mentioned studies), rather than by its downexpression, cannot be excluded.
In malaria, the role of CD47 has been recently addressed (Banerjee et al., 2015; Ayi et al., 2016). Parasites of the nonlethal strain of P. yoelii 17XNL were mostly detected infecting young RBCs expressing high levels of CD47, suggesting that malaria parasites could take advantage of negative signaling of CD47 to avoid early elimination by phagocytosis (Banerjee et al., 2015). The authors of this study also demonstrated that absence of this molecule in deficient mice attenuated the course of parasitemia and notably, converted the lethal infection of P. yoelii YM into a nonlethal, self-resolving infection characterized by a very low parasite load (peak of 0.06%). Similar results in a more recent study (Ayi et al., 2016) showed that CD47-deficiency attenuated parasitemia and abolished the development of cerebral malaria in P. berghei ANKA-infected mice, as well as P. falciparum-pRBC had decreased levels of CD47, a phenomenon that was associated with both in vitro and in vivo clearance of P. falciparum—and P. berghei-pRBCs via SIRPα, respectively.
These data raise the possibility that alteration of CD47 expression on apoptotic pRBCs operates as a regulator of malaria parasite clearance. Although elimination of pRBCs has a role in pathogenesis of malarial anemia, contrary to RBC lysis that allows the propagation of merozoites at the end of schizogony, RBC apoptosis could play a beneficial role leading intraerythrocytic-developing parasites to degradation by phagocytosis and consequently reducing parasite burden that directly promotes anemia through RBC lysis. This protective role of erythrocytic apoptosis was previously demonstrated by treating P. berghei-infected mice with the drug aurothiomalate, which triggers apoptosis selectively in pRBCs with no effect on intraerythrocytic parasite development (Alesutan et al., 2010). This in vivo stimulation of pRBC apoptosis delayed the course of parasitemia and mortality of mice, suggesting that the use of drugs to selectively trigger apoptosis pathways in pRBCs could be a strategy to treat malaria in a manner not propitious to generation of parasite resistance, since drugs would target the host cell and not the parasite.
While CD47 changes on pRBCs could play a protector effect, the same is not expected for nRBCs. As mentioned above, CD47 deficiency is associated with development of anemia as a result of increased susceptibility of CD47−/− RBC to phagocytosis under pathological circumstances. Thus, if on one hand RBC clearance promoted by the absence of CD47 attenuates malaria parasitemia (pRBC), on the other hand it leads to anemia (nRBC), as observed in CD47-deficient mice with P. berghei malaria (Ayi et al., 2016). This connection between CD47, nRBC and anemia was also confirmed in a study with wild type mice infected by P. yoelii 17XNL that found a decline in the number of nRBCs expressing high levels CD47 when RBC counts were falling. An increase in newly formed RBCs (reticulocytes) that express high levels of CD47 was detected later at the recovery stage (Fernandez-Arias et al., 2016), implicating CD47 in the pathogenesis of malarial anemia through elimination of nRBCs.
Paradoxically, deflagration of apoptosis in nRBCs could play a protective role in malaria infection. It is well-known that apoptotic processes represent an innate mechanism against intracellular parasitism that altruistically drives parasites to degradation by phagocytosis along with host parasitized cells, thereby preventing infection of adjacent cells and pathogen propagation (Williams, 1994). Alternatively, apoptosis could act in the control of parasitemia limiting the availability of non-parasitized targeted cells susceptible to infection, as shown in P. falciparum in vitro culture where apoptotic nRBCs were refractory to parasite invasion (Totino et al., 2011). However, this phenomenon offering as a host protective mechanism for parasite burden control in malaria while adversely induces anemia, needs to be better examined.
Apoptosis and Defective Erythropoiesis
Besides participating in the pathogenesis of malarial anemia by mediating precocious elimination of nRBC, apoptosis could interfere with the production of new RBCs, as previously reported in severe anemia associated to Leishmania donovani infection in golden hamsters (Lafuse et al., 2013) as well as in anemia of chronic disease in patients with rheumatoid arthritis (Papadaki et al., 2002), in which cytokine-mediated apoptosis of erythroid cells seems to operate.
Anemia in both malaria experimental models and human infection is followed by an unexpected frequency of peripheral reticulocytes considering the loss of RBCs, indicating that impaired erythropoietic activity is an important mechanism in the pathogenesis of malarial anemia (Chang et al., 2004; Leowattana et al., 2008; Fendel et al., 2010; Anyona et al., 2012; Thawani et al., 2014). Infection by P. chabaudi, P. yoelii, and P. berghei was shown to inhibit development of erythroid precursors induced by erythropoietin in mice (Thawani et al., 2014), an effect also achieved by in vitro incubation of human erythroid cells with hemozoin or proinflamatory cytokines such as TNF and IFN-γ, whose levels are augmented during malaria (Means and Krantz, 1991; Casals-Pascual et al., 2006; Awandare et al., 2011). In addition, in a study with children presenting several degrees of malarial anemia, ineffective erythropoiesis was associated with the amount of circulating hemozoin and TNF, and deposition of hemozoin in bone marrow was related to abnormal erythroid development in postmortem analysis of children with severe anemia (Casals-Pascual et al., 2006).
In this context, proapoptotic effects of hemozoin, TNF and interferon-γ on erythroid precursors have been described in vitro (Dai and Krantz, 1999; Lamikanra et al., 2009; Vittori et al., 2010) and high levels of apoptotic erythroid cells were observed in bone marrow aspirates of severe malaria patients presenting erythroid hypoplasia and low percentage of peripheral reticulocytes (Anantrakulsil et al., 2005). Nevertheless, the capacity of both TNF and hemozoin to depress erythropoiesis by inducing apoptosis has not been consensually demonstrated in vitro (Skorokhod et al., 2010; Awandare et al., 2011) and apoptosis was not implicated in impaired maturation of erythroblasts during anemia of experimental P. chabaudi infection (Chang et al., 2004).
These apparently contradictory results could be related to the differences between cell lines, since in vitro studies with hemozoin and TFN were performed with cells from different sources, i.e., erythroid cell lines or fresh isolated CD34+ stem cells from PBMC, which have been shown to exhibit different susceptibility to apoptosis induced by TNF or hemozoin (Lamikanra et al., 2009; Skorokhod et al., 2010; Vittori et al., 2010; Awandare et al., 2011). For instance, erythroid cells derived from human erythroleukaemia K562 cell line were susceptible to apoptosis induced by TNF, but not hemozoin, while those cells derived from normal peripheral blood CD34+ cells were only susceptible to hemozoin-induced apoptosis, suggesting that these compounds interfere in the development of erythroid cells through distinct pathways, with hemozoin inducing apoptosis and TNF triggering non-apoptotic mechanisms in healthy (non-leukemia) cells. Indeed, a gene expression profile study of purified primary erythroid cultures incubated with either TNF or hemozoin showed recently that both stimuli were able to inhibit erythroid development, with activation of largely distinct transcriptional programs: hemozoin up-regulated genes related to regulation of transcription, cellular stress response and apoptosis, and the majority of genes up-regulated by TNF was involved in innate and adaptive immune responses to infection (Lamikanra et al., 2015).
