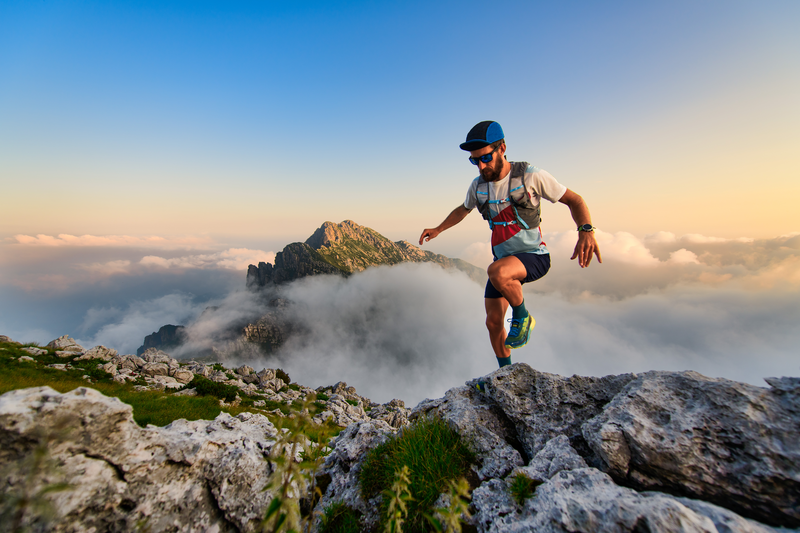
94% of researchers rate our articles as excellent or good
Learn more about the work of our research integrity team to safeguard the quality of each article we publish.
Find out more
REVIEW article
Front. Cell Dev. Biol.
Sec. Cancer Cell Biology
Volume 13 - 2025 | doi: 10.3389/fcell.2025.1489958
The final, formatted version of the article will be published soon.
You have multiple emails registered with Frontiers:
Please enter your email address:
If you already have an account, please login
You don't have a Frontiers account ? You can register here
Nicotinic acetylcholine receptors (nAChRs) are widely expressed in a variety of cell types and are involved in multiple physiological regulatory mechanisms in cells, tissues and systems.Increasing evidence suggests that the α5 nicotinic acetylcholine receptor (α5-nAChR), encoded by the CHRNA5 gene, is one of a key mediator involved in lung cancer development and immune responses. Several studies have shown that it is a regulator that stimulates processes via various signaling pathways, including STAT3 in lung cancer. In addition, α5-nAChR has a profound effect on lung immune response through multiple immune-related factor pathways. In this review, we focus on the perspectives on α5-nAChR in lung cancer progression, which indicates that targeting α5-nAChR could provide novel anticancer and immune therapy strategies for lung cancer.
Keywords: α5-nAChR, Growth, Epithelial mesenchymal transition (EMT), immune escape, lung cancer
Received: 02 Sep 2024; Accepted: 26 Feb 2025.
Copyright: © 2025 Jiaying, Jingting, Zengping, Jing, Jia and Ma. This is an open-access article distributed under the terms of the Creative Commons Attribution License (CC BY). The use, distribution or reproduction in other forums is permitted, provided the original author(s) or licensor are credited and that the original publication in this journal is cited, in accordance with accepted academic practice. No use, distribution or reproduction is permitted which does not comply with these terms.
* Correspondence:
Xiaoli Ma, Central Hospital Affiliated to Shandong First Medical University, Jinan, 271000, Shandong Province, China
Disclaimer: All claims expressed in this article are solely those of the authors and do not necessarily represent those of their affiliated organizations, or those of the publisher, the editors and the reviewers. Any product that may be evaluated in this article or claim that may be made by its manufacturer is not guaranteed or endorsed by the publisher.
Research integrity at Frontiers
Learn more about the work of our research integrity team to safeguard the quality of each article we publish.