- 1Kunshan Hospital of Chinese Medicine, Affiliated Hospital of Yangzhou University, Kunshan, China
- 2College of Pharmaceutical Sciences, Soochow University, Suzhou, Jiangsu, China
- 3Jiangsu Province Engineering Research Center of Precision Diagnostics and Therapeutics Development, Soochow University, Suzhou, China
- 4Department of Clinical Medical Research Center, Affiliated Hospital of Nantong University, Nantong, China
Gastric cancer is a common malignancy characterized by an insidious onset and high mortality rate. Exosomes, a special type of extracellular vesicle, contain various bioactive molecules and have been found to play crucial roles in maintaining normal physiological functions and homeostasis in the body. Recent research has shown that the contents of exosome play a significant role in the progression and metastasis of gastric cancer through communication and regulatory functions. These mechanisms involve promoting gastric cancer cell proliferation and drug resistance. Additionally, other cells in the gastric cancer microenvironment can regulate the progression of gastric cancer through exosomes. These include exosomes derived from fibroblasts and immune cells, which modulate gastric cancer cells. Therefore, in this review, we provide a brief overview of recent advances in the contents and occurrence mechanisms of exosome. This review specifically focused on the regulatory mechanisms of exosomes derived from gastric cancer and other cellular subtypes in the tumor microenvironment. Subsequently, we summarize the latest research progress on the use of exosomes in liquid biopsy, discussing the potential of gastric cancer exosomes in clinical applications.
1 Introduction
Gastric cancer (GC) was ranked as one of the most common cancers globally. Due to its insidious onset, diagnosis often occurs at an advanced stage, contributing to a high mortality rate (Smyth et al., 2020). Studies indicated that more than one million people are diagnosed with gastric cancer each year, with a higher incidence in East Asia, possibly linked to dietary habits and lifestyle factors (Oliveira et al., 2015; Lee et al., 2016; Thrift and El-Serag, 2020). Therefore, early detection of treatable GC is critical to improving patients’ long-term prognosis, underscoring the need for the development of innovative noninvasive biomarkers with high specificity and sensitivity for early GC screening.
Various classification systems exist for GC. As early as 1971, Japanese scholars classified early GC into three types based on morphological features: protruding, superficial, and depressed. The Paris endoscopic classification supplemented this, incorporating contributions from research groups worldwide (Participants in the Paris Workshop, 2003). Other classification methods include the Bormann classification and the Lauren classification (Yang et al., 2007; Panani, 2008). Researchers commonly use histological classification when studying the pathophysiological mechanisms of GC, with 90% of cases exhibiting adenocarcinoma. In clinical practice, the TNM staging system is employed to assess the progression of GC and guide appropriate treatment. Although new staging methods have been proposed after neoadjuvant therapy, further clinical validation is needed. Molecular advances have increased our understanding of GC biomarkers (Chia and Tan, 2016), and single-cell techniques may offer insights into molecular and biological markers for future classification and staging, although these methods have not yet been standardized. Advancements in medical technology, increased health awareness, and the development of diagnostic tools such as endoscopy, novel targeted drugs and immunotherapy have contributed to a gradual decline in the diagnosis and mortality rates of GC. The increasing average lifespan and progress in healthcare suggest that cancer, including GC, may become more prevalent as a chronic disease in the future (Sarfati et al., 2016; De Magalhaes, 2013). Early diagnosis, reducing mortality, and improving patients’ quality of life pose key challenges for future healthcare professionals and researchers. This underscores the importance of in-depth research into GC, especially exploring its pathogenesis and developing new diagnostic methods to address future challenges (Rocken, 2023; Zhang C. et al., 2023; Sugano et al., 2023).
Exosomes are a class of Extracellular Vesicles (EVs) produced by donor cells, which can be used as an effective intercellular communication tool to transmit a series of molecules to recipient cells (Kalluri and LeBleu, 2020; Zhang M. et al., 2023). Exosomes are normally around 30 nm–150 nm. On the surface of exosomes, proteins or recognition components that enhance their targeting ability can potentially aid in exosomes evasion of recognition and attack by the immune system (Yang et al., 2019). Exosomes were initially considered platelet dust, vesicles involved in the cellular excretion of metabolic waste (Couch et al., 2021). However, current research indicates that exosomes possess the following characteristics: they play a highly targeted role in cellular communication and participate in physiological activities of cells, including the regulation of cell growth and apoptosis (Wu et al., 2022). In studies related to exosomes and cancer, exosomes were shown to influence the metastasis and growth of tumors, including the regulation of immune cell functions (Liu et al., 2016; Li M. Y. et al., 2021). Additionally, tumor cells can transfer certain characteristics, such as drug resistance, through exosomes (Mashouri et al., 2019) and the identities of the tumor cells could be reflected in the exosomal cargos. As substances secreted by almost all cells, exosomes from different cell sources exhibit functional diversity; for instance, immune cells can regulate the growth of tumor cells through exosomes (Xu et al., 2020). Currently, exosomes play a crucial role in the occurrence and development of several cancers, such as lung cancer, pancreatic cancer, and liver cancer (Ariston Gabriel et al., 2020; Wang et al., 2019; Khan et al., 2023). Similarly, exosomes also impact on the occurrence and development of GC. Furthermore, exosomes can be maintained steadily in a variety of body fluids because the lipid bilayer structure adequately shields the contents from degradation, which suggests that exosomes could be an effective option for dependable biomarkers (Wu et al., 2021). Exosomes and their derived cargos have been exploited as new indicators for cancer evaluation and prediction, as evidenced by an increasing volume of examples in the literature (Tang et al., 2021). Exosomes have also been utilized for cancer treatment to transport biological materials and chemotherapeutic medications, due to its transport and stability characteristics. For instance, Exosomes are thought to be effective delivery systems for RNA-based treatment approaches, which open a new horizon for nano-therapeutic strategies in caner field (Zhang M. et al., 2023; Wandrey et al., 2023). Exosomes that have been edited to include internal therapeutic compounds and surface ornamentation are commonly referred to as engineered exosomes. Engineered exosomes may effectively and accurately carry anticancer medications to tumor locations with fewer treatment-related side effects (Figure 1) (Sadeghi et al., 2023; Zhang F. et al., 2023).
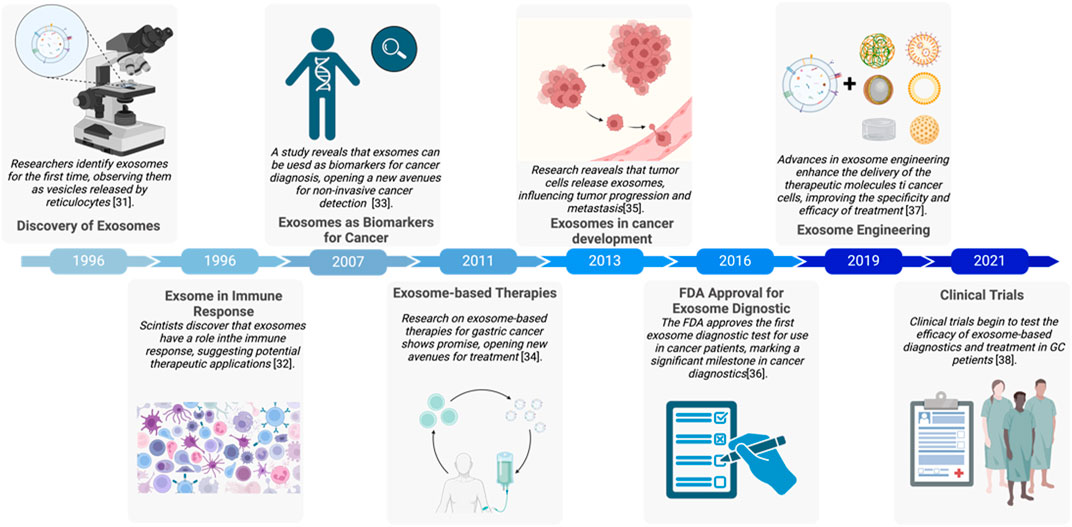
Figure 1. Major events of exosomes in gastric cancer development (Raposo et al., 1996; Chaput et al., 2005; Kok and Yu, 2020; Zhang Y. et al., 2020; Azmi et al., 2013; Masyuk et al., 2013; Liang et al., 2021; Rezaie et al., 2022). The figure was created by Biorender (https://www.biorender.com/).
This article primarily summarizes recent advances in exosome-related research in the field of basic GC, including the regulation of tumor growth by tumor-derived exosomes, the interplay between tumor microenvironment (TME) and tumor-derived exosomes, and the study of GC exosomes in clinical applications. Finally, we discuss engineered strategies for exosome therapy and provide an outlook on future research findings.
2 Classification and main contents of exosomes
2.1 Difference between exosomes and microvesicles
In fact, exosomes are a subtype of EVs produced by donor cells exosomes. The EVs mainly include exosomes and microvesicles, and if we do not consider their biological functions, their differences are primarily reflected in their origin and size. Microvesicles are formed by budding directly from the plasma membrane; these vesicles have diameters ranging from 50 to 500 nm and can even reach 1 μm. Exosomes, on the other hand, are formed by the fusion of multivesicular bodies (MVBs) with the plasma membrane, releasing intraluminal vesicles (ILVs) from the luminal cavity of MVBs, resulting in exosomes with diameters ranging from 30 to 150 nm (van Niel et al., 2018). More than two fold as many exosomes are found in the blood of cancer patients than in the blood of healthy people, highlighting the importance of exosomes and their very active intercellular communication in cancer (Zhang and Yu, 2019). Some cell types have been found to employ unique methods for exosome generation; for example, T cells can produce exosomes on the cell surface by utilizing the plasma membrane (Booth et al., 2006). It is noticeable that microvesicles and exosomes are overlapping in size and have similar appearances, so exosomes are primarily identified by marker proteins.
2.2 Exosomes play different functions depending on source and cargoes
Exosomes from different sources exhibit distinct biological functions, which may be related to the specific structures of the source cells, and they also differ in their targeting ability (Zhang M. et al., 2023; Gao et al., 2018). Furthermore, in practical research, the process of extracting exosomes may involve other vesicle structures, such as apoptotic bodies and ectosomes, collectively leading to the formation of extracellular vesicles (Willms et al., 2016).
Exosomes biogenesis initiates with endosome formation, which occurs when cells internalize extraneous materials via endocytosis. Over time, early endosomes progress into late endosomes, giving rise to endocytic vesicles through membrane invagination. These vesicles become enclosed within endosomes and ultimately develop into MVBs, a pivotal stage in exosomes generation. Endocytic vesicles transport distinct proteins, RNA, lipids, and various molecules (Kalluri and LeBleu, 2020). Exosomes biogenesis initiates with endosome formation, which occurs when cells internalize extraneous materials via endocytosis. Over time, early endosomes progress into late endosomes, giving rise to endocytic vesicles through membrane invagination. These vesicles become enclosed within endosomes and ultimately develop into MVBs, a pivotal stage in exosomes generation. Endocytic vesicles transport distinct proteins, RNA, lipids, and various molecules (Colombo et al., 2014). The ESCRT system comprises four main complexes (ESCRT-0, ESCRT-I, ESCRT-II, and ESCRT-III) along with associated cofactors. Responsible for cargo sorting and MVBs formation, ESCRT plays a crucial role. Rab GTPases, such as Rab 27a, Rab 27b, and Rab35 (Ostrowski et al., 2010), govern the transport and fusion of MVBs with plasma membranes. Exosomal cargoes (e.g., miRNAs, proteins, lipids) undergo selective, rather than random, loading through specific mechanisms. miRNAs are regulated by RNA-binding proteins (e.g., hnRNPA2B1, YBX1) or specific sequence signals (Villarroya-Beltri et al., 2013; Garcia-Martin et al., 2022). Proteins are packaged into exosomes through ubiquitination, ESCRT-dependent or ESCRT-independent pathways (Figure 2) (Thery et al., 2001; Li X. et al., 2020). Lipids are sorted via lipid rafts or specific lipid transporters (Crivelli et al., 2022; Wei et al., 2021). Furthermore, heat shock proteins (e.g., HSP70) play a role in endosomal sorting, highlighting the diverse mechanisms involved in their biogenesis (Gobbo et al., 2016).The type and abundance of exosomal contents are typically determined by the state of the donor cells, including the physiological and pathological conditions of these cells. Various interventions may also influence the production of exosomes (Debbi et al., 2022; Jafari et al., 2020). The contents of exosomes include RNA, proteins, lipids, DNA, etc. (Figure 2), and exosomes carrying specific cargo can perform particular functions (Kimiz-Gebologlu and Oncel, 2022; Tenchov et al., 2022). This topic is a primary focus of current exosomes research. Understanding the contents of exosomes is crucial for studying GC, as it helps in comprehending how exosome secretion may vary with changes in TME.
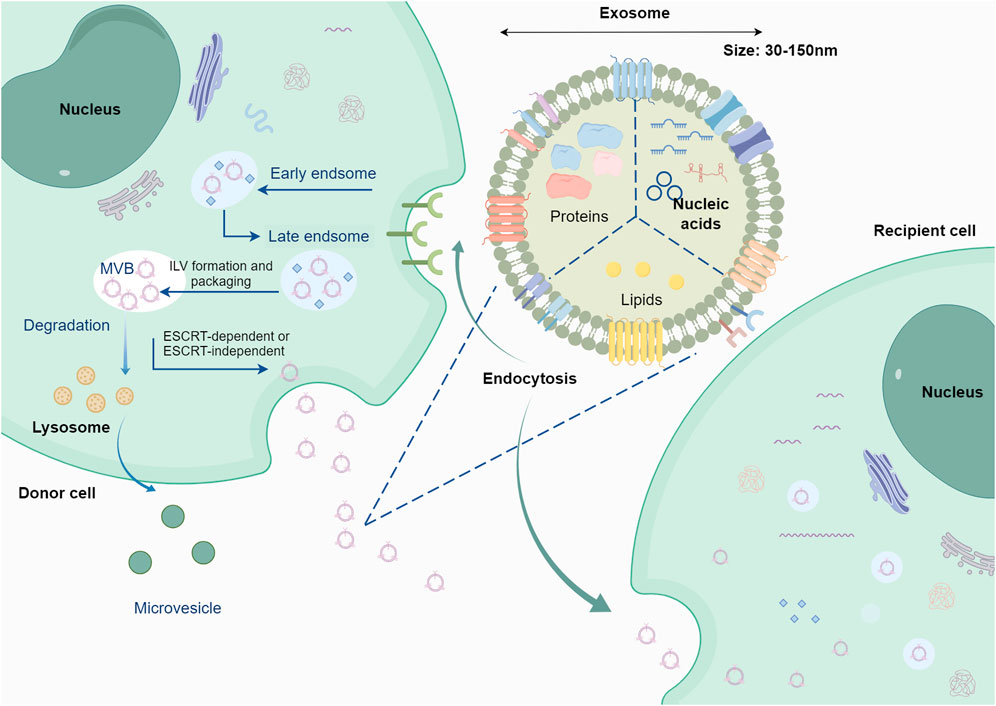
Figure 2. Origin and cargo of exosomes. Following the processes of early endosomes and late endosomes, exosomes are formed through the fusion of multivesicular endosomes with the plasma membrane, releasing intraluminal vesicles into the lumen of the multivesicular endosome. Exosomes contain various cargoes associated with the parent cell, including nucleic acids, proteins, lipids, etc. The figure was created by Figdraw (www.figdraw.com).
