- School of Biosciences, University of Birmingham, Birmingham, United Kingdom
The role of caspases, or cysteine-aspartic proteases, in apoptosis has been well-studied across multiple organisms. These apoptotic caspases can be divided into initiator and effector caspases, with the former cleaving and activating the latter to trigger cell death. However, emerging evidence is supporting non-lethal roles of caspases in development, tissue homeostasis and disease. In comparison to effector caspases, less is known about the non-apoptotic functions of initiator caspases because of their more restricted activities and fewer known substrates. This review focuses on some recent findings in Drosophila on non-lethal roles of the initiator caspase Dronc. We discuss their biological importance, underlying regulatory mechanisms, and implications for our understanding of their mammalian counterparts. Deciphering the non-apoptotic functions of Dronc will provide valuable insights into the multifaceted functions of caspases during development and in diseases including cancer.
Introduction
The caspase family is comprised of cysteine proteases which are found as inactive zymogens, but once activated, cleave their substrates mainly at aspartate residues (McIlwain et al., 2013; Julien and Wells, 2017). Roles of caspases have been intensively studied in apoptosis, a major form of programmed cell death (Vandenabeele et al., 2010). In addition to apoptosis, caspases also play critical roles in immune responses by cleaving and activating inflammatory cytokines and stalling bacterial replication (Jiménez Fernández and Lamkanfi, 2015; Ross et al., 2022). Therefore, caspases are categorized as apoptotic and inflammatory caspases. Apoptotic caspases are further divided into initiator or effector caspases depending on their structures and functions in apoptosis (Riedl and Shi, 2004). Initiator caspases have long N-terminal domains, including the death effector domain (DED) or the caspase activation and recruitment domain (CARD). In contrast, effector caspases have short or no N-terminal prodomains. Once activated, initiator caspases cleave and activate downstream effector caspases, which in turn cleave a substantial number of substrates to execute apoptosis. Intriguingly, caspases also possess a broad spectrum of non-lethal functions (Arama et al., 2021). Advanced genetic tools developed in the model organism Drosophila have revealed widespread and sublethal activation of caspases, particularly effector caspases, during development (Tang et al., 2015; Ding et al., 2016). This supports widely observed non-lethal caspase functions. Compared to effector caspases, initiator caspases have more restricted activities and fewer known substrates. However, their non-lethal roles are also emerging. This review will discuss evidence for this by focusing on several recent studies in Drosophila.
Caspases in Drosophila
The core components, including caspases, of the apoptotic machinery are well conserved between mammals and Drosophila (Figure 1) (Fuchs and Steller, 2011; Hounsell and Fan, 2021). There are seven caspases in Drosophila: the initiator caspases Dronc, Dredd, and Strica (Chen et al., 1998; Dorstyn et al., 1999a; Meier et al., 2000; Doumanis et al., 2001), and the effector caspases DrICE, Dcp-1, Damm, and Decay (Fraser and Evan, 1997; Song et al., 1997; Dorstyn et al., 1999b; Harvey et al., 2001). The major apoptotic caspases in Drosophila somatic tissues are Dronc, DrICE and Dcp-1 (Chew et al., 2004; Xu et al., 2005; Xu et al., 2006). Dronc is the Drosophila ortholog of Caspase-2 and -9 in mammals and, like its counterparts, contains a CARD prodomain. In response to apoptotic stimuli such as heat, hypoxia and toxins, expression of pro-apoptotic proteins Hid, Rpr and Grim antagonizes the Drosophila inhibitor of apoptosis proteins (Diap1) (Fuchs and Steller, 2011; Hounsell and Fan, 2021). Like the mammalian IAPs, Diap1 suppresses caspases. Once released from Diap1 inhibition, Dronc associates with Dark, the Drosophila equivalent of the adaptor protein Apaf-1, to form the apoptosome. Dronc then auto-cleaves and becomes activated. Activated Dronc further cleaves and activates the effector caspases DrICE and Dcp-1, leading to the execution of apoptosis. Similarly to mammals, apoptosis can also be triggered by Eiger (Egr), the Drosophila Tumor Necrosis Factor (TNF), through activation of c-Jun N-terminal kinase (JNK) signaling resulting in the expression of pro-apoptotic genes (Figure 1). In addition to apoptosis, Dronc also plays a role in other forms of cell death, such as in TNF-induced necrosis (Li et al., 2019).
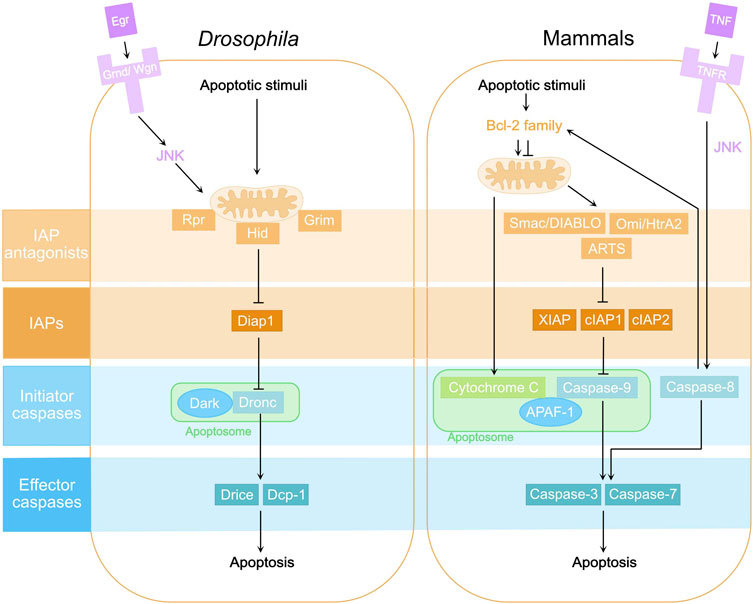
FIGURE 1. Core components of the apoptosis pathway in Drosphila and mammals. In Drosophila, apoptotic stimuli trigger the expression of IAP antagonists, mainly Rpr, Hid and Grim, which localize to mitochondria and inhibit the Drosophila inhibitor of apoptosis protein 1 (Diap1). Consequently, the initiator caspase Dronc, the Drosophila homolog of Caspase-2 and -9, is released from the inhibition of Diap1. Dronc then binds to Drosophila Ark (Dark), the Apaf-1 homolog, forming the apoptosome and becoming activated. Activated Dronc further cleaves and activates effector caspases DrICE and Dcp-1 leading to apoptosis. Eiger (Egr), the Drosophila Tumor Necrosis Factor (TNF), can trigger apoptosis via its receptors Grindelwald (Grnd) and Wengen (Wgn) and activation of c-Jun N-terminal Kinase (JNK) signaling. Their counterparts in the mammalian apoptosis pathway are highlighted in the same color.
