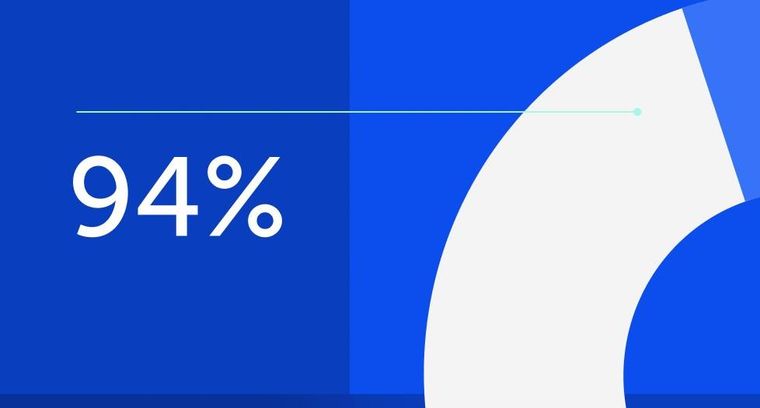
94% of researchers rate our articles as excellent or good
Learn more about the work of our research integrity team to safeguard the quality of each article we publish.
Find out more
REVIEW article
Front. Cell Dev. Biol., 20 November 2024
Sec. Signaling
Volume 12 - 2024 | https://doi.org/10.3389/fcell.2024.1506450
Follicle-stimulating hormone (FSH), a glycoprotein hormone synthesized and secreted by the anterior pituitary gland, plays a critical role in reproductive development and regulation by binding to FSH receptor (FSHR). Beyond reproductive tissue, FSHRs have been identified in various non-reproductive tissues, indicating broader functions. FSH levels chronically rise during menopause and remain elevated in postmenopausal life. This increase in FSH level has been indicated to be associated with heightened risk of several non-reproductive diseases, including osteoporosis, hypercholesterolemia, type 2 diabetes mellitus, obesity, cardiovascular disease, Alzheimer’s disease, and certain cancers. In this review, we will examine the role of FSH-FSHR signaling in the pathogenesis of these non-reproductive diseases and explore therapeutic strategies targeting FSH-FSHR signaling pathways.
Follicle-stimulating hormone (FSH), a glycoprotein hormone secreted by the anterior pituitary, is primarily recognized for its essential role in reproductive physiology. It can stimulate the development of ovarian follicles in females and spermatogenesis in males, and is regulated by gonadotropin-releasing hormone (GnRH) from the hypothalamus and by steroids feedback of sexual gland, such as estrogen and progesterone (Bernard et al., 2010). Over the past few decades, most research on FSH has been dominated by this classical understanding, which focuses on its crucial roles in reproduction.
However, recent advances in endocrinology have revealed that the influence of FSH extends far beyond its reproductive roles. This is primarily evidenced by the increased identification of FSH extragonadal targets across various tissues and cell types, including bone, pancreatic islets, adipose tissue in different species, such as human and mice (Cheng et al., 2023; Lin and Ge, 2009; Xiong et al., 2023). Growing evidence also suggests that FSH plays a significant role in the pathogenesis of various non-reproductive diseases that have been shown to be more prevalent during menopause, including osteoporosis, type 2 diabetes mellitus, obesity, cardiovascular disease, Alzheimer’s diseases and several cancers (Santoro et al., 2015; Taneja et al., 2019). This paradigm shift in knowledge calls for a more thorough investigation of the non-reproductive role of FSH and its impact on disease development.
Perimenopause, a complex physiological transition typically occurring around the fourth decade of life, is characterized by irregular menstrual cycles and significant hormonal alterations. A decline in estrogen levels concomitant with elevated FSH and luteinizing hormone (LH) concentrations is most notable during this transition (Santoro et al., 2021). While much of the existing literature has focused on the implications of declining estrogen in perimenopausal pathologies, recent studies suggest that elevated FSH may play a more direct and substantial role in the etiology of certain conditions (Gallagher et al., 2010). For instance, the well-documented association between FSH and osteoporosis has catalyzed further exploration into the broader non-reproductive functions of FSH (Cheng et al., 2023; El Khoudary, 2017; Yeh et al., 1996).
FSH mediates its biological effects through interaction with specific G protein-coupled receptors, known as FSHRs. Currently, four isoforms of FSHR (FSHR1, FSHR2, FSHR3, and FSHR4) have been identified across various tissues, each isoform being linked to distinct G protein interactions and subsequent downstream signaling pathways (Simoni et al., 2002). While FSHR1 predominantly governs reproductive functions via cyclic adenosine monophosphate (cAMP)-mediated signaling, other isoforms, such as FSHR3, are implicated in mitogen-activated protein kinase/extracellular signal-regulated kinase (MAPK/ERK) signaling and calcium influx pathways, thereby influencing a wide spectrum of cellular processes beyond reproduction (Li et al., 2007). The presence of FSHRs in non-reproductive tissues, including osteoclasts, adipocytes, vascular endothelial cells, hepatocytes, and neural cells, suggests the potential involvement of FSH in the pathogenesis of various non-reproductive diseases (Casarini and Crepieux, 2019).
This review endeavors to provide a comprehensive update on the current understanding of the non-reproductive functions of FSH and their underlying molecular mechanisms. By examining the influence of FSH on various tissues and its association with disease pathophysiology, this review seeks to elucidate how alterations in FSH levels contribute to increased disease risks. Additionally, this review will explore the potential of FSH-related treatment strategies and discuss future potential directions for research focusing on the non-reproductive roles of FSH.
Osteoporosis is a systemic bone disease characterized by reduced bone mass and microstructural damage to bone tissue, leading to increased bone fragility and susceptibility to fractures (Akhter et al., 2007). In women, the risk of developing osteoporosis increases at the perimenopausal ages, which is accompanied by an increase in FSH levels, supporting the hypothesis that osteoporosis is closely linked to hormonal changes during the menopausal transition (Cannarella et al., 2019; Bergamini et al., 2024). In men with hypogonadism, resulting in excess FSH, observational studies have been conducted. The result also shown that elevated FSH posts a negative effect on male bone mess (Giovanelli et al., 2022). Although previous hypotheses largely agree that the drop in estrogen levels during perimenopause contributes to bone loss, research indicates that an increase in FSH is more closely associated with postmenopausal bone loss (Sun et al., 2006). Early research has shown that bone loss is observed in ovariectomized mice with reduced estrogen level and increased FSH level, but in mice that underwent both ovariectomy and hypophysectomy, the degrade of bone loss is reduced. This result suggests a possible effect of pituitary hormone in the process of bone loss (Yeh et al., 1996). In 2006, Sun et al. (2006) showed that FSHβ or FSHR knockout mice do not develop bone loss, but both develop severe hypogonadism with reduced estrogen levels, further suggesting the effect of FSH on bone resorption.
Subsequent studies have elucidated the mechanisms by which FSH contributes to bone loss. FSHRs coupled to Giα are present in osteoclasts and their progenitors, but not in osteoblasts (Ghinea et al., 2024). By binding to Giα-coupled FSHR on osteoclasts and osteoclast precursors, FSH activates the phosphorylation of extracellular signal-regulated kinase 1 and 2 (ERK1/2), inhibitor of kappa B alpha (IkBa), and protein kinase B (AKT) (Sun et al., 2006). ERK1/2 and IkBa phosphorylation downstream stimulate osteoclast differentiation. The activated AKT signaling pathway does not appear to have a direct influence on osteoclast formation. However, without AKT signaling, FSH-induced osteoclast formation is reduced (Sun et al., 2006). Overall, the main effect of FSH to induce osteoclastogenesis is exerted downstream via the ERK1/2 and IkBa signaling pathways, while AKT acts as a mediator (Sun et al., 2006). In healthy individuals, moderate FSH levels regulate bone remodeling to maintain stable bone mass. However, in pathological conditions or in periods such as postmenopause, the amount of FSH exceeds the normal value and triggers additional differentiation of osteoclasts, which disrupts the balance of bone remodeling (Gorai et al., 1995; Kawai et al., 2004).
Given the uncovered mechanisms of postmenopausal osteoporosis, treatment strategies targeting FSH signaling pathways has been investigated. Initially, GnRH is blocked to downstream suppress FSH level. However, the result shows that the blockade of GnRH signaling not only reduces FSH levels but also leads to hypogonadism and a decrease in LH levels, resulting in serious side effects such as hypogonadism and LH depression (Rendina et al., 2010). To achieve a better therapeutic effect with fewer side effects, further studies are focusing on the development of FSH blocking methods. In 2022, an FSH-blocking antibody, MS-Hu6, was developed and evaluated for safety and efficacy of FSH blocking in mice and monkeys. The results suggest that MS-Hu6 safely and effectively blocks FSH functions without affecting the function of other hormones, such as estrogen and LH. This may be due to the abundance of FSHRs in the ovary, allowing the maintenance of normal FSH functions despite reduced FSH levels. In addition, experiments focused on the therapeutic effect of MS-Hu6 in the treatment of osteoporosis and showed that MS-Hu6 can be distributed into the bone, providing the potential to use it to treat osteoporosis with high FSH levels (Gera et al., 2022). However, these pioneering studies did not establish a way to specifically target the bone tissue, and the use of MS-Hu6 has shown a broad spectrum of effects on adipose tissue, central nervous system and cardiovascular system. Future studies could focus on modifying this antibody through different methods like genetic modification and nanotechnology to specifically target FSHRs on osteoclasts, thereby enabling more precise and specific treatment of osteoporosis.
Metabolic disorders include a number of diseases that result from disruptions in normal metabolic processes. Metabolic disorders often have a poor prognosis due to the complexity of associated complications, including cardiovascular disease and obesity. Several metabolic disorders have been shown to pose a higher risk in postmenopausal women.
Postmenopausal women often exhibit elevated cholesterol levels. A 2019 study involving 278 pre- and postmenopausal women found significantly higher serum FSH, total cholesterol (TC), and low-density lipoprotein cholesterol (LDL-C) levels in postmenopausal women. To isolate the effect estrogen, further experiments were performed using ovariectomized mice treated with exogenous estrogen to avoid estrogen changes via FSH-estrogen feedback loop. Estrogen levels were carefully matched between the experimental and control groups. The results showed that ovariectomized mice administrated additional exogenous FSH had higher TC and LDL-C levels compared to the control group (Guo et al., 2019). This research demonstrates that FSH can affect cholesterol levels independent of estrogen.
Type 2 diabetes mellitus is another common metabolic disorder that often develops following menopause (LeBlanc et al., 2017; Tatulashvili et al., 2021). Type 2 diabetes mellitus is characterized by peripheral insulin resistance or relative insulin deficiency. Several menopause-related factors, including central obesity, insulin resistance, and decreased lean muscle mass, contribute to the increased risk of type 2 diabetes mellitus (Tatulashvili et al., 2021; Paschou et al., 2019; Vryonidou et al., 2015). Elevated FSH levels have been recognized as a significant risk factor in the pathogenesis of this diseases, possibly due to the role of FSH in promoting fat accumulation, inhibiting insulin secretion, and reducing lean muscle mass (Cheng et al., 2023).
