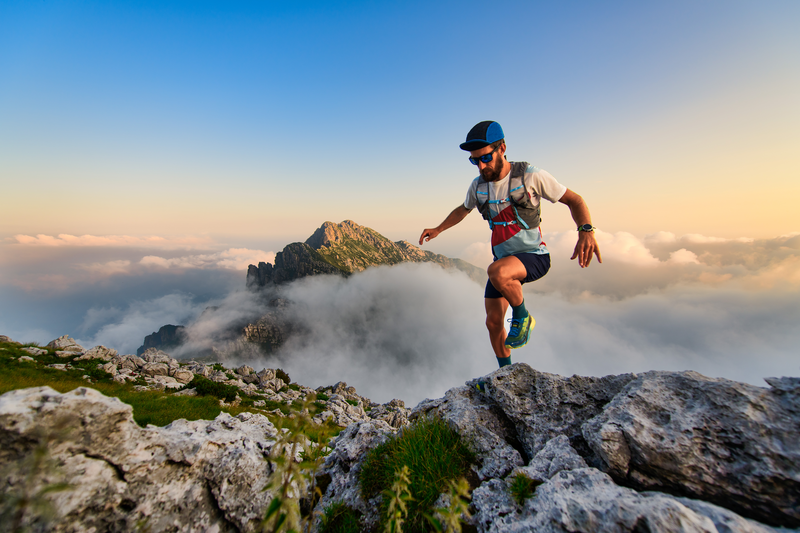
95% of researchers rate our articles as excellent or good
Learn more about the work of our research integrity team to safeguard the quality of each article we publish.
Find out more
REVIEW article
Front. Cell Dev. Biol. , 26 March 2025
Sec. Molecular and Cellular Pathology
Volume 13 - 2025 | https://doi.org/10.3389/fcell.2025.1556359
This article is part of the Research Topic Advances in Immunobiology of Inflammation View all articles
Rheumatoid arthritis (RA) is a chronic autoimmune disease that causes synovial joint inflammation as well as bone destruction and erosion, typically characterized by joint pain, swelling, and stiffness, with complications and persistent pain after remission posing a significant health burden for RA patients. The etiology of RA has not yet been fully elucidated, but a large number of studies have shown that the initiation of inflammation in RA is closely related to T-cell activation, the production of a variety of pro-inflammatory cytokines, macrophage M1/M2 imbalance, homeostatic imbalance of the intestinal flora, fibroblast-like synoviocytes (FLSs) and synovial tissue macrophages (STMs) in the synovial lumen of joints that exhibit an aggressive phenotype. While the resolution of RA is less discussed, therefore, we provided a systematic review of the relevant remission mechanisms including blocking T cell activation, regulating macrophage polarization status, modulating the signaling pathway of FLSs, modulating the subpopulation of STMs, and inhibiting the relevant inflammatory factors, as well as the probable causes of persistent arthritis pain after the remission of RA and its pain management methods. Achieving resolution in RA is crucial for improving the quality of life and long-term prognosis of patients. Thus, understanding these mechanisms provide novel potential for further drug development and treatment of RA.
RA is a chronic autoimmune disease that causes inflammation of the synovial joints and bone destruction and erosion, typically characterized by joint pain, swelling and stiffness (Brown et al., 2024; Conrad et al., 2023; McInnes and Schett, 2011; Mueller et al., 2021). In addition, RA also causes damage to other tissues and organs such as the heart, kidneys, lungs, digestive system, eyes, skin, and nervous system (Giles, 2019; Wu et al., 2022). It is worth noting that about 40% of RA patients suffer from complications, and the incidence of serious complications is 8.3%, with cardiovascular disease, interstitial lung disease, osteoporosis, and metabolic syndrome predominating (Taylor et al., 2021). RA is widespread worldwide, with a prevalence of 0.5%–2%, with a higher prevalence in women, smokers, and those with a family history (Myasoedova et al., 2020).
At present, the etiology of RA has not yet been fully elucidated, and its heterogeneity precludes a single, unified description of pathogenesis. It has been suggested that a combination of genetic factors [e.g., variation at human leukocyte antigen (HLA) loci], environmental exposures (e.g., smoking), and the microbiota contribute to the development of RA (Brown et al., 2024; Smolen et al., 2018). Among them, HLA alleles, especially the HLA-DR1 and HLA-DR4 loci, play an important role in the development of RA by mechanisms that may be related to the formation of citrullinated antigens and immune tolerance of CD4+ T cells (Wysocki et al., 2020; Nagafuchi et al., 2022). Sustained antigenic stimulation provided by environmental factors leads to an immune response by inducing the activation of peptidylarginine deiminase (PAD) to produce citrullinated antigens, which stimulate autoantibody production and form immune complexes (Klareskog et al., 2005; Malmström et al., 2016). In addition, pathogenic microorganisms can influence the homeostatic imbalance of the intestinal flora, thereby affecting the immune system and damaging the mesenteric mucosal barrier, promoting joint damage in RA (Maeda et al., 2016). But the most noteworthy immune processes occur in the synovial membrane and synovial fluid (Nygaard and Firestein, 2020; Hu et al., 2019). During the process of RA, joint damage or transient infections can activate the vascular system and allow the entry of autoantibodies. They form immune complexes with joint tissue antigens, activate stromal cells, resident macrophages, mast cells, and osteoclasts, release vasoactive mediators and chemokines, recruit leukocytes, and exacerbate inflammation (Malmström et al., 2016; Smolen et al., 2016). For example, STMs release cytokines such as tumor necrosis factor α (TNF-α), interleukin-1 (IL-1), and interleukin-6 (IL-6) (Ding et al., 2023; McInnes and Schett, 2017). FLSs are stimulated by these cytokines to release inflammatory mediators, chemokines, etc., which promote the influx and proliferation of immune cells. And then, inflammatory factors and FLSs stimulate osteoclast activity, leading to the progression of bone erosion (Yoshitomi, 2019). In addition, activated FLSs produce large amounts of matrix metalloproteinase (MMPs) and receptor activator of nuclear factor-κB ligand (RANKL), which further accelerate the degradation of intra-articular cartilage and bone tissue, leading to further bone erosion development (Ospelt et al., 2008).
Previous treatments often used glucocorticoids or nonsteroidal anti-inflammatory drugs (NSAIDs) (e.g., Ibuprofen and Celecoxib), which, at the cost of significant toxicity, slowed down joint damage and disability at best. In contrast, contemporary therapeutic strategies aim to induce remission of RA and prevent damage before it occurs (Schett et al., 2021; Prasad et al., 2023). In this review, we will focus on summarizing the mechanisms of dissipation and remission of inflammation during the period of RA. We will also discuss the possible causes of persistent arthritis pain after RA remission and its pain management approaches.
Macrophages are pivotal effector cells that play a pivotal role in the pathogenesis of RA through their capacity to differentiate into various functional phenotypes (Wang et al., 2019). An imbalance in immune homeostasis is created when there are more pro-inflammatory M1-type macrophages than anti-inflammatory M2-type macrophages (Cutolo et al., 2022; Stein et al., 1992; Pan et al., 2021). This imbalance leads to an exacerbation of joint inflammation. Reprogramming of pro-inflammatory macrophages toward anti-inflammatory phenotypes is therefore considered to be an effective way to target the M1/M2 imbalance. Modulating macrophage polarization in order to correct the M1/M2 imbalance represents a promising therapeutic strategy (Zheng et al., 2024a). Metabolically engineered exosomes enable targeted reprogramming of macrophages. When systemically administered into mice with collagen-induced arthritis (CIA), these exosomes effectively accumulated in the inflamed joints, induce M2 polarization, substantially suppresses inflammation in joints and provides strong chondroprotection and osteoprotection (You et al., 2021). Encapsulated a plasmid DNA encoding the anti-inflammatory cytokine interleukin-10 (IL-10pDNA) and the chemotherapeutic drug betamethasone sodium phosphate (BSP) into biomimetic vector M2 exosomes (M2 Exo) derived from M2-type macrophages from M2-type macrophages to form the M2 Exo/pDNA/BSP co-delivery system. This system can promote macrophage polarization from M1 to M2 through the synergistic effect of IL-10 pDNA and BSP in reducing the secretion of pro-inflammatory cytokines (IL-1β, TNF-α) and increasing the expression of IL-10 cytokine (Li et al., 2022). Meanwhile, M2 macrophages-derived extracellular vesicle (EVs) could also convert activated M1 macrophages into M2 macrophages through in situ macrophage reprogramming and producing a distinct protein expression pattern characteristic of anti-inflammatory M2 macrophages. After administration of M2-EVs into the joint of a CIA mouse model, the STMs polarization was significantly shifted from M1 to M2 phenotype in decreased joint swelling, arthritic index score and synovial inflammation, with corresponding reductions in bone erosion and articular cartilage damage (Kim et al., 2022a). In addition to this, nanoparticle-based drug delivery is a feasible and common strategy for the treatment of RA. Folic acid-modified silver nanoparticles (FA-AgNPs) are bioactive nanoparticles. After entering the cells, FA-AgNPs dissolved and released Ag+ in response to intracellular glutathione, which exerts a series of anti-inflammatory functions, induces apoptosis in M1 macrophages, scavenges reactive oxygen species (ROS), and promotes polarization of M2 macrophages, targeted RA therapy via simultaneous M1 macrophage apoptosis and M1/M2 macrophages reprogramming (Yang et al., 2021). Celastrol-loaded enzyme-responsive nanoparticles dually target osteoclasts and inflammatory macrophages derived from patients with RA, leading to increased apoptosis of these cells. And In an adjuvant-induced arthritis (AIA) rat model, it effectively reduced the number of osteoclasts and inflammatory macrophages in these joints, relieving inflammation and repairing bone erosion (Deng et al., 2021). Nuclear factor-kappa B (NF-κB), Janus kinase (JAK) 1/2 and Extracellular signal-regulated kinase (ERK1)/Notch1 are also potential targets that could selectively suppress proinflammatory macrophages (Zerrouk et al., 2024). JAK inhibitors are potential RA drugs. Phosphodiesterase 3B (PDE3B) is a member of the phosphohydrolase family, which plays a role in numerous signal transduction pathways. The intravenous administration of liposomes loaded with PDE3B siRNA was observed to promote the macrophage anti-inflammatory program, reduce the inflammatory response and synoviocyte infiltration, and attenuate bone and cartilage erosion in CIA mice. A mechanistic study revealed that the depletion of PDE3B increased cAMP levels, which enhanced the PKA-CREB-C/EBPβ pathway and resulted in the transcription of anti-inflammatory program-related genes (Chen et al., 2024). Polyphyllin I (PPI), a principal constituent of Paris polyphyllin, exhibits a selective inhibitory effect on a range of tumor cells. PPI has been shown to suppress the NF-κB-mediated production of pro-inflammatory effectors in activated macrophages, thereby demonstrating effective amelioration of synovial inflammation in the ankle joint of CIA mice (Wang et al., 2018). In addition, by down-regulating hypoxia-associated peptidyl arginine deiminase 4 (PADI4) expression, the number of M1 macrophages was reduced, and the degree of joint swelling and destruction was effectively alleviated in CIA rats, implying that the inflammatory environment can be eased by decreasing PADI4 expression and improving the hypoxic environment (Cheng et al., 2021). In addition, many other mechanisms are involved in regulating the phenotypic transition from M1 to M2 (Feng et al., 2024; Ross et al., 2021).
T cells are deeply involved in the pathogenesis of RA, especially CD4+ T cells drive inflammation in RA joints (Takeshita et al., 2019; Farh et al., 2014; Hu et al., 2011). Th17 can promote synovitis by producing a variety of pro-inflammatory cytokines, and notably, regulatory T cells (Tregs) can suppress inflammatory responses and maintain immune tolerance (Inamo et al., 2021; Wu et al., 2020). Therefore, this paragraph explores the alleviation of RA symptoms by blocking T-cell activation, inhibiting CD4+ T-cell pyroptosis, reducing Th17 cell differentiation, and modulating the balance of Th17/Treg and T follicular helper (Tfh)/T follicular regulatory cells (Tfr) (Wu et al., 2020; Yang et al., 2024; Jiang et al., 2023; Zhang et al., 2020).
