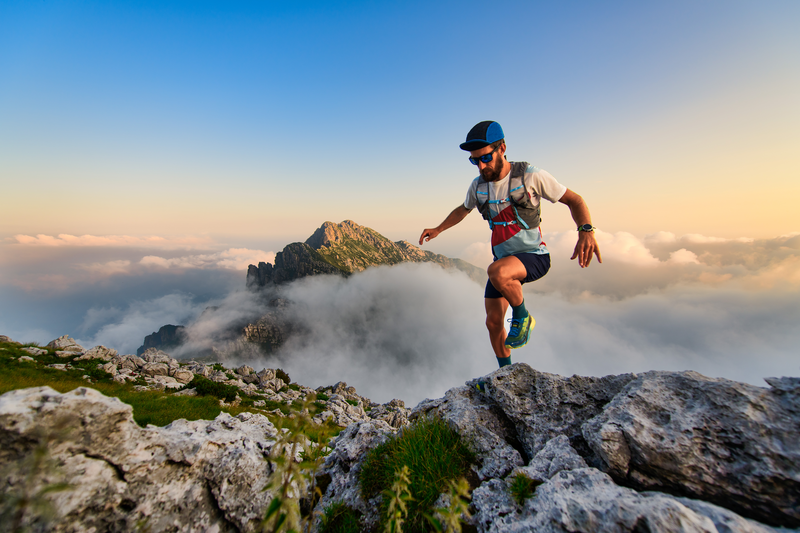
95% of researchers rate our articles as excellent or good
Learn more about the work of our research integrity team to safeguard the quality of each article we publish.
Find out more
REVIEW article
Front. Cell Dev. Biol. , 05 August 2024
Sec. Cancer Cell Biology
Volume 12 - 2024 | https://doi.org/10.3389/fcell.2024.1442193
The role of long noncoding RNA (lncRNA) in tumors, particularly in gastrointestinal tumors, has gained significant attention. Accumulating evidence underscores the interaction between various lncRNAs and diverse molecular pathways involved in cancer progression. One such pivotal pathway is the PI3K/AKT pathway, which serves as a crucial intracellular mechanism maintaining the balance among various cellular physiological processes for normal cell growth and survival. Frequent dysregulation of the PI3K/AKT pathway in cancer, along with aberrant activation, plays a critical role in driving tumorigenesis. LncRNAs modulate the PI3K/AKT signaling pathway through diverse mechanisms, primarily by acting as competing endogenous RNA to regulate miRNA expression and associated genes. This interaction significantly influences fundamental biological behaviors such as cell proliferation, metastasis, and drug resistance. Abnormal expression of numerous lncRNAs in gastrointestinal tumors often correlates with clinical outcomes and pathological features in patients with cancer. Additionally, these lncRNAs influence the sensitivity of tumor cells to chemotherapy in multiple types of gastrointestinal tumors through the abnormal activation of the PI3K/AKT pathway. These findings provide valuable insights into the mechanisms underlying gastrointestinal tumors and potential therapeutic targets. However, gastrointestinal tumors remain a significant global health concern, with increasing incidence and mortality rates of gastrointestinal tumors over recent decades. This review provides a comprehensive summary of the latest research on the interactions of lncRNA and the PI3K/AKT pathway in gastrointestinal tumor development. Additionally, it focuses on the functions of lncRNAs and the PI3K/AKT pathway in carcinogenesis, exploring expression profiles, clinicopathological characteristics, interaction mechanisms with the PI3K/AKT pathway, and potential clinical applications.
Long noncoding RNAs (lncRNAs) are a set of noncoding RNAs comprising more than 200 nucleotides in length and constitute approximately 60% of human cell transcriptional output (Mattick and Makunin, 2006; Djebali et al., 2012; Tao et al., 2023). As RNA sequencing methods and epigenomic techniques advance, novel lncRNA types and their distinct cellular functions continue to emerge (Anastasiadou et al., 2018b; Qian et al., 2019; Saw et al., 2021; Herman et al., 2022). Despite lacking protein-coding ability, lncRNAs play crucial roles in regulating gene expression, maintaining chromosomal stability, and influencing alternative splicing, depending on their cellular location (Bridges et al., 2021; Tan et al., 2021; Zhao et al., 2022). Recent studies highlight their significance as regulators of diverse physiological and pathological processes (Romeo et al., 2023). Interacting with various RNAs, lncRNAs affect multiple molecular targets, modulating their stability and downstream gene expression (Kopp and Mendell, 2018; Shaath et al., 2022; Yao et al., 2022; Li et al., 2024). Aberrant lncRNA expressions have been identified across various human tumors and diseases, spanning gastric cancer (GC), breast cancer, liver cancer (LC), melanoma, and cardiovascular diseases (Anastasiadou et al., 2018a; Yan and Bu, 2021). Given the distinct expression profiles and association with patient prognosis, various lncRNAs serve as potent biomarkers for diagnosing and predicting human disorders (Ozawa et al., 2017; Peng et al., 2018; Costa et al., 2023). In particular, lncRNAs have emerged as critical regulators and exert their functions mainly through intricate competing endogenous RNA (ceRNA) networks in gastrointestinal tumors including GC, LC, colorectal cancer (CRC), pancreatic cancer (PC), and esophageal cancer (EC) (Guo et al., 2015; Chan and Tay, 2018; Wang et al., 2019a; Mo et al., 2022; Xue et al., 2023). The expression levels of certain lncRNAs, whether upregulated or downregulated, are strongly associated with the clinicopathological characteristics of patients with gastrointestinal tumors (Xiao et al., 2021; Lulli et al., 2022; Wei et al., 2022). Dysregulated lncRNAs actively contribute to cell proliferation, migration, invasion, epithelial-to-mesenchymal transition (EMT), apoptosis, and drug resistance during the initiation and development of multiple gastrointestinal tumors (Panzitt et al., 2007; Wang X. et al., 2017; Zheng et al., 2018; Wang N. et al., 2019; Lv et al., 2021). As RNA-based therapeutics gain traction in clinical settings, understanding intricate lncRNA mechanisms is essential for the effective diagnosis and treatment of gastrointestinal tumors (Ozawa et al., 2017; Wang et al., 2019b; Sun et al., 2023).
The phosphatidylinositol 3-kinase (PI3K) pathway serves as a crucial intracellular signaling pathway governing various cellular processes across different tissues, including cell growth, proliferation, metabolism, migration, and immune response (Katso et al., 2001; Engelman et al., 2006; Xue et al., 2021). PI3K, a pivotal lipid kinase, can be activated through multiple upstream pathways, giving rise to downstream effects (MacDougall et al., 1995; Vanhaesebroeck et al., 1997). In humans, three classes of PI3Ks—class I, II, and III—exist, with an extensive focus on class I PI3Ks (Vanhaesebroeck et al., 2010; Okkenhaug, 2013; Jean and Kiger, 2014). Class I PI3Ks form heterodimers from the merging of p110 catalytic subunits and p85 regulatory subunits (Cui et al., 2014; Zhang et al., 2020; Hart et al., 2022). Four isoforms of p110—p110α, p110β, p110γ, and p110δ—encoded by PIK3CA, PIK3CB, PIK3CG, and PIK3CD, respectively, are part of this class. The regulatory subunit p85 exists in three forms, namely, p85α, p85β, and p85γ, with p85α being the most abundantly expressed regulatory subunit (Haug et al., 1991; Fox et al., 2020; Chaudhuri et al., 2023). Class II PI3Ks lack regulatory subunits, primarily characterized as monomer enzymes. Class II PI3Ks exist as three catalytic subtypes, including PI3KC2α and PI3KC2β, which are widely distributed, while PI3KC2γ is specifically found in the liver (Gulluni et al., 2019; Thibault et al., 2023). Moreover, VPS34, the sole member of class III PI3K, forms tetrameric complexes I and II with regulatory and catalytic subunits (Backer, 2008; Reidick et al., 2017; Ohashi et al., 2019; Alkhoury et al., 2023). Generally, mutations in the PI3K gene, amplifications, increased activity of oncogenic proteins upstream, and loss of tumor suppressor genes all contribute to elevated PI3K activity in tumors. Activation of PI3K occurs through the combination of receptor tyrosine kinases and various growth factor ligands such as platelet-derived growth factor, epidermal growth factor (EGF), and insulin-like growth factor (IGF) (Chang et al., 2003). Activated PI3K generates phosphatidylinositol 3,4,5-trisphosphate (PIP3) from phosphatidylinositol 4,5-bisphosphate (PIP2), recruiting downstream signaling proteins to activate downstream signaling pathways such as AGC serine/threonine kinases (AKT) (Calamito et al., 2010; Chin and Toker, 2011). Subsequently, AKT phosphorylates downstream effectors in a sequence-dependent manner, influencing a wide array of functions, commonly recognizing the motif (R-X-R-X-X-S/T; Figure 1) (Obata et al., 2000; Nag et al., 2023; Zhou et al., 2023).
Figure 1. Activation process of the PI3K/AKT pathway. Upon activation by receptor-coupled tyrosine kinases in response to extracellular stimuli, PI3K phosphorylates phosphatidylinositol 4,5-bisphosphate (PIP2) to generate phosphatidylinositol 3,4,5-trisphosphate (PIP3). Subsequently, PI3P recruits and phosphorylates AKT at the plasma membrane, thereby exerting a spectrum of biological effects.
Hyperactivation of the PI3K pathway has been extensively studied in multiple tumors, highlighting its integral role in tumor development and progression (Altomare and Testa, 2005; Ediriweera et al., 2019; Miricescu et al., 2020). In addition, PI3K mutations, particularly in the PIK3CA gene, are frequently observed in colorectal, gastric, and liver cancers. These mutations lead to constitutive activation of the PI3K-AKT pathway, promoting uncontrolled cell proliferation, survival, metastasis, and invasion (Huang D. et al., 2018; Liu W. et al., 2022; Chong et al., 2022). Animal models have been instrumental in elucidating the role of PI3K-AKT pathway mutations in gastrointestinal tumors. For instance, transgenic mice with hepatocyte-specific PTEN deficiency leads to the development of liver tumors, highlights the oncogenic potential of PI3K activation (Horie et al., 2004). Similarly, mouse models with intestinal epithelial-specific PIK3CA mutations exhibit increased tumorigenesis, supporting the role of PI3K-Akt signaling in colorectal cancer development (Cathomas, 2014; Chen et al., 2022; Wang et al., 2023). Therapeutic strategies targeting the PI3K pathway, owing to the core role of PI3K signaling in numerous tumor pathogenic processes, are rapidly evolving and entering preclinical and early clinical trials (Beck et al., 2014; Alzahrani, 2019; Tewari et al., 2022; Yu et al., 2022). Dysregulation of the PI3K pathway, stimulated by various factors such as epigenetic alterations and environmental influences, is observed in gastrointestinal tumors, contributing to uncontrolled cell proliferation, survival, cell cycle progression, resistance to apoptosis, and chemoresistance (Chang et al., 2003; Danielsen et al., 2015; Papadatos-Pastos et al., 2015; Liu R. et al., 2020). The PI3K pathway’s involvement in mediating the oncogenic effects of several lncRNAs has been identified to contribute to tumor growth and metastasis in gastrointestinal tumors (Wang Y. et al., 2020; Zhao et al., 2020; Fang L. et al., 2022). Preclinical and clinical studies have highlighted the potential of targeting the PI3K pathway as a therapeutic intervention for gastrointestinal tumors mainly based on the influence on tumor resistance to antineoplastic drugs (Ng et al., 2000; O’gorman and Cotter, 2001; Mueller et al., 2012; Du J. et al., 2022). Continued exploration of the mechanisms underlying PI3K pathway dysregulation will aid in developing targeted therapies, potentially improving outcomes for patients with gastrointestinal tumors (Michl and Downward, 2005; Zhang et al., 2011; Su et al., 2022).
This review comprehensively outlines the dysregulated expression patterns of lncRNAs and their correlation with clinicopathological features in patients with gastrointestinal tumors. Additionally, we also elucidate the mechanisms underlying the crosstalk between lncRNAs and the PI3K/AKT pathway in gastrointestinal tumors. Given the pivotal role of this interaction, the potential implications for precise diagnosis, prognosis, and therapeutic interventions have been explored.
Recent research indicates that lncRNAs can modulate the activity of the PI3K signaling pathway through various mechanisms, thereby influencing the development of gastrointestinal tumors (Table 1) (Shang et al., 2019; Luo J. et al., 2021; Almalki, 2023). Numerous studies have highlighted the role of lncRNAs as competing endogenous RNAs (ceRNAs), where they competitively bind to microRNAs (miRNAs), mediating the expression of downstream target genes and regulating the PI3K signaling pathway (Table 2) (Wei et al., 2019; Rahmani et al., 2020; Kumar et al., 2023). Additionally, the PI3K signaling pathway can influence the expression levels of lncRNAs through a series of downstream effector proteins (Dai et al., 2020; Zhong et al., 2022). The abnormal interaction between lncRNAs and the PI3K/AKT pathway ultimately affects multiple cellular processes and tumor progression. This section emphasizes the molecular mechanisms by which lncRNAs modulate the oncogenic PI3K/AKT pathway, impacting various biological processes in gastrointestinal tumors, including cell proliferation, migration, invasion, and chemoresistance (Figure 2) (He et al., 2021).