Additionally, the reduction in lean muscle mass associated with elevated FSH levels contributes to development of varying degrees of sarcopenia in menopausal women (Sowers et al., 2007; Douchi et al., 2002). Sarcopenia in the elderly can lead to physical frailty, disability, and a reduced quality of life. Severe sarcopenia is also linked to an increased risk of complications, including cardiovascular disease, diabetes, and obesity (Damluji et al., 2023).
The role of FSH in cholesterol metabolism has been increasingly studied, with recent findings identifying FSHR in the liver (Rossouw et al., 2002). Current evidence suggests that FSH contributes to hypercholesterolemia through two primary pathways. First, FSH binding to hepatocytes is thought to activate sterol regulatory element-binding protein, a transcription factor that subsequently upregulates the expression of HMG-CoA reductase (Guo et al., 2019). HMG-CoA reductase is a key enzyme in hepatic cholesterol biosynthesis and its overexpression is associated with the overproduction of cholesterol (Pertusa et al., 2007). Second, FSH can hinder the clearance of LDL-C from the bloodstream. In ovariectomized mice with elevated FSH levels, reduced expression of LDL receptors on hepatocytes was observed, resulting in decreased LDL-C uptake and an overall increase in serum cholesterol levels (Song et al., 2016).
The primary mechanism by which elevated FSH levels may contribute to the development of type 2 diabetes mellitus is the inhibition of insulin secretion from pancreatic islets. FSHRs have been identified on β-cells of human pancreatic islets, which exhibit a biphasic response to FSH. Under normal physiological conditions, FSH binds to FSHRs coupled to the Gαs protein, activating the cAMP/protein kinase A (PKA) and calcium signaling pathways. This pathway enhances glucose-stimulated insulin secretion, helping to maintain normal blood glucose levels. However, when FSH levels are elevated, FSHRs coupled to Gαi are activated, subsequently leading to the inhibition of the cAMP/PKA signaling pathway. This consequently leads to reduced insulin secretion. Thus, prolonged exposure to high FSH levels can lead to insulin deficiency and eventual development of type 2 diabetes mellitus (Cheng et al., 2023).
FSHRs have been identified in muscle cells, though the direct effects of FSH on muscle tissue is still not well understood (Chen et al., 2018). Nevertheless, several FSH-induced changes have been implicated in the development of sarcopenia. For example, the reduction in insulin production due to increased FSH levels can impair glucose uptake by muscle cells, disrupting the maintenance of muscle mass and function (Li et al., 2022). Furthermore, the increased bone resorption associated with high FSH levels may contribute to sarcopenia through bone-muscle crosstalk, as bone loss is often associated with reduction in lean muscle mass (Li et al., 2019).
Efforts to alleviate FSH-induced hypercholesterolemia have included the use of FSH antibodies, although results have been inconsistent. A study investigating the effects of FSH antibody treatment in mice with diet-induced obesity has shown that the treatment successfully reversed triglyceride levels, but it did not significantly alter cholesterol (Liu et al., 2017). In contrast, a 2019 study demonstrated that treatment with FSH antibody could inhibit FSH-induced cholesterol synthesis in ovariectomized mice treated with exogenous estrogen (Guo et al., 2019). The different results of FSH antibodies treatment on cholesterol metabolism highlight the need for further research to explore the relationship between FSH and cholesterol metabolism. In addition to FSH antibodies, reduced lipid and cholesterol levels were also observed in women whose FSH levels decreased by more than 30% following estrogen replacement therapy (Song et al., 2016).
For type 2 diabetes, a lifestyle programme involving 1,007 men with impaired glucose tolerance or type 2 diabetes has shown that testosterone treatment can effectively prevent or revert type 2 diabetes. The group receiving testosterone therapy reports a significantly lower oral glucose tolerance compared to the placebo group. However, 7% of patients in the testosterone-treated group experienced serious adverse events, likely attributable to excess testosterone administration (Wittert et al., 2021). The interaction between FSH and testosterone levels is well established (Oduwole et al., 2021). Therefore, future studies should explore the potential of adjuvant therapies targeting FSH to mitigate the adverse effects associated with excessive exogenous testosterone administration.
Currently, there are no specific treatments directly targeting FSH for type 2 diabetes mellitus and sarcopenia. This may be due to the complex pathogenic mechanisms of these diseases, in which changes in FSH levels play only a minor role. However, treatments such as FSH antibodies, hormone replacement therapy, and FSHR blockade have been successfully applied in related conditions, such as osteoporosis, obesity, and hypercholesterolemia. Thus, targeting FSH in the treatment of type 2 diabetes mellitus and sarcopenia presents a promising area for future research (Bergamini et al., 2024; Rendina et al., 2010; Song et al., 2016).
Postmenopausal women often experience weight gain and changes in body composition, which further contribute to the development of obesity. Obesity development in the elderly is multifactorial, while aging is the most predominant factor. However, postmenopausal obesity is characterized by specific features, including increased visceral adiposity, central obesity, and reduced lean muscle mass (Sutton-Tyrrell et al., 2010). And an increase in FSH level has been indicated to be a major risk factor for the progression of postmenopausal obesity in women (Zsakai et al., 2015; Floyd et al., 2016).
FSH is implicated in various metabolic changes contributing to obesity, such as cholesterol accumulation and the progression of type 2 diabetes mellitus. These FSH-induced alterations are particularly problematic for elderly women, who may have reduced physiological resistance to such disorders (Jaff et al., 2014; Crowther and Norris, 2012). Among these changes, fat accumulation remains the most potent driver of obesity progression.
FSH has been shown to promote adipogenesis, with FSH treatment leading to an increase in adipose tissue, particularly in the visceral organs (Liu et al., 2017). This results in central and abdominal obesity as prominent features (Liu et al., 2017). The accumulation of fat in these regions triggers a cascade of physiological changes, including increased body mass index and altered fat distribution, which ultimately constitute the progression of obesity (Davis et al., 2012; Donato et al., 2006). Fat accumulations in visceral areas significantly increase the risk of complications such as coronary artery disease and respiratory disorders (Alberti et al., 2005). Postmenopausal obesity is further characterized by increases in waist circumference and waist-hip ratio, which is due to central obesity (Gavaler and Rosenblum, 2003; Sternfeld et al., 1999).
The effect of FSH to promote fat accumulation is achieved by targeting adipocytes in different adipose tissues. Among three types of adipose tissues in the human body, FSH mainly affects white adipose tissue and beige adipose tissue, while the effect of FSH on brown adipose tissue is unclear due to a small number of FSHR on adipocytes of brown adipose tissue (Bartke, 2017). In white adipose tissue adipocytes, the activation of FSHRs downstream increases cAMP levels, which downstream activate PKA. PKA phosphorylates a series of transcription factors including peroxisome proliferator-activated receptor gamma (PPARγ), CCAAT/Enhancer binding protein alpha (C/EBPα) and cAMP response element-binding receptors (CREB). These phosphorylated transcription factors then facilitate the expression of lipogenic genes such as lipoprotein lipase (Lpl), fatty acid synthase (Fas) and PPARγ in white adipose tissue, promoting the maturation of preadipocytes into mature adipocytes to perform their fat producing and storage functions (Cui et al., 2012).
Beige adipose tissue is specialized tissue that transforms between white adipose tissue and brown adipose tissue. Studies have shown that FSHR activation blocks the beiging of beige tissue, leading to its fate of white adipose tissue with activation of lipogenesis and inhibition of thermogenesis. In beige tissue, FSHR is coupled to Gαi, which can reduce cAMP and subsequently block the activation of a mitochondrial protein, uncoupling protein-1 (UCP1). UCP1 is a major regulator in beige adipose tissue (Cypess et al., 2009). Normally in the adipocytes of beige adipose tissue, cold exposure triggers the activation of UCP1 mediated by the release of norepinephrine, which facilitates ATP production and oxidation process in abundant mitochondria, allowing the adipose tissue to consume its fat and accelerate metabolic rate. Failure of UCP1 activation in adipose tissue through FSH binding then reduces the consumption of fat tissue and leads to its accumulation and can finally develop into obesity (Marlatt and Ravussin, 2017). The reduction of cAMP also inhibits the process of beige adipose differentiating into brown adipocytes and further increases the expression of lipogenic genes Fas and Lpl (Liu et al., 2017) (Figure 1). In addition, FSH can increase the expression of FSHR in adipocytes and thus amplify its effect (Cui et al., 2012).
Figure 1. Beige adipose tissue activation under different stimuli. This figure compares the intracellular activities of beige adipose tissue in response to cold exposure (left panel) and elevated follicle-stimulating hormone (FSH) levels (right panel). Under cold exposure, norepinephrine activates β-adrenergic receptors associated with Gαs, stimulating an increased cyclic adenosine monophosphate (cAMP) level which activates protein kinase A (PKA). This pathway upregulates uncoupling protein-1 (UCP1) in mitochondria, promoting lipolysis, ATP production, fatty acid oxidation, and the differentiation of preadipocytes into brown adipose tissue (BAT). Conversely, with elevated FSH levels, FSH binds to follicle-stimulating hormone receptor (FSHR), coupled with Gαi proteins, leading to decreased cAMP levels and reduced PKA activity. This inhibition reduces UCP1 activation, decreasing mitochondrial function and energy expenditure, thereby increasing fat storage and reducing thermogenesis. Created in BioRender. gN, fh. (2024) BioRender.com/d80o007.
To overcome the FSH-induced obesity, FSH antibody have been developed and evaluated for their effectiveness and potential as further treatment. Initial experiments were carried out in vitro on mice obesity cell line, 3T3-L1 cells. The result shows that FSH antibody treatment to the cell culture can induce the expression of UCP1 as well as other thermogenic genes, including C/EBPα, cell death-inducing DFFA-like effector A (CIDEA), and vascular endothelial growth factor A (VEGFA) (Liu et al., 2017). This finding supports the hypothesis that blocking FSH signaling pathways can stimulate the formation of thermogenic brown adipose tissue, thereby reducing body fat and potentially preventing or curing obesity (Liu et al., 2017).
Subsequent studies utilized the monoclonal FSH antibody, Hf2, to investigate its potential in treating obesity caused by FSH overexpression. Primary research demonstrated that Hf2 can reduce obesity caused by a high fat diet in both female and male mice, indicating the ability of FSH antibody in treating obesity. To further validate the effect of FSH antibody in conditions of excessive FSH production, Hf2 was administrated to ovariectomized mice. The result shows that FSH antibody can reduce the increase of adipose tissue in ovariectomized mice (Greenhill, 2017).