RA patients have more pyroptotic CD4+ T cells in the blood and synovium compared to healthy individuals. Knockdown or pharmacological inhibition of arachidonate 5-lipoxygenase (ALOX5) was found to inhibit CD4+ T cell pyroptosis and ameliorate symptoms in two rodent models of RA. The underlying mechanism involves an increase in the activity of ALOX5 in RA CD4+ T cells was found to enhance the production of the Leukotriene A4 (LTA4) derivative Leukotriene B4 (LTB4), which stimulated Ca2+ influx through Orai protein 3 (ORAI3) channels. This resulted in the activation of NLRP3 inflammasomes and pyroptosis (Cai et al., 2024). Interfering with T cell activation Rho GTPase activating protein (TAGAP) significantly reduced the levels of RhoA and NLRP3 thereby decreasing the differentiation of Th17 cells, alleviating the inflammation and swelling of ankle joints and slowing down the progression of RA in CIA rats (Sun et al., 2023). α-Mangostin (MG) also inhibits Th17 cell differentiation through activation of choline anti-inflammatory pathway (CAP), reduces secretion of TNF-α and IL-1β under inflammatory conditions, and improves the peripheral immune milieu and alleviates inflammatory responses in CIA rats (Yin et al., 2020). Studies have found that Tregs are functionally defective in RA patients (Firestein and McInnes, 2017). Therefore, restoring their function not only controls inflammation, but also restores tolerance in these patients (Jang et al., 2022; Mueller et al., 2021; Byng-Maddick and Ehrenstein, 2015). Interleukin-2 (IL-2) is a pleiotropic cytokine that promote inflammatory response and maintains immune tolerance, and low-dose IL-2 therapy can mediate the progression toward immune tolerance in a variety of autoimmune diseases (Spolski et al., 2018; Rosenzwajg et al., 2015; Hartemann et al., 2013; Malek, 2008). Studies have shown that IL-2 exerts anti-inflammatory effects and maintains immune tolerance by regulating the balance of Th17/Treg and Tfh/Tfr, and is a potential treatment for RA (Wu et al., 2020). Specifically, Tregs highly express the IL-2 receptor alpha chain (CD25), making them highly sensitive to IL-2. Although other T cells also express IL-2 receptors, at low doses, IL-2 has a weaker effect on their proliferation and activation. Clinically relevant drugs that improve arthritis by targeting T cells are available. Abatacept is the only T cell co-stimulation modulator approved thus far for the treatment of moderate-to-severe RA (Schiff, 2015). Abatacept treatment significantly reduced the proportions of Tregs and PD-1+ TFh cells and is effective in clinical patients with RA (Aldridge et al., 2022). While abatacept is a potential RA target drug, it may also leading side effects like infection, allergy and tumor. In addition to this, tocilizumab (TCZ), a monoclonal antibody of IL-6R, significantly reduced the number of synovial T cells (Chatzidionysiou et al., 2021). TCZ may also increase the risk of infection, allergy and tumor. The DNA-methylation inhibitors decitabine a potential to ameliorate chronic and acute animal models of RA. by relying on the fact that indoleamine 2,3-dioxygenase (IDO) can generate a regulatory T-cell population that promotes apoptosis of Th1 and Th17 cells (Huang et al., 2021a). While decitabine may also cause myelosuppression, increased risk of infection, gastrointestinal reactions, fatigue and fever. Notably, recent studies have demonstrated sustained drug-free remission using chimeric antigen receptor T cell (CAR-T) therapy for a longstanding case of RA with diffuse large B-cell lymphoma (DLBCL) (Szabo et al., 2024). Besides, there are several clinical trails processed in RA patients under CAR-T treatment. For example, KYV101, an anti-CD19 CAR-T, was developed to against autologous B cells. SBT777101, a CAR-Treg, was also developed to promote Treg proliferation (Table 1). Although these CAR-T treatments possess huge potential for RA patients, they may also leading side effects (e.g., Cytokine Release Syndrome, Immune Effector Cell-Associated Neurotoxicity Syndrome, B-cell Aplasia and Hypogammaglobulinemia, Tumor Lysis Syndrome and off-taget). Moreover, certain T-cell subsets employ their anti-inflammatory attributes to facilitate joint repair. Consequently, the artificial reprogramming of T-cell subsets through the modulation of their metabolic state is anticipated to pave the way for the development of novel molecules that could potentially address RA in the future (Mondal et al., 2024) (Table 1).
Besides CD4+ T cells, CD8+ T cells also drive inflammation in RA. CD8+ T cells are notably abundant in synovium and make more interferon (IFN)-γ and nearly as much TNF as CD4+ T cells (Jonsson et al., 2022). The majority of these synovium CD8+ T cells are granzyme K (GzmK) and granzyme B (GzmB) double positive and acts as cytokines producers to drive inflammation (Jonsson et al., 2022). In addition, CD8+ T cells sourced GzmK, a tryptase-like protease, can cleaving fibroblasts produced complement proteins to activate the complement cascade and drive RA inflammation (Donado et al., 2025). GzmK is distinct from GzmB, and currently lack corresponding inhibitors due to limited research. GzmB is produced by NK cells, Cytotoxic T lymphocytes (CTLs) and macrophages during RA and validated for RA treatment. ZINC000004557101, a natural compound, is a potential drug targeting GzmB for treating RA (Wang et al., 2022). B cells are also central to the pathogenesis of RA, contributing to autoantibody production, inflammation, and immune dysregulation, and B cells depletion with anti-CD20 antibody significantly improve RA symptoms (Roll et al., 2006) (Figure 1).
Figure 1. Resolution of Inflammation during RA. Resolution of RA requires the balance between M1/M2 macrophage, the function of T helper cells, STMs and FLSs, and the function of cytokines like IL-6. Besides, gut microbiota also contributes to RA resolution. EVs, extracellular vesicle; FA-AgNPs, Folic acid-modified silver nanoparticles; NF-κB, Nuclear factor-kappa B; JAK, Janus kinase; ERK1, Extracellular signal-regulated kinase; MAPK/ERK, Mitogen-Activated Protein Kinase/Extracellular Signal-Regulated Kinase; PDE3B, Phosphodiesterase 3B; PPI, Polyphyllin I; PADI4, peptidyl arginine deiminase 4; ALOX5, arachidonate 5-lipoxygenase; TAGAP, T cell activation Rho GTPase activating protein; TCZ, tocilizumab; CAR-T, chimeric antigen receptor T cell; SHH, Sonic Hedgehog; EMS, Er Miao San; SFRP1, Secreted frizzled-related protein 1; microRNA, miRNA; lncRNAs, long noncoding RNAs; FLIP, FLICE-like inhibitory protein; DBZP, Polysaccharides from Dianbaizhu; SIOL, Saussurea involucrata oral liquid; THH, Tripterygium hypoglaucum Hutch; SPP, Sporidiobolus pararoseus polysaccharide; FGF10, fibroblast growth factor 10; TLR-2, Toll-like receptor 2; STMs, synovial macrophages; M2 Exo, M2 exosomes; BSP, betamethasone sodium phosphate; IL-10pDNA, Encapsulated a plasmid DNA encoding the anti-inflammatory cytokine interleukin-10; RSK2, Ribosomal S6 Kinase 2.
In RA, FLSs become a key driver of synovial inflammation and joint damage (Nygaard and Firestein, 2020; Tsaltskan and Firestein, 2022; Bartok and Firestein, 2009). FLSs play a pro-inflammatory role through the production of MMPs and cytokines, osteoclast activation, and immune cell recruitment (Diarra et al., 2007; Yamamura et al., 2001; Reparon-Schuijt et al., 2000). This paragraph therefore summarizes the mechanisms involved in alleviating RA by modulating the signaling pathways, metabolism, and apoptosis of FLSs.
It was found that the Sonic Hedgehog (SHH) signaling pathway may mediate RA-through mitogen-activated protein kinases/extracellular signal-regulated kinases (MAPK/ERK) signaling pathway by regulate proliferation and migration of FLSs, which are involved in the development of RA. In contrast, inhibition of the SHH signaling pathway reduces the proliferation and migration of RA patient-derived FLS (RA-FLS), thereby alleviating RA (Liu et al., 2018; Wang et al., 2014; Elia et al., 2007). Artesunate, an anti-malarial drug, can prevent cartilage and bone destruction in rats with CIA by suppression of PDK1-induced activation of Akt and RSK2 phosphorylation, downregulating the expression of MMP-2 and MMP-9, and inhibiting the migration and invasion of RA-FLS (Ma et al., 2019; Gagliardi et al., 2015). Er Miao San (EMS) may be able to inhibit the activation of the Wnt/β-catenin signaling pathway and inhibit the abnormal activation and angiogenesis of FLSs, thus exerting a protective effect against RA (Chen et al., 2023; Huang et al., 2019). Similarly, Secreted frizzled-related protein 1 (SFRP1) also regulates RA-FLS pyroptosis through Wnt/β-catenin and Notch signaling pathways, suggesting that it could be a promising RA biomarker and therapeutic target (Jiang et al., 2022; Park et al., 2015; Collu et al., 2014; Ando et al., 2003). Aberrant activation of the NF-κB signaling cascade has been identified as the underlying cause of the elevated proliferation and impaired apoptosis of FLSs in RA, which subsequently leads to the onset of inflammation (Li et al., 2013; Roman-Blas and Jimenez, 2006). Piperine was observed to directly reduce the phosphorylation of NF-κB and the expression of NF-κB target genes associated with RA-FLS proliferation, apoptosis inhibition, and inflammation. Additionally, it was noted to increase the expression of apoptosis-related genes and to decrease the protein levels of cytokines and chemokines secreted by FLSs, which resulted in an anti-inflammatory effect (Baito et al., 2023; Pradeep and Kuttan, 2004).
Recent metabolomic studies have demonstrated that metabolic changes, including those in glucose, lipid, and amino acid metabolism, occur prior to the onset of synovitis (Hu et al., 2024; Garcia-Carbonell et al., 2016). RA-FLS exhibit specific overexpression of glycolytic enzymes, resulting in elevated glycolysis. This elevated glycolysis serves to generate ATP and plays a pivotal role in immune regulation, angiogenesis, and adaptation to hypoxia (Li et al., 2023; Singh et al., 2015). Inhibition of glycolysis may exert a direct effect on synoviocyte-mediated inflammatory functions. Glucose deprivation or incubation of the FLS with glycolytic inhibitors has been observed to impair cytokine secretion and decrease the rate of proliferation and migration of the cells. Furthermore, this has been demonstrated to result in a notable reduction in the severity of arthritis in this mouse model (Garcia-Carbonell et al., 2016; Ekwall et al., 2011). Furthermore, glycolytic enzymes, such as hexokinase 2 (HK2), are markedly elevated in RA-FLS cells. Consequently, targeting the HK2 enzyme may represent a promising, selective therapeutic target for arthritis, offering a safer alternative to global glycolysis inhibition (Masoumi et al., 2020). Non-coding RNAs have been demonstrated to regulate a number of key biological processes in FLSs, including proliferation, migration, invasion, apoptosis, and inflammatory responses. Additionally, they influence DNA methylation and osteogenic differentiation in FLSs. Given these observations, non-coding RNAs have emerged as a promising avenue for the development of biomarkers for the diagnosis of RA. Local targeting of non-coding RNAs in FLSs represents a promising approach for future therapeutic strategies in RA (Zheng et al., 2024b; Yang et al., 2020; Ye et al., 2017). Several studies have found that microRNA (miRNA) affects the biological properties of RA-FLS. Overexpression of miRNA in RA-FLS significantly inhibited RA-FLS proliferation and promoted apoptosis (Yiou et al., 2023; Hu et al., 2022; Lin et al., 2020). Notably, it has been found that silencing long noncoding RNAs (lncRNAs) nuclear paraspeckle assembly transcript 1 (NEAT1) promotes microRNA-129 and microRNA-204 inhibition of the MAPK/ERK signaling pathway and attenuates FLS synovitis in RA (Chen et al., 2021).