Figure 2. Overview of the interaction of lncRNA and the PI3K/AKT pathway in gastrointestinal tumors. Dysregulation of long non-coding RNAs (lncRNAs) is common in gastrointestinal tumors, playing intricate roles in both oncogenic and tumor-suppressor processes contingent upon the specific molecular interactions in the context of cancer. Specifically, various lncRNAs act as sponges, binding completely with miRNAs, consequently modulating target gene expression and influencing the activity of the PI3K/AKT pathway. This altered pathway activity is considered pivotal in mediating the oncogenic effects induced by lncRNAs, contributing to aberrant cellular processes and tumor progression.
In GC, upregulated expression of the lncRNA AK023391 was observed in clinical samples and cell lines, and the expression level was positively associated with poor overall survival (OS) among patients with GC. Both in vitro and in vivo experiments demonstrated that overexpression of AK023391 prompted cell growth, colony formation, and invasion and hindered cell apoptosis and cell cycle arrest. Mechanistic exploration revealed that the lncRNA AK023391 interacted with the PI3K/AKT signaling pathway, subsequently triggering NF-κB and inactivating FOXO3a to exert its oncogenic effects. This was accompanied by upregulated expression of c-myb, cyclinB1/g2, and bcl-6, as well as downregulation of the p53 pathways in GC (Huang et al., 2017). Furthermore, the lncRNA THAP7-AS1 exhibited overexpression in GC tissues with lymph node metastasis, and it is closely associated with the poorer American Joint Committee on Cancer staging and prognosis. Analysis using receiver operating characteristic curves indicated that the lncRNA THAP7-AS1 effectively distinguished between GC cases with and without lymph node metastasis (area under the curve = 0.7367). Cellular experiments demonstrated that high expression of THAP7-AS1 promoted the proliferation, invasion, and migration abilities of GC cells (Liu J. et al., 2023). Mechanistic investigations unveiled that the transcription factor SP1 activates the transcription of THAP7-AS1, while METTL3 mediates m6A modification of THAP7-AS1 through an IGF2BP1-dependent mechanism, enhancing its expression level. Upregulated expression of THAP7-AS1 facilitates the nuclear entry of the CUL4B protein, inhibiting the transcription of miR-22-3p and miR-320a, subsequently activating the PI3K/AKT signaling pathway and exerting oncogenic properties in GC (Liu H. T. et al., 2022). Moreover, LINC00152 has shown upregulated expression in GC tissues compared with that in noncancerous tissue samples. Clinical feature analysis demonstrated a significant correlation between LINC00152 expression in GC tissues and pathological features such as tumor size. Functional experiments using shRNA-mediated knockdown of LINC00152 in MGC803 and HGC-27 cells confirmed that its downregulation inhibited cell proliferation. RNA pulldown experiments and RNA immunoprecipitation (RIP) assays showed that LINC00152 interacted with EGF receptor (EGFR) to constitutively activate EGFR and subsequently, the PI3K/AKT signaling pathway, leading to increased tumor cell proliferation (Zhou et al., 2015). Additionally, upregulated expression of LINC01559 in GC tissues was identified to be transmitted from mesenchymal stem cells to GC cells in the form of exosomes. After uptake and entry into GC cells, LINC01559 promotes cell proliferation, migration, and stemness by upregulating phosphoglycerate kinase 1 (PGK1) through miR-1343-3p sponge activity and inducing phosphatase and tensin homolog (PTEN) promoter methylation, resulting in downregulation. These processes ultimately activated the PI3K/AKT pathway, accelerating GC progression (Wang et al., 2020a). Analysis of data from The Cancer Genome Atlas (TCGA) and Gene Expression Omnibus (GEO) online databases revealed that the expression of both LOC101929709 and LIN28B is significantly elevated in GC tissues. LOC101929709, through its interaction with LIN28B, facilitated the recognition and stabilization of m6A-modified c-MYC mRNA, activating the PI3K/AKT pathway and promoting proliferation, migration, and glycolysis in GC cells (Xu et al., 2023). Exosomes derived from Fusobacterium nucleatum–infected GC cells (Fn-GCEx) contained increased HOTTIP. Elevated HOTTIP in exosomes acted as an oncogene by sponging miRNA-885-3p, contributing to the overexpression of ephrin type B receptor 2 (EphB2) in uninfected AGS and HGC-27 cells. Further experiments confirmed that EphB2 enhanced GC progression through the activation of the PI3K/AKT pathway (Xin et al., 2023). Moreover, the relative expression of colon cancer–associated transcript-1 (CCAT1) was upregulated in GC tissues. CCAT1 expression exerts promising clinical reference value because of the close correlation with clinicopathological features such as tumor diameter, venous/lymphatic invasion, and clinical biochemical indexes. Cantharidin exerts an antitumor effect by significantly inhibiting the invasion and metastasis of GC cells through the downregulation of CCAT1, thereby blocking PI3K/AKT pathway signaling (Song et al., 2020). Additionally, FOXD1-AS1 exhibits high expression levels in GC cell lines and tissues. FOXD1-AS1 interacts with miR-466, stimulating the PI3K/AKT signaling pathway and increasing FOXD1 protein levels through the eIF4G-eIF4E-eIF4A translation complex. Ultimately, FOXD1 overexpression enhanced cell growth, migration, and chemoresistance of GC cells mediated by FOXD1-AS1 (Wu Q. et al., 2021). Furthermore, upregulated expression of LINC00511 in GC cells promotes cell proliferation, migration, stemness, and the EMT process while inhibiting apoptosis. Mechanistic experiments revealed that the transcription of the oncogene LINC00511 is triggered by SRY-box transcription factor 4 (SOX4). High levels of LINC00511 recruit enhancers of zeste 2 polycomb repressive complex 2 subunit (EZH2) to boost PTEN promoter methylation, inhibiting PTEN expression and driving the PI3K/AKT signaling pathway. Moreover, LINC00511 increases SOX4 expression by sponging miR-195-5p (Wang et al., 2021). However, lncRNA TP53TG1 acted as a tumor suppressor, inhibiting proliferation, metastasis, and cell cycle progression of GC cells while promoting apoptosis. TP53TG1 was downregulated in GC, possibly due to m6A modifications of demethylase ALKBH5 reducing its stability. Low TP53TG1 expression was associated with poor OS, larger tumors, poorer differentiation, advanced TNM stage, and metastasis in patients with GC. TP53TG1 interacts with the oncogenic protein phosphatase 2A inhibitor (CIP2A), triggering its ubiquitination-mediated degradation and inhibiting the PI3K/AKT pathway, underscoring its antitumor significance in GC progression (Fang D. et al., 2022). In summary, these findings suggest that multiple lncRNAs play pivotal roles in regulating various processes of GC cells by modulating the PI3K/AKT signaling pathway.
Compared with adjacent normal tissues, lncRNA HULC expression is elevated in LC tissues. Experimental findings indicate that HULC plays a role in LC tumorigenesis by boosting the autophagy process, thereby accelerating the proliferation ability of LC cells. Mechanistically, increased HULC levels reduce mature miR15a levels, upregulate autophagy-related protein P62, and enhance the expression of histone deacetylase Sirt1, promoting autophagy in LC cells. Moreover, HULC suppresses PTEN expression through the ubiquitin-proteasome system induced by P62, activating the AKT-PI3K-mTOR signaling pathway (Xin et al., 2018). Strikingly, HULC plays a carcinogenic role together with miR24-2. miR24-2 increases the transcriptional activity of the HULC protein by inhibiting the expression of arginine methyltransferase 7 (PRMT7) in human LC stem cells. The significantly increased HULC further enhances Nanog expression and assists cellular autophagy dependent on the PI3K/AKT pathway (Wang et al., 2020b). Microarray analysis revealed high expression of the lncRNA PTTG3P in HCC tissues compared with that in adjacent normal tissues. Elevated PTTG3P levels in patients with HCC are often associated with unfavorable TNM stage, larger tumors, and poor survival. As for the tumor-promoting mechanism, PTTG3P overexpression upregulates pituitary tumor-transforming 1 (PTTG1), activating the PI3K/AKT pathway and enhancing HCC growth, cell cycle progression, EMT, and metastasis in HCC (Huang J. L. et al., 2018; Li et al., 2022).
Expression differences of the lncRNA DUXAP10 in HCC and neighboring tissues, showing higher expression in HCC tissues. DUXAP10 overexpression negatively regulates miR-1914, weakening its anticarcinogenic effects. Further experiments involving MHCC-97L and Hep3B cells clarified that DUXAP10 overexpression triggers G-protein–coupled receptor 39 (GPR39) expression and PI3K/AKT signaling, intensifying HCC malignant processes including cell proliferation, colony formation, cell cycle progression, and antiapoptosis (Sun et al., 2019). LncRNA CDKN2B-AS1 is universally overexpressed in several online HCC databases and cell lines, exerting oncogenic functions by enhancing HCC cell proliferation, mobility, migration, and invasion. Moreover, CDKN2B-AS1 has clinical significance owing to its close association with advanced tumor grade, size, stage, vascular invasion, and undesirable OS. CDKN2B-AS1 also acts as a sponge of let-7c-5p to facilitate NAP1L1 expression. Elevated expression of NAP1L1 further stimulates the activation of the PI3K/AKT pathway and promotes the oncogenic role of CDKN2B-AS1 (Huang Y. et al., 2018). Noteworthy, LINC01468 exhibits overexpression in HCC, particularly in cases related to nonalcoholic fatty liver disease–driven HCC. Elevated levels of LINC01468 correspond to poorer outcomes in terms of OS and various unfavorable pathological indicators such as uncontrolled serum biochemical levels, tumor size, TNM stage, and microvascular invasion. Experimental data suggest that enforced expression of PTTG3P significantly accelerates lipid metabolism, subsequently fostering chemoresistance and tumorigenesis. This effect occurs through the facilitation of the Src homology 2-domain-containing PtdIns (3,4,5) P3 5-phosphatase-2 (SHIP2)-cullin 4A (CUL4A) interaction, leading to the ubiquitination degradation of SHIP2. The resultant destabilization of SHIP2 further triggers the activation of the PI3K/AKT/mTOR pathway, thereby contributing to HCC progression (Wang H. et al., 2022). Additionally, the novel lncRNA-AWPPH displays heightened levels in HCC tissues and is deemed an independent prognostic factor in HCC. Its elevation is positively associated with disease-free survival, recurrence-free survival, and OS rates among patients with HCC. The lncRNA-AWPPH interacts with YBX1, facilitating the transcriptional activation of the PI3K/AKT pathway. These findings underscore the malignant roles of lncRNA-AWPPH, including its involvement in promoting cell proliferation and migration, tumor growth, and metastasis (Zhao et al., 2017). Notably, decreased levels of lncRNA PTENP1 were observed in highly metastatic HCC Mahlavu cells through quantitative reverse transcription–polymerase chain reaction (qRT-PCR) analysis. Ectopic PTENP1 expression through Sleeping Beauty–based hybrid baculovirus vectors significantly augments PTEN levels, dampens the activation of the PI3K/AKT pathway, induces autophagy and apoptosis, and suppresses cell proliferation, migration, invasion, angiogenesis, and neo vasculature maturation (Chen et al., 2015). Furthermore, the lncRNA TCL6 displays conspicuous downregulation in HCC tissues derived from the GEO dataset (GSE14520) and in HepG2, SMMC-7721, and MHCC-97H cells. Functioning as a tumor-suppressive lncRNA, TCL6 curbs the carcinogenic effects of the PI3K/AKT signaling pathway by directly interacting with miR-106a-5p, subsequently upregulating PTEN expression in HCC (Luo et al., 2020). In cholangiocarcinoma, studies demonstrate abnormal enrichment of lncRNA HCG18 in tumor tissues sourced from the TCGA database and QBC939 cells. Functional experiments affirm the cancer-promoting role of HCG18, influencing cell viability, migration, and invasion as well as the EMT process in QBC939 cells. Additionally, investigations indicate the overexpression of HCG18 in exosomes released by these cells, leading to reduced expression of miR-424-5p in surrounding cancer cells. Decreased miR-424-5p levels subsequently elevate the expression of SRY-box transcription factor 9 (SOX9) and activate the PI3K/AKT pathway, culminating in accelerated tumor progression in cholangiocarcinoma (Ni et al., 2023). Additionally, in hepatoblastoma tissues, analysis based on the GEO database showed upregulated expression of the lncRNA MIR205HG. This upregulation was confirmed in the cell cytoplasm of HepG2 and HuH-6 cells. Functional assays demonstrated that decreased MIR205HG expression hindered the proliferation, migratory, and invasive potential of HepG2 and HuH-6 cells. Furthermore, a series of experiments indicated that MIR205HG acts as a strong sponge for miR-205-5p, thereby enhancing the activity of MAPK and the PI3K/AKT pathway (Zhang et al., 2022). These observations collectively illustrate how lncRNAs intricately activate the PI3K/AKT pathway through complex mechanisms, thereby modulating various pathological functions such as apoptosis, proliferation, migration, and invasion in LC cells (Figure 3).
