- 1Center of Obstetrics and Gynecology, Peking University Shenzhen Hospital, Shenzhen, China
- 2Institute of Obstetrics and Gynecology, Shenzhen PKU-HKUST Medical Center, Shenzhen, China
- 3Shenzhen Key Laboratory on Technology for Early Diagnosis of Major Gynecologic Diseases, Shenzhen, China
- 4Department of Obstetrics and Gynecology, Longquan People’s Hospital, Lishui, China
Copper (Cu) is an essential trace element involved in a variety of biological processes, such as antioxidant defense, mitochondrial respiration, and bio-compound synthesis. In recent years, a novel theory called cuproptosis has emerged to explain how Cu induces programmed cell death. Cu targets lipoylated enzymes in the tricarboxylic acid cycle and subsequently triggers the oligomerization of lipoylated dihydrolipoamide S-acetyltransferase, leading to the loss of Fe–S clusters and induction of heat shock protein 70. Gynecological malignancies including cervical cancer, ovarian cancer and uterine corpus endometrial carcinoma significantly impact women’s quality of life and even pose a threat to their lives. Excessive Cu can promote cancer progression by enhancing tumor growth, proliferation, angiogenesis and metastasis through multiple signaling pathways. However, there are few studies investigating gynecological cancers in relation to cuproptosis. Therefore, this review discusses Cu homeostasis and cuproptosis while exploring the potential use of cuproptosis for prognosis prediction as well as its implications in the progression and treatment of gynecological cancers. Additionally, we explore the application of Cu ionophore therapy in treating gynecological malignancies.
1 Introduction
As an essential trace element, copper (Cu) plays a pivotal role in various biological processes, encompassing antioxidant defense, mitochondrial respiration, and bio-compound synthesis (Chen et al., 2022). Within the human body, Cu maintains a delicate equilibrium and achieves homeostasis. Furthermore, the accumulation of Cu is associated with the manifestation of Wilson disease, a severe hereditary disorder (Walshe, 2007). Recently elucidated by Tsvetkov et al. (2022) in March 2022, cuproptosis represents a novel mechanism of cell death induced by excessive Cu ions. This phenomenon is distinct from other forms of programmed cell death such as apoptosis, ferroptosis, and necroptosis and relies on mitochondrial respiration. Specifically targeting lipoylated enzymes within the tricarboxylic acid (TCA) cycle primarily through Cu+ ions binding to them leads to subsequent oligomerization of lipoylated dihydrolipoamide S-acetyltransferase (DLAT), loss of Fe-S clusters and induction of heat shock protein 70 (HSP70), ultimately culminating in acute proteotoxic stress-induced cellular demise.
Gynecological cancers, including cervical cancer (CC), ovarian cancer (OC), and uterine corpus endometrial carcinoma (UCEC), significantly impact women’s quality of life and pose a threat to their survival. According to the Global Cancer Statistics 2020 report, CC ranks as the fourth leading cause of cancer-related deaths and is among the top four most commonly diagnosed cancers in women across all populations (Sung et al., 2021). OC, particularly epithelial ovarian cancer, stands out as the most lethal gynecological malignancy with 5-year survival rates below 45%. The lack of effective early diagnostic methods results in over 70% of OC cases being detected at an advanced stage (Webb and Jordan, 2017). UCEC represents a prevalent gynecological malignancy originating from the endometrium (Yang et al., 2020). Despite achieving a cure rate of up to 95% for early-stage UCEC cases, its prognosis remains poor due to high recurrence and mortality rates, causing over 50,000 deaths annually (Holst et al., 2019; Wang et al., 2020; Cui et al., 2021). It is evident that the detrimental impact of gynecological cancers on women’s health cannot be overlooked.
In recent years, numerous reports have emerged regarding the correlation between elevated levels of Cu and cancer progression. Evidence has demonstrated that many types of cancer exhibit increased intratumoral copper levels and/or altered systemic distribution of copper; for instance, in the early 1980s, significantly higher levels of Cu were found in malignant tissues within female reproductive organs (Margalioth et al., 1983; Denoyer et al., 2015). Excessive amounts of Cu are believed to play a crucial role in promoting cell proliferation, angiogenesis, and metastasis during cancer progression (Garber, 2015). However, few studies have investigated the association between gynecological cancers and cuproptosis, especially the effect of cuproptosis on the progression and treatment of gynecological cancers. This review not only discusses Cu homeostasis and cuproptosis mechanisms, but also explored the prospects for using cuproptosis as a prognostic tool for predicting outcomes in gynecological cancers, the role played by cuproptosis during gynecological cancer progression processes, as well as potential applications for Cu ionophores treatment. This review provides new insights into the application of coproptosis in gynecological cancers.
2 Copper homeostasis and cuproptosis
2.1 Copper homeostasis
After the ingestion of Cu-containing food and water, the majority of dietary Cu is absorbed in the small intestine by the copper transporter 1 (CTR1), a homotrimeric Cu transporter belonging to the solute carrier 31 (SLC31, CTR) class of proteins (Bossak et al., 2018). Prior to absorption by CTR1, Cu2+ in food is initially reduced to Cu+ at the apical membrane of enterocytes, most likely facilitated by Steap proteins which are a family of metalloreductases (Lönnerdal, 2008). Additionally, it has been suggested that divalent metal transporter 1 (DMT1), a major iron transporter, may also transport Cu at the apical membrane of enterocytes (Gunshin et al., 1997). Following absorption, Cu ions are exported from enterocytes into the bloodstream via ATPase copper transporting alpha (ATP7A) - one type of Cu-transporting ATPase (Cu-ATPase). Cu-ATPases are polytopic membrane proteins that regulate transmembrane transport of Cu ions through ATP hydrolysis energy utilization; this includes ATP7A and ATPase copper transporting beta (ATP7B) (Lutsenko et al., 2007). Cu ions primarily circulate in blood bound to various proteins. In human blood serum, approximately 75% of Cu2+ ions bind nonexchangeably with ceruloplasmin; among exchangeable Cu ions: about 25% bind with human serum albumin, while around 0.2% exist as ternary complexes comprising Cu2+-His-Xaa moieties (Kirsipuu et al., 2020). Subsequently, Cu is transported to the liver which plays a crucial role in regulating systemic copper homeostasis as it serves as a major captor and distributor while facilitating excretion via bile ducts (Li, 2020). The liver’s storage function for Cu is mediated by two thiol-rich proteins known as metallothionein1/2, capable of binding copper ions through their cysteine residues in pH-dependent manner (Xie et al., 2023). Furthermore, the liver can eliminate excesses amounts of Cu into the bile ducts through the function of ATP7B and release Cu into the circulation to redistribute Cu throughout the body (Hernandez et al., 2008). Ultimately, Cu is primarily excreted from the body through biliary secretion and unabsorbed metal ions, with a minor fraction being eliminated via urine, sweat, and menstruation (Aggett, 1999; Chen et al., 2022).
After cellular uptake, monovalent Cu ions are transported into cells via the Steap proteins and CTR1. Subsequently, Cu+ is either sequestered by metallothioneins (MTs) for storage or conveyed within the cytoplasm by copper chaperones. During the storage process, Cu ions initially bind to glutathione (GSH), and then Cu+ is delivered to MTs in the form of a Cu-GSH complex (Harris, 2000). Simultaneously, a precise regulatory network of high-affinity copper chaperones facilitates trafficking of non-stored Cu ions. Cu is directed towards superoxide dismutase 1 (SOD1) through its dedicated chaperone called copper chaperone for superoxide dismutase (CCS), which aids in detoxifying reactive oxygen species (ROS) and maintaining copper homeostasis. Animal experiments have demonstrated that both CCS and SOD1 are found in both the cytoplasm and mitochondrial intermembrane space, where they play a role in scavenging superoxides (Okado-Matsumoto and Fridovich, 2001; Sturtz et al., 2001). Copper ions are most likely transferred to antioxidant-1 protein (ATOX1), which acts as an intermediary for delivering Cu+ to ATP7A and ATP7B within the secretory pathway. These two transporters play crucial roles in supplying Cu for biosynthetic processes while also exporting excess intracellular Cu from cells through targeted localization on different cellular membranes (Lutsenko et al., 2007). In addition to transferring Cu to secretory compartments and cytosolic proteins, Cu+ is transported into mitochondria via cytochrome oxidase 17 protein (COX17), a specific chaperone for delivery to cytochrome c oxidase (CCO) involved in oxidative phosphorylation and mitochondrial function. Furthermore, within the cell nucleus, Cu+ can regulate CTR1 expression through Sp1 zinc finger domain acting as a sensor for monitoring intracellular Cu levels (Li, 2020). Figure 1 illustrates cellular mechanisms governing Cu homeostasis.