In addition to this, it is also important to alleviate the development of RA by down-regulating various cytokines. It was found that Phospholipase C-like 1 (PLCL1) may promote the inflammatory response in RA-FLS by regulating NLRP3 inflammatory vesicles. Silencing PLCL1 with the NLRP3 inhibitor INF39 resulted in the downregulation of cytokine levels of IL-6, IL-1β, and CXCL8, and therefore, INF39 could counteract the release of inflammatory cytokines caused by overexpression of PLCL1 (Luo et al., 2022; Shi et al., 2021). Bortezomib, a proteasome inhibitor, showed significant inhibitory and proapoptotic activity in splenocytes and FLSs in a rat model of AIA, altered the inflammatory cytokine pattern by down-regulating the expression of Toll-like receptor 2 (TLR-2), TLR-3, and TLR-4 in FLSs and reduced the invasiveness of FLSs from rats with AIA (Ospelt et al., 2008; Yannaki et al., 2010; Seibl et al., 2003). Meanwhile, 8-shogaol, a potent molecule, has been shown to have significant inhibitory effects against TNF-α, IL-1β, and IL-17 mediated inflammation and migration in RA-FLS and 3D synovial culture systems. These effects have been observed in the context of RA, where 8-shogaol has been demonstrated to reverse pathologies of the inflamed synovium by targeting TAK1 (Jo et al., 2022; Courties et al., 2010). Furthermore, fibroblast growth factor (FGF) lining FLSs is highly activated in patients with relapse RA. FGF10 knockdown by small interfering RNA in FLSs significantly reduced the expression of receptor activator of NF-κB ligand. Targeted tissue-specific inhibition of FGF10/FGFR1 may therefore provide new opportunities to treat patients with relapse RA (Meng et al., 2023; Tan et al., 2018). Besides, there are FAPα+THY1− fibroblasts selectively mediate bone and cartilage damage with little effect on inflammation, and FAPα+ THY1+ fibroblasts resulted in a more severe and persistent inflammatory arthritis, with minimal effect on bone and cartilage in RA (Croft et al., 2019). Emodin promote the secretion of STMs exosomes, which inhibit the secretion of pro-inflammatory factors by FAPα+THY1+ FLSs and promote the secretion of anti-inflammatory factors by FAPα+THY1+ FLSs, thereby inhibiting FAPα+THY1−FLS proliferation and migration inhibiting RA damage (Cheng and Rong, 2024).
Different subpopulations of STMs exist in the human synovium, and different STMs subpopulations have different homeostatic and regulatory inflammatory functions, and STM have a key role in restoring joint homeostasis (Kurowska-Stolarska and Alivernini, 2017; Alivernini et al., 2017; De Rycke et al., 2005; Koch et al., 1991). It has been found that a subpopulation of STMs, MerTK+TREM2+ and MerTK+LYVE1+, have a unique mitigating transcriptome signature and are enriched for negative regulators of inflammation. These STMs induce synovial fibroblasts to undergo repair in vitro and promote the abrogation of inflammatory lipid-mediated disorders. Thus, therapeutic enhancement of the functions of MerTK+CD206+ STM clusters by activation of MerTK with agonists, could facilitate restoration of synovial homeostasis (Huang et al., 2021b; Boutet et al., 2021; Alivernini et al., 2020; A-Gonzalez et al., 2017). At the same time, STMs form an internal immune barrier at the synovial lining and physically isolate the joint, and these barrier-forming macrophages exhibit features typical of epithelial cells, with epithelioid CX3CR1+ lining macrophages limiting the inflammatory response by providing tight junction-mediated shielding for intra-articular structures (Kurowska-Stolarska and Alivernini, 2022; Jaitin et al., 2019; Culemann et al., 2019). It has also been found that the synovial tissue macrophage ecological niche plays a key role in maintaining joint homeostasis and suppressing chronic inflammation, and that FLICE-like inhibitory protein (FLIP) is a risk locus for RA. When FLIP is absent, monocytes are regulated to shift toward pro-inflammatory STMs (Huang et al., 2021b; Huang et al., 2015; Huang et al., 2010). Recent studies have all demonstrated important heterogeneity in macrophage phenotype and function, but the specific mechanisms by which STMs subsets that might amplify the inflammatory response or promote the restoration of tissue homeostasis are unclear (You et al., 2021; Knab et al., 2022; Hannemann et al., 2021; Udalova et al., 2016).
Tumor necrosis factor (TNF) is a pleiotropic cytokine involved in many aspects of immune regulation, and anti-TNF biotherapies are considered a breakthrough in the treatment of RA (Davignon et al., 2018; Kalliolias and Ivashkiv, 2015; Feldmann and Maini, 2010). There have been many studies in the past confirming the clinical effectiveness of RA treatments targeting TNF-α (Guo et al., 2018; Mateen et al., 2016; Bjarnadottir et al., 2014; Geiler et al., 2011). TNF inhibitors, such as etanercept, infliximab, adalimumab, golimumab, and certolizumab, have been found to significantly improve treatment outcomes in RA (Jang et al., 2022; Ma and Xu, 2013). However, these conventional anti-TNF-α antibodies are somewhat immunogenicity and form resistant antibodies when used (Ishiwatari-Ogata et al., 2022; Rubbert-Roth et al., 2019). Currently, ozoralizumab, as a new generation antibody, has demonstrated low immunogenicity in the animal models used (Ishiwatari-Ogata et al., 2022), offering the possibility of early improvement of clinical symptoms, optimization of drug bioavailability, enhancement of tissue penetration and reduction of side effects. Ozoralizumab is therefore an ideal candidate for the treatment of RA patients not only at the initial stage of RA onset, but also during secondary failure of anti-TNF-α therapy (Ishiwatari-Ogata et al., 2022; Tsumoto and Takeuchi, 2024; Takeuchi et al., 2022; Keam, 2022) (Table 1).
IL-6 is a typical cytokine with pleiotropic and redundant functions (Pandolfi et al., 2020; Kang et al., 2020). Activation of the IL-6 pathway induces inhibitory molecules as well as the presence of sIL-6R and gp130 forms in the blood to regulate its signaling. Both overproduction of IL-6 and dysregulation of the IL-6 signaling pathway can contribute to the onset and progression of RA (Lee, 2018; Hirano et al., 1985). TCZ is an IL-6 inhibitor commonly used in the treatment of RA (Ogata et al., 2019; Ogata et al., 2012). Furthermore, it was shown that curcumin has been found to significantly reduce H3ac levels in the IL-6 promoter as well as IL-6 mRNA expression in RA synovial fibroblasts (RASFs) (Makuch et al., 2021; Wada et al., 2014). Studies have found that simultaneous inhibition of TNF and IL-6 improves efficacy. The combination of anti-TNF and anti-IL-6 antibodies in a mouse model of CIA resulted in sustained long-term remission, histological improvement, and effects on bone remodeling pathways (Wada et al., 2014; Phillips, 2023). Notably, the IL-6 signaling pathway attenuates TNF-α production by plasmacytoid dendritic cells (pDCs) in RA and reduces the inflammatory potential of synovial fibroblasts in RA patients. The study reveals an anti-inflammatory mechanism that can limit pDC-derived TNF-α secretion via IL-6 (Papadaki et al., 2022; Alculumbre et al., 2019; Takagi et al., 2011). In summary, IL-6 exhibits a dual role in RA, acting as both a pro-inflammatory and anti-inflammatory mediator. Therefore, the role of IL-6 in RA requires further investigation to better understand its mechanisms and implications.
An increasing number of studies have reported that the gut microbiota plays an important role in relieving RA through multiple pathways, therefore, this segment investigates whether modulation of the gut-joint axis, gut microbiota, metabolites of intestinal flora, and the intestinal barrier have an effect on relieving RA. Has been hypothesized that the disease begins in the mucosal sites and then transitions to involve the synovial joints, affecting RA through the gut-joint axis (Lin et al., 2023; Zaiss et al., 2021; Xu et al., 2020). Licorice (GC) can repair the intestinal barrier, improve the relative abundance of gut microbiota, adjusts the gut-joint axis to manage immunological imbalance in RA (Yang et al., 2024). The use of vitamin C to interfere with the gut-joint axis to improve gut microbiota imbalance and inhibit the levels of pro-inflammatory cytokines IL-6 and TNF-α associated with RA can effectively alleviate arthritis symptoms in mice (Zhang et al., 2024). Many studies have also shown that drugs can also provide relief from RA by targeting the gut microbiota. Sinomenine (SIN) can alleviate the symptoms of CIA by targeting Lactobacillus and microbial tryptophan metabolites to activate the aryl hydrocarbon receptor (AhR) and modulate the Th17/Treg balance (Jiang et al., 2023; Rosser et al., 2020). Polysaccharides from Dianbaizhu (DBZP) alleviate RA by altering the abundance of specific bacteria such as Lactobacillus and Bacteroides and a number of metabolites in a process that includes affecting the digestion and metabolism of carbohydrates and protein, altering sex hormones levels, and regulating intestinal immune function (Dong et al., 2024). Meanwhile, Saussurea involucrata oral liquid (SIOL) may play a therapeutic role in RA by improving the tricarboxylic acid (TCA) cycle through modulating the relative abundance of Lactobacillus, Romboutsia, Bacteroides and Alloprevotella (Cong et al., 2022). Tuna oil (TO) regulates gut microbiota and repairs the damaged intestinal epithelial barrier, thereby relieved arthritis severity and joint bone erosion, and ameliorated systemic inflammation (Lu et al., 2021). Tripterygium hypoglaucum Hutch (THH) extract significantly restored the dysbiosis of regulates gut C57BL/6 mice with AIA, with an increase in Bifidobacterium, Akkermansia, and Lactobacillus, and a decrease in Butyricimonas, Parabacteroides, and Anaeroplasma significantly alleviated swollen ankle, joint cavity exudation, and articular cartilage destruction in AIA mice (Zheng et al., 2023; Hu et al., 2023). Notably, prevotella histicola from human gut microbiota suppressed the development of arthritis,but the exact mechanism of action is unclear. All of these studies validate the integral role of gut flora in RA remission (Maeda and Takeda, 2017). Short-chain fatty acids (SCFAs) are metabolites from gut microbes involved in the host’s inflammatory response and immunity (Yao et al., 2022). The production of SCFAs (especially acetic acid, propionic acid and butyric acid) indeed increased significantly by the intervention of Sporidiobolus pararoseus polysaccharide (SPP). These SCFAs play an important role in contributing to the reduction of the inflammatory response and alleviation of the symptoms of RA, in maintaining the intestinal barrier function and in regulating immune homeostasis (Liao et al., 2024; Hu et al., 2021). Butyrate is used as a key intermediate linking the regulates gut to RA. Decreased butyrate alters the immune function and intestinal permeability of the intestinal mucosa, leading to a compromised intestinal barrier, where bacteria and their metabolites can enter the bloodstream and reach the distant target tissues of the host, resulting in local inflammation and aggravating arthritis (Cao et al., 2024; He et al., 2022). It has been reported that madecassoside can expansion of the richness of butyrate-producing bacteria-up-regulation of intestinal butyrate level-induction of Treg cell differentiation and IL-10 expression thereby improving arthritis symptoms (Qiao et al., 2020). Moreover, exogenous acetate was observed to reverse CIA mice with exacerbated gut microbial disruption, thereby further confirming the crucial role of the effect of gut microbial metabolite acetate on neutrophils in vivo in immune regulation (Jin et al., 2023; Balakrishnan et al., 2021).