Except for Hf2, recent studies have also demonstrated that another FSHβ antibody, Hu6, also has the effect to reduce adipose tissue in FSH-induced obesity (Bergamini et al., 2024). These findings highlight the potential of using FSH antibodies to prevent and treat obesity driven by FSH overproduction and other factors, such as aging.
Cardiovascular diseases are currently the leading cause of mortality worldwide, with a significant increased risk observed in postmenopausal women (El Khoudary et al., 2020). Atherosclerosis is the primary underlying mechanism for most cardiovascular disease conditions. The pathogenesis of atherosclerosis involves the accumulation of lipids, inflammatory cells, and fibrous tissue within the arterial walls, which are intricately linked to the endothelium function and lipid metabolism (Yang et al., 2023; Zhao et al., 2019). Previously, the reduction of estrogen during menopause has been considered a key factor in the progression of postmenopausal cardiovascular disease (El Khoudary et al., 2012). However, estrogen replacement therapy has shown limited effectiveness in treating cardiovascular disease, and high-dose estrogen has not been consistently shown to significantly increase the risk of cardiovascular disease, indicating the limited role of estrogen in cardiovascular disease progression (Grodstein et al., 2000; Henderson et al., 1991).
Given this, recent studies have increasingly focused on the role of FSH in cardiovascular disease. Research by Munir et al. (2012) demonstrated a direct correlation between elevated FSH levels and an increased number of aortic plaques. FSH has been linked to conditions that contribute to atherosclerosis, including increased bone resorption and the accumulation of fat and cholesterol (Thomson et al., 2024; Huo et al., 2023). These changes lead to elevated levels of free calcium, fat, and LDL-C within coronary arteries, increasing the risk of carotid intima-media thickness and eventually leading to atherosclerosis (Celestino et al., 2013). Additionally, FSH has been shown to initiate several key processes in the development of atherosclerosis, including adhesion of immune cells and angiogenesis (Moore et al., 2013). In males, recent studies suggest that FSH can exacerbate cardiovascular diseases in the condition of testosterone deficiency, further indicating the FSH effect to promote cardiovascular disease (Duivenvoorden et al., 2024).
Beyond its impact on fat and calcium metabolism, FSH primarily targets endothelial cells in the progression of cardiovascular disease. Endothelial cell activation is crucial in atherosclerosis development. In most conditions, accumulated lipids and local inflammatory factors trigger the expression of adhesion molecules like intracellular adhesion molecule 1 (ICAM-1) and vascular cell adhesion molecule 1 (VCAM-1) on endothelial cells, recruiting immune cells and forming plaques which further damage the vascular wall and block the lumen (Moore et al., 2013). Elevated FSH level activates endothelial cells through an alternative pathway mediated by FSHR. FSHR in endothelial cells are coupled with Gαs proteins, whose activation downstream increases cAMP concentrations and activate PKA. PKA upregulates VCAM-1 expression and initiate atherosclerosis (Li et al., 2017).
FSH also facilitates angiogenesis, a critical step in atherosclerosis. In most conditions of cardiovascular disease, angiogenesis is driven by VEGF (Shaw et al., 2024). However, studies have indicated that FSH can induce angiogenesis without VEGF. FSH perform their angiogenic function also through the FSHR on endothelial cells, but through the phosphoinositide 3-kinase (PI3K)/AKT pathway. The activation of PI3K/AKT pathway in endothelial cells will mimic the function of VEGF and further trigger angiogenesis (Stilley et al., 2014).
Currently, there is no specific treatment for cardiovascular disease targeting FSH or FSHR. FSH antibody or replacement therapies have not significantly reduced atherosclerosis or other conditions of cardiovascular disease. The complexity of cardiovascular disease, involving multiple risk factors such as lifestyle, genetics, and environment, may limit the efficacy of targeting a single risk factor like FSH. However, by targeting FSH signaling, treatment strategies aimed to alleviate symptoms, prevent severe complications, and ensure better prognosis for cardiovascular diseases still hold the potential for future study. For example, we have previously mentioned that FSH-targeting therapies can reduce bone resorption, fat and cholesterol accumulation, which are risk factors that contribute to cardiovascular diseases.
Observational studies utilizing multimodal brain imaging have revealed an increase in amyloid-β (Aβ) deposition in peri-menopausal women (Mosconi et al., 2017). This finding suggests that changes during menopausal transition may contribute to Alzheimer’s disease development. Initial research focused on the role of declining estrogen levels in Alzheimer’s disease progression, but the outcomes of estrogen-replacement therapy in postmenopausal women have been inconsistent, showing effects ranging from exacerbation to improvement of Alzheimer’s disease symptoms (Matyi et al., 2019; Mulnard et al., 2000; Shumaker et al., 2004). In contrast, elevated FSH levels have been shown to correlate with Alzheimer’s disease progression, implicating the elevated FSH as a potential contributor to Alzheimer’s disease pathogenesis (Short et al., 2001).
To specifically investigate the effects of FSH, Xiong et al. (2023) developed an Alzheimer’s disease mouse model through ovariectomy, which disrupts sex hormone levels. Estrogen replacement was employed to maintain normal estrogen levels. This study found an increase in Alzheimer’s disease related markers in the brain, including Aβ and hyperphosphorylated Tau368, indicating that FSH may promote Alzheimer’s disease independently of estrogen. Additionally, treatment with FSH antibodies led to a reduction in these pathological markers, further supporting the notion that FSH plays a role in Alzheimer’s disease development.
These findings establish a relationship between elevated FSH levels and the progression of Alzheimer’s disease, evidenced by increased Alzheimer’s disease hallmarks. More recent studies have demonstrated that FSH directly affects neurons (Short et al., 2001; Xiong et al., 2022; Bowen et al., 2000). Immunofluorescence studies have shown that FSH can increase the levels of Aβ and Tau368 in neurons, which are characteristic of the neurodegenerative process in Alzheimer’s disease (Xiong et al., 2022). Furthermore, FSH administration has been associated with plaque accumulation in the hippocampus and cortex, regions critical for memory and cognition, which led to increased neuronal apoptosis in these areas and increased risk of Alzheimer’s disease (Xiong et al., 2022).
FSHRs are identified in the central nervous system, particularly in neurons within the cortex and hippocampus (Xiong et al., 2022). In neurons, FSHRs are coupled with the Gαi protein, which downstream induces the phosphorylation of AKT, ERK1/2, and SRPK2. The phosphorylation of these molecules leads to the upregulation of the C/EBPβ/asparagine endopeptidase (AEP) pathway, promoting the accumulation of Aβ and Tau368 (Xiong et al., 2023). C/EBPβ is a transcription factor involved in several biological processes, including energy metabolism, cell proliferation, differentiation, and inflammation (Greenbaum et al., 1998; Poli, 1998; Straccia et al., 2011). In Alzheimer’s disease, C/EBPβ has been associated with neurotoxicity and can be induced by pro-inflammatory factors, contributing to the pathological processes in Alzheimer’s disease. Upregulated C/EBPβ expression subsequently enhances the transcription of δ-secretase, also known as AEP. AEP plays a critical role in cleaving amyloid precursor protein (APP) and Tau, thereby facilitating the accumulation of Aβ and hyperphosphorylated Tau368 (Wang et al., 2018). This sequence of events is a significant contributor to the development and progression of Alzheimer’s disease (Figure 2).
Figure 2. The role of FSH-FSHR signaling in Alzheimer’s disease pathogenesis. This figure illustrates the signaling pathways activated by FSH in neurons located within the cortex and hippocampus. This sequence of molecular events contributes to the neurodegenerative processes that are indicated to cause Alzheimer’s disease (AD), highlighting a novel mechanism by which follicle-stimulating hormone (FSH) could influence AD pathology independent of its canonical reproductive roles. In this pathway, FSH activates follicle-stimulating hormone receptor (FSHR) coupled with Gαi, which downstream phosphorylate CCAAT/Enhancer binding protein alpha (C/EBPβ) and stress-activated protein kinase (SRPK2). These two molecules subsequently enhance the expression and effect of asparagine endopeptidase (AEP), leading to an increased cleavage of amyloid precursor protein (APP) and Tau protein. This pathway ends with the formation of neurodegenerative hallmarks amyloid-β (Aβ) and Tau368. Created in BioRender. gN, fh. (2024) BioRender.com/s03s437.
Studies have investigated the potential of targeting FSH signaling in the treatment of Alzheimer’s disease. Notably, the knockdown of neuronal FSHR has been shown to inhibit Alzheimer’s disease pathogenesis, with a significant reduction in the production of Aβ and Tau368 (Xiong et al., 2022). This pioneering research highlights the potential therapeutic strategy of blocking FSH action in neurons to mitigate Alzheimer’s disease progression.
Building on this, a recent study employed an FSHβ antibody to treat Alzheimer’s disease mouse models, corroborating earlier findings by demonstrating a reduction in both Aβ levels and AEP activity. The results suggest that inhibiting FSH could have a neuroprotective effect. Interestingly, the study utilized intraperitoneal administration of the FSH antibody, yielding promising results in preventing Alzheimer’s disease pathology. This finding implies that peripheral blockade of FSH might be sufficient to prevent its pathological effects in the central nervous system (Xiong et al., 2023). Although FSH antibodies have shown significant effects in treating FSH-induced neural impairments, future research is in need to explore other treatment strategies such as FSHR blockade specifically in neurons for better treatment effect targeting central nervous system.
FSH has been demonstrated to take part in the pathogenesis of several cancers, including ovarian and breast cancers in females and prostate cancer in males.
Ovarian cancer has the highest mortality rate among all gynecological cancers and ranks fifth in cancer-related mortality in women. A study involving 1,411 women with epithelial ovarian cancer has shown that the incidence of ovarian cancer increases with elevated FSH levels, particularly post-menopause (Schildkraut et al., 2001). Another study observed increased FSH levels in the peritoneal fluid of ovarian cancer patients, further suggesting a link between high FSH levels and ovarian cancer pathogenesis (Halperin et al., 1999). Moreover, studies have indicated that FSH exerts similar effects on ovarian cancer cells as on follicular development, promoting key cancerous processes such as proliferation, migration, invasion, and angiogenesis (Li et al., 2007; Stilley et al., 2014; Liu et al., 2015).
Breast cancer is the most prevalent cancer type among women globally. Estrogen is the primary hormone implicated in the development of breast cancer. Under normal physiological conditions, estrogen, predominantly produced by the ovaries, plays a crucial role in the development and maintenance of breast tissue. However, in the context of malignancy, estrogen signaling can promote the growth and survival of cancerous cells (Blander, 2006). Beyond estrogen, studies have identified FSHRs on various breast cancer cell lines, such as SK-BR-3, MDA-MB-231, MCF-7, and T-47D. In vitro studies demonstrate that FSH can enhance the survival and proliferation of these cancerous cells (Sanchez et al., 2016). Additionally, FSH, as a major regulator of estrogen, may contribute to the dysregulation of estrogen levels, further influencing the pathogenesis of breast cancer. The interactions between FSH and estrogen may explain the complexity of breast cancer treatment in postmenopausal patients (Yager and Davidson, 2006).