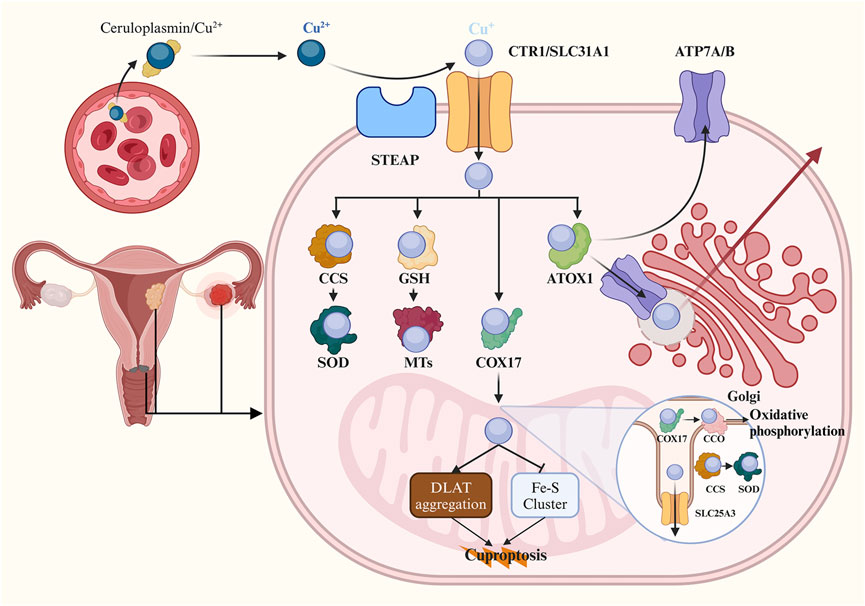
Figure 1. Schematic of copper homeostasis and cuproptosis. The majority of Cu2+ ions are transported by binding to ceruloplasmin in human blood serum. Cells uptake Cu+ ions through the Steap proteins and CTR1. Cellular Cu ions can bind to GSH and are then delivered to metallothioneins for storage in the form of a Cu-GSH complex. Cu can also target SOD1 through CCS to detoxify ROS. In the secretory pathway, Cu ions bind to ATOX1 and are delivered to ATP7A and ATP7B, which are involved in delivering Cu to the biosynthetic pathway and exporting it from the cell by targeting Cu-ATPases to different cellular membranes. Additionally, Cu is transported to CCO within mitochondria for oxidative phosphorylation and mitochondrial function via COX17. Within mitochondria, it is proposed that Cu directly binds to lipoylated proteins DLAT leading to lipoylation-dependent oligomerization in the TCA cycle. Furthermore, copper-induced programmed cell death occurs as a result of its ability induce loss of Fe-S cluster proteins in an FDX1-dependent manner. CTR1, Cu transporter 1; GSH, glutathione; SOD1, superoxide dismutase 1; CCS, copper chaperone for superoxide dismutase; ROS, reactive oxygen species; ATOX1, antioxidant-1 protein; ATP7A, ATPase copper transporting alpha; ATP7B, ATPase copper transporting beta; Cu-ATPase, Cu-transporting ATPase; CCO, cytochrome c oxidase; COX17, cytochrome oxidase 17 protein; DLAT, dihydrolipoamide S-acetyltransferase; TCA, tricarboxylic acid; FDX1, ferredoxin 1.
2.2 Cuproptosis
In the early 1980s, Cu was reported to induce cell death (Halliwell and Gutteridge, 1984). Over subsequent decades, there has been ongoing debate regarding the mechanism of programmed cell death associated with excessive Cu levels. However, in 2022, a novel mechanism called cuproptosis was elucidated and validated as the process by which Cu induce programmed cell death, distinguishing it from apoptosis, ferroptosis, pyroptosis, and necroptosis (Tsvetkov et al., 2022). Specifically, it was discovered that increased intracellular Cu levels resulting from pulsed treatment with the Cu ionophore elesclomol at concentrations as low as 40 nM could trigger cuproptosis. Importantly, pharmacological inhibitors known to block various cell death pathways were found to be ineffective in suppressing Cu-induced cell death. These inhibitors included necrostatin-1 (a necroptosis inhibitor), ferrostatin-1 (a ferroptosis inhibitor), N-acetyl cysteine (an inhibitor of oxidative stress-induced death), Z-VAD-FMK and Boc-D-FMK (apoptosis inhibitors). Conversely, only a Cu chelator demonstrated the ability to rescue cells from excess Cu-induced cell death. This finding highlights that cuproptosis is distinct from all other known forms of programmed cell death.
The mitochondrion is a crucial site for the biological role of Cu within cells and represents a primary target in cuproptosis. Excessive Cu ions are known to induce oxidative damage to the mitochondrial membrane and disrupt enzyme function in the TCA cycle (Sheline and Choi, 2004; Arciello et al., 2005). Previous studies have demonstrated that Cu impact the mitochondrial respiratory chain, resulting in elevated levels of intracellular ROS (Jiao et al., 2016; Kwan et al., 2016). In 2022, Tsvetkov et al. elucidated the strong association between cuproptosis and the TCA cycle rather than the electron transport chain or ATP production. They observed time-dependent dysregulation of numerous metabolites associated with the TCA cycle following pulse treatment with elesclomol. Conversely, treatment with elesclomol did not significantly reduce basal or ATP-linked respiration, while mitochondrial uncoupler had no effect on Cu toxicity (Tsvetkov et al., 2022). Subsequently, they identified and validated critical cuproptosis key genes (CKGs) through genome-wide knockout screens, metabolism screens, and individual gene knockout studies. Knockout of seven CKGs was found to rescue cuproptosis. Among these genes, Ferredoxin 1 (FDX1) encodes a reductase that converts Cu2+ to Cu+, thereby enhancing copper toxicity by directly binding to elesclomol-Cu complex and inhibiting Fe-S cluster formation (Tsvetkov et al., 2019). The remaining six genes can be divided into two groups: lipolytransferase1 (LIPT1), lipoyl synthase (LIAS), dihydrolipoamide dehydrogenase (DLD), which encode components of lipoic acid pathway; pyruvate dehydrogenase (PDH) complex including DLAT, pyruvate dehydrogenase E1 subunit alpha 1(PDHA1), pyruvate dehydrogenase E1 subunit beta (PDHB), which encode protein targets for lipoylation. Through immunohistochemical staining and gene knockout experiments, FDX1 was identified as an upstream regulator of protein lipoylation, a highly conserved lysine posttranslational modification found in only four enzymes involved in metabolic complexes within the TCA cycle (Rowland et al., 2018; Solmonson and DeBerardinis, 2018). It has been proposed that Cu directly binds to lipoylated proteins in the TCA cycle, leading to a toxic gain of function (Tsvetkov et al., 2022). DLAT, an essential component of the PDH complex, undergoes lipoylation-dependent oligomerization upon binding Cu ions. Additionally, Tsvetkov et al. (2022) also discovered that treatment with copper ionophore induces loss of Fe-S cluster proteins in an FDX1-dependent manner and triggers acute proteotoxic stress characterized by excessive HSP70 expression. The mechanism underlying cuproptosis reveals the interplay between mitochondrial respiration, the TCA cycle, and the Cu-induced programmed cell death (Figure 1).
3 Excessive Cu and cancer progression
Numerous studies have consistently reported elevated levels of Cu in both serum and tumor tissues across various types of cancers, including gynecologic malignancies (Gupte and Mumper, 2009). For instance, patients with CC exhibited significantly higher serum Cu levels compared to healthy individuals (Zhang et al., 2018), while cancerous ovary tissues showed a greater concentration of Cu than noncancerous ovary samples (Yaman et al., 2007). Besides, it has been reported that chronic exposure to elevated levels of Cu in drinking water can stimulate the proliferation of tumor cells and pancreatic cancer growth in mice by regulating the oxidative phosphorylation process (Ishida et al., 2013). These results suggest that excess Cu may be associated with the development and progression of cancers.
Recent studies have demonstrated that excessive Cu can participate in multiple signaling pathways, thereby promoting cancer progression by enhancing tumor growth, proliferation, angiogenesis, and metastasis. Here we summarized several pivotal signaling pathways implicated in the progression of cancer. In terms of cell proliferation, mitogen-activated protein kinase 1/2 (MEK1/2) was the first-identified copper-binding kinase belonging to the RAS/RAF/MEK/ERK pathway, which is believed to be involved in cell proliferation and cancer progression (Chang et al., 2003; Montagut and Settleman, 2009; Vitaliti et al., 2022). Copper ions can also act on the PI3K-AKT signaling pathway leading to downstream AKT activation through direct activation or binding to histidine 117 and histidine 203 sites of pyruvate dehydrogenase kinase 1 (Ostrakhovitch et al., 2002; Guo et al., 2021). Subsequently, AKT activation further catalyzes the phosphorylation and subcellular redistribution of forkhead box O1a and forkhead box O4, resulting in cancer cell proliferation (Walter et al., 2006). Additionally, ATOX1, one of the Cu chaperones, has been documented to augment cellular proliferation as a Cu-dependent transcription factor while promoting inflammatory neovascularization (Itoh et al., 2008; Chen et al., 2015).
Angiogenesis is another crucial step in tumor progression with excess Cu considered an essential co-factor for angiogenesis (Tisato et al., 2010). The role of Cu in the regulation of blood vessel formation has been demonstrated through its mediation of angiogenin’s biological activity, promotion of microvessel invasion and vascular infiltration, as well as upregulation of vascular endothelial growth factor (VEGF) expression level (Soncin et al., 1997; Gérard et al., 2010; Qiu et al., 2012). Hypoxia inducible factors (HIFs) play a crucial role in tumor angiogenesis by activating the transcription of VEGF, angiopoietin 2, stromal-derived factor 1, cyclooxygenase 2, and stem cell factor (Jun et al., 2017). Importantly, Cu is essential for HIF-1 activation through its regulation of the binding between HIF-1α and the hypoxia-responsive element as well as the formation of the HIF-1 transcriptional complex (Feng et al., 2009).
Moreover, some studies have demonstrated that Cu can exert its effects on various proteins and pathways to promote tumor invasion and metastasis. Cu has been shown to activate lysyl oxidase/lysyl oxidase like proteins secreted by cancer cells, thereby promoting tumor metastasis through remodeling of the tumor microenvironment and induction of epithelial-mesenchymal transition (EMT), a critical process in cancer cell invasion (Xiao and Ge, 2012; Vitaliti et al., 2022). Additionally, Cu can activate phosphotyrosine-binding protein mediator of ErbB2-driven cell motility 1, which triggers EMT by binding to insulin receptor substrate 1 and activating downstream PI3K-AKT signaling pathway (Sorokin and Chen, 2013; Vitaliti et al., 2022). Furthermore, copper has been reported to regulate intracellular levels of cyclic adenosine monophosphate (cAMP) by binding to and inhibiting cAMP-degrading phosphodiesterase 3B activity (Krishnamoorthy et al., 2016). The primary target of cAMP - protein kinase A - is believed to enhance cancer cell metastatic capacity (Tonucci et al., 2019). Recent findings indicate that ATOX1 is essential for the migration of breast cancer cells (Blockhuys et al., 2020). The autophagy pathway plays an essential role in cancer cell proliferation as it facilitates recycling metabolic waste for further energy supply or aids in evading apoptosis (Xie et al., 2023). Tsang et al. discovered a direct interaction between Cu and the autophagic kinases unc-51 like autophagy activating kinase 1 (ULK1) and ULK2, demonstrating that intracellular excessive Cu ions are associated with starvation-induced autophagy, thereby enhancing ULK1 kinase activity and promoting autophagic flux (Tsang et al., 2020). Collectively, elevated levels of Cu can modulate various proteins and signaling pathways involved in cancer progression.