Persistent arthritic pain following the resolution of joint inflammation is a common and challenging issue in RA. Despite effective control of inflammation through disease-modifying antirheumatic drugs (DMARDs) or biologic therapies, a large proportion of RA patients have persistent moderate to high pain in a state of inflammatory remission, which significantly impacts their quality of life (McWilliams et al., 2019). A study has revealed that over half of patients experienced severe pain at the outset of, or during, the transition from one biological DMARD (e.g., Adalimumab, Tocilizumab, Rituximab, Abatacept and Anakinra) or targeted synthetic DMARD (e.g., Tofacitinib, Baricitinib and Upadacitinib) to another (Kim et al., 2022b; Vergne-Salle et al., 2020). Pain progression is heterogeneous in people with RA. Persistent arthritic pain may be caused by a variety of underlying factors, including systemic cytokine, simple mechanical stimulations, and increase neuronal innervation or sensitize peripheral nociceptors at the joint site, but the exact mechanisms are unknown (Kim et al., 2022b; Lim, 2022; Lee et al., 2014). Specifically, chronic inflammation in RA can lead to long-term changes in the central nervous system (CNS), resulting in heightened pain sensitivity (hyperalgesia) and pain from non-painful stimuli (allodynia) (Sağ et al., 2020; Lee et al., 2011). Inflammatory mediators such as cytokines, growth factors and chemokines and immune cells can cause direct damage to peripheral nerves, leading to neuropathic pain (Asbury and Fields, 1984; McWilliams and Walsh, 2017). Even after inflammation resolves, structural damage (e.g., cartilage loss, bone erosion, and joint deformity) can persist, causing mechanical pain (McWilliams et al., 2012). Residual inflammation may persist in joints or surrounding tissues, even when clinical markers (e.g., CRP, ESR) and imaging appear normal (McWilliams and Walsh, 2017). Chronic pain in RA is often associated with psychological comorbidities such as depression, anxiety, and catastrophizing, which can amplify pain perception and disability (McWilliams et al., 2012; Odegård et al., 2007).
Interestingly a few patients displayed superior pain-reducing ability with Janus kinase (Jak) inhibitors and baricitinib monotherapy (Kim et al., 2022b; Lim, 2022). It has also been found that neuroinflammation within the dorsal root ganglion (DRG) may upregulate FcγRI (a key immune receptor) signaling after regression of joint inflammation in a mouse model of collagen antibody-induced arthritis (CAIA), and that knocking down the expression of Fcgr1 in the DRG produces a similar analgesic effect and reduces persistent pain (Liu et al., 2022). The persistence of pain and discomfort during the remission period represents a significant challenge. To enhance the alleviation of patients’ suffering and improve their quality of life, it is imperative to continue investigating the specific mechanisms of remission (Kim et al., 2022b).
RA causes severe joint pain, swelling, and stiffness with many types of complications and persistent pain after remission poses a significant health burden to the patient. Previously, glucocorticosteroids or NSAIDs were commonly used, which at best slowed joint damage and disability at the cost of significant toxicity. In contrast, contemporary therapeutic strategies aim to induce remission of RA and prevent damage before it occurs. Therefore, this paper summarizes and discusses the relevant inflammatory mechanisms of remission in RA, and concludes that remission of RA can be achieved by blocking T-cell activation, modulating macrophage polarization status, modulating FLS signaling pathways, modulating STMs subpopulations, and inhibiting relevant inflammatory factors. An in-depth understanding of the complex mechanisms of disease remission can help develop new therapies that more precisely target disease-causing molecules. On the other hand, the presence of persistent arthritic pain and discomfort after RA remission is still a problem, so further research on the specific mechanisms of remission can also help to better alleviate patients suffering and improve their quality of life.
Besides these above-mentioned targets and drugs, there are classical and supportive RA drugs. Methotrexate (MTX) is a cornerstone drug in the treatment of RA, primarily functioning through multiple mechanisms: inhibiting the production of pro-inflammatory cytokines, modulating the Th1/Th2 balance, and suppressing the proliferation of immune cells like T and B cells. Curcumin and quercetin are two natural compounds that have been studied for their potential benefits in managing RA, while they are not a replacement for conventional RA treatments (e.g., DMARDs). Vitamin supplementation can play a supportive role in RA management by addressing nutrient deficiencies, reducing inflammation, and protecting joint and bone health. Key vitamins like D, C, E, B6, B9, and K have shown promise in improving RA outcomes. However, supplementation should be tailored to individual needs and used in conjunction with conventional RA therapies under medical guidance. Probiotics represent a promising complementary therapy for RA, with potential benefits in immune modulation, inflammation reduction, and gut health improvement. Classical and supportive RA drugs are more affordable due to their relative low price and higher medical insurance cover, while biological DMARDs are expensive and less insurance covered. To get a better management of RA, a personalized combination of these drugs is recommended.
Although there are already many potential targets and available drugs for current RA treatment. Novel targets and treatment strategies are still required to meet the low response and huge side effects under current treatment. Here are some possible future research directions for RA treatment: precision medicine and personalized therapy with specific biomarkers and genetic variations and/or epigenetic modifications, development of novel targets and mechanisms of RA, new drug formats (bispecific antibodies, improve targeting and reduce side effects), and most of all combination therapy of kinds targets and drugs.
XY: Writing–original draft. DR: Writing–original draft. QC: Conceptualization, Writing–original draft. JS: Supervision, Writing–review and editing. BW: Writing–review and editing. SW: Funding acquisition, Writing–review and editing. HZ: Funding acquisition, Writing–review and editing.
The author(s) declare that financial support was received for the research and/or publication of this article. HZ is supported by the National Natural Science Foundation of China (82402072), the Zhejiang Provincial Natural Science Foundation of China under Grant No. QN25H100002, the Medical School Joint Innovation Open Fund of Lishui university (X111015). BW was supported by the Medicine and Health Technology Funding Project of Zhejiang (2022RC303; 2025KY514), and Lishui Technology Plan Project (2023SJZC060; 2023GYX04; 2024SJZC133). SW is supported by the National Natural Science Foundation of China (81570013).
The authors declare that they have no known competing financial interests or personal relationships that could have appeared to influence the work reported in this paper.
The authors declare that the research was conducted in the absence of any commercial or financial relationships that could be construed as a potential conflict of interest.
The author(s) declare that no Generative AI was used in the creation of this manuscript.
All claims expressed in this article are solely those of the authors and do not necessarily represent those of their affiliated organizations, or those of the publisher, the editors and the reviewers. Any product that may be evaluated in this article, or claim that may be made by its manufacturer, is not guaranteed or endorsed by the publisher.
A-Gonzalez, N., Quintana, J. A., García-Silva, S., Mazariegos, M., González de la Aleja, A., Nicolás-Ávila, J. A., et al. (2017). Phagocytosis imprints heterogeneity in tissue-resident macrophages. J. Exp. Med. 214, 1281–1296. doi:10.1084/jem.20161375
Alculumbre, S., Raieli, S., Hoffmann, C., Chelbi, R., Danlos, F.-X., and Soumelis, V. (2019). Plasmacytoid pre-dendritic cells (pDC): from molecular pathways to function and disease association. Seminars Cell and Dev. Biol. 86, 24–35. doi:10.1016/j.semcdb.2018.02.014
Aldridge, J., Andersson, K., Gjertsson, I., Hultgård Ekwall, A.-K., Hallström, M., van Vollenhoven, R., et al. (2022). Blood PD-1+TFh and CTLA-4+CD4+ T cells predict remission after CTLA-4Ig treatment in early rheumatoid arthritis. Rheumatology 61, 1233–1242. doi:10.1093/rheumatology/keab454
Alivernini, S., MacDonald, L., Elmesmari, A., Finlay, S., Tolusso, B., Gigante, M. R., et al. (2020). Distinct synovial tissue macrophage subsets regulate inflammation and remission in rheumatoid arthritis. Nat. Med. 26, 1295–1306. doi:10.1038/s41591-020-0939-8
Alivernini, S., Tolusso, B., Petricca, L., Bui, L., Di Sante, G., Peluso, G., et al. (2017). Synovial features of patients with rheumatoid arthritis and psoriatic arthritis in clinical and ultrasound remission differ under anti-TNF therapy: a clue to interpret different chances of relapse after clinical remission? Ann. Rheumatic Dis. 76, 1228–1236. doi:10.1136/annrheumdis-2016-210424
Ando, K., Kanazawa, S., Tetsuka, T., Ohta, S., Jiang, X., Tada, T., et al. (2003). Induction of Notch signaling by tumor necrosis factor in rheumatoid synovial fibroblasts. Oncogene 22, 7796–7803. doi:10.1038/sj.onc.1206965
Asbury, A. K., and Fields, H. L. (1984). Pain due to peripheral nerve damage: an hypothesis. Neurology 34, 1587. doi:10.1212/wnl.34.12.1587
Baito, Q. N., Jaafar, H. M., and Mohammad, T. A. M. (2023). Piperine suppresses inflammatory fibroblast-like synoviocytes derived from rheumatoid arthritis patients via NF-κB inhibition. Cell. Immunol. 391-392, 104752. doi:10.1016/j.cellimm.2023.104752
Balakrishnan, B., Luckey, D., Bodhke, R., Chen, J., Marietta, E., Jeraldo, P., et al. (2021). Prevotella histicola protects from arthritis by expansion of allobaculum and augmenting butyrate production in humanized mice. Front. Immunol. 12, 609644. doi:10.3389/fimmu.2021.609644
Bartok, B., and Firestein, G. S. (2009). Fibroblast-like synoviocytes: key effector cells in rheumatoid arthritis. Immunol. Rev. 233, 233–255. doi:10.1111/j.0105-2896.2009.00859.x
Bjarnadottir, U., Lemarquis, A. L., Halldorsdottir, S., Freysdottir, J., and Ludviksson, B. R. (2014). The suppressive function of human CD8+ iTregs is inhibited by IL-1β and TNFα. Scand. J. Immunol. 80, 313–322. doi:10.1111/sji.12212
Boutet, M.-A., Courties, G., Nerviani, A., Le Goff, B., Apparailly, F., Pitzalis, C., et al. (2021). Novel insights into macrophage diversity in rheumatoid arthritis synovium. Autoimmun. Rev. 20, 102758. doi:10.1016/j.autrev.2021.102758
Brown, P., Pratt, A. G., and Hyrich, K. L. (2024). Therapeutic advances in rheumatoid arthritis. Bmj, e070856. doi:10.1136/bmj-2022-070856
Byng-Maddick, R., and Ehrenstein, M. R. (2015). The impact of biological therapy on regulatory T cells in rheumatoid arthritis. Rheumatology 54, 768–775. doi:10.1093/rheumatology/keu487
Cai, H., Zhang, J., Xu, H., Sun, W., Wu, W., Dong, C., et al. (2024). ALOX5 drives the pyroptosis of CD4 + T cells and tissue inflammation in rheumatoid arthritis. Sci. Signal. 17, eadh1178. doi:10.1126/scisignal.adh1178