These data explain, at least in part, the lack of a relationship between apoptosis and depressed erythropoiesis in severe anemia of P. chabaudi infection in A/J mice (Chang et al., 2004), with TNF probably overwhelming the proapoptotic property of hemozoin. Although there are no studies concerning the effect of TNF on hemozoin-induced apoptosis, it has been shown that P. chabaudi infection in A/J mice is marked by increased levels of TNF at the time of decreased reticulocyte response (Jacobs et al., 1996). Additionally, these data illustrate the well-known concept of the complex multifactorial pathogenesis of malarial anemia, in which the molecular mechanisms regulating eythropoiesis in malaria are still poorly understood; a scenario that reflects a research gap that remains challenging due to practical and ethical difficulties in obtaining bone marrow samples from patients with severe malarial anemia, whose population majority comprises children of African endemic regions.
Concluding Remarks
It has become evident that deflagration of cell death processes by apoptosis is not restrict to nucleated cells, occurring also in enucleated RBCs, and consequently, contributing to the development of anemia through precocious elimination of RBCs. In malaria infection, erythrocytic apoptosis occurs and seems to play both detrimental and protective roles by inducing anemia and controlling parasitemia, respectively (Figure 1). Although the magnitude and dynamics of erythrocytic apoptosis in malaria are still to be better established, the above reported connections between apoptosis and anemia open new options for development of therapy for severe malarial anemia by exploring the apoptotic machinery, as considered in other pathologies related to increased induction of apoptosis such as Alzheimer's disease, Parkinson's disease, and sepsis (Jana and Paliwal, 2007; Hattori et al., 2010). Advances that allow therapy improvement for severe malaria anemia are, indeed, urgently needed. The use of adjunctive therapy for severe malarial anemia has not been well explored, possibly due to the common requirement for blood transfusion. However, the prevalence of blood-borne infections such as HIV in malaria endemic areas limits the availability and safety of blood transfusion (Osaro and Charles, 2011). Furthermore, blood transfusion shows a trend toward presentation of severe adverse events and does not significantly decrease mortality rates, underscoring the need for new strategies for treating severe malarial anemia (Meremikwu and Smith, 2000; Obonyo et al., 2007). Thus, inhibition of cell signaling pathways leading to erythrocytic apoptosis could be explored as an alternative strategy to mitigate the impact of severe anemia in mortality caused by malaria.
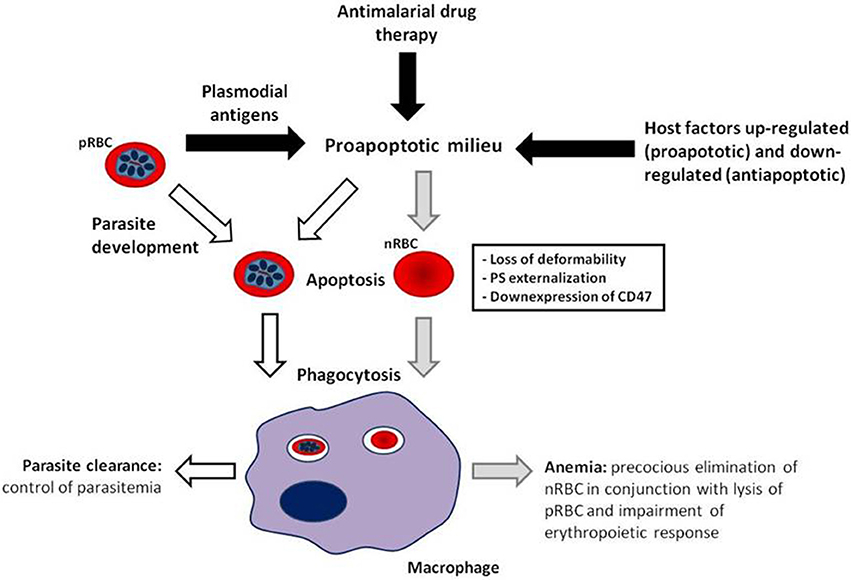
Figure 1. Model of erythrocytic apoptosis involvement in the pathogenesis of malarial anemia. Both parasitic antigens and host factors modulated by malaria infection generate a favorable environment for precocious induction of erythrocytic apoptosis (black arrows), which can be enhanced by antimalarial drug treatment, and in the case of parasitized red blood cells (pRBCs), by intraerythrocytic parasite development. Erythrocytic apoptosis provokes cellular changes, i.e., cell rigidity increase, exposure of phosphatidylserine and downexpression of CD47, that drive pRBC (white arrows) and non-parasitized RBC (nRBC; gray arrows) to phagocytosis. Elimination of apoptotic nRBCs along with pRBC lysis and erythropoiesis impairment contribute to the development of malarial anemia. Uptake of apoptotic pRBCs can help control parasite load. In this manner, the pathogenic or protective role of erythrocytic apoptosis in malaria could be a result of increased apoptosis induction differentially in nRBC and pRBC, respectively.
Author Contributions
PT wrote the manuscript; CD-R and MF critically revised it for intellectual content. All authors read and approved the final manuscript.
Conflict of Interest Statement
The authors declare that the research was conducted in the absence of any commercial or financial relationships that could be construed as a potential conflict of interest.
Acknowledgments
This work was supported by Instituto Oswaldo Cruz-Fiocruz; Fundação de Amparo à Pesquisa do Estado do Rio de Janeiro (Faperj) and; Conselho Nacional de Desenvolvimento Científico e Tecnológico (CNPq). CD-R and MF are recipients of a Research Productivity Fellowship from CNPq and Full Scientists of the Faperj.
References
Abdalla, S. H. (1986). Red cell associated IgG in patients suffering from Plasmodium falciparum malaria. Br. J. Haematol. 62, 13–19. doi: 10.1111/j.1365-2141.1986.tb02895.x
Abdalla, S. H. (1988). Peripheral blood and bone marrow leucocytes in Gambian children with malaria: numerical changes and evaluation of phagocytosis. Ann. Trop. Paediatr. 8, 250–258. doi: 10.1080/02724936.1988.11748582
Abed, M., Towhid, S. T., Pakladok, T., Alesutan, I., Götz, F., Gulbins, E., et al. (2013). Effect of bacterial peptidoglycan on erythrocyte death and adhesion to endothelial cells. Int. J. Med. Microbiol. 303, 182–189. doi: 10.1016/j.ijmm.2013.01.004
Abolaji, A. O., Eteng, M. U., Omonua, O., and Adenrele, Y. (2013). Influence of coadministration of artemether and lumefantrine on selected plasma biochemical and erythrocyte oxidative stress indices in female Wistar rats. Hum. Exp. Toxicol. 32, 206–215. doi: 10.1177/0960327112464666
Aguilar-Dorado, I. C., Hernández, G., Quintanar-Escorza, M. A., Maldonado-Vega, M., Rosas-Flores, M., and Calderón-Salinas, J. V. (2014). Eryptosis in lead-exposed workers. Toxicol. Appl. Pharmacol. 281, 195–202. doi: 10.1016/j.taap.2014.10.003
Ahrens, N., Pagenkopf, C., Kiesewetter, H., and Salama, A. (2006). CD47 is expressed at normal levels in patients with autoimmune haemolytic anaemia and/or immune thrombocytopenia. Transfus. Med. 16, 397–402. doi: 10.1111/j.1365-3148.2006.00688.x
Akinosoglou, K. S., Solomou, E. E., and Gogos, C. A. (2012). Malaria: a haematological disease. Hematology 17, 106–114. doi: 10.1179/102453312X13221316477336
Alesutan, I., Bobbala, D., Qadri, S. M., Estremera, A., Föller, M., and Lang, F. (2010). Beneficial effect of aurothiomalate on murine malaria. Malar. J. 9:118. doi: 10.1186/1475-2875-9-118
Alzoubi, K., Alktifan, B., Oswald, G., Fezai, M., Abed, M., and Lang, F. (2014a). Breakdown of phosphatidylserine asymmetry following treatment of erythrocytes with lumefantrine. Toxins 6, 650–664. doi: 10.3390/toxins6020650
Alzoubi, K., Calabrò, S., Bissinger, R., Abed, M., Faggio, C., and Lang, F. (2014b). Stimulation of suicidal erythrocyte death by artesunate. Cell. Physiol. Biochem. 34, 2232–2244. doi: 10.1159/000369666
Anantrakulsil, S., Maneerat, Y., Wilairatana, P., Krudsood, S., Arunsuriyasak, C., Atichartakarn, V., et al. (2005). Hematopoietic feature and apoptosis in the bone marrow of severe Plasmodium falciparum-infected patients: preliminary study. Southeast Asian J. Trop. Med. Public Health 36, 543–551.