Prostate cancer is the most common cancer among men and holds second highest cancer-related mortality in this population. Androgen plays a significant role in prostate cancer pathogenesis. Given this, androgen deprivation therapy, which blocks androgen signaling, is currently the standard treatment strategy for prostate cancer (Shafi et al., 2013; Desai et al., 2021; Zhang et al., 2022). The relationship between FSH and prostate cancer remains unclear due to a lack of clinical evidence. However, studies have detected FSHRs in several human prostate cancer cell lines, including PC-3, LNCaP, and C4-2. In these studies, administration of FSH has been shown to increase prostate-specific antigen levels and promote the proliferation of PC-3 cells (Dizeyi et al., 2019). Additionally, FSH signals to Sertoli cells to stimulate the production of androgen-binding proteins, which concentrate testosterone in the reproductive system (Oduwole et al., 2021). This effect of FSH suggests a potential role in amplifying androgen effects within the prostate gland, warranting further investigation into the impact of FSH on androgen levels in the prostate gland and its potential role in prostate cancer progression.
FSH primarily targets the reproductive tissues, including the ovarian surface epithelium. FSHRs are abundantly expressed on ovaries and exist in various isoforms that activate distinct downstream pathways involved in non-reproductive functions. These pathways support the growth, survival, metastasis, and apoptosis evasion of ovarian cancer cells (Zheng et al., 1996).
FSH stimulates ovarian cancer cell growth by interacting with the FSH receptor variant FSHR3. Unlike FSHR1, which primarily operates through cAMP signaling in reproductive cells, FSHR3 activates the MAPK/ERK pathway and increases calcium influx independent of cAMP (Li et al., 2007; Sullivan et al., 2013). In the ovarian cancer cell line ID8, studies have demonstrated that FSHR3 activation is associated with the proliferation of ovarian surface epithelial cells through ERK phosphorylation (Zheng et al., 1996). Inhibiting this pathway with the MEK inhibitor PD98059 reduces both ERK activation and cell proliferation, highlighting the significance of MAPK/ERK signaling in FSH-driven tumor growth (Zheng et al., 1996). Additionally, ERK1/2 activation via FSHR1 is implicated in promoting epithelial-mesenchymal transition in ovarian cancer cells, with epithelial growth factor playing a key role (Vergara et al., 2010).
OCT4 is a key regulator of stem cell pluripotency and self-renewal. Studies have shown that the dysregulation of OCT4 has been highly associated with the pathogenesis and advancement of various cancers. Overexpression of OCT4 has been observed in multiple cancers, including lung, prostate, liver, and cervical cancers, as well as ovarian cancer (Liu et al., 2011; Iki and Pour, 2006; Wang et al., 2010). In ovarian cancer, nuclear OCT4 overexpression has been reported in ovarian cells, further suggesting OCT4 involvement in ovarian cancer progression (Zhang et al., 2013).
In ovarian cancer, FSH upregulates OCT4 expression via the ERK1/2 signaling pathway, contributing to tumor progression (Liu et al., 2015). Elevated OCT4 promotes ovarian cancer cell proliferation and growth by upregulating bone morphogenetic protein 4 (BMP4) and interacting with Lin28 (Ma et al., 2013). Additionally, OCT4 overexpression in cancer stem cells increases the expression of Notch, SRY-Box transcription factor 2 (Sox2) and Nanog, which are crucial for stem cell proliferation and differentiation (Zhang et al., 2013).
OCT4 is also critical for promoting epithelial-mesenchymal transition in ovarian cancer cells. FSH-induced OCT4 overexpression increases Snail levels, leading to the downregulation of E-cadherin and the upregulation of N-cadherin, both of which are markers of epithelial-mesenchymal transition (Liu et al., 2015). Furthermore, OCT4 is involved in inhibiting apoptosis in ovarian cancer stem cells, linked to the activation of the AKT-survivin pathway (Hu et al., 2008). Increased OCT4 expression increases AKT and survivin levels, highlighting the importance of the OCT4-AKT-survivin axis in FSH-mediated apoptosis resistance (Zhang et al., 2013).
In addition to FSH-induced OCT4 upregulation that increases Snail expression, another pathway involving FSH binding to FSHR1 has been identified. This binding activates CREB via the Gαs-cAMP-PKA signaling pathway. PKA moves to the nucleus, where it phosphorylates CREB, allowing it to act as a transcription factor (Zhang et al., 2021). Phosphorylated CREB then boosts human Alk B homolog 5 (ALKBH5) expression, a demethylase that removes m6A modifications from Snail mRNA. This epigenetic change extends the half-life of Snail mRNA, enhancing its role in promoting epithelial-mesenchymal transition by downregulating E-cadherin and upregulating N-cadherin, aiding cancer metastasis (Wang et al., 2023; Xu et al., 2024; Aborisade et al., 2022).
Survivin, an inhibitor of apoptosis, is upregulated in various cancers, including ovarian cancer, and plays a critical role in cell proliferation (Kondapuram et al., 2023). Yan et al. discovered that FSH binding to FSHR1 on ovarian cancer cells increases survivin expression through the PI3K/AKT pathway. This rise in survivin boosts VEGF expression, promoting ovarian cancer cell proliferation (Huang et al., 2008). Additionally, FSH treatment raises cyclin D1 and cyclin E levels while reducing programmed cell death gene 6 (PDCD6) and death receptor 5 (DR5) levels (Chu et al., 2021; Montalto and De Amicis, 2020). Further investigations using RNA interference to knock down survivin have shown that the changes in cyclin D1, cyclin E, and DR5 are survivin-dependent, while PDCD6 regulation appears independent of survivin. Moreover, FSH-induced survivin upregulation and PDCD6 downregulation are indicated to be downstream to the PI3K/AKT and stress-activated protein kinase/Jun N-terminal kinase (SAPK/JNK) signaling pathway, which are activated by the FSH binding to FSHR1 (Huang et al., 2011) (Figure 3).
Figure 3. FSH signaling pathways involved in various mechanisms of ovarian cancer pathogenesis. Key molecules, extracellular signal-regulated kinase (ERK) (orange), octamer-binding transcription factor 4 (OCT4) (red), Snail (purple), and Survivin (blue), are highlighted to clearly delineate their downstream signaling roles in promoting tumor growth, metastasis, and anti-apoptosis. This figure illustrates the complex interactions between FSH receptors (FSHR1 and FSHR3) and various downstream effectors, leading to distinct cellular responses that contribute to the progression of ovarian cancer. Specifically, it showcases the roles of the mitogen-activated protein kinase (MAPK)/ERK pathway, OCT4 overexpression in epithelial-mesenchymal transition and cancer stem cell maintenance, Snail activation in metastasis, and Survivin-mediated resistance to apoptosis.
FSH contributes to breast cancer growth and survival by promoting cell migration and invasion. It achieves this by modulating the actin cytoskeleton through the upregulation of Moesin and focal adhesion kinase (FAK) via ROCK-2 activation, which is downstream of Gα13 signaling and a Gαi and c-Src-dependent pathway triggered by FSH binding to FSHR. These pathways reorganize the cytoskeleton, enhancing cancer cell motility (Sanchez et al., 2016).
Additionally, FSH increases chemoresistance in breast cancer cells to drugs like doxorubicin and cyclophosphamide. This resistance is driven by the activation of hypoxia-inducible factor-1α (HIF-1α), a key regulatory protein that promotes the expression of genes in angiogenesis and glycolysis, thereby boosting the resilience of cancer cells to hypoxia and chemotherapy. FSH activates the PI3K/AKT pathway through FSHR interaction with Gαq/11, subsequently resulting in the inhibition of prolyl hydroxylases and an increase in intracellular HIF-1α levels (Bergandi et al., 2019).
Currently, there is no established clinical therapy targeting FSH singling for cancer treatment. However, preclinical studies suggest that blocking FSH-FSHR signaling with either FSH antagonists or FSHR antibodies is effective and safe to eliminate ovarian cancer in animal models (Blaakaer et al., 1995; Bordoloi et al., 2022; Perales-Puchalt et al., 2017).
Given the numerous signaling pathways involved in FSH-induced ovarian cancer progression and survival, targeted therapies against OCT4, ERK, Snail, and PDCD6 are also under investigation. Blocking these pathways has shown promise in inhibiting tumor growth, metastasis, and apoptosis escape in ovarian cancer cells (Liang et al., 2023; Ashraf and Kumar, 2022; Hojo et al., 2018; Yuan et al., 2017). For example, using short hairpin RNA to knockdown Snail in ovarian cancer cell lines largely reduced the proliferation rate of cancer cells, as well as reversed their chemoresistance to cisplatin, providing a better treatment effect and prognosis with chemotherapies (Hojo et al., 2018). Another therapeutic strategy using miR-124 also showed promising effect in the treatment of ovarian cancer. And the underlying mechanism is that miR-124 suppresses the expression of PDCD6, thus inhibiting the invasion and apoptosis evasion of cancer cells (Yuan et al., 2017). Although the precise targeting of cancer cells remains challenging, advancements in nanotechnology, histological techniques, and a deeper understanding of cancer biology may enable these therapeutic strategies to be translated into clinical practice, offering new hope to patients with FSH-related ovarian cancer or even other cancers with these pathogenic pathways.
In the context of breast and prostate cancer, hormonal therapies that reduce estrogen and androgen levels, respectively, have been well established and have proven effective in limiting cancer progression. For breast cancer, methods downregulating estrogen level, such as estrogen receptor modulators, aromatase inhibitors and ovarian suppressors, are widely employed. In prostate cancer, androgen deprivation therapy is the standard treatment (Nabid et al., 2018; Elsamany et al., 2022). However, adverse effects associated with the upregulated FSH, arising from negative feedback mechanisms due to reduced estrogen or androgen levels, can significantly worsen the physiological conditions of patients. These effects may compromise the overall outcomes of primary cancer treatments and contribute to the development of complications, including osteoporosis, sexual dysfunction, infertility, and cardiovascular disease (Maughan et al., 2010; Nguyen et al., 2015). As a result, the exploration of adjuvant therapies that specifically target FSH regulation, in order to maintain its physiological balance, represents a potential area for future research.
However, the direct role of FSH in breast cancer and prostate cancer remains poorly understood, and no FSH-targeted therapies have been developed to date. Given the known interactions between FSH and estrogen or androgen pathways, future research may focus on targeting FSH to indirectly suppress tumor initiation or progression.
The non-reproductive effects of FSH and their role in the pathogenesis of various diseases have been increasingly elucidated, revealing complex interactions between FSH signaling and a multitude of biological processes in various tissues and organs. The signaling pathways activated by FSH binding to its receptor, FSHR, have been well-characterized in many contexts. However, due to the complexity of FSHRs and their underlying signaling pathways, some downstream pathways remain incompletely understood. For instance, the downstream pathways of FSHR3 in ovarian cancer is not fully established even with a clear result of enhanced cell proliferation. Future advancements in the understanding of FSH signaling and intracellular molecular interactions are expected to further clarify these mechanisms, potentially leading to more precise and accurate models of FSH-related signaling cascades.