Figure 3. Molecular interactions of abnormal lncRNAs and the PI3K/AKT pathway in liver cancer. The aberrant interplay between lncRNAs and the PI3K/AKT pathway significantly impacts the carcinogenesis of liver cancer. Dysregulated expression of diverse lncRNAs is observed in liver cancer, closely associated with clinical characteristics and patient outcomes. Several studies have elucidated the regulatory mechanisms of lncRNAs in activating the PI3K/AKT pathway during malignant biological processes, crucial for cell growth, metastasis, survival, metabolism, and even drug resistance. Considering their pivotal expression patterns, clinical implications, and roles in carcinogenesis, the interactions between lncRNAs and the PI3K/AKT pathway offer a theoretical foundation for potential future clinical applications such as precise diagnosis, risk stratification, and targeted therapies in liver cancer.
LINC01296 exhibits a remarkable increase in CRC and shows a close association with advanced tumor stage and distant metastasis. Kaplan-Meier analysis further illustrates that elevated levels of LINC01296 correlate with poor clinical outcomes in patients with CRC. LINC01296 levels significantly influence the proliferation and invasion abilities of CRC cell lines and affect their resistance to 5-fluorouracil (5-FU) chemotherapy. Mechanistically, LINC01296 directly diminishes the levels of miR-26a, thereby augmenting the expression of GALNT3, a key enzyme involved in O-glycosylation. This elevation of GALNT3 subsequently enhances the O-glycosylation of mucin1 (MUC1) and activates the PI3K/AKT signaling pathway. The crosstalk of LINC01296-miR-26a-GALNT3-MUC1-PI3K/AKT underscores its role in promoting tumor growth, liver metastasis, and conferring chemo-resistance to 5-FU in CRC (Liu et al., 2018). Additionally, high levels of lncRNA HOTAIR are observed in CRC tissues and cell lines. HOTAIR appears to sponge miR-326, leading to increased levels of fucosyltransferase 6 (FUT6) and subsequent facilitation of CD44 fucosylation. The α1, 3-fucosylated CD44, in turn, amplifies the PI3K/AKT pathway, mediating HOTAIR’s pro-tumorigenic effects in CRC, such as accelerated cell proliferation, migration, invasion, tumorigenesis, and distant metastasis. Kaplan-Meier analysis also confirms the association between high HOTAIR expression and poor prognosis in CRC (Pan et al., 2019). Additionally, elevated levels of lncRNA TCONS_00012883 are observed in CRC tissues, as confirmed by qRT-PCR analysis. This study demonstrates that TCONS_00012883 exhibits higher expression in tumor samples with advanced tumor stages and metastasis. Moreover, Kaplan-Meier survival analysis reveals that patients with elevated levels of TCONS_00012883 experience shorter OS and progression-free survival. In vitro and in vivo experiments further establish that TCONS_00012883 amplifies cell proliferation and metastasis, thus enhancing tumor growth and metastasis. TCONS_00012883, primarily localized in the cell nucleus, upregulates the target gene matrix metallopeptidase 1 (MMP1) by interacting with DEAD-box helicase 3 (DDX3) protein and mediating reverse activation of the transcription factor YY1. This interaction ultimately contributes to the activation of the PI3K/AKT pathway, mediating the tumor-promoting functions of TCONS_00012883 in CRC (Yang et al., 2020). Recent studies have highlighted the significant increase in LINC00659 levels within CRC tissues, linking it to an oncogenic role in lncRNA. This upsurge in LINC00659 expression is associated with shortened patient survival. Knockdown of LINC00659 disrupts the regulation of the PI3K-Akt signaling pathway, exerting a suppressive influence on CRC cell cycle progression, proliferation, drug resistance, and colony formation (Tsai et al., 2018). Furthermore, lncRNA microarray analysis revealed elevated small nucleolar RNA host gene 7 (SNHG7) expression profiles in CRC tissues and metastatic CRC cell lines. SNHG7 promotes malignant progression both in vitro and in vivo. High SNHG7 levels correlate with adverse tumor characteristics such as size, lymphatic metastasis, distant metastasis, tumor stage, and OS. SNHG7 interacts with miR-34a, reducing its expression through a ceRNA mechanism. Consequently, the downstream acetylgalactosaminyltransferase GALNT7 is upregulated, causing abnormal O-glycosylation and activating mTOR, thereby initiating the PI3K/Akt pathway (Li et al., 2018). Moreover, highly metastatic CRC cells release exosomes containing elevated levels of MALAT1. These exosome-derived MALAT1 molecules enhance the proliferative, invasive, and metastatic capabilities and confer resistance to 5-FU in primary CRC cells. MALAT1 interacts with miR-26a/26b, elevating fucosyltransferase 4 (FUT4) levels and related fucosylation. This interaction further activates the PI3K/AKT/mTOR pathway, thereby mediating CRC progression and metastasis (Xu et al., 2020). The lncRNA AB073614 has shown differential expression in CRC cells and tissues from patients with CRC. Elevated AB073614 levels in CRC tissues, when compared with paired normal tissues, are closely associated with adverse clinical pathological features such as tumor grade, size, cell differentiation status, and distant metastasis. Knockdown of AB073614 in SW480 cells significantly impedes the PI3K/AKT pathway, inhibiting proliferation, migration, and invasion while promoting apoptosis and G1-phase cell cycle arrest (Wang Y. et al., 2017). The lncRNA ST3GAL6-AS1 has demonstrated inhibitory effects on CRC progression through glycosylation processes. Its expression level shows a negative correlation with key aspects such as tumor size, lymph node metastasis, distant metastasis, and tumor stage. In CRC cells, ST3GAL6-AS1 triggers the transcription of ST3GAL6, leading to an elevation in α-2,3 sialylation levels of glycoproteins. This, in turn, inhibits the activation of the PI3K/Akt signaling pathway and phosphorylation of Forkhead box1 (FOXO1). These outcomes underscore the significance of interactions involving ST3GAL6-AS1, ST3GAL6, PI3K/Akt, and FOXO1 in regulating CRC progression. Understanding these mechanisms holds promise for developing therapeutic strategies against CRC (Hu et al., 2019). Additionally, analysis of CRC specimens in the TCGA and GTEx databases revealed decreased expression of LINC02038 in CRC tissues compared with that in adjacent para-cancerous tissues. Low LINC02038 expression correlates positively with higher invasiveness, distant metastasis, higher TNM staging, and poorer OS of patients. In LoVo and SW620 cells, LINC02038 functions as a molecular sponge for miR-552-5p, thereby inhibiting the degradation of FAM172A. This inhibition suppresses the activation of the PI3K/AKT pathway. These findings strongly indicate the inhibitory roles of LINC02038 in CRC cell proliferation, vitality, migration, and invasive abilities (Liu W. et al., 2023).
Research has highlighted the significant role of LINC01268 contained in exosomes, emphasizing its pivotal involvement in tumor growth and invasion within the context of PC. Specifically, LINC01268 operates as an oncogene, orchestrating malignant processes such as cell proliferation, migration, EMT, and invasion in PC. RNA-seq analysis uncovered a substantial upsurge in LINC01268 expression in plasma samples obtained from patients with PC, a finding further corroborated through qRT-PCR analysis conducted on PC tumor tissues. Subsequent investigations unveiled that elevated LINC01268 expression leads to a reduction in miR-217 levels and an increase in KIF2A expression in PC cells AsPC-1 and PANC-1. These alterations trigger the activation of the PI3K/AKT pathway, consequently fueling tumor cell growth, proliferation, migration, invasion, and EMT progression (Liu S. et al., 2023). Moreover, analysis from the TCGA database indicates a notably high expression of the lncRNA HOXA10-AS in PC tissues. Survival analysis underscores the substantial prognostic value of HOXA10-AS for patients with PC. Further exploration has illuminated the indispensable role of HOXA10-AS in driving the malignant biological behavior of PC, largely mediated by the PI3K-AKT pathway. Molecular analysis confirms that HOXA10-AS acts as a sponge for miR-340-3p, thereby augmenting the expression of the hydroxytryptamine receptor 1D (HTR1D). This regulatory mechanism significantly affects the proliferation, migration, and differentiation of PC cells by upregulating the PI3K-AKT pathway (Wu et al., 2022). Similarly, LINC01094 exhibits significant overexpression in PC tissues based on the GEO database and correlates with unfavorable clinical features, including tumor size, lymphatic metastasis, and TNM classification. Silencing of LINC01094 in xenograft mouse models notably reduces the proliferation, migration, and metastasis of PC cells, elucidating its role in promoting tumor growth and metastasis. Functional experiments of the carcinogenic mechanism have revealed that LINC01094 serves as an endogenous sponge for miR-577, elevating the RNA-binding protein lin-28 homolog B (LIN28B) levels, thereby activating the PI3K/AKT pathway (Luo C. et al., 2021). Additionally, marked downregulation of lncRNA MEG3 has been observed in tissue samples from patients with PC. Reduced MEG3 expression aligns with more aggressive tumor characteristics such as increased size, metastasis, and vascular invasion. Cellular experiments have confirmed the suppressive role of MEG3 in PC by inhibiting the PI3K/AKT signaling pathway, resulting in impaired cell proliferation, invasive capacity, and migration (Gu et al., 2017). Furthermore, microarray analysis of six human PC tissues has identified aberrantly decreased LINC00671 expression. Patients exhibiting lower LINC00671 levels typically present adverse tumor differentiation and TNM staging, correlating with poor outcomes. Both in vitro and in vivo experiments have demonstrated the involvement of LINC00671 in inhibiting the PI3K-Akt signaling pathway, thereby restraining PC cell proliferation, invasion, and migration (Qu et al., 2021). These collective findings underscore the pivotal role of lncRNAs in regulating PC progression by modulating the activity of the PI3K pathway. This discovery provides a promising avenue for potential targeted therapies in the treatment of PC.
Whole-genome sequencing and RNA sequencing analysis of EC have identified significant mutation patterns in multiple lncRNAs and frequently abnormal PI3K/AKT pathways. These findings suggest their pivotal roles in the occurrence and development of EC (Chang et al., 2017). Further functional assays are needed to validate the interaction between lncRNAs and the PI3K/AKT pathway for precision treatment and prevention of EC in patients. Multiple studies indicate that dysregulated lncRNAs contribute to chemotherapy resistance across various types of cancer. Recent research specifically highlights LINC01014’s involvement in regulating drug resistance in EC. Overexpression of LINC01014 notably inhibits gefitinib-induced apoptosis in FLO-1 cells. Additionally, silencing of LINC01014 in gefitinib-resistant FLO-1 cell lines significantly enhances the chemosensitivity of gefitinib-resistant FLO-1 cells to gefitinib, impairing the PI3K-AKT-mTOR signaling pathway (Fu et al., 2020). Moreover, the lncRNA MCEI (lncRNA mediated the chemosensitivity of ESCC by regulating IGF2) plays a role in the tumorigenesis of EC and suppresses EC cell chemosensitivity to cisplatin. Analysis of the GSE89102 dataset identifies MCEI as significantly upregulated in EC tissues. Further assessment using the TCGA dataset reveals a strong association between MCEI expression and reduced OS rates in patients with EC. MCEI binds to miR-6759-5p, increasing IGF2 concentration, thus promoting AKT phosphorylation. These findings highlight the oncogenic functions of MCEI, mediating EC cell chemosensitivity to cisplatin through the miR-6759-5p/IGF2/PI3K/AKT axis (Liu G. et al., 2020). Furthermore, high expression of lncRNA CASC9 correlates with worse tumor differentiation, greater depth of primary tumor infiltration, lymph node metastasis, and advanced TNM staging in EC. Prognostic analysis confirms the association between high CASC9 expression and poor OS in patients with EC. Additional analysis reveals that CASC8 enhances CREB-binding protein (CBP) enrichment in the LAMC2 promoter region, upregulating LAMC2 expression in EC KYSE150 and KYSE450 cells. Subsequently, upregulated expression of LAMC2 activates focal adhesion kinase, PI3K, and AKT phosphorylation, facilitating the pro-metastatic effects mediated by CASC9 in EC progression (Liang et al., 2018). Moreover, dysregulation of the lncRNA SNHG17 closely associates with the EMT process in EC development. Upregulated expression of SNHG17 induced by TGF-β1 in EC Eca109 cells directly regulates c-Myc transcription, activating the PI3K/AKT signaling pathway and promoting cell proliferation, migration, invasion, and EMT. High SNHG17 expression correlates positively with poorer survival and unfavorable clinicopathologic features of patients with EC in the GEO dataset (GSE20347) and GEPIA (Shen et al., 2022). Additionally, high expression of lncRNA ESCCAL-1 in EC tumors is associated with poorer OS, disease-free survival (DEF), and clinicopathological indexes. Knockdown of ESCCAL-1 in EC-9706 and EC-109 cells inhibits malignant cellular processes, including proliferation, migration, invasion, and resistance to apoptosis. Mechanistic experiments suggest that ESCCAL-1 acts as a sponge for miR-590-3p, preventing the suppression of APOBEC3G expression by miR-590-3p. This action activates the PI3K/Akt signaling pathway, thereby promoting malignant characteristics induced by ESCCAL-1 in EC (Liu J. et al., 2020). These results underscore the critical role of the interaction between lncRNAs and miRNAs, along with their involvement in the PI3K/AKT pathway, in the progression of EC.