Anstey, N. M., Russell, B., Yeo, T. W., and Price, R. N. (2009). The pathophysiology of vivax malaria. Trends Parasitol. 25, 220–227. doi: 10.1016/j.pt.2009.02.003
Anyona, S. B., Kempaiah, P., Raballah, E., Davenport, G. C., Were, T., Konah, S. N., et al. (2012). Reduced systemic bicyclo-prostaglandin-E2 and cyclooxygenase-2 gene expression are associated with inefficient erythropoiesis and enhanced uptake of monocytic hemozoin in children with severe malarial anemia. Am. J. Hematol. 87, 782–789. doi: 10.1002/ajh.23253
Awandare, G. A., Kempaiah, P., Ochiel, D. O., Piazza, P., Keller, C. C., and Perkins, D. J. (2011). Mechanisms of erythropoiesis inhibition by malarial pigment and malaria-induced proinflammatory mediators in an in vitro model. Am. J. Hematol. 86, 155–162. doi: 10.1002/ajh.21933
Ayi, K., Lu, Z., Serghides, L., Ho, J. M., Finney, C., Wang, J. C., et al. (2016). CD47-SIRPα interactions regulate macrophage uptake of Plasmodium falciparum-infected erythrocytes and clearance of malaria in vivo. Infect. Immun. 84, 2002–2011. doi: 10.1128/IAI.01426-15
Ayi, K., Patel, S. N., Serghides, L., Smith, T. G., and Kain, K. C. (2005). Nonopsonic phagocytosis of erythrocytes infected with ring-stage Plasmodium falciparum. Infect. Immun. 73, 2559–2563. doi: 10.1128/IAI.73.4.2559-2563.2005
Azuma, Y., Nakagawa, H., Dote, K., Higai, K., and Matsumoto, K. (2011). Decreases in CD31 and CD47 levels on the cell surface during etoposide-induced Jurkat cell apoptosis. Biol. Pharm. Bull. 34, 1828–1834. doi: 10.1248/bpb.34.1828
Banerjee, R., Khandelwal, S., Kozakai, Y., Sahu, B., and Kumar, S. (2015). CD47 regulates the phagocytic clearance and replication of the Plasmodium yoelii malaria parasite. Proc. Natl. Acad. Sci. U.S.A. 112, 3062–3067. doi: 10.1073/pnas.1418144112
Barros, M. M. O., Yamamoto, M., Figueiredo, M. S., Cançado, R., Kimura, E. Y., Langhi, D. M. Jr., et al. (2009). Expression levels of CD47, CD35, CD55, and CD59 on red blood cells and signal-regulatory protein-alpha, beta on monocytes from patients with warm autoimmune hemolytic anemia. Transfusion 49, 154–160. doi: 10.1111/j.1537-2995.2008.01936.x
Beaumont, C., and Delaby, C. (2009). Recycling iron in normal and pathological states. Semin. Hematol. 46, 328–338. doi: 10.1053/j.seminhematol.2009.06.004
Bi, S. H., Cheng, L. T., and Wang, T. (2006). The role of erythrocytes phosphatidylserine exposure in anemia in peritoneal dialysis patients. Ren. Fail. 28, 573–576. doi: 10.1080/08860220600839647
Biemba, G., Dolmans, D., Thuma, P. E., Weiss, G., and Gordeuk, V. R. (2000). Severe anaemia in Zambian children with Plasmodium falciparum malaria. Trop. Med. Int. Health 5, 9–16. doi: 10.1046/j.1365-3156.2000.00506.x
Bonomini, M., Sirolli, V., Reale, M., and Arduini, A. (2001). Involvement of phosphatidylserine exposure in the recognition and phagocytosis of uremic erythrocytes. Am. J. Kidney Dis. 37, 807–814. doi: 10.1016/S0272-6386(01)80130-X
Bosman, G. J., Willekens, F. L., and Werre, J. M. (2005). Erythrocyte aging: a more than superficial resemblance to apoptosis? Cell. Physiol. Biochem. 16, 1–8. doi: 10.1159/000087725
Bratosin, D., Estaquier, J., Petit, F., Arnoult, D., Quatannens, B., Tissier, J. P., et al. (2001). Programmed cell death in mature erythrocytes: a model for investigating death effector pathways operating in the absence of mitochondria. Cell Death Differ. 8, 1143–1156. doi: 10.1038/sj.cdd.4400946
Bratosin, D., Mazurier, J., Tissier, J. P., Estaquier, J., Huart, J. J., Ameisen, J. C., et al. (1998). Cellular and molecular mechanisms of senescent erythrocyte phagocytosis by macrophages. A review. Biochimie 80, 173–195. doi: 10.1016/S0300-9084(98)80024-2
Bratosin, D., Mazurier, J., Tissier, J. P., Slomianny, C., Estaquier, J., Russo-Marie, F., et al. (1997). Molecular mechanisms of erythrophagocytosis. Characterization of the senescent erythrocytes that are phagocytized by macrophages. C. R. Acad. Sci. III 320, 811–818. doi: 10.1016/S0764-4469(97)85017-2
Bratosin, D., Tcacenco, L., Sidoroff, M., Cotoraci, C., Slomianny, C., Estaquier, J., et al. (2009). Active caspases-8 and -3 in circulating human erythrocytes purified on immobilized annexin-V: a cytometric demonstration. Cytometry A 75, 236–244. doi: 10.1002/cyto.a.20693
Burger, P., de Korte, D., van den Berg, T. K., and van Bruggen, R. (2012). CD47 in erythrocyte ageing and clearance – the Dutch point of view. Transfus. Med. Hemother. 39, 348–352. doi: 10.1159/000342231
Burgmann, H., Looareesuwan, S., Kapiotis, S., Viravan, C., Vanijanonta, S., Hollenstein, U., et al. (1996). Serum levels of erythropoietin in acute Plasmodium falciparum malaria. Am. J.Trop. Med. Hyg. 54, 280–283.