Although caspases are best known at executing cell death, they also have non-lethal functions. Effector caspases, in particular, have been reported to have various roles in processes such as cell migration, growth control, neurite pruning, and tissue remodeling (Williams et al., 2006; Gorelick-Ashkenazi et al., 2018; Shinoda et al., 2019; Villars et al., 2022). In contrast, non-lethal roles of initiator caspases are less understood. Here, we will discuss the reported non-lethal roles of Dronc during Drosophila development and tissue homeostasis, as well as their implications for tumorigenesis.
Roles of Dronc during development and morphogenesis
During development of the central nervous system (CNS) in Drosophila, neuroblasts undergo asymmetric cell division to self-renew while also giving rise to more fate-specified cells such as the intermediate neural precursors (INP) and ganglion mother cells (GMC), depending on the context (Homem and Knoblich, 2012; Harding and White, 2018). GMC can further divide and produce two differentiated cells such as neurons and glial cells. Numb is a membrane-associated protein during asymmetric cell division (Knoblich et al., 1997). It segregates into the more differentiated daughter cell and inhibits Notch signaling, contributing to divergent cell fates. Interestingly, Dronc was revealed as a binding partner of Numb, and this interaction is abolished by Numb phosphorylation (Ouyang et al., 2011). Expression of a phospho-mimetic form of Numb in the larval brain produces supernumerary neuroblasts but fewer differentiated neurons. Moreover, expression of a catalytically inactive form of Dronc (DroncC>A) (Meier et al., 2000), but not the effector caspase inhibitor P35 (Hay et al., 1994), attenuates this phospho-Numb-induced neuroblast production (Ouyang et al., 2011). Therefore, Dronc has a non-apoptotic role in the maintenance of neuroblast homeostasis via its interaction with Numb (Figure 2A). The catalytic activity of Dronc, but not effector caspases, is required in this process.
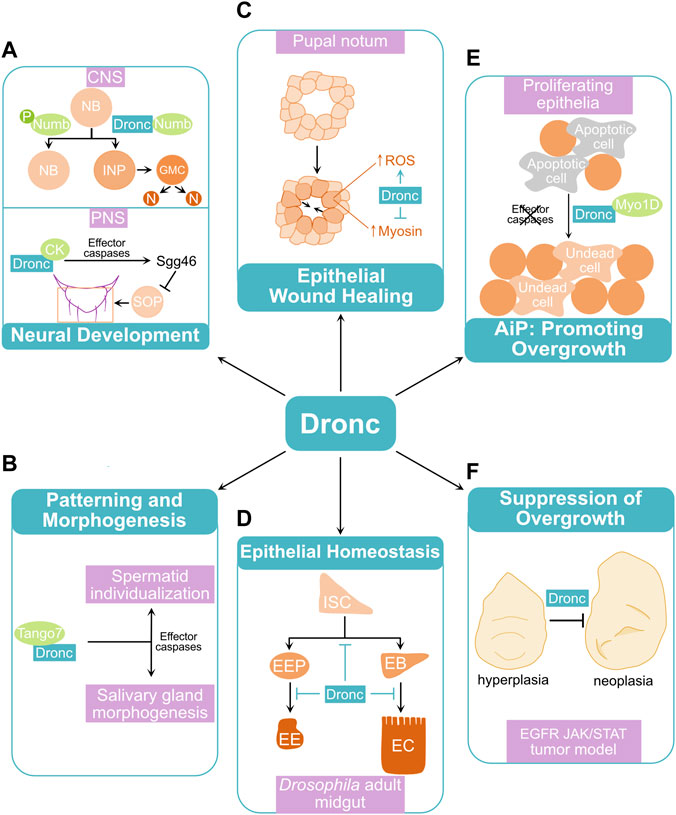
FIGURE 2. Examples of non-lethal roles of Dronc in Drosophila. (A) Roles of Dronc in neural development. In the larval central nervous system (CNS), Dronc interacts with Numb to promote cell differentiation from neuroblasts (NB) to intermediate neural precursors (INP), ganglion mother cells (GMC), and differentiated cells such as neurons (N). Phosphorylation (P) of Numb abolishes the Numb-Dronc interaction and promotes excess neuroblast proliferation. In the peripheral nervous system (PNS), Dronc promotes cleavage of Shaggy kinase 46 (Sgg46) via its interaction with Crinkled (CK) and effector caspases. This cleavage activates Sgg leading to division of sensory organ precursors (SOP) and formation of sensory organs such as macrochaetaes. (B) Tango7 binds to Dronc and controls its function during spermatid individualization and salivary gland morphogenesis, via catalytic activities of effector caspases. (C) In the pupal notum, Dronc counteracts wound closure by promoting production of reactive oxygen species (ROS) and inhibiting myosin accumulation at the wound sites. (D) During gut epithelial homeostasis, Dronc contributes to maintenance of quiescent intestinal stem cells (ISC) and inhibits differentiation of enteroblasts (EB) into enterocytes (EC) and enteroendocrine progenitor cells (EEP) into enteroendocrine cells (EE). (E) In larval proliferating epithelia, Dronc drives tissue overgrowth via apoptosis-induced proliferation (AiP) when effector caspases are deficient or inhibited. (F) Dronc suppresses tumor progression in a Drosophila tumor model in which the EGFR and JAK/STAT signaling pathways are simultaneously activated. See the main text for detailed mechanisms regulating non-lethal roles of Dronc in these processes.
