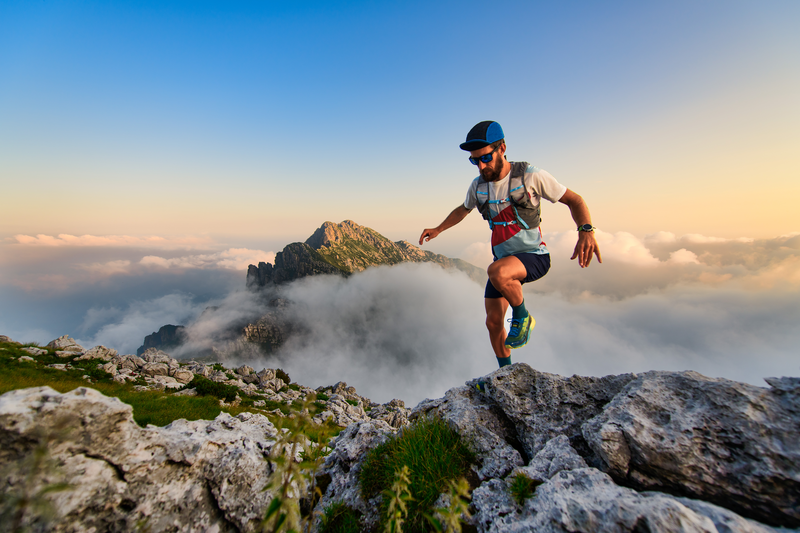
94% of researchers rate our articles as excellent or good
Learn more about the work of our research integrity team to safeguard the quality of each article we publish.
Find out more
ORIGINAL RESEARCH article
Front. Cell Dev. Biol.
Sec. Molecular and Cellular Pathology
Volume 13 - 2025 | doi: 10.3389/fcell.2025.1549063
The final, formatted version of the article will be published soon.
You have multiple emails registered with Frontiers:
Please enter your email address:
If you already have an account, please login
You don't have a Frontiers account ? You can register here
Connexins are gap junction proteins that play pivotal roles in intercellular communication. Connexin 43 (Cx43) is one of the most ubiquitously expressed connexin isoforms in human. Cx43 has been demonstrated to be involved in the pathological process of various diseases, including arrhythmias. Recently, translationally controlled tumor protein (TCTP), a highly conserved anti-apoptotic protein, has been shown to play an important role in protecting against the development of heart failure. However, its role in arrhythmogenesis remains unclear. In this study, we aimed to examine the interaction between TCTP and Cx43 and investigate the roles of TCTP in the formation of Cx43 gap junction channels and gap junctional intercellular communication (GJIC) in cardiomyocytes. Methods and Results: We found that TCTP was predominantly expressed in the intercalated discs of mouse heart tissue. Cx43 in adult mouse hearts was coimmunoprecipitated using a TCTP-specific antibody. Additionally, co-localization of TCTP and Cx43 was demonstrated using a proximity ligation assay in iPS cell-derived human cardiomyocytes. TCTP silencing reduced the formation of Cx43 gap junction channels at the intercellular contacts between cardiomyocytes.Moreover, TCTP silencing significantly attenuated GJIC among cardiomyocytes. Interestingly, the development of ventricular arrhythmia was attenuated in cardiomyocyte-specific TCTPoverexpressing mice. Conclusions: These findings indicate that TCTP regulates GJIC. Thus, TCTP may be a therapeutic target for preventing Cx43-related pathogenesis.
Keywords: Translationally Controlled Tumor Protein, Cx43, Heart, gap junctional intercellular communication, cardiac arrhythmias
Received: 20 Dec 2024; Accepted: 25 Feb 2025.
Copyright: © 2025 Hu, Cai, Hidaka, Hiraishi, Cang, Umemura, Yokoyama, Knollmann, Ishikawa and Fujita. This is an open-access article distributed under the terms of the Creative Commons Attribution License (CC BY). The use, distribution or reproduction in other forums is permitted, provided the original author(s) or licensor are credited and that the original publication in this journal is cited, in accordance with accepted academic practice. No use, distribution or reproduction is permitted which does not comply with these terms.
* Correspondence:
Takayuki Fujita, Department of Physiology, Fukuoka University School of Medicine, Fukuoka, Japan
Disclaimer: All claims expressed in this article are solely those of the authors and do not necessarily represent those of their affiliated organizations, or those of the publisher, the editors and the reviewers. Any product that may be evaluated in this article or claim that may be made by its manufacturer is not guaranteed or endorsed by the publisher.
Research integrity at Frontiers
Learn more about the work of our research integrity team to safeguard the quality of each article we publish.