Although we have illustrated some of the underlying signaling pathways downstream FSH-FSHR signaling in various non-reproductive cells, the complexity of FSH reactions and signaling in the whole human body remains poorly understood. Several studies indicate that elevated FSH levels do not significantly impact bone, adipose tissue, cholesterol metabolism, or other non-reproductive functions (Liu et al., 2017; Wu et al., 2021; Elenkov et al., 2023). However, these studies primarily focus on changes in FSH levels without considering additional variables such as age, physiological conditions, and the levels of other hormones. Such gaps in endocrinological research highlight the necessity for comprehensive investigations into the effects of FSH within the entire organism.
Another critical area that underscores the importance of understanding FSH in the broader context is its role in male non-reproductive diseases, which remains inadequately explored. Although some studies suggest that elevated FSH may exacerbate several conditions, including cardiovascular disease, type 2 diabetes mellitus, and prostate cancer, the underlying mechanisms are often linked to the amplification of androgen effects resulting from FSH-androgen interactions (Wittert et al., 2021; Oduwole et al., 2021; Duivenvoorden et al., 2024; Shafi et al., 2013).
Additionally, while FSH influences various physiological processes that may, in turn, affect its own function, external factors can also modulate FSH levels. For instance, we previously noted that FSH may promote fat accumulation; however, a 2007 clinical trial involving Asian women found that lower FSH levels were associated with higher body mass index in postmenopausal women (Ausmanas et al., 2007). This condition may be attributed to excessive adipose tissue producing estrogen via aromatization, which exerts negative feedback on FSH production and secretion (De et al., 2006). Furthermore, estrogen from ovarian sources also complicates the interpretation of FSH functions, as FSH and estrogen levels are often interrelated under physiological conditions. Although many studies have demonstrated that FSH can perform non-reproductive functions independent of estrogen, the influence of estrogen on overall health cannot be overlooked. As research continues to delineate the effects of FSH on various non-reproductive tissues or cells, we might acquire a more comprehensive understanding of the FSH role in overall health.
Eventually, various treatments targeting FSH signaling, including FSH antibodies, FSHR blockade, and hormone replacement therapies, have been extensively studied across a range of diseases, with some now advancing to clinical trials. In conditions strongly associated with elevated FSH, such as osteoporosis, obesity, and Alzheimer’s disease, FSH-targeted therapies have shown significant promise. However, in diseases where FSH elevation is not a primary driver of pathogenesis, such as cardiovascular disease and certain cancers, FSH-suppressing treatments have not demonstrated clear therapeutic efficacy. This observation may suggest that FSH plays a less significant role in the development of these conditions. Nonetheless, FSH modulation may still hold potential as an adjunctive therapy, enhancing the efficacy of existing treatments and contributing to better clinical outcomes. This approach underscores the importance of precision medicine in the ongoing development of targeted therapies. To summarize, treatment strategies targeting FSH-FSHR signaling hold strong potential in both disease prevention and therapeutic interventions.
CL: Visualization, Writing–original draft, Writing–review and editing. YL: Resources, Validation, Visualization, Writing–review and editing. HK: Project administration, Resources, Validation, Visualization, Writing–review and editing.
The author(s) declare that no financial support was received for the research, authorship, and/or publication of this article.
The authors declare that the research was conducted in the absence of any commercial or financial relationships that could be construed as a potential conflict of interest.
The author(s) declare that no Generative AI was used in the creation of this manuscript.
All claims expressed in this article are solely those of the authors and do not necessarily represent those of their affiliated organizations, or those of the publisher, the editors and the reviewers. Any product that may be evaluated in this article, or claim that may be made by its manufacturer, is not guaranteed or endorsed by the publisher.
Aborisade, A., Akinyele, A., Aregbesola, B., Adesina, O., and Ladeji, A. (2022). Immunohistochemical expression of E-cadherin, N-cadherin and Snail/slug in ameloblastoma. J. Stomatol. Oral Maxillofac. Surg. 123 (6), e801–e807. doi:10.1016/j.jormas.2022.05.026
Akhter, M. P., Lappe, J. M., Davies, K. M., and Recker, R. R. (2007). Transmenopausal changes in the trabecular bone structure. Bone 41 (1), 111–116. doi:10.1016/j.bone.2007.03.019
Alberti, K. G. M. M., Zimmet, P., and Shaw, J.IDF Epidemiology Task Force Consensus Group (2005). The metabolic syndrome--a new worldwide definition. Lancet 366 (9491), 1059–1062. doi:10.1016/S0140-6736(05)67402-8
Ashraf, R., and Kumar, S. (2022). Mfn2-mediated mitochondrial fusion promotes autophagy and suppresses ovarian cancer progression by reducing ROS through AMPK/mTOR/ERK signaling. Cell Mol. Life Sci. 79 (11), 573. doi:10.1007/s00018-022-04595-6
Ausmanas, M. K., Tan, D. A., Jaisamrarn, U., Tian, X. W., and Holinka, C. F. (2007). Estradiol, FSH and LH profiles in nine ethnic groups of postmenopausal Asian women: the Pan-Asia Menopause (PAM) study. Climacteric 10 (5), 427–437. doi:10.1080/13697130701610780
Bergamini, M., Dalla Volta, A., Palumbo, C., Zamboni, S., Triggiani, L., Zamparini, M., et al. (2024). Relationship between circulating FSH levels and body composition and bone health in patients with prostate cancer who undergo androgen deprivation therapy: the BLADE study. Elife 13, e92655. doi:10.7554/eLife.92655
Bergandi, L., Canosa, S., Pittatore, G., Silvagno, F., Doublier, S., Gennarelli, G., et al. (2019). Human recombinant FSH induces chemoresistance in human breast cancer cells via HIF-1α activation. Biol. Reprod. 100 (6), 1521–1535. doi:10.1093/biolre/ioz050
Bernard, D. J., Fortin, J., Wang, Y., and Lamba, P. (2010). Mechanisms of FSH synthesis: what we know, what we don't, and why you should care. Fertil. Steril. 93 (8), 2465–2485. doi:10.1016/j.fertnstert.2010.03.034
Blaakaer, J., Baeksted, M., Micic, S., Albrectsen, P., Rygaard, J., and Bock, J. (1995). Gonadotropin-releasing hormone agonist suppression of ovarian tumorigenesis in mice of the Wx/Wv genotype. Biol. Reprod. 53 (4), 775–779. doi:10.1095/biolreprod53.4.775
Blander, C. L. (2006). Estrogens and breast cancer. N. Engl. J. Med. 354 (15), 1647–1648. doi:10.1056/NEJMc060364
Bordoloi, D., Bhojnagarwala, P. S., Perales-Puchalt, A., Kulkarni, A. J., Zhu, X., Liaw, K., et al. (2022). A mAb against surface-expressed FSHR engineered to engage adaptive immunity for ovarian cancer immunotherapy. JCI Insight 7 (22), e162553. doi:10.1172/jci.insight.162553
Bowen, R. L., Isley, J. P., and Atkinson, R. L. (2000). An association of elevated serum gonadotropin concentrations and Alzheimer disease? J. Neuroendocrinol. 12 (4), 351–354. doi:10.1046/j.1365-2826.2000.00461.x
Cannarella, R., Barbagallo, F., Condorelli, R. A., Aversa, A., La Vignera, S., and Calogero, A. E. (2019). Osteoporosis from an endocrine perspective: the role of hormonal changes in the elderly. J. Clin. Med. 8 (10), 1564. doi:10.3390/jcm8101564
Casarini, L., and Crepieux, P. (2019). Molecular mechanisms of action of FSH. Front. Endocrinol. (Lausanne) 10, 305. doi:10.3389/fendo.2019.00305
Celestino, C.D. S. D., Nogueira De Almeida, V. A., Cleto, M. C. J., De Oliveira Cipriano Torres, D., Oliveira Dos Santos, A. C., De Lima Ferreira Fernandes, C. H., et al. (2013). Endogenous sex hormones are not associated with subclinical atherosclerosis in menopausal women. Minerva Ginecol. 65 (3), 297–302.