In the Drosophila peripheral nervous system (PNS), typical sensory organs on the adult thorax include large (macrochaete) and small (microchaete) bristles (Simpson and Marcellini, 2006). Each consists of four cells, including a socket cell, a shaft (hair) cell, a sheath cell, and a neuron, which are derived from asymmetric divisions of a sensory organ precursor (SOP). Wild-type flies have two pairs of macrochaetes on the scutellum of the notum (Figure 2A) (Kanuka et al., 2005; Koto et al., 2009). Interestingly, dark mutants, expression of a dominant negative mutant form of Dronc (DroncDN), or RNAi knockdown of Dronc in the scutellum frequently gave rise to flies with extra macrochaetes (Kanuka et al., 1999; Rodriguez et al., 1999; Kanuka et al., 2005). These suggest a role for caspases in bristle pattern formation. An isoform of Shaggy kinase Sgg46, an antagonist of Wg/Wnt signaling during macrochaete formation, was identified as a substrate of effector caspases (Kanuka et al., 2005). Dronc regulates this process through its interaction with Crinkled (CK), a non-conventional myosin acting as an adaptor of Dronc to facilitate the cleavage of Sgg46 (Figure 2A) (Orme et al., 2016). This regulatory role of CK on Dronc has also been implicated in antenna arista morphogenesis and border cell migration during oogenesis (Geisbrecht and Montell, 2004; Kiehart et al., 2004; Orme et al., 2016).
Intriguingly, another adaptor protein, Tango7, was found to mediate non-lethal roles of Dronc in regulating patterning and morphogenesis, specifically during spermatogenesis and development of the salivary gland (Figure 2B) (D'Brot et al., 2013; Kang et al., 2017). During spermatid individualization, a large proportion of cytoplasm is removed and cytoplasmic bridges between spermatids are broken, resulting in the formation of individual sperms (Arama et al., 2003; Huh et al., 2003). This non-apoptotic process requires localized activation of the apoptosome and effector caspases which depends on a physical binding of Tango7 to both Dronc and Dark (D'Brot et al., 2013). A similar role of Tango7 was also observed during development of the salivary gland, which becomes morphologically stretched due to an enlarged lumen at late larval stage (Kang et al., 2017). During this morphogenetic process, Dronc localizes to and dismantles the cortical actin meshwork leading to its redistribution to the cytoplasm. Tango7, but not Dark, is required for the localization and proteolytic function of Dronc in this process. In contrast, the cytoplasmic localization and activation of Dronc depends on Dark and is critical for cell death that occurs in salivary glands starting at early pupal stage. These suggest that Dark and Tango7 determine the subcellular localization of Dronc and its lethal versus non-lethal functions.
The identification of Numb, CK and Tango7 as binding partners and mediators of Dronc function during development and morphogenesis is intriguing. Notably, MYO7A, the mammalian ortholog of CK, can selectively bind to Caspase-8 and regulate its non-apoptotic cleavage activity (Orme et al., 2016). Therefore, adaptor proteins modulating caspase specificity appears to be an evolutionarily conserved phenomenon. However, the mechanisms controlling the context-specific interactions between caspases and their unique adaptor proteins remain elusive.
Roles of Dronc in epithelial wound healing and homeostasis
Tissue regeneration and repair frequently rely on wound healing and stem cell renewal. During Drosophila pupal development, two wing imaginal discs, the epithelial primordia of adult notum and wings, fuse anteriorly to form an intact thorax (Zeitlinger and Bohmann, 1999). This process, termed thorax closure, has been used as a model of epithelial wound closure. During this process, non-apoptotic caspase activity was detected at the leading edge cells (Fujisawa et al., 2019). Similarly, a sublethal caspase activation was observed close to the wound sites following laser ablation of pupa nota. Interestingly, expression of Diap1, droncRNAi, or DroncDN, but not P35, promoted wound healing. In agreement with this finding, co-expression of Rpr and P35, hence activating Dronc but inhibiting effector caspases, resulted in slower wound closure and flies with abnormal thoraxes. Therefore, Dronc, but not effector caspases, negatively regulates epithelial wound closure. Notably, active JNK signaling is required for wound healing. However, Dronc does not regulate JNK in this process. In contrast, production of reactive oxygen species (ROS) at wound sites, which regulates wound closure, depends on Dronc. Moreover, the level of myosin, a motor protein critical for actin cytoskeleton remodeling and wound closure, is significantly increased in response to loss of dronc. These support an inhibitory role of Dronc in wound healing by promoting ROS production and inhibiting myosin accumulation (Figure 2C).
The adult Drosophila midgut, a functional equivalent of the mammalian small intestine, is an established model for the study of stem cell biology and epithelial tissue homeostasis (Micchelli and Perrimon, 2006; Ohlstein and Spradling, 2006; Jiang and Edgar, 2011). In the midgut, multipotent intestinal stem cells (ISC) can self-renew and give rise to enteroblasts (EB) or enteroendocrine progenitor cells (EEP) (Hung et al., 2021). EBs can further differentiate into absorptive enterocytes (EC), while EEPs can develop into hormone-secreting enteroendocrine cells (EE) (Figure 2D). Dronc is critical for gut epithelial tissue homeostasis as a defective intestinal barrier function was observed in survived dronc homozygous mutants, which are strong semi-lethal (Lindblad et al., 2021). In support of this, expression of Dronc was detected in progenitor cells including EBs (Arthurton et al., 2020; Lindblad et al., 2021). A conditional knockout of Dronc in both ISCs and EBs or RNAi knockdown of Dronc specifically in EBs resulted in a hyperplastic phenotype characterized by an increased number of both progenitor cells such as EBs and differentiated cells including ECs and EEs. Therefore, Dronc contributes to the maintenance of quiescent progenitor cells as well as inhibition of cell differentiation (Figure 2D). Moreover, this hyperplastic phenotype was rescued by expressing wild-type Dronc, but not DroncC>A, suggesting that Dronc catalytic activity is required in this process. However, blocking apoptosis by downregulating key apoptosis pathway components including Dark, DrICE and Dcp-1 or expression of P35 in EBs did not replicate the phenotype observed with loss of dronc. Hence, Dronc appears to have apoptosis cascade-independent roles in regulating gut epithelial tissue homeostasis.
