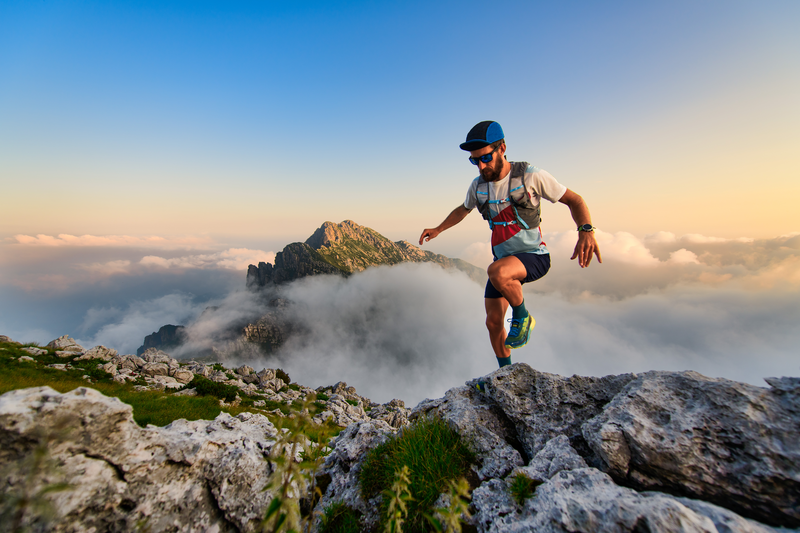
95% of researchers rate our articles as excellent or good
Learn more about the work of our research integrity team to safeguard the quality of each article we publish.
Find out more
ORIGINAL RESEARCH article
Front. Cell. Infect. Microbiol. , 17 April 2015
Sec. Fungal Pathogenesis
Volume 5 - 2015 | https://doi.org/10.3389/fcimb.2015.00036
This article is part of the Research Topic Emerging Enterobacteriaceae infections: antibiotic resistance and novel treatment options View all 7 articles
Host Defense Peptides (HDPs) are small cationic peptides found in several organisms. They play a vital role in innate immunity response and immunomodulatory stimulation. This investigation was designed to study the antimicrobial activities of β-defensin peptide-4 (sAvBD-4) and 10 (sAvBD-4) derived from chickens against pathogenic organisms including bacteria and fungi. Ten bacterial strains and three fungal species were used in investigation. The results showed that the sAvBD-10 displayed a higher bactericidal potency against all the tested bacterial strains than that of sAvBD-4. The exhibited bactericidal activity was significant against almost the different bacterial strains at different peptide concentrations except for that of Pseudomonas aeruginosa (P. aeruginosa) and Streptococcus bovis (Str. bovis) strains where a moderate effect was noted. Both peptides were effective in the inactivation of fungal species tested yielding a killing rate of up to 95%. The results revealed that the synthetic peptides were resistant to salt at a concentration of 50 mM NaCl. However, they lost antimicrobial potency when applied in the presence of high salt concentrations. Based on blood hemolysis studies, a little hemolytic effect was showed in the case of both peptides even when applied at high concentrations. The data obtained from this study indicated that synthetic avian peptides exhibit strong antibacterial and antifungal activity. In conclusion, future work and research should be tailored to a better understanding of the mechanisms of action of those peptides and their potential use in the pharmaceutical industry to help reduce the incidence and impact of infectious agent and be marketed as a naturally occurring antibiotic.
Host Defense Peptides (HDPs) are a diverse group of small and cationic peptides that are present in several organisms (Cuperus et al., 2013). Originally, they were called Antimicrobial Peptides (AMPs) due to their ability to inactivate and control bacteria in vitro. Several studies showed that these peptides have additional functions, mainly immunomodulatory ones (Cuperus et al., 2013). Therefore, they were named as “Host Defense Peptides (HDPs).” Recently, some reports indicated that HDPs are responsible for differentiation, activation and chemotaxis of leukocytes. They inhibited Lipopolysaccharide (LPS), enhance phagocytosis, DNA uptake and wound healing (Zanetti, 2005; Semple and Dorin, 2012).
HDPs were first discovered in the 1970's by extraction from tissues of avian species (Evans et al., 1994). Based on new advances in the field of bioinformatics and available sequence data, a number of new HDPs have been identified. The first avian HDPs were confirmed in the mid 1990's, and included five defensins isolated from the leukocytes of some avian species like chickens and turkeys (Evans et al., 1994). Recently, the complete defensin and cathelicidin genes clusters have been sequenced for chickens. Due to the interest in their role as potential therapeutically agents, there is a remarkable amount of information based on various studies using other avian species (Cuperus et al., 2013). Currently, there is a lot of research on the role of these peptides as templates for possible novel anti-microbial agents (Cuperus et al., 2013). Accordingly, they have been sought as possible alternatives to antibiotics which lost their shine due to the increasing resistance that is exhibited against a wide range of bacteria which is a major public health issue especially during the last decade (Cuperus et al., 2013). Defensins peptides are cysteine-rich, cationic in nature and composed of three conserved disulfide bridges, a β-sheet and both hydrophobic and cationic amino acids (Ganz, 2003; Selsted and Ouellette, 2005). The defensin peptides are classified into three main groups: α−, β−, and θ−defensins (Yang et al., 2004; Selsted and Ouellette, 2005). Alpha-defensins are found in mammals and form disulfide bridges between Cys1–Cys6, Cys2–Cys4, and Cys3–Cys5 (Lehrer and Ganz, 2002; Yang et al., 2004). Theta-defensins are present in all vertebrates and form disulfide bonds between Cys1–Cys5, Cys2–Cys4, and Cys3–Cys6 (Lehrer and Ganz, 2002; Klotman and Chang, 2006). Based on extensive search and based on chicken genome analysis, β-defensins were the first to be confirmed which derived from avians species. Using the chicken genome, they were first to be confirmed in avian species (Lehrer and Ganz, 2002; Klotman and Chang, 2006). The underlying mechanism by which AMPs exert their effect is through their interaction with the negatively charged phosolipid bilayer found in the cell membrane under hydrophobic conditions, thus resulting in disruption and killing of the pathogen (Higgs et al., 2005; Lynn et al., 2007). It has been reported that avian β-defensin are more efficient against Gram-positive bacteria than Gram-negative bacteria due to their structural conformation (Cuperus et al., 2013). The mode of action of theses peptides in bacterial killing was reported in some studies due to its net charge. Some studies revealed that the duck β−defensin-12 has the lowest net charge and lower antibacterial activity as compared to duck β−defensin 4 and 7 peptides (Powers and Hancock, 2003; Ganz, 2004; Brogden, 2005).