Chen, H., Cui, Y., and Yu, S. (2018). Expression and localisation of FSHR, GHR and LHR in different tissues and reproductive organs of female yaks. Folia Morphol. Warsz. 77 (2), 301–309. doi:10.5603/FM.a2016.0095
Cheng, Y., Zhu, H., Ren, J., Wu, H.-Y., Yu, J.-E., Jin, L.-Y., et al. (2023). Follicle-stimulating hormone orchestrates glucose-stimulated insulin secretion of pancreatic islets. Nat. Commun. 14 (1), 6991. doi:10.1038/s41467-023-42801-6
Chu, C., Geng, Y., Zhou, Y., and Sicinski, P. (2021). Cyclin E in normal physiology and disease states. Trends Cell Biol. 31 (9), 732–746. doi:10.1016/j.tcb.2021.05.001
Crowther, N. J., and Norris, S. A. (2012). The current waist circumference cut point used for the diagnosis of metabolic syndrome in sub-Saharan African women is not appropriate. PLoS One 7 (11), e48883. doi:10.1371/journal.pone.0048883
Cui, H., Zhao, G., Liu, R., Zheng, M., Chen, J., and Wen, J. (2012). FSH stimulates lipid biosynthesis in chicken adipose tissue by upregulating the expression of its receptor FSHR. J. Lipid Res. 53 (5), 909–917. doi:10.1194/jlr.M025403
Cypess, A. M., Lehman, S., Williams, G., Tal, I., Rodman, D., Goldfine, A. B., et al. (2009). Identification and importance of brown adipose tissue in adult humans. N. Engl. J. Med. 360 (15), 1509–1517. doi:10.1056/NEJMoa0810780
Damluji, A. A., Alfaraidhy, M., AlHajri, N., Rohant, N. N., Kumar, M., Al, M. C., et al. (2023). Sarcopenia and cardiovascular diseases. Circulation 147 (20), 1534–1553. doi:10.1161/CIRCULATIONAHA.123.064071
Davis, S. R., Castelo-Branco, C., Chedraui, P., Lumsden, M. A., Nappi, R. E., Shah, D., et al. (2012). Understanding weight gain at menopause. Climacteric 15 (5), 419–429. doi:10.3109/13697137.2012.707385
De, P. G., Maldera, S., Tartagni, M., Pannacciulli, N., Loverro, G., and Giorgino, R. (2006). Inhibitory effect of obesity on gonadotropin, estradiol, and inhibin B levels in fertile women. Obes. (Silver Spring) 14 (11), 1954–1960. doi:10.1038/oby.2006.228
Desai, K., McManus, J. M., and Sharifi, N. (2021). Hormonal therapy for prostate cancer. Endocr. Rev. 42 (3), 354–373. doi:10.1210/endrev/bnab002
Dizeyi, N., Trzybulska, D., Al-Jebari, Y., Huhtaniemi, I., and Lundberg Giwercman, Y. (2019). Cell-based evidence regarding the role of FSH in prostate cancer. Urol. Oncol. 37 (4), e1–e290. doi:10.1016/j.urolonc.2018.12.011
Donato, G. B., Fuchs, S. C., Oppermann, K., Bastos, C., and Spritzer, P. M. (2006). Association between menopause status and central adiposity measured at different cutoffs of waist circumference and waist-to-hip ratio. Menopause 13 (2), 280–285. doi:10.1097/01.gme.0000177907.32634.ae
Douchi, T., Yamamoto, S., Yoshimitsu, N., Andoh, T., Matsuo, T., and Nagata, Y. (2002). Relative contribution of aging and menopause to changes in lean and fat mass in segmental regions. Maturitas 42 (4), 301–306. doi:10.1016/s0378-5122(02)00161-5
Duivenvoorden, W. C. M., Margel, D., Subramony Gayathri, V., Duceppe, E., Yousef, S., Naeim, M., et al. (2024). Follicle-stimulating hormone exacerbates cardiovascular disease in the presence of low or castrate testosterone levels. JACC Basic Transl. Sci. 9 (3), 364–379. doi:10.1016/j.jacbts.2023.10.010
Elenkov, A., Wirestrand, E., Hagsund, A., Huhtaniemi, I., Giwercman, Y. L., and Giwercman, A. (2023). Non-reproductive effects of follicle-stimulating hormone in young men. Andrology 11 (3), 471–477. doi:10.1111/andr.13335
El Khoudary, S. R. (2017). Gaps, limitations and new insights on endogenous estrogen and follicle stimulating hormone as related to risk of cardiovascular disease in women traversing the menopause: a narrative review. Maturitas 104, 44–53. doi:10.1016/j.maturitas.2017.08.003
El Khoudary, S. R., Aggarwal, B., Beckie, T. M., Hodis, H. N., Johnson, A. E., Langer, R. D., et al. (2020). Menopause transition and cardiovascular disease risk: implications for timing of early prevention: a scientific statement from the American heart association. Circulation 142 (25), e506–e532. doi:10.1161/CIR.0000000000000912
El Khoudary, S. R., Wildman, R. P., Matthews, K., Thurston, R. C., Bromberger, J. T., and Sutton-Tyrrell, K. (2012). Endogenous sex hormones impact the progression of subclinical atherosclerosis in women during the menopausal transition. Atherosclerosis 225 (1), 180–186. doi:10.1016/j.atherosclerosis.2012.07.025
Elsamany, S. A., Alghanmi, H., Albaradei, A., Abdelhamid, R., Madi, E., and Ramzan, A. (2022). Assessment of compliance with hormonal therapy in early breast cancer patients with positive hormone receptor phenotype: a single institution study. Breast 62, 69–74. doi:10.1016/j.breast.2022.01.017
Floyd, Z. E., Gawronska-Kozak, B., Tam, C. S., and Gimble, J. M. (2016). Mechanisms of metabolism, aging and obesity. Biochimie 124, 1–2. doi:10.1016/j.biochi.2016.03.005
Gallagher, C. M., Moonga, B. S., and Kovach, J. S. (2010). Cadmium, follicle-stimulating hormone, and effects on bone in women age 42-60 years, NHANES III. Environ. Res. 110 (1), 105–111. doi:10.1016/j.envres.2009.09.012
Gavaler, J. S., and Rosenblum, E. (2003). Predictors of postmenopausal body mass index and waist hip ratio in the Oklahoma postmenopausal health disparities study. J. Am. Coll. Nutr. 22 (4), 269–276. doi:10.1080/07315724.2003.10719303
Gera, S., Kuo, T.-C., Gumerova, A. A., Korkmaz, F., Sant, D., DeMambro, V., et al. (2022). FSH-blocking therapeutic for osteoporosis. Elife 11, e78022. doi:10.7554/eLife.78022
Ghinea, N., Liehn, E. A., Grommes, J., Delattre, D. D., and Olesen, T. K. (2024). Follicle-stimulating hormone receptor expression in advanced atherosclerotic plaques. Sci. Rep. 14 (1), 10176. doi:10.1038/s41598-024-60962-2
Giovanelli, L., Quinton, R., Cangiano, B., Colombo, S., Persani, L., Bonomi, M., et al. (2022). FSH and bone: comparison between males with central versus primary hypogonadism. Front. Endocrinol. 13, 939897. doi:10.3389/fendo.2022.939897
Gorai, I., Chaki, O., Nakayama, M., and Minaguchi, H. (1995). Urinary biochemical markers for bone resorption during the menstrual cycle. Calcif. Tissue Int. 57 (2), 100–104. doi:10.1007/BF00298428
Greenbaum, L. E., Li, W., Cressman, D. E., Peng, Y., Ciliberto, G., Poli, V., et al. (1998). CCAAT enhancer-binding protein beta is required for normal hepatocyte proliferation in mice after partial hepatectomy. J. Clin. Invest. 102 (5), 996–1007. doi:10.1172/JCI3135
Greenhill, C. (2017). Adipose tissue: FSH antibody prevents obesity and loss of bone. Nat. Rev. Endocrinol. 13 (7), 375. doi:10.1038/nrendo.2017.70
Grodstein, F., Manson, J. E., Colditz, G. A., Willett, W. C., Speizer, F. E., and Stampfer, M. J. (2000). A prospective, observational study of postmenopausal hormone therapy and primary prevention of cardiovascular disease. Ann. Intern Med. 133 (12), 933–941. doi:10.7326/0003-4819-133-12-200012190-00008
Guo, Y., Zhao, M., Bo, T., Ma, S., Yuan, Z., Chen, W., et al. (2019). Blocking FSH inhibits hepatic cholesterol biosynthesis and reduces serum cholesterol. Cell Res. 29 (2), 151–166. doi:10.1038/s41422-018-0123-6
Halperin, R., Hadas, E., Langer, R., Bukovsky, I., and Schneider, D. (1999). Peritoneal fluid gonadotropins and ovarian hormones in patients with ovarian cancer. Int. J. Gynecol. Cancer 9 (6), 502–507. doi:10.1046/j.1525-1438.1999.99075.x
Henderson, B. E., Paganini-Hill, A., and Ross, R. K. (1991). Decreased mortality in users of estrogen replacement therapy. Arch. Intern Med. 151 (1), 75–78. doi:10.1001/archinte.151.1.75
Hojo, N., Huisken, A. L., Wang, H., Chirshev, E., Kim, N. S., Nguyen, S. M., et al. (2018). Snail knockdown reverses stemness and inhibits tumour growth in ovarian cancer. Sci. Rep. 8 (1), 8704. doi:10.1038/s41598-018-27021-z
Hu, T., Liu, S., Breiter, D. R., Wang, F., Tang, Y., and Sun, S. (2008). Octamer 4 small interfering RNA results in cancer stem cell-like cell apoptosis. Cancer Res. 68 (16), 6533–6540. doi:10.1158/0008-5472.CAN-07-6642
Huang, Y., Hua, K., Zhou, X., Jin, H., Chen, X., Lu, X., et al. (2008). Activation of the PI3K/AKT pathway mediates FSH-stimulated VEGF expression in ovarian serous cystadenocarcinoma. Cell Res. 18 (7), 780–791. doi:10.1038/cr.2008.70
Huang, Y., Jin, H., Liu, Y., Zhou, J., Ding, J., Cheng, K. W., et al. (2011). FSH inhibits ovarian cancer cell apoptosis by up-regulating survivin and down-regulating PDCD6 and DR5. Endocr. Relat. Cancer 18 (1), 13–26. doi:10.1677/ERC-09-0308
Huo, X., Clarke, R., Halsey, J., Jackson, R., Lehman, A., Prince, R., et al. (2023). Calcium supplements and risk of CVD: a meta-analysis of randomized trials. Curr. Dev. Nutr. 7 (3), 100046. doi:10.1016/j.cdnut.2023.100046
Iki, K., and Pour, P. M. (2006). Expression of Oct4, a stem cell marker, in the hamster pancreatic cancer model. Pancreatology 6 (4), 406–413. doi:10.1159/000094317
Jaff, N. G., Snyman, T., Norris, S. A., and Crowther, N. J. (2014). Staging reproductive aging using stages of reproductive aging workshop + 10 in black urban african women in the study of women entering and in endocrine transition. Menopause 21 (11), 1225–1233. doi:10.1097/GME.0000000000000235
Kawai, H., Furuhashi, M., and Suganuma, N. (2004). Serum follicle-stimulating hormone level is a predictor of bone mineral density in patients with hormone replacement therapy. Arch. Gynecol. Obstet. 269 (3), 192–195. doi:10.1007/s00404-003-0532-7
Kondapuram, S. K., Ramachandran, H. K., Arya, H., and Coumar, M. S. (2023). Targeting survivin for cancer therapy: strategies, small molecule inhibitors and vaccine based therapeutics in development. Life Sci. 335, 122260. doi:10.1016/j.lfs.2023.122260
LeBlanc, E. S., Kapphahn, K., Hedlin, H., Desai, M., Parikh, N. I., Liu, S., et al. (2017). Reproductive history and risk of type 2 diabetes mellitus in postmenopausal women: findings from the Women's Health Initiative. Menopause 24 (1), 64–72. doi:10.1097/GME.0000000000000714
Li, C.-W., Yu, K., Shyh-Chang, N., Jiang, Z., Liu, T., Ma, S., et al. (2022). Pathogenesis of sarcopenia and the relationship with fat mass: descriptive review. J. Cachexia Sarcopenia Muscle 13 (2), 781–794. doi:10.1002/jcsm.12901
Li, G., Zhang, L., Wang, D., Aiqudsy, L., Jiang, J. X., Xu, H., et al. (2019). Muscle-bone crosstalk and potential therapies for sarco-osteoporosis. J. Cell Biochem. 120 (9), 14262–14273. doi:10.1002/jcb.28946
Li, X., Chen, W., Li, P., Wei, J., Cheng, Y., Liu, P., et al. (2017). Follicular stimulating hormone accelerates atherogenesis by increasing endothelial VCAM-1 expression. Theranostics 7 (19), 4671–4688. doi:10.7150/thno.21216
Li, Y., Ganta, S., Cheng, C., Craig, R., Ganta, R. R., and Freeman, L. C. (2007). FSH stimulates ovarian cancer cell growth by action on growth factor variant receptor. Mol. Cell Endocrinol. 267 (1-2), 26–37. doi:10.1016/j.mce.2006.11.010
Liang, K., Pan, X., Chen, Y., and Huang, S. (2023). Anti-ovarian cancer actions and pharmacological targets of plumbagin. Naunyn Schmiedeb. Arch. Pharmacol. 396 (6), 1205–1210. doi:10.1007/s00210-023-02393-w
Lin, S.-W., and Ge, W. (2009). Differential regulation of gonadotropins (FSH and LH) and growth hormone (GH) by neuroendocrine, endocrine, and paracrine factors in the zebrafish--an in vitro approach. Gen. Comp. Endocrinol. 160 (2), 183–193. doi:10.1016/j.ygcen.2008.11.020
Liu, D., Zhou, P., Zhang, L., Wu, G., Zheng, Y., and He, F. (2011). Differential expression of Oct4 in HPV-positive and HPV-negative cervical cancer cells is not regulated by DNA methyltransferase 3A. Tumour Biol. 32 (5), 941–950. doi:10.1007/s13277-011-0196-z
Liu, L., Zhang, J., Fang, C., Zhang, Z., Feng, Y., and Xi, X. (2015). OCT4 mediates FSH-induced epithelial-mesenchymal transition and invasion through the ERK1/2 signaling pathway in epithelial ovarian cancer. Biochem. Biophys. Res. Commun. 461 (3), 525–532. doi:10.1016/j.bbrc.2015.04.061
Liu, P., Ji, Y., Yuen, T., Rendina-Ruedy, E., DeMambro, V. E., Dhawan, S., et al. (2017). Blocking FSH induces thermogenic adipose tissue and reduces body fat. Nature 546 (7656), 107–112. doi:10.1038/nature22342
Ma, W., Ma, J., Xu, J., Qiao, C., Branscum, A., Cardenas, A., et al. (2013). Lin28 regulates BMP4 and functions with Oct4 to affect ovarian tumor microenvironment. Cell Cycle 12 (1), 88–97. doi:10.4161/cc.23028
Marlatt, K. L., and Ravussin, E. (2017). Brown adipose tissue: an update on recent findings. Curr. Obes. Rep. 6 (4), 389–396. doi:10.1007/s13679-017-0283-6
Matyi, J. M., Rattinger, G. B., Schwartz, S., Buhusi, M., and Tschanz, J. T. (2019). Lifetime estrogen exposure and cognition in late life: the Cache County Study. Menopause 26 (12), 1366–1374. doi:10.1097/GME.0000000000001405
Maughan, K. L., Lutterbie, M. A., and Ham, P. S. (2010). Treatment of breast cancer. Am. Fam. Physician 81 (11), 1339–1346.