Cao, Y., Chen, J., Xiao, J., Hong, Y., Xu, K., and Zhu, Y. (2024). Butyrate: a bridge between intestinal flora and rheumatoid arthritis. Front. Immunol. 15, 1475529. doi:10.3389/fimmu.2024.1475529
Chatzidionysiou, K., Circiumaru, A., Rethi, B., Joshua, V., Engstrom, M., Hensvold, A., et al. (2021). Tocilizumab decreases T cells but not macrophages in the synovium of patients with rheumatoid arthritis while it increases the levels of serum interleukin-6 and RANKL. RMD Open 7, e001662. doi:10.1136/rmdopen-2021-001662
Chen, J., Luo, X., Liu, M., Peng, L., Zhao, Z., He, C., et al. (2021). Silencing long non-coding RNA NEAT1 attenuates rheumatoid arthritis via the MAPK/ERK signalling pathway by downregulating microRNA-129 and microRNA-204. RNA Biol. 18, 657–668. doi:10.1080/15476286.2020.1857941
Chen, L., Zhou, Q., Fang, X., Xu, Q., Zou, Y., and Zhang, J. (2024). Administration of liposomal-based Pde3b gene therapy protects mice against collagen-induced rheumatoid arthritis via modulating macrophage polarization. Int. J. Nanomedicine 19, 4411–4427. doi:10.2147/IJN.S454445
Chen, S., Wang, J., Wang, J., Jia, X., Xuan, Z., Cheng, Z., et al. (2023). Wnt/β-catenin signaling pathway promotes abnormal activation of fibroblast-like synoviocytes and angiogenesis in rheumatoid arthritis and the intervention of Er Miao San. Phytomedicine 120, 155064. doi:10.1016/j.phymed.2023.155064
Cheng, L., and Rong, X. (2024). Emodin promotes the recovery of rheumatoid arthritis by regulating the crosstalk between macrophage subsets and synovial fibroblast subsets. Animal Models Exp. Med. 8, 44–56. doi:10.1002/ame2.12387
Cheng, Y., Si, Y., Wang, L., Ding, M., Yu, S., Lu, L., et al. (2021). The regulation of macrophage polarization by hypoxia-PADI4 coordination in Rheumatoid arthritis. Int. Immunopharmacol. 99, 107988. doi:10.1016/j.intimp.2021.107988
Collu, G. M., Hidalgo-Sastre, A., and Brennan, K. (2014). Wnt–Notch signalling crosstalk in development and disease. Cell. Mol. Life Sci. 71, 3553–3567. doi:10.1007/s00018-014-1644-x
Cong, S., Wang, L., Meng, Y., Cai, X., Zhang, C., Gu, Y., et al. (2022). Saussurea involucrata oral liquid regulates gut microbiota and serum metabolism during alleviation of collagen-induced arthritis in rats. Phytotherapy Res. 37, 1242–1259. doi:10.1002/ptr.7681
Conrad, N., Misra, S., Verbakel, J. Y., Verbeke, G., Molenberghs, G., Taylor, P. N., et al. (2023). Incidence, prevalence, and co-occurrence of autoimmune disorders over time and by age, sex, and socioeconomic status: a population-based cohort study of 22 million individuals in the UK. Lancet 401, 1878–1890. doi:10.1016/S0140-6736(23)00457-9
Courties, G., Seiffart, V., Presumey, J., Escriou, V., Scherman, D., Zwerina, J., et al. (2010). In vivo RNAi-mediated silencing of TAK1 decreases inflammatory Th1 and Th17 cells through targeting of myeloid cells. Blood 116, 3505–3516. doi:10.1182/blood-2010-02-269605
Croft, A. P., Campos, J., Jansen, K., Turner, J. D., Marshall, J., Attar, M., et al. (2019). Distinct fibroblast subsets drive inflammation and damage in arthritis. Nature 570, 246–251. doi:10.1038/s41586-019-1263-7
Culemann, S., Grüneboom, A., Nicolás-Ávila, J. Á., Weidner, D., Lämmle, K. F., Rothe, T., et al. (2019). Locally renewing resident synovial macrophages provide a protective barrier for the joint. Nature 572, 670–675. doi:10.1038/s41586-019-1471-1
Cutolo, M., Campitiello, R., Gotelli, E., and Soldano, S. (2022). The role of M1/M2 macrophage polarization in rheumatoid arthritis synovitis. Front. Immunol. 13, 867260. doi:10.3389/fimmu.2022.867260
Davignon, J.-L., Rauwel, B., Degboé, Y., Constantin, A., Boyer, J.-F., Kruglov, A., et al. (2018). Modulation of T-cell responses by anti-tumor necrosis factor treatments in rheumatoid arthritis: a review. Arthritis Res. and Ther. 20, 229. doi:10.1186/s13075-018-1725-6
Deng, C., Zhang, Q., He, P., Zhou, B., He, K., Sun, X., et al. (2021). Targeted apoptosis of macrophages and osteoclasts in arthritic joints is effective against advanced inflammatory arthritis. Nat. Commun. 12, 2174. doi:10.1038/s41467-021-22454-z
De Rycke, L., Baeten, D., Foell, D., Kruithof, E., Veys, E. M., Roth, J., et al. (2005). Differential expression and response to anti-TNFα treatment of infiltrating versus resident tissue macrophage subsets in autoimmune arthritis. J. Pathology 206, 17–27. doi:10.1002/path.1758
Diarra, D., Stolina, M., Polzer, K., Zwerina, J., Ominsky, M. S., Dwyer, D., et al. (2007). Dickkopf-1 is a master regulator of joint remodeling. Nat. Med. 13, 156–163. doi:10.1038/nm1538
Ding, Q., Hu, W., Wang, R., Yang, Q., Zhu, M., Li, M., et al. (2023). Signaling pathways in rheumatoid arthritis: implications for targeted therapy. Signal Transduct. Target. Ther. 8, 68. doi:10.1038/s41392-023-01331-9
Donado, C. A., Theisen, E., Zhang, F., Nathan, A., Fairfield, M. L., Rupani, K. V., et al. (2025). Granzyme K activates the entire complement cascade. Nature. doi:10.1038/s41586-025-08713-9
Dong, Y., Wang, Y., Zhang, F., Ma, J., Li, M., Liu, W., et al. (2024). Polysaccharides from Gaultheria leucocarpa var. yunnanensis (DBZP) alleviates rheumatoid arthritis through ameliorating gut microbiota. Int. J. Biol. Macromol. 281, 136250. doi:10.1016/j.ijbiomac.2024.136250
Ekwall, A.-K. H., Eisler, T., Anderberg, C., Jin, C., Karlsson, N., Brisslert, M., et al. (2011). The tumour-associated glycoprotein podoplanin is expressed in fibroblast-like synoviocytes of the hyperplastic synovial lining layer in rheumatoid arthritis. Arthritis Res. and Ther. 13, R40. doi:10.1186/ar3274
Elia, D., Madhala, D., Ardon, E., Reshef, R., and Halevy, O. (2007). Sonic hedgehog promotes proliferation and differentiation of adult muscle cells: involvement of MAPK/ERK and PI3K/Akt pathways. Biochimica Biophysica Acta (BBA) - Mol. Cell Res. 1773, 1438–1446. doi:10.1016/j.bbamcr.2007.06.006
Farh, K.K.-H., Marson, A., Zhu, J., Kleinewietfeld, M., Housley, W. J., Beik, S., et al. (2014). Genetic and epigenetic fine mapping of causal autoimmune disease variants. Nature 518, 337–343. doi:10.1038/nature13835
Feldmann, M., and Maini, R. N. (2010). Anti-TNF therapy, from rationale to standard of care: what lessons has it taught us? J. Immunol. 185, 791–794. doi:10.4049/jimmunol.1090051
Feng, Z., Meng, F., Huo, F., Zhu, Y., Qin, Y., Gui, Y., et al. (2024). Inhibition of ferroptosis rescues M2 macrophages and alleviates arthritis by suppressing the HMGB1/TLR4/STAT3 axis in M1 macrophages. Redox Biol. 75, 103255. doi:10.1016/j.redox.2024.103255
Firestein, G. S., and McInnes, I. B. (2017). Immunopathogenesis of rheumatoid arthritis. Immunity 46, 183–196. doi:10.1016/j.immuni.2017.02.006
Gagliardi, P. A., di Blasio, L., and Primo, L. (2015). PDK1: a signaling hub for cell migration and tumor invasion. Biochimica Biophysica Acta (BBA) - Rev. Cancer 1856, 178–188. doi:10.1016/j.bbcan.2015.07.003
Garcia-Carbonell, R., Divakaruni, A. S., Lodi, A., Vicente-Suarez, I., Saha, A., Cheroutre, H., et al. (2016). Critical role of glucose metabolism in rheumatoid arthritis fibroblast-like synoviocytes. Arthritis and Rheumatology 68, 1614–1626. doi:10.1002/art.39608
Geiler, J., Buch, M., and McDermott, M. F. (2011). Anti-TNF treatment in rheumatoid arthritis. Curr. Pharm. Des. 17, 3141–3154. doi:10.2174/138161211798157658
Giles, J. T. (2019). Extra-articular manifestations and comorbidity in rheumatoid arthritis: potential impact of pre–rheumatoid arthritis prevention. Clin. Ther. 41, 1246–1255. doi:10.1016/j.clinthera.2019.04.018
Guo, Q., Wang, Y., Xu, D., Nossent, J., Pavlos, N. J., and Xu, J. (2018). Rheumatoid arthritis: pathological mechanisms and modern pharmacologic therapies. Bone Res. 6, 15. doi:10.1038/s41413-018-0016-9
Hannemann, N., Apparailly, F., and Courties, G. (2021). New insights into macrophage heterogeneity in rheumatoid arthritis. Jt. Bone Spine 88, 105091. doi:10.1016/j.jbspin.2020.105091
Hartemann, A., Bensimon, G., Payan, C. A., Jacqueminet, S., Bourron, O., Nicolas, N., et al. (2013). Low-dose interleukin 2 in patients with type 1 diabetes: a phase 1/2 randomised, double-blind, placebo-controlled trial. Lancet Diabetes and Endocrinol. 1, 295–305. doi:10.1016/S2213-8587(13)70113-X
He, J., Chu, Y., Li, J., Meng, Q., Liu, Y., Jin, J., et al. (2022). Intestinal butyrate-metabolizing species contribute to autoantibody production and bone erosion in rheumatoid arthritis. Sci. Adv. 8, eabm1511. doi:10.1126/sciadv.abm1511
Hirano, T., Taga, T., Nakano, N., Yasukawa, K., Kashiwamura, S., Shimizu, K., et al. (1985). Purification to homogeneity and characterization of human B-cell differentiation factor (BCDF or BSFp-2). Proc. Natl. Acad. Sci. 82, 5490–5494. doi:10.1073/pnas.82.16.5490
Hu, B., Liu, C., Jiang, W., Zhu, H., Zhang, H., Qian, H., et al. (2021). Chronic in vitro fermentation and in vivo metabolism: extracellular polysaccharides from Sporidiobolus pararoseus regulate the intestinal microbiome of humans and mice. Int. J. Biol. Macromol. 192, 398–406. doi:10.1016/j.ijbiomac.2021.09.127
Hu, J., Ni, J., Zheng, J., Guo, Y., Yang, Y., Ye, C., et al. (2023). Tripterygium hypoglaucum extract ameliorates adjuvant-induced arthritis in mice through the gut microbiota. Chin. J. Nat. Med. 21, 730–744. doi:10.1016/s1875-5364(23)60466-2
Hu, X., Kim, H., Stahl, E., Plenge, R., Daly, M., and Raychaudhuri, S. (2011). Integrating autoimmune risk loci with gene-expression data identifies specific pathogenic immune cell subsets. Am. J. Hum. Genet. 89, 682–506. doi:10.1016/j.ajhg.2011.10.010
Hu, X.-X., Wu, Y.-j., Zhang, J., and Wei, W. (2019). T-cells interact with B cells, dendritic cells, and fibroblast-like synoviocytes as hub-like key cells in rheumatoid arthritis. Int. Immunopharmacol. 70, 428–434. doi:10.1016/j.intimp.2019.03.008
Hu, Z., Chen, J., Wang, M., Weng, W., Chen, Y., and Pan, Y. (2022). Rheumatoid arthritis fibroblast-like synoviocytes maintain tumor-like biological characteristics through ciRS-7-dependent regulation of miR-7. Mol. Biol. Rep. 49, 8473–8483. doi:10.1007/s11033-022-07666-w
Hu, Z., Li, Y., Zhang, L., Jiang, Y., Long, C., Yang, Q., et al. (2024). Metabolic changes in fibroblast-like synoviocytes in rheumatoid arthritis: state of the art review. Front. Immunol. 15, 1250884. doi:10.3389/fimmu.2024.1250884
Huang, P., Yan, R., Zhang, X., Wang, L., Ke, X., and Qu, Y. (2019). Activating Wnt/β-catenin signaling pathway for disease therapy: challenges and opportunities. Pharmacol. and Ther. 196, 79–90. doi:10.1016/j.pharmthera.2018.11.008
Huang, Q.-Q., Doyle, R., Chen, S.-Y., Sheng, Q., Misharin, A. V., Mao, Q., et al. (2021b). Critical role of synovial tissue–resident macrophage niche in joint homeostasis and suppression of chronic inflammation. Sci. Adv. 7. doi:10.1126/sciadv.abd0515
Huang, Q.-Q., Perlman, H., Birkett, R., Doyle, R., Fang, D., Haines, G. K., et al. (2015). CD11c-mediated deletion of Flip promotes autoreactivity and inflammatory arthritis. Nat. Commun. 6, 7086. doi:10.1038/ncomms8086
Huang, Q.-Q., Perlman, H., Huang, Z., Birkett, R., Kan, L., Agrawal, H., et al. (2010). FLIP: a novel regulator of macrophage differentiation and granulocyte homeostasis. Blood 116, 4968–4977. doi:10.1182/blood-2009-11-252841
Huang, Y. S., Tseng, W. Y., Clanchy, F. I. L., Topping, L. M., Ogbechi, J., McNamee, K., et al. (2021a). Pharmacological modulation of T cell immunity results in long-term remission of autoimmune arthritis, 118. Proceedings of the National Academy of Sciences.