3 Interactions of exosomes in the progression of GC
Tumors constantly interact with their surrounding environment through interactions involving immune regulation, such as immune cells attacking tumors. As tumors undergo continuous changes, they could evade immune cell attack (Demaria et al., 2019; Miller and Sadelain, 2015). The interactions between exosomes and tumors are similar to this dynamic. Tumor cells themselves can secrete exosomes, known as tumor-derived exosomes, and surrounding immune cells can also release exosomes. Exosomes from different sources exhibit heterogeneity in their contents and biological functions. For example, tumor-derived exosomes can transfer drug resistance between tumor cells, regulate tumor immune evasion and promote tumor cell growth or metastasis by activating or inhibiting certain signaling pathways of recipient cells through transferring certain chemical compounds to modulate the development of the tumor process (Isaac et al., 2021). Noncoding RNA (ncRNA) is one of the groups of bioactive molecules included in exosomes that work as the main carrier of information transfer between tumors cells. Examples of ncRNA include microRNAs (miRNAs), circular RNAs (circRNAs), and long noncoding RNAs (lncRNAs) (Lakshmi et al., 2021; Chang and Wang, 2019). On the other hand, exosomes from other cell sources, such as immune cell-derived exosomes generated from NK cells and T cells, play a role in killing tumor cells and inhibiting tumor growth (Kang et al., 2021; Zhu et al., 2017). Therefore, when discussing the regulatory role of exosomes in the occurrence and development of GC, it is essential to clarify the source of exosomes and discuss them separately based on their origins. From the perspective of tumor interactions, tumor-derived exosomes may be potential biomarkers for predicting tumor development. From the perspective of tumor killing, immune cell-derived exosomes could be used as therapeutic targets for controlling tumor development.
3.1 Research on the key components in GC exosomes that promote cancer proliferation and metastasis
The first study on GC exosomes and their association with tumor proliferation was published in 2009. Qu et al. reported that exosomes produced by the GC cell line SGC7901 could promote tumor cell proliferation by activating the PI3K/AKT and MAPK/ERK pathways (Qu et al., 2009). In comparison to early studies on GC and exosomes, current studies delve deeper into the specific components that play a role in exosomes. In a study by Qiu et al., exosomes produced by GC cells were shown to impact tumor metastasis. They observed significantly elevated levels of miR-519a-3p in the serum of patients with GC and liver metastasis compared to patients without liver metastasis. Researchers have also noted that exosomes produced by GC cells are internalized by macrophages in the liver. MiR-519a-3p in exosomes targeted DUSP2, activating the MAPK/ERK pathway and inducing M2 polarization in macrophages. This process promotes angiogenesis and accelerates GC liver metastasis (Qiu et al., 2022).
Exosomes can deliver miRNAs, and abundant research has been conducted in this area (Sun et al., 2018a). GC exosomes have been found to induce peritoneal fibrosis and mesothelial-to-mesenchymal transition (MMT), promoting GC metastasis (Deng et al., 2017). Researchers identified miRNA-21-5p in exosomes produced by GC cells, which can be internalized by peritoneal mesothelial cells (PMCs). This internalization activates the TGF-/Smad pathway, promoting peritoneal metastasis in cancer (Li et al., 2018). miRNA-106aβ in GC exosomes is believed to promote peritoneal metastasis by regulating Smad7, and the histological basis for this promoting mechanism is disruption of the mesothelial barrier (Zhu et al., 2020; Zhu et al., 2022). miRNA-15b-3p was detected in the serum of GC patients and in exosomes derived from GC cells. It is associated with poor overall survival in patients and enhances GC migration and proliferation by inhibiting the expression of the apoptosis-related proteins caspase-3, caspase-9, and DYNLT1 (Zhu et al., 2022). GC exosomes can also increase adhesion between GC cells and mesothelial cells, promoting tumor metastasis (Arita et al., 2016). These findings suggested that researchers may be able to inhibit GC metastasis by downregulating the expression of specific miRNAs in exosomes.
In comparison to miRNAs, circRNAs exhibit better stability and can function as regulators of gene expression by serving as miRNA sponges (Chen, 2020; Memczak et al., 2013; Hansen et al., 2013). CircRNAs have also been found in exosomes and play crucial roles in cell proliferation and disease progression (Dai et al., 2020; Li C. et al., 2021). Studies have shown that circNRIP1 can be transmitted through GC cell exosomes, where it promotes GC cell proliferation by regulating the AKT1/mTOR pathway. This finding suggested that specific circRNAs carried by GC exosomes can enhance GC cell proliferation (Zhang et al., 2019). Similarly, another study revealed that circITTCH can act as a sponge for miRNA-199a-5p, suppressing GC metastasis by increasing Klotho expression (Wang Y. et al., 2021). CircNEK9 was found to promote GC progression through the miRNA-409-3p/MAP7 axis (Yu et al., 2021). Circ_0004104 was shown to target RNF2, accelerating GC progression (Yue et al., 2021). CircUBE2Q2 was shown to promote malignant progression in GC by mediating autophagy and the glycolytic pathway (Yang J. et al., 2021). In addition, exosome-derived circATP8A1 from GC cells induce macrophages M2 polarization via the circATP8A1/miR-1-3p/STAT6 axis, and tumor progression (Deng et al., 2024). Similarly, exosomal circMAN1A2 competed with FBXW11 for binding to SFPQ, preventing FBXW11-mediated k48-linked ubiquitination and SFPQ protein degradation, thereby stabilizing SFPQ expression.CircMAN1A2 can be encapsulated by hnRNPA2B1 in exosomes and can be taken up by T cells, thus affecting antitumour immunity (Shen et al., 2025). Additionally, circRNAs, including RELL1, regulate g GC progression through the autophagy pathway (Sang et al., 2022).
Exosomes can also carry lncRNAs (Liu R. et al., 2019; Sun et al., 2018b). HOX transcript antisense RNA (HOTAIR) has been detected in the peripheral blood serum and tumor tissues of GC patients, and its overexpression can increase tumor growth and metastatic capabilities (Chen P. et al., 2023; Zhang J. et al., 2020). Similarly, the lncRNA TTN-AS1 promotes GC cell growth and migration by enhancing CDX2 expression (Wang et al., 2023). The lncRNA SPRY4-IT1 regulates cell proliferation and migration in GC by modulating the AMPK pathway (Fan et al., 2019). Exosomes secreted by GC cells containing a lncRNA (lncAKR1C2) can enhance lymph node metastasis in GC (Zhu et al., 2023). Exosomal LINC01480 can promote the proliferation, migration and invasion of GC cells by upregulating VCAM1 expression through competitive binding with miR-204-5p (Zhang Y. et al., 2024). Similarly, exosomal LINC00355 promotes the malignant progression of GC through histone deacetylase HDAC3-mediated TP53INP1 transcriptional inhibition (Zhao et al., 2023).
Proteins in exosomes include membrane proteins and glycoproteins anchored on the surface, and these proteins have been found to play important roles in biological processes (Wang X. et al., 2022; Salunkhe et al., 2020). Exosomes secreted by GC cells contain CD97 on the surface. In vitro experiments have shown that knocking down CD97 expression can inhibit tumor metastasis. This promoting mechanism occurs by increasing the expression of epithelial adhesion molecules, and CD97-enriched exosomes can facilitate lymphatic metastasis of GC cells (Shen X. et al., 2022). CD44 in exosomes derived from GC cells has also been found to promote lymphatic metastasis (Wang M. et al., 2022). Exosomes secreted by GC cells can carry epidermal growth factor receptor (EGFR) and integrate EGFR into the plasma membrane of liver cells. This translocation of EGFR from cancer cells to liver cells can inhibit the action of miRNA-26a/b, activate hepatocyte growth factor (HGF), and promote liver metastasis of cancer cells (Zhang et al., 2017). Another example of such a protein is FZD10, which can be identified as a protein in exosomes from various cancers that can sustain the proliferation of GC cells (Scavo et al., 2019). Exosomal proteins are expressed mainly in parent cells, reflecting their biological characteristics. Moreover, membrane proteins such as CD97 can enhance the adhesion efficiency of exosomes, suggesting that regulating the expression of specific proteins on the surface of exosomes can also modulate their function. Therefore, Inhibiting the biological effects of some exosomal proteins can effectively inhibit their growth-promoting and migration-promoting effects on recipient cells.
3.2 The regulatory role of GC-derived exosomes in malignant tumor behavior
During the development and treatment of GC, tumor cells continuously adapt through self-renewal or progressive mutation, enhancing their ability to spread and develop resistance to therapeutic drugs. Malignant behaviors such as angiogenesis, epithelial–mesenchymal transition (EMT), and drug resistance in tumor cells are closely associated with the difficulty of cancer treatment and the metastatic potential of tumor cells. Recent research has also revealed the regulatory role of GC exosomes in these behaviors (Yan et al., 2017; Eguchi et al., 2022).
Tumor angiogenesis is a common malignant event in the growth and metastasis of tumors and provides oxygen and nutrients for the growth of tumor cells through the formation of new blood vessels (Jiang et al., 2020). In exosomes derived from GC, the significantly elevated expression of the X26 nt target VE-cadherin promoted angiogenesis and vascular permeability (Chen et al., 2021). Exosomes produced by GC cells involved in peritoneal metastasis exhibit a decreased level of miRNA-486-5p, promoting the EMT process and facilitating peritoneal metastasis (Lin et al., 2021). Additionally, researchers have shown that miRNA-196a-1 contained in exosomes from GC cells can mediate the transfer of invasive cells between highly invasive and minimally invasive cells (Feng et al., 2019). Studies have shown that ascites-derived exosomes enhanced tumor invasiveness and neo-angiogenesis. A common conclusion from these studies is that exosomes serve as carriers for malignant behaviors in tumors (Hyung et al., 2023).
Chemotherapy is the primary approach for the late-stage treatment of GC patients (Johnston and Beckman, 2019). Drug resistance is one of the reasons for poor patient prognosis, and exosomes can serve as tools for the treatment of drug resistance. Studies have shown that GC cells resistant to cisplatin can transfer the resistant phenotype to recipient cells through exosomes. The mechanism involves miR-769-5p in exosomes regulating Caspase9, promoting the ubiquitination and degradation of p53, thus achieving drug resistance (Jing et al., 2022). Resistant GC cells can transfer drug resistance through miRNA-501 in the exosomes they produce (Liu X. et al., 2019).
Given the significant role of GC cell-derived exosomes in the progression of GC, researchers have wondered whether controlling the secretion of exosomes could be a way to control the progression of GC. It has been observed that reducing the expression level of Rab27b can decrease exosome secretion, thereby alleviating peritoneal metastasis in GC. Additionally, exosomes from other organs have been found to induce cancer-derived exosomes apoptosis (Chen et al., 2023b). This once again confirmed that exosomes from different sources have distinct biological functions.
Exosomes are also intermediate mediators of other molecules that regulate the development of cancer. Study reported that when RAB31 expression was decreased, there was a decrease in both the quantity and size of exosomes released by GC cells, as shown by exosome nanoparticle tracking analysis and electron microscopy. This discovery demonstrated that RAB31 regulates exosome secretion, a critical function in GC metastasis (Wu et al., 2023).
Exosomes may also serve as tools that are actively expelled by cancer cells and are unfavorable for cancer progression. Researchers have shown differential expression of miRNA-410-3p in exosomes from the supernatant of primary GC tissue and cultured GC cells. Increased expression levels of miRNA-410-3p were shown to inhibit the progression of primary GC (Liu and Chu, 2023). However, this raises the question of whether tumor-suppressive miRNAs can be targeted for drug delivery. This still needs to be approached with caution, as in the case of this study, while miRNA-410-3p acts as an anticancer gene in GC, it may function as an oncogene in other organs, such as the liver or lungs. Different miRNAs may play anticancer roles in one type of cancer due to distinct biological principles, while in another type of cancer, the same pathway could be procarcinogenic (Alessandrini et al., 2018; Xu et al., 2023).
3.3 GC exosomes and the composition and function of the GC TME
The concept of the TME originated from the “seed and soil” theory and the relationship between inflammation and cancer (Paget, 1989). The TME constitutes the stroma of the tumor and consists of the tumor lesion itself, surrounding malignant or nonmalignant cells, blood vessels, immune cells, nerves, cellular metabolites, and more. Its main characteristics include low oxygen levels, low nutrient levels, and high lactate levels. The TME can be subdivided into various categories, such as the hypoxic microenvironment, immune microenvironment, metabolic microenvironment, acidic microenvironment, and neural regulatory microenvironment and mechanical microenvironment (Jin and Jin, 2020). At the same time, we must not ignore the exosomal microenvironment composed of exosomes regulated by the interaction between tumor cells and surrounding cells (Figure 3).
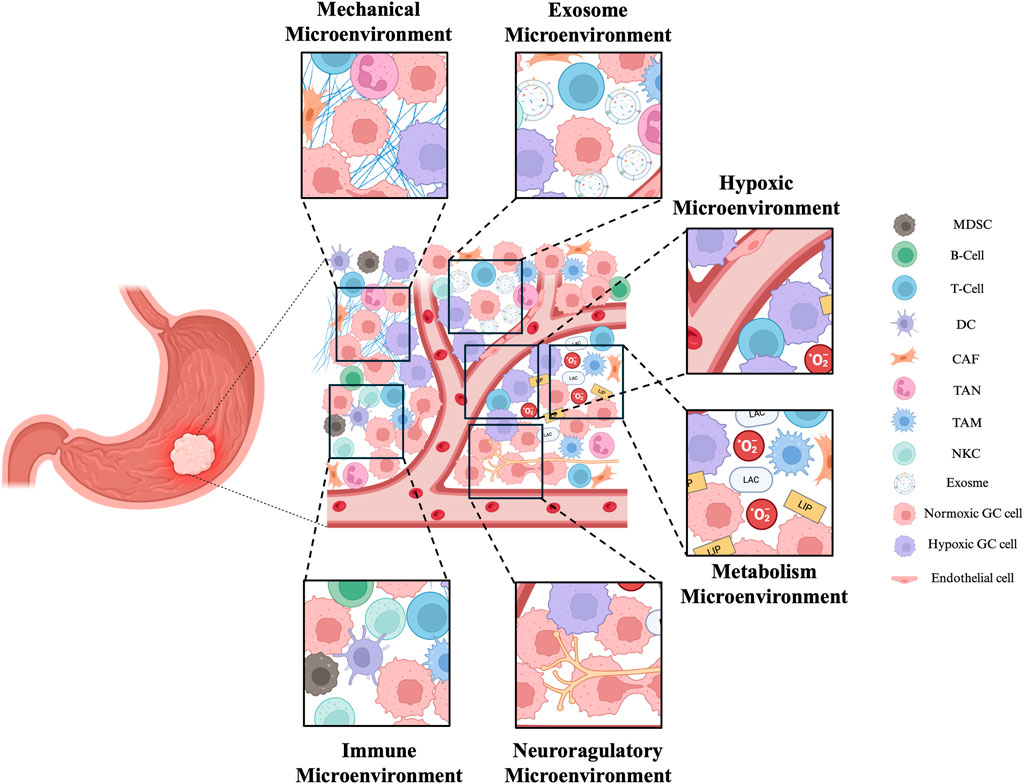
Figure 3. Exosomes have important roles in the TME. The complex TME in GC includes mechanical microenvironment, exosome microenvironment, hypoxic microenvironment, immune microenvironment, metabolism microenvironment and neuroregulatory microenvironment. Together, they affect the development of tumors. MDSC (Myeloid-derived suppressor cell), DC (Dendritic cell), CAF (Cancer-associated fibroblast), TAN (Tumor-associated neutrophile),TAM (tumor-associated macrophage), NK (natural killer cell). The figure was created by Biorender (https://www.biorender.com/).