Calderón-Salinas, J. V., Muñoz-Reyes, E. G., Guerrero-Romero, J. F., Rodríguez-Morán, M., Bracho-Riquelme, R. L., Carrera-Gracia, M. A., et al. (2011). Eryptosis and oxidative damage in type 2 diabetic mellitus patients with chronic kidney disease. Mol. Cell. Biochem. 357, 171–179. doi: 10.1007/s11010-011-0887-1
Camacho, L. H., Gordeuk, V. R., Wilairatana, P., Pootrakul, P., Brittenham, G. M., and Looareesuwan, S. (1998). The course of anaemia after the treatment of acute, falciparum malaria. Ann. Trop. Med. Parasitol. 92, 525–537. doi: 10.1080/00034983.1998.11813310
Carrero, J. A., and Unanue, E. R. (2012). Mechanisms and immunological effects of apoptosis caused by Listeria monocytogenes. Adv. Immunol. 113, 157–174. doi: 10.1016/B978-0-12-394590-7.00001-4
Carvalho, L. J., Alves, F. A., de Oliveira, S. G., do Valle Rdel, R., Fernandes, A. A., Muniz, J. A., et al. (2003). Severe anemia affects both splenectomized and non- splenectomized Plasmodium falciparum-infected Aotus infulatus monkeys. Mem. Inst. Oswaldo Cruz 98, 679–686. doi: 10.1590/S0074-02762003000500016
Carvalho, L. J., Moreira Ada, S., Daniel-Ribeiro, C. T., and Martins, Y. C. (2014). Vascular dysfunction as a target for adjuvant therapy in cerebral malaria. Mem. Inst. Oswaldo Cruz 109, 577–588. doi: 10.1590/0074-0276140061
Casals-Pascual, C., Kai, O., Cheung, J. O. P., Williams, S., Lowe, B., Nyanoti, M., et al. (2006). Suppression of erythropoiesis in malarial anemia is associated with hemozoin in vitro and in vivo. Blood 108, 2569–2577. doi: 10.1182/blood-2006-05-018697
Castedo, M., Hirsch, T., Susin, S. A., Zamzami, N., Marchetti, P., Macho, A., et al. (1996). Sequential acquisition of mitochondrial and plasma membrane alterations during early lymphocyte apoptosis. J. Immunol. 157, 512–521.
Chang, K. H., Tam, M., and Stevenson, M. M. (2004). Inappropriately low reticulocytosis in severe malarial anemia correlates with suppression in the development of late erythroid precursors. Blood 103, 3727–3735. doi: 10.1182/blood-2003-08-2887
Chilongola, J., Balthazary, S., Mpina, M., Mhando, M., and Mbugi, E. (2009). CD36 deficiency protects against malarial anaemia in children by reducing Plasmodium falciparum-infected red blood cell adherence to vascular endothelium. Trop. Med. Int. Health 14, 810–816. doi: 10.1111/j.1365-3156.2009.02298.x
Collins, W. E., Jeffery, G. M., and Roberts, J. M. (2003). A retrospective examination of anemia during infection of humans with Plasmodium vivax. Am. J. Trop. Med. Hyg. 68, 410–412.
Dai, C., and Krantz, S. B. (1999). Interferon gamma induces upregulation and activation of caspases 1, 3, and 8 to produce apoptosis in human erythroid progenitor cells. Blood 93, 3309–3316.
Dalko, E., Das, B., Herbert, F., Fesel, C., Pathak, S., Tripathy, R., et al. (2015). Multifaceted role of heme during severe Plasmodium falciparum infections in India. Infect. Immun. 83, 3793–3799. doi: 10.1128/IAI.00531-15
Daniel-Ribeiro, C. T., Banic, D. M., Issa-Ahmed, I., and Galvão-Castro, B. (1986). Polyclonal B-lymphocyte activation and sensitization of erythrocytes by IgG in human malaria: relevance to the development of anaemia in a holoendemic area in northwestern Brazil (Ariquemes - Rondônia). Mem. Inst. Oswaldo Cruz 81, 169–176. doi: 10.1590/S0074-02761986000600028
Daniel-Ribeiro, C. T., and Zanini, G. (2000). Autoimmunity and malaria: what are they doing together? Acta Trop. 76, 205–221. doi: 10.1016/S0001-706X(00)00099-1
Das, B. S., Patnaik, J. K., Mohanty, S., Mishra, S. K., Mohanty, D., Satpathy, S. K., et al. (1993). Plasma antioxidants and lipid peroxidation products in falciparum malaria. Am. J. Trop. Med. Hyg. 49, 720–725.
De Nardo, P., Oliva, A., Giancola, M. L., Ghirga, P., Mencarini, P., Bibas, M., et al. (2013). Haemolytic anaemia after oral artemether-lumefantrine treatment in a patient affected by severe imported falciparum malaria. Infection 41, 863–865. doi: 10.1007/s15010-013-0451-x
Dondorp, A. M., Angus, B. J., Chotivanich, K., Silamut, K., Ruangveerayuth, R., Hardeman, M. R., et al. (1999). Red blood cell deformability as a predictor of anemia in severe falciparum malaria. Am. J. Trop. Med. Hyg. 60, 733–737.