4 Cuproptosis in gynecological cancers
Gynecological cancer is a significant threat to women’s health and life, encompassing CC, OC, and UCEC. Recent advancements in next-generation sequencing technology and bioinformatic analysis methods have led to the discovery of numerous cuproptosis-related genes (CRGs) and cuproptosis-related long non-coding RNAs (CRLs) in patients with gynecological cancers. Utilizing these CRGs and CRLs, researchers have developed prognostic prediction models for patients with gynecological cancers through LASSO algorithms and multivariate regression analysis. The samples are stratified into high-risk and low-risk groups based on the risk score that exhibits a negative correlation with disease prognosis. Subsequently, they performed functional analyses of differentially expressed genes between high-risk and low-risk groups to identify potential biomarkers and therapeutic targets associated with cuproptosis-related signatures in gynecological cancers. Immunotherapy, such as anti-PD-1/PD-L1 antibodies represents an important milestone in gynecological cancer treatment. Nevertheless, the low response rate observed in unselected patients and the tendency of therapeutic resistance continue to pose significant challenges to their clinical application (Li T. et al., 2023). Thus, the potential benefits of immunotherapy for patients with different risks were also explored. Additionally, several studies suggest that copper ionophores such as elesclomol and disulfiram (DSF) may hold promise as treatments for gynecological malignancies.
4.1 Construction of risk models and functional analysis using CRGs/CRLs
4.1.1 CC
CC is a prevalent tumor that poses a significant threat to the physical and mental wellbeing of women. To date, several studies have identified CRGs or CRLs associated with CC prognosis, developed prognostic models, and elucidated potential mechanisms influencing prognosis and therapeutic strategies through functional analysis of distinct risk groups (Table 1). Lei et al. (2022) investigated the differential expression levels of 13 known CRGs in cervical cancer samples and constructed a prognostic prediction model incorporating seven CRGs. Their findings revealed that DBT, FDX1, LIPT1, and PDHA1 acted as positive predictors for survival in patients with CC, while ATP7A, DLAT, and GCSH were negative predictors. Notably, LIPT1 and PDHA1 were found to positively regulate cuproptosis whereas ATP7A inhibited cuproptosis by reducing intracellular Cu levels (Tsvetkov et al., 2019; Tsvetkov et al., 2022), suggesting reduced susceptibility to cuproptosis in the high-risk group (Lei et al., 2022). Among these CRGs, FDX1 has been reported to impact the prognosis of lung adenocarcinoma by modulating glucose metabolism, fatty acid oxidation, and amino acid metabolism without affecting tumor cell growth rate apoptosis or abnormal cell cycle distribution (Zhang Z. et al., 2021). Furthermore, ATP7A has been implicated in poor survival outcomes across various cancer types due to its role in promoting tumorigenesis metastasis and conferring platinum drug resistance (Samimi et al., 2003; Shanbhag et al., 2019; Yu et al., 2021). Moreover, Kang et al. (2024) discovered that SFT2D1, a cuproptosis-related angiogenesis gene, exhibited high expression in CC and displayed a positive correlation with microvascular density. Knockdown of SFT2D1 significantly inhibited the proliferation, migration, and invasiveness of CC cells. Meanwhile, several significant CRLs have been validated to be included in risk prediction models. LINC01305 has been shown to promote the progression of CC cells and is associated with a lower survival rate through its interaction with the RNA-binding protein KHSRP (Huang et al., 2021). Liu et al. (2022b)’s study revealed that AL441992.1 is a protective factor in CC, which is consistent with previous research conducted by Chen et al. (2020). In CC, SOX21-AS1 exhibits hypomethylation and plays a role in cell proliferation, migration, invasion, and EMT progress (Zhang et al., 2019; Du et al., 2021). ATP2A1-AS1 has been confirmed as a prognostic biomarker for CC due to its involvement in autophagy-related processes (Feng et al., 2021). Additionally, Liu et al. (2023b) was the first to discover that CNNM3-DT was differentially expressed between radiosensitive and non-radiosensitive groups, suggesting it may serve as a potential target for treatment.
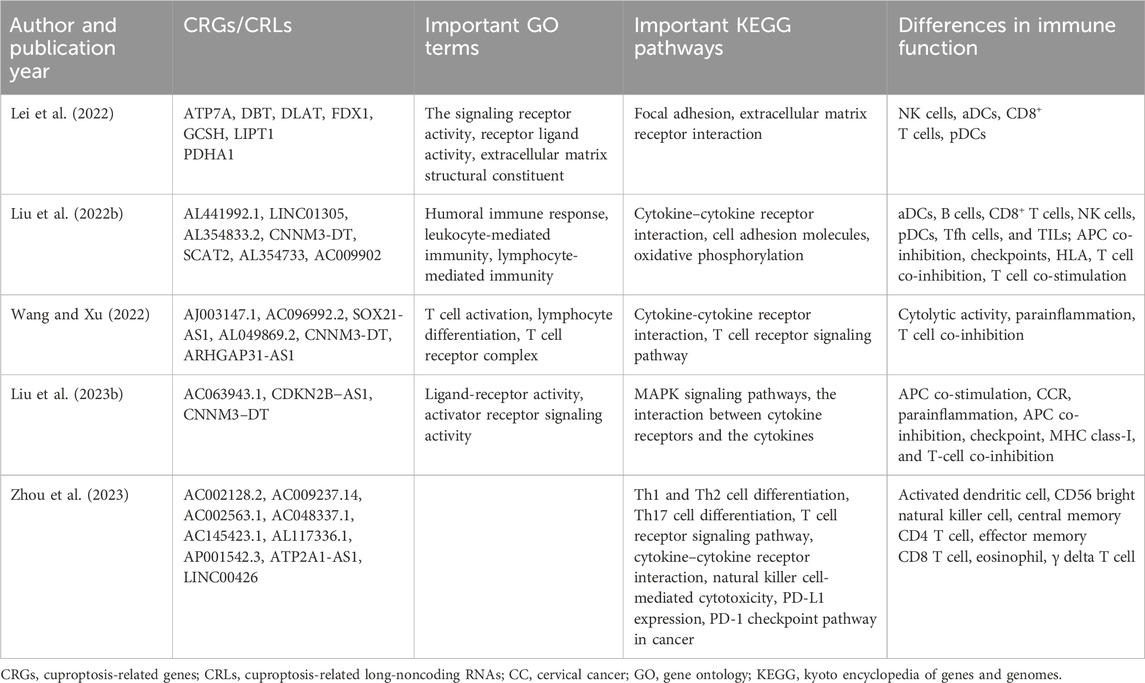
Table 1. CRGs/CRLs in the risk model and results of functional analysis between two risk groups in CC.
The results of Kyoto Encyclopedia of Genes and Genomes (KEGG) functional analysis revealed enrichment of immune-related pathways, including cytokine–cytokine receptor interaction, T cell receptor signaling pathway, Th1 and Th2 cell differentiation, as well as PD-L1 expression and PD-1 checkpoint pathway in cancer between two risk groups. These findings suggest that CRLs may mediate immune-related processes and ultimately impact the progression of CC in different risk groups (Liu et al., 2022b; Wang and Xu, 2022; Zhou et al., 2023). Furthermore, KEGG analysis demonstrated that differentially expressed CRGs were enriched in focal adhesion and extracellular matrix receptor interaction pathways closely associated with invasion and metastasis processes in cancer cells. This indicates a potential relationship between cuproptosis and CC invasion (Lei et al., 2022). Given the strong association between CRLs and immune regulation along with the widespread use of immunotherapy for CC treatment, an immune function analysis was conducted. Significant differences were observed in various immune cell types and functions between two risk groups. For instance, Liu et al. (2023b) reported significantly lower expression levels of CD8+ T-cells in the high-risk group, which is known to contribute to cancer progression through exhaustion mechanisms (Dolina et al., 2021). Additionally, key immune checkpoint genes exhibited higher expression levels in the low-risk group including PD-1, CTLA4, LAG-3, and TIGIT suggesting that immunotherapy such as immune checkpoint inhibitors (ICI) may be more suitable for patients with low-risk (Liu et al., 2022b). These conclusions provide novel insights into CC progression mechanisms as well as treatment strategies tailored to patients with different risk.
4.1.2 OC
OC is the most lethal malignant gynecological tumor, with 5-year survival rates below 45% (Webb and Jordan, 2017). We identified four articles that elucidated the construction of prognostic prediction models and revealed significant differences in signal pathways and immune functions between high- and low-risk subgroups (Table 2). Among the candidate CRGs/CRLs involved in constructing the prediction model, functional experiments confirmed that WASF2 was associated with cuproptotic resistance, promoting cancer cell proliferation and platinum resistance. Moreover, its expression level showed a negative correlation with prognosis in OC patients (Wang et al., 2023). The lncRNA ZFHX4-AS1 was identified as a prognostic biomarker involved in cell proliferation, metabolism, infiltration, and distribution of tumor-infiltrating immune cells in OC. Its over-expression was significantly associated with poor overall survival and progression-free survival (PFS) (Wang et al., 2022). Additionally, AP001372.2 was reported as a novel biomarker for predicting prognosis in head and neck squamous cell cancer (Liu et al., 2022a). Liu et al. (2023a) found that the differentially expressed genes were enriched in extracellular matrix (ECM) related signaling pathways and biological processes in Gene Ontology (GO) and KEGG analysis. The ECM, which plays role in the transmission of information between cells, is remodeled by the uncontrolled growth of cells in cancer (Girigoswami et al., 2021). A recent review highlighted the pivotal involvement of ECM in serous OC development and progression including initiation at precursor lesions within fallopian tube fimbriae, metastatic progression, and drug resistance development (Brown et al., 2023). Moreover, significant differences in immune function analysis were observed among different risk groups, including immunomodulatory pathways, immune cell types, and immune cell infiltration. Zhang J. et al. (2022) found a negative association between the risk score and the immune score, activated immune inflammatory cells, the number of immune-related pathways, and particularly the immune cell infiltration. This suggests that patients in the high-risk group may experience immunosuppression. Liu et al. (2023a) reached a similar conclusion as they identified abundant Tregs in the tumor microenvironment of high-risk patients. Tregs have been reported to play a role in suppressing antitumor immune responses and are associated with poor survival rates (Göschl et al., 2019). The high-risk group also exhibited enrichment of pathways involved in promoting cancer progression such as WNT β-catenin signaling and EMT receptor interaction (Li et al., 2024). Meanwhile, patients in the low-risk group showed high expression levels of molecules related to immune checkpoints such as PDL1 and CTLA4 (Zhang J. et al., 2022; Li et al., 2024). In contrary, Liu et al. (2023a) noted overexpression of PD1, CTLA4, PD-L1, and HAVCR2 in patients from the high-risk group. Therefore, ICI treatment for OC patients should be individualized.