Starting from the carcinogenic mutations produced by malignant cells, surrounding non-transformed cells are recruited, releasing cytokines. Together with tumor cells, these cells mutually regulate and collectively influence tumor progression (Figure 4, Table 1) (Balkwill et al., 2012; Deng et al., 2019). The TME of GC is composed of various cell types, including the extracellular matrix (ECM), fibroblasts, endothelial cells, mesenchymal stem cells, macrophages, T cells, dendritic cells, NK, neutrophils, and endothelial cells (Pitt et al., 2016; Chhabra and Weeraratna, 2023). These cells, in addition to the extracellular components they produce, constitute the TME, and the components of the TME in GC play their respective roles in inducing immune tolerance and promoting the development of GC (Liu et al., 2022; Mao et al., 2017). A pro-cancer local inflammatory environment is formed between GC cells and fibroblasts through INHBB/NF-κB/IL-1β (Jin et al., 2023). In GC peritoneal metastasis, SOX9 in tumor cells can inhibit T-cell cytotoxicity by promoting the secretion of interleukin 6 family cytokines and promoting the polarization of M2 macrophages, creating a microenvironment conducive to tumor growth and facilitating GC peritoneal metastasis (Fan et al., 2023). Tumor-associated macrophages (TAMs) are the predominant infiltrating immune cells in the TME (Mantovani and Allavena, 2015; Noy and Pollard, 2014). Research has shown that TAMs have protumor activity and are involved in mediating immunosuppression, angiogenesis and promoting tumor resistance and metastasis in immune reactions.
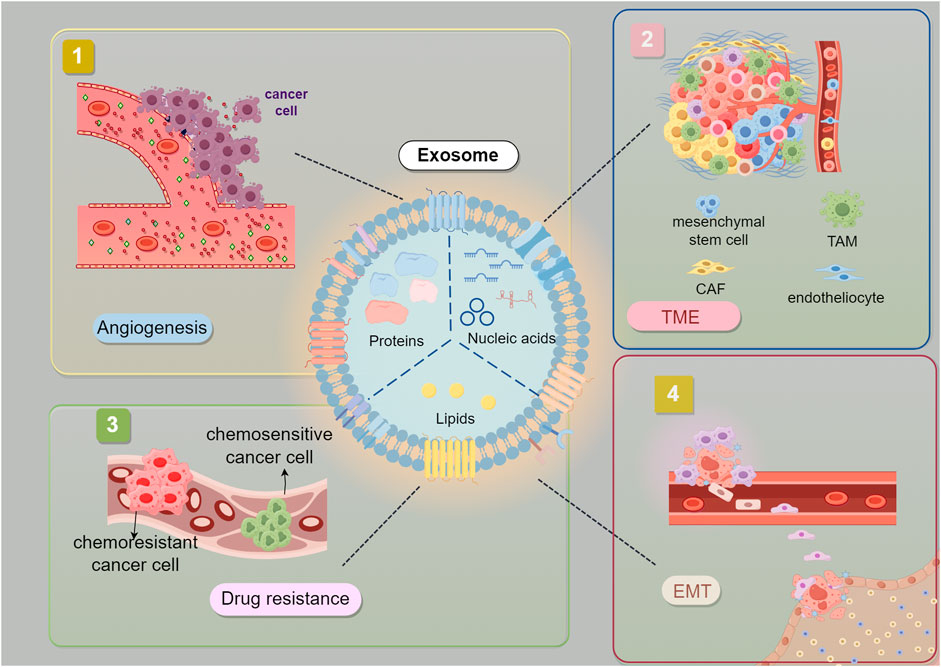
Figure 4. Interaction between Gastric Cancer-Derived Exosomes and TME. Exosomes derived from GC cells are believed to promote tumor EMT, angiogenesis, drug resistance. EMT (epithelial-mesenchymal transition) CAF (Cancer-associated fibroblast) TAM (tumor-associated macrophage).The figure was created by Figdraw (www.figdraw.com).
Research has revealed that cells present in the TME can regulate GC cells through exosomes. For instance, exosomes secreted by GC mesenchymal stem cells, which contain miR-221 and miR-374a-5p, promote the proliferation of GC cells (Wang et al., 2014; Ma et al., 2017; Ji et al., 2023). The research also demonstrates that ferroptosis-mediated oxaliplatin resistance and malignant transformation in GC are encouraged by the loss of cancer-associated fibroblast-derived exosomal DACT3-AS1 (Qu et al., 2023). Increased platelet levels also enhance the ability of bone marrow-derived mesenchymal stem cells (BMSCs) to promote cancer metastasis (Wang Q. et al., 2018). E Exosomes highly enriched with miRNA-223 from macrophage-derived exosomes can boost the migration and invasion abilities of GC cells (Zheng P. M. et al., 2020). Activated fibroblasts in the TME release exosomes overexpressing MMP11, and knocking down MMP11 has been confirmed to be related to the migration of GC cells (Xu et al., 2019). Interestingly, exosomes secreted by activated fibroblasts contain the miRNA-139, which is negatively correlated with MMP11 and can inhibit tumor progression by suppressing MMP11. GC cells secrete exosomes containing PKM2, activating the NF-κB pathway in fibroblasts and inducing an immunosuppressive microenvironment in GC (Fan et al., 2019). In addition to specific cell types, exosomes enriched with the circRNA hsa_circ_0000437 from adjacent tissues have been found to regulate the proliferative capacity of GC cells (Salunkhe et al., 2020). Melatonin has been shown to inhibit the invasion and proliferation of GC cells by modulating the expression of miRNA-27b-3p in GC exosomes (Zhang Y. Q. et al., 2023).
Hypoxia is a common microenvironment in the TME, and recent research has suggested that hypoxia in the TME may also be an indirect factor regulating the function of tumor exosomes. For instance, tumor-derived exosomes generated under hypoxic conditions have been found to promote tumor metastasis by mediating M2 macrophage polarization, and this effect is regulated by exosomal miRNA-301a, which modulates the PTEN/PI3Kγ pathway (Wang et al., 2018b). Researchers simulated a hypoxic environment under 1% oxygen and found that exosomes derived from GC cells cultured under hypoxic conditions enhanced the invasive capacity of GC cells cultured under normoxic conditions (20% O2). Subsequently, this phenomenon was found to be mediated by the overexpression of the lncRNA prostate cancer gene expression marker 1 (PCGEM1) in exosomes (Piao et al., 2021). Exosomes released from GC-derived exosomes under hypoxic conditions containing miRNA-301a-3p can promote the malignant behavior of GC by inhibiting HIF-1α degradation (Xia et al., 2020). Similarly, miRNA-199a-3p, which is released from exosomes under hypoxic conditions, promotes GC metastasis through MAP3K4 (Li L. et al., 2023).
Exosomes produced by GC cells can also regulate cells in the TME. For instance, researchers have shown that exosomes derived from GC cells accumulate extensively in the lungs and are phagocytosed by macrophages. This process induces immunosuppression in macrophages and increases PD-L1 expression, establishing a Pre-metastatic niche (PMN) for GC lung metastasis (Wang Y. et al., 2021). miR-605-3p suppresses vesicle-associated membrane protein 3 (VAMP3) expression, decreases exosome secretion, and concurrent inhibition of nitric oxide synthase 3 (NOS3) synthesis in cells, resulting in reduced NOS3 levels in exosomes. This process impeding multivesicular body trafficking to the cell membrane, thereby inhibiting tumor angiogenesis and PMN, consequently delaying the advancement of liver metastasis in GC (Hu et al., 2024). This regulatory effect is reciprocal, as exosomes from M2 macrophages containing miRNA-487a can promote GC progression (Xin et al., 2023). Exosomes from TAMs that carry miRNA-513b-5p promote GC progression (Zhang R. et al., 2024). GC exosomes also influence the gene expression of CD8+ T cells, shaping an appropriate TME for lung metastasis (Liu et al., 2020).
Exosomes secreted by GC cells can shape cells in the TME. One study indicated that GC cells from lymph node metastases regulate stromal stem cells at metastatic sites through exosome secretion to promote the malignant progression of GC (Wang M. et al., 2021). Another study revealed that GC cells can induce M2 polarization through exosomes, which results in the formation of a microenvironment conducive to tumor progression (Xin et al., 2021). GC cells induce glucose metabolism reprogramming of neutrophils through exosomes via the HMGB1/NF-κB pathway. This process mediates neutrophil N2 polarization by inhibiting SLIT2 expression through miR-4745-5p/3911, ultimately promoting GC metastasis (Zhang J. et al., 2024). Research has revealed that M2 TAMs-derived exosomes transported MALAT1 to GC cells, where MALAT1 interacted with β-catenin and inhibited its ubiquitination and degradation through β-TRCP. Furthermore, MALAT1 upregulated HIF-1α expression by acting as a sponge for miR-217-5p. M2-type TAM exosomes activate β-catenin and HIF-1α signaling pathways together to enhance aerobic glycolysis of GC cells (Wang et al., 2024).
In this section, we summarize recent research on the biological functions of GC exosomes. We also highlighted the role of exosomes from the TME in GC, including those produced by different cell types within the microenvironment. The composition of the TME is complex, with hypoxia and immune components being the two main signatures of the TME. These findings may lead to potential targets for exosome-based therapeutic strategies. Understanding the interplay between exosomes and various TME subtypes could enhance the combined therapeutic potential of exosomes. Research has suggested that exosomes in the TME are diverse, as almost every cell type in the TME can secrete exosomes (Yang et al., 2020). The potential interactions between the regulatory effects of these exosomes are a topic that warrants further investigation.
4 GC exosomes in clinical applications
4.1 As early diagnosis biomarkers
A low diagnostic rate, high metastasis rate, and high recurrence rate are common factors contributing to the poor prognosis of GC patients (Thrift and El-Serag, 2020). Currently used tumor markers for the clinical diagnosis of GC lack sensitivity and specificity. There are few clinically validated markers related to metastasis. As a potential noninvasive diagnostic tool, exosome biopsy has shown significant value in the early diagnosis and prognostic assessment of GC (Table 2). New and more sensitive screening strategies have also been proposed (Lopez et al., 2018).
In theory, the contents of exosomes have the potential to serve as detection markers. Research has focused extensively on miRNA as a predictive marker (Chen et al., 2020). However, studies have also analyzed the proteomics of exosomes to determine their tumor metastatic potential (Chen et al., 2023c). Several miRNAs, including miRNA-134 (Jin et al., 2022), miRNA-23b (Zhang R. et al., 2024), and serum miRNA-92a-3p, are suitable detection markers (Lu et al., 2021). Additionally, miRNAs within exosomes hold promise as biomarkers for assessing postoperative metastatic risk in GC patients. For instance, in a study analyzing serum samples from 89 stage II/III GC patients, researchers found that elevated levels of exosomal miRNA-379-5p and miRNA-410-3p were associated with shorter progression-free survival (Liu and Chu, 2020). Circulating exosomal miRNA-19b-3p and miRNA-106p-5p in GC patients may be related to cancer staging and lymphatic metastasis (Zhang et al., 2015). The previously mentioned miR-769-5p might also serve as a potential biomarker for assessing GC drug resistance (Jing et al., 2022).
Proteins in exosomes have also been found to potentially serve as predictive markers, although relatively fewer studies have evaluated these proteins than miRNAs. Researchers isolated exosomes from gastric serosal venous samples of 61 GC patients and found that the expression level of TGF-β1 in exosomes is associated with lymphatic metastasis in GC (Yen et al., 2017). In another study, researchers collected peripheral blood serum from late-stage GC patients with metastasis and extracted and purified exosomes for analysis and discovered that ILK1 and CD14 can influence the colony-forming ability of GC cells and are associated with organ-specific metastasis (Zhou et al., 2023). Proteins in exosomes are primarily membranes and glycoproteins. Given the characteristics of exosome formation, exosomal membrane proteins are related to the source of target cells. Analysis of the total protein profile of serum exosomes from healthy controls and GC patients revealed that TRIM3 could be a potential biomarker for GC diagnosis (Fu et al., 2018). PD-L1 in GC exosomes can reflect the immune status of GC patients (Shen D. D. et al., 2022). Based on these findings, future studies could investigate whether different membrane protein markers or miRNAs are associated with specific organ metastasis or the metastatic growth characteristics of cancer cells (Zhou et al., 2023).
LncRNAs also have the potential to serve as potential liquid biopsy markers GC growth and metastasis. For example, as mentioned earlier, HOTAIR can be detected in both serum and tumor tissue (Zhou et al., 2023). lnc-GNAQ-6:1 is considered a potential marker for exosomal liquid biopsy in GC patients (Li S. et al., 2020), and the serum exosomal lnc-SLC2A12-10:1 can also be used for diagnosis and prognosis monitoring (Zheng P. et al., 2020). Furthermore, there was a strong correlation found between the invasion depth and TNM stage and the elevated expression of serum exosomal HOTTIP in GC (Zhao et al., 2018). Comparing exosomal HOTTIP to traditional biomarkers CEA, CA19–9, and CA72-4, the former showed superior diagnostic accuracy. Also, a negative overall survival rate was highly linked with elevated exosomal HOTTIP levels, establishing exosomal HOTTIP as a separate prognostic factor in GC patients. Research on circRNAs in GC -related tumors started relatively late, but researchers have found that they also have the potential to be detection markers. For instance, the previously mentioned circRNA Has_circ_0000437 can be detected in patient serum (Shen X. et al., 2022). Circ-RanGAP1 is upregulated in the serum exosomes of GC patients (Lu et al., 2020) and promotes tumor progression by regulating VEGFA. CircFCHO2 can also be detected in the serum of GC patients (Zhang et al., 2022). Like the aforementioned circRNAs, those verified in studies include hsa_circ_000200 (Huang et al., 2023), hsa_circ_0000419 (Tao et al., 2020), and hsa_circ_0015286 (Zheng et al., 2022). Some circRNAs can also serve as indicators for assessing the prognosis and metastatic risk of GC patients. For example, the circRNA CDR1as may be a prognostic marker for GC (Li R. et al., 2023), and researchers have found that the circRNA KIAA1244 can predict the risk of GC lymph node metastasis (Tang et al., 2018).