This study was designed to evaluate the antimicrobial activities of synthetic chicken β-defensin peptides (sAvBD-4 and 10) against both pathogenic Gram-negative and Gram-positive bacteria and fungi. The kinetics were also investigated and the effect of salinity on their activity was delineated.
Bacterial species used included both Gram-negative and Gram-positive strains. The five Gram-positive bacterial strains used were: Micrococcus luteus ATCC 49732 (M. Luteus), Enterococcus faecalis ATCC 29212 (Ent. faecalis), Streptococcus bovis ATCC 49147 (Str. bovis), Staphylococcus epidermidis ATCC 12228 (Staph. epidermidis) and Methicillin-Resistance Staphylococcus aureus ATCC 43330 (MRSA). Five strains of Gram-negative bacteria included: Pseudomonas aeruginosa ATCC 27853 (P. aeruginosa), Escherichia coli ATCC 25922 (E. coli), Salmonella typhimurium ATCC 14028 (Salm. typhimurium), Klebsiella pneumonia ATCC 700603(Kleb. pneumonia), Shigella sonnei ATCC 25931(Sh. sonnei). The bacterial strains were cultured on tryptone soy agar and incubated for 24 h at 37°C and stored in slants at 4°C.
Three types of fungal species were used; Candida albicans ATCC 10231 (C. albicans), Aspergillus flavus (Asp. flavus), and Aspergillus niger (Asp. niger) isolates (obtained from the Department of Biological Sciences, King Abdulaziz University, Jeddah, Saudi Arabia. Filamentous fungi and Candida were cultured in Sabouraud dextrose agar and incubated at 25–28°C for 72 h for multicellular fungi and h at 30°C for 24 in the case of Candida and stored in slants at 4°C.
The mature peptide of chicken β-defensin (AvBD-4 and 10) were custom synthesized and purified to 80% level using high-performance liquid chromatography (HPLC) by GL Biochem Ltd. (Shanghai, China) (http://www.glschina.com/en/profile.htm). The full sequence of amino acids of chicken β-defensin (AvBD-4 and 10) are listed in Table 1.
MIC assays for the peptides were performed by two-fold broth dilution method with Mueller Hinton II broth according to the procedures as suggested by the CLSI (Clinical and Laboratory Standards Institute) (Wayne, 2009, 2012). In summary, Bacteria and Candida were grew to reach out the exponential phase. Cells were then centrifuged at 2000 × g for 15 min. The resulting pellets were washed and resuspended in 10 mM sodium phosphate buffer saline solution (pH 7.0). Two-fold serial dilutions of the sAvBD-4 and 10 were prepared in appropriate culture medium in 96-microwell plates. 100 μL inoculum from the culture with a bacterial density of 106 CFU/ml were added to each individual well containing 100 μL of either M-H alone, or M-H containing two-fold diluted peptide to give a final concentration of the peptide ranging from 0 to 200 μM. MIC values were recorded as the minimum concentration that showed no visible growth after overnight incubation at 37°C. The cell survival percentage was determined by measuring the absorbance at λ = 570 nm with a microplate reader. Cell survival % = [(mean optical density of the sample − blank)/(mean optical density of the control ((no treatment) − blank)] × 100.
The minimum bactericidal concentration (MBC) or the minimum fungicidal concentration (MFC) were evaluated by subculture of the contents of the first two clear wells obtained in the MIC assay onto minimal M-H agar plates. The lowest peptide concentration yielding more than 99% of either bacterial or fungal growth inhibition was noted as MBC or MFC.
Two multicellular fungi (Asp. niger and Asp. flavus) were used to evaluate the anti-fungal activity of those two peptides. Overnight cultures of the fungi were prepared by inoculating 100 ml of Sabouraud dextrose broth with a 105 spores/ml concentration. Tetracycline used as a reference in the case of both Gram-negative and positive bacteria. On the other hand, Ketoconazole was use as a reference in the case of the fungus C. albicans, Asp. Niger, and Asp. flavus. The both reference antibiotics were used at concentration of (30 μg/ml).
In order to study the kinetics of bacterial inactivation for both of the test peptides, three organisms were used: MRSA, and E. coli (1 × 108 CFU/ml) and C. albicans (1 × 108 CFU/ml). The concentration used was two times of that of the MIC. Overnight bacterial cultures were prepared. The cultures were spun down and resuspended in fresh M-H medium at a concentration of 1 × 108 CFU/ml. sAvBD-4 and 10 were then added to the bacterial suspension, at a concentration equivalent to two times that of the MIC. The mixture was incubated under 35°C. Ten-microliter aliquots were removed with a sterile calibrated loop at (0, 15, 30, 60, 120, and 180 min) and uniformly seeded on M-H medium (Ma et al., 2011). The plates were incubated at 35°C for 24–48 h. After the incubation period, the number of viable cells was counted and expressed in CFU/ml. The results were analyzed and represented graphically, a microbial death curve as a function of time.