Notably, similar roles of initiator caspases in wound healing and intestinal tissue homeostasis have been reported in mammals. For example, Caspase-8 deficiency in mouse skin resulted in elevated wound closure due to hyperproliferation of epidermal cells and enhanced local immune responses (Lee et al., 2009). Downregulation of Caspase-9 was associated with less differentiated, or higher grade, colon cancer (Xu et al., 2013). However, it is not yet known how caspase expression is locally regulated and what caspase substrates mediate these processes. Answers to these questions will provide novel insight into tissue-specific functions of initiator caspases.
Roles of Dronc in apoptosis-induced proliferation and tumorigenesis
Apoptosis-induced proliferation (AiP) is a mechanism of compensatory cell proliferation in response to apoptotic stress (Fan and Bergmann, 2008a; Mollereau et al., 2013). Caspases play critical roles in the activation of AiP in multiple organisms including hydra, Drosophila, and mouse (Ryoo and Bergmann, 2012). AiP was first reported in the Drosophila larval wing imaginal disc, a proliferating tissue in which most cells are dividing (Huh et al., 2004; Pérez-Garijo et al., 2004; Ryoo et al., 2004). It was later discovered that initiator and effector caspases can trigger different mechanisms of AiP in a context-dependent manner (Fan and Bergmann, 2008b). In response to apoptotic stress, DrICE and Dcp-1 drive AiP via activation of Hedgehog signaling in differentiating tissues, in which most cells have exited the cell cycle. In contrast, Dronc activates mitogenic signals, such as Wg/Wnt, Dpp/TGF-β, and Spi/EGF signaling, leading to AiP in proliferating tissues. Myo1D, an unconventional myosin, physically interacts with and relocates cytoplasmic Dronc to the plasma membrane, allowing Dronc to activate Duox, a NADPH-oxidase, leading to production of extracellular ROS (Fogarty et al., 2016; Amcheslavsky et al., 2018). These ROS attract hemocytes, the Drosophila macrophages, which secret Egr to activate JNK signaling non-cell autonomously at the apoptotic or damage sites. Interestingly, a similar role for Myo1D-Dronc interaction was observed in old ECs contributing to midgut tissue homeostasis (Amcheslavsky et al., 2020). Furthermore, Myo1D mediates Dronc-dependent regulation of F-actin dynamics which is critical for AiP, through controlling ROS production and JNK activation in apoptotic cells (Farrell et al., 2022). Therefore, Myo1D is a key adaptor which mediates distinct roles of Dronc in AiP (Figure 2E). Moreover, expression of darkRNAi or DroncC>A suppresses AiP, suggesting that apoptosome formation and Dronc catalytic activity are required (Huh et al., 2004; Fan et al., 2014). Notably, expression of P35 or knockdown of both DrICE and Dcp-1 suppresses apoptosis, but not Dronc-induced AiP, in the proliferating eye and wing tissues, leading to tissue overgrowth (Kondo et al., 2006; Fan et al., 2014). Hence, the role of Dronc in AiP can be tumorigenic. Indeed, AiP has been implicated in promoting tumor growth and cancer recurrence in mammals (Huang et al., 2011; Ford et al., 2015). However, compared to effector caspases, roles of initiator caspases in these processes are less known.
Dronc also possesses a tumor suppressive role independent of apoptosis. This was observed recently in a Drosophila tumor model with synergistic activation of EGFR and JAK/STAT signaling (EJS) in wing imaginal discs (Xu et al., 2022). In EJS tumors, a high level of Dronc activity was observed. Inhibition of Dronc activity, RNAi knockdown, or a conditional knockout of Dronc resulted in an enhanced tumor overgrowth phenotype characterized by increased cell proliferation and cell sizes. In contrast, reduction of the pro-apoptotic proteins Rpr, Hid and Grim or expression of P35 suppressed apoptosis but did not cause elevated EJS tumor growth. Therefore, Dronc activity counteracts EJS tumor progression independent of apoptosis (Figure 2F). Loss of dronc further enhances JNK activity. This promotes tumor progression by inducing the expression of Unpaired (Upd) ligands, which activate JAK/STAT signaling. Activation of JNK also leads to a higher number and proliferation of hemocytes, which attach to the EJS tumor cells and secrete Upd to further enhance tumor growth. Therefore, Dronc regulates both EJS tumor and its microenvironment by modulating JNK activity.
These examples in Drosophila suggest both pro- and anti-cancer activities of Dronc. In mammals, Caspase-8 promotes tumorigenesis in gliomas and is associated with poor patient prognosis (Fianco et al., 2017). In contrast, Caspase-2 deficiency in mouse models of lymphoma and lung cancer results in greater chemotherapeutic resistance, but also enhances tumor growth (Ho et al., 2009; Terry et al., 2015). Therefore, mammalian initiator caspases also have pro- or anti-oncogenic functions. However, how these initiator caspases are directed to execute their vital, but not lethal, roles in these processes remains elusive.
Discussion
The initiator caspase Dronc in Drosophila, like its counterparts Caspase-2, -8 and -9 in mammals, appears to exert its diverse functions in a context-dependent manner. These roles could be determined by the adaptor proteins with which Dronc interacts, the subcellular localization and activation of Dronc, or the Dronc-mediated cleavage of non-lethal substrates. Notably, these mechanisms are frequently interlinked and integrated. For example, the membrane-associated Numb interacts with Dronc to promote neuroblast differentiation via asymmetric localization and segregation of Numb (Figure 2A). Similarly, non-conventional myosins such as CK, Tango7 and Myo1D physically interact with Dronc and potentially regulate its subcellular localization for non-lethal functions (Figures 2A, B, E). However, it remains to be seen whether similar adaptors exist to mediate the functions of Dronc in wound healing, gut epithelial homeostasis, and tumor growth control (Figures 2C, D, F).