4.2 As prognostic indicators
Tumor tissue pathology is currently the gold standard for diagnosing GC and enables the analysis of patient tumor stage and the selection of appropriate treatment strategies based on pathological results. Liquid biopsy is not intended to replace pathological examination but serves more as a supplementary choice for investigation. For instance, certain small-molecule markers have been found to have the potential to predict the preferred metastatic site and prognosis of tumors. Therefore, exosomal liquid biopsy as a complementary auxiliary examination is meaningful. Notably, the heterogeneity of exosomes derived from blood may affect the accuracy of detection (Lopez et al., 2018). Changes in a patient’s general condition can influence exosome secretion and content alterations. Therefore, a meticulous experimental design is required to address these considerations.
Serum exosomal liquid biopsy is not necessarily the sole approach for exosomal analysis. Peritoneal lavage fluid or ascites can also serve as alternative sources for sample collection (Ohzawa et al., 2020a; Tokuhisa et al., 2015; Hu et al., 2019). The miRNA-29s in peritoneal fluid are predictive factors for tumor recurrence (Ohzawa et al., 2020b). Exosomal biomarkers such as has_let_7g_3p and has_miR_10395-3p in peritoneal lavage fluid can predict the occurrence of peritoneal metastasis and systemic chemotherapy efficacy (Luo et al., 2023). Exosomal miRNAs from neutrophils act as accurate biomarkers for GC diagnosis (Yu et al., 2024). Moreover, researchers have isolated exosomes from gastric juice, expanding the possibilities for sample sources (Kagota et al., 2019). Currently, in liquid biopsy studies related to tumor exosomes, sample sources include saliva, serum, urine, etc. However, whether exosomes from different sources have sufficient representativeness remains a question for further investigation when considering practical clinical applications. It is crucial to determine which sample type provides better representativeness and stability in detection through additional research.
4.3 As therapeutic target
Exosomes, serving as innovative vehicles for cancer vaccines, present a more promising approach than conventional vaccines because of their reduced immunogenicity, tumor heterogeneity accommodation, and superior delivery efficiency. These vesicles exhibit inherent low immunogenicity, precise targeting abilities, biological durability, and intercellular communication attributes, rendering them well-suited for cancer vaccine advancement. They have the capacity to transport bioactive substances like DNA, ncRNAs, and proteins that modulate the immune characteristics of recipient cells. Furthermore, exosomes can convey tumor neoantigens and immune stimulants, triggering persistent and wide-ranging anti-tumor immune reactions (Sheikhhossein et al., 2024).
In cancer immunotherapy, tumor-derived exosomes (TEX) as potent cell-free peptide-based antitumour vaccine through dendritic cell-released MHC class I/peptide complexes for efficient CD8+ T cell priming to suppress tumour growth or prime naive Tc1 lymphocytes leading to tumor rejection (Andre et al., 2004; Chaput et al., 2004). With additional preclinical and clinical validation, the TEX vaccine may emerge as a potential tool in the fight against cancer. Notably, Zitvogel et al. pioneered the investigation of tumor suppression through exosome-based vaccines in 1998 (Zitvogel et al., 1998). The major research applications of TEX Vaccinnes in solid tumors including lung cancer (Yaddanapudi et al., 2012) and breast caner (Xie et al., 2018). However, the application of these treatments in GC is slow to progress.
4.4 Drug delivery system
Recently, exosomes have garnered significant interest in serving as natural nanocarriers for delivering anti-GC drugs due to their high biocompatibility, low immunogenicity, ability to target tumor cells, enhance drug efficacy, and mitigate adverse reactions. Researchers have demonstrated the important role of SALL4 in enhancing tumor angiogenesis through the modulation of VEGF expression. It also suggested an exosome-based nano-drug delivery system loaded for the concurrent administration of siRNA directed against the SALL4/VEGF pathway and the anticancer medication thalidomide. The study validated the prospective utility of the exosomal drug delivery system in anti-angiogenic and therapy of GC by suppressing SALL4/VEGF pathway (Abouelnazar et al., 2023). Research on a novel delivery strategy was found to transfect synthetic miR-200a mimics into exosomes via electroporation. In a mechanistic way, miR-200a increased E-cadherin water and decreased the expression levels of β-catenin, vimentin, ZEB1 and Snail1, leading to EMT inhibition in GC cells (Mirzaei et al., 2023). In addition, researchers proposed that 17-DMAG-loaded MKN45-targeting exosomes effectively deliver 17-DMAG to GC cells, resulting in enhanced antitumor effects. This research aims to provide insights into the design of targeted exosome-based therapies for GC in terms of potential clinical applications (Park et al., 2024).
4.5 Chemoresistance
Recent studies have identified exosomes as mediators of tumor chemotherapy resistance through various mechanisms. These mechanisms include direct drug efflux, transportation via drug efflux pumps, and intercellular exchange of miRNA. Moreover, exosomes contribute to tumor chemotherapy resistance by facilitating the activity of efflux pump transporters such as P-glycoprotein(P-gp), multidrug resistance protein-1 (MRP-1), ATP-binding cassette transporter A3 (ABCA-3), and ATP-binding cassette transporter G2 (ABCG-2) (Shedden et al., 2003; Levchenko et al., 2005; Ambudkar et al., 2005). Exosomes loaded with miRNAs, mRNAs, and other ncRNAs can regulate drug resistance. Researchers explored the potential therapeutic approach of exosome delivery of anti-miR-214 to reverse cisplatin (DDP) resistance in GC. They confirmed that anti-miR-214 could enhance the sensitivity of GC cells to DDP by cell experiments. Then they constructed and verified that exosome-loaded anti-miR-214 (Exo-anti-214) could effectively enhance the sensitivity of GC cells to DDP by down-regulating the expression of tumor miR-214 and up-regulating the related target proteins. This study provides a new therapeutic strategy based on a combination of systemic injection of exosomes containing anti-miR-214 and intraperitoneal DDP administration was proposed for overcoming DDP-resistant GC (Wang et al., 2018c). In GC resistant cell line MGC-803/5-FU, the expression level of TFAP2E was decreased due to hypermethylation, which directly promoted the chemotherapy resistance to 5-FU. Treatment with the demethylating drug 5-aza-2 ′-deoxycytidine (5AZA) can reverse this effect. miR-106a-5p and miR-421 are significantly overexpressed in exosomes secreted by drug-resistant cells, which are involved in mediating the methylation-related resistance mechanism of TFAP2E by targeting regulatory genes such as E2F1, MTOR, and STAT3. After co-culture with sensitive cells, exosomes may enhance drug resistance by delivering these miRNAs (Jingyue et al., 2019). Similarly, miR-500a-3p was significantly highly expressed in DDP-resistant GC cells and their secreted exosomes. Drug-resistant cells deliver miR-500a-3p to sensitive cells via exosomes, inhibiting the expression of tumor suppressor FBXW7 and enhancing the DDP resistance and stemness of recipient cells. These study revealed the key role of exosomes and their miRNAs in chemotherapy resistance (Lin et al., 2020). A recent study revealed a new mechanism by which M2-polarized macrophages deliver miR-3681-3p via exosomes to promote DDP resistance in GC cells. Experiments have shown that M2 macrophage-derived exosomes target miR-3681-3p to inhibit the expression of MLH1, a key of DNA repair protein, and significantly enhance DDP resistance in GC cells, while MLH1 overexpression can reverse drug resistance (Wei et al., 2025). Similarly, research demonstrated that the exosome secreted by M2 macrophages delivered circTEX2 to GC cells and relieved its inhibition of drug effector protein ABCC1 by adsorption of miR-145, thus enhancing the resistance of tumor cells to DDP. These study suggested that targeting exosome-dependent signaling pathways between M2 macrophage and cancer cells may be a potential therapeutic strategy to overcome DDP resistance in GC (Qu et al., 2024).
5 Discussion
Cancer is a complex systemic disease involving the participation of the immune system, vascular system, nervous system, and other factors in its onset and progression. As the primary mode of intercellular communication, exosomes possess the capacity to influence the fate of both their originating cells and recipient cells. Their impact on tumors manifests in various ways, including stimulation of tumor growth and resistance to drugs, initiation of myofibroblast differentiation, facilitation of angiogenesis, promotion of PMN formation, and induction of immunosuppression. Extensive research has demonstrated the involvement of exosomes in critical biological processes such as angiogenesis, proliferation, invasion, migration, and recurrence in GC. Moreover, exosomes play a pivotal role in modulating the drug resistance of GC cells, thereby advancing the disease. The presence of diverse bioactive molecules within tumor cell-derived exosomes positions them as promising candidates for early GC detection (Figure 5). Furthermore, the potential of exosomes to serve as targeted drug delivery vehicles underscores their significant future applications. In previous overall landscape of research on exosome regulation of tumor progression and growth, there is a predominant focus on miRNA studies. This is evident not only in the biological functions of miRNAs but also in the noninvasive early diagnosis of tumors through liquid biopsy using characteristic miRNA profiles. With technological advancements, there is a growing recognition of the ability of exosomes to transport various molecules for intercellular communication with a range of cell types, including mesenchymal stem cells and different immune cells. Simultaneously, some scholars have raised questions about the physiological functions of exosomes. For instance, concerns have been raised about whether the quantity of exosomes is sufficient to generate biological functions. Consequently, manipulating the exosome biogenesis process to enhance the production of exosomes/EVs with specific functions in targeted cell types holds significant promise for clinical utility. Additionally, there are questions about whether exosomes, when used as exogenous drugs, can evade recognition by immune cells. Future researchers should delve deeper, considering a systemic organ perspective and comprehensively assessing the role of tumor exosomes. Furthermore, due to the specific location of primary GC occurrence, certain microbiota in the gastrointestinal tract may also produce exosomes, influencing the onset and progression of GC. For instance, H. pylori has been found to inhibit the immune response gamma-glutamyltransferase (GGT) through exosome secretion (Franzini et al., 2014). These findings suggest that when exosome-related studies are conducted in GC patients with concurrent bacterial infections, the situation may become more complex, and the mixture of various factors could lead to inaccurate results.
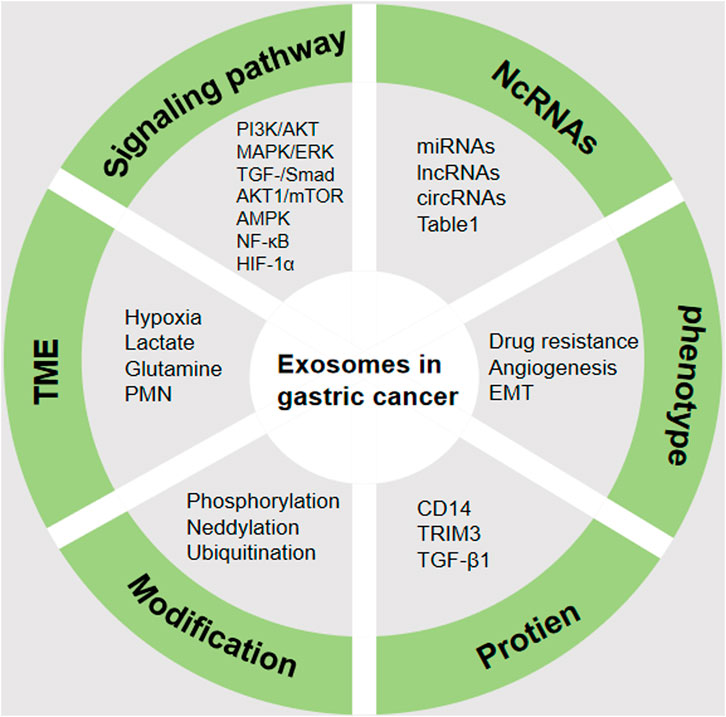
Figure 5. Regulatory mechanism of exosomes in GC. The complex mechanism in GC includes the aberrant expression of NcRNAs, protein, phenotype, signaling pathway, TME and modification. TME (tumor microenvironment), NcRNAs (Non-coding RNAs), EMT (Epithelial-mesenchymal transition), PMN (Pre-metastatic niche), HIF-1α (hypoxia inducible factor-1), AMPK (Adenosine 5′-monophosphate -activated protein kinase), NF-κB (nuclear factor-kappa B). The figure was created by power point.
6 Conclusion
Exosomes play a pivotal role in GC progression, acting as key regulators in tumor growth, metastasis, and immune evasion. They carry a variety of bioactive molecules, including miRNAs, lncRNAs, circRNAs, and proteins, which can serve as potential biomarkers for early diagnosis and prognosis in GC. The interaction between GC-derived exosomes and the TME is complex, with exosomes from various cellular components influencing tumor behavior. Engineered exosomes offer a promising therapeutic strategy by delivering anticancer drugs directly to tumor sites. Further research is needed to fully understand the multifaceted roles of exosomes in GC and to develop effective exosome-based diagnostic and therapeutic approaches.
7 Future directions
In the context of exosome therapeutic strategies, engineering exosomes may be a promising approach (Zhang M. et al., 2023). Engineering modifications of exosomes can enable them to carry specific drugs, enhance their targeting to cancer cells, and evade immune surveillance. These advantages make engineered exosomes therapy potentially more advantageous than traditional methods. Scientists have employed various contemporary techniques such as transmission electron microscopy, dynamic light scattering, nanoparticle tracking analysis, protein quantification, Western blotting, and flow cytometry to characterize exosomes. However, due to the alterations in exosomes morphology, purity, and biological function during isolation and processing, their characterization remains a challenging and pivotal issue in current research. Consequently, there is a huge amount of space for further exploration and advancement in understanding the composition and attributes of exosomes. Enhanced methodologies are essential for the comprehensive characterization and analysis of exosomes. Exosomes serve as a double-edged sword for tumor cells. Exosomes from tumor cells typically promote cancer, while those derived from immune cells often have anticancer functions. Distinguishing and defining exosomes secreted by different subtypes of cells are critical aspects of future research. Currently, exosomes extraction and identification are primarily performed in cells, limiting research within the organism. Encouragingly, researchers are exploring techniques to identify exosomes in tissues (Crescitelli et al., 2021). It is anticipated that future studies can isolate exosomes from tissues, bringing related research closer to clinical applications. Despite the broad potential of exosomes as drug delivery vehicles, challenges remain regarding their yield, stability, targeted delivery efficiency, and immunogenicity. Exosomes inherently transport biologically active molecules from their parent cells. However, using them directly for therapeutic purposes may induce off-target effects or increase the risk of metastasis. Therefore, experts advocate for the implementation of rigorous standards for exosome modification and quality control to improve targeting through surface modifications (e.g., targeted peptide coupling) or content editing (e.g., CRISPR-mediated regulation of specific RNA) and to mitigate off-target risks. With the emergence of AI/ML, it is believed that future exosomes can better serve the clinic.