Inamo, J., Suzuki, K., Takeshita, M., Kondo, Y., Okuzono, Y., Koga, K., et al. (2021). Molecular remission at T cell level in patients with rheumatoid arthritis. Sci. Rep. 11, 16691. doi:10.1038/s41598-021-96300-z
Ishiwatari-Ogata, C., Kyuuma, M., Ogata, H., Yamakawa, M., Iwata, K., Ochi, M., et al. (2022). Ozoralizumab, a humanized anti-tnfα NANOBODY® compound, exhibits efficacy not only at the onset of arthritis in a human TNF transgenic mouse but also during secondary failure of administration of an anti-tnfα IgG. Front. Immunol. 13. doi:10.3389/fimmu.2022.853008
Jaitin, D. A., Adlung, L., Thaiss, C. A., Weiner, A., Li, B., Descamps, H., et al. (2019). Lipid-associated macrophages control metabolic homeostasis in a trem2-dependent manner. Cell 178, 686–698.e14. doi:10.1016/j.cell.2019.05.054
Jang, S., Kwon, E.-J., and Lee, J. J. (2022). Rheumatoid arthritis: pathogenic roles of diverse immune cells. Int. J. Mol. Sci. 23, 905. doi:10.3390/ijms23020905
Jiang, P., Wei, K., Chang, C., Zhao, J., Zhang, R., Xu, L., et al. (2022). SFRP1 negatively modulates pyroptosis of fibroblast-like synoviocytes in rheumatoid arthritis: a review. Front. Immunol. 13, 903475. doi:10.3389/fimmu.2022.903475
Jiang, Z.-M., Zeng, S.-L., Huang, T.-Q., Lin, Y., Wang, F.-F., Gao, X.-J., et al. (2023). Sinomenine ameliorates rheumatoid arthritis by modulating tryptophan metabolism and activating aryl hydrocarbon receptor via gut microbiota regulation. Sci. Bull. 68, 1540–1555. doi:10.1016/j.scib.2023.06.027
Jin, L., Zhang, Z., Pan, P., Zhao, Y., Zhou, M., Liu, L., et al. (2023). Low-dose ethanol consumption inhibits neutrophil extracellular traps formation to alleviate rheumatoid arthritis. Commun. Biol. 6, 1088. doi:10.1038/s42003-023-05473-y
Jo, S., Samarpita, S., Lee, J. S., Lee, Y. J., Son, J. E., Jeong, M., et al. (2022). 8-Shogaol inhibits rheumatoid arthritis through targeting TAK1. Pharmacol. Res. 178, 106176. doi:10.1016/j.phrs.2022.106176
Jonsson, A. H., Zhang, F., Dunlap, G., Gomez-Rivas, E., Watts, G. F. M., Faust, H. J., et al. (2022). Granzyme K(+) CD8 T cells form a core population in inflamed human tissue. Sci. Transl. Med. 14, eabo0686. doi:10.1126/scitranslmed.abo0686
Kalliolias, G. D., and Ivashkiv, L. B. (2015). TNF biology, pathogenic mechanisms and emerging therapeutic strategies. Nat. Rev. Rheumatol. 12, 49–62. doi:10.1038/nrrheum.2015.169
Kang, S., Narazaki, M., Metwally, H., and Kishimoto, T. (2020). Historical overview of the interleukin-6 family cytokine. J. Exp. Med. 217, e20190347. doi:10.1084/jem.20190347
Kim, H., Back, J. H., Han, G., Lee, S. J., Park, Y. E., Gu, M. B., et al. (2022a). Extracellular vesicle-guided in situ reprogramming of synovial macrophages for the treatment of rheumatoid arthritis. Biomaterials 286, 121578. doi:10.1016/j.biomaterials.2022.121578
Kim, H.-A., Park, S. Y., and Shin, K. (2022b). Implications of persistent pain in patients with rheumatoid arthritis despite remission status: data from the KOBIO registry. J. Rheumatic Dis. 29, 215–222. doi:10.4078/jrd.22.0005
Klareskog, L., Stolt, P., Lundberg, K., Källberg, H., Bengtsson, C., Grunewald, J., et al. (2005). A new model for an etiology of rheumatoid arthritis: smoking may trigger HLA–DR (shared epitope)–restricted immune reactions to autoantigens modified by citrullination. Arthritis and Rheumatism 54, 38–46. doi:10.1002/art.21575
Knab, K., Chambers, D., and Krönke, G. (2022). Synovial macrophage and fibroblast heterogeneity in joint homeostasis and inflammation. Front. Med. 9, 862161. doi:10.3389/fmed.2022.862161
Koch, A. E., Burrows, J. C., Skoutelis, A., Marder, R., Domer, P. H., Anderson, B., et al. (1991). Monoclonal antibodies detect monocyte/macrophage activation and differentiation antigens and identify functionally distinct subpopulations of human rheumatoid synovial tissue macrophages. Am. J. Pathol. 138, 165–173.
Kurowska-Stolarska, M., and Alivernini, S. (2017). Synovial tissue macrophages: friend or foe? RMD Open 3, e000527. doi:10.1136/rmdopen-2017-000527
Kurowska-Stolarska, M., and Alivernini, S. (2022). Synovial tissue macrophages in joint homeostasis, rheumatoid arthritis and disease remission. Nat. Rev. Rheumatol. 18, 384–397. doi:10.1038/s41584-022-00790-8
Lee, G. R. (2018). The balance of Th17 versus Treg cells in autoimmunity. Int. J. Mol. Sci. 19, 730. doi:10.3390/ijms19030730
Lee, Y. C., Frits, M. L., Iannaccone, C. K., Weinblatt, M. E., Shadick, N. A., Williams, D. A., et al. (2014). Subgrouping of patients with rheumatoid arthritis based on pain, fatigue, inflammation, and psychosocial factors. Arthritis and Rheumatology 66, 2006–2014. doi:10.1002/art.38682
Lee, Y. C., Nassikas, N. J., and Clauw, D. J. (2011). The role of the central nervous system in the generation and maintenance of chronic pain in rheumatoid arthritis, osteoarthritis and fibromyalgia. Arthritis Res. and Ther. 13, 1–10.
Li, G., Zhang, Y., Qian, Y., Zhang, H., Guo, S., Sunagawa, M., et al. (2013). Interleukin-17A promotes rheumatoid arthritis synoviocytes migration and invasion under hypoxia by increasing MMP2 and MMP9 expression through NF-κB/HIF-1α pathway. Mol. Immunol. 53, 227–236. doi:10.1016/j.molimm.2012.08.018
Li, H., Feng, Y., Zheng, X., Jia, M., Mei, Z., Wang, Y., et al. (2022). M2-type exosomes nanoparticles for rheumatoid arthritis therapy via macrophage re-polarization. J. Control. Release 341, 16–30. doi:10.1016/j.jconrel.2021.11.019
Li, Q., Chen, Y., Liu, H., Tian, Y., Yin, G., and Xie, Q. (2023). Targeting glycolytic pathway in fibroblast-like synoviocytes for rheumatoid arthritis therapy: challenges and opportunities. Inflamm. Res. 72, 2155–2167. doi:10.1007/s00011-023-01807-y
Liao, T., Shen, F., Zhu, H., Mu, W., Qian, H., and Liu, Y. (2024). Extracellular polysaccharides from Sporidiobolus pararoseus alleviates rheumatoid through ameliorating gut barrier function and gut microbiota. Int. J. Biol. Macromol. 260, 129436. doi:10.1016/j.ijbiomac.2024.129436
Lim, D.-H. (2022). A challenging target: persistent pain during the remission state in rheumatoid arthritis patients. J. Rheumatic Dis. 30, 1–2. doi:10.4078/jrd.22.0047
Lin, L., Zhang, K., Xiong, Q., Zhang, J., Cai, B., Huang, Z., et al. (2023). Gut microbiota in pre-clinical rheumatoid arthritis: from pathogenesis to preventing progression. J. Autoimmun. 141, 103001. doi:10.1016/j.jaut.2023.103001
Lin, S., Wang, S., Zhang, Z., Lu, Y., Yang, M., Chen, P., et al. (2020). MiRNA-6089 inhibits rheumatoid arthritis fibroblast-like synoviocytes proliferation and induces apoptosis by targeting CCR4. Archives Physiology Biochem. 128, 1426–1433. doi:10.1080/13813455.2020.1773862
Liu, F., Feng, X. X., Zhu, S. L., Huang, H. Y., Chen, Y. D., Pan, Y. F., et al. (2018). Sonic hedgehog signaling pathway mediates proliferation and migration of fibroblast-like synoviocytes in rheumatoid arthritis via MAPK/ERK signaling pathway. Front. Immunol. 9, 2847. doi:10.3389/fimmu.2018.02847
Liu, Y., Caterina, M. J., and Qu, L. (2022). Sensory neuron expressed FcγRI mediates postinflammatory arthritis pain in female mice. Front. Immunol. 13. doi:10.3389/fimmu.2022.889286
Lu, C., Chen, J., Yi, C., Han, J., Shi, Q., Li, J., et al. (2021). Gut microbiota mediated the protective effects of tuna oil on collagen-induced arthritis in mice. Food and Funct. 12, 5387–5398.
Luo, S., Li, X.-F., Yang, Y.-L., Song, B., Wu, S., Niu, X.-N., et al. (2022). PLCL1 regulates fibroblast-like synoviocytes inflammation via NLRP3 inflammasomes in rheumatoid arthritis. Adv. Rheumatology 62, 25. doi:10.1186/s42358-022-00252-5
Ma, J.-D., Jing, J., Wang, J.-W., Yan, T., Li, Q.-H., Mo, Y.-Q., et al. (2019). A novel function of artesunate on inhibiting migration and invasion of fibroblast-like synoviocytes from rheumatoid arthritis patients. Arthritis Res. and Ther. 21, 153. doi:10.1186/s13075-019-1935-6
Ma, X., and Xu, S. (2013). TNF inhibitor therapy for rheumatoid arthritis. Biomed. Rep. 1, 177–184. doi:10.3892/br.2012.42
Maeda, Y., Kurakawa, T., Umemoto, E., Motooka, D., Ito, Y., Gotoh, K., et al. (2016). Dysbiosis contributes to arthritis development via activation of autoreactive T cells in the intestine. Arthritis and Rheumatology 68, 2646–2661. doi:10.1002/art.39783
Maeda, Y., and Takeda, K. (2017). Role of gut microbiota in rheumatoid arthritis. J. Clin. Med. 6, 60. doi:10.3390/jcm6060060
Makuch, S., Więcek, K., and Woźniak, M. (2021). The immunomodulatory and anti-inflammatory effect of curcumin on immune cell populations, cytokines, and in vivo models of rheumatoid arthritis. Pharmaceuticals 14, 309. doi:10.3390/ph14040309
Malek, T. R. (2008). The biology of interleukin-2. Annu. Rev. Immunol. 26, 453–479. doi:10.1146/annurev.immunol.26.021607.090357
Malmström, V., Catrina, A. I., and Klareskog, L. (2016). The immunopathogenesis of seropositive rheumatoid arthritis: from triggering to targeting. Nat. Rev. Immunol. 17, 60–75. doi:10.1038/nri.2016.124
Masoumi, M., Mehrabzadeh, M., Mahmoudzehi, S., Mousavi, M. J., Jamalzehi, S., Sahebkar, A., et al. (2020). Role of glucose metabolism in aggressive phenotype of fibroblast-like synoviocytes: latest evidence and therapeutic approaches in rheumatoid arthritis. Int. Immunopharmacol. 89, 107064. doi:10.1016/j.intimp.2020.107064
Mateen, S., Zafar, A., Moin, S., Khan, A. Q., and Zubair, S. (2016). Understanding the role of cytokines in the pathogenesis of rheumatoid arthritis. Clin. Chim. Acta 455, 161–171. doi:10.1016/j.cca.2016.02.010
McInnes, I. B., and Schett, G. (2011). The pathogenesis of rheumatoid arthritis. N. Engl. J. Med. 365, 2205–2219. doi:10.1056/NEJMra1004965
McInnes, I. B., and Schett, G. (2017). Pathogenetic insights from the treatment of rheumatoid arthritis. Lancet 389, 2328–2337. doi:10.1016/S0140-6736(17)31472-1
McWilliams, D. F., Dawson, O., Young, A., Kiely, P. D., Ferguson, E., and Walsh, D. A. (2019). Discrete trajectories of resolving and persistent pain in people with rheumatoid arthritis despite undergoing treatment for inflammation: results from three UK cohorts. J. Pain 20, 716–727. doi:10.1016/j.jpain.2019.01.001
McWilliams, D. F., and Walsh, D. A. (2017). Pain mechanisms in rheumatoid arthritis. Clin. Exp. Rheumatol. 35, 94–101.