Montalto, F. I., and De Amicis, F. (2020). Cyclin D1 in cancer: a molecular connection for cell cycle control, adhesion and invasion in tumor and stroma. Cells 9 (12), 2648. doi:10.3390/cells9122648
Moore, K. J., Sheedy, F. J., and Fisher, E. A. (2013). Macrophages in atherosclerosis: a dynamic balance. Nat. Rev. Immunol. 13 (10), 709–721. doi:10.1038/nri3520
Mosconi, L., Berti, V., Quinn, C., McHugh, P., Petrongolo, G., Varsavsky, I., et al. (2017). Sex differences in Alzheimer risk: brain imaging of endocrine vs chronologic aging. Neurology 89 (13), 1382–1390. doi:10.1212/WNL.0000000000004425
Mulnard, R. A., Cotman, C. W., Kawas, C., van Dyck, C. H., Sano, M., Doody, R., et al. (2000). Estrogen replacement therapy for treatment of mild to moderate Alzheimer disease: a randomized controlled trial. Alzheimer's Disease Cooperative Study. JAMA 283 (8), 1007–1015. doi:10.1001/jama.283.8.1007
Munir, J. A., Wu, H., Bauer, K., Bindeman, J., Byrd, C., Feuerstein, I. M., et al. (2012). The perimenopausal atherosclerosis transition: relationships between calcified and noncalcified coronary, aortic, and carotid atherosclerosis and risk factors and hormone levels. Menopause 19 (1), 10–15. doi:10.1097/gme.0b013e318221bc8d
Nabid, A., Carrier, N., Martin, A.-G., Bahary, J.-P., Lemaire, C., Vass, S., et al. (2018). Duration of androgen deprivation therapy in high-risk prostate cancer: a randomized phase III trial. Eur. Urol. 74 (4), 432–441. doi:10.1016/j.eururo.2018.06.018
Nguyen, P. L., Alibhai, S. M. H., Basaria, S., D'Amico, A. V., Kantoff, P. W., Keating, N. L., et al. (2015). Adverse effects of androgen deprivation therapy and strategies to mitigate them. Eur. Urol. 67 (5), 825–836. doi:10.1016/j.eururo.2014.07.010
Oduwole, O. O., Huhtaniemi, I. T., and Misrahi, M. (2021). The roles of luteinizing hormone, follicle-stimulating hormone and testosterone in spermatogenesis and folliculogenesis revisited. Int. J. Mol. Sci. 22 (23), 12735. doi:10.3390/ijms222312735
Paschou, S. A., Anagnostis, P., Pavlou, D. I., Vryonidou, A., Goulis, D. G., and Lambrinoudaki, I. (2019). Diabetes in menopause: risks and management. Curr. Vasc. Pharmacol. 17 (6), 556–563. doi:10.2174/1570161116666180625124405
Perales-Puchalt, A., Svoronos, N., Rutkowski, M. R., Allegrezza, M. J., Tesone, A. J., Payne, K. K., et al. (2017). Follicle-stimulating hormone receptor is expressed by most ovarian cancer subtypes and is a safe and effective immunotherapeutic target. Clin. Cancer Res. 23 (2), 441–453. doi:10.1158/1078-0432.CCR-16-0492
Pertusa, M., Morenilla-Palao, C., Carteron, C., Viana, F., and Cabedo, H. (2007). Transcriptional control of cholesterol biosynthesis in Schwann cells by axonal neuregulin 1. J. Biol. Chem. 282 (39), 28768–28778. doi:10.1074/jbc.M701878200
Poli, V. (1998). The role of C/EBP isoforms in the control of inflammatory and native immunity functions. J. Biol. Chem. 273 (45), 29279–29282. doi:10.1074/jbc.273.45.29279
Rendina, D., Gianfrancesco, F., De Filippo, G., Merlotti, D., Esposito, T., Mingione, A., et al. (2010). FSHR gene polymorphisms influence bone mineral density and bone turnover in postmenopausal women. Eur. J. Endocrinol. 163 (1), 165–172. doi:10.1530/EJE-10-0043
Rossouw, J. E., Anderson, G. L., Prentice, R. L., LaCroix, A. Z., Kooperberg, C., Stefanick, M. L., et al. (2002). Risks and benefits of estrogen plus progestin in healthy postmenopausal women: principal results from the Women's Health Initiative randomized controlled trial. JAMA 288 (3), 321–333. doi:10.1001/jama.288.3.321
Sanchez, A. M., Flamini, M. I., Russo, E., Casarosa, E., Pacini, S., Petrini, M., et al. (2016). LH and FSH promote migration and invasion properties of a breast cancer cell line through regulatory actions on the actin cytoskeleton. Mol. Cell Endocrinol. 437, 22–34. doi:10.1016/j.mce.2016.08.009
Santoro, N., Epperson, C. N., and Mathews, S. B. (2015). Menopausal symptoms and their management. Endocrinol. Metab. Clin. North Am. 44 (3), 497–515. doi:10.1016/j.ecl.2015.05.001
Santoro, N., Roeca, C., Peters, B. A., and Neal-Perry, G. (2021). The menopause transition: signs, symptoms, and management options. J. Clin. Endocrinol. Metabolism 106 (1), 1–15. doi:10.1210/clinem/dgaa764
Schildkraut, J. M., Cooper, G. S., Halabi, S., Calingaert, B., Hartge, P., and Whittemore, A. S. (2001). Age at natural menopause and the risk of epithelial ovarian cancer. Obstet. Gynecol. 98 (1), 85–90. doi:10.1016/s0029-7844(01)01388-6
Shafi, A. A., Yen, A. E., and Weigel, N. L. (2013). Androgen receptors in hormone-dependent and castration-resistant prostate cancer. Pharmacol. & Ther. 140 (3), 223–238. doi:10.1016/j.pharmthera.2013.07.003
Shaw, P., Dwivedi, S. K. D., Bhattacharya, R., Mukherjee, P., and Rao, G. (2024). VEGF signaling: role in angiogenesis and beyond. Biochim. Biophys. Acta Rev. Cancer 1879 (2), 189079. doi:10.1016/j.bbcan.2024.189079
Short, R. A., Bowen, R. L., O'Brien, P. C., and Graff-Radford, N. R. (2001). Elevated gonadotropin levels in patients with Alzheimer disease. Mayo Clin. Proc. 76 (9), 906–909. doi:10.4065/76.9.906
Shumaker, S. A., Legault, C., Kuller, L., Rapp, S. R., Thal, L., Lane, D. S., et al. (2004). Conjugated equine estrogens and incidence of probable dementia and mild cognitive impairment in postmenopausal women: women's Health Initiative Memory Study. JAMA 291 (24), 2947–2958. doi:10.1001/jama.291.24.2947
Simoni, M., Nieschlag, E., and Gromoll, J. (2002). Isoforms and single nucleotide polymorphisms of the FSH receptor gene: implications for human reproduction. Hum. Reprod. Update 8 (5), 413–421. doi:10.1093/humupd/8.5.413
Song, Y., Wang, E.-S., Xing, L.-L., Shi, S., Qu, F., Zhang, D., et al. (2016). Follicle-stimulating hormone induces postmenopausal dyslipidemia through inhibiting hepatic cholesterol metabolism. J. Clin. Endocrinol. Metabolism 101 (1), 254–263. doi:10.1210/jc.2015-2724
Sowers, M., Zheng, H., Tomey, K., Karvonen-Gutierrez, C., Jannausch, M., Li, X., et al. (2007). Changes in body composition in women over six years at midlife: ovarian and chronological aging. J. Clin. Endocrinol. Metabolism 92 (3), 895–901. doi:10.1210/jc.2006-1393
Sternfeld, B., Quesenberry, C. P., and Husson, G. (1999). Habitual physical activity and menopausal symptoms: a case-control study. J. Womens Health 8 (1), 115–123. doi:10.1089/jwh.1999.8.115
Stilley, J. A., Guan, R., Duffy, D. M., and Segaloff, D. L. (2014). Signaling through FSH receptors on human umbilical vein endothelial cells promotes angiogenesis. J. Clin. Endocrinol. Metabolism 99 (5), E813–E820. doi:10.1210/jc.2013-3186
Straccia, M., Gresa-Arribas, N., Dentesano, G., Ejarque-Ortiz, A., Tusell, J. M., Serratosa, J., et al. (2011). Pro-inflammatory gene expression and neurotoxic effects of activated microglia are attenuated by absence of CCAAT/enhancer binding protein β. J. Neuroinflammation 8, 156. doi:10.1186/1742-2094-8-156
Sullivan, R. R., Faris, B. R., Eborn, D., Grieger, D. M., Cino-Ozuna, A. G., and Rozell, T. G. (2013). Follicular expression of follicle stimulating hormone receptor variants in the Ewe. Reprod. Biol. Endocrinol. 11, 113. doi:10.1186/1477-7827-11-113
Sun, L., Peng, Y., Sharrow, A. C., Iqbal, J., Zhang, Z., Papachristou, D. J., et al. (2006). FSH directly regulates bone mass. Cell 125 (2), 247–260. doi:10.1016/j.cell.2006.01.051
Sutton-Tyrrell, K., Zhao, X., Santoro, N., Lasley, B., Sowers, M., Johnston, J., et al. (2010). Reproductive hormones and obesity: 9 years of observation from the study of women's health across the nation. Am. J. Epidemiol. 171 (11), 1203–1213. doi:10.1093/aje/kwq049
Taneja, C., Gera, S., Kim, S. M., Iqbal, J., Yuen, T., and Zaidi, M. (2019). FSH-metabolic circuitry and menopause. J. Mol. Endocrinol. 63 (3), R73-R80–R80. doi:10.1530/JME-19-0152