Eda, S., and Sherman, I. W. (2002). Cytoadherence of malaria-infected red blood cells involves exposure of phosphatidylserine. Cell. Physiol. Biochem. 12, 373–384. doi: 10.1159/000067908
Ekvall, H. (2003). Malaria and anemia. Curr. Opin. Hematol. 10, 108–114. doi: 10.1097/00062752-200303000-00002
Evans, K. J., Hansen, D. S., van Rooijen, N., Buckingham, L. A., and Schofield, L. (2006). Severe malarial anemia of low parasite burden in rodent models results from accelerated clearance of uninfected erythrocytes. Blood 107, 1192–1199. doi: 10.1182/blood-2005-08-3460
Felder, K. M., Hoelzle, K., Ritzmann, M., Kilchling, T., Schiele, D., Heinritzi, K., et al. (2011). Hemotrophic mycoplasmas induce programmed cell death in red blood cells. Cell. Physiol. Biochem. 27, 557–564. doi: 10.1159/000329957
Fendel, R., Brandts, C., Rudat, A., Kreidenweiss, A., Steur, C., Appelmann, I., et al. (2010). Hemolysis is associated with low reticulocyte production index and predicts blood transfusion in severe malarial anemia. PLoS ONE 5:e10038. doi: 10.1371/journal.pone.0010038
Fernandez-Arias, C., Rivera-Correa, J., Gallego-Delgado, J., Rudlaff, R., Fernandez, C., Roussel, C., et al. (2016). Anti-self phosphatidylserine antibodies recognize uninfected erythrocytes promoting malarial anemia. Cell Host Microbe 19, 194–203. doi: 10.1016/j.chom.2016.01.009
Föller, M., Shumilina, E., Lam, R., Mohamed, W., Kasinathan, R., Huber, S., et al. (2007). Induction of suicidal erythrocyte death by listeriolysin from Listeria monocytogenes. Cell. Physiol. Biochem. 20, 1051–1060. doi: 10.1159/000110715
Gao, M., Cheung, K. L., Lau, I. P., Yu, W. S., Fung, K. P., Yu, B., et al. (2012). Polyphyllin D induces apoptosis in human erythrocytes through Ca2+ rise and membrane permeabilization. Arch. Toxicol. 86, 741–752. doi: 10.1007/s00204-012-0808-4
Goka, B. Q., Kwarko, H., Kurtzhals, J. A., Gyan, B., Ofori-Adjei, E., Ohene, S. A., et al. (2001). Complement binding to erythrocytes is associated with macrophage activation and reduced haemoglobin in Plasmodium falciparum malaria. Trans. R. Soc. Trop. Med. Hyg. 95, 545–549. doi: 10.1016/S0035-9203(01)90036-7
Grau, G. E., and Craig, A. G. (2012). Cerebral malaria pathogenesis: revisiting parasite and host contributions. Future Microbiol. 7, 291–302. doi: 10.2217/fmb.11.155
Greenberg, M. E., Sun, M., Zhang, R., Febbraio, M., Silverstein, R., and Hazen, S. L. (2006). Oxidized phosphatidylserine-CD36 interactions play an essential role in macrophage-dependent phagocytosis of apoptotic cells. J. Exp. Med. 203, 2613–2625. doi: 10.1084/jem.20060370
Guo, Y. L., Liu, D. Q., Bian, Z., Zhang, C. Y., and Zen, K. (2009). Down-regulation of platelet surface CD47 expression in Escherichia coli O157:H7 infection-induced thrombocytopenia. PLoS ONE 4:e7131. doi: 10.1371/journal.pone.0007131
Harris, J. V., Bohr, T. M., Stracener, C., Landmesser, M. E., Torres, V., Mbugua, A., et al. (2012). Sequential Plasmodium chabaudi and Plasmodium berghei infections provide a novel model of severe malarial anemia. Infect. Immun. 80, 2997–3007. doi: 10.1128/IAI.06185-11
Hattori, Y., Takano, K., Teramae, H., Yamamoto, S., Yokoo, H., and Matsuda, N. (2010). Insights into sepsis therapeutic design based on the apoptotic death pathway. J. Pharmacol. Sci. 114, 354–365. doi: 10.1254/jphs.10R04CR
Helleberg, M., Goka, B. Q., Akanmori, B. D., Obeng-Adjei, G., Rodriques, O., and Kurtzhals, J. A. (2005). Bone marrow suppression and severe anaemia associated with persistent Plasmodium falciparum infection in African children with microscopically undetectable parasitaemia. Malar. J. 4:56. doi: 10.1186/1475-2875-4-56
Hermsen, C. C., Konijnenberg, Y., Mulder, L., Loé, C., van Deuren, M., van der Meer, J. W., et al. (2003). Circulating concentrations of soluble granzyme A and B increase during natural and experimental Plasmodium falciparum infections. Clin. Exp. Immunol. 132, 467–472. doi: 10.1046/j.1365-2249.2003.02160.x
Huang, S., Amaladoss, A., Liu, M., Chen, H., Zhang, R., Preiser, P. R., et al. (2014). In vivo splenic clearance correlates with in vitro deformability of red blood cells from Plasmodium yoelii-infected mice. Infect. Immun. 82, 2532–2541. doi: 10.1128/IAI.01525-13
Issifou, S., Mavoungou, E., Borrmann, S., Bouyou-Akotet, M. K., Matsiegui, P. B., and Kremsner, P. G. (2003). Severe malarial anemia associated with increased soluble Fas ligand (sFasL) concentrations in Gabonese children. Eur. Cytokine Netw. 14, 238–241.
Jacobs, P., Radzioch, D., and Stevenson, M. M. (1996). A Th1-associated increase in tumor necrosis factor alpha expression in the spleen correlates with resistance to blood-stage malaria in mice. Infect. Immun. 64, 535–541.
Jacobson, M. D., Burne, J. F., and Raff, M. C. (1994). Programmed cell death and Bcl-2 protection in the absence of a nucleus. EMBO J. 13, 1899–1910.
Jakeman, G. N., Saul, A., Hogarth, W. L., and Collins, W. E. (1999). Anaemia of acute malaria infections in non-immune patients primarily results from destruction of uninfected erythrocytes. Parasitology 119, 127–133. doi: 10.1017/S0031182099004564
Jana, S., and Paliwal, J. (2007). Apoptosis: potential therapeutic targets for new drug discovery. Curr. Med. Chem. 14, 2369–2379. doi: 10.2174/092986707781745569
Jang, W. H., Lim, K. M., Kim, K., Noh, J. Y., Kang, S., Chang, Y. K., et al. (2011). Low level of lead can induce phosphatidylserine exposure and erythrophagocytosis: a new mechanism underlying lead-associated anemia. Toxicol. Sci. 122, 177–184. doi: 10.1093/toxsci/kfr079
Jiang, H., Fu, R., Wang, H., Li, L., Liu, H., and Shao, Z. (2013). CD47 is expressed abnormally on hematopoietic cells in myelodysplastic syndrome. Leuk. Res. 37, 907–910. doi: 10.1016/j.leukres.2013.04.008
Jilani, K., Lupescu, A., Zbidah, M., Abed, M., Shaik, N., and Lang, F. (2012). Enhanced apoptotic death of erythrocytes induced by the mycotoxin ochratoxin A. Kidney Blood Press. Res. 36, 107–118. doi: 10.1159/000341488
Kasinathan, R. S., and Greenberg, R. M. (2010). Schistosoma mansoni soluble egg antigens trigger erythrocyte cell death. Cell. Physiol. Biochem. 26, 767–774. doi: 10.1159/000322344
Kempe, D. S., Akel, A., Lang, P. A., Hermle, T., Biswas, R., Muresanu, J., et al. (2007). Suicidal erythrocyte death in sepsis. J. Mol. Med. 85, 273–281. doi: 10.1007/s00109-006-0123-8
Kerr, J. F., Wyllie, A. H., and Currie, A. R. (1972). Apoptosis: a basic biological phenomenon with wide-ranging implications in tissue kinetics. Br. J. Cancer 26, 239–257. doi: 10.1038/bjc.1972.33
Khandelwal, S., van Rooijen, N., and Saxena, R. K. (2007). Reduced expression of CD47 during murine red blood cell (RBC) senescence and its role in RBC clearance from the circulation. Transfusion 47, 1725–1732. doi: 10.1111/j.1537-2995.2007.01348.x