Recent studies have unveiled the dysregulation of diverse lncRNAs in gastrointestinal tumors, closely linked with clinical and pathological features as well as patient prognosis (Huang et al., 2023; Ma and Hu, 2023; Samadi et al., 2023; Xiao et al., 2023). Experimental findings underscore the pivotal regulatory roles of lncRNAs in the initiation and progression of gastrointestinal tumors, influencing vital biological behaviors such as cell proliferation, migration, invasion, and chemoresistance (Khajehdehi et al., 2021). Notably, lncRNAs often act as ceRNAs, binding with miRNAs to modulate mRNA expression of downstream target genes, subsequently affecting the PI3K/AKT pathway’s activity (Liu et al., 2017; Zhang et al., 2017; Lee et al., 2019; Qian et al., 2019; Ghafouri-Fard et al., 2021). In CRC, the ceRNA network formed by lncRNA/miRNA/mRNA interaction plays a vital role in biological processes such as EMT, metastasis, and treatment resistance (Wang et al., 2019a). The altered activity of the PI3K/AKT pathway, mediated by lncRNAs, plays a crucial role in tumor development (Duan et al., 2018; Luo et al., 2019; Duan et al., 2020; Fattahi et al., 2020; Shang et al., 2023). The intricate interplay between lncRNAs and the PI3K/AKT pathway offers crucial insights into gastrointestinal tumor progression (Moafian et al., 2021). Molecular targeted therapy has made substantial strides in cancer treatment, emphasizing the increasing importance of targeting lncRNAs or the PI3K/AKT signaling pathway for therapeutic intervention for gastrointestinal tumors (Gao et al., 2022; Mortazavi et al., 2022; Maharati and Moghbeli, 2023). However, developing drugs targeting specific lncRNAs is challenging because of their diverse structures and functions, complicating a comprehensive understanding of their precise mechanisms (Ferrè et al., 2016; Kopp and Mendell, 2018; Ali and Grote, 2020; Bridges et al., 2021; Herman et al., 2022). Additionally, the dual capacity of lncRNAs to promote and inhibit tumor growth adds complexity to their clinical application in gastrointestinal cancer therapy. Moreover, evidence suggests limited single-agent efficacy of inhibitors targeting PI3K or AKT under tolerable doses (Liu et al., 2009; Cho, 2014; Fruman and Rommel, 2014). Moreover, it remains uncertain whether available PI3K or AKT inhibitors effectively inhibit the pathway for anti-tumor effects. The lack of a qualified biomarker hampers identifying responsive patient populations and projecting clinical benefits from PI3K inhibitors (Rodon et al., 2013; Polivka and Janku, 2014; Yang et al., 2019). The association between lncRNA and PI3K signaling pathways involves multiple cellular signaling pathways. By intervening in specific lncRNA-PI3K signaling pathway interactions, it is possible to simultaneously target multiple downstream signaling pathways, which increases the difficulty of targeted drug development. Further comprehensive experimental and clinical studies are essential to grasp the molecular mechanisms and clinical significance of lncRNA-PI3K/AKT pathway interactions fully.
Identifying specific patterns of lncRNA-PI3K/AKT pathway interactions in gastrointestinal tumors not only lays a theoretical foundation for related therapeutic avenues but also aids in discovering valuable biomarkers for disease progression and prognosis. Multiple studies highlight significantly elevated levels of various lncRNAs in the plasma or tissues of patients with gastrointestinal tumors (Bhan et al., 2017). The detection of specific lncRNA levels in the plasma or tissues can serve as novel sensitive biomarkers for diagnosis. For instance, three lncRNAs (NEAT1:11, lnc-PDZD8-1:5, and LINC00910:16) exhibited significant upregulation in CRC through RNA sequencing. Combined detection of these lncRNAs in peripheral blood mononuclear cells demonstrated 74.5% sensitivity and 84.5% specificity in diagnosing CRC (Li et al., 2023). Online databases such as TCGA and GEO offer rich patient resources, facilitating the identification of prognosis-related lncRNAs that can evaluate adverse clinical characteristics and prognoses, aiding in personalized treatment strategies (Charles and Eichhorn, 2018; Wu Z. et al., 2021; Grammatikakis and Lal, 2022). Univariate and multivariate analyses have revealed the independent prognostic potential of multiple lncRNAs in predicting digestive tract cancer prognosis. For instance, lncRNA AWPPH expression levels serve as an independent prognostic factor for assessing recurrence-free and OS rates in patients with HCC (Zhao et al., 2017). Similarly, the lncRNA PTTG3P predicts the prognosis of patients with HCC independently. The upregulated expression of SNHG17 serves as an independent prognostic marker (Shen et al., 2022). In GC, expression levels of the lncRNA AK023391 independently predict the clinical outcomes of patients with GC, while the lncRNA TP53TG1 acts as a potential indicator for early diagnosis and prognosis (Huang et al., 2017; Fang D. et al., 2022). Additionally, lncRNA THAP7-AS1 expression levels can differentiate whether patients with GC experience lymph node metastasis (Liu H. T. et al., 2022). These collective studies demonstrate the significant clinical diagnostic and prognostic value of lncRNAs in gastrointestinal tumors. In light of the positive outcomes from current research, the potential therapeutic impact of interactions between lncRNA and PI3K as well as their role as diagnostic and prognostic indicators for gastrointestinal tumors is evident.
Given the non-selectivity of the PI3K-AKT pathway, several innovative strategies can be expected to improve the treatment efficacy of PI3K-AKT pathway inhibitor (Asati et al., 2016; Fattahi et al., 2020; Mortazavi et al., 2022; Khezri et al., 2024). One promising approach involves developing inhibitors that specifically target mutations or alterations in the PI3K-AKT pathway unique to tumor cells (Adil et al., 2021; Leiphrakpam and Are, 2024; Morgos et al., 2024). Utilizing gene-editing technologies such as CRISPR/Cas9 to selectively knock out components of the PI3K-AKT pathway in tumor cells while sparing normal cells offers high specificity for precise targeting of cancer cells without affecting healthy tissue (Matano et al., 2015; Faulkner et al., 2020; Bao et al., 2022; Singh et al., 2023). Similarly, nanoparticle-based delivery systems can be designed to selectively deliver PI3K-AKT pathway inhibitors to tumor cells (Sandhiutami et al., 2020; Du A. et al., 2022; Wang R. et al., 2022; Sanaei et al., 2022). Another effective strategy is combining PI3K-AKT pathway inhibitors with other treatments that are more selective for tumor cells, such as targeted therapies against tumor-specific antigens or immunotherapies (Fresno et al., 2004; Alzahrani, 2019; Fattahi et al., 2020; Yu et al., 2022). This combined approach can improve the overall specificity and efficacy of the treatment by attacking the cancer cells through multiple mechanisms. Crucial to these strategies is the identification and validation of biomarkers that predict responsiveness to PI3K-AKT pathway inhibitors (Huang T. et al., 2018; Xu et al., 2024). By stratifying patients based on the presence of these biomarkers, treatments can be personalized and applied more selectively, ensuring that patients most likely to benefit from PI3K-AKT inhibitors receive them.
Extensive studies have been conducted on various inhibitors and clinical drugs targeting the PI3K-AKT pathway as RNA based cancer gene therapy, including their applications in gastrointestinal tumors (Table 3). Both basic and clinical trials have evaluated the efficacy of these inhibitors, including PI3K inhibitor Alpelisib, AKT inhibitor Ipatasertib, and dual PI3K/mTOR inhibitor Dactolisib (Saura et al., 2017; Bang et al., 2019; Yu et al., 2020; Xu et al., 2021; Liao et al., 2023). These inhibitors represent promising treatment options for gastrointestinal tumors, either as monotherapies or in combination with other therapies. Currently, there are some clinical trials have investigated inhibitors targeting this pathway to determine their efficacy and safety in different types of cancers. For example, a Phase II trial explores the PI3K inhibitor SF1126 in treating patients with advanced or metastatic cancer (ClinicalTrials.gov IDNCT02644122).
Table 3. Key clinical trials on inhibiting of PI3K-Akt signaling pathways and RNA based cancer gene therapy.
Despite emerging research on the mechanisms between lncRNAs and the PI3K-AKT pathway, there remains a lack of effective inhibitors targeting the interaction between lncRNAs and the PI3K-AKT pathway. Given the vital role of the interaction between lncRNAs and the PI3K-AKT pathway in gastrointestinal tumors, further research is essential to fill this gap and develop viable treatment strategies that can significantly improve patient outcomes.
Gastrointestinal tumors remain a formidable challenge within public health. Numerous studies have demonstrated the critical role of the interplay between lncRNAs and the PI3K/AKT pathway to the carcinogenesis of gastrointestinal tumors. The dysregulated expression of lncRNAs, primarily through sponging specific miRNAs to regulate downstream target genes, significantly influences the activity of the PI3K/AKT pathway. This involvement plays a crucial role in the initiation and progression of gastrointestinal tumors, resulting in uncontrolled cell proliferation, invasion, reduced apoptosis, metastasis, and angiogenesis. Moreover, prior research has underscored the correlation between aberrant lncRNA expression levels and the clinical characteristics and outcomes of patients with gastrointestinal tumors, suggesting that lncRNAs have significant potential as vital diagnostic and prognostic markers.
In recent years, the burgeoning exploration of lncRNAs in gastrointestinal tumors has underscored its significance as a plausible therapeutic target. As previously reported, targeting lncRNAs and the PI3K/AKT pathway offers a promising avenue to counter chemoresistance and enhance the effectiveness of tumor treatment. This interaction presents an innovative therapeutic approach deserving of further investigation within the context of gastrointestinal tumors. Through a deeper exploration of the underlying mechanisms of lncRNAs and the PI3K/AKT pathway in these tumors, coupled with the development of targeted therapeutic interventions, we can envision substantial advancements in precise diagnosis, prognosis, and treatment methodologies for gastrointestinal tumors.
PL: Writing–original draft. XM: Writing–original draft. XG: Writing–review and editing, Writing–original draft, Project administration, Investigation, Funding acquisition, Formal Analysis, Conceptualization.
The author(s) declare that no financial support was received for the research, authorship, and/or publication of this article.
We sincerely appreciate all contributors to the references listed. Figures are created using BioRender.
The authors declare that the research was conducted in the absence of any commercial or financial relationships that could be construed as a potential conflict of interest.
All claims expressed in this article are solely those of the authors and do not necessarily represent those of their affiliated organizations, or those of the publisher, the editors and the reviewers. Any product that may be evaluated in this article, or claim that may be made by its manufacturer, is not guaranteed or endorsed by the publisher.