McWilliams, D. F., Zhang, W., Mansell, J. S., Kiely, P. D., Young, A., and Walsh, D. A. (2012). Predictors of change in bodily pain in early rheumatoid arthritis: an inception cohort study. Arthritis Care Res. Hob. 64, 1505–1513. doi:10.1002/acr.21723
Meng, X., Chen, Z., Li, T., Nie, Z., Han, H., Zhong, S., et al. (2023). Role and therapeutic potential for targeting fibroblast growth factor 10/FGFR1 in relapsed rheumatoid arthritis. Arthritis and Rheumatology 76, 32–47. doi:10.1002/art.42674
Mondal, S., Saha, S., and Sur, D. (2024). Immuno-metabolic reprogramming of T cell: a new frontier for pharmacotherapy of Rheumatoid arthritis. Immunopharmacol. Immunotoxicol. 46, 330–340. doi:10.1080/08923973.2024.2330636
Mueller, A.-L., Payandeh, Z., Mohammadkhani, N., Mubarak, S. M., Zakeri, A., Alagheband Bahrami, A., et al. (2021). Recent advances in understanding the pathogenesis of rheumatoid arthritis: new treatment strategies. Cells 10, 3017. doi:10.3390/cells10113017
Myasoedova, E., Davis, J., Matteson, E. L., and Crowson, C. S. (2020). Is the epidemiology of rheumatoid arthritis changing? Results from a population-based incidence study, 1985–2014. Ann. Rheumatic Dis. 79, 440–444. doi:10.1136/annrheumdis-2019-216694
Nagafuchi, Y., Ota, M., Hatano, H., Inoue, M., Kobayashi, S., Okubo, M., et al. (2022). Control of naive and effector CD4 T cell receptor repertoires by rheumatoid-arthritis-risk HLA alleles. J. Autoimmun. 133, 102907. doi:10.1016/j.jaut.2022.102907
Nygaard, G., and Firestein, G. S. (2020). Restoring synovial homeostasis in rheumatoid arthritis by targeting fibroblast-like synoviocytes. Nat. Rev. Rheumatol. 16, 316–333. doi:10.1038/s41584-020-0413-5
Odegård, S., Finset, A., Mowinckel, P., Kvien, T. K., and Uhlig, T. (2007). Pain and psychological health status over a 10-year period in patients with recent onset rheumatoid arthritis. Ann. Rheum. Dis. 66, 1195–1201. doi:10.1136/ard.2006.064287
Ogata, A., Hirano, T., Hishitani, Y., and Tanaka, T. (2012). Safety and efficacy of tocilizumab for the treatment of rheumatoid arthritis. Clin. Med. Insights Arthritis Musculoskelet. Disord. 5, 27–42. doi:10.4137/CMAMD.S7371
Ogata, A., Kato, Y., Higa, S., and Yoshizaki, K. (2019). IL-6 inhibitor for the treatment of rheumatoid arthritis: a comprehensive review. Mod. Rheumatol. 29, 258–267. doi:10.1080/14397595.2018.1546357
Ospelt, C., Brentano, F., Rengel, Y., Stanczyk, J., Kolling, C., Tak, P. P., et al. (2008). Overexpression of toll-like receptors 3 and 4 in synovial tissue from patients with early rheumatoid arthritis: Toll-like receptor expression in early and longstanding arthritis. Arthritis and Rheumatism 58, 3684–3692. doi:10.1002/art.24140
Pan, H., Huang, W., Wang, Z., Ren, F., Luo, L., Zhou, J., et al. (2021). The ACE2-ang-(1-7)-mas Axis modulates M1/M2 macrophage polarization to relieve CLP-induced inflammation via TLR4-mediated NF-кb and MAPK pathways. J. Inflamm. Res. 14, 2045–2060. doi:10.2147/JIR.S307801
Pandolfi, F., Franza, L., Carusi, V., Altamura, S., Andriollo, G., and Nucera, E. (2020). Interleukin-6 in rheumatoid arthritis. Int. J. Mol. Sci. 21, 5238. doi:10.3390/ijms21155238
Papadaki, G., Goutakoli, P., Tiniakou, I., Grün, J. R., Grützkau, A., Pavlopoulos, G. A., et al. (2022). IL-6 signaling attenuates TNF-α production by plasmacytoid dendritic cells in rheumatoid arthritis. J. Immunol. 209, 1906–1917. doi:10.4049/jimmunol.2100882
Park, J.-S., Kim, S.-H., Kim, K., Jin, C.-H., Choi, K. Y., Jang, J., et al. (2015). Inhibition of Notch signalling ameliorates experimental inflammatory arthritis. Ann. Rheumatic Dis. 74, 267–274. doi:10.1136/annrheumdis-2013-203467
Phillips, R. (2023). Nanobody targets TNF and IL-6 for additive efficacy. Nat. Rev. Rheumatol. 19, 194. doi:10.1038/s41584-023-00947-z
Pradeep, C. R., and Kuttan, G. (2004). Piperine is a potent inhibitor of nuclear factor-κB (NF-κB), c-Fos, CREB, ATF-2 and proinflammatory cytokine gene expression in B16F-10 melanoma cells. Int. Immunopharmacol. 4, 1795–1803. doi:10.1016/j.intimp.2004.08.005
Prasad, P., Verma, S., Surbhi, , Ganguly, N. K., Chaturvedi, V., and Mittal, S. A. (2023). Rheumatoid arthritis: advances in treatment strategies. Mol. Cell Biochem. 478 69–88. doi:10.1007/s11010-022-04492-3
Qiao, S., Lian, X., Yue, M., Zhang, Q., Wei, Z., Chen, L., et al. (2020). Regulation of gut microbiota substantially contributes to the induction of intestinal Treg cells and consequent anti-arthritis effect of madecassoside. Int. Immunopharmacol. 89, 107047. doi:10.1016/j.intimp.2020.107047
Reparon-Schuijt, C. C., Van Esch, W. J. E., Van Kooten, C., Rozier, B. C. D., Levarht, E. W. N., Breedveld, F. C., et al. (2000). Regulation of synovial B cell survival in rheumatoid arthritis by vascular cell adhesion molecule 1 (CD106) expressed on fibroblast-like synoviocytes. Arthritis and Rheumatism 43, 1115–1121. doi:10.1002/1529-0131(200005)43:5<1115::AID-ANR22>3.0.CO;2-A
Roll, P., Palanichamy, A., Kneitz, C., Dorner, T., and Tony, H. P. (2006). Regeneration of B cell subsets after transient B cell depletion using anti-CD20 antibodies in rheumatoid arthritis. Arthritis and Rheumatism Official J. Am. Coll. Rheumatology 54, 2377–2386. doi:10.1002/art.22019
Roman-Blas, J. A., and Jimenez, S. A. (2006). NF-kappaB as a potential therapeutic target in osteoarthritis and rheumatoid arthritis. Osteoarthr. Cartil. 14, 839–848. doi:10.1016/j.joca.2006.04.008
Rosenzwajg, M., Churlaud, G., Mallone, R., Six, A., Dérian, N., Chaara, W., et al. (2015). Low-dose interleukin-2 fosters a dose-dependent regulatory T cell tuned milieu in T1D patients. J. Autoimmun. 58, 48–58. doi:10.1016/j.jaut.2015.01.001
Ross, E. A., Devitt, A., and Johnson, J. R. (2021). Macrophages: the good, the bad, and the gluttony. Front. Immunol. 12, 708186. doi:10.3389/fimmu.2021.708186
Rosser, E. C., Piper, C. J. M., Matei, D. E., Blair, P. A., Rendeiro, A. F., Orford, M., et al. (2020). Microbiota-derived metabolites suppress arthritis by amplifying aryl-hydrocarbon receptor activation in regulatory B cells. Cell Metab. 31, 837–851.e10. doi:10.1016/j.cmet.2020.03.003
Rubbert-Roth, A., Szabó, M. Z., Kedves, M., Nagy, G., Atzeni, F., and Sarzi-Puttini, P. (2019). Failure of anti-TNF treatment in patients with rheumatoid arthritis: the pros and cons of the early use of alternative biological agents. Autoimmun. Rev. 18, 102398. doi:10.1016/j.autrev.2019.102398
Sağ, S., Sağ, M. S., Tekeoğlu, I., Kamanlı, A., Nas, K., and Acar, B. A. (2020). Central nervous system involvement in rheumatoid arthritis: possible role of chronic inflammation and tnf blocker therapy. Acta Neurol. Belg. 120, 25–31. doi:10.1007/s13760-017-0879-3
Schett, G., Tanaka, Y., and Isaacs, J. D. (2021). Why remission is not enough: underlying disease mechanisms in RA that prevent cure. Nat. Rev. Rheumatol. 17, 135–144. doi:10.1038/s41584-020-00543-5
Schiff, M. (2015). Co-Stimulation therapy in rheumatoid arthritis: today and tomorrow. Curr. Treat. Options Rheumatology 1, 334–349. doi:10.1007/s40674-015-0029-0
Seibl, R., Birchler, T., Loeliger, S., Hossle, J. P., Gay, R. E., Saurenmann, T., et al. (2003). Expression and regulation of toll-like receptor 2 in rheumatoid arthritis synovium. Am. J. Pathology 162, 1221–1227. doi:10.1016/S0002-9440(10)63918-1
Shi, Y., Lv, Q., Zheng, M., Sun, H., and Shi, F. (2021). NLRP3 inflammasome inhibitor INF39 attenuated NLRP3 assembly in macrophages. Int. Immunopharmacol. 92, 107358. doi:10.1016/j.intimp.2020.107358
Singh, P. K., Yang, X. Y., Zheng, K. D., Lin, K., Zheng, G., Zou, H., et al. (2015). Energy metabolism disorder as a contributing factor of rheumatoid arthritis: a comparative proteomic and metabolomic study. Plos One 10, e0132695. doi:10.1371/journal.pone.0132695
Smolen, J. S., Aletaha, D., Barton, A., Burmester, G. R., Emery, P., Firestein, G. S., et al. (2018). Rheumatoid arthritis. Nat. Rev. Dis. Prim. 4, 18001. doi:10.1038/nrdp.2018.1
Smolen, J. S., Aletaha, D., and McInnes, I. B. (2016). Rheumatoid arthritis. Lancet 388, 2023–2038. doi:10.1016/S0140-6736(16)30173-8
Spolski, R., Li, P., and Leonard, W. J. (2018). Biology and regulation of IL-2: from molecular mechanisms to human therapy. Nat. Rev. Immunol. 18, 648–659. doi:10.1038/s41577-018-0046-y
Stein, M., Keshav, S., Harris, N., and Gordon, S. (1992). Interleukin 4 potently enhances murine macrophage mannose receptor activity: a marker of alternative immunologic macrophage activation. J. Exp. Med. 176, 287–292. doi:10.1084/jem.176.1.287
Sun, H.-G., Jiang, Q., Fan, W.-J., Shen, X.-Y., Wang, Z.-W., and Wang, X. (2023). TAGAP activates Th17 cell differentiation by promoting RhoA and NLRP3 to accelerate rheumatoid arthritis development. Clin. Exp. Immunol. 214, 26–35. doi:10.1093/cei/uxad084
Szabo, D., Balogh, A., Gopcsa, L., Giba-Kiss, L., Lakatos, G., Paksi, M., et al. (2024). Sustained drug-free remission in rheumatoid arthritis associated with diffuse large B-cell lymphoma following tandem CD20-CD19-directed non-cryopreserved CAR-T cell therapy using zamtocabtagene autoleucel. RMD Open 10, e004727. doi:10.1136/rmdopen-2024-004727
Takagi, H., Fukaya, T., Eizumi, K., Sato, Y., Sato, K., Shibazaki, A., et al. (2011). Plasmacytoid dendritic cells are crucial for the initiation of inflammation and T cell immunity in vivo. Immunity 35, 958–971. doi:10.1016/j.immuni.2011.10.014