Another factor regulating the context-dependent activation of Dronc is its upstream inhibitor Diap1. For instance, Hippo signaling, a growth and tissue size control signal, monitors macrochaete formation by regulating Diap1 expression (Wang and Baker, 2019). Consistent with this finding, a dynamic expression of Diap1 was observed during sensory organ development, affecting bristle morphogenesis (Koto et al., 2009). Moreover, diap1 expression and its protein stability are subject to the control of multiple cell type-specific mechanisms (Fan and Bergmann, 2014). Therefore, Diap1 can be a critical factor modulating sublethal Dronc activities. It is worth noting that anastasis, a phenomenon referring to cell recovery from activation of apoptotic caspases, can also contribute to caspase activation widely observed during development, regeneration, and tumorigenesis (Tang et al., 2015; Ding et al., 2016; Sun et al., 2020). Although physiological roles of anastasis remain to be clarified, it poses a challenge for future studies to distinguish it from the non-lethal roles of caspases.
Finally, the question of which substrates confer context-dependent functions of Dronc remains to be answered. Bioinformatics searches to identify Dronc substrates are not currently feasible as cleavage sites of Dronc are highly variable (Hawkins et al., 2000; Snipas et al., 2008). This, together with the spatial and temporal nature of Dronc activation, presents another challenge for future studies. However, recent technical advance on developing sensitive caspase reporters and proximity labeling of Dronc in vivo has offered unique opportunities to address this challenge in Drosophila (Baena-Lopez et al., 2018; Shinoda et al., 2019). Therefore, studies using Drosophila models, which have uncovered the versatility of Dronc in processes underpinning tissue homeostasis and development, will continue to provide valuable insights into our understanding of caspase biology and its implication for human diseases.
Author contributions
DD and YF conducted literature research and wrote the manuscript.
Funding
This work has been supported by grant BB/S015701/1 from the UKRI BBSRC. DD is a Ph.D. student supported by the Midlands Integrative Biosciences Training Partnership (MIBTP) programme.
Acknowledgments
We apologise to our colleagues for omitting many relevant publications owing to space limitations.
Conflict of interest
The authors declare that the research was conducted in the absence of any commercial or financial relationships that could be construed as a potential conflict of interest.
Publisher’s note
All claims expressed in this article are solely those of the authors and do not necessarily represent those of their affiliated organizations, or those of the publisher, the editors and the reviewers. Any product that may be evaluated in this article, or claim that may be made by its manufacturer, is not guaranteed or endorsed by the publisher.
References
Amcheslavsky, A., Lindblad, J. L., and Bergmann, A. (2020). Transiently "undead" enterocytes mediate homeostatic tissue turnover in the adult Drosophila midgut. Cell Rep. 33 (8), 108408. doi:10.1016/j.celrep.2020.108408
Amcheslavsky, A., Wang, S., Fogarty, C. E., Lindblad, J. L., Fan, Y., and Bergmann, A. (2018). Plasma membrane localization of apoptotic caspases for non-apoptotic functions. Dev. Cell 45 (4), 450–464.e3. doi:10.1016/j.devcel.2018.04.020
Arama, E., Agapite, J., and Steller, H. (2003). Caspase activity and a specific cytochrome C are required for sperm differentiation in Drosophila. Dev. Cell 4 (5), 687–697. doi:10.1016/S1534-5807(03)00120-5
Arama, E., Baena-Lopez, L. A., and Fearnhead, H. O. (2021). Non-lethal message from the Holy Land: The first international conference on nonapoptotic roles of apoptotic proteins. FEBS J. 288 (7), 2166–2183. doi:10.1111/febs.15547
Arthurton, L., Nahotko, D. A., Alonso, J., Wendler, F., and Baena-Lopez, L. A. (2020). Non-apoptotic caspase activation preserves Drosophila intestinal progenitor cells in quiescence. EMBO Rep. 21 (12), e48892. doi:10.15252/embr.201948892
Baena-Lopez, L. A., Arthurton, L., Bischoff, M., Vincent, J.-P., Alexandre, C., and McGregor, R. (2018). Novel initiator caspase reporters uncover previously unknown features of caspase-activating cells. Development 145 (23), dev170811. doi:10.1242/dev.170811
Chen, P., Rodriguez, A., Erskine, R., Thach, T., and Abrams, J. M. (1998). Dredd, a novel effector of the apoptosis activators reaper, grim, and hid in Drosophila. Dev. Biol. 201 (2), 202–216. doi:10.1006/dbio.1998.9000
Chew, S. K., Akdemir, F., Chen, P., Lu, W. J., Mills, K., Daish, T., et al. (2004). The apical caspase dronc governs programmed and unprogrammed cell death in Drosophila. Dev. Cell 7 (6), 897–907. doi:10.1016/j.devcel.2004.09.016
D'Brot, A., Chen, P., Vaishnav, M., Yuan, S., Akey, C. W., and Abrams, J. M. (2013). Tango7 directs cellular remodeling by the Drosophila apoptosome. Genes Dev. 27 (15), 1650–1655. doi:10.1101/gad.219287.113
Ding, A. X., Sun, G., Argaw, Y. G., Wong, J. O., Easwaran, S., and Montell, D. J. (2016). CasExpress reveals widespread and diverse patterns of cell survival of caspase-3 activation during development in vivo. Elife 5, e10936. doi:10.7554/eLife.10936