Tatulashvili, S., Gusto, G., Cosson, E., Balkau, B., Gourdy, P., Bonnet, F., et al. (2021). Gonadal hormonal factors before menopause and incident type 2 diabetes in women: a 22-year follow-up of 83 799 women from the E3N cohort study. J. Diabetes 13 (4), 330–338. doi:10.1111/1753-0407.13129
Thomson, C. A., Aragaki, A. K., Prentice, R. L., Stefanick, M. L., Manson, J. E., Wactawski-Wende, J., et al. (2024). Long-term effect of randomization to calcium and vitamin D supplementation on health in older women: postintervention follow-up of a randomized clinical trial. Ann. Intern Med. 177 (4), 428–438. doi:10.7326/M23-2598
Vergara, D., Merlot, B., Lucot, J.-P., Collinet, P., Vinatier, D., Fournier, I., et al. (2010). Epithelial-mesenchymal transition in ovarian cancer. Cancer Lett. 291 (1), 59–66. doi:10.1016/j.canlet.2009.09.017
Vryonidou, A., Paschou, S. A., Muscogiuri, G., Orio, F., and Goulis, D. G. (2015). Mechanisms in endocrinology: metabolic syndrome through the female life cycle. Eur. J. Endocrinol. 173 (5), R153–R163. doi:10.1530/EJE-15-0275
Wang, X. Q., Ongkeko, W. M., Chen, L., Yang, Z. F., Lu, P., Chen, K. K., et al. (2010). Octamer 4 (Oct4) mediates chemotherapeutic drug resistance in liver cancer cells through a potential Oct4-AKT-ATP-binding cassette G2 pathway. Hepatology 52 (2), 528–539. doi:10.1002/hep.23692
Wang, Y., Wang, Y., Patel, H., Chen, J., Wang, J., Chen, Z.-S., et al. (2023). Epigenetic modification of m6A regulator proteins in cancer. Mol. Cancer 22 (1), 102. doi:10.1186/s12943-023-01810-1
Wang, Z.-H., Gong, K., Liu, X., Zhang, Z., Sun, X., Wei, Z. Z., et al. (2018). C/EBPβ regulates delta-secretase expression and mediates pathogenesis in mouse models of Alzheimer's disease. Nat. Commun. 9 (1), 1784. doi:10.1038/s41467-018-04120-z
Wittert, G., Bracken, K., Robledo, K. P., Grossmann, M., Yeap, B. B., Handelsman, D. J., et al. (2021). Testosterone treatment to prevent or revert type 2 diabetes in men enrolled in a lifestyle programme (T4DM): a randomised, double-blind, placebo-controlled, 2-year, phase 3b trial. Lancet Diabetes Endocrinol. 9 (1), 32–45. doi:10.1016/S2213-8587(20)30367-3
Wu, K. C., Ewing, S. K., Li, X., Sigurðsson, S., Guðnason, V., Kado, D. M., et al. (2021). FSH level and changes in bone mass and body composition in older women and men. J. Clin. Endocrinol. Metabolism 106 (10), 2876–2889. doi:10.1210/clinem/dgab481
Xiong, J., Kang, S. S., Wang, M., Wang, Z., Xia, Y., Liao, J., et al. (2023). FSH and ApoE4 contribute to Alzheimer's disease-like pathogenesis via C/EBPβ/δ-secretase in female mice. Nat. Commun. 14 (1), 6577. doi:10.1038/s41467-023-42282-7
Xiong, J., Kang, S. S., Wang, Z., Liu, X., Kuo, T.-C., Korkmaz, F., et al. (2022). FSH blockade improves cognition in mice with Alzheimer's disease. Nature 603 (7901), 470–476. doi:10.1038/s41586-022-04463-0
Xu, X., Zhuang, X., Yu, H., Li, P., Li, X., Lin, H., et al. (2024). FSH induces EMT in ovarian cancer via ALKBH5-regulated Snail m6A demethylation. Theranostics 14 (5), 2151–2166. doi:10.7150/thno.94161
Yager, J. D., and Davidson, N. E. (2006). Estrogen carcinogenesis in breast cancer. N. Engl. J. Med. 354 (3), 270–282. doi:10.1056/NEJMra050776
Yang, K., Hou, R., Zhao, J., Wang, X., Wei, J., Pan, X., et al. (2023). Lifestyle effects on aging and CVD: a spotlight on the nutrient-sensing network. Ageing Res. Rev. 92, 102121. doi:10.1016/j.arr.2023.102121
Yeh, J. K., Chen, M. M., and Aloia, J. F. (1996). Ovariectomy-induced high turnover in cortical bone is dependent on pituitary hormone in rats. Bone 18 (5), 443–450. doi:10.1016/8756-3282(96)00045-2
Yuan, L., Li, S., Zhou, Q., Wang, D., Zou, D., Shu, J., et al. (2017). MiR-124 inhibits invasion and induces apoptosis of ovarian cancer cells by targeting programmed cell death 6. Oncol. Lett. 14 (6), 7311–7317. doi:10.3892/ol.2017.7157
Zhang, H., Zhou, Y., Xing, Z., Sah, R. K., Hu, J., and Hu, H. (2022). Androgen metabolism and response in prostate cancer anti-androgen therapy resistance. Int. J. Mol. Sci. 23 (21), 13521. doi:10.3390/ijms232113521
Zhang, M., Wang, Y., Huan, Z., Liu, Y., Zhang, W., Kong, D., et al. (2021). FSH modulated cartilage ECM metabolism by targeting the PKA/CREB/SOX9 pathway. J. Bone Min. Metab. 39 (5), 769–779. doi:10.1007/s00774-021-01232-3
Zhang, Z., Zhu, Y., Lai, Y., Wu, X., Feng, Z., Yu, Y., et al. (2013). Follicle-stimulating hormone inhibits apoptosis in ovarian cancer cells by regulating the OCT4 stem cell signaling pathway. Int. J. Oncol. 43 (4), 1194–1204. doi:10.3892/ijo.2013.2054
Zhao, D., Liu, J., Wang, M., Zhang, X., and Zhou, M. (2019). Epidemiology of cardiovascular disease in China: current features and implications. Nat. Rev. Cardiol. 16 (4), 203–212. doi:10.1038/s41569-018-0119-4
Zheng, W., Magid, M. S., Kramer, E. E., and Chen, Y. T. (1996). Follicle-stimulating hormone receptor is expressed in human ovarian surface epithelium and fallopian tube. Am. J. Pathol. 148 (1), 47–53.
Zsakai, A., Mascie-Taylor, N., and Bodzsar, E. B. (2015). Relationship between some indicators of reproductive history, body fatness and the menopausal transition in Hungarian women. J. Physiol. Anthropol. 34, 35. doi:10.1186/s40101-015-0076-0
Aβ amyloid-β
AEP asparagine endopeptidase
AKT protein kinase B
ALKBH5 human Alk B homolog 5
APP amyloid precursor protein
BAT brown adipose tissue
BMP4 bone morphogenetic protein 4
cAMP cyclic adenosine monophosphate
C/EBPα CCAAT/Enhancer binding protein alpha
CIDEA cell death-inducing DFFA-like effector A
CREB cAMP response element-binding receptors
DR5 death receptor 5
ERK extracellular signal-regulated kinase
FAK focal adhesion kinase
Fas fatty acid synthase
FSH follicle-stimulating hormone
FSHR follicle-stimulating hormone receptor
GnRH gonadotropin-releasing hormone
HMG-CoA reductase 3-hydroxy-3-methyl glutaryl coenzyme A reductase
ICAM-1 intracellular adhesion molecule 1
IkBa inhibitor of kappa B alpha
JNK Jun N-terminal kinase
LDL-C low-density lipoprotein cholesterol
LH luteinizing hormone
Lpl lipoprotein lipase
MAPK mitogen-activated protein kinase
OCT4 octamer-binding transcription factor 4
PDCD6 programmed cell death gene 6
PI3K phosphoinositide 3-kinase
PKA protein kinase A
PPARγ peroxisome proliferator-activated receptor gamma
SAPK stress-activated protein kinase
Sox2 SRY-Box transcription factor 2
TC total cholesterol
UCP1 uncoupling protein-1
VCAM-1 vascular cell adhesion molecule 1
VEGFA vascular endothelial growth factor A
Keywords: FSH, FSHR, reproduction, menopause, non-reproductive diseases
Citation: Li C, Ling Y and Kuang H (2024) Research progress on FSH-FSHR signaling in the pathogenesis of non-reproductive diseases. Front. Cell Dev. Biol. 12:1506450. doi: 10.3389/fcell.2024.1506450
Received: 05 October 2024; Accepted: 05 November 2024;
Published: 20 November 2024.
Edited by:
Bilal Çiğ, Ahi Evran University, TürkiyeReviewed by:
Yves Combarnous, Centre National de la Recherche Scientifique (CNRS), FranceCopyright © 2024 Li, Ling and Kuang. This is an open-access article distributed under the terms of the Creative Commons Attribution License (CC BY). The use, distribution or reproduction in other forums is permitted, provided the original author(s) and the copyright owner(s) are credited and that the original publication in this journal is cited, in accordance with accepted academic practice. No use, distribution or reproduction is permitted which does not comply with these terms.
*Correspondence: Haibin Kuang, a3VhbmdoYWliaW5AbmN1LmVkdS5jbg==; Yan Ling, bGluZ3lhbjEyMDVAMTYzLmNvbQ==
Disclaimer: All claims expressed in this article are solely those of the authors and do not necessarily represent those of their affiliated organizations, or those of the publisher, the editors and the reviewers. Any product that may be evaluated in this article or claim that may be made by its manufacturer is not guaranteed or endorsed by the publisher.
Research integrity at Frontiers
Learn more about the work of our research integrity team to safeguard the quality of each article we publish.