E. coli was used as a test model to evaluate the effects of ionic strength on the antibacterial activity of the two peptides. E. coli was subcultured at 37°C to the mid-log phase, and suspended to 106 CFU/ml in MH. A suspension of E. coli (1 ml) was incubated with different concentrations of peptides (0–200 μg/mL), with different concentrations of NaCl (0, 20, 50, 150 mM) in 10 mM sodium phosphate buffer, pH 7.4. The tested bacteria was cultured at 37°C for 2 h before 1000 times of dilution followed by plating. Survived bacteria were counted (Ma et al., 2011; Wang et al., 2011; Baricelli et al., 2015).
The hemolytic activities of the synthetic defensin were investigated according to what has been reported in the literature (Shin et al., 2001; Yu et al., 2001). Briefly, fresh chicken blood was collected from King Abdulaziz University farm, Jeddah, KSA. The blood was spun down for erythrocytes harvesting by centrifugation (3000 rpm, 10 min, at 20°C). The resulting erythrocytes were then washed twice with sterile PBS at a concentration of 0.5% vol/vol and were used for the assay, by dispensing 90 μl into each well of the 96-well plates. Ten microliters of different peptides concentrations were added to the cells and incubated at 37°C for 2 h. After incubation, the microtiter was spun down at 800 × g for 10 min. The supernatants were withdrawn and transferred to a new 96-well plate and checked for released hemoglobin as measured spectrophotometerically at 405 nm. For (0 hemolysis) as well as (100% hemolysis) controls, cells were resuspended in PBS only and in 1% Triton X-100, respectively (Ma et al., 2013).
Data were entered using IBM SPSS Statistics 20, and was analyzed by Kaplan–Meier analysis. A P level of < 0.05 was considered to significant.
The chicken β-defensin (sAvBD-4 and 10) used in this study were analogs of the natural peptides. These were custom made having the linear N-terminal acetylated as is the case with naturally occurring mature chicken β-defensin peptides. These custom-made peptides were evaluated for their anti-bacterial and anti-fungal activities against 10 bacterial strains and three fungal species. There was a variation in the response of the bacteria to the tested peptides with sAvBD10 showing a better efficacy on average against all the bacteria tested. Statistical analyses showed that the difference was significant at the 95% as shown in (Table 2, Figures 1A,B). The results showed that sAvBD-4 inhibited the growth of both Gram-negative and positive bacteria with MIC concentrations as follows: 25 μg/ml [(Staph. epidermidis, Kleb. pneumonia, Sh. sonnei, C. albicans), 50 μg /ml (MRSA, M. luteus, Salm. typhimurium, E. coli, Asp. flavus) and 100 μg/ml for (Str. bovis, Ent. faecalis, Asp. niger)]. However, sAvBD-10 was more efficient in achieving bacterial inactivation at the following MIC concentrations: 25 μg/ml (M. luteus, Kleb. pneumonia, C. albicans, and Asp. flavus) and 50 μg/ml (Str. bovis, Ent. faecalis, MRSA, Salm. typhimurium, E. coli, Staph. epidermidis, Sh. sonnei, Asp. niger, and P. aeruginosa). The MBC levels were also determined and found to be two-fold higher than those of the corresponding MIC values (MBC range, 50–200 μg/ml) (Table 2). At those lower concentrations, sAvBD-10 had a significantly better antimicrobial activity as compared to sAvBD-4 against all the bacteria tested (P < 0.002). However, at higher concentrations of 100 μg/ml, both peptides showed no significant difference in their bactericidal efficacy (Table 2).
Figure 1. Antimicrobial activities of synthetic chicken β-defensin-4 and 10-derived peptide (sAvBD) against (A) bacteria and (B) Fungal species. All assays were performed in three independent experiments and each point is the mean ± SE, (P < 0.002).
There was a dose dependent decline in antifungal inhibition and inactivation by both peptides (Table 2). Again, sAvBD-10 showed a significantly better anti-fungal activity as compared to sAvBD-4 as far as both MIC and MFC are concerned. In the case of sAvBD-10 (Figure 1B), the MIC varied among the three fungi with 25 μg/ml needed for both C. albicans and Asp. flavus and 50 μg/ml for Asp. niger. There was a significant difference in the MIC reported regarding sAvBD-4 among the three fungal species tested (Table 2, Figure 1B) with highest MIC observed in the case of Asp. niger.
The data showed that the MFC activity of sAvBD-10 was 25 μg/ml in the case of C. albicans and Asp. flavus and 50 μg/ml for Asp. niger. A much higher concentration was needed concerning sAvBD-4 (Table 2, Figure 1B). A better bactericidal activity was noted in the case of tetracycline as compared to the tested peptides. This, also, applies in the case of ketoconazole.
In the kinetics study, MRSA, E. coli, and C. albicans were used (Figure 2). Both peptides showed similar efficacy against MRSA during the first hour of application. However, faster decline was prominent in the case of sAvBD-4 for the next 2 h. This was not the case in term of E. coli where the two peptides started the same for the first hour and sAvBD-10 produced higher bactericidal efficacy for the remaining 2 h. As far as the kinetics of killing of C. albicans is concerned, there was a consistent better efficacy of sAvBD-10 over sAvBD-4.
Figure 2. The kinetic inactivation of synthetic chicken β-defensin-4 and 10-derived peptide (sAvBD) against MRSA, E. coli and C. albicans species. All assays were performed in three independent experiments and each point is the mean ± SE.