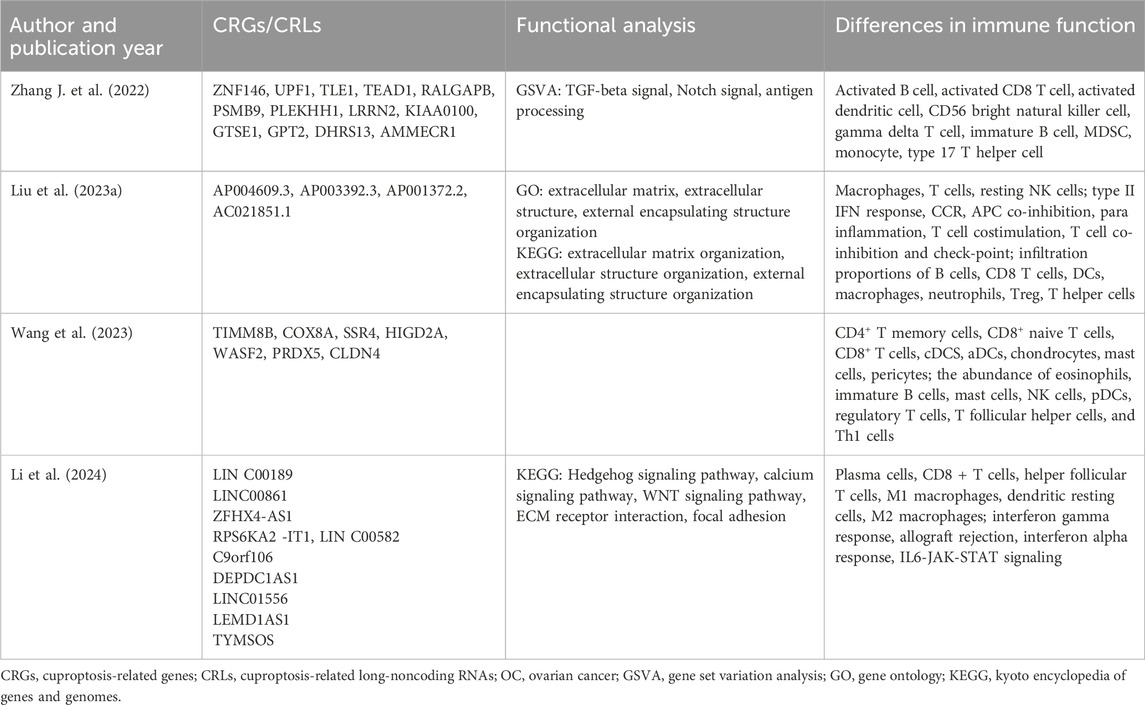
Table 2. CRGs/CRLs in the risk model and results of functional analysis between two risk groups in OC.
4.1.3 UCEC
UCEC, which originates from the endometrium, is a prevalent gynecological cancer worldwide. Table 3 summarizes the CRGs/CRLs in prognosis prediction models and the results of various functional analyses. Chen (2022) discovered that CDKN2A is overexpressed in UCEC cells and proposed that the regulatory axis lncRNA XIST/miR-125a-5p/CDKN2A plays a critical role in UCEC progression. Other studies have also reported CDKN2A as a prognostic biomarker in UCEC (Zhang et al., 2020; Lin et al., 2023). The CRG PC has been validated to be significantly upregulated in some UCEC cell lines (Lin et al., 2023) and has been shown to be essential for cell proliferation and progression in various malignancies (Sellers et al., 2015; Kiesel et al., 2021). lncRNA AC084117.1, associated with glutaminase, has been identified as a risk factor, and its silence significantly inhibits the proliferation and migration of UCEC cells (Cai et al., 2023). Another risk factor, BX322234.1, was found to be negatively correlated with prognosis in UCEC patients (Li B. et al., 2023), consistent with previous studies (Wang et al., 2021; Huo et al., 2022).
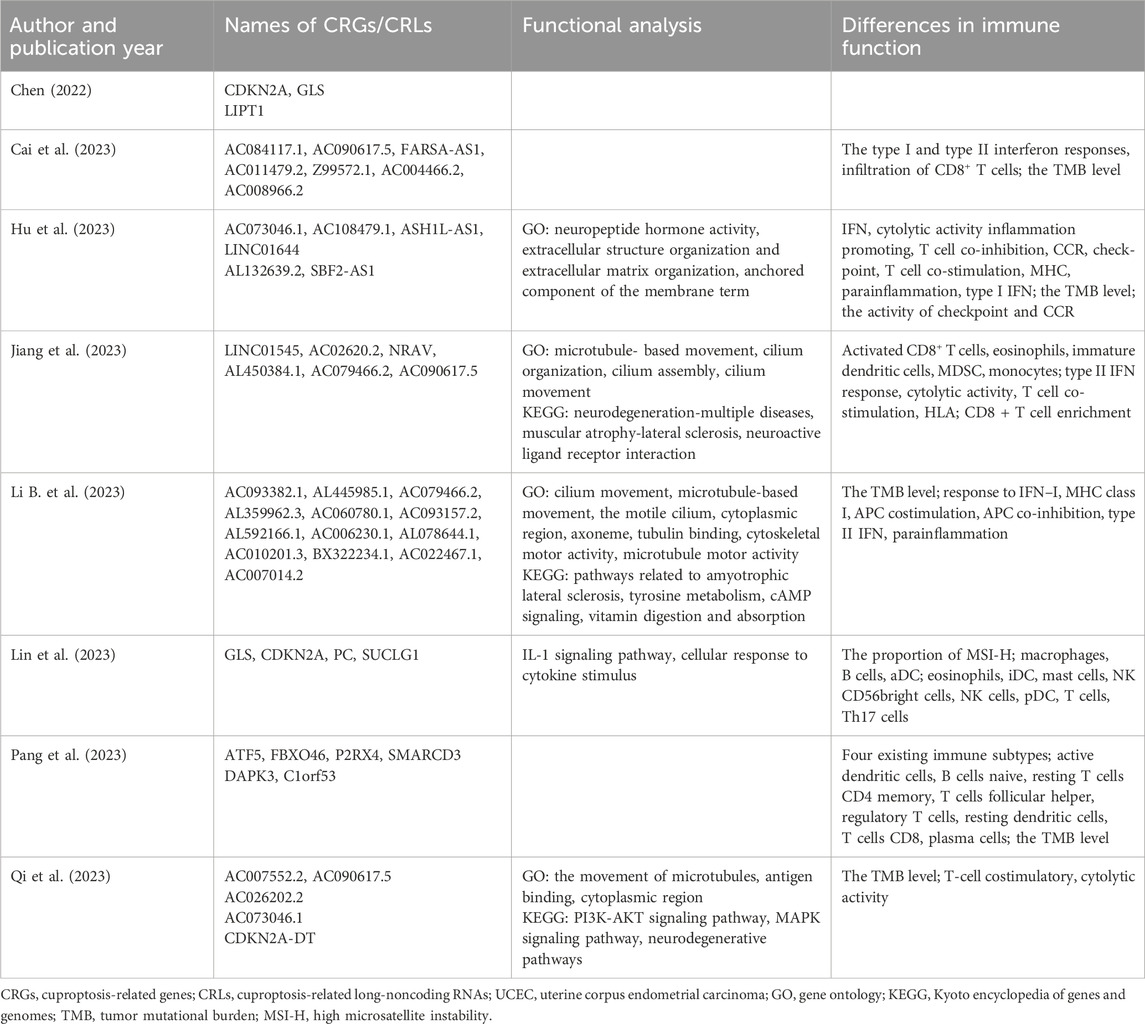
Table 3. CRGs/CRLs in the risk model and results of functional analysis between two risk groups in UCEC.
The results of GO, KEGG, and immune functional analysis revealed significant differences between the low-risk and high-risk groups. Hu et al. (2023) identified neuropeptide hormone activity as the top-ranked term in GO analysis, with extracellular structure organization and extracellular matrix organization also enriched. These findings suggest an association between UCEC prognosis and cellular recognition and hormone modulation (Hu et al., 2023). In terms of KEGG analysis, several critical signaling pathways in cancer research were enriched, including cAMP signaling, PI3K-AKT signaling pathway, and MAPK signaling pathway (Li B. et al., 2023; Qi et al., 2023). The activated cAMP/PKA signaling pathway has been shown to inhibit proliferation, invasion, migration, and growth of UCEC cells in mice (Li et al., 2022). Aberrant activation of the PI3K-AKT signaling pathway has been reported in various malignancies including UCEC (Noorolyai et al., 2019; Roncolato et al., 2019). In UCEC, excessive estrogen can activate the PI3K-AKT pathway to promote cell proliferation (Qu et al., 2015), while inhibitors targeting this pathway have demonstrated potential for suppressing progestin-resistant cancer cell proliferation through autophagy promotion (Liu et al., 2017). Furthermore, numerous studies have focused on exploring potential therapies by targeting the PI3K-AKT pathway in UCEC (Roncolato et al., 2019; Lin et al., 2022; Liang et al., 2023). The MAPK pathway, consisting of a cascade of three kinases, is known to respond to various physiological signals such as hormones, cytokines, and growth factors (Lee et al., 2020). It has been found to play a role in the migration and invasion of cancer cells in UCEC (Zhang F. et al., 2021). Additionally, immune analysis revealed significant differences in immune cell types, immune function, and immune cell infiltration between the high-risk and low-risk groups. The low-risk group exhibited higher checkpoint and CCR activity, higher tumor mutational burden (TMB), as well as a higher proportion of high microsatellite instability compared to the high-risk group (Hu et al., 2023; Li B. et al., 2023; Lin et al., 2023). Theoretically speaking, a higher TMB leads to more neo-antigens which increases the chances for T cell recognition and improves outcomes in clinical ICI treatment (Jardim et al., 2021). Consequently, patients in the low-risk group may potentially benefit more from immunotherapy.