Takeshita, M., Suzuki, K., Kondo, Y., Morita, R., Okuzono, Y., Koga, K., et al. (2019). Multi-dimensional analysis identified rheumatoid arthritis-driving pathway in human T cell. Ann. Rheumatic Dis. 78, 1346–1356. doi:10.1136/annrheumdis-2018-214885
Takeuchi, T., Kawanishi, M., Nakanishi, M., Yamasaki, H., and Tanaka, Y. (2022). Phase II/III results of a trial of anti–tumor necrosis factor multivalent NANOBODY compound ozoralizumab in patients with rheumatoid arthritis. Arthritis and Rheumatology 74, 1776–1785. doi:10.1002/art.42273
Tan, Q., Chen, B., Wang, Q., Xu, W., Wang, Y., Lin, Z., et al. (2018). A novel FGFR1-binding peptide attenuates the degeneration of articular cartilage in adult mice. Osteoarthr. Cartil. 26, 1733–1743. doi:10.1016/j.joca.2018.08.012
Taylor, P. C., Atzeni, F., Balsa, A., Gossec, L., Müller-Ladner, U., and Pope, J. (2021). The key comorbidities in patients with rheumatoid arthritis: a narrative review. J. Clin. Med. 10, 509. doi:10.3390/jcm10030509
Tsaltskan, V., and Firestein, G. S. (2022). Targeting fibroblast-like synoviocytes in rheumatoid arthritis. Curr. Opin. Pharmacol. 67, 102304. doi:10.1016/j.coph.2022.102304
Tsumoto, K., and Takeuchi, T. (2024). Next-generation anti-tnfα agents: the example of ozoralizumab. BioDrugs 38, 341–351. doi:10.1007/s40259-024-00648-3
Udalova, I. A., Mantovani, A., and Feldmann, M. (2016). Macrophage heterogeneity in the context of rheumatoid arthritis. Nat. Rev. Rheumatol. 12, 472–485. doi:10.1038/nrrheum.2016.91
Vergne-Salle, P., Pouplin, S., Trouvin, A. P., Bera-Louville, A., Soubrier, M., Richez, C., et al. (2020). The burden of pain in rheumatoid arthritis: impact of disease activity and psychological factors. Eur. J. Pain 24, 1979–1989. doi:10.1002/ejp.1651
Wada, T. T., Araki, Y., Sato, K., Aizaki, Y., Yokota, K., Kim, Y. T., et al. (2014). Aberrant histone acetylation contributes to elevated interleukin-6 production in rheumatoid arthritis synovial fibroblasts. Biochem. Biophysical Res. Commun. 444, 682–686. doi:10.1016/j.bbrc.2014.01.195
Wang, L.-x., Zhang, S.-x., Wu, H.-j., Rong, X.-l., and Guo, J. (2019). M2b macrophage polarization and its roles in diseases. J. Leukoc. Biol. 106, 345–358. doi:10.1002/JLB.3RU1018-378RR
Wang, M., Zhu, S., Peng, W., Li, Q., Li, Z., Luo, M., et al. (2014). Sonic hedgehog signaling drives proliferation of synoviocytes in rheumatoid arthritis: a possible novel therapeutic target. J. Immunol. Res. 2014, 401903–401910. doi:10.1155/2014/401903
Wang, Q., Zhou, X., Zhao, Y., Xiao, J., Lu, Y., Shi, Q., et al. (2018). Polyphyllin I ameliorates collagen-induced arthritis by suppressing the inflammation response in macrophages through the NF-κB pathway. Front. Immunol. 9. doi:10.3389/fimmu.2018.02091
Wang, X., Jiang, Y., Zhou, P., Lin, L., Yang, Y., Yang, Q., et al. (2022). Effective natural inhibitors targeting granzyme B in rheumatoid arthritis by computational study. Front. Med. 9, 1052792. doi:10.3389/fmed.2022.1052792
Wu, D., Luo, Y., Li, T., Zhao, X., Lv, T., Fang, G., et al. (2022). Systemic complications of rheumatoid arthritis: focus on pathogenesis and treatment. Front. Immunol. 13, 1051082. doi:10.3389/fimmu.2022.1051082
Wu, R., Li, N., Zhao, X., Ding, T., Xue, H., Gao, C., et al. (2020). Low-dose Interleukin-2: biology and therapeutic prospects in rheumatoid arthritis. Autoimmun. Rev. 19, 102645. doi:10.1016/j.autrev.2020.102645
Wysocki, T., Olesińska, M., and Paradowska-Gorycka, A. (2020). Current understanding of an emerging role of HLA-DRB1 gene in rheumatoid arthritis–from research to clinical practice. Cells 9, 1127. doi:10.3390/cells9051127
Xu, H., Zhao, H., Fan, D., Liu, M., Cao, J., Xia, Y., et al. (2020). Interactions between gut microbiota and immunomodulatory cells in rheumatoid arthritis. Mediat. Inflamm. 2020, 1430605–1430614. doi:10.1155/2020/1430605
Yamamura, Y., Gupta, R., Morita, Y., He, X., Pai, R., Endres, J., et al. (2001). Effector function of resting T cells: activation of synovial fibroblasts. J. Immunol. 166, 2270–2275. doi:10.4049/jimmunol.166.4.2270
Yang, D., Lv, G., Wu, Y., Guo, W., Wang, Y., Hu, J., et al. (2024). Licorice-regulated gut–joint axis for alleviating collagen-induced rheumatoid arthritis. Phytomedicine 135, 156203. doi:10.1016/j.phymed.2024.156203
Yang, S., Yin, W., Ding, Y., and Liu, F. (2020). Lnc RNA ZFAS1 regulates the proliferation, apoptosis, inflammatory response and autophagy of fibroblast-like synoviocytes via miR-2682-5p/ADAMTS9 axis in rheumatoid arthritis. Biosci. Rep. 40. doi:10.1042/BSR20201273
Yang, Y., Guo, L., Wang, Z., Liu, P., Liu, X., Ding, J., et al. (2021). Targeted silver nanoparticles for rheumatoid arthritis therapy via macrophage apoptosis and Re-polarization. Biomaterials 264, 120390. doi:10.1016/j.biomaterials.2020.120390
Yannaki, E., Papadopoulou, A., Athanasiou, E., Kaloyannidis, P., Paraskeva, A., Bougiouklis, D., et al. (2010). The proteasome inhibitor bortezomib drastically affects inflammation and bone disease in adjuvant-induced arthritis in rats. Arthritis and Rheumatism 62, 3277–3288. doi:10.1002/art.27690
Yao, Y., Cai, X., Zheng, Y., Zhang, M., Fei, W., Sun, D., et al. (2022). Short-chain fatty acids regulate B cells differentiation via the FFA2 receptor to alleviate rheumatoid arthritis. Br. J. Pharmacol. 179, 4315–4329. doi:10.1111/bph.15852
Ye, Y., Gao, X., and Yang, N. (2017). LncRNA ZFAS1 promotes cell migration and invasion of fibroblast-like synoviocytes by suppression of miR-27a in rheumatoid arthritis. Hum. Cell 31, 14–21. doi:10.1007/s13577-017-0179-5
Yin, Q., Wu, Y.-j., Pan, S., Wang, D.-d., Tao, M.-q., Pei, W.-y., et al. (2020). Activation of cholinergic anti-inflammatory pathway in peripheral immune cells involved in therapeutic actions of α-mangostin on collagen-induced arthritis in rats [corrigendum]. Drug Des. Dev. Ther. 14, 2235–2236. doi:10.2147/dddt.s264751
Yiou, W., Zhihong, W., Shibai, Z., Shanni, L., and Wenwei, Q. (2023). MiR-326 regulates cell proliferation and apoptosis in fibroblast-like synoviocytes in rheumatoid arthritis. Hum. Cell 36, 987–996. doi:10.1007/s13577-023-00873-y
Yoshitomi, H. (2019). Regulation of immune responses and chronic inflammation by fibroblast-like synoviocytes. Front. Immunol. 10, 1395. doi:10.3389/fimmu.2019.01395
You, D. G., Lim, G. T., Kwon, S., Um, W., Oh, B. H., Song, S. H., et al. (2021). Metabolically engineered stem cell–derived exosomes to regulate macrophage heterogeneity in rheumatoid arthritis. Sci. Adv. 7, eabe0083. doi:10.1126/sciadv.abe0083
Zaiss, M. M., Joyce Wu, H.-J., Mauro, D., Schett, G., and Ciccia, F. (2021). The gut–joint axis in rheumatoid arthritis. Nat. Rev. Rheumatol. 17, 224–237. doi:10.1038/s41584-021-00585-3
Zerrouk, N., Alcraft, R., Hall, B. A., Augé, F., and Niarakis, A. (2024). Large-scale computational modelling of the M1 and M2 synovial macrophages in rheumatoid arthritis. npj Syst. Biol. Appl. 10, 10. doi:10.1038/s41540-024-00337-5
Zhang, L., Song, P., Zhang, X., Metea, C., Schleisman, M., Karstens, L., et al. (2020). Alpha-glucosidase inhibitors alter gut microbiota and ameliorate collagen-induced arthritis. Front. Pharmacol. 10, 1684. doi:10.3389/fphar.2019.01684
Zhang, Y., Zhen, S., Xu, H., Sun, S., Wang, Z., Li, M., et al. (2024). Vitamin C alleviates rheumatoid arthritis by modulating gut microbiota balance. Biosci. Trends 18, 187–194. doi:10.5582/bst.2024.01037
Zheng, J., Hu, J., Yang, Y., Xiong, L., Yang, H., Zhang, Z., et al. (2023). Suppressive effect of Tripterygium hypoglaucum (Levl.) Hutch extract on rheumatoid arthritis in mice by modulating inflammasome and bile acid metabolism. Biomed. and Pharmacother. 167, 115494. doi:10.1016/j.biopha.2023.115494
Zheng, Y., Cai, X., Ren, F., and Yao, Y. (2024b). The role of non-coding RNAs in fibroblast-like synoviocytes in rheumatoid arthritis. Int. J. Rheumatic Dis. 27. doi:10.1111/1756-185x.15376
Keywords: rheumatoid arthritis, inflammation resolution, macrophages, fibroblast-like synoviocytes, cytokines
Citation: Ye X, Ren D, Chen Q, Shen J, Wang B, Wu S and Zhang H (2025) Resolution of inflammation during rheumatoid arthritis. Front. Cell Dev. Biol. 13:1556359. doi: 10.3389/fcell.2025.1556359
Received: 06 January 2025; Accepted: 12 March 2025;
Published: 26 March 2025.
Edited by:
Kihong Lim, University of Rochester, United StatesReviewed by:
Florian Barthelemy, University of California, Los Angeles, United StatesCopyright © 2025 Ye, Ren, Chen, Shen, Wang, Wu and Zhang. This is an open-access article distributed under the terms of the Creative Commons Attribution License (CC BY). The use, distribution or reproduction in other forums is permitted, provided the original author(s) and the copyright owner(s) are credited and that the original publication in this journal is cited, in accordance with accepted academic practice. No use, distribution or reproduction is permitted which does not comply with these terms.
*Correspondence: Hongliang Zhang, emhsQGxzdS5lZHUuY24=
Disclaimer: All claims expressed in this article are solely those of the authors and do not necessarily represent those of their affiliated organizations, or those of the publisher, the editors and the reviewers. Any product that may be evaluated in this article or claim that may be made by its manufacturer is not guaranteed or endorsed by the publisher.
Research integrity at Frontiers
Learn more about the work of our research integrity team to safeguard the quality of each article we publish.