Adil, M. S., Khulood, D., and Somanath, P. R. (2021). Targeting Akt-associated microRNAs for cancer therapeutics. Biochem. Pharmacol. 189, 114384. doi:10.1016/j.bcp.2020.114384
Ali, T., and Grote, P. (2020). Beyond the RNA-dependent function of LncRNA genes. Elife 9, e60583. doi:10.7554/eLife.60583
Alkhoury, C., Henneman, N. F., Petrenko, V., Shibayama, Y., Segaloni, A., Gadault, A., et al. (2023). Class 3 PI3K coactivates the circadian clock to promote rhythmic de novo purine synthesis. Nat. Cell Biol. 25 (7), 975–988. doi:10.1038/s41556-023-01171-3
Almalki, W. H. (2023). Beyond the genome: lncRNAs as regulators of the PI3K/AKT pathway in lung cancer. Pathol. Res. Pract. 251, 154852. doi:10.1016/j.prp.2023.154852
Altomare, D. A., and Testa, J. R. (2005). Perturbations of the AKT signaling pathway in human cancer. Oncogene 24 (50), 7455–7464. doi:10.1038/sj.onc.1209085
Alzahrani, A. S. (2019). PI3K/Akt/mTOR inhibitors in cancer: at the bench and bedside. Semin. Cancer Biol. 59, 125–132. doi:10.1016/j.semcancer.2019.07.009
Anastasiadou, E., Faggioni, A., Trivedi, P., and Slack, F. J. (2018a). The nefarious nexus of noncoding RNAs in cancer. Int. J. Mol. Sci. 19 (7), 2072. doi:10.3390/ijms19072072
Anastasiadou, E., Jacob, L. S., and Slack, F. J. (2018b). Non-coding RNA networks in cancer. Nat. Rev. Cancer 18 (1), 5–18. doi:10.1038/nrc.2017.99
Asati, V., Mahapatra, D. K., and Bharti, S. K. (2016). PI3K/Akt/mTOR and Ras/Raf/MEK/ERK signaling pathways inhibitors as anticancer agents: structural and pharmacological perspectives. Eur. J. Med. Chem. 109, 314–341. doi:10.1016/j.ejmech.2016.01.012
Backer, J. M. (2008). The regulation and function of Class III PI3Ks: novel roles for Vps34. Biochem. J. 410 (1), 1–17. doi:10.1042/bj20071427
Bang, Y. J., Kang, Y. K., Ng, M., Chung, H. C., Wainberg, Z. A., Gendreau, S., et al. (2019). A phase II, randomised study of mFOLFOX6 with or without the Akt inhibitor ipatasertib in patients with locally advanced or metastatic gastric or gastroesophageal junction cancer. Eur. J. Cancer 108, 17–24. doi:10.1016/j.ejca.2018.11.017
Bao, Y. Y., Zhong, J. T., Shen, L. F., Dai, L. B., Zhou, S. H., Fan, J., et al. (2022). Effect of Glut-1 and HIF-1α double knockout by CRISPR/CAS9 on radiosensitivity in laryngeal carcinoma via the PI3K/Akt/mTOR pathway. J. Cell Mol. Med. 26 (10), 2881–2894. doi:10.1111/jcmm.17303
Beck, J. T., Ismail, A., and Tolomeo, C. (2014). Targeting the phosphatidylinositol 3-kinase (PI3K)/AKT/mammalian target of rapamycin (mTOR) pathway: an emerging treatment strategy for squamous cell lung carcinoma. Cancer Treat. Rev. 40 (8), 980–989. doi:10.1016/j.ctrv.2014.06.006
Bhan, A., Soleimani, M., and Mandal, S. S. (2017). Long noncoding RNA and cancer: a new paradigm. Cancer Res. 77 (15), 3965–3981. doi:10.1158/0008-5472.Can-16-2634
Bridges, M. C., Daulagala, A. C., and Kourtidis, A. (2021). LNCcation: lncRNA localization and function. J. Cell Biol. 220 (2), e202009045. doi:10.1083/jcb.202009045
Calamito, M., Juntilla, M. M., Thomas, M., Northrup, D. L., Rathmell, J., Birnbaum, M. J., et al. (2010). Akt1 and Akt2 promote peripheral B-cell maturation and survival. Blood 115 (20), 4043–4050. doi:10.1182/blood-2009-09-241638
Chan, J. J., and Tay, Y. (2018). Noncoding RNA:RNA regulatory networks in cancer. Int. J. Mol. Sci. 19 (5), 1310. doi:10.3390/ijms19051310
Chang, F., Lee, J. T., Navolanic, P. M., Steelman, L. S., Shelton, J. G., Blalock, W. L., et al. (2003). Involvement of PI3K/Akt pathway in cell cycle progression, apoptosis, and neoplastic transformation: a target for cancer chemotherapy. Leukemia 17 (3), 590–603. doi:10.1038/sj.leu.2402824
Chang, J., Tan, W., Ling, Z., Xi, R., Shao, M., Chen, M., et al. (2017). Genomic analysis of oesophageal squamous-cell carcinoma identifies alcohol drinking-related mutation signature and genomic alterations. Nat. Commun. 8, 15290. doi:10.1038/ncomms15290
Charles, R. J. L., and Eichhorn, P. J. A. (2018). Platforms for investigating LncRNA functions. SLAS Technol. 23 (6), 493–506. doi:10.1177/2472630318780639
Chaudhuri, P., Putta, P., Graham, L. M., and Rosenbaum, M. A. (2023). p85α regulatory subunit isoform controls PI3-kinase and TRPC6 membrane translocation. Cell Calcium 111, 102718. doi:10.1016/j.ceca.2023.102718
Chen, C. L., Tseng, Y. W., Wu, J. C., Chen, G. Y., Lin, K. C., Hwang, S. M., et al. (2015). Suppression of hepatocellular carcinoma by baculovirus-mediated expression of long non-coding RNA PTENP1 and MicroRNA regulation. Biomaterials 44, 71–81. doi:10.1016/j.biomaterials.2014.12.023
Chen, H., Qi, Q., Wu, N., Wang, Y., Feng, Q., Jin, R., et al. (2022). Aspirin promotes RSL3-induced ferroptosis by suppressing mTOR/SREBP-1/SCD1-mediated lipogenesis in PIK3CA-mutant colorectal cancer. Redox Biol. 55, 102426. doi:10.1016/j.redox.2022.102426
Chin, Y. R., and Toker, A. (2011). Akt isoform-specific signaling in breast cancer: uncovering an anti-migratory role for palladin. Cell Adh Migr. 5 (3), 211–214. doi:10.4161/cam.5.3.15790
Cho, D. C. (2014). Targeting the PI3K/Akt/mTOR pathway in malignancy: rationale and clinical outlook. BioDrugs 28 (4), 373–381. doi:10.1007/s40259-014-0090-5
Chong, X., Chen, J., Zheng, N., Zhou, Z., Hai, Y., Chen, S., et al. (2022). PIK3CA mutations-mediated downregulation of circLHFPL2 inhibits colorectal cancer progression via upregulating PTEN. Mol. Cancer 21 (1), 118. doi:10.1186/s12943-022-01531-x
Costa, P., Sales, S. L. A., Pinheiro, D. P., Pontes, L. Q., Maranhão, S. S., Pessoa, C., et al. (2023). Epigenetic reprogramming in cancer: from diagnosis to treatment. Front. Cell Dev. Biol. 11, 1116805. doi:10.3389/fcell.2023.1116805
Cui, W., Cai, Y., and Zhou, X. (2014). Advances in subunits of PI3K class I in cancer. Pathology 46 (3), 169–176. doi:10.1097/pat.0000000000000066
Dai, Q., Zhang, T., Pan, J., and Li, C. (2020). LncRNA UCA1 promotes cisplatin resistance in gastric cancer via recruiting EZH2 and activating PI3K/AKT pathway. J. Cancer 11 (13), 3882–3892. doi:10.7150/jca.43446
Danielsen, S. A., Eide, P. W., Nesbakken, A., Guren, T., Leithe, E., and Lothe, R. A. (2015). Portrait of the PI3K/AKT pathway in colorectal cancer. Biochim. Biophys. Acta 1855 (1), 104–121. doi:10.1016/j.bbcan.2014.09.008
Djebali, S., Davis, C. A., Merkel, A., Dobin, A., Lassmann, T., Mortazavi, A., et al. (2012). Landscape of transcription in human cells. Nature 489 (7414), 101–108. doi:10.1038/nature11233
Du, A., Li, S., Zhou, Y., Disoma, C., Liao, Y., Zhang, Y., et al. (2022a). M6A-mediated upregulation of circMDK promotes tumorigenesis and acts as a nanotherapeutic target in hepatocellular carcinoma. Mol. Cancer 21 (1), 109. doi:10.1186/s12943-022-01575-z
Du, J., Xu, Q., Zhao, H., Jia, X., Ba, N., Peng, F., et al. (2022b). PI3K inhibitor 3-MA promotes the antiproliferative activity of esomeprazole in gastric cancer cells by downregulating EGFR via the PI3K/FOXO3a pathway. Biomed. Pharmacother. 148, 112665. doi:10.1016/j.biopha.2022.112665
Duan, S., Huang, W., Liu, X., Liu, X., Chen, N., Xu, Q., et al. (2018). IMPDH2 promotes colorectal cancer progression through activation of the PI3K/AKT/mTOR and PI3K/AKT/FOXO1 signaling pathways. J. Exp. Clin. Cancer Res. 37 (1), 304. doi:10.1186/s13046-018-0980-3
Duan, Y., Haybaeck, J., and Yang, Z. (2020). Therapeutic potential of PI3K/AKT/mTOR pathway in gastrointestinal stromal tumors: rationale and progress. Cancers (Basel) 12 (10), 2972. doi:10.3390/cancers12102972
Ediriweera, M. K., Tennekoon, K. H., and Samarakoon, S. R. (2019). Role of the PI3K/AKT/mTOR signaling pathway in ovarian cancer: biological and therapeutic significance. Semin. Cancer Biol. 59, 147–160. doi:10.1016/j.semcancer.2019.05.012
Engelman, J. A., Luo, J., and Cantley, L. C. (2006). The evolution of phosphatidylinositol 3-kinases as regulators of growth and metabolism. Nat. Rev. Genet. 7 (8), 606–619. doi:10.1038/nrg1879
Fang, D., Ou, X., Sun, K., Zhou, X., Li, Y., Shi, P., et al. (2022a). m6A modification-mediated lncRNA TP53TG1 inhibits gastric cancer progression by regulating CIP2A stability. Cancer Sci. 113 (12), 4135–4150. doi:10.1111/cas.15581
Fang, L., Ford-Roshon, D., Russo, M., O'brien, C., Xiong, X., Gurjao, C., et al. (2022b). RNF43 G659fs is an oncogenic colorectal cancer mutation and sensitizes tumor cells to PI3K/mTOR inhibition. Nat. Commun. 13 (1), 3181. doi:10.1038/s41467-022-30794-7
Fattahi, S., Amjadi-Moheb, F., Tabaripour, R., Ashrafi, G. H., and Akhavan-Niaki, H. (2020). PI3K/AKT/mTOR signaling in gastric cancer: epigenetics and beyond. Life Sci. 262, 118513. doi:10.1016/j.lfs.2020.118513
Faulkner, J., Jiang, P., Farris, D., Walker, R., and Dai, Z. (2020). CRISPR/CAS9-mediated knockout of Abi1 inhibits p185(Bcr-Abl)-induced leukemogenesis and signal transduction to ERK and PI3K/Akt pathways. J. Hematol. Oncol. 13 (1), 34. doi:10.1186/s13045-020-00867-5
Ferrè, F., Colantoni, A., and Helmer-Citterich, M. (2016). Revealing protein-lncRNA interaction. Brief. Bioinform 17 (1), 106–116. doi:10.1093/bib/bbv031
Fox, M., Mott, H. R., and Owen, D. (2020). Class IA PI3K regulatory subunits: p110-independent roles and structures. Biochem. Soc. Trans. 48 (4), 1397–1417. doi:10.1042/bst20190845
Fresno, V. J. A., Casado, E., De Castro, J., Cejas, P., Belda-Iniesta, C., and González-Barón, M. (2004). PI3K/Akt signalling pathway and cancer. Cancer Treat. Rev. 30 (2), 193–204. doi:10.1016/j.ctrv.2003.07.007
Fruman, D. A., and Rommel, C. (2014). PI3K and cancer: lessons, challenges and opportunities. Nat. Rev. Drug Discov. 13 (2), 140–156. doi:10.1038/nrd4204
Fu, X., Cui, G., Liu, S., and Zhao, S. (2020). Linc01014 regulates gefitinib resistance in oesophagus cancer via EGFR-PI3K-AKT-mTOR signalling pathway. J. Cell Mol. Med. 24 (2), 1670–1675. doi:10.1111/jcmm.14860
Gao, J., Yang, S., Xie, G., Pan, J., and Zhu, F. (2022). Integrating network pharmacology and experimental verification to explore the pharmacological mechanisms of aloin against gastric cancer. Drug Des. Devel Ther. 16, 1947–1961. doi:10.2147/dddt.S360790
Ghafouri-Fard, S., Abak, A., Tondro, A. F., Shoorei, H., Majidpoor, J., and Taheri, M. (2021). The emerging role of non-coding RNAs in the regulation of PI3K/AKT pathway in the carcinogenesis process. Biomed. Pharmacother. 137, 111279. doi:10.1016/j.biopha.2021.111279
Grammatikakis, I., and Lal, A. (2022). Significance of lncRNA abundance to function. Mamm. Genome 33 (2), 271–280. doi:10.1007/s00335-021-09901-4
Gu, L., Zhang, J., Shi, M., Zhan, Q., Shen, B., and Peng, C. (2017). lncRNA MEG3 had anti-cancer effects to suppress pancreatic cancer activity. Biomed. Pharmacother. 89, 1269–1276. doi:10.1016/j.biopha.2017.02.041
Gulluni, F., De Santis, M. C., Margaria, J. P., Martini, M., and Hirsch, E. (2019). Class II PI3K functions in cell biology and disease. Trends Cell Biol. 29 (4), 339–359. doi:10.1016/j.tcb.2019.01.001
Guo, L. L., Song, C. H., Wang, P., Dai, L. P., Zhang, J. Y., and Wang, K. J. (2015). Competing endogenous RNA networks and gastric cancer. World J. Gastroenterol. 21 (41), 11680–11687. doi:10.3748/wjg.v21.i41.11680
Hart, J. R., Liu, X., Pan, C., Liang, A., Ueno, L., Xu, Y., et al. (2022). Nanobodies and chemical cross-links advance the structural and functional analysis of PI3Kα. Proc. Natl. Acad. Sci. U. S. A. 119 (38), e2210769119. doi:10.1073/pnas.2210769119
Haug, R. H., Wible, R. T., Likavec, M. J., and Conforti, P. J. (1991). Cervical spine fractures and maxillofacial trauma. J. Oral Maxillofac. Surg. 49 (7), 725–729. doi:10.1016/s0278-2391(10)80236-8
He, Y., Sun, M. M., Zhang, G. G., Yang, J., Chen, K. S., Xu, W. W., et al. (2021). Targeting PI3K/Akt signal transduction for cancer therapy. Signal Transduct. Target Ther. 6 (1), 425. doi:10.1038/s41392-021-00828-5
Herman, A. B., Tsitsipatis, D., and Gorospe, M. (2022). Integrated lncRNA function upon genomic and epigenomic regulation. Mol. Cell 82 (12), 2252–2266. doi:10.1016/j.molcel.2022.05.027
Horie, Y., Suzuki, A., Kataoka, E., Sasaki, T., Hamada, K., Sasaki, J., et al. (2004). Hepatocyte-specific Pten deficiency results in steatohepatitis and hepatocellular carcinomas. J. Clin. Invest. 113 (12), 1774–1783. doi:10.1172/jci20513