Dorstyn, L., Colussi, P. A., Quinn, L. M., Richardson, H., and Kumar, S. (1999a). DRONC, an ecdysone-inducible Drosophila caspase. Proc. Natl. Acad. Sci. U. S. A. 96 (8), 4307–4312. doi:10.1073/pnas.96.8.4307
Dorstyn, L., Read, S. H., Quinn, L. M., Richardson, H., and Kumar, S. (1999b). DECAY, a novel Drosophila caspase related to mammalian caspase-3 and caspase-7. J. Biol. Chem. 274 (43), 30778–30783. doi:10.1074/jbc.274.43.30778
Doumanis, J., Quinn, L., Richardson, H., and Kumar, S. (2001). STRICA, a novel Drosophila melanogaster caspase with an unusual serine/threonine-rich prodomain, interacts with DIAP1 and DIAP2. Cell Death Differ. 8 (4), 387–394. doi:10.1038/sj.cdd.4400864
Fan, Y., and Bergmann, A. (2014). Multiple mechanisms modulate distinct cellular susceptibilities toward apoptosis in the developing Drosophila eye. Dev. Cell 30 (1), 48–60. doi:10.1016/j.devcel.2014.05.007
Fan, Y., and Bergmann, A. (2008a). Apoptosis-induced compensatory proliferation. The Cell is dead. Long live the Cell!. Trends Cell Biol. 18 (10), 467–473. doi:10.1016/j.tcb.2008.08.001
Fan, Y., and Bergmann, A. (2008b). Distinct Mechanisms of Apoptosis-Induced Compensatory Proliferation in Proliferating and Differentiating Tissues in the Drosophila Eye. Dev. Cell 14 (3), 399–410. doi:10.1016/j.devcel.2008.01.003
Fan, Y., Wang, S., Hernandez, J., Yenigun, V. B., Hertlein, G., Fogarty, C. E., et al. (2014). Genetic models of apoptosis-induced proliferation decipher activation of JNK and identify a requirement of EGFR signaling for tissue regenerative responses in Drosophila. PLoS Genet. 10 (1), e1004131. doi:10.1371/journal.pgen.1004131
Farrell, L., Puig-Barbe, A., Haque, M. I., Amcheslavsky, A., Yu, M., Bergmann, A., et al. (2022). Actin remodeling mediates ROS production and JNK activation to drive apoptosis-induced proliferation. PLOS Genetics 18 (12), e1010533. doi:10.1371/journal.pgen.1010533
Fianco, G., Mongiardi, M. P., Levi, A., De Luca, T., Desideri, M., Trisciuoglio, D., et al. (2017). Caspase-8 contributes to angiogenesis and chemotherapy resistance in glioblastoma. Elife 6, e22593. doi:10.7554/eLife.22593
Fogarty, C. E., Diwanji, N., Lindblad, J. L., Tare, M., Amcheslavsky, A., Makhijani, K., et al. (2016). Extracellular reactive oxygen species drive apoptosis-induced proliferation via Drosophila macrophages. Curr. Biol. 26 (5), 575–584. doi:10.1016/j.cub.2015.12.064
Ford, C. A., Petrova, S., Pound, J. D., Voss, J. J., Melville, L., Paterson, M., et al. (2015). Oncogenic properties of apoptotic tumor cells in aggressive B cell lymphoma. Curr. Biol. 25 (5), 577–588. doi:10.1016/j.cub.2014.12.059
Fraser, A. G., and Evan, G. I. (1997). Identification of a Drosophila melanogaster ICE/CED-3-related protease, drICE. EMBO J. 16 (10), 2805–2813. doi:10.1093/emboj/16.10.2805
Fuchs, Y., and Steller, H. (2011). Programmed cell death in animal development and disease. Cell 147 (4), 742–758. doi:10.1016/j.cell.2011.10.033
Fujisawa, Y., Kosakamoto, H., Chihara, T., and Miura, M. (2019). Non-apoptotic function of Drosophila caspase activation in epithelial thorax closure and wound healing. Development 146 (4), dev169037. doi:10.1242/dev.169037
Geisbrecht, E. R., and Montell, D. J. (2004). A role for Drosophila IAP1-mediated caspase inhibition in rac-dependent cell migration. Cell 118 (1), 111–125. doi:10.1016/j.cell.2004.06.020
Gorelick-Ashkenazi, A., Weiss, R., Sapozhnikov, L., Florentin, A., Tarayrah-Ibraheim, L., Dweik, D., et al. (2018). Caspases maintain tissue integrity by an apoptosis-independent inhibition of cell migration and invasion. Nat. Commun. 9 (1), 2806. doi:10.1038/s41467-018-05204-6
Harding, K., and White, K. (2018). Drosophila as a model for developmental biology: Stem cell-fate decisions in the developing nervous system. J. Dev. Biol. [Online] 6 (4), 25. doi:10.3390/jdb6040025
Harvey, N. L., Daish, T., Mills, K., Dorstyn, L., Quinn, L. M., Read, S. H., et al. (2001). Characterization of the Drosophila caspase, DAMM. J. Biol. Chem. 276 (27), 25342–25350. doi:10.1074/jbc.M009444200
Hawkins, C. J., Yoo, S. J., Peterson, E. P., Wang, S. L., Vernooy, S. Y., and Hay, B. A. (2000). The Drosophila caspase DRONC cleaves following glutamate or aspartate and is regulated by DIAP1, HID, and GRIM. J. Biol. Chem. 275 (35), 27084–27093. doi:10.1074/jbc.M000869200
Hay, B. A., Wolff, T., and Rubin, G. M. (1994). Expression of baculovirus P35 prevents cell death in Drosophila. Development 120 (8), 2121–2129. doi:10.1242/dev.120.8.2121
Ho, L. H., Taylor, R., Dorstyn, L., Cakouros, D., Bouillet, P., and Kumar, S. (2009). A tumor suppressor function for caspase-2. Proc. Natl. Acad. Sci. U. S. A. 106 (13), 5336–5341. doi:10.1073/pnas.0811928106
Homem, C. C., and Knoblich, J. A. (2012). Drosophila neuroblasts: A model for stem cell biology. Development 139 (23), 4297–4310. doi:10.1242/dev.080515
Hounsell, C., and Fan, Y. (2021). The duality of caspases in cancer, as told through the fly. Int. J. Mol. Sci. 22 (16), 8927. doi:10.3390/ijms22168927
Huang, Q., Li, F., Liu, X., Li, W., Shi, W., Liu, F. F., et al. (2011). Caspase 3-mediated stimulation of tumor cell repopulation during cancer radiotherapy. Nat. Med. 17 (7), 860–866. doi:10.1038/nm.2385