Author contributions
XwL: Writing - original draft, Writing - review and editing. XaL: Data curation, Writing - original draft. ML: Methodology, Writing - review and editing. JC: Conceptualization, Methodology, Writing - original draft, Writing - review and editing. XrL: Conceptualization, Methodology, Writing - original draft, Writing - review and editing.
Funding
The author(s) declare that financial support was received for the research and/or publication of this article. This paper was supported by Kunshan and Technology Bureau (China), Grant Reference Number (KS2329); The Natural Science Foundation of Nanjing University of Traditional Chinese Medicine (XZR2021075) and The project of Suzhou Medical Health Science and Technology Innovation (SKYD2022053); The TCM science and technology development plan of Jiangsu Province (YB2020062) and Clinical Key Disease Diagnosis and Treatment Technology Special Project of Suzhou (LCZX202341).
Conflict of interest
The authors declare that the research was conducted in the absence of any commercial or financial relationships that could be construed as a potential conflict of interest.
Generative AI statement
The author(s) declare that no Gen AI was used in the creation of this manuscript.
Publisher’s note
All claims expressed in this article are solely those of the authors and do not necessarily represent those of their affiliated organizations, or those of the publisher, the editors and the reviewers. Any product that may be evaluated in this article, or claim that may be made by its manufacturer, is not guaranteed or endorsed by the publisher.
References
Abouelnazar, F. A., Zhang, X., Zhang, J., Wang, M., Yu, D., Zang, X., et al. (2023). SALL4 promotes angiogenesis in gastric cancer by regulating VEGF expression and targeting SALL4/VEGF pathway inhibits cancer progression. Cancer Cell Int. 23 (1), 149. doi:10.1186/s12935-023-02985-9
Alessandrini, L., Manchi, M., De Re, V., Dolcetti, R., and Canzonieri, V. (2018). Proposed molecular and miRNA classification of gastric cancer. Int. J. Mol. Sci. 19 (6), 1683. doi:10.3390/ijms19061683
Ambudkar, S. V., Sauna, Z. E., Gottesman, M. M., and Szakacs, G. (2005). A novel way to spread drug resistance in tumor cells: functional intercellular transfer of P-glycoprotein (ABCB1). Trends Pharmacol. Sci. 26 (8), 385–387. doi:10.1016/j.tips.2005.06.001
Andre, F., Chaput, N., Schartz, N. E. C., Flament, C., Aubert, N., Bernard, J., et al. (2004). Exosomes as potent cell-free peptide-based vaccine. I. Dendritic cell-derived exosomes transfer functional MHC class I/peptide complexes to dendritic cells. J. Immunol. 172 (4), 2126–2136. doi:10.4049/jimmunol.172.4.2126
Ariston Gabriel, A. N., Wang, F., Jiao, Q., Yvette, U., Yang, X., Al-Ameri, S. A., et al. (2020). The involvement of exosomes in the diagnosis and treatment of pancreatic cancer. Mol. Cancer 19 (1), 132. doi:10.1186/s12943-020-01245-y
Arita, T., Ichikawa, D., Konishi, H., Komatsu, S., Shiozaki, A., Ogino, S., et al. (2016). Tumor exosome-mediated promotion of adhesion to mesothelial cells in gastric cancer cells. Oncotarget 7 (35), 56855–56863. doi:10.18632/oncotarget.10869
Azmi, A. S., Bao, B., and Sarkar, F. H. (2013). Exosomes in cancer development, metastasis, and drug resistance: a comprehensive review. Cancer Metastasis Rev. 32 (3-4), 623–642. doi:10.1007/s10555-013-9441-9
Balkwill, F. R., Capasso, M., and Hagemann, T. (2012). The tumor microenvironment at a glance. J. Cell Sci. 125 (Pt 23), 5591–5596. doi:10.1242/jcs.116392
Booth, A. M., Fang, Y., Fallon, J. K., Yang, J. M., Hildreth, J. E. K., and Gould, S. J. (2006). Exosomes and HIV Gag bud from endosome-like domains of the T cell plasma membrane. J. Cell Biol. 172 (6), 923–935. doi:10.1083/jcb.200508014
Chang, W., and Wang, J. (2019). Exosomes and their noncoding RNA cargo are emerging as new modulators for diabetes mellitus. Cells 8 (8), 853. doi:10.3390/cells8080853
Chaput, N., Schartz, N. E. C., André, F., Taïeb, J., Novault, S., Bonnaventure, P., et al. (2004). Exosomes as potent cell-free peptide-based vaccine. II. Exosomes in CpG adjuvants efficiently prime naive Tc1 lymphocytes leading to tumor rejection. J. Immunol. 172 (4), 2137–2146. doi:10.4049/jimmunol.172.4.2137
Chaput, N., Taïeb, J., André, F., and Zitvogel, L. (2005). The potential of exosomes in immunotherapy. Expert Opin. Biol. Ther. 5 (6), 737–747. doi:10.1517/14712598.5.6.737
Chen, L. L. (2020). The expanding regulatory mechanisms and cellular functions of circular RNAs. Nat. Rev. Mol. Cell Biol. 21 (8), 475–490. doi:10.1038/s41580-020-0243-y
Chen, P., Liu, Z., Xiao, H., Yang, X., Li, T., Huang, W., et al. (2023a). Effect of tumor exosome-derived Lnc RNA HOTAIR on the growth and metastasis of gastric cancer. Clin. Transl. Oncol. 25 (12), 3447–3459. doi:10.1007/s12094-023-03208-3
Chen, X., Zhang, S., Du, K., Zheng, N., Liu, Y., Chen, H., et al. (2021). Gastric cancer-secreted exosomal X26nt increases angiogenesis and vascular permeability by targeting VE-cadherin. Cancer Sci. 112 (5), 1839–1852. doi:10.1111/cas.14740
Chen, Y., Cai, G., Jiang, J., Chen, Y., Ding, Y., Lu, J., et al. (2023c). Proteomic profiling of gastric cancer with peritoneal metastasis identifies a protein signature associated with immune microenvironment and patient outcome. Gastric Cancer 26 (4), 504–516. doi:10.1007/s10120-023-01379-0
Chen, Y., Feng, H., Wu, Y., Wang, R., Li, Z., Chen, J., et al. (2020). Evaluation of plasma exosomal microRNAs as circulating biomarkers for progression and metastasis of gastric cancer. Clin. Transl. Med. 10 (6), e171. doi:10.1002/ctm2.171
Chen, Y., Li, Y., Wu, Y., Chen, S., Jin, X., Chen, X., et al. (2023b). Yiwei decoction promotes apoptosis of gastric cancer cells through spleen-derived exosomes. Front. Pharmacol. 14, 1144955. doi:10.3389/fphar.2023.1144955
Chhabra, Y., and Weeraratna, A. T. (2023). Fibroblasts in cancer: unity in heterogeneity. Cell 186 (8), 1580–1609. doi:10.1016/j.cell.2023.03.016
Chia, N. Y., and Tan, P. (2016). Molecular classification of gastric cancer. Ann. Oncol. 27 (5), 763–769. doi:10.1093/annonc/mdw040
Colombo, M., Raposo, G., and Thery, C. (2014). Biogenesis, secretion, and intercellular interactions of exosomes and other extracellular vesicles. Annu. Rev. Cell Dev. Biol. 30, 255–289. doi:10.1146/annurev-cellbio-101512-122326
Couch, Y., Buzàs, E. I., Di Vizio, D., Gho, Y. S., Harrison, P., Hill, A. F., et al. (2021). A brief history of nearly EV-erything - the rise and rise of extracellular vesicles. J. Extracell. Vesicles 10 (14), e12144. doi:10.1002/jev2.12144
Crescitelli, R., Lasser, C., and Lotvall, J. (2021). Isolation and characterization of extracellular vesicle subpopulations from tissues. Nat. Protoc. 16 (3), 1548–1580. doi:10.1038/s41596-020-00466-1
Crivelli, S. M., Giovagnoni, C., Zhu, Z., Tripathi, P., Elsherbini, A., Quadri, Z., et al. (2022). Function of ceramide transfer protein for biogenesis and sphingolipid composition of extracellular vesicles. J. Extracell. Vesicles 11 (6), e12233. doi:10.1002/jev2.12233
Dai, J., Su, Y., Zhong, S., Cong, L., Liu, B., Yang, J., et al. (2020). Exosomes: key players in cancer and potential therapeutic strategy. Signal Transduct. Target Ther. 5 (1), 145. doi:10.1038/s41392-020-00261-0
Debbi, L., Guo, S., Safina, D., and Levenberg, S. (2022). Boosting extracellular vesicle secretion. Biotechnol. Adv. 59, 107983. doi:10.1016/j.biotechadv.2022.107983
de Magalhaes, J. P. (2013). How ageing processes influence cancer. Nat. Rev. Cancer 13 (5), 357–365. doi:10.1038/nrc3497
Demaria, O., Cornen, S., Daëron, M., Morel, Y., Medzhitov, R., and Vivier, E. (2019). Harnessing innate immunity in cancer therapy. Nature 574 (7776), 45–56. doi:10.1038/s41586-019-1593-5
Deng, C., Huo, M., Chu, H., Zhuang, X., Deng, G., Li, W., et al. (2024). Exosome circATP8A1 induces macrophage M2 polarization by regulating the miR-1-3p/STAT6 axis to promote gastric cancer progression. Mol. Cancer 23 (1), 49. doi:10.1186/s12943-024-01966-4
Deng, G., Qu, J., Zhang, Y., Che, X., Cheng, Y., Fan, Y., et al. (2017). Gastric cancer-derived exosomes promote peritoneal metastasis by destroying the mesothelial barrier. FEBS Lett. 591 (14), 2167–2179. doi:10.1002/1873-3468.12722
Deng, S., Clowers, M. J., Velasco, W. V., Ramos-Castaneda, M., and Moghaddam, S. J. (2019). Understanding the complexity of the tumor microenvironment in K-ras mutant lung cancer: finding an alternative path to prevention and treatment. Front. Oncol. 9, 1556. doi:10.3389/fonc.2019.01556
Eguchi, T., Sheta, M., Fujii, M., and Calderwood, S. K. (2022). Cancer extracellular vesicles, tumoroid models, and tumor microenvironment. Semin. Cancer Biol. 86 (Pt 1), 112–126. doi:10.1016/j.semcancer.2022.01.003
Fan, Y., Che, X., Qu, J., Hou, K., Wen, T., Li, Z., et al. (2019). Exosomal PD-L1 retains immunosuppressive activity and is associated with gastric cancer prognosis. Ann. Surg. Oncol. 26 (11), 3745–3755. doi:10.1245/s10434-019-07431-7
Fan, Y., Li, Y., Yao, X., Jin, J., Scott, A., Liu, B., et al. (2023). Epithelial SOX9 drives progression and metastases of gastric adenocarcinoma by promoting immunosuppressive tumour microenvironment. Gut 72 (4), 624–637. doi:10.1136/gutjnl-2021-326581
Feng, C., She, J., Chen, X., Zhang, Q., Zhang, X., Wang, Y., et al. (2019). Exosomal miR-196a-1 promotes gastric cancer cell invasion and metastasis by targeting SFRP1. Nanomedicine (Lond) 14 (19), 2579–2593. doi:10.2217/nnm-2019-0053
Franzini, M., Corti, A., Fierabracci, V., and Pompella, A. (2014). Helicobacter, gamma-glutamyltransferase and cancer: further intriguing connections. World J. Gastroenterol. 20 (47), 18057–18058. doi:10.3748/wjg.v20.i47.18057
Fu, H., Yang, H., Zhang, X., Wang, B., Mao, J., Li, X., et al. (2018). Exosomal TRIM3 is a novel marker and therapy target for gastric cancer. J. Exp. Clin. Cancer Res. 37 (1), 162. doi:10.1186/s13046-018-0825-0
Gao, X., Ran, N., Dong, X., Zuo, B., Yang, R., Zhou, Q., et al. (2018). Anchor peptide captures, targets, and loads exosomes of diverse origins for diagnostics and therapy. Sci. Transl. Med. 10 (444), eaat0195. doi:10.1126/scitranslmed.aat0195
Garcia-Martin, R., Wang, G., Brandão, B. B., Zanotto, T. M., Shah, S., Kumar Patel, S., et al. (2022). MicroRNA sequence codes for small extracellular vesicle release and cellular retention. Nature 601 (7893), 446–451. doi:10.1038/s41586-021-04234-3
Gobbo, J., Marcion, G., Cordonnier, M., Dias, A. M. M., Pernet, N., Hammann, A., et al. (2016). Restoring anticancer immune response by targeting tumor-derived exosomes with a HSP70 peptide aptamer. J. Natl. Cancer Inst. 108 (3), djv330. doi:10.1093/jnci/djv330
Hansen, T. B., Jensen, T. I., Clausen, B. H., Bramsen, J. B., Finsen, B., Damgaard, C. K., et al. (2013). Natural RNA circles function as efficient microRNA sponges. Nature 495 (7441), 384–388. doi:10.1038/nature11993
Hu, Y., Qi, C., Liu, X., Zhang, C., Gao, J., Wu, Y., et al. (2019). Malignant ascites-derived exosomes promote peritoneal tumor cell dissemination and reveal a distinct miRNA signature in advanced gastric cancer. Cancer Lett. 457, 142–150. doi:10.1016/j.canlet.2019.04.034
Hu, Y., Zang, W., Feng, Y., Mao, Q., Chen, J., Zhu, Y., et al. (2024). mir-605-3p prevents liver premetastatic niche formation by inhibiting angiogenesis via decreasing exosomal nos3 release in gastric cancer. Cancer Cell Int. 24 (1), 184. doi:10.1186/s12935-024-03359-5
Huang, X. J., Wang, Y., Wang, H. T., Liang, Z. F., Ji, C., Li, X. X., et al. (2023). Exosomal hsa_circ_000200 as a potential biomarker and metastasis enhancer of gastric cancer via miR-4659a/b-3p/HBEGF axis. Cancer Cell Int. 23 (1), 151. doi:10.1186/s12935-023-02976-w
Hyung, S., Ko, J., Heo, Y. J., Blum, S. M., Kim, S. T., Park, S. H., et al. (2023). Patient-derived exosomes facilitate therapeutic targeting of oncogenic MET in advanced gastric cancer. Sci. Adv. 9 (47), eadk1098. doi:10.1126/sciadv.adk1098
Isaac, R., Reis, F. C. G., Ying, W., and Olefsky, J. M. (2021). Exosomes as mediators of intercellular crosstalk in metabolism. Cell Metab. 33 (9), 1744–1762. doi:10.1016/j.cmet.2021.08.006
Jafari, D., Malih, S., Eini, M., Jafari, R., Gholipourmalekabadi, M., Sadeghizadeh, M., et al. (2020). Improvement, scaling-up, and downstream analysis of exosome production. Crit. Rev. Biotechnol. 40 (8), 1098–1112. doi:10.1080/07388551.2020.1805406
Ji, R., Lin, J., Gu, H., Ma, J., Fu, M., and Zhang, X. (2023). Gastric cancer derived mesenchymal stem cells promote the migration of gastric cancer cells through miR-374a-5p. Curr. Stem Cell Res. Ther. 18 (6), 853–863. doi:10.2174/1574888X18666221124145847
Jiang, X., Wang, J., Deng, X., Xiong, F., Zhang, S., Gong, Z., et al. (2020). The role of microenvironment in tumor angiogenesis. J. Exp. Clin. Cancer Res. 39 (1), 204. doi:10.1186/s13046-020-01709-5
Jin, M. Z., and Jin, W. L. (2020). The updated landscape of tumor microenvironment and drug repurposing. Signal Transduct. Target Ther. 5 (1), 166. doi:10.1038/s41392-020-00280-x
Jin, Y., Cai, Q., Wang, L., Ji, J., Sun, Y., Jiang, J., et al. (2023). Paracrine activin B-NF-κB signaling shapes an inflammatory tumor microenvironment in gastric cancer via fibroblast reprogramming. J. Exp. Clin. Cancer Res. 42 (1), 269. doi:10.1186/s13046-023-02861-4
Jin, Z., Song, Y., Lian, C., and Gao, L. (2022). Decreased serum exosomal microRNA-134 expression and its prognostic value in gastric cancer. Ann. Clin. Lab. Sci. 52 (4), 563–570.