Hu, J., Shan, Y., Ma, J., Pan, Y., Zhou, H., Jiang, L., et al. (2019). LncRNA ST3Gal6-AS1/ST3Gal6 axis mediates colorectal cancer progression by regulating α-2,3 sialylation via PI3K/Akt signaling. Int. J. Cancer 145 (2), 450–460. doi:10.1002/ijc.32103
Huang, D., Sun, W., Zhou, Y., Li, P., Chen, F., Chen, H., et al. (2018a). Mutations of key driver genes in colorectal cancer progression and metastasis. Cancer Metastasis Rev. 37 (1), 173–187. doi:10.1007/s10555-017-9726-5
Huang, H., Liang, X., Wu, W., Yuan, T., Chen, Z., Wang, L., et al. (2023). FOXP3-regulated lncRNA NONHSAT136151 promotes colorectal cancer progression by disrupting QKI interaction with target mRNAs. J. Cell Mol. Med. 28, e18068. doi:10.1111/jcmm.18068
Huang, J. L., Cao, S. W., Ou, Q. S., Yang, B., Zheng, S. H., Tang, J., et al. (2018b). The long non-coding RNA PTTG3P promotes cell growth and metastasis via up-regulating PTTG1 and activating PI3K/AKT signaling in hepatocellular carcinoma. Mol. Cancer 17 (1), 93. doi:10.1186/s12943-018-0841-x
Huang, T., Wang, M., Huang, B., Chang, A., Liu, F., Zhang, Y., et al. (2018c). Long noncoding RNAs in the mTOR signaling network: biomarkers and therapeutic targets. Apoptosis 23 (5-6), 255–264. doi:10.1007/s10495-018-1453-z
Huang, Y., Xiang, B., Liu, Y., Wang, Y., and Kan, H. (2018d). LncRNA CDKN2B-AS1 promotes tumor growth and metastasis of human hepatocellular carcinoma by targeting let-7c-5p/NAP1L1 axis. Cancer Lett. 437, 56–66. doi:10.1016/j.canlet.2018.08.024
Huang, Y., Zhang, J., Hou, L., Wang, G., Liu, H., Zhang, R., et al. (2017). LncRNA AK023391 promotes tumorigenesis and invasion of gastric cancer through activation of the PI3K/Akt signaling pathway. J. Exp. Clin. Cancer Res. 36 (1), 194. doi:10.1186/s13046-017-0666-2
Jean, S., and Kiger, A. A. (2014). Classes of phosphoinositide 3-kinases at a glance. J. Cell Sci. 127 (Pt 5), 923–928. doi:10.1242/jcs.093773
Katso, R., Okkenhaug, K., Ahmadi, K., White, S., Timms, J., and Waterfield, M. D. (2001). Cellular function of phosphoinositide 3-kinases: implications for development, homeostasis, and cancer. Annu. Rev. Cell Dev. Biol. 17, 615–675. doi:10.1146/annurev.cellbio.17.1.615
Khajehdehi, M., Khalaj-Kondori, M., Ghasemi, T., Jahanghiri, B., and Damaghi, M. (2021). Long noncoding RNAs in gastrointestinal cancer: tumor suppression versus tumor promotion. Dig. Dis. Sci. 66 (2), 381–397. doi:10.1007/s10620-020-06200-x
Khezri, M. R., Hsueh, H. Y., Mohammadipanah, S., Khalili Fard, J., and Ghasemnejad-Berenji, M. (2024). The interplay between the PI3K/AKT pathway and circadian clock in physiologic and cancer-related pathologic conditions. Cell Prolif. 57 (7), e13608. doi:10.1111/cpr.13608
Kopp, F., and Mendell, J. T. (2018). Functional classification and experimental dissection of long noncoding RNAs. Cell 172 (3), 393–407. doi:10.1016/j.cell.2018.01.011
Kumar, A., Girisa, S., Alqahtani, M. S., Abbas, M., Hegde, M., Sethi, G., et al. (2023). Targeting autophagy using long non-coding RNAs (LncRNAs): new landscapes in the arena of cancer therapeutics. Cells 12 (5), 810. doi:10.3390/cells12050810
Lee, K. T., Gopalan, V., and Lam, A. K. (2019). Roles of long-non-coding RNAs in cancer therapy through the PI3K/Akt signalling pathway. Histol. Histopathol. 34 (6), 593–609. doi:10.14670/hh-18-081
Leiphrakpam, P. D., and Are, C. (2024). PI3K/Akt/mTOR signaling pathway as a target for colorectal cancer treatment. Int. J. Mol. Sci. 25 (6), 3178. doi:10.3390/ijms25063178
Li, G., Yao, Q., Liu, P., Zhang, H., Liu, Y., Li, S., et al. (2024). Critical roles and clinical perspectives of RNA methylation in cancer. MedComm (2020) 5 (5), e559. doi:10.1002/mco2.559
Li, Y., Yin, Y., He, Y., He, K., and Li, J. (2022). SOS1 regulates HCC cell epithelial-mesenchymal transition via the PI3K/AKT/mTOR pathway. Biochem. Biophys. Res. Commun. 637, 161–169. doi:10.1016/j.bbrc.2022.11.015
Li, Y., Zeng, C., Hu, J., Pan, Y., Shan, Y., Liu, B., et al. (2018). Long non-coding RNA-SNHG7 acts as a target of miR-34a to increase GALNT7 level and regulate PI3K/Akt/mTOR pathway in colorectal cancer progression. J. Hematol. Oncol. 11 (1), 89. doi:10.1186/s13045-018-0632-2
Li, Z., Wang, D., Zhang, W., Shi, H., and Zhu, M. (2023). Novel PBMC LncRNA signatures as diagnostic biomarkers for colorectal cancer. Pathol. Res. Pract. 253, 154985. doi:10.1016/j.prp.2023.154985
Liang, Y., Chen, X., Wu, Y., Li, J., Zhang, S., Wang, K., et al. (2018). LncRNA CASC9 promotes esophageal squamous cell carcinoma metastasis through upregulating LAMC2 expression by interacting with the CREB-binding protein. Cell Death Differ. 25 (11), 1980–1995. doi:10.1038/s41418-018-0084-9
Liao, W., Du, J., Li, L., Wu, X., Chen, X., Feng, Q., et al. (2023). CircZNF215 promotes tumor growth and metastasis through inactivation of the PTEN/AKT pathway in intrahepatic cholangiocarcinoma. J. Exp. Clin. Cancer Res. 42 (1), 125. doi:10.1186/s13046-023-02699-w
Liu, B., Pan, S., Xiao, Y., Liu, Q., Xu, J., and Jia, L. (2018). LINC01296/miR-26a/GALNT3 axis contributes to colorectal cancer progression by regulating O-glycosylated MUC1 via PI3K/AKT pathway. J. Exp. Clin. Cancer Res. 37 (1), 316. doi:10.1186/s13046-018-0994-x
Liu, G., Guo, W., Chen, G., Li, W., Cui, Y., Qin, J., et al. (2020a). Lnc-MCEI mediated the chemosensitivity of esophageal squamous cell carcinoma via miR-6759-5p to competitively regulate IGF2. Int. J. Biol. Sci. 16 (15), 2938–2950. doi:10.7150/ijbs.47051
Liu, H. T., Zou, Y. X., Zhu, W. J., Sen, L., Zhang, G. H., Ma, R. R., et al. (2022a). lncRNA THAP7-AS1, transcriptionally activated by SP1 and post-transcriptionally stabilized by METTL3-mediated m6A modification, exerts oncogenic properties by improving CUL4B entry into the nucleus. Cell Death Differ. 29 (3), 627–641. doi:10.1038/s41418-021-00879-9
Liu, J., Gu, X., Guan, Z., Huang, D., Xing, H., and Zheng, L. (2023a). Role of m6A modification in regulating the PI3K/AKT signaling pathway in cancer. J. Transl. Med. 21 (1), 774. doi:10.1186/s12967-023-04651-0
Liu, J., Mayekar, M. K., Wu, W., Yan, M., Guan, H., Wang, J., et al. (2020b). Long non-coding RNA ESCCAL-1 promotes esophageal squamous cell carcinoma by down regulating the negative regulator of APOBEC3G. Cancer Lett. 493, 217–227. doi:10.1016/j.canlet.2020.09.001
Liu, P., Cheng, H., Roberts, T. M., and Zhao, J. J. (2009). Targeting the phosphoinositide 3-kinase pathway in cancer. Nat. Rev. Drug Discov. 8 (8), 627–644. doi:10.1038/nrd2926
Liu, R., Chen, Y., Liu, G., Li, C., Song, Y., Cao, Z., et al. (2020c). PI3K/AKT pathway as a key link modulates the multidrug resistance of cancers. Cell Death Dis. 11 (9), 797. doi:10.1038/s41419-020-02998-6
Liu, S., Di, Y., Li, Q., Chen, L., Ma, Y., He, X., et al. (2023b). Exosomal lncRNA LINC01268 promotes pancreatic cancer progression via the miR-217-KIF2A-PI3K/AKT axis. Genes Dis. 10 (5), 1799–1801. doi:10.1016/j.gendis.2022.12.018
Liu, W., Zhang, Z., Luo, X., Qian, K., Huang, B., Liang, J., et al. (2023c). m(6)A-mediated LINC02038 inhibits colorectal cancer progression via regulation of the FAM172A/PI3K/AKT pathway via competitive binding with miR-552-5p. Int. J. Oncol. 63 (1), 81. doi:10.3892/ijo.2023.5529
Liu, W., Zheng, L., Zhang, R., Hou, P., Wang, J., Wu, L., et al. (2022b). Circ-ZEB1 promotes PIK3CA expression by silencing miR-199a-3p and affects the proliferation and apoptosis of hepatocellular carcinoma. Mol. Cancer 21 (1), 72. doi:10.1186/s12943-022-01529-5
Liu, X. X., Xiong, H. P., Huang, J. S., Qi, K., and Xu, J. J. (2017). Highly expressed long non-coding RNA CRNDE promotes cell proliferation through PI3K/AKT signalling in non-small cell lung carcinoma. Clin. Exp. Pharmacol. Physiol. 44 (8), 895–902. doi:10.1111/1440-1681.12780
Lulli, M., Napoli, C., Landini, I., Mini, E., and Lapucci, A. (2022). Role of non-coding RNAs in colorectal cancer: focus on long non-coding RNAs. Int. J. Mol. Sci. 23 (21), 13431. doi:10.3390/ijms232113431
Luo, C., Cen, S., Ding, G., and Wu, W. (2019). Mucinous colorectal adenocarcinoma: clinical pathology and treatment options. Cancer Commun. (Lond). 39 (1), 13. doi:10.1186/s40880-019-0361-0
Luo, C., Lin, K., Hu, C., Zhu, X., Zhu, J., and Zhu, Z. (2021a). LINC01094 promotes pancreatic cancer progression by sponging miR-577 to regulate LIN28B expression and the PI3K/AKT pathway. Mol. Ther. Nucleic Acids 26, 523–535. doi:10.1016/j.omtn.2021.08.024
Luo, J., Jiang, Y., Wu, L., Zhuo, D., Zhang, S., Jiang, X., et al. (2021b). Long non-coding RNA ABHD11-AS1 promotes colorectal cancer progression and invasion through targeting the integrin subunit alpha 5/focal adhesion kinase/phosphoinositide 3 kinase/Akt signaling pathway. Aging (Albany NY) 13 (16), 20179–20191. doi:10.18632/aging.203342
Luo, L. H., Jin, M., Wang, L. Q., Xu, G. J., Lin, Z. Y., Yu, D. D., et al. (2020). Long noncoding RNA TCL6 binds to miR-106a-5p to regulate hepatocellular carcinoma cells through PI3K/AKT signaling pathway. J. Cell Physiol. 235 (9), 6154–6166. doi:10.1002/jcp.29544
Lv, E., Sheng, J., Yu, C., Rao, D., and Huang, W. (2021). LncRNA influence sequential steps of hepatocellular carcinoma metastasis. Biomed. Pharmacother. 136, 111224. doi:10.1016/j.biopha.2021.111224
Ma, W., and Hu, J. (2023). The linear ANRIL transcript P14AS regulates the NF-κB signaling to promote colon cancer progression. Mol. Med. 29 (1), 162. doi:10.1186/s10020-023-00761-z
Macdougall, L. K., Domin, J., and Waterfield, M. D. (1995). A family of phosphoinositide 3-kinases in Drosophila identifies a new mediator of signal transduction. Curr. Biol. 5 (12), 1404–1415. doi:10.1016/s0960-9822(95)00278-8
Maharati, A., and Moghbeli, M. (2023). PI3K/AKT signaling pathway as a critical regulator of epithelial-mesenchymal transition in colorectal tumor cells. Cell Commun. Signal 21 (1), 201. doi:10.1186/s12964-023-01225-x
Matano, M., Date, S., Shimokawa, M., Takano, A., Fujii, M., Ohta, Y., et al. (2015). Modeling colorectal cancer using CRISPR-Cas9-mediated engineering of human intestinal organoids. Nat. Med. 21 (3), 256–262. doi:10.1038/nm.3802
Mattick, J. S., and Makunin, I. V. (2006). Non-coding RNA. Hum. Mol. Genet. 15 (Spec No 1), R17–R29. doi:10.1093/hmg/ddl046
Michl, P., and Downward, J. (2005). Mechanisms of disease: PI3K/AKT signaling in gastrointestinal cancers. Z Gastroenterol. 43 (10), 1133–1139. doi:10.1055/s-2005-858638
Miricescu, D., Totan, A., Stanescu, S., Ii, B. S. C., Stefani, C., and Greabu, M. (2020). PI3K/AKT/mTOR signaling pathway in breast cancer: from molecular landscape to clinical aspects. Int. J. Mol. Sci. 22 (1), 173. doi:10.3390/ijms22010173
Mo, H., Wang, X., Ji, G., Liang, X., Yang, Y., Sun, W., et al. (2022). The effect of SNPs in lncRNA as ceRNA on the risk and prognosis of hepatocellular carcinoma. BMC Genomics 23 (1), 769. doi:10.1186/s12864-022-09010-9
Moafian, Z., Maghrouni, A., Soltani, A., and Hashemy, S. I. (2021). Cross-talk between non-coding RNAs and PI3K/AKT/mTOR pathway in colorectal cancer. Mol. Biol. Rep. 48 (5), 4797–4811. doi:10.1007/s11033-021-06458-y
Morgos, D. T., Stefani, C., Miricescu, D., Greabu, M., Stanciu, S., Nica, S., et al. (2024). Targeting PI3K/AKT/mTOR and MAPK signaling pathways in gastric cancer. Int. J. Mol. Sci. 25 (3), 1848. doi:10.3390/ijms25031848
Mortazavi, M., Moosavi, F., Martini, M., Giovannetti, E., and Firuzi, O. (2022). Prospects of targeting PI3K/AKT/mTOR pathway in pancreatic cancer. Crit. Rev. Oncol. Hematol. 176, 103749. doi:10.1016/j.critrevonc.2022.103749
Mueller, A., Bachmann, E., Linnig, M., Khillimberger, K., Schimanski, C. C., Galle, P. R., et al. (2012). Selective PI3K inhibition by BKM120 and BEZ235 alone or in combination with chemotherapy in wild-type and mutated human gastrointestinal cancer cell lines. Cancer Chemother. Pharmacol. 69 (6), 1601–1615. doi:10.1007/s00280-012-1869-z
Nag, S., Rahaman, S., Guha, A., and Ray, P. S. (2023). An AKT1-and TRIM21-mediated phosphodegron controls proteasomal degradation of HuR enabling cell survival under heat shock. iScience 26 (4), 106307. doi:10.1016/j.isci.2023.106307
Ng, S. S. W., Tsao, M. S., Chow, S., and Hedley, D. W. (2000). Inhibition of phosphatidylinositide 3-kinase enhances gemcitabine-induced apoptosis in human pancreatic cancer cells. Cancer Res. 60 (19), 5451–5455.