Huh, J. R., Guo, M., and Hay, B. A. (2004). Compensatory proliferation induced by cell death in the Drosophila wing disc requires activity of the apical cell death caspase dronc in a nonapoptotic role. Curr. Biol. 14 (14), 1262–1266. doi:10.1016/j.cub.2004.06.015
Huh, J. R., Vernooy, S. Y., Yu, H., Yan, N., Shi, Y., Guo, M., et al. (2003). Multiple apoptotic caspase cascades are required in nonapoptotic roles for Drosophila spermatid individualization. PLOS Biol. 2 (1), e15. doi:10.1371/journal.pbio.0020015
Hung, R.-J., Li, J. S. S., Liu, Y., and Perrimon, N. (2021). Defining cell types and lineage in the Drosophila midgut using single cell transcriptomics. Curr. Opin. Insect Sci. 47, 12–17. doi:10.1016/j.cois.2021.02.008
Jiang, H., and Edgar, B. A. (2011). Intestinal stem cells in the adult Drosophila midgut. Exp. Cell Res. 317 (19), 2780–2788. doi:10.1016/j.yexcr.2011.07.020
Jiménez Fernández, D., and Lamkanfi, M. (2015). Inflammatory caspases: Key regulators of inflammation and cell death. Biol. Chem. 396 (3), 193–203. doi:10.1515/hsz-2014-0253
Julien, O., and Wells, J. A. (2017). Caspases and their substrates. Cell Death Differ. 24 (8), 1380–1389. doi:10.1038/cdd.2017.44
Kang, Y., Neuman, S. D., and Bashirullah, A. (2017). Tango7 regulates cortical activity of caspases during reaper-triggered changes in tissue elasticity. Nat. Commun. 8 (1), 603. doi:10.1038/s41467-017-00693-3
Kanuka, H., Kuranaga, E., Takemoto, K., Hiratou, T., Okano, H., and Miura, M. (2005). Drosophila caspase transduces Shaggy/GSK-3beta kinase activity in neural precursor development. EMBO J. 24 (21), 3793–3806. doi:10.1038/sj.emboj.7600822
Kanuka, H., Sawamoto, K., Inohara, N., Matsuno, K., Okano, H., and Miura, M. (1999). Control of the cell death pathway by dapaf-1, a Drosophila apaf-1/CED-4-related caspase activator. Mol. Cell 4 (5), 757–769. doi:10.1016/S1097-2765(00)80386-X
Kiehart, D. P., Franke, J. D., Chee, M. K., Montague, R. A., Chen, T.-l., Roote, J., et al. (2004). Drosophila crinkled, mutations of which disrupt morphogenesis and cause lethality, encodes fly myosin VIIA. Genetics 168 (3), 1337–1352. doi:10.1534/genetics.104.026369
Knoblich, J. A., Jan, L. Y., and Jan, Y. N. (1997). The N terminus of the Drosophila Numb protein directs membrane association and actin-dependent asymmetric localization. Proc. Natl. Acad. Sci. 94 (24), 13005–13010. doi:10.1073/pnas.94.24.13005
Kondo, S., Senoo-Matsuda, N., Hiromi, Y., and Miura, M. (2006). DRONC coordinates cell death and compensatory proliferation. Mol. Cell. Biol. 26 (19), 7258–7268. doi:10.1128/MCB.00183-06
Koto, A., Kuranaga, E., and Miura, M. (2009). Temporal regulation of Drosophila IAP1 determines caspase functions in sensory organ development. J. Cell Biol. 187 (2), 219–231. doi:10.1083/jcb.200905110
Lee, P., Lee, D. J., Chan, C., Chen, S. W., Ch'en, I., and Jamora, C. (2009). Dynamic expression of epidermal caspase 8 simulates a wound healing response. Nature 458 (7237), 519–523. doi:10.1038/nature07687
Li, M., Sun, S., Priest, J., Bi, X., and Fan, Y. (2019). Characterization of TNF-induced cell death in Drosophila reveals caspase- and JNK-dependent necrosis and its role in tumor suppression. Cell Death Dis. 10 (8), 613. doi:10.1038/s41419-019-1862-0
Lindblad, J. L., Tare, M., Amcheslavsky, A., Shields, A., and Bergmann, A. (2021). Non-apoptotic enteroblast-specific role of the initiator caspase Dronc for development and homeostasis of the Drosophila intestine. Sci. Rep. 11 (1), 2645. doi:10.1038/s41598-021-81261-0
McIlwain, D. R., Berger, T., and Mak, T. W. (2013). Caspase functions in cell death and disease. Cold Spring Harb. Perspect. Biol. 5 (4), a008656. doi:10.1101/cshperspect.a008656
Meier, P., Silke, J., Leevers, S. J., and Evan, G. I. (2000). The Drosophila caspase DRONC is regulated by DIAP1. EMBO J. 19 (4), 598–611. doi:10.1093/emboj/19.4.598
Micchelli, C. A., and Perrimon, N. (2006). Evidence that stem cells reside in the adult Drosophila midgut epithelium. Nature 439 (7075), 475–479. doi:10.1038/nature04371
Mollereau, B., Perez-Garijo, A., Bergmann, A., Miura, P., Gerlitz, O., Ryoo, H. D., et al. (2013). Compensatory proliferation and apoptosis-induced proliferation: a need for clarification. Cell Death Differ. 20 (1), 181. doi:10.1038/cdd.2012.82
Ohlstein, B., and Spradling, A. (2006). The adult Drosophila posterior midgut is maintained by pluripotent stem cells. Nature 439 (7075), 470–474. doi:10.1038/nature04333
Orme, M. H., Liccardi, G., Moderau, N., Feltham, R., Wicky-John, S., Tenev, T., et al. (2016). The unconventional myosin CRINKLED and its mammalian orthologue MYO7A regulate caspases in their signalling roles. Nat. Commun. 7 (1), 10972. doi:10.1038/ncomms10972
Ouyang, Y., Petritsch, C., Wen, H., Jan, L., Jan, Y. N., and Lu, B. (2011). Dronc caspase exerts a non-apoptotic function to restrain phospho-Numb-induced ectopic neuroblast formation in Drosophila. Development 138 (11), 2185–2196. doi:10.1242/dev.058347
Pérez-Garijo, A., Martín, F. A., and Morata, G. s. (2004). Caspase inhibition during apoptosis causes abnormal signalling and developmental aberrations in Drosophila. Development 131 (22), 5591–5598. doi:10.1242/dev.01432
Riedl, S. J., and Shi, Y. (2004). Molecular mechanisms of caspase regulation during apoptosis. Nat. Rev. Mol. Cell Biol. 5 (11), 897–907. doi:10.1038/nrm1496