4.2 Application of copper ionophores in gynecological cancers
Copper ionophores are lipid-soluble molecules capable of increasing intracellular Cu levels and inducing cuproptosis by reversibly binding with Cu (Tsvetkov et al., 2022). Elesclomol and DSF, as prominent copper ionophore drugs, have been shown to play a crucial role in cancer therapy (Oliveri, 2022). For instance, a phase III trial demonstrated that the combination of elesclomol and paclitaxel significantly extended median PFS compared to paclitaxel alone in advanced melanoma patients with normal baseline lactate dehydrogenase levels (O’Day et al., 2013). DSF, an inhibitor of aldehyde dehydrogenase, has been suggested to confer protective effects against prostate and breast cancer (Askgaard et al., 2014).
Several studies have demonstrated the potential application of elesclomol and DSF in the therapy of gynecological cancers. In CC cell lines, DSF showed cytotoxicity in a Cu-dependent manner and this cytotoxic effect was mediated by apoptosis and autophagy simultaneously. Additionally, the DSF/Cu complex was found to reduce cisplatin resistance by targeting cancer stem cell-like LGR5(+) cells (Cao et al., 2022; Zhang W. et al., 2022). Furthermore, Zheng et al. (2023) revealed that DSF could bind to HSP90A to inhibit tumor growth and metastasis through the HSP90A/NDRG1/β-catenin pathway in CC cells. Combination treatment with DSF and Cu significantly reduced tumor volume and improved survival rate in a murine OC xenograft model (Gan et al., 2023). Moreover, DSF was shown to enhance drug sensitivity of poly (ADP-ribose) polymerases inhibitors (PARPis), an important targeted drug for OC treatment (Tang et al., 2023). Additionally, both elesclomol and DSF increased cell death when combined with carboplatin treatment compared to carboplatin alone in OC cells. The combination of DSF and cisplatin also enhanced apoptosis of cancer cells, suggesting that Cu ionophores could augment the anti-tumor effect of platinum drugs (Harrington et al., 2020; Yuan et al., 2023). In Ishikawa cells (endometrial cancer cells), the combined application of DSF and a novel Cu-cysteamine compound has been shown to promote apoptosis and exert potent anti-tumor effects by inducing mitochondrial impairment (Yang et al., 2024). Overall, DSF and elesclomol not only have efficacy against gynecological malignancies, but also show the potential to enhance the drug sensitivity of other anti-tumor chemotherapy drugs or targeted therapies.
5 Conclusion
The essential micronutrient Cu serves as a critical catalytic cofactor in various biological processes and maintains homeostasis. Excessive intracellular Cu is associated with the development of diseases such as Wilson disease. Cu from dietary sources or water is absorbed in the small intestine and transported into the bloodstream by ATP7A. The liver plays a crucial role in regulating systemic Cu homeostasis by storing Cu, excreting excess Cu into bile ducts, and releasing Cu into the blood for redistribution. Within cells, Cu is either stored bound to MTs or transported within the cytoplasm by copper chaperones. It can target SOD1 through CCS to detoxify ROS, bind to ATOX1 for delivery to secretory and biosynthetic pathways, or be transported to CCO within mitochondria for oxidative phosphorylation and mitochondrial function via COX17. In March 2022, Tsvetkov et al. elucidated a novel mechanism of cell death induced by Cu called cuproptosis, which differs from other programmed cell death mechanisms. Copper ions (Cu+) can target lipoylated enzymes in the TCA cycle, subsequently inducing oligomerization of lipoylated DLAT, loss of Fe-S clusters, and induction of HSP70 expression leading to acute proteotoxic stress-induced cell death.
The levels of Cu are elevated in both serum and tumor tissues in numerous malignancies. Recent studies have demonstrated that excessive Cu can promote cancer progression by enhancing tumor growth, proliferation, angiogenesis, and metastasis through various signaling pathways, such as the RAS/RAF/MEK/ERK pathway and the PI3K-AKT signaling pathway. ATOX1, a copper chaperone, is considered to function as a Cu-dependent transcription factor promoting inflammatory neovascularization. Additionally, copper has been reported to be involved in regulating vascular formation and infiltration, activating tumor microenvironment remodeling, inducing EMT, and participating in autophagy processes that contribute to cancer progression.
CC, OC, and UCEC are prevalent gynecological malignancies that have inflicted suffering and claimed the lives of women worldwide. Recent studies have identified specific CRGs or CRLs as prognostic markers for these cancers, aiming to elucidate the role of cuproptosis in their development by constructing risk prediction models. Multiple pathways, including immune-related pathways, WNT β-catenin signaling, cAMP signaling, PI3K-AKT signaling, and MAPK signaling pathway, have been implicated in the progression of these cancers. Furthermore, significant differences in immune function were observed between high-risk and low-risk groups, suggesting that immune therapy may be more effective in the latter group. Additionally, copper ionophores such as elesclomol and DSF hold promise for treating gynecological malignancies due to their direct effects on tumor cells as well as their ability to enhance sensitivity to other anti-tumor drugs like PARPis and platinum-based agents.
Author contributions
XH: Writing–original draft, Writing–review and editing. ML: Visualization, Writing–review and editing. CL: Conceptualization, Funding acquisition, Supervision, Writing–review and editing.
Funding
The authors declare that financial support was received for the research, authorship, and/or publication of this article. This work was supported by Shenzhen High-level Hospital Construction Fund (YBH2019-260), Shenzhen Key Medical Discipline Construction Fund (No. SZXK027), and Sanming Project of Medicine in Shenzhen (No. SZSM202011016).
Acknowledgments
The authors would like to thank the study participants for their contributions. Additionally, the figure was generated using Biorender.
Conflict of interest
The authors declare that the research was conducted in the absence of any commercial or financial relationships that could be construed as a potential conflict of interest.
Publisher’s note
All claims expressed in this article are solely those of the authors and do not necessarily represent those of their affiliated organizations, or those of the publisher, the editors and the reviewers. Any product that may be evaluated in this article, or claim that may be made by its manufacturer, is not guaranteed or endorsed by the publisher.
References
Arciello, M., Rotilio, G., and Rossi, L. (2005). Copper-dependent toxicity in SH-SY5Y neuroblastoma cells involves mitochondrial damage. Biochem. Biophys. Res. Commun. 327 (2), 454–459. doi:10.1016/j.bbrc.2004.12.022
Askgaard, G., Friis, S., Hallas, J., Thygesen, L. C., and Pottegård, A. (2014). Use of disulfiram and risk of cancer: a population-based case-control study. Eur. J. Cancer Prev. 23 (3), 225–232. doi:10.1097/CEJ.0b013e3283647466
Blockhuys, S., Zhang, X., and Wittung-Stafshede, P. (2020). Single-cell tracking demonstrates copper chaperone Atox1 to be required for breast cancer cell migration. Proc. Natl. Acad. Sci. U. S. A. 117 (4), 2014–2019. doi:10.1073/pnas.1910722117
Bossak, K., Drew, S. C., Stefaniak, E., Płonka, D., Bonna, A., and Bal, W. (2018). The Cu(II) affinity of the N-terminus of human copper transporter CTR1: comparison of human and mouse sequences. J. Inorg. Biochem. 182, 230–237. doi:10.1016/j.jinorgbio.2018.01.011
Brown, Y., Hua, S., and Tanwar, P. S. (2023). Extracellular matrix in high-grade serous ovarian cancer: advances in understanding of carcinogenesis and cancer biology. Matrix Biol. 118, 16–46. doi:10.1016/j.matbio.2023.02.004