Ni, Q., Zhang, H., Shi, X., and Li, X. (2023). Exosomal lncRNA HCG18 contributes to cholangiocarcinoma growth and metastasis through mediating miR-424-5p/SOX9 axis through PI3K/AKT pathway. Cancer Gene Ther. 30 (4), 582–595. doi:10.1038/s41417-022-00500-2
Obata, T., Yaffe, M. B., Leparc, G. G., Piro, E. T., Maegawa, H., Kashiwagi, A., et al. (2000). Peptide and protein library screening defines optimal substrate motifs for AKT/PKB. J. Biol. Chem. 275 (46), 36108–36115. doi:10.1074/jbc.M005497200
O'gorman, D. M., and Cotter, T. G. (2001). Molecular signals in anti-apoptotic survival pathways. Leukemia 15 (1), 21–34. doi:10.1038/sj.leu.2401998
Ohashi, Y., Tremel, S., and Williams, R. L. (2019). VPS34 complexes from a structural perspective. J. Lipid Res. 60 (2), 229–241. doi:10.1194/jlr.R089490
Okkenhaug, K. (2013). Signaling by the phosphoinositide 3-kinase family in immune cells. Annu. Rev. Immunol. 31, 675–704. doi:10.1146/annurev-immunol-032712-095946
Ozawa, T., Matsuyama, T., Toiyama, Y., Takahashi, N., Ishikawa, T., Uetake, H., et al. (2017). CCAT1 and CCAT2 long noncoding RNAs, located within the 8q.24.21 'gene desert', serve as important prognostic biomarkers in colorectal cancer. Ann. Oncol. 28 (8), 1882–1888. doi:10.1093/annonc/mdx248
Pan, S., Liu, Y., Liu, Q., Xiao, Y., Liu, B., Ren, X., et al. (2019). HOTAIR/miR-326/FUT6 axis facilitates colorectal cancer progression through regulating fucosylation of CD44 via PI3K/AKT/mTOR pathway. Biochim. Biophys. Acta Mol. Cell Res. 1866 (5), 750–760. doi:10.1016/j.bbamcr.2019.02.004
Panzitt, K., Tschernatsch, M. M., Guelly, C., Moustafa, T., Stradner, M., Strohmaier, H. M., et al. (2007). Characterization of HULC, a novel gene with striking up-regulation in hepatocellular carcinoma, as noncoding RNA. Gastroenterology 132 (1), 330–342. doi:10.1053/j.gastro.2006.08.026
Papadatos-Pastos, D., Rabbie, R., Ross, P., and Sarker, D. (2015). The role of the PI3K pathway in colorectal cancer. Crit. Rev. Oncol. Hematol. 94 (1), 18–30. doi:10.1016/j.critrevonc.2014.12.006
Peng, K., Liu, R., Yu, Y., Liang, L., Yu, S., Xu, X., et al. (2018). Identification and validation of cetuximab resistance associated long noncoding RNA biomarkers in metastatic colorectal cancer. Biomed. Pharmacother. 97, 1138–1146. doi:10.1016/j.biopha.2017.11.031
Polivka, J., and Janku, F. (2014). Molecular targets for cancer therapy in the PI3K/AKT/mTOR pathway. Pharmacol. Ther. 142 (2), 164–175. doi:10.1016/j.pharmthera.2013.12.004
Qian, X., Zhao, J., Yeung, P. Y., Zhang, Q. C., and Kwok, C. K. (2019). Revealing lncRNA structures and interactions by sequencing-based approaches. Trends Biochem. Sci. 44 (1), 33–52. doi:10.1016/j.tibs.2018.09.012
Qu, S., Niu, K., Wang, J., Dai, J., Ganguly, A., Gao, C., et al. (2021). LINC00671 suppresses cell proliferation and metastasis in pancreatic cancer by inhibiting AKT and ERK signaling pathway. Cancer Gene Ther. 28 (3-4), 221–233. doi:10.1038/s41417-020-00213-4
Rahmani, F., Ziaeemehr, A., Shahidsales, S., Gharib, M., Khazaei, M., Ferns, G. A., et al. (2020). Role of regulatory miRNAs of the PI3K/AKT/mTOR signaling in the pathogenesis of hepatocellular carcinoma. J. Cell Physiol. 235 (5), 4146–4152. doi:10.1002/jcp.29333
Reidick, C., Boutouja, F., and Platta, H. W. (2017). The class III phosphatidylinositol 3-kinase Vps34 in Saccharomyces cerevisiae. Biol. Chem. 398 (5-6), 677–685. doi:10.1515/hsz-2016-0288
Rodon, J., Dienstmann, R., Serra, V., and Tabernero, J. (2013). Development of PI3K inhibitors: lessons learned from early clinical trials. Nat. Rev. Clin. Oncol. 10 (3), 143–153. doi:10.1038/nrclinonc.2013.10
Romeo, M., Dallio, M., Scognamiglio, F., Ventriglia, L., Cipullo, M., Coppola, A., et al. (2023). Role of non-coding RNAs in hepatocellular carcinoma progression: from classic to novel clinicopathogenetic implications. Cancers (Basel) 15 (21), 5178. doi:10.3390/cancers15215178
Samadi, P., Shahnazari, M., Shekari, A., Maghool, F., and Jalali, A. (2023). A pan-cancer analysis indicates long noncoding RNA HAND2-AS1 as a potential prognostic, immunomodulatory and therapeutic biomarker in various cancers including colorectal adenocarcinoma. Cancer Cell Int. 23 (1), 307. doi:10.1186/s12935-023-03163-7
Sanaei, M. J., Baghery Saghchy Khorasani, A., Pourbagheri-Sigaroodi, A., Shahrokh, S., Zali, M. R., and Bashash, D. (2022). The PI3K/Akt/mTOR axis in colorectal cancer: oncogenic alterations, non-coding RNAs, therapeutic opportunities, and the emerging role of nanoparticles. J. Cell Physiol. 237 (3), 1720–1752. doi:10.1002/jcp.30655
Sandhiutami, N. M. D., Arozal, W., Louisa, M., Rahmat, D., and Wuyung, P. E. (2020). Curcumin nanoparticle enhances the anticancer effect of cisplatin by inhibiting PI3K/AKT and JAK/STAT3 pathway in rat ovarian carcinoma induced by DMBA. Front. Pharmacol. 11, 603235. doi:10.3389/fphar.2020.603235
Saura, C., Roda, D., Roselló, S., Oliveira, M., Macarulla, T., Pérez-Fidalgo, J. A., et al. (2017). A first-in-human phase I study of the ATP-competitive AKT inhibitor ipatasertib demonstrates robust and safe targeting of AKT in patients with solid tumors. Cancer Discov. 7 (1), 102–113. doi:10.1158/2159-8290.Cd-16-0512
Saw, P. E., Xu, X., Chen, J., and Song, E. W. (2021). Non-coding RNAs: the new central dogma of cancer biology. Sci. China Life Sci. 64 (1), 22–50. doi:10.1007/s11427-020-1700-9
Shaath, H., Vishnubalaji, R., Elango, R., Kardousha, A., Islam, Z., Qureshi, R., et al. (2022). Long non-coding RNA and RNA-binding protein interactions in cancer: experimental and machine learning approaches. Semin. Cancer Biol. 86 (Pt 3), 325–345. doi:10.1016/j.semcancer.2022.05.013
Shang, A. Q., Wang, W. W., Yang, Y. B., Gu, C. Z., Ji, P., Chen, C., et al. (2019). Knockdown of long noncoding RNA PVT1 suppresses cell proliferation and invasion of colorectal cancer via upregulation of microRNA-214-3p. Am. J. Physiol. Gastrointest. Liver Physiol. 317 (2), G222–g232. doi:10.1152/ajpgi.00357.2018
Shang, L., Wang, Y., Li, J., Zhou, F., Xiao, K., Liu, Y., et al. (2023). Mechanism of Sijunzi Decoction in the treatment of colorectal cancer based on network pharmacology and experimental validation. J. Ethnopharmacol. 302 (Pt A), 115876. doi:10.1016/j.jep.2022.115876
Shen, S., Liang, J., Liang, X., Wang, G., Feng, B., Guo, W., et al. (2022). SNHG17, as an EMT-related lncRNA, promotes the expression of c-Myc by binding to c-Jun in esophageal squamous cell carcinoma. Cancer Sci. 113 (1), 319–333. doi:10.1111/cas.15184
Singh, P., Ali, S. A., Kumar, S., and Mohanty, A. K. (2023). CRISPR-Cas9 based knockout of S100A8 in mammary epithelial cells enhances cell proliferation and triggers oncogenic transformation via the PI3K-Akt pathway: insights from a deep proteomic analysis. J. Proteomics 288, 104981. doi:10.1016/j.jprot.2023.104981
Song, M., Wang, X., Luo, Y., Liu, Z., Tan, W., Ye, P., et al. (2020). Cantharidin suppresses gastric cancer cell migration/invasion by inhibiting the PI3K/Akt signaling pathway via CCAT1. Chem. Biol. Interact. 317, 108939. doi:10.1016/j.cbi.2020.108939
Su, W., Feng, B., Hu, L., Guo, X., and Yu, M. (2022). MUC3A promotes the progression of colorectal cancer through the PI3K/Akt/mTOR pathway. BMC Cancer 22 (1), 602. doi:10.1186/s12885-022-09709-8
Sun, L., Wang, L., Chen, T., Yao, B., Wang, Y., Li, Q., et al. (2019). microRNA-1914, which is regulated by lncRNA DUXAP10, inhibits cell proliferation by targeting the GPR39-mediated PI3K/AKT/mTOR pathway in HCC. J. Cell Mol. Med. 23 (12), 8292–8304. doi:10.1111/jcmm.14705
Sun, Y., Sun, F., Jin, J., Xu, W., and Qian, H. (2023). Exosomal LncRNAs in gastrointestinal cancer: biological functions and emerging clinical applications. Cancers (Basel) 15 (3), 959. doi:10.3390/cancers15030959
Tan, Y. T., Lin, J. F., Li, T., Li, J. J., Xu, R. H., and Ju, H. Q. (2021). LncRNA-mediated posttranslational modifications and reprogramming of energy metabolism in cancer. Cancer Commun. (Lond). 41 (2), 109–120. doi:10.1002/cac2.12108
Tao, S., Hou, Y., Diao, L., Hu, Y., Xu, W., Xie, S., et al. (2023). Long noncoding RNA study: genome-wide approaches. Genes Dis. 10 (6), 2491–2510. doi:10.1016/j.gendis.2022.10.024
Tewari, D., Patni, P., Bishayee, A., Sah, A. N., and Bishayee, A. (2022). Natural products targeting the PI3K-Akt-mTOR signaling pathway in cancer: a novel therapeutic strategy. Semin. Cancer Biol. 80, 1–17. doi:10.1016/j.semcancer.2019.12.008
Thibault, B., Ramos-Delgado, F., and Guillermet-Guibert, J. (2023). Targeting class I-II-III PI3Ks in cancer therapy: recent advances in tumor biology and preclinical research. Cancers (Basel). 15 (3), 784. doi:10.3390/cancers15030784
Tsai, K. W., Lo, Y. H., Liu, H., Yeh, C. Y., Chen, Y. Z., Hsu, C. W., et al. (2018). Linc00659, a long noncoding RNA, acts as novel oncogene in regulating cancer cell growth in colorectal cancer. Mol. Cancer 17 (1), 72. doi:10.1186/s12943-018-0821-1
Vanhaesebroeck, B., Guillermet-Guibert, J., Graupera, M., and Bilanges, B. (2010). The emerging mechanisms of isoform-specific PI3K signalling. Nat. Rev. Mol. Cell Biol. 11 (5), 329–341. doi:10.1038/nrm2882
Vanhaesebroeck, B., Leevers, S. J., Panayotou, G., and Waterfield, M. D. (1997). Phosphoinositide 3-kinases: a conserved family of signal transducers. Trends Biochem. Sci. 22 (7), 267–272. doi:10.1016/s0968-0004(97)01061-x