Rodriguez, A., Oliver, H., Zou, H., Chen, P., Wang, X., and Abrams, J. M. (1999). Dark is a Drosophila homologue of Apaf-1/CED-4 and functions in an evolutionarily conserved death pathway. Nat. Cell Biol. 1 (5), 272–279. doi:10.1038/12984
Ross, C., Chan, A. H., von Pein, J. B., Maddugoda, M. P., Boucher, D., and Schroder, K. (2022). Inflammatory caspases: Toward a unified model for caspase activation by inflammasomes. Annu. Rev. Immunol. 40 (1), 249–269. doi:10.1146/annurev-immunol-101220-030653
Ryoo, H. D., Gorenc, T., and Steller, H. (2004). Apoptotic cells can induce compensatory cell proliferation through the JNK and the wingless signaling pathways. Dev. Cell 7 (4), 491–501. doi:10.1016/j.devcel.2004.08.019
Ryoo, H. D., and Bergmann, A. (2012). The role of apoptosis-induced proliferation for regeneration and cancer. Cold Spring Harb Perspect Biol 4 (8), a008797. doi:10.1101/cshperspect.a008797
Shinoda, N., Hanawa, N., Chihara, T., Koto, A., and Miura, M. (2019). Dronc-independent basal executioner caspase activity sustains Drosophila imaginal tissue growth. Proc. Natl. Acad. Sci. 116 (41), 20539–20544. doi:10.1073/pnas.1904647116
Simpson, P., and Marcellini, S. (2006). The origin and evolution of stereotyped patterns of macrochaetes on the nota of cyclorraphous Diptera. Heredity 97 (3), 148–156. doi:10.1038/sj.hdy.6800874
Snipas, S. J., Drag, M., Stennicke, H. R., and Salvesen, G. S. (2008). Activation mechanism and substrate specificity of the Drosophila initiator caspase DRONC. Cell Death Differ. 15 (5), 938–945. doi:10.1038/cdd.2008.23
Song, Z., McCall, K., and Steller, H. (1997). DCP-1, a Drosophila cell death protease essential for development. Science 275 (5299), 536–540. doi:10.1126/science.275.5299.536
Sun, G., Ding, X. A., Argaw, Y., Guo, X., and Montell, D. J. (2020). Akt1 and dCIZ1 promote cell survival from apoptotic caspase activation during regeneration and oncogenic overgrowth. Nat. Commun. 11 (1), 5726. doi:10.1038/s41467-020-19068-2
Tang, H. L., Tang, H. M., Fung, M. C., and Hardwick, J. M. (2015). In vivo CaspaseTracker biosensor system for detecting anastasis and non-apoptotic caspase activity. Sci. Rep. 5, 9015. doi:10.1038/srep09015
Terry, M. R., Arya, R., Mukhopadhyay, A., Berrett, K. C., Clair, P. M., Witt, B., et al. (2015). Caspase-2 impacts lung tumorigenesis and chemotherapy response in vivo. Cell Death Differ. 22 (5), 719–730. doi:10.1038/cdd.2014.159
Vandenabeele, P., Galluzzi, L., Vanden Berghe, T., and Kroemer, G. (2010). Molecular mechanisms of necroptosis: An ordered cellular explosion. Nat. Rev. Mol. Cell Biol. 11 (10), 700–714. doi:10.1038/nrm2970
Villars, A., Matamoro-Vidal, A., Levillayer, F., and Levayer, R. (2022). Microtubule disassembly by caspases is an important rate-limiting step of cell extrusion. Nat. Commun. 13 (1), 3632. doi:10.1038/s41467-022-31266-8
Wang, L.-H., and Baker, N. E. (2019). Salvador–Warts–Hippo pathway regulates sensory organ development via caspase-dependent nonapoptotic signaling. Cell Death Dis. 10 (9), 669. doi:10.1038/s41419-019-1924-3
Williams, D. W., Kondo, S., Krzyzanowska, A., Hiromi, Y., and Truman, J. W. (2006). Local caspase activity directs engulfment of dendrites during pruning. Nat. Neurosci. 9 (10), 1234–1236. doi:10.1038/nn1774
Xu, D., Li, Y., Arcaro, M., Lackey, M., and Bergmann, A. (2005). The CARD-carrying caspase Dronc is essential for most, but not all,developmental cell death in Drosophila. Development 132 (9), 2125–2134. doi:10.1242/dev.01790
Xu, D., Wang, C., Shen, X., Yu, Y., Rui, Y., Zhang, D., et al. (2013). Apoptotic block in colon cancer cells may be rectified by lentivirus mediated overexpression of caspase-9. Acta Gastroenterol. Belg 76 (4), 372–380.
Xu, D., Wang, Y., Willecke, R., Chen, Z., Ding, T., and Bergmann, A. (2006). The effector caspases drICE and dcp-1 have partially overlapping functions in the apoptotic pathway in Drosophila. Cell Death Differ. 13 (10), 1697–1706. doi:10.1038/sj.cdd.4401920
Xu, D. C., Wang, L., Yamada, K. M., and Baena-Lopez, L. A. (2005). Non-apoptotic activation of Drosophila caspase-2/9 modulates JNK signaling, the tumor microenvironment, and growth of wound-like tumors. Cell Reports 39 (3), 110718. doi:10.1016/j.celrep.2022.110718
Keywords: apoptosis, initiator caspase, Dronc, non-lethal role, non-apoptotic function, Drosophila
Citation: Dominguez D and Fan Y (2023) Non-lethal roles of the initiator caspase Dronc in Drosophila. Front. Cell. Death 2:1184041. doi: 10.3389/fceld.2023.1184041
Received: 10 March 2023; Accepted: 30 March 2023;
Published: 06 April 2023.
Edited by:
Bertrand Mollereau, Université de Lyon, FranceReviewed by:
Luis Alberto Baena-Lopez, University of Oxford, United KingdomRomain Levayer, Institut Pasteur, France
Copyright © 2023 Dominguez and Fan. This is an open-access article distributed under the terms of the Creative Commons Attribution License (CC BY). The use, distribution or reproduction in other forums is permitted, provided the original author(s) and the copyright owner(s) are credited and that the original publication in this journal is cited, in accordance with accepted academic practice. No use, distribution or reproduction is permitted which does not comply with these terms.
*Correspondence: Yun Fan, y.fan@bham.ac.uk