Koka, S., Huber, S. M., Boini, K. M., Lang, C., Föller, M., and Lang, F. (2007). Lead decreases parasitemia and enhances survival of Plasmodium berghei-infected mice. Biochem. Biophys. Res. Commun. 363, 484–489. doi: 10.1016/j.bbrc.2007.08.173
Kongpatanakul, S., Chatsiricharoenkul, S., Khuhapinant, A., Atipas, S., and Kaewkungwal, J. (2009). Comparative study of dihydroartemisinin and artesunate safety in healthy Thai volunteers. Int. J. Clin. Pharmacol. Ther. 47, 579–586. doi: 10.5414/CPP47579
Kulkarni, A. G., Suryakar, A. N., Sardeshmukh, A. S., and Rathi, D. B. (2003). Studies on biochemical changes with special reference to oxidant and antioxidants in malaria patients. Indian J. Clin. Biochem. 18, 136–149. doi: 10.1007/BF02867380
Lafuse, W. P., Story, R., Mahylis, J., Gupta, G., Varikuti, S., Steinkamp, H., et al. (2013). Leishmania donovani infection induces anemia in hamsters by differentially altering erythropoiesis in bone marrow and spleen. PLoS ONE 8:e59509. doi: 10.1371/journal.pone.0059509
Lamikanra, A. A., Brown, D., Potocnik, A., Casals-Pascual, C., Langhorne, J., and Roberts, D. J. (2007). Malarial anemia: of mice and men. Blood 110, 18–28. doi: 10.1182/blood-2006-09-018069
Lamikanra, A. A., Merryweather-Clarke, A. T., Tipping, A. J., and Roberts, D. J. (2015). Distinct mechanisms of inadequate erythropoiesis induced by tumor necrosis factor alpha or malarial pigment. PLoS ONE 10:e0119836. doi: 10.1371/journal.pone.0119836
Lamikanra, A. A., Theron, M., Kooij, T. W. A., and Roberts, D. J. (2009). Hemozoin (malaria pigment) directly promotes apoptosis of erythroid precursors. PLoS ONE 4:e8446. doi: 10.1371/journal.pone.0008446
Lang, E., and Lang, F. (2015). Triggers, inhibitors, mechanisms, and significance of eryptosis: the suicidal erythrocyte death. BioMed. Res. Int. 2015:513518. doi: 10.1155/2015/513518
Lang, E., Qadri, S. M., and Lang, F. (2012). Killing me softly – suicidal erythrocyte death. Int. J. Biochem. Cell Biol. 44, 1236–1243. doi: 10.1016/j.biocel.2012.04.019
Lang, F., Lang, P. A., Lang, K. S., Brand, V., Tanneur, V., Duranton, C., et al. (2004a). Channel-induced apoptosis of infected host cells-the case of malaria. Pflugers Arch. 448, 319–324. doi: 10.1007/s00424-004-1254-9
Lang, K. S., Roll, B., Myssina, S., Schittenhelm, M., Scheel-Walter, H. G., Kanz, L., et al. (2002). Enhanced erythrocyte apoptosis in sickle cell anemia, thalassemia and glucose-6-phosphate dehydrogenase deficiency. Cell. Physiol. Biochem. 12, 365–372. doi: 10.1159/000067907
Lang, P. A., Kaiser, S., Myssina, S., Birka, C., Weinstock, C., Northoff, H., et al. (2004b). Effect of Vibrio parahaemolyticus haemolysin on human erythrocytes. Cell. Microbiol. 6, 391–400. doi: 10.1111/j.1462-5822.2004.00369.x
La Raja, M. (2002). Erythrophagocytosis by peripheral monocytes in Plasmodium falciparum malaria. Haematologica 87:EIM14.
Lawrence, D. W., King, S. B., Frazier, W. A., and Koenig, J. M. (2009). Decreased CD47 expression during spontaneous apoptosis targets neutrophils for phagocytosis by monocyte-derived macrophages. Early Hum. Dev. 85, 659–663. doi: 10.1016/j.earlhumdev.2009.09.005
Layez, C., Nogueira, P., Combes, V., Costa, F. T., Juhan-Vague, I., da Silva, L. H., et al. (2005). Plasmodium falciparum rhoptry protein RSP2 triggers destruction of the erythroid lineage. Blood 106, 3632–3638. doi: 10.1182/blood-2005-04-1574
Leowattana, W., Krudsood, S., Tangpukdee, N., Brittenham, G., and Looareesuwan, S. (2008). Defective erythropoietin production and reticulocyte response in acute Plasmodium falciparum malaria-associated anemia. Southeast Asian J. Trop. Med. Public Health 39, 581–588.
Lv, Z., Bian, Z., Shi, L., Niu, S., Ha, B., Tremblay, A., et al. (2015). Loss of cell surface CD47 clustering formation and binding avidity to SIRPα facilitate apoptotic cell clearance by macrophages. J. Immunol. 195, 661–671. doi: 10.4049/jimmunol.1401719
Mandal, D., Moitra, P. K., Saha, S., and Basu, J. (2002). Caspase 3 regulates phosphatidylserine externalization and phagocytosis of oxidatively stressed erythrocytes. FEBS Lett. 513, 184–188. doi: 10.1016/S0014-5793(02)02294-9
Mao, T. Y., Fu, L. L., and Wang, J. S. (2011). Hypoxic exercise training causes erythrocyte senescence and rheological dysfunction by depressed Gardos channel activity. J. Appl. Physiol. 111, 382–391. doi: 10.1152/japplphysiol.00096.2011
McGilvray, I. D., Serghides, L., Kapus, A., Rotstein, O. D., and Kain, K. C. (2000). Nonopsonic monocyte/macrophage phagocytosis of Plasmodium falciparum-parasitized erythrocytes: a role for CD36 in malarial clearance. Blood 96, 3231–3240.
Means, R. T. Jr., and Krantz, S. B. (1991). Inhibition of human erythroid colony-forming units by gamma interferon can be corrected by recombinant human erythropoietin. Blood 78, 2564–2567.
Menendez, C., Fleming, A. F., and Alonso, P. L. (2000). Malaria-related anaemia. Parasitol. Today 16, 469–476. doi: 10.1016/S0169-4758(00)01774-9
Meremikwu, M. M., and Smith, H. J. (2000). Blood transfusion for treating malarial anaemia. Cochrane Database Syst. Rev. 2:CD001475. doi: 10.1002/14651858.CD001475
Merry, A. H., Looareesuwan, S., Phillips, R. E., Chanthavanich, P., Supanaranond, W., Warrell, D. A., et al. (1986). Evidence against immune haemolysis in falciparum malaria in Thailand. Br. J. Haematol. 64, 187–194. doi: 10.1111/j.1365-2141.1986.tb07586.x
Mindukshev, I. V., Rukoiatkina, N. I., Dobrylko, I. A., Skverchinskaia, E. A., Nikitina, E. R., Krivoshlyk, V. V., et al. (2013). Characterisation of enucleated cells apoptosis: human platelets and erythrocytes. Ross. Fiziol. Zh. Im. I. M. Sechenova 99, 92–110.
Muoneke, V.U., Ibekwe, R.C., Nebe-Agumadu, H.U., and Ibe, B.C. (2012). Factors associated with mortality in under-five children with severe anemia in Ebonyi, Nigeria. Idian. Pediatr. 49, 119–123. doi: 10.1007/s13312-012-0026-4
Myssina, S., Huber, S. M., Birka, C., Lang, P. A., Lang, K. S., Friedrich, B., et al. (2003). Inhibition of erythrocyte cation channels by erythropoietin. J. Am. Soc. Nephrol. 14, 2750–2757. doi: 10.1097/01.ASN.0000093253.42641.C1
Noh, J. Y., Park, J. S., Lim, K. M., Kim, K., Bae, O. N., Chung, S. M., et al. (2010). A naphthoquinone derivative can induce anemia through phosphatidylserine exposure-mediated erythrophagocytosis. J. Pharmacol. Exp. Ther. 333, 414–420. doi: 10.1124/jpet.109.164608
Obonyo, C. O., Vulule, J., Akhwale, W. S., and Grobbee, D. E. (2007). In-hospital morbidity and mortality due to severe malarial anemia in western Kenya. Am. J. Trop. Med. Hyg. 77, 23–28.