Cai, L., Cai, L., Zhou, L., Zhao, Y., and Qian, J. (2023). Identification and validation of a seven cuproptosis-associated lncRNA signature to predict the prognosis of endometrial cancer. J. Int. Med. Res. 51 (12), 3000605231213435. doi:10.1177/03000605231213435
Cao, H. Z., Yang, W. T., and Zheng, P. S. (2022). Cytotoxic effect of disulfiram/copper on human cervical cancer cell lines and LGR5-positive cancer stem-like cells. BMC Cancer 22 (1), 521. doi:10.1186/s12885-022-09574-5
Chang, F., Steelman, L. S., Lee, J. T., Shelton, J. G., Navolanic, P. M., Blalock, W. L., et al. (2003). Signal transduction mediated by the Ras/Raf/MEK/ERK pathway from cytokine receptors to transcription factors: potential targeting for therapeutic intervention. Leukemia 17 (7), 1263–1293. doi:10.1038/sj.leu.2402945
Chen, G. F., Sudhahar, V., Youn, S. W., Das, A., Cho, J., Kamiya, T., et al. (2015). Copper transport protein antioxidant-1 promotes inflammatory neovascularization via chaperone and transcription factor function. Sci. Rep. 5, 14780. doi:10.1038/srep14780
Chen, P., Gao, Y., Ouyang, S., Wei, L., Zhou, M., You, H., et al. (2020). A prognostic model based on immune-related long non-coding RNAs for patients with cervical cancer. Front. Pharmacol. 11, 585255. doi:10.3389/fphar.2020.585255
Chen, Y. (2022). Identification and validation of cuproptosis-related prognostic signature and associated regulatory Axis in uterine corpus endometrial carcinoma. Front. Genet. 13, 912037. doi:10.3389/fgene.2022.912037
Chen, L., Min, J., and Wang, F. (2022). Copper homeostasis and cuproptosis in health and disease. Signal Transduct. Target Ther. 7 (1), 378. doi:10.1038/s41392-022-01229-y
Cui, Y. Q., Xiang, Y., Meng, F., Ji, C. H., Xiao, R., Li, J. P., et al. (2021). ALDH2 promotes uterine corpus endometrial carcinoma proliferation and construction of clinical survival prognostic model. Aging (Albany NY) 13 (20), 23588–23602. doi:10.18632/aging.203605
Denoyer, D., Masaldan, S., La Fontaine, S., and Cater, M. A. (2015). Targeting copper in cancer therapy: “copper that cancer”. Metallomics 7 (11), 1459–1476. doi:10.1039/c5mt00149h
Dolina, J. S., Van Braeckel-Budimir, N., Thomas, G. D., and Salek-Ardakani, S. (2021). CD8(+) T cell exhaustion in cancer. Front. Immunol. 12, 715234. doi:10.3389/fimmu.2021.715234
Du, P., Zhi, Y., Wang, R., Li, Y., Li, H., Zhang, X., et al. (2021). Aberrant methylation of the SOX21-AS1 promoter region promotes gene expression and its clinical value in cervical cancer. Reprod. Sci. 28 (2), 532–540. doi:10.1007/s43032-020-00335-y
Feng, W., Ye, F., Xue, W., Zhou, Z., and Kang, Y. J. (2009). Copper regulation of hypoxia-inducible factor-1 activity. Mol. Pharmacol. 75 (1), 174–182. doi:10.1124/mol.108.051516
Feng, Q., Wang, J., Cui, N., Liu, X., and Wang, H. (2021). Autophagy-related long non-coding RNA signature for potential prognostic biomarkers of patients with cervical cancer: a study based on public databases. Ann. Transl. Med. 9 (22), 1668. doi:10.21037/atm-21-5156
Gan, Y., Liu, T., Feng, W., Wang, L., Li, L. I., and Ning, Y. (2023). Drug repositioning of disulfiram induces endometrioid epithelial ovarian cancer cell death via the both apoptosis and cuproptosis pathways. Oncol. Res. 31 (3), 333–343. doi:10.32604/or.2023.028694
Garber, K. (2015). Cancer’s copper connections. Science 349 (6244), 129. doi:10.1126/science.349.6244.129
Gérard, C., Bordeleau, L. J., Barralet, J., and Doillon, C. J. (2010). The stimulation of angiogenesis and collagen deposition by copper. Biomaterials 31 (5), 824–831. doi:10.1016/j.biomaterials.2009.10.009
Girigoswami, K., Saini, D., and Girigoswami, A. (2021). Extracellular matrix remodeling and development of cancer. Stem Cell. Rev. Rep. 17 (3), 739–747. doi:10.1007/s12015-020-10070-1
Göschl, L., Scheinecker, C., and Bonelli, M. (2019). Treg cells in autoimmunity: from identification to Treg-based therapies. Semin. Immunopathol. 41 (3), 301–314. doi:10.1007/s00281-019-00741-8
Gunshin, H., Mackenzie, B., Berger, U. V., Gunshin, Y., Romero, M. F., Boron, W. F., et al. (1997). Cloning and characterization of a mammalian proton-coupled metal-ion transporter. Nature 388 (6641), 482–488. doi:10.1038/41343
Guo, J., Cheng, J., Zheng, N., Zhang, X., Dai, X., Zhang, L., et al. (2021). Copper promotes tumorigenesis by activating the PDK1-AKT oncogenic pathway in a copper transporter 1 dependent manner. Adv. Sci. (Weinh) 8 (18), e2004303. doi:10.1002/advs.202004303
Gupte, A., and Mumper, R. J. (2009). Elevated copper and oxidative stress in cancer cells as a target for cancer treatment. Cancer Treat. Rev. 35 (1), 32–46. doi:10.1016/j.ctrv.2008.07.004
Halliwell, B., and Gutteridge, J. M. (1984). Oxygen toxicity, oxygen radicals, transition metals and disease. Biochem. J. 219 (1), 1–14. doi:10.1042/bj2190001
Harrington, B. S., Ozaki, M. K., Caminear, M. W., Hernandez, L. F., Jordan, E., Kalinowski, N. J., et al. (2020). Drugs targeting tumor-initiating cells prolong survival in a post-surgery, post-chemotherapy ovarian cancer relapse model. Cancers (Basel) 12 (6), 1645. doi:10.3390/cancers12061645
Harris, E. D. (2000). Cellular copper transport and metabolism. Annu. Rev. Nutr. 20, 291–310. doi:10.1146/annurev.nutr.20.1.291
Hernandez, S., Tsuchiya, Y., García-Ruiz, J. P., Lalioti, V., Nielsen, S., Cassio, D., et al. (2008). ATP7B copper-regulated traffic and association with the tight junctions: copper excretion into the bile. Gastroenterology 134 (4), 1215–1223. doi:10.1053/j.gastro.2008.01.043
Holst, F., Werner, H. M. J., Mjøs, S., Hoivik, E. A., Kusonmano, K., Wik, E., et al. (2019). PIK3CA amplification associates with aggressive phenotype but not markers of AKT-MTOR signaling in endometrial carcinoma. Clin. Cancer Res. 25 (1), 334–345. doi:10.1158/1078-0432.Ccr-18-0452
Hu, P., Wang, Y., Chen, X., Zhao, L., Qi, C., and Jiang, G. (2023). Development and verification of a newly established cuproptosis-associated lncRNA model for predicting overall survival in uterine corpus endometrial carcinoma. Transl. Cancer Res. 12 (8), 1963–1979. doi:10.21037/tcr-23-61
Huang, X., Liu, X., Du, B., Liu, X., Xue, M., Yan, Q., et al. (2021). LncRNA LINC01305 promotes cervical cancer progression through KHSRP and exosome-mediated transfer. Aging (Albany NY) 13 (15), 19230–19242. doi:10.18632/aging.202565
Huo, X. L., Wang, S. F., Yang, Q., Yu, X. L., Gu, T., Hua, H. X., et al. (2022). Diagnostic and prognostic value of genomic instability-derived long non-coding RNA signature of endometrial cancer. Taiwan J. Obstet. Gynecol. 61 (1), 96–101. doi:10.1016/j.tjog.2021.11.018
Ishida, S., Andreux, P., Poitry-Yamate, C., Auwerx, J., and Hanahan, D. (2013). Bioavailable copper modulates oxidative phosphorylation and growth of tumors. Proc. Natl. Acad. Sci. U. S. A. 110 (48), 19507–19512. doi:10.1073/pnas.1318431110
Itoh, S., Kim, H. W., Nakagawa, O., Ozumi, K., Lessner, S. M., Aoki, H., et al. (2008). Novel role of antioxidant-1 (Atox1) as a copper-dependent transcription factor involved in cell proliferation. J. Biol. Chem. 283 (14), 9157–9167. doi:10.1074/jbc.M709463200
Jardim, D. L., Goodman, A., de Melo Gagliato, D., and Kurzrock, R. (2021). The challenges of tumor mutational burden as an immunotherapy biomarker. Cancer Cell. 39 (2), 154–173. doi:10.1016/j.ccell.2020.10.001
Jiang, X. Y., Hu, J. J., Wang, R., Zhang, W. Y., Jin, Q. Q., Yang, Y. T., et al. (2023). Cuproptosis-associated lncRNA gene signature establishes new prognostic profile and predicts immunotherapy response in endometrial carcinoma. Biochem. Genet. doi:10.1007/s10528-023-10574-8
Jiao, Y., Hannafon, B. N., and Ding, W. Q. (2016). Disulfiram’s anticancer activity: evidence and mechanisms. Anticancer Agents Med. Chem. 16 (11), 1378–1384. doi:10.2174/1871520615666160504095040
Jun, J. C., Rathore, A., Younas, H., Gilkes, D., and Polotsky, V. Y. (2017). Hypoxia-inducible factors and cancer. Curr. Sleep. Med. Rep. 3 (1), 1–10. doi:10.1007/s40675-017-0062-7
Kang, J., Jiang, J., Xiang, X., Zhang, Y., Tang, J., and Li, L. (2024). Identification of a new gene signature for prognostic evaluation in cervical cancer: based on cuproptosis-associated angiogenesis and multi-omics analysis. Cancer Cell. Int. 24 (1), 23. doi:10.1186/s12935-023-03189-x
