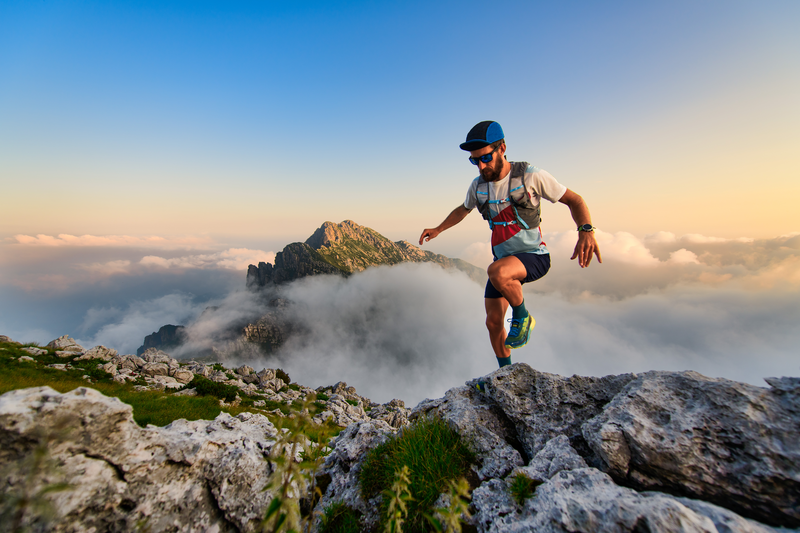
94% of researchers rate our articles as excellent or good
Learn more about the work of our research integrity team to safeguard the quality of each article we publish.
Find out more
ORIGINAL RESEARCH article
Front. Cell Dev. Biol.
Sec. Embryonic Development
Volume 13 - 2025 | doi: 10.3389/fcell.2025.1530953
This article is part of the Research Topic Cellular Micro-Environment of the Endometrium: Reproduction, Embryo Implantation, and Placentation - From Bench to Bedside and Beyond to Tissue Engineering View all 5 articles
The final, formatted version of the article will be published soon.
You have multiple emails registered with Frontiers:
Please enter your email address:
If you already have an account, please login
You don't have a Frontiers account ? You can register here
Background: Endometrial thickness (EMT) is a crucial indicator of endometrial receptivity in assisted reproductive technology (ART). However, its relationship with pregnancy outcomes remains unclear, especially across different cycle types such as fresh in vitro fertilization-embryo transfer (IVF-ET), frozen-thawed embryo transfer (FET), and preimplantation genetic testing for aneuploidy embryo transfer (PGT-ET).The clinical significance of EMT and its optimal range for improving ART outcomes remain subjects of debate. Methods: This retrospective cohort study analyzed data from 80,585 ART cycles conducted between July 2008 and December 2022 at a private reproductive center, including 25,683 fresh IVF-ET, 33,112 FET, and 1,071 PGT-ET cycles. EMT was measured via ultrasound on the day of HCG administration and grouped into ranges for comparison. Primary outcomes included live birth rates (LBR) and clinical pregnancy rates (CPR) across EMT ranges. Statistical analyses, including chi-square tests, receiver operating characteristic (ROC) analysis, and adjusted risk ratio (aRR) calculations, were performed to evaluate the association between EMT and pregnancy outcomes.The relationship between EMT and LBR was non-linear, with no single cutoff value. LBR varied significantly across EMT ranges, peaking at approximately 12 mm in fresh IVF-ET cycles and around 10 mm in FET and PGT-ET cycles. Higher EMT was generally associated with improved LBR and CPR, but predictive power was limited (AUC: 0.56-0.60). Compared to an EMT of 10-11.9 mm, fresh IVF-ET cycles with EMT <10 mm had significantly lower LBR (aRR: 0.60-0.86), while those with EMT ≥12 mm had higher LBR (aRR: 1.12-1.17). Similar trends were observed in FET and PGT-ET cycles, although sensitivity to EMT variations was lower, particularly in PGT-ET cycles. Miscarriage rates (MR) showed no significant differences across EMT groups.This study demonstrates that EMT has a non-linear association with LBR and CPR across fresh IVF-ET, FET, and PGT-ET cycles, with no single cutoff value.While higher EMT generally correlates with improved outcomes, its overall predictive value for LBR is limited. The findings underscore the need for individualized evaluation of EMT based on cycle type to optimize reproductive outcomes in ART.
Keywords: Endometrial thickness, live birth rates, Art, IVF, pregnancy outcomes
Received: 19 Nov 2024; Accepted: 24 Feb 2025.
Copyright: © 2025 Huang, Tang, Wei, Nong, Tang, Wei, Zhang, Yao, Li and Fan. This is an open-access article distributed under the terms of the Creative Commons Attribution License (CC BY). The use, distribution or reproduction in other forums is permitted, provided the original author(s) or licensor are credited and that the original publication in this journal is cited, in accordance with accepted academic practice. No use, distribution or reproduction is permitted which does not comply with these terms.
* Correspondence:
Li Fan, Liuzhou Maternal and Child Health Hospital, Liuzhou, China
Disclaimer: All claims expressed in this article are solely those of the authors and do not necessarily represent those of their affiliated organizations, or those of the publisher, the editors and the reviewers. Any product that may be evaluated in this article or claim that may be made by its manufacturer is not guaranteed or endorsed by the publisher.
Research integrity at Frontiers
Learn more about the work of our research integrity team to safeguard the quality of each article we publish.