Oldenborg, P. A., Gresham, H. D., Chen, Y., Izui, S., and Lindberg, F. P. (2002). Lethal autoimmune hemolytic anemia in CD47-deficient nonobese diabetic (NOD) mice. Blood 99, 3500–3504. doi: 10.1182/blood.V99.10.3500
Oldenborg, P. A., Gresham, H. D., and Lindberg, F. P. (2001). CD47-signal regulatory protein α (SIRPα) regulates Fcγ and complement receptor-mediated phagocytosis. J. Exp. Med. 193, 855–862. doi: 10.1084/jem.193.7.855
Oldenborg, P. A., Zheleznyak, A., Fang, Y. F., Lagenaur, C. F., Gresham, H. D., and Lindberg, F. P. (2000). Role of CD47 as a marker of self on red blood cells. Science 288, 2051–2054. doi: 10.1126/science.288.5473.2051
Olsson, M., and Oldenborg, P. A. (2008). CD47 on experimentally senescent murine RBCs inhibits phagocytosis following Fcγ receptor-mediated but not scavenger receptor-mediated recognition by macrophages. Blood 112, 4259–4267. doi: 10.1182/blood-2008-03-143008
Omodeo-Salè, F., Motti, A., Dondorp, A., White, N. J., and Taramelli, D. (2005). Destabilisation and subsequent lysis of human erythrocytes induced by Plasmodium falciparum haem products. Eur. J. Haematol. 74, 324–332. doi: 10.1111/j.1600-0609.2004.00352.x
Osaro, E., and Charles, A. T. (2011). The challenges of meeting the blood transfusion requirements in Sub-Saharan Africa: the need for the development of alternatives to allogenic blood. J. Blood. Med. 2, 7–21. doi: 10.2147/JBM.S17194
Pain, A., Urban, B. C., Kai, O., Casals-Pascual, C., Shafi, J., Marsh, K., et al. (2001). A non-sense mutation in Cd36 gene is associated with protection from severe malaria. Lancet 357, 1502–1503. doi: 10.1016/S0140-6736(00)04662-6
Papadaki, H. A., Kritikos, H. D., Valatas, V., Boumpas, D. T., and Eliopoulos, G. D. (2002). Anemia of chronic disease in rheumatoid arthritis is associated with increased apoptosis of bone marrow erythroid cells: improvement following anti-tumor necrosis factor-alpha antibody therapy. Blood 100, 474–482. doi: 10.1182/blood-2002-01-0136
Patel, S. N., Serghides, L., Smith, T. G., Febbraio, M., Silverstein, R. L., Kurtz, T. W., et al. (2004). CD36 mediates the phagocytosis of Plasmodium falciparum-infected erythrocytes by rodent macrophages. J. Infect. Dis. 189, 204–213. doi: 10.1086/380764
Pattanapanyasat, K., Sratongno, P., Chimma, P., Chitjamnongchai, S., Polsrila, K., and Chotivanich, K. (2010). Febrile temperature but not proinflammatory cytokines promotes phosphatidylserine expression on Plasmodium falciparum malaria-infected red blood cells during parasite maturation. Cytometry A 77, 515–523. doi: 10.1002/cyto.a.20879
Paul, A., Pallavi, R., Tatu, U. S., and Natarajan, V. (2013). The bystander effect in optically trapped red blood cells due Plasmodium falciparum infection. Trans. R. Soc. Trop. Med. Hyg. 107, 220–223. doi: 10.1093/trstmh/trt010
Phillips, R. E., Looareesuwan, S., Warrell, D. A., Lee, S. H., Karbwang, J., Warrell, M. J., et al. (1986). The importance of anaemia in cerebral and uncomplicated falciparum malaria: role of complications, dyserythropoiesis and iron sequestration. Q. J. Med. 58, 305–323.
Phillips, R. E., and Pasvol, G. (1992). Anaemia of Plasmodium falciparum malaria. Baillières Clin. Haematol. 5, 315–330. doi: 10.1016/S0950-3536(11)80022-3
Pino, P., Vouldoukis, I., Kolb, J. P., Mahmoudi, N., Desportes-Livage, I., Bricaire, F., et al. (2003). Plasmodium falciparum-infected erythrocyte adhesion induces caspase activation and apoptosis in human endothelial cells. J. Infect. Dis. 187, 1283–1290. doi: 10.1086/373992
Raffray, L., Receveur, M. C., Beguet, M., Lauroua, P., Pistone, T., and Malvy, D. (2014). Severe delayed autoimmune haemolytic anaemia following artesunate administration in severe malaria: a case report. Malar. J. 13:398. doi: 10.1186/1475-2875-13-398
Rodrigues, V., Cordeiro-da-Silva, A., Laforge, M., Ouaissi, A., Silvestre, R., and Estaquier, J. (2012). Modulation of mammalian apoptotic pathways by intracellular protozoan parasites. Cell. Microbiol. 14, 325–333. doi: 10.1111/j.1462-5822.2011.01737.x
Salmon, M. G., De Souza, J. B., Butcher, G. A., and Playfair, J. H. (1997). Premature removal of uninfected erythrocytes during malarial infection of normal and immunodeficient mice. Clin. Exp. Immunol. 108, 471–476. doi: 10.1046/j.1365-2249.1997.3991297.x
Satpathy, S. K., Mohanty, N., Nanda, P., and Samal, G. (2004). Severe falciparum malaria. Indian J. Pediatr. 71, 133–135. doi: 10.1007/BF02723094
Schantz-Dunn, J., and Nour, N. M. (2009). Malaria and pregnancy: a global health perspective. Rev. Obstet. Gynecol. 2, 186–192.