Kiesel, V. A., Sheeley, M. P., Coleman, M. F., Cotul, E. K., Donkin, S. S., Hursting, S. D., et al. (2021). Pyruvate carboxylase and cancer progression. Cancer Metab. 9 (1), 20. doi:10.1186/s40170-021-00256-7
Kirsipuu, T., Zadorožnaja, A., Smirnova, J., Friedemann, M., Plitz, T., Tõugu, V., et al. (2020). Copper(II)-binding equilibria in human blood. Sci. Rep. 10 (1), 5686. doi:10.1038/s41598-020-62560-4
Krishnamoorthy, L., Cotruvo, J. A., Chan, J., Kaluarachchi, H., Muchenditsi, A., Pendyala, V. S., et al. (2016). Copper regulates cyclic-AMP-dependent lipolysis. Nat. Chem. Biol. 12 (8), 586–592. doi:10.1038/nchembio.2098
Kwan, S. Y., Cheng, X., Tsang, Y. T., Choi, J. S., Kwan, S. Y., Izaguirre, D. I., et al. (2016). Loss of ARID1A expression leads to sensitivity to ROS-inducing agent elesclomol in gynecologic cancer cells. Oncotarget 7 (35), 56933–56943. doi:10.18632/oncotarget.10921
Lee, S., Rauch, J., and Kolch, W. (2020). Targeting MAPK signaling in cancer: mechanisms of drug resistance and sensitivity. Int. J. Mol. Sci. 21 (3), 1102. doi:10.3390/ijms21031102
Lei, L., Tan, L., and Sui, L. (2022). A novel cuproptosis-related gene signature for predicting prognosis in cervical cancer. Front. Genet. 13, 957744. doi:10.3389/fgene.2022.957744
Li, Y. (2020). Copper homeostasis: emerging target for cancer treatment. IUBMB Life 72 (9), 1900–1908. doi:10.1002/iub.2341
Li, W., Gu, Y., Liu, S., Ruan, F., and Lv, W. (2022). GLP1R inhibits the progression of endometrial carcinoma through activation of cAMP/PKA pathway. J. Clin. Lab. Anal. 36 (10), e24604. doi:10.1002/jcla.24604
Li, B., Li, X., Ma, M., Shi, J., and Wu, C. (2023). Identification of a novel cuproptosis-associated lncRNA model that can improve prognosis prediction in uterine corpus endometrial carcinoma. Heliyon 9 (12), e22665. doi:10.1016/j.heliyon.2023.e22665
Li, T., Wang, X., Niu, M., Wang, M., Zhou, J., Wu, K., et al. (2023). Bispecific antibody targeting TGF-β and PD-L1 for synergistic cancer immunotherapy. Front. Immunol. 14, 1196970. doi:10.3389/fimmu.2023.1196970
Li, N., Yu, K., Huang, D., Li, S., Zeng, D., Li, J., et al. (2024). Molecular characterization of cuproptosis-related lncRNAs: defining molecular subtypes and a prognostic signature of ovarian cancer. Biol. Trace Elem. Res. 202 (4), 1428–1445. doi:10.1007/s12011-023-03780-3
Liang, C., Jiang, Y., and Sun, L. (2023). Vitexin suppresses the proliferation, angiogenesis and stemness of endometrial cancer through the PI3K/AKT pathway. Pharm. Biol. 61 (1), 581–589. doi:10.1080/13880209.2023.2190774
Lin, L., Gao, Y., Hu, X., Ouyang, J., and Liu, C. (2022). Pentamidine inhibits proliferation, migration and invasion in endometrial cancer via the PI3K/AKT signaling pathway. BMC Womens Health 22 (1), 470. doi:10.1186/s12905-022-02078-1
Lin, S., Xu, Y., Liu, B., Zheng, L., Cao, C., Wu, P., et al. (2023). A novel cuproptosis-related gene signature for overall survival prediction in uterine corpus endometrial carcinoma (UCEC). Heliyon 9 (4), e14613. doi:10.1016/j.heliyon.2023.e14613
Liu, H., Zhang, L., Zhang, X., and Cui, Z. (2017). PI3K/AKT/mTOR pathway promotes progestin resistance in endometrial cancer cells by inhibition of autophagy. Onco Targets Ther. 10, 2865–2871. doi:10.2147/ott.S95267
Liu, X., Cheng, W., Li, H., and Song, Y. (2022a). Identification and validation of cuproptosis-related LncRNA signatures as a novel prognostic model for head and neck squamous cell cancer. Cancer Cell. Int. 22 (1), 345. doi:10.1186/s12935-022-02762-0
Liu, X., Zhou, L., Gao, M., Dong, S., Hu, Y., and Hu, C. (2022b). Signature of seven cuproptosis-related lncRNAs as a novel biomarker to predict prognosis and therapeutic response in cervical cancer. Front. Genet. 13, 989646. doi:10.3389/fgene.2022.989646
Liu, L., Wang, Q., Zhou, J. Y., and Zhang, B. (2023a). Developing four cuproptosis-related lncRNAs signature to predict prognosis and immune activity in ovarian cancer. J. Ovarian Res. 16 (1), 88. doi:10.1186/s13048-023-01165-7
Liu, L., Zheng, J., Xia, H., Wu, Q., Cai, X., Ji, L., et al. (2023b). Construction and comprehensive analysis of a curoptosis-related lncRNA signature for predicting prognosis and immune response in cervical cancer. Front. Genet. 14, 1023613. doi:10.3389/fgene.2023.1023613
Lönnerdal, B. (2008). Intestinal regulation of copper homeostasis: a developmental perspective. Am. J. Clin. Nutr. 88 (3), 846S–850s. doi:10.1093/ajcn/88.3.846S
Lutsenko, S., Barnes, N. L., Bartee, M. Y., and Dmitriev, O. Y. (2007). Function and regulation of human copper-transporting ATPases. Physiol. Rev. 87 (3), 1011–1046. doi:10.1152/physrev.00004.2006
Margalioth, E. J., Schenker, J. G., and Chevion, M. (1983). Copper and zinc levels in normal and malignant tissues. Cancer 52 (5), 868–872. doi:10.1002/1097-0142(19830901)52:5<868::aid-cncr2820520521>3.0.co;2-k
Montagut, C., and Settleman, J. (2009). Targeting the RAF-MEK-ERK pathway in cancer therapy. Cancer Lett. 283 (2), 125–134. doi:10.1016/j.canlet.2009.01.022
Noorolyai, S., Shajari, N., Baghbani, E., Sadreddini, S., and Baradaran, B. (2019). The relation between PI3K/AKT signalling pathway and cancer. Gene 698, 120–128. doi:10.1016/j.gene.2019.02.076
O’Day, S. J., Eggermont, A. M., Chiarion-Sileni, V., Kefford, R., Grob, J. J., Mortier, L., et al. (2013). Final results of phase III SYMMETRY study: randomized, double-blind trial of elesclomol plus paclitaxel versus paclitaxel alone as treatment for chemotherapy-naive patients with advanced melanoma. J. Clin. Oncol. 31 (9), 1211–1218. doi:10.1200/jco.2012.44.5585
Okado-Matsumoto, A., and Fridovich, I. (2001). Subcellular distribution of superoxide dismutases (SOD) in rat liver: Cu,Zn-SOD in mitochondria. J. Biol. Chem. 276 (42), 38388–38393. doi:10.1074/jbc.M105395200
Oliveri, V. (2022). Selective targeting of cancer cells by copper ionophores: an overview. Front. Mol. Biosci. 9, 841814. doi:10.3389/fmolb.2022.841814
Ostrakhovitch, E. A., Lordnejad, M. R., Schliess, F., Sies, H., and Klotz, L. O. (2002). Copper ions strongly activate the phosphoinositide-3-kinase/Akt pathway independent of the generation of reactive oxygen species. Arch. Biochem. Biophys. 397 (2), 232–239. doi:10.1006/abbi.2001.2559
Pang, X., Li, F., Lu, M., and Zhu, L. (2023). Coordination of single-cell and bulk RNA sequencing to construct a cuproptosis-related gene prognostic index for endometrial cancer prognosis, immune microenvironment infiltration, and immunotherapy treatment options. J. Cancer 14 (16), 3078–3098. doi:10.7150/jca.86325
Qi, S., Feng, H., and Li, X. (2023). LncRNAs signatures associated with cuproptosis predict the prognosis of endometrial cancer. Front. Genet. 14, 1120089. doi:10.3389/fgene.2023.1120089
Qiu, L., Ding, X., Zhang, Z., and Kang, Y. J. (2012). Copper is required for cobalt-induced transcriptional activity of hypoxia-inducible factor-1. J. Pharmacol. Exp. Ther. 342 (2), 561–567. doi:10.1124/jpet.112.194662
Qu, T., Zhang, S. M., Yu, L. L., Zhang, S., Yuan, D. Z., Xu, Q., et al. (2015). Relocalisation and activation of integrins induced rapidly by oestrogen via G-protein-coupled receptor 30 in mouse blastocysts. Reprod. Fertil. Dev. 28, 1679. doi:10.1071/rd14227
Roncolato, F., Lindemann, K., Willson, M. L., Martyn, J., and Mileshkin, L. (2019). PI3K/AKT/mTOR inhibitors for advanced or recurrent endometrial cancer. Cochrane Database Syst. Rev. 10 (10), Cd012160. doi:10.1002/14651858.CD012160.pub2
Rowland, E. A., Snowden, C. K., and Cristea, I. M. (2018). Protein lipoylation: an evolutionarily conserved metabolic regulator of health and disease. Curr. Opin. Chem. Biol. 42, 76–85. doi:10.1016/j.cbpa.2017.11.003
Samimi, G., Varki, N. M., Wilczynski, S., Safaei, R., Alberts, D. S., and Howell, S. B. (2003). Increase in expression of the copper transporter ATP7A during platinum drug-based treatment is associated with poor survival in ovarian cancer patients. Clin. Cancer Res. 9 (16 Pt 1), 5853–5859.