The effect of salinity on the antibacterial efficacy of both peptides was evaluated using various salt concentrations ranging from 0 to 150 mM (Figure 3). The results revealed that the synthetic peptide's efficacy was not reduced in the presence of salt concentrations ranging from 0 to 50 mM. However, such an effect was significantly compromised in a dose dependent manner when higher concentrations of salt were present (Figure 3) (P < 0.001).
Figure 3. Effects of salinity on the antibacterial activity of synthetic chicken β-defensin-4 and 10-derived peptide (sAvBD) against E. coli. All assays were performed in three independent experiments and each point is the mean ± SE, (P < 0.001).
The hemolytic activities of the two peptides were evaluated using freshly isolated chicken erythrocytes. The hemolysis was done using spectrophotometric measurements at a wavelength of 405 nm (Figure 4). The results showed that the peptides did not produce significant hemolytic effects.
Figure 4. Hemolytic activities of synthetic chicken β-defensin-4 and 10-derived peptides (sAvBD). All assays were performed in three independent experiments and each point is the mean ± SE.
Around 1000 HDP has been discovered so far. The β-defensins, cathelicidens and liver antimicrobial peptide-2 are three of those HDPs that originated from chickens. In this investigation, the antimicrobial potential of two synthetic peptides (AvBD-4 and 10) was evaluated against 10 Gram-positive and -negative bacterial strains, as well as unicellular and multicellular fungi. This work was initiated to better understand the efficacy of those novel peptides against bacteria and fungi and their possible application in conjunction either with already existing antimicrobial agents or with possible substitutes for them. The uncontrolled use, misuse, and abuse of commonly administered antimicrobial agents has resulted in the horizontal gene transfer among microorganisms and led to the stimulation of the evolutionary potential of the bacteria to develop some kind of resistance against the antimicrobial agents and consequently resulted in the emergence of multi-drug resistant bacteria. Such a resistance to antimicrobials is a worldwide problem in the treatment of infectious diseases especially in patients who are immunocompromised patients with hospital-acquired infections. For this reason, alternative agents have been sought to deal with the issue of multi-drug resistance. The AMPs are naturally occurring products, which have undergone a long-term evolution in nature. These peptides may have a promising potential as possible substitutes for antimicrobial agents or to potentiate the efficacy of already existing drugs used for the treatment of infectious agents.
The findings of this study revealed that the synthetic AvBD-4 and 10 displayed a potent efficacy against a broad-spectrum of bacteria. The current results were compatible with those of other studies on the antimicrobial activity of other known AvBDs (Evans et al., 1994, 1995; Harwig et al., 1994; Thouzeau et al., 2003; Lynn et al., 2004; Higgs et al., 2005; van Dijk et al., 2008; Ma et al., 2009a,b, 2011, 2012a,b,c; Soman et al., 2010; Wang et al., 2010; Zhang et al., 2011, 2012). For instance, geese derived synthetic peptides showed an effect against both Gram-positive and -negative bacteria, like S. aureus and E. coli, thus consistent with the findings on synthetic β-defensins potency in their natural forms as reported elsewhere (Sass et al., 2010; Ma et al., 2013). The results of a study on recombinant AvBD2 derived from ducks exhibited a strong bactericidal potential against B. cereus, S. aureus, and P. multocid, and weak bactericidal activity against E. coli and S. choleraesuis like the results obtained using defensins (Evans et al., 1994; Harwig et al., 1994; Yu et al., 2001; Thouzeau et al., 2003; Sugiarto and Yu, 2006), were chemically synthesized (Higgs et al., 2005; van Dijk et al., 2007) or were produced by recombinant expression (Milona et al., 2007; van Dijk et al., 2007; Ma et al., 2008). In another study using Recombinant HBD2 showed that it inhibited three Gram-negative bacteria known for their resistant to antimicrobial agents (S. marcescen, P. aeruginosa, A. baumannii) that are opportunistic nosocomial pathogens present among immunocompromised individuals (Baricelli et al., 2015).
Interestingly, besides the broad spectrum of antibacterial effect, the synthetic AvBD-4 and 10 exhibited a fungicidal activity against both unicellular and multicellular fungi. More than 90% fungicidal activity was reported in this study. It was interesting to note that avian derived peptides have an anti-fungal potential. The results showed for the first time that those avian peptides had inhibitory and cidal effects against fungi. Also, it was reported that defensin secreted by filamentous fungi displayed strong inhibitory potential against human and plant infectious agents (Lacadena et al., 1995; Meyer, 2002, 2008; Marx, 2004; Galgóczy et al., 2010).
The differences in the inactivation kinetics between the two peptides may be structure related and/or dependent on the type bacteria used. Studies demonstrated that pheasant cathelicidin-1 (Pc-CATH1) inhibited E. coli growth during the first hour of application. However, it took 6 h for the bacterial growth to be resumed (Wang et al., 2011). The killing kinetics for synthetic sAvBD-10 was more effective than sAvBD-4 in eliminating fungal species than bacteria as indicated in this investigation.
Salt resistance is a defiance that is highly pertinent for HDPs in order for them to exert their full potential under physiological conditions. The results showed that the NaCl levels as those found under physiological conditions found in the human body did not compromise the efficacy of the tested peptides. Similar to those present in the Similar to other defensins (Sang et al., 2005; Ma et al., 2011). The efficacies of those peptides were compromised in the presence of high salt concentrations. This is consistent with the findings reported by others (Soman et al., 2010) and those of others using ostrich (Ma et al., 2013; Lu et al., 2014). The results revealed that the effect of ionic strength on the antimicrobial impact of investigated peptides were compatible with other reports on most tested defensins and other AMPs (Porter et al., 1997; Bals et al., 1998; Zucht et al., 1998; Veldhuizen et al., 2008).