Schulze-Osthoff, K., Walczak, H., Drodge, W., and Krammer, P. H. (1994). Cell nucleus and DNA fragmentation are not required for apoptosis. J. Cell. Biol. 127, 15–20. doi: 10.1083/jcb.127.1.15
Shi, B., Ma, G. G., Liu, H. X., Liu, J. Q., Yang, W. H., Zhang, H. Z., et al. (2013). Significance of erythrocytes phosphatidylserine exposure on occurrence of anemia in sepsis. Chin. Crit. Care. Med. 25, 614–617. doi: 10.3760/cma.j.issn.2095-4352.2013.10.009
Skorokhod, O. A., Caione, L., Marrocco, T., Migliardi, G., Barrera, V., Arese, P., et al. (2010). Inhibition of erythropoiesis in malaria anemia: role of hemozoin and hemozoin-generated 4-hydroxynonenal. Blood 116, 4328–4337. doi: 10.1182/blood-2010-03-272781
Sobolewski, P., Gramaglia, I., Frangos, J., Intaglietta, M., and van der Heyde, H. C. (2005). Nitric oxide bioavailability in malaria. Trends Parasitol. 21, 415–422. doi: 10.1016/j.pt.2005.07.002
Sosale, N. G., Spinler, K. R., Alvey, C., and Discher, D. E. (2015). Macrophage engulfment of a cell or nanoparticle is regulated by unavoidable opsonization, a species-specific ‘marker of self’ CD47, and target physical properties. Curr. Opin. Immunol. 35, 107–112. doi: 10.1016/j.coi.2015.06.013
Sowunmi, A., Akano, K., Ayede, A. I., Ntadom, G., Fatunmbi, B., Aderoyeje, T., et al. (2015). Temporal changes in haematocrit following artemisinin-based combination treatments of uncomplicated falciparum malaria in children. BMC Infect. Dis. 15:454. doi: 10.1186/s12879-015-1219-y
Spottiswoode, N., Duffy, P. E., and Drakesmith, H. (2014). Iron, anemia and hepcidin in malaria. Front. Pharmacol. 5:125. doi: 10.3389/fphar.2014.00125
Stewart, A., Urbaniak, S., Turner, M., and Bessos, H. (2005). The application of a new quantitative assay for the monitoring of integrin-associated protein CD47 on red blood cells during storage and comparison with the expression of CD47 and phosphatidylserine with flow cytometry. Transfusion 45, 1496–1503. doi: 10.1111/j.1537-2995.2005.00564.x
Tait, J. F., and Smith, C. (1999). Phosphatidylserine receptors: role of CD36 in binding of anionic phospholipid vesicles to monocytic cells. J. Biol. Chem. 274, 3048–3054. doi: 10.1074/jbc.274.5.3048
Thawani, N., Tam, M., Bellemare, M. J., Bohle, D. S., Olivier, M., de Souza, J. B., et al. (2014). Plasmodium products contribute to severe malarial anemia by inhibiting erythropoietin-induced proliferation of erythroid precursors. J. Infect. Dis. 209, 140–149. doi: 10.1093/infdis/jit417
Totino, P. R., Daniel-Ribeiro, C. T., and Ferreira-da-Cruz, M. F. (2009). Pro-apoptotic effects of antimalarial drugs do not affect mature human erythrocytes. Acta Trop. 112, 236–238. doi: 10.1016/j.actatropica.2009.08.002
Totino, P. R., Daniel-Ribeiro, C. T., and Ferreira-da-Cruz, M. F. (2011). Refractoriness of eryptotic red blood cells to Plasmodium falciparum infection: a putative host defense mechanism limiting parasitaemia. PLoS ONE 6:e26575. doi: 10.1371/journal.pone.0026575
Totino, P. R., Magalhães, A. D., Alves, E. B., Costa, M. R. F., Lacerda, M. V. G., Daniel-Ribeiro, C. T., et al. (2014). Plasmodium falciparum, but not P. vivax, can induce erythrocytic apoptosis. Parasit. Vectors 7:484. doi: 10.1186/s13071-014-0484-8
Totino, P. R., Magalhães, A. D., Silva, L. A., Banic, D. M., Daniel-Ribeiro, C. T., and Ferreira-da-Cruz, M. F. (2010). Apoptosis of non-parasitized red blood cells in malaria: a putative mechanism involved in the pathogenesis of anaemia. Malar. J. 9:350. doi: 10.1186/1475-2875-9-350
Totino, P. R., Pinna, R. A., Oliveira, A. C., Banic, D. M., Daniel-Ribeiro, C. T., and Ferreira-da-Cruz, M. F. (2013). Apoptosis of non-parasitised red blood cells in Plasmodium yoelii malaria. Mem. Inst. Oswaldo Cruz 108, 686–690. doi: 10.1590/0074-0276108062013003
Totino, P. R., Riccio, E. K., Corte-Real, S., Daniel-Ribeiro, C. T., and de Fátima Ferreira-da-Cruz, M. (2006). Dexamethasone has pro-apoptotic effects on non-activated fresh peripheral blood mononuclear cells. Cell. Biol. Int. 30, 133–137. doi: 10.1016/j.cellbi.2005.09.002
Ulett, G. C., and Adderson, E. E. (2006). Regulation of apoptosis by gram-positive bacteria: mechanistic diversity and consequences for immunity. Curr. Immunol. Rev. 2, 119–141. doi: 10.2174/157339506776843033
Vittori, D., Vota, D., Callero, M., Chamorro, M. E., and Nesse, A. (2010). c-FLIP is involved in erythropoietin-mediated protection of erythroid-differentiated cells from TNF-α-induced apoptosis. Cell Biol. Int. 34, 621–630. doi: 10.1042/CBI20090085
Waitumbi, J. N., Opollo, M. O., Muga, R. O., Misore, A. O., and Stoute, J. A. (2000). Red cell surface changes and erythrophagocytosis in children with severe Plasmodium falciparum anemia. Blood 95, 1481–1486.
Wennicke, K., Debierre-Grockiego, F., Wichmann, D., Brattig, N. W., Pankuweit, S., Maisch, B., et al. (2008). Glycosylphosphatidylinositol-induced cardiac myocyte death might contribute to the fatal outcome of Plasmodium falciparum malaria. Apoptosis 13, 857–866. doi: 10.1007/s10495-008-0217-6
Williams, G. T. (1994). Programmed cell death: a fundamental protective response to pathogens. Trends Microbiol. 2, 463–464. doi: 10.1016/0966-842X(94)90648-3
Wilson, N. O., Huang, M. B., Anderson, W., Bond, V., Powell, M., Thompson, W. E., et al. (2008). Soluble factors from Plasmodium falciparum-infected erythrocytes induce apoptosis in human brain vascular endothelial and neuroglia cells. Mol. Biochem. Parasitol. 162, 172–176. doi: 10.1016/j.molbiopara.2008.09.003
Wu, Y., Tibrewal, N., and Birge, R. B. (2006). Phosphatidylserine recognition by phagocytes: a view to a kill. Trends Cell Biol. 16, 189–197. doi: 10.1016/j.tcb.2006.02.003
Wyllie, A. H. (1980). Glucocorticoid-induced thymocyte apoptosis is associated with endogenous endonuclease activation. Nature 284, 555–556. doi: 10.1038/284555a0
Keywords: malaria, anemia, apoptosis, red blood cells, phagocytosis
Citation: Totino PRR, Daniel-Ribeiro CT and Ferreira-da-Cruz MF (2016) Evidencing the Role of Erythrocytic Apoptosis in Malarial Anemia. Front. Cell. Infect. Microbiol. 6:176. doi: 10.3389/fcimb.2016.00176
Received: 16 September 2016; Accepted: 21 November 2016;
Published: 09 December 2016.
Edited by:
Joao Santana Silva, University of São Paulo, BrazilCopyright © 2016 Totino, Daniel-Ribeiro and Ferreira-da-Cruz. This is an open-access article distributed under the terms of the Creative Commons Attribution License (CC BY). The use, distribution or reproduction in other forums is permitted, provided the original author(s) or licensor are credited and that the original publication in this journal is cited, in accordance with accepted academic practice. No use, distribution or reproduction is permitted which does not comply with these terms.
*Correspondence: Paulo R. R. Totino, cHJ0b3Rpbm9AaW9jLmZpb2NydXouYnI=