Sellers, K., Fox, M. P., Bousamra, M., Slone, S. P., Higashi, R. M., Miller, D. M., et al. (2015). Pyruvate carboxylase is critical for non-small-cell lung cancer proliferation. J. Clin. Investig. 125 (2), 687–698. doi:10.1172/jci72873
Shanbhag, V., Jasmer-McDonald, K., Zhu, S., Martin, A. L., Gudekar, N., Khan, A., et al. (2019). ATP7A delivers copper to the lysyl oxidase family of enzymes and promotes tumorigenesis and metastasis. Proc. Natl. Acad. Sci. U. S. A. 116 (14), 6836–6841. doi:10.1073/pnas.1817473116
Sheline, C. T., and Choi, D. W. (2004). Cu2+ toxicity inhibition of mitochondrial dehydrogenases in vitro and in vivo. Ann. Neurol. 55 (5), 645–653. doi:10.1002/ana.20047
Solmonson, A., and DeBerardinis, R. J. (2018). Lipoic acid metabolism and mitochondrial redox regulation. J. Biol. Chem. 293 (20), 7522–7530. doi:10.1074/jbc.TM117.000259
Soncin, F., Guitton, J. D., Cartwright, T., and Badet, J. (1997). Interaction of human angiogenin with copper modulates angiogenin binding to endothelial cells. Biochem. Biophys. Res. Commun. 236 (3), 604–610. doi:10.1006/bbrc.1997.7018
Sorokin, A. V., and Chen, J. (2013). MEMO1, a new IRS1-interacting protein, induces epithelial-mesenchymal transition in mammary epithelial cells. Oncogene 32 (26), 3130–3138. doi:10.1038/onc.2012.327
Sturtz, L. A., Diekert, K., Jensen, L. T., Lill, R., and Culotta, V. C. (2001). A fraction of yeast Cu,Zn-superoxide dismutase and its metallochaperone, CCS, localize to the intermembrane space of mitochondria. A physiological role for SOD1 in guarding against mitochondrial oxidative damage. J. Biol. Chem. 276 (41), 38084–38089. doi:10.1074/jbc.M105296200
Sung, H., Ferlay, J., Siegel, R. L., Laversanne, M., Soerjomataram, I., Jemal, A., et al. (2021). Global cancer Statistics 2020: GLOBOCAN estimates of incidence and mortality worldwide for 36 cancers in 185 countries. CA Cancer J. Clin. 71 (3), 209–249. doi:10.3322/caac.21660
Tang, B., Wu, M., Zhang, L., Jian, S., Lv, S., Lin, T., et al. (2023). Combined treatment of disulfiram with PARP inhibitors suppresses ovarian cancer. Front. Oncol. 13, 1154073. doi:10.3389/fonc.2023.1154073
Tisato, F., Marzano, C., Porchia, M., Pellei, M., and Santini, C. (2010). Copper in diseases and treatments, and copper-based anticancer strategies. Med. Res. Rev. 30 (4), 708–749. doi:10.1002/med.20174
Tonucci, F. M., Almada, E., Borini-Etichetti, C., Pariani, A., Hidalgo, F., Rico, M. J., et al. (2019). Identification of a CIP4 PKA phosphorylation site involved in the regulation of cancer cell invasiveness and metastasis. Cancer Lett. 461, 65–77. doi:10.1016/j.canlet.2019.07.006
Tsang, T., Posimo, J. M., Gudiel, A. A., Cicchini, M., Feldser, D. M., and Brady, D. C. (2020). Copper is an essential regulator of the autophagic kinases ULK1/2 to drive lung adenocarcinoma. Nat. Cell. Biol. 22 (4), 412–424. doi:10.1038/s41556-020-0481-4
Tsvetkov, P., Detappe, A., Cai, K., Keys, H. R., Brune, Z., Ying, W., et al. (2019). Mitochondrial metabolism promotes adaptation to proteotoxic stress. Nat. Chem. Biol. 15 (7), 681–689. doi:10.1038/s41589-019-0291-9
Tsvetkov, P., Coy, S., Petrova, B., Dreishpoon, M., Verma, A., Abdusamad, M., et al. (2022). Copper induces cell death by targeting lipoylated TCA cycle proteins. Science 375 (6586), 1254–1261. doi:10.1126/science.abf0529
Vitaliti, A., De Luca, A., and Rossi, L. (2022). Copper-dependent kinases and their role in cancer inception, progression and metastasis. Biomolecules 12 (10), 1520. doi:10.3390/biom12101520
Walter, P. L., Kampkötter, A., Eckers, A., Barthel, A., Schmoll, D., Sies, H., et al. (2006). Modulation of FoxO signaling in human hepatoma cells by exposure to copper or zinc ions. Arch. Biochem. Biophys. 454 (2), 107–113. doi:10.1016/j.abb.2006.08.016
Wang, P., Zeng, Z., Shen, X., Tian, X., and Ye, Q. (2020). Identification of a multi-RNA-type-based signature for recurrence-free survival prediction in patients with uterine corpus endometrial carcinoma. DNA Cell. Biol. 39 (4), 615–630. doi:10.1089/dna.2019.5148
Wang, Q., and Xu, Y. (2022). Comprehensive analysis of cuproptosis-related lncRNAs model in tumor immune microenvironment and prognostic value of cervical cancer. Front. Pharmacol. 13, 1065701. doi:10.3389/fphar.2022.1065701
Wang, X., Dai, C., Ye, M., Wang, J., Lin, W., and Li, R. (2021). Prognostic value of an autophagy-related long-noncoding-RNA signature for endometrial cancer. Aging (Albany NY) 13 (4), 5104–5119. doi:10.18632/aging.202431
Wang, X., Wang, Y., Sun, F., Xu, Y., Zhang, Z., Yang, C., et al. (2022). Novel LncRNA ZFHX4-AS1 as a potential prognostic biomarker that affects the immune microenvironment in ovarian cancer. Front. Oncol. 12, 945518. doi:10.3389/fonc.2022.945518
Wang, K., Zhang, Y., Ao, M., Luo, H., Mao, W., and Li, B. (2023). Multi-omics analysis defines a cuproptosis-related prognostic model for ovarian cancer: implication of WASF2 in cuproptosis resistance. Life Sci. 332, 122081. doi:10.1016/j.lfs.2023.122081
Webb, P. M., and Jordan, S. J. (2017). Epidemiology of epithelial ovarian cancer. Best. Pract. Res. Clin. Obstet. Gynaecol. 41, 3–14. doi:10.1016/j.bpobgyn.2016.08.006
Xiao, Q., and Ge, G. (2012). Lysyl oxidase, extracellular matrix remodeling and cancer metastasis. Cancer Microenviron. 5 (3), 261–273. doi:10.1007/s12307-012-0105-z
Xie, J., Yang, Y., Gao, Y., and He, J. (2023). Cuproptosis: mechanisms and links with cancers. Mol. Cancer 22 (1), 46. doi:10.1186/s12943-023-01732-y
Yaman, M., Kaya, G., and Simsek, M. (2007). Comparison of trace element concentrations in cancerous and noncancerous human endometrial and ovary tissues. Int. J. Gynecol. Cancer 17 (1), 220–228. doi:10.1111/j.1525-1438.2006.00742.x
Yang, X., Zhang, S., He, C., Xue, P., Zhang, L., He, Z., et al. (2020). METTL14 suppresses proliferation and metastasis of colorectal cancer by down-regulating oncogenic long non-coding RNA XIST. Mol. Cancer 19 (1), 46. doi:10.1186/s12943-020-1146-4
Yang, L., Yao, C., Su, Z., Fang, Y., Pandey, N. K., Amador, E., et al. (2024). Combination of disulfiram and Copper-Cysteamine nanoparticles induces mitochondria damage and promotes apoptosis in endometrial cancer. Bioact. Mater 36, 96–111. doi:10.1016/j.bioactmat.2024.02.009
Yu, H., Wang, H., Qie, A., Wang, J., Liu, Y., Gu, G., et al. (2021). FGF13 enhances resistance to platinum drugs by regulating hCTR1 and ATP7A via a microtubule-stabilizing effect. Cancer Sci. 112 (11), 4655–4668. doi:10.1111/cas.15137
Yuan, M., Wu, Q., Zhang, M., Lai, M., Chen, W., Yang, J., et al. (2023). Disulfiram enhances the antitumor activity of cisplatin by inhibiting the Fanconi anemia repair pathway. J. Zhejiang Univ. Sci. B 24 (3), 207–220. doi:10.1631/jzus.B2200405
Zhang, M., Shi, M., and Zhao, Y. (2018). Association between serum copper levels and cervical cancer risk: a meta-analysis. Biosci. Rep. 38 (4). doi:10.1042/bsr20180161
Zhang, X., Zhao, X., Li, Y., Zhou, Y., and Zhang, Z. (2019). Long noncoding RNA SOX21-AS1 promotes cervical cancer progression by competitively sponging miR-7/VDAC1. J. Cell. Physiol. 234 (10), 17494–17504. doi:10.1002/jcp.28371
Zhang, J., Wang, Z., Zhao, R., An, L., Zhou, X., Zhao, Y., et al. (2020). An integrated autophagy-related gene signature predicts prognosis in human endometrial Cancer. BMC Cancer 20 (1), 1030. doi:10.1186/s12885-020-07535-4
Zhang, F., Ni, Z. J., Ye, L., Zhang, Y. Y., Thakur, K., Cespedes-Acuña, C. L., et al. (2021). Asparanin A inhibits cell migration and invasion in human endometrial cancer via Ras/ERK/MAPK pathway. Food Chem. Toxicol. 150, 112036. doi:10.1016/j.fct.2021.112036
Zhang Z., , Ma, Y., Guo, X., Du, Y., Zhu, Q., Wang, X., et al. (2021). FDX1 can impact the prognosis and mediate the metabolism of lung adenocarcinoma. Front. Pharmacol. 12, 749134. doi:10.3389/fphar.2021.749134
Zhang, J., Lu, M., Xu, H., Ren, F., and Zhu, L. (2022). Molecular subtypes based on cuproptosis-related genes and tumor microenvironment infiltration characterization in ovarian cancer. Cancer Cell. Int. 22 (1), 328. doi:10.1186/s12935-022-02756-y
Zhang, W., Zhai, Q., Li, M., Huang, S., Sun, Z., Yan, Z., et al. (2022). Anti-cancer effects of disulfiram in cervical cancer cell lines are mediated by both autophagy and apoptosis. Bull. Exp. Biol. Med. 172 (5), 642–648. doi:10.1007/s10517-022-05447-w
Zheng, P., Wu, Y., Wang, Y., and Hu, F. (2023). Disulfiram suppresses epithelial-mesenchymal transition (EMT), migration and invasion in cervical cancer through the HSP90A/NDRG1 pathway. Cell. Signal 109, 110771. doi:10.1016/j.cellsig.2023.110771
Zhou, J., Xu, L., Zhou, H., Wang, J., and Xing, X. (2023). Prediction of prognosis and chemotherapeutic sensitivity based on cuproptosis-associated lncRNAs in cervical squamous cell carcinoma and endocervical adenocarcinoma. Genes. (Basel) 14 (7), 1381. doi:10.3390/genes14071381
Glossary
Keywords: copper homeostasis, cuproptosis, gynecological cancers, prognosis prediction, functional analysis, copper ionophore
Citation: Huang X, Lian M and Li C (2024) Copper homeostasis and cuproptosis in gynecological cancers. Front. Cell Dev. Biol. 12:1459183. doi: 10.3389/fcell.2024.1459183
Received: 03 July 2024; Accepted: 06 September 2024;
Published: 25 September 2024.
Edited by:
Ming Yi, Zhejiang University, ChinaReviewed by:
Qian Yang, Zhejiang University, ChinaRuidi Yu, Huazhong University of Science and Technology, China
Copyright © 2024 Huang, Lian and Li. This is an open-access article distributed under the terms of the Creative Commons Attribution License (CC BY). The use, distribution or reproduction in other forums is permitted, provided the original author(s) and the copyright owner(s) are credited and that the original publication in this journal is cited, in accordance with accepted academic practice. No use, distribution or reproduction is permitted which does not comply with these terms.
*Correspondence: Changzhong Li, 15168888909@163.com