Furthermore, the antimicrobial potency of synthetic AvBD-4 and 10, these synthetic peptides had low hemolytic effect and low toxicity at higher concentrations. These results are in agreement with other studies on other avian β-defensin and also for mammals defensin (Milona et al., 2007; Veldhuizen et al., 2008; Ma et al., 2011, 2012a,c). Some published studies indicated that the little toxic effect of defensin peptide on animal cells might be due to the partly higher cholesterol levels and a lack of negatively charged phospholipids in the outer leaflet of animal membranes, which inhibit the binding of many AMPs (Matsuzaki et al., 1995; Ishitsuka et al., 2006). This in agreement with the data obtained by other scientists using geese and duck related defensins (Ma et al., 2013; Lu et al., 2014).
The mechanism of action by which those peptides these peptides exert their effects is not well-understood. It is likely to be due to interaction of the peptides which are positively charged with specific structures like the phospholipid membranes found on the negatively charged bacterial membrane. The result of the interaction of the peptides with the phospholipid membranes, will lead to an increase in the pore formation of the membrane which result in increased permeability leading to the demise of the bacterial cell (Zasloff, 2002; van Dijk et al., 2008; Derache et al., 2009; Ma et al., 2013; Lu et al., 2014).
The antimicrobial activity of synthetic chicken β-defensin-4 and 10 were studied against wide spectrum of infectious pathogens, including bacterial and fungal species. These peptides have exhibited significant antimicrobial activities against a broad spectrum of Gram-positive and Gram-negative bacteria of concern to the public health and have been involved in many outbreaks. Interestingly, besides their broad spectrum of antibacterial activities, synthetic AvBD (4 and 10) have shown fungicidal potential against C. albicans and Asp. flavus. The results showed that the synthetic AvBD (4 and 10) were more efficacious as an anti-fungal agents causing more than 95% reduction in fungi as compared to the control. Both of those synthetic peptides were resistant to salt concentrations of 50 mM NaCl, but lost their antimicrobial potential in a milieu containing salt concentration of 100 and 150 mM NaCl. Based on the data, it would be concluded that synthetic β-defensin peptides have a potent antimicrobial activities against a broad spectrum of pathogens and would merit the need for more studies to investigate their full potential in the treatment of disease in both humans and animals. They are likely to be a possible alternative to antibiotics and serve as natural antimicrobial properties with the hope that they do not produce bacteria that are resistant due to their naturally occurring properties.
HY and AE collection and assembly of the data, manuscript writing, and data analysis; HY, AE, MM, and SH discussion, manuscript revision; AA, MM, and OA data analysis and discussion; HY and AE concept and design, data analysis, manuscript revision, and final approval of the manuscript.
The authors declare that the research was conducted in the absence of any commercial or financial relationships that could be construed as a potential conflict of interest.
The authors thank DSR technical and financial support. The authors are solely responsible for the contents of this paper. The Deanship of Scientific Research (DSR), King Abdulaziz University, Jeddah, funded this project, under Grant No. (273-130-1433).
Bals, R., Wang, X., Wu, Z., Freeman, T., Bafna, V., Zasloff, M., et al. (1998). Human beta-defensin 2 is a salt-sensitive peptide antibiotic expressed in human lung. J. Clin. Invest. 102, 874–880.
Baricelli, J., Rocafull, M. A., Vázquez, D., Bastidas, B., Báez-Ramirez, E., and Thomas, L. E. (2015). β-defensin-2 in breast milk displays a broad antimicrobial activity against pathogenic bacteria. J. Pediatr. 91, 36–43. doi: 10.1016/j.jped.2014.05.006
PubMed Abstract | Full Text | CrossRef Full Text | Google Scholar
Brogden, K. A. (2005). Antimicrobial peptides: pore formers or metabolic inhibitors in bacteria? Nat. Rev. Microbiol. 3, 238–250. doi: 10.1038/nrmicro1098
PubMed Abstract | Full Text | CrossRef Full Text | Google Scholar
Cuperus, T., Coorens, M., van Dijk, A., and Haagsman, H. P. (2013). Avian host defense peptides. Dev. Comp. Immunol. 41, 352–369. doi: 10.1016/j.dci.2013.04.019
PubMed Abstract | Full Text | CrossRef Full Text | Google Scholar
Derache, C., Labas, V., Aucagne, V., Meudal, H., Landon, C., Delmas, A. F., et al. (2009). Primary structure and antibacterial activity of chicken bone marrow-derived beta-defensins. Antimicrob. Agents Chemother. 53, 4647–4655. doi: 10.1128/AAC.00301-09
PubMed Abstract | Full Text | CrossRef Full Text | Google Scholar
Evans, E. W., Beach, F. G., Moore, K. M., Jackwood, M. W., Glisson, J. R., and Harmon, B. G. (1995). Antimicrobial activity of chicken and turkey heterophil peptides CHP1, CHP2, THP1, and THP3. Vet. Microbiol. 47, 295–303.
Evans, E. W., Beach, G. G., Wunderlich, J., and Harmon, B. G. (1994). Isolation of antimicrobial peptides from avian heterophils. J. Leukoc. Biol. 56, 661–665.
Galgóczy, L., Kovács, L., Vágvölgyi, Cs., and Defensin-like antifungal proteins secreted by filamentous fungi. (2010). “Current research, technology and education topics in applied microbiology and microbial biotechnology,” ed A. Mendez-Vials (Badajoz: FORMATEX).