Jing, X., Xie, M., Ding, K., Xu, T., Fang, Y., Ma, P., et al. (2022). Exosome-transmitted miR-769-5p confers cisplatin resistance and progression in gastric cancer by targeting CASP9 and promoting the ubiquitination degradation of p53. Clin. Transl. Med. 12 (5), e780. doi:10.1002/ctm2.780
Jingyue, S., Xiao, W., Juanmin, Z., Wei, L., Daoming, L., and Hong, X. (2019). TFAP2E methylation promotes 5-fluorouracil resistance via exosomal miR-106a-5p and miR-421 in gastric cancer MGC-803 cells. Mol. Med. Rep. 20 (1), 323–331. doi:10.3892/mmr.2019.10237
Johnston, F. M., and Beckman, M. (2019). Updates on management of gastric cancer. Curr. Oncol. Rep. 21 (8), 67. doi:10.1007/s11912-019-0820-4
Kagota, S., Taniguchi, K., Lee, S. W., Ito, Y., Kuranaga, Y., Hashiguchi, Y., et al. (2019). Analysis of extracellular vesicles in gastric juice from gastric cancer patients. Int. J. Mol. Sci. 20 (4), 953. doi:10.3390/ijms20040953
Kalluri, R., and LeBleu, V. S. (2020). The biology, function, and biomedical applications of exosomes. Science 367 (6478), eaau6977. doi:10.1126/science.aau6977
Kang, Y. T., Niu, Z., Hadlock, T., Purcell, E., Zeinali, M., Owen, S., et al. (2021). On-chip biogenesis of circulating NK cell-derived exosomes in non-small cell lung cancer exhibits antitumoral activity. Adv. Sci. (Weinh) 8 (6), 2003747. doi:10.1002/advs.202003747
Khan, N. A., Asim, M., Biswas, K. H., Alansari, A. N., Saman, H., Sarwar, M. Z., et al. (2023). Exosome nanovesicles as potential biomarkers and immune checkpoint signaling modulators in lung cancer microenvironment: recent advances and emerging concepts. J. Exp. Clin. Cancer Res. 42 (1), 221. doi:10.1186/s13046-023-02753-7
Kimiz-Gebologlu, I., and Oncel, S. S. (2022). Exosomes: large-scale production, isolation, drug loading efficiency, and biodistribution and uptake. J. Control Release 347, 533–543. doi:10.1016/j.jconrel.2022.05.027
Kok, V. C., and Yu, C. C. (2020). Cancer-derived exosomes: their role in cancer biology and biomarker development. Int. J. Nanomedicine 15, 8019–8036. doi:10.2147/IJN.S272378
Lakshmi, S., Hughes, T. A., and Priya, S. (2021). Exosomes and exosomal RNAs in breast cancer: a status update. Eur. J. Cancer 144, 252–268. doi:10.1016/j.ejca.2020.11.033
Lee, Y. C., Chiang, T. H., Chou, C. K., Tu, Y. K., Liao, W. C., Wu, M. S., et al. (2016). Association between Helicobacter pylori eradication and gastric cancer incidence: a systematic review and meta-analysis. Gastroenterology 150 (5), 1113–1124 e5. doi:10.1053/j.gastro.2016.01.028
Levchenko, A., Mehta, B. M., Niu, X., Kang, G., Villafania, L., Way, D., et al. (2005). Intercellular transfer of P-glycoprotein mediates acquired multidrug resistance in tumor cells. Proc. Natl. Acad. Sci. U. S. A. 102 (6), 1933–1938. doi:10.1073/pnas.0401851102
Li, C., Ni, Y. Q., Xu, H., Xiang, Q. Y., Zhao, Y., Zhan, J. K., et al. (2021b). Roles and mechanisms of exosomal non-coding RNAs in human health and diseases. Signal Transduct. Target Ther. 6 (1), 383. doi:10.1038/s41392-021-00779-x
Li, L., Wang, L., Yang, J. L., Wang, H. J., and Wang, Y. Y. (2023a). Hypoxic tumor-derived exosomal miR-199a-3p promote gastric cancer metastasis via MAP3K4. J. Cancer 14 (11), 2161–2172. doi:10.7150/jca.83909
Li, M. Y., Liu, L. Z., and Dong, M. (2021a). Progress on pivotal role and application of exosome in lung cancer carcinogenesis, diagnosis, therapy and prognosis. Mol. Cancer 20 (1), 22. doi:10.1186/s12943-021-01312-y
Li, Q., Li, B., Wei, S., He, Z., Huang, X., Wang, L., et al. (2018). Exosomal miR-21-5p derived from gastric cancer promotes peritoneal metastasis via mesothelial-to-mesenchymal transition. Cell Death Dis. 9 (9), 854. doi:10.1038/s41419-018-0928-8
Li, R., Tian, X., Jiang, J., Qian, H., Shen, H., and Xu, W. (2023b). CircRNA CDR1as: a novel diagnostic and prognostic biomarker for gastric cancer. Biomarkers 28 (5), 448–457. doi:10.1080/1354750X.2023.2206984
Li, S., Zhang, M., Zhang, H., Hu, K., Cai, C., Wang, J., et al. (2020b). Exosomal long noncoding RNA lnc-GNAQ-6:1 may serve as a diagnostic marker for gastric cancer. Clin. Chim. Acta 501, 252–257. doi:10.1016/j.cca.2019.10.047
Li, X., Li, C., Zhang, L., Wu, M., Cao, K., Jiang, F., et al. (2020a). The significance of exosomes in the development and treatment of hepatocellular carcinoma. Mol. Cancer 19 (1), 1. doi:10.1186/s12943-019-1085-0
Liang, Y., Duan, L., Lu, J., and Xia, J. (2021). Engineering exosomes for targeted drug delivery. Theranostics 11 (7), 3183–3195. doi:10.7150/thno.52570
Lin, H., Zhang, L., Zhang, C., and Liu, P. (2020). Exosomal MiR-500a-3p promotes cisplatin resistance and stemness via negatively regulating FBXW7 in gastric cancer. J. Cell Mol. Med. 24 (16), 8930–8941. doi:10.1111/jcmm.15524
Lin, X. M., Wang, Z. J., Lin, Y. X., and Chen, H. (2021). Decreased exosome-delivered miR-486-5p is responsible for the peritoneal metastasis of gastric cancer cells by promoting EMT progress. World J. Surg. Oncol. 19 (1), 312. doi:10.1186/s12957-021-02381-5
Liu, J., Wu, S., Zheng, X., Zheng, P., Fu, Y., Wu, C., et al. (2020). Immune suppressed tumor microenvironment by exosomes derived from gastric cancer cells via modulating immune functions. Sci. Rep. 10 (1), 14749. doi:10.1038/s41598-020-71573-y
Liu, M. X., and Chu, K. M. (2023). MiR-410-3p suppresses primary gastric cancer and exosomes regulate endogenous expression of miR-410-3p. Am. J. Cancer Res. 13 (6), 2670–2680.
Liu, R., Li, X., Zhu, W., Wang, Y., Zhao, D., Wang, X., et al. (2019a). Cholangiocyte-derived exosomal long noncoding RNA H19 promotes hepatic stellate cell activation and cholestatic liver fibrosis. Hepatology 70 (4), 1317–1335. doi:10.1002/hep.30662
Liu, X., and Chu, K. M. (2020). Exosomal miRNAs as circulating biomarkers for prediction of development of haematogenous metastasis after surgery for stage II/III gastric cancer. J. Cell Mol. Med. 24 (11), 6220–6232. doi:10.1111/jcmm.15253
Liu, X., Lu, Y., Xu, Y., Hou, S., Huang, J., Wang, B., et al. (2019b). Exosomal transfer of miR-501 confers doxorubicin resistance and tumorigenesis via targeting of BLID in gastric cancer. Cancer Lett. 459, 122–134. doi:10.1016/j.canlet.2019.05.035
Liu, Y., Gu, Y., Han, Y., Zhang, Q., Jiang, Z., Zhang, X., et al. (2016). Tumor exosomal RNAs promote lung pre-metastatic niche formation by activating alveolar epithelial TLR3 to recruit neutrophils. Cancer Cell 30 (2), 243–256. doi:10.1016/j.ccell.2016.06.021
Liu, Y., Li, C., Lu, Y., Liu, C., and Yang, W. (2022). Tumor microenvironment-mediated immune tolerance in development and treatment of gastric cancer. Front. Immunol. 13, 1016817. doi:10.3389/fimmu.2022.1016817
Lopez, A., Harada, K., Mizrak Kaya, D., Dong, X., Song, S., and Ajani, J. A. (2018). Liquid biopsies in gastrointestinal malignancies: when is the big day? Expert Rev. Anticancer Ther. 18 (1), 19–38. doi:10.1080/14737140.2018.1403320
Lu, J., Wang, Y. H., Yoon, C., Huang, X. Y., Xu, Y., Xie, J. W., et al. (2020). Circular RNA circ-RanGAP1 regulates VEGFA expression by targeting miR-877-3p to facilitate gastric cancer invasion and metastasis. Cancer Lett. 471, 38–48. doi:10.1016/j.canlet.2019.11.038
Lu, X., Lu, J., Wang, S., Zhang, Y., Ding, Y., Shen, X., et al. (2021). Circulating serum exosomal miR-92a-3p as a novel biomarker for early diagnosis of gastric cancer. Future Oncol. 17 (8), 907–919. doi:10.2217/fon-2020-0792
Luo, J., Jiang, L., Shi, M., Yang, Z. Y., Shi, M., Lu, S., et al. (2023). Exosomal hsa-let-7g-3p and hsa-miR-10395-3p derived from peritoneal lavage predict peritoneal metastasis and the efficacy of neoadjuvant intraperitoneal and systemic chemotherapy in patients with gastric cancer. Gastric Cancer 26 (3), 364–378. doi:10.1007/s10120-023-01368-3
Ma, M., Chen, S., Liu, Z., Xie, H., Deng, H., Shang, S., et al. (2017). miRNA-221 of exosomes originating from bone marrow mesenchymal stem cells promotes oncogenic activity in gastric cancer. Onco Targets Ther. 10, 4161–4171. doi:10.2147/OTT.S143315
Mantovani, A., and Allavena, P. (2015). The interaction of anticancer therapies with tumor-associated macrophages. J. Exp. Med. 212 (4), 435–445. doi:10.1084/jem.20150295
Mao, J., Liang, Z., Zhang, B., Yang, H., Li, X., Fu, H., et al. (2017). UBR2 enriched in p53 deficient mouse bone marrow mesenchymal stem cell-exosome promoted gastric cancer progression via wnt/β-catenin pathway. Stem Cells 35 (11), 2267–2279. doi:10.1002/stem.2702
Mashouri, L., Yousefi, H., Aref, A. R., Ahadi, A. M., Molaei, F., and Alahari, S. K. (2019). Exosomes: composition, biogenesis, and mechanisms in cancer metastasis and drug resistance. Mol. Cancer 18 (1), 75. doi:10.1186/s12943-019-0991-5
Masyuk, A. I., Masyuk, T. V., and Larusso, N. F. (2013). Exosomes in the pathogenesis, diagnostics and therapeutics of liver diseases. J. Hepatol. 59 (3), 621–625. doi:10.1016/j.jhep.2013.03.028
Memczak, S., Jens, M., Elefsinioti, A., Torti, F., Krueger, J., Rybak, A., et al. (2013). Circular RNAs are a large class of animal RNAs with regulatory potency. Nature 495 (7441), 333–338. doi:10.1038/nature11928
Miller, J. F., and Sadelain, M. (2015). The journey from discoveries in fundamental immunology to cancer immunotherapy. Cancer Cell 27 (4), 439–449. doi:10.1016/j.ccell.2015.03.007
Mirzaei, S., Gholami, M. H., Aghdaei, H. A., Hashemi, M., Parivar, K., Karamian, A., et al. (2023). Exosome-mediated miR-200a delivery into TGF-beta-treated AGS cells abolished epithelial-mesenchymal transition with normalization of ZEB1, vimentin and Snail1 expression. Environ. Res. 231 (Pt 1), 116115. doi:10.1016/j.envres.2023.116115
Noy, R., and Pollard, J. W. (2014). Tumor-associated macrophages: from mechanisms to therapy. Immunity 41 (1), 49–61. doi:10.1016/j.immuni.2014.06.010
Ohzawa, H., Kumagai, Y., Yamaguchi, H., Miyato, H., Sakuma, Y., Horie, H., et al. (2020a). Exosomal microRNA in peritoneal fluid as a biomarker of peritoneal metastases from gastric cancer. Ann. Gastroenterol. Surg. 4 (1), 84–93. doi:10.1002/ags3.12296
Ohzawa, H., Saito, A., Kumagai, Y., Kimura, Y., Yamaguchi, H., Hosoya, Y., et al. (2020b). Reduced expression of exosomal miR-29s in peritoneal fluid is a useful predictor of peritoneal recurrence after curative resection of gastric cancer with serosal involvement. Oncol. Rep. 43 (4), 1081–1088. doi:10.3892/or.2020.7505
Oliveira, C., Pinheiro, H., Figueiredo, J., Seruca, R., and Carneiro, F. (2015). Familial gastric cancer: genetic susceptibility, pathology, and implications for management. Lancet Oncol. 16 (2), e60–e70. doi:10.1016/S1470-2045(14)71016-2
Ostrowski, M., Carmo, N. B., Krumeich, S., Fanget, I., Raposo, G., Savina, A., et al. (2010). Rab27a and Rab27b control different steps of the exosome secretion pathway. Nat. Cell Biol. 12 (1), 19–13. sup pp 1-13. doi:10.1038/ncb2000
Paget, S. (1989). The distribution of secondary growths in cancer of the breast. 1889. Cancer Metastasis Rev. 8 (2), 98–101.