Ganz, T. (2003). Defensins: antimicrobial peptides of innate immunity. Nat. Rev. Immunol. 3, 710–720. doi: 10.1038/nri1180
PubMed Abstract | Full Text | CrossRef Full Text | Google Scholar
Ganz, T. (2004). Defensins: antimicrobial peptides of vertebrates. C. R. Biol. 327, 539–549. doi: 10.1016/j.crvi.2003.12.007
PubMed Abstract | Full Text | CrossRef Full Text | Google Scholar
Harwig, S. S., Swiderek, K. M., Kokryakov, V. N., Tan, L., Lee, T. D., Panyutich, E. A., et al. (1994). Gallinacins: cysteine-rich antimicrobial peptides of chicken leukocytes. FEBS Lett. 342, 281–285.
Higgs, R., Lynn, D. J., Gaines, S., McMahon, J., Tierney, J., James, T., et al. (2005). The synthetic form of a novel chicken beta-defensin identified in silico is predominantly active against intestinal pathogens. Immunogenetics 57, 90–98. doi: 10.1007/s00251-005-0777-3
PubMed Abstract | Full Text | CrossRef Full Text | Google Scholar
Ishitsuka, Y., Pham, D. S., Waring, A. J., Lehrer, R. I., and Lee, K. Y. (2006). Insertion selectivity of antimicrobial peptide protegrin-1 into lipid monolayers: effect of head group electrostatics and tail group packing. Biochem. Biophys. Acta 1758, 1450–1460. doi: 10.1016/j.bbamem.2006.08.001
PubMed Abstract | Full Text | CrossRef Full Text | Google Scholar
Klotman, M. E., and Chang, T. L. (2006). Defensins in innate antiviral immunity. Nat. Rev. Immunol. 6, 447–456. doi: 10.1038/nri1860
PubMed Abstract | Full Text | CrossRef Full Text | Google Scholar
Lacadena, J., Martinez del Pozo, A., Gasset, M., Patino, B., Campos-Olivas, R., Vazquez, C., et al. (1995). Characterization of the antifungal protein secreted by the mould Aspergillus giganteus. Arch. Biochem. Biophys. 324, 273–281.
Lehrer, R. I., and Ganz, T. (2002). Defensins of vertebrate animals. Curr. Opin. Immunol. 14, 96–102. doi: 10.1016/S0952-7915(01)00303-X
PubMed Abstract | Full Text | CrossRef Full Text | Google Scholar
Lu, S., Peng, K., Gao, Q., Xiang, M., Liu, H., and Song, H. (2014). Molecular cloning, characterization and tissue distribution of two ostrich β-defensins: AvBD2 and AvBD7. Gene 552, 1–7. doi: 10.1016/j.gene.2014.08.019
PubMed Abstract | Full Text | CrossRef Full Text | Google Scholar
Lynn, D. J., Higgs, R., Gaines, S., Tierney, J., James, T., Lloyd, A. T., et al. (2004). Bioinformatic discovery and initial characterisation of nine novel antimicrobial peptide genes in the chicken. Immunogenetics 56, 170–177. doi: 10.1007/s00251-004-0675-0
PubMed Abstract | Full Text | CrossRef Full Text | Google Scholar
Lynn, D. J., Higgs, R., Lloyd, A. T., O'Farrelly, C., Herve-Grepinet, V., Nys, Y., et al. (2007). Avian beta-defensin nomenclature: a community proposed update. Immunol. Lett. 110, 86–89. doi: 10.1016/j.imlet.2007.03.007
Ma, D. Y., Liao, W. Y., Wang, R. Q., Han, Z. X., and Liu, S. W. (2009a). Two novel duck antibacterial peptides, avian beta-defensins 9 and 10, with antimicrobial activity. J. Microbiol. Biotechnol. 19, 1447–1455.
Ma, D. Y., Liao, W. Y., Wang, R. Q., Han, Z. X., and Liu, S. W. (2009b). Identification and characterization of a novel antibacterial peptide, avian β-defensin 2 from ducks. J. Microbiol. 47, 610–618. doi: 10.1007/s12275-009-0068-z
PubMed Abstract | Full Text | CrossRef Full Text | Google Scholar
Ma, D. Y., Lin, L. J., Zhang, K. X., Han, Z. X., Shao, Y. H., Liu, X. L., et al. (2011). Three novel Anas platyrhynchos avian beta-defensins, upregulated by duck hepatitis virus, with antibacterial and antiviral activities. Mol. Immunol. 49, 84–96. doi: 10.1016/j.molimm.2011.07.019
PubMed Abstract | Full Text | CrossRef Full Text | Google Scholar
Ma, D., Lin, L., Zhan, K., Han, Z., Shao, Y., Wang, R., et al. (2012b). Discovery and characterization of Coturnix chinensis avian β-defensin 10, with broad antibacterial activity. J. Pept. Sci. 18, 224–232. doi: 10.1002/psc.1437
PubMed Abstract | Full Text | CrossRef Full Text | Google Scholar
Ma, D. Y., Liu, S. W., Han, Z. X., Li, Y. J, and Shan, A. S. (2008). Expression and characterization of recombinant gallinacin-9 and gallinacin-8 in Escherichia coli. Protein Expr. Puri. 58, 284–291. doi: 10.1016/j.pep.2007.11.017
PubMed Abstract | Full Text | CrossRef Full Text | Google Scholar
Ma, D., Zhou, C. Y., Zhang, M. Y., Han, Z. X., Shao, Y. H., and Liu, S. W. (2012a). Functional analysis and induction of four novel goose (Anser cygnoides) avian β-defensins in response to Salmonella enteritidis infection. Comp. Immunol. Microbiol. Infect. Dis. 35, 197–207. doi: 10.1016/j.cimid.2012.01.006
PubMed Abstract | Full Text | CrossRef Full Text | Google Scholar
Ma, D., Zhang, K., Zhang, M., Xin, S., Liu, X., Han, Z., et al. (2012c). Identification, expression and activity analyses of five novel duck β-defensins. PLoS ONE 7:e47743. doi: 10.1371/journal.pone.0047743
PubMed Abstract | Full Text | CrossRef Full Text | Google Scholar
Ma, D., Zhang, M., Zhang, K., Liu, X., Han, Z., Shao, Y., et al. (2013). Identification of three novel avian beta-defensins from goose and their significance in the pathogenesis of Salmonella. Mol. Immunol. 56, 521–529. doi: 10.1016/j.molimm.2013.05.227
PubMed Abstract | Full Text | CrossRef Full Text | Google Scholar
Marx, F. (2004). Small, basic antifungal proteins secreted from filamentous ascomycetes: a comparative study regarding expression, structure, function and potential application. Appl. Microbiol. Biotechnol. 65, 133–142. doi: 10.1007/s00253-004-1600-z
PubMed Abstract | Full Text | CrossRef Full Text | Google Scholar
Matsuzaki, K., Sugishit, K., Fujii, N., and Mijajima, K. (1995). Molecular basis for membrane selectivity of an antimicrobial peptide, magainin 2. Biochemistry 34. 3423–3429. doi: 10.1021/bi00010a034
PubMed Abstract | Full Text | CrossRef Full Text | Google Scholar
Meyer, V. (2002). Stahl U. New insights in the regulation of the afp gene encoding the antifungal protein of Aspergillus giganteus. Curr.Genet. 42, 36–42. doi: 10.1007/s00294-002-0324-9
PubMed Abstract | Full Text | CrossRef Full Text | Google Scholar
Meyer, V. (2008). A small protein that fights fungi: AFP as a new promising antifungal agent of biotechnological value. Appl. Microbiol. Biotechnol. 78, 17–28. doi: 10.1007/s00253-007-1291-3
PubMed Abstract | Full Text | CrossRef Full Text | Google Scholar
Milona, P., Townes, C. L., Bevan, R. M., and Hall, J. (2007). The chicken host peptides, gallinacins 4, 7, and 9 have antimicrobial activity against Salmonella serovars. Biochem. Biophys. Res. Commun 356, 169–174. doi: 10.1016/j.bbrc.2007.02.098
PubMed Abstract | Full Text | CrossRef Full Text | Google Scholar
Porter, E. M., van Dam, E., Valore, E. V., and Ganz, T. (1997). Broad-spectrum antimicrobial activity of human intestinal defensin 5. Infect. Immun. 65, 2396–2401.
Powers, J. P., and Hancock, R. E. (2003). The relationship between peptide structure and antibacterial activity. Peptides 24, 1681–1691. doi: 10.1016/j.peptides.2003.08.023
PubMed Abstract | Full Text | CrossRef Full Text | Google Scholar
Sang, Y., Ortega, M. T., Blecha, F., Prakash, O., and Melgarejo, T. (2005). Molecular cloning and characterization of three beta-defensins from canine testes. Infect. Immun. 73, 2611–2620.
Sass, V., Schneider, T., Wilmes, M., Körner, C., Tossi, A., Novikova, N., et al. (2010). Human beta-defensin 3 inhibits cell wall biosynthesis in Staphylococci. Infect Immun. 78, 2793–2800.
Selsted, M. E., and Ouellette, A. J. (2005). Mammalian defensins in the antimicrobial immune response. Nat. Immunol. 6, 551–557. doi: 10.1038/ni1206
PubMed Abstract | Full Text | CrossRef Full Text | Google Scholar
Semple, F., and Dorin, J. R. (2012). Beta-defensins: multifunctional modulators of 1701 infection, inflammation and more? J. Innate Immun. 4, 337–348. doi: 10.1159/000336619
PubMed Abstract | Full Text | CrossRef Full Text | Google Scholar
Shin, S., Lee, S. H., Yang, S. T., Park, E. J., Lee, D. G., Lee, M. K., et al. (2001). Antibacterial, antitumor and hemolytic activities of alpha-helical antibiotic peptide, P18 and its analogs. J. Pept. Res. 58, 504–514. doi: 10.1034/j.1399-3011.2001.00934.x
PubMed Abstract | Full Text | CrossRef Full Text | Google Scholar
Soman, S. S., Sivakumar, K. C., and Sreekumar, E. (2010). Molecular dynamics simulation studies and in vivo site directed mutagenesis of avian beta-defensin Apl-AvBD2. BMC Bioinformatics 11:S7. doi: 10.1186/1471-2105-11-s1-s7
PubMed Abstract | Full Text | CrossRef Full Text | Google Scholar
Sugiarto, H., and Yu, P. L. (2006). Identification of three novel ostricacins: an update on the phylogenetic perspective of beta-defensins. Int. J. Antimicrob. Agents. 27, 229–235.