Panani, A. D. (2008). Cytogenetic and molecular aspects of gastric cancer: clinical implications. Cancer Lett. 266 (2), 99–115. doi:10.1016/j.canlet.2008.02.053
Park, J. H., Kim, S. J., Kim, O. H., and Kim, D. J. (2024). Enhanced efficacy of gastric cancer treatment through targeted exosome delivery of 17-DMAG anticancer agent. Int. J. Mol. Sci. 25 (16), 8762. doi:10.3390/ijms25168762
Participants in the Paris Workshop, (2003). The Paris endoscopic classification of superficial neoplastic lesions: esophagus, stomach, and colon. Gastrointest. Endosc. 58 (6 Suppl. l), S3–S43. doi:10.1016/s0016-5107(03)02159-x
Piao, H. Y., Guo, S., Wang, Y., and Zhang, J. (2021). Exosome-transmitted lncRNA PCGEM1 promotes invasive and metastasis in gastric cancer by maintaining the stability of SNAI1. Clin. Transl. Oncol. 23 (2), 246–256. doi:10.1007/s12094-020-02412-9
Pitt, J. M., Marabelle, A., Eggermont, A., Soria, J. C., Kroemer, G., and Zitvogel, L. (2016). Targeting the tumor microenvironment: removing obstruction to anticancer immune responses and immunotherapy. Ann. Oncol. 27 (8), 1482–1492. doi:10.1093/annonc/mdw168
Qiu, S., Xie, L., Lu, C., Gu, C., Xia, Y., Lv, J., et al. (2022). Gastric cancer-derived exosomal miR-519a-3p promotes liver metastasis by inducing intrahepatic M2-like macrophage-mediated angiogenesis. J. Exp. Clin. Cancer Res. 41 (1), 296. doi:10.1186/s13046-022-02499-8
Qu, B., Liu, J., Peng, Z., Xiao, Z., Li, S., Wu, J., et al. (2024). Macrophages enhance cisplatin resistance in gastric cancer through the transfer of circTEX2. J. Cell Mol. Med. 28 (5), e18070. doi:10.1111/jcmm.18070
Qu, J. L., Qu, X. J., Zhao, M. F., Teng, Y. E., Zhang, Y., Hou, K. Z., et al. (2009). Gastric cancer exosomes promote tumour cell proliferation through PI3K/Akt and MAPK/ERK activation. Dig. Liver Dis. 41 (12), 875–880. doi:10.1016/j.dld.2009.04.006
Qu, X., Liu, B., Wang, L., Liu, L., Zhao, W., Liu, C., et al. (2023). Loss of cancer-associated fibroblast-derived exosomal DACT3-AS1 promotes malignant transformation and ferroptosis-mediated oxaliplatin resistance in gastric cancer. Drug Resist Updat 68, 100936. doi:10.1016/j.drup.2023.100936
Raposo, G., Nijman, H. W., Stoorvogel, W., Liejendekker, R., Harding, C. V., Melief, C. J., et al. (1996). B lymphocytes secrete antigen-presenting vesicles. J. Exp. Med. 183 (3), 1161–1172. doi:10.1084/jem.183.3.1161
Rezaie, J., Feghhi, M., and Etemadi, T. (2022). A review on exosomes application in clinical trials: perspective, questions, and challenges. Cell Commun. Signal 20 (1), 145. doi:10.1186/s12964-022-00959-4
Rocken, C. (2023). Predictive biomarkers in gastric cancer. J. Cancer Res. Clin. Oncol. 149 (1), 467–481. doi:10.1007/s00432-022-04408-0
Sadeghi, S., Tehrani, F. R., Tahmasebi, S., Shafiee, A., and Hashemi, S. M. (2023). Exosome engineering in cell therapy and drug delivery. Inflammopharmacology 31 (1), 145–169. doi:10.1007/s10787-022-01115-7
Salunkhe, S., Dheeraj, , Basak, M., Chitkara, D., and Mittal, A. (2020). Surface functionalization of exosomes for target-specific delivery and in vivo imaging and tracking: strategies and significance. J. Control Release 326, 599–614. doi:10.1016/j.jconrel.2020.07.042
Sang, H., Zhang, W., Peng, L., Wei, S., Zhu, X., Huang, K., et al. (2022). Exosomal circRELL1 serves as a miR-637 sponge to modulate gastric cancer progression via regulating autophagy activation. Cell Death Dis. 13 (1), 56. doi:10.1038/s41419-021-04364-6
Sarfati, D., Koczwara, B., and Jackson, C. (2016). The impact of comorbidity on cancer and its treatment. CA Cancer J. Clin. 66 (4), 337–350. doi:10.3322/caac.21342
Scavo, M. P., Depalo, N., Rizzi, F., Ingrosso, C., Fanizza, E., Chieti, A., et al. (2019). FZD10 carried by exosomes sustains cancer cell proliferation. Cells 8 (8), 777. doi:10.3390/cells8080777
Shedden, K., Xie, X. T., Chandaroy, P., Chang, Y. T., and Rosania, G. R. (2003). Expulsion of small molecules in vesicles shed by cancer cells: association with gene expression and chemosensitivity profiles. Cancer Res. 63 (15), 4331–4337.
Sheikhhossein, H. H., Iommelli, F., Di Pietro, N., Curia, M. C., Piattelli, A., Palumbo, R., et al. (2024). Exosome-like systems: from therapies to vaccination for cancer treatment and prevention-exploring the state of the art. Vaccines (Basel) 12 (5), 519. doi:10.3390/vaccines12050519
Shen, D. D., Pang, J. R., Bi, Y. P., Zhao, L. F., Li, Y. R., Zhao, L. J., et al. (2022b). LSD1 deletion decreases exosomal PD-L1 and restores T-cell response in gastric cancer. Mol. Cancer 21 (1), 75. doi:10.1186/s12943-022-01557-1
Shen, X., Kong, S., Ma, S., Shen, L., Zheng, M., Qin, S., et al. (2022a). Hsa_circ_0000437 promotes pathogenesis of gastric cancer and lymph node metastasis. Oncogene 41 (42), 4724–4735. doi:10.1038/s41388-022-02449-w
Shen, Y., Lin, J., Jiang, T., Shen, X., Li, Y., Fu, Y., et al. (2025). GC-derived exosomal circMAN1A2 promotes cancer progression and suppresses T-cell antitumour immunity by inhibiting FBXW11-mediated SFPQ degradation. J. Exp. Clin. Cancer Res. 44 (1), 24. doi:10.1186/s13046-025-03288-9
Smyth, E. C., Nilsson, M., Grabsch, H. I., van Grieken, N. C., and Lordick, F. (2020). Gastric cancer. Lancet 396 (10251), 635–648. doi:10.1016/S0140-6736(20)31288-5
Sugano, K., Moss, S. F., and Kuipers, E. J. (2023). Gastric intestinal metaplasia: real culprit or innocent bystander as a precancerous condition for gastric cancer? Gastroenterology 165 (6), 1352–1366 e1. doi:10.1053/j.gastro.2023.08.028
Sun, Z., Shi, K., Yang, S., Liu, J., Zhou, Q., Wang, G., et al. (2018a). Effect of exosomal miRNA on cancer biology and clinical applications. Mol. Cancer 17 (1), 147. doi:10.1186/s12943-018-0897-7
Sun, Z., Yang, S., Zhou, Q., Wang, G., Song, J., Li, Z., et al. (2018b). Emerging role of exosome-derived long non-coding RNAs in tumor microenvironment. Mol. Cancer 17 (1), 82. doi:10.1186/s12943-018-0831-z
Tang, W., Fu, K., Sun, H., Rong, D., Wang, H., and Cao, H. (2018). CircRNA microarray profiling identifies a novel circulating biomarker for detection of gastric cancer. Mol. Cancer 17 (1), 137. doi:10.1186/s12943-018-0888-8
Tang, X. H., Guo, T., Gao, X. Y., Wu, X. L., Xing, X. F., Ji, J. F., et al. (2021). Exosome-derived noncoding RNAs in gastric cancer: functions and clinical applications. Mol. Cancer 20 (1), 99. doi:10.1186/s12943-021-01396-6
Tao, X., Shao, Y., Lu, R., Ye, Q., Xiao, B., Ye, G., et al. (2020). Clinical significance of hsa_circ_0000419 in gastric cancer screening and prognosis estimation. Pathol. Res. Pract. 216 (1), 152763. doi:10.1016/j.prp.2019.152763
Tenchov, R., Sasso, J. M., Wang, X., Liaw, W. S., Chen, C. A., and Zhou, Q. A. (2022). Exosomes─Nature's lipid nanoparticles, a rising star in drug delivery and diagnostics. ACS Nano 16 (11), 17802–17846. doi:10.1021/acsnano.2c08774
Thery, C., Boussac, M., Véron, P., Ricciardi-Castagnoli, P., Raposo, G., Garin, J., et al. (2001). Proteomic analysis of dendritic cell-derived exosomes: a secreted subcellular compartment distinct from apoptotic vesicles. J. Immunol. 166 (12), 7309–7318. doi:10.4049/jimmunol.166.12.7309
Thrift, A. P., and El-Serag, H. B. (2020). Burden of gastric cancer. Clin. Gastroenterol. Hepatol. 18 (3), 534–542. doi:10.1016/j.cgh.2019.07.045
Tokuhisa, M., Ichikawa, Y., Kosaka, N., Ochiya, T., Yashiro, M., Hirakawa, K., et al. (2015). Exosomal miRNAs from peritoneum lavage fluid as potential prognostic biomarkers of peritoneal metastasis in gastric cancer. PLoS One 10 (7), e0130472. doi:10.1371/journal.pone.0130472
van Niel, G., D'Angelo, G., and Raposo, G. (2018). Shedding light on the cell biology of extracellular vesicles. Nat. Rev. Mol. Cell Biol. 19 (4), 213–228. doi:10.1038/nrm.2017.125
Villarroya-Beltri, C., Gutiérrez-Vázquez, C., Sánchez-Cabo, F., Pérez-Hernández, D., Vázquez, J., Martin-Cofreces, N., et al. (2013). Sumoylated hnRNPA2B1 controls the sorting of miRNAs into exosomes through binding to specific motifs. Nat. Commun. 4, 2980. doi:10.1038/ncomms3980
Wandrey, M., Jablonska, J., Stauber, R. H., and Gül, D. (2023). Exosomes in cancer progression and therapy resistance: molecular insights and therapeutic opportunities. Life (Basel) 13 (10), 2033. doi:10.3390/life13102033
Wang, H., Lu, Z., and Zhao, X. (2019). Tumorigenesis, diagnosis, and therapeutic potential of exosomes in liver cancer. J. Hematol. Oncol. 12 (1), 133. doi:10.1186/s13045-019-0806-6
Wang, M., Yu, W., Cao, X., Gu, H., Huang, J., Wu, C., et al. (2022b). Exosomal CD44 transmits lymph node metastatic capacity between gastric cancer cells via YAP-cpt1a-mediated FAO reprogramming. Front. Oncol. 12, 860175. doi:10.3389/fonc.2022.860175
Wang, M., Zhao, C., Shi, H., Zhang, B., Zhang, L., Zhang, X., et al. (2014). Deregulated microRNAs in gastric cancer tissue-derived mesenchymal stem cells: novel biomarkers and a mechanism for gastric cancer. Br. J. Cancer 110 (5), 1199–1210. doi:10.1038/bjc.2014.14
Wang, M., Zhao, X., Qiu, R., Gong, Z., Huang, F., Yu, W., et al. (2021b). Lymph node metastasis-derived gastric cancer cells educate bone marrow-derived mesenchymal stem cells via YAP signaling activation by exosomal Wnt5a. Oncogene 40 (12), 2296–2308. doi:10.1038/s41388-021-01722-8
Wang, Q., Li, Z., Sun, L., Chen, B., Zhao, Y., Shen, B., et al. (2018a). Platelets enhance the ability of bone-marrow mesenchymal stem cells to promote cancer metastasis. Onco Targets Ther. 11, 8251–8263. doi:10.2147/OTT.S181673
Wang, X., Huang, J., Chen, W., Li, G., Li, Z., and Lei, J. (2022a). The updated role of exosomal proteins in the diagnosis, prognosis, and treatment of cancer. Exp. Mol. Med. 54 (9), 1390–1400. doi:10.1038/s12276-022-00855-4
Wang, X., Luo, G., Zhang, K., Cao, J., Huang, C., Jiang, T., et al. (2018b). Hypoxic tumor-derived exosomal miR-301a mediates M2 macrophage polarization via PTEN/PI3Kγ to promote pancreatic cancer metastasis. Cancer Res. 78 (16), 4586–4598. doi:10.1158/0008-5472.CAN-17-3841
Wang, X., Zhang, H., Bai, M., Ge, S., Deng, T., Liu, R., et al. (2018c). Exosomes serve as nanoparticles to deliver anti-miR-214 to reverse chemoresistance to cisplatin in gastric cancer. Mol. Ther. 26 (3), 774–783. doi:10.1016/j.ymthe.2018.01.001
Wang, Y., Jiang, R., Zhao, H., Li, F., Li, Y., and Zhu, M. (2023). TTN-AS1 delivered by gastric cancer cell-derived exosome induces gastric cancer progression through in vivo and in vitro studies. Cell Biol. Toxicol. 39 (2), 557–571. doi:10.1007/s10565-022-09762-w
Wang, Y., Wang, H., Zheng, R., Wu, P., Sun, Z., Chen, J., et al. (2021a). Circular RNA ITCH suppresses metastasis of gastric cancer via regulating miR-199a-5p/Klotho axis. Cell Cycle 20 (5-6), 522–536. doi:10.1080/15384101.2021.1878327
Wang, Y., Zhang, J., Shi, H., Wang, M., Yu, D., Fu, M., et al. (2024). M2 tumor-associated macrophages-derived exosomal MALAT1 promotes glycolysis and gastric cancer progression. Adv. Sci. (Weinh) 11 (24), e2309298. doi:10.1002/advs.202309298
Wei, D., Zhan, W., Gao, Y., Huang, L., Gong, R., Wang, W., et al. (2021). RAB31 marks and controls an ESCRT-independent exosome pathway. Cell Res. 31 (2), 157–177. doi:10.1038/s41422-020-00409-1
Wei, W., Li, J., Huang, J., Jiang, Q., Lin, C., Hu, R., et al. (2025). Exosomal miR-3681-3p from M2-polarized macrophages confers cisplatin resistance to gastric cancer cells by targeting MLH1. Mol. Med. Rep. 31 (4), 94. doi:10.3892/mmr.2025.13459
Willms, E., Johansson, H. J., Mäger, I., Lee, Y., Blomberg, K. E. M., Sadik, M., et al. (2016). Cells release subpopulations of exosomes with distinct molecular and biological properties. Sci. Rep. 6, 22519. doi:10.1038/srep22519
Wu, H., Fu, M., Liu, J., Chong, W., Fang, Z., Du, F., et al. (2021). The role and application of small extracellular vesicles in gastric cancer. Mol. Cancer 20 (1), 71. doi:10.1186/s12943-021-01365-z
Wu, S., Tang, C., Zhang, Q. W., Zhuang, Q., Ye, X., Xia, J., et al. (2023). Overexpression of RAB31 in gastric cancer is associated with released exosomes and increased tumor cell invasion and metastasis. Cancer Med. 12 (12), 13497–13510. doi:10.1002/cam4.6007
Wu, Y., Li, J., Zeng, Y., Pu, W., Mu, X., Sun, K., et al. (2022). Exosomes rewire the cartilage microenvironment in osteoarthritis: from intercellular communication to therapeutic strategies. Int. J. Oral Sci. 14 (1), 40. doi:10.1038/s41368-022-00187-z
Xia, X., Wang, S., Ni, B., Xing, S., Cao, H., Zhang, Z., et al. (2020). Hypoxic gastric cancer-derived exosomes promote progression and metastasis via MiR-301a-3p/PHD3/HIF-1α positive feedback loop. Oncogene 39 (39), 6231–6244. doi:10.1038/s41388-020-01425-6
Xie, Y., Wu, J., Xu, A., Ahmeqd, S., Sami, A., Chibbar, R., et al. (2018). Heterologous human/rat HER2-specific exosome-targeted T cell vaccine stimulates potent humoral and CTL responses leading to enhanced circumvention of HER2 tolerance in double transgenic HLA-A2/HER2 mice. Vaccine 36 (11), 1414–1422. doi:10.1016/j.vaccine.2018.01.078
Xin, L., Wu, Y., Liu, C., Zeng, F., Wang, J. L., Wu, D. Z., et al. (2021). Exosome-mediated transfer of lncRNA HCG18 promotes M2 macrophage polarization in gastric cancer. Mol. Immunol. 140, 196–205. doi:10.1016/j.molimm.2021.10.011
Xin, Y., Li, X., Zhang, M., Shang, Z., Luo, Z., Wang, Y., et al. (2023). Fusobacterium nucleatum-induced exosomal HOTTIP promotes gastric cancer progression through the microRNA-885-3p/EphB2 axis. Cancer Sci. 114 (6), 2360–2374. doi:10.1111/cas.15781
Xu, G., Zhang, B., Ye, J., Cao, S., Shi, J., Zhao, Y., et al. (2019). Exosomal miRNA-139 in cancer-associated fibroblasts inhibits gastric cancer progression by repressing MMP11 expression. Int. J. Biol. Sci. 15 (11), 2320–2329. doi:10.7150/ijbs.33750
Xu, X. H., Shao, S. L., Guo, D., Ge, L. N., Wang, Z., Liu, P., et al. (2023). Roles of microRNAs and exosomes in Helicobacter pylori associated gastric cancer. Mol. Biol. Rep. 50 (1), 889–897. doi:10.1007/s11033-022-08073-x
Xu, Z., Zeng, S., Gong, Z., and Yan, Y. (2020). Exosome-based immunotherapy: a promising approach for cancer treatment. Mol. Cancer 19 (1), 160. doi:10.1186/s12943-020-01278-3
Yaddanapudi, K., Mitchell, R. A., Putty, K., Willer, S., Sharma, R. K., Yan, J., et al. (2012). Vaccination with embryonic stem cells protects against lung cancer: is a broad-spectrum prophylactic vaccine against cancer possible? PLoS One 7 (7), e42289. doi:10.1371/journal.pone.0042289
Yan, Y., Fu, G., and Ming, L. (2017). Exosomes participate in the carcinogenesis and the malignant behavior of gastric cancer. Scand. J. Gastroenterol. 52 (5), 499–504. doi:10.1080/00365521.2016.1278458
Yang, B., Chen, Y., and Shi, J. (2019). Exosome biochemistry and advanced nanotechnology for next-generation theranostic platforms. Adv. Mater 31 (2), e1802896. doi:10.1002/adma.201802896
Yang, C. S., Chou, G., Jan, Y. J., Wang, J., Yeh, D. C., and Teng, C. L. (2007). Primary lymphohistiocytic variant of anaplastic large cell lymphoma of the stomach. J. Chin. Med. Assoc. 70 (2), 71–75. doi:10.1016/S1726-4901(09)70305-3
Yang, E., Wang, X., Gong, Z., Yu, M., Wu, H., and Zhang, D. (2020). Exosome-mediated metabolic reprogramming: the emerging role in tumor microenvironment remodeling and its influence on cancer progression. Signal Transduct. Target Ther. 5 (1), 242. doi:10.1038/s41392-020-00359-5
Yang, J., Zhang, X., Cao, J., Xu, P., Chen, Z., Wang, S., et al. (2021a). Circular RNA UBE2Q2 promotes malignant progression of gastric cancer by regulating signal transducer and activator of transcription 3-mediated autophagy and glycolysis. Cell Death Dis. 12 (10), 910. doi:10.1038/s41419-021-04216-3
Yang, X., Cai, S., Shu, Y., Deng, X., Zhang, Y., He, N., et al. (2021b). Exosomal miR-487a derived from m2 macrophage promotes the progression of gastric cancer. Cell Cycle 20 (4), 434–444. doi:10.1080/15384101.2021.1878326
Yen, E. Y., Miaw, S. C., Yu, J. S., and Lai, I. R. (2017). Exosomal TGF-β1 is correlated with lymphatic metastasis of gastric cancers. Am. J. Cancer Res. 7 (11), 2199–2208.
Yu, D., Zhang, J., Wang, M., Ji, R., Qian, H., Xu, W., et al. (2024). Exosomal miRNAs from neutrophils act as accurate biomarkers for gastric cancer diagnosis. Clin. Chim. Acta 554, 117773. doi:10.1016/j.cca.2024.117773
Yu, L., Xie, J., Liu, X., Yu, Y., and Wang, S. (2021). Plasma exosomal CircNEK9 accelerates the progression of gastric cancer via miR-409-3p/MAP7 Axis. Dig. Dis. Sci. 66 (12), 4274–4289. doi:10.1007/s10620-020-06816-z
Yue, F., Peng, K., Zhang, L., and Zhang, J. (2021). Circ_0004104 accelerates the progression of gastric cancer by regulating the miR-539-3p/RNF2 Axis. Dig. Dis. Sci. 66 (12), 4290–4301. doi:10.1007/s10620-020-06802-5
Zang, X., Wang, R., Wang, Z., Qiu, S., Zhang, F., Zhou, L., et al. (2024). Exosomal circ50547 as a potential marker and promotor of gastric cancer progression via miR-217/HNF1B axis. Transl. Oncol. 45, 101969. doi:10.1016/j.tranon.2024.101969
Zhang, C., Huang, W., Xu, X., and Zuo, S. (2023a). Flexible endoscopic instrument for diagnosis and treatment of early gastric cancer. Med. Biol. Eng. Comput. 61 (11), 2815–2828. doi:10.1007/s11517-023-02911-1
Zhang, F., Jiang, J., Qian, H., Yan, Y., and Xu, W. (2023c). Exosomal circRNA: emerging insights into cancer progression and clinical application potential. J. Hematol. Oncol. 16 (1), 67. doi:10.1186/s13045-023-01452-2
Zhang, H., Deng, T., Liu, R., Bai, M., Zhou, L., Wang, X., et al. (2017). Exosome-delivered EGFR regulates liver microenvironment to promote gastric cancer liver metastasis. Nat. Commun. 8, 15016. doi:10.1038/ncomms15016
Zhang, J., Qiu, W. q., Zhu, H., Liu, H., Sun, J. h., Chen, Y., et al. (2020b). HOTAIR contributes to the carcinogenesis of gastric cancer via modulating cellular and exosomal miRNAs level. Cell Death Dis. 11 (9), 780. doi:10.1038/s41419-020-02946-4
Zhang, J., Song, Y., Zhang, C., Zhi, X., Fu, H., Ma, Y., et al. (2015). Circulating MiR-16-5p and MiR-19b-3p as two novel potential biomarkers to indicate progression of gastric cancer. Theranostics 5 (7), 733–745. doi:10.7150/thno.10305
Zhang, J., Yu, D., Ji, C., Wang, M., Fu, M., Qian, Y., et al. (2024c). Exosomal miR-4745-5p/3911 from N2-polarized tumor-associated neutrophils promotes gastric cancer metastasis by regulating SLIT2. Mol. Cancer 23 (1), 198. doi:10.1186/s12943-024-02116-6
Zhang, L., and Yu, D. (2019). Exosomes in cancer development, metastasis, and immunity. Biochim. Biophys. Acta Rev. Cancer 1871 (2), 455–468. doi:10.1016/j.bbcan.2019.04.004
Zhang, M., Hu, S., Liu, L., Dang, P., Liu, Y., Sun, Z., et al. (2023b). Engineered exosomes from different sources for cancer-targeted therapy. Signal Transduct. Target Ther. 8 (1), 124. doi:10.1038/s41392-023-01382-y
Zhang, R., Chen, X., Miao, C., Chen, Y., Li, Y., Shen, J., et al. (2024b). Tumor-associated macrophage-derived exosomal miR-513b-5p is a target of jianpi yangzheng decoction for inhibiting gastric cancer. J. Ethnopharmacol. 318 (Pt B), 117013. doi:10.1016/j.jep.2023.117013
Zhang, X., Wang, S., Wang, H., Cao, J., Huang, X., Chen, Z., et al. (2019). Circular RNA circNRIP1 acts as a microRNA-149-5p sponge to promote gastric cancer progression via the AKT1/mTOR pathway. Mol. Cancer 18 (1), 20. doi:10.1186/s12943-018-0935-5
Zhang, Y., Bi, J., Huang, J., Tang, Y., and Du, S. (2020a). Exosome: a review of its classification, isolation techniques, storage, diagnostic and targeted therapy applications. Int. J. Nanomedicine 15, 6917–6934. doi:10.2147/IJN.S264498
Zhang, Y., Guo, S., Mao, T., Guo, J., Zhang, Q., Tian, Z., et al. (2024a). Tumor-derived exosomal LINC01480 upregulates VCAM1 expression by acting as a competitive endogenous RNA of miR-204-5p to promote gastric cancer progression. ACS Biomater. Sci. Eng. 10 (1), 550–562. doi:10.1021/acsbiomaterials.3c00394
Zhang, Y. Q., Shi, S. S., Li, Y. F., Yang, Y., Bai, P., and Qiao, C. H. (2023d). Melatonin inhibits gastric cancer cell proliferation by suppressing exosome miR-27b-3p expression. Anticancer Res. 43 (10), 4413–4424. doi:10.21873/anticanres.16637
Zhang, Z., Sun, C., Zheng, Y., and Gong, Y. (2022). circFCHO2 promotes gastric cancer progression by activating the JAK1/STAT3 pathway via sponging miR-194-5p. Cell Cycle 21 (20), 2145–2164. doi:10.1080/15384101.2022.2087280
Zhao, R., Zhang, Y., Zhang, X., Yang, Y., Zheng, X., Li, X., et al. (2018). Exosomal long noncoding RNA HOTTIP as potential novel diagnostic and prognostic biomarker test for gastric cancer. Mol. Cancer 17 (1), 68. doi:10.1186/s12943-018-0817-x
Zhao, W., Zhang, Y., Zhang, W., Sun, Y., Zheng, B., Wang, J., et al. (2023). Exosomal LINC00355 promotes the malignant progression of gastric cancer through histone deacetylase HDAC3-mediated TP53INP1 transcriptional inhibition. Life Sci. 315, 121387. doi:10.1016/j.lfs.2023.121387
Zheng, P., Gao, H., Xie, X., and Lu, P. (2022). Plasma exosomal hsa_circ_0015286 as a potential diagnostic and prognostic biomarker for gastric cancer. Pathol. Oncol. Res. 28, 1610446. doi:10.3389/pore.2022.1610446
Zheng, P., Zhang, H., Gao, H., Sun, J., Li, J., Zhang, X., et al. (2020b). Plasma exosomal long noncoding RNA lnc-SLC2A12-10:1 as a novel diagnostic biomarker for gastric cancer. Onco Targets Ther. 13, 4009–4018. doi:10.2147/OTT.S253600
Zheng, P. M., Gao, H. J., Li, J. M., Zhang, P., and Li, G. (2020a). Effect of exosome-derived miR-223 from macrophages on the metastasis of gastric cancer cells. Zhonghua Yi Xue Za Zhi 100 (22), 1750–1755. doi:10.3760/cma.j.cn112137-20200425-01309
Zhou, C., Qiao, C., Ji, J., Xi, W., Jiang, J., Guo, L., et al. (2023). Plasma exosome proteins ILK1 and CD14 correlated with organ-specific metastasis in advanced gastric cancer patients. Cancers (Basel) 15 (15), 3986. doi:10.3390/cancers15153986
Zhu, K. G., Yang, J., Zhu, Y., Zhu, Q., Pan, W., Deng, S., et al. (2023). The microprotein encoded by exosomal lncAKR1C2 promotes gastric cancer lymph node metastasis by regulating fatty acid metabolism. Cell Death Dis. 14 (10), 708. doi:10.1038/s41419-023-06220-1
Zhu, L., Kalimuthu, S., Gangadaran, P., Oh, J. M., Lee, H. W., Baek, S. H., et al. (2017). Exosomes derived from natural killer cells exert therapeutic effect in melanoma. Theranostics 7 (10), 2732–2745. doi:10.7150/thno.18752
Zhu, M., Zhang, N., He, S., and Lu, X. (2020). Exosomal miR-106a derived from gastric cancer promotes peritoneal metastasis via direct regulation of Smad7. Cell Cycle 19 (10), 1200–1221. doi:10.1080/15384101.2020.1749467
Zhu, M., Zhang, N., and Ma, J. (2022). Integration of exosomal miR-106a and mesothelial cells facilitates gastric cancer peritoneal dissemination. Cell Signal 91, 110230. doi:10.1016/j.cellsig.2021.110230
Keywords: gastric cancer, exosome, tumor microenvironment, regulation, interaction
Citation: Li X, Lu X, Liu M, Chen J and Lu X (2025) Extracellular vesicles: messengers of cross-talk between gastric cancer cells and the tumor microenvironment. Front. Cell Dev. Biol. 13:1561856. doi: 10.3389/fcell.2025.1561856
Received: 16 January 2025; Accepted: 31 March 2025;
Published: 16 April 2025.
Edited by:
Yi Yao, Renmin Hospital of Wuhan University, ChinaReviewed by:
Adeel Khan, Southeast University, ChinaSagnik Nag, Monash University Malaysia, Malaysia
Copyright © 2025 Li, Lu, Liu, Chen and Lu. This is an open-access article distributed under the terms of the Creative Commons Attribution License (CC BY). The use, distribution or reproduction in other forums is permitted, provided the original author(s) and the copyright owner(s) are credited and that the original publication in this journal is cited, in accordance with accepted academic practice. No use, distribution or reproduction is permitted which does not comply with these terms.
*Correspondence: Xirong Lu, MTM4MTI3MDAwOTVAMTYzLmNvbQ==; Junjie Chen, bnRmeWNqakBudHUuZWR1LmNu
†These authors have contributed equally to this work