Thouzeau, C., Le Maho, Y., Froget, G., Sabatier, L., Le Bohec, C., Hoffmann, J. A., et al. (2003). Spheniscins, avian beta-defensins in preserved stomach contents of the king penguin, Aptenodytes patagonicus. J. Biol. Chem. 27, 51053–51058. doi: 10.1074/jbc.M306839200
PubMed Abstract | Full Text | CrossRef Full Text | Google Scholar
van Dijk, A., Veldhuizen, E. J., and Haagsman, H. P. (2008). Avian defensins. Vet. Immunol. Immunopathol. 124, 1–18. doi: 10.1016/j.vetimm.2007.12.006
PubMed Abstract | Full Text | CrossRef Full Text | Google Scholar
van Dijk, A., Veldhuizen, E. J., Kalkhove, S. I., Tjeerdsma-van Bokhoven, J. L., Romijn, R. A., and Haagsman, H. P. (2007). The beta-defensin gallinacin-6 is expressed in the chicken digestive tract and has antimicrobial activity against food-borne pathogens. Antimicrob. Agents Chemother. 51, 912–922. doi: 10.1128/AAC.00568-06
PubMed Abstract | Full Text | CrossRef Full Text | Google Scholar
Veldhuizen, E. J., Rijnders, M., Claassen, E. A., van Dijk, A., and Haagsman, H. P. (2008). Porcine beta-defensin 2 displays broad antimicrobial activity against pathogenic intestinal bacteria. Mol. Immunol. 45, 386–394. doi: 10.1016/j.molimm.2007.06.001
PubMed Abstract | Full Text | CrossRef Full Text | Google Scholar
Wang, R., Ma, D., Lin, L., Zhou, C., Han, Z., Shao, Y., et al. (2010). Identification and characterization of an avian beta-defensin orthologue, avian beta-defensin 9, from quails. Appl. Microbiol. Biotechnol. 87, 1395–1405. doi: 10.1007/s00253-010-2591-6
PubMed Abstract | Full Text | CrossRef Full Text | Google Scholar
Wang, Y., Lu, Z., Feng, F., Zhu, W., Guang, H., Liu, J., et al. (2011). Molecular cloning and characterization of novel cathelicidin derived myeloid antimicrobial peptide from Phasianus colchicus. Dev. Comp. Immunol. 35, 314–322. doi: 10.1016/j.dci.2010.10.004
PubMed Abstract | Full Text | CrossRef Full Text | Google Scholar
Wayne, P. (2009). Methods for Dilution Antimicrobial Susceptibility Tests for Bacteria that Grow Aerobically; Approved Standard, 8th Edn., M07-A8. Wayne, PA: Clinical and Laboratory Standards Institute.
Wayne, P. (2012). Performance Standards for Antimicrobial Susceptibility Testing, 22nd Informational Suppl., M100-S22. Wayne, PA: Clinical and Laboratory Standards Institute.
Yang, D., Biragyn, A., Hoover, D. M., Lubkowski, J., and Oppenheim, J. J. (2004). Multiple roles of antimicrobial defensins, cathelicidins, and eosinophil-derived neurotoxin in host defense. Annu. Rev. Immunol. 22, 181–215. doi: 10.1146/annurev.immunol.22.012703.104603
PubMed Abstract | Full Text | CrossRef Full Text | Google Scholar
Yu, P. L., Choudhury, S. D., and Ahrens, K. (2001). Purification and characterization of the antimicrobial peptide, ostricacin. Biotechnol. Lett. 23, 207–210. doi: 10.1023/A:1005623806445
Zanetti, M. (2005). The role of cathelicidins in the innate host defenses of mammals. Curr. Issues Mol. Biol. 7, 179–196. Available online at: http://www.horizonpress.com/cimb/v/v7/12.pdf
Zasloff, M. (2002). Antimicrobial peptides of multicellular organisms. Nature 415, 389–395. doi: 10.1038/415389a
PubMed Abstract | Full Text | CrossRef Full Text | Google Scholar
Zhang, M. Y., Zhou, C. Y., Han, Z. X., Shao, T. Y., Liu, S. W., and Ma, D. Y. (2011). Isolation, identification and bioactivity characterization of goose avian beta-defensin. Chin. J. Biotechnol. 27, 1711–1721.
Zhang, M. Y., Zhang, K. X., Xin, S. N., Han, Z. X., Shao, Y. H., Liu, X. L., et al. (2012). Preliminary exploration of the signal transduction mechanisms of the goose beta defensing 1 resistance to Salmonella enteritidis infection mediated by toll-like receptor 4. Acta Veterinary et Zootechnica Sinica 43, 1938–1948.
Keywords: antimicrobial activity, synthetic AvBD-4 and 10, bacteria, fungi, natural antibiotic
Citation: Yacoub HA, Elazzazy AM, Abuzinadah OAH, Al-Hejin AM, Mahmoud MM and Harakeh SM (2015) Antimicrobial activities of chicken β-defensin (4 and 10) peptides against pathogenic bacteria and fungi. Front. Cell. Infect. Microbiol. 5:36. doi: 10.3389/fcimb.2015.00036
Received: 25 December 2014; Accepted: 29 March 2015;
Published: 17 April 2015.
Edited by:
Ghassan M. Matar, American University of Beirut, LebanonReviewed by:
Torsten Eckstein, Colorado State University, USACopyright © 2015 Yacoub, Elazzazy, Abuzinadah, Al-Hejin, Mahmoud and Harakeh. This is an open-access article distributed under the terms of the Creative Commons Attribution License (CC BY). The use, distribution or reproduction in other forums is permitted, provided the original author(s) or licensor are credited and that the original publication in this journal is cited, in accordance with accepted academic practice. No use, distribution or reproduction is permitted which does not comply with these terms.
*Correspondence: Haitham A. Yacoub, Biological Sciences Department, Faculty of Sciences, King Abdulaziz University, Building #71, Abdullah Sulayman street, Jeddah, Saudi ArabiaaGFpdGhhbXlhY291YjQ2QGdtYWlsLmNvbQ==
Disclaimer: All claims expressed in this article are solely those of the authors and do not necessarily represent those of their affiliated organizations, or those of the publisher, the editors and the reviewers. Any product that may be evaluated in this article or claim that may be made by its manufacturer is not guaranteed or endorsed by the publisher.
Research integrity at Frontiers
Learn more about the work of our research integrity team to safeguard the quality of each article we publish.