Wang, H., Wang, Y., Lai, S., Zhao, L., Liu, W., Liu, S., et al. (2022a). LINC01468 drives NAFLD-HCC progression through CUL4A-linked degradation of SHIP2. Cell Death Discov. 8 (1), 449. doi:10.1038/s41420-022-01234-8
Wang, L., Bo, X., Yi, X., Xiao, X., Zheng, Q., Ma, L., et al. (2020a). Exosome-transferred LINC01559 promotes the progression of gastric cancer via PI3K/AKT signaling pathway. Cell Death Dis. 11 (9), 723. doi:10.1038/s41419-020-02810-5
Wang, L., Cho, K. B., Li, Y., Tao, G., Xie, Z., and Guo, B. (2019a). Long noncoding RNA (lncRNA)-Mediated competing endogenous RNA networks provide novel potential biomarkers and therapeutic targets for colorectal cancer. Int. J. Mol. Sci. 20 (22), 5758. doi:10.3390/ijms20225758
Wang, L., Duan, W., Yan, S., Xie, Y., and Wang, C. (2019b). Circulating long non-coding RNA colon cancer-associated transcript 2 protected by exosome as a potential biomarker for colorectal cancer. Biomed. Pharmacother. 113, 108758. doi:10.1016/j.biopha.2019.108758
Wang, L., Li, X., Zhang, W., Yang, Y., Meng, Q., Wang, C., et al. (2020b). miR24-2 promotes malignant progression of human liver cancer stem cells by enhancing tyrosine kinase Src epigenetically. Mol. Ther. 28 (2), 572–586. doi:10.1016/j.ymthe.2019.10.015
Wang, N., Yu, Y., Xu, B., Zhang, M., Li, Q., and Miao, L. (2019c). Pivotal prognostic and diagnostic role of the long non-coding RNA colon cancer-associated transcript 1 expression in human cancer (Review). Mol. Med. Rep. 19 (2), 771–782. doi:10.3892/mmr.2018.9721
Wang, Q., Mao, X., Luo, F., and Wang, J. (2021). LINC00511 promotes gastric cancer progression by regulating SOX4 and epigenetically repressing PTEN to activate PI3K/AKT pathway. J. Cell Mol. Med. 25 (19), 9112–9127. doi:10.1111/jcmm.16656
Wang, R., Ha, K. Y., Dhandapani, S., and Kim, Y. J. (2022b). Biologically synthesized black ginger-selenium nanoparticle induces apoptosis and autophagy of AGS gastric cancer cells by suppressing the PI3K/Akt/mTOR signaling pathway. J. Nanobiotechnology 20 (1), 441. doi:10.1186/s12951-022-01576-6
Wang, T., Sun, L., Chen, C., Zhang, Y., He, B., Zhang, Y., et al. (2023). Phosphorylation at tyrosine 317 and 508 are crucial for PIK3CA/p110α to promote CRC tumorigenesis. Cell Biosci. 13 (1), 164. doi:10.1186/s13578-023-01102-7
Wang, X., Dong, J., Jia, L., Zhao, T., Lang, M., Li, Z., et al. (2017a). HIF-2-dependent expression of stem cell factor promotes metastasis in hepatocellular carcinoma. Cancer Lett. 393, 113–124. doi:10.1016/j.canlet.2017.01.032
Wang, Y., Kuang, H., Xue, J., Liao, L., Yin, F., and Zhou, X. (2017b). LncRNA AB073614 regulates proliferation and metastasis of colorectal cancer cells via the PI3K/AKT signaling pathway. Biomed. Pharmacother. 93, 1230–1237. doi:10.1016/j.biopha.2017.07.024
Wang, Y., Lyu, Z., Qin, Y., Wang, X., Sun, L., Zhang, Y., et al. (2020c). FOXO1 promotes tumor progression by increased M2 macrophage infiltration in esophageal squamous cell carcinoma. Theranostics 10 (25), 11535–11548. doi:10.7150/thno.45261
Wei, H., Hu, J., Pu, J., Tang, Q., Li, W., Ma, R., et al. (2019). Long noncoding RNA HAGLROS promotes cell proliferation, inhibits apoptosis and enhances autophagy via regulating miR-5095/ATG12 axis in hepatocellular carcinoma cells. Int. Immunopharmacol. 73, 72–80. doi:10.1016/j.intimp.2019.04.049
Wei, S., Hu, W., Feng, J., and Geng, Y. (2022). Promotion or remission: a role of noncoding RNAs in colorectal cancer resistance to anti-EGFR therapy. Cell Commun. Signal 20 (1), 150. doi:10.1186/s12964-022-00960-x
Wu, Q., Ma, J., Wei, J., Meng, W., Wang, Y., and Shi, M. (2021a). FOXD1-AS1 regulates FOXD1 translation and promotes gastric cancer progression and chemoresistance by activating the PI3K/AKT/mTOR pathway. Mol. Oncol. 15 (1), 299–316. doi:10.1002/1878-0261.12728
Wu, W., Li, Q., Zhu, Z., Li, C., Lu, P., Zhou, X., et al. (2022). HTR1D functions as a key target of HOXA10-AS/miR-340-3p axis to promote the malignant outcome of pancreatic cancer via PI3K-AKT signaling pathway. Int. J. Biol. Sci. 18 (9), 3777–3794. doi:10.7150/ijbs.70546
Wu, Z., Lu, Z., Li, L., Ma, M., Long, F., Wu, R., et al. (2021b). Identification and validation of ferroptosis-related LncRNA signatures as a novel prognostic model for colon cancer. Front. Immunol. 12, 783362. doi:10.3389/fimmu.2021.783362
Xiao, L., Zhang, Y., Luo, Q., Guo, C., Chen, Z., and Lai, C. (2023). DHRS4-AS1 regulate gastric cancer apoptosis and cell proliferation by destabilizing DHX9 and inhibited the association between DHX9 and ILF3. Cancer Cell Int. 23 (1), 304. doi:10.1186/s12935-023-03151-x
Xiao, Z. S., Zhao, L., Zhang, X. N., Li, H. X., and Yin, Z. H. (2021). Effect of rs67085638 in long non-coding RNA (CCAT1) on colon cancer chemoresistance to paclitaxel through modulating the microRNA-24-3p and FSCN1. J. Cell Mol. Med. 25 (8), 3744–3753. doi:10.1111/jcmm.16210
Xin, X., Wu, M., Meng, Q., Wang, C., Lu, Y., Yang, Y., et al. (2018). Long noncoding RNA HULC accelerates liver cancer by inhibiting PTEN via autophagy cooperation to miR15a. Mol. Cancer 17 (1), 94. doi:10.1186/s12943-018-0843-8
Xin, Y., Li, X., Zhang, M., Shang, Z., Luo, Z., Wang, Y., et al. (2023). Fusobacterium nucleatum-induced exosomal HOTTIP promotes gastric cancer progression through the microRNA-885-3p/EphB2 axis. Cancer Sci. 114 (6), 2360–2374. doi:10.1111/cas.15781
Xu, H., Chen, K., Shang, R., Chen, X., Zhang, Y., Song, X., et al. (2021). Alpelisib combination treatment as novel targeted therapy against hepatocellular carcinoma. Cell Death Dis. 12 (10), 920. doi:10.1038/s41419-021-04206-5
Xu, J., Xiao, Y., Liu, B., Pan, S., Liu, Q., Shan, Y., et al. (2020). Exosomal MALAT1 sponges miR-26a/26b to promote the invasion and metastasis of colorectal cancer via FUT4 enhanced fucosylation and PI3K/Akt pathway. J. Exp. Clin. Cancer Res. 39 (1), 54. doi:10.1186/s13046-020-01562-6
Xu, T. P., Yu, T., Xie, M. Y., Fang, Y., Xu, T. T., Pan, Y. T., et al. (2023). LOC101929709 promotes gastric cancer progression by aiding LIN28B to stabilize c-MYC mRNA. Gastric Cancer 26 (2), 169–186. doi:10.1007/s10120-022-01348-z
Xu, Y. J., He, Y., Chen, C., Shi, J., He, M., Liu, Y., et al. (2024). Multiomics analysis revealed colorectal cancer pathogenesis. J. Proteome Res. 23 (6), 2100–2111. doi:10.1021/acs.jproteome.3c00894
Xue, C., Li, G., Lu, J., and Li, L. (2021). Crosstalk between circRNAs and the PI3K/AKT signaling pathway in cancer progression. Signal Transduct. Target Ther. 6 (1), 400. doi:10.1038/s41392-021-00788-w
Xue, C., Yao, Q., Gu, X., Shi, Q., Yuan, X., Chu, Q., et al. (2023). Evolving cognition of the JAK-STAT signaling pathway: autoimmune disorders and cancer. Signal Transduct. Target Ther. 8 (1), 204. doi:10.1038/s41392-023-01468-7
Yan, H., and Bu, P. (2021). Non-coding RNA in cancer. Essays Biochem. 65 (4), 625–639. doi:10.1042/ebc20200032
Yang, J., Nie, J., Ma, X., Wei, Y., Peng, Y., and Wei, X. (2019). Targeting PI3K in cancer: mechanisms and advances in clinical trials. Mol. Cancer 18 (1), 26. doi:10.1186/s12943-019-0954-x
Yang, P., Li, J., Peng, C., Tan, Y., Chen, R., Peng, W., et al. (2020). TCONS_00012883 promotes proliferation and metastasis via DDX3/YY1/MMP1/PI3K-AKT axis in colorectal cancer. Clin. Transl. Med. 10 (6), e211. doi:10.1002/ctm2.211
Yao, Z. T., Yang, Y. M., Sun, M. M., He, Y., Liao, L., Chen, K. S., et al. (2022). New insights into the interplay between long non-coding RNAs and RNA-binding proteins in cancer. Cancer Commun. (Lond). 42 (2), 117–140. doi:10.1002/cac2.12254
Yu, C. C., Huang, S. Y., Chang, S. F., Liao, K. F., and Chiu, S. C. (2020). The synergistic anti-cancer effects of NVP-BEZ235 and regorafenib in hepatocellular carcinoma. Molecules 25 (10), 2454. doi:10.3390/molecules25102454
Yu, L., Wei, J., and Liu, P. (2022). Attacking the PI3K/Akt/mTOR signaling pathway for targeted therapeutic treatment in human cancer. Semin. Cancer Biol. 85, 69–94. doi:10.1016/j.semcancer.2021.06.019
Zhang, D., Sun, G., Zhang, H., Tian, J., and Li, Y. (2017). Long non-coding RNA ANRIL indicates a poor prognosis of cervical cancer and promotes carcinogenesis via PI3K/Akt pathways. Biomed. Pharmacother. 85, 511–516. doi:10.1016/j.biopha.2016.11.058
Zhang, J., Roberts, T. M., and Shivdasani, R. A. (2011). Targeting PI3K signaling as a therapeutic approach for colorectal cancer. Gastroenterology 141 (1), 50–61. doi:10.1053/j.gastro.2011.05.010
Zhang, M., Jang, H., and Nussinov, R. (2020). Structural features that distinguish inactive and active PI3K lipid kinases. J. Mol. Biol. 432 (22), 5849–5859. doi:10.1016/j.jmb.2020.09.002
Zhang, W., Liang, F., Li, Q., Sun, H., Li, F., Jiao, Z., et al. (2022). LncRNA MIR205HG accelerates cell proliferation, migration and invasion in hepatoblastoma through the activation of MAPK signaling pathway and PI3K/AKT signaling pathway. Biol. Direct 17 (1), 2. doi:10.1186/s13062-021-00309-3
Zhao, C., Xie, W., Zhu, H., Zhao, M., Liu, W., Wu, Z., et al. (2022). LncRNAs and their RBPs: how to influence the fate of stem cells? Stem Cell Res. Ther. 13 (1), 175. doi:10.1186/s13287-022-02851-x
Zhao, Q., Zhao, Y., Hu, W., Zhang, Y., Wu, X., Lu, J., et al. (2020). m(6)A RNA modification modulates PI3K/Akt/mTOR signal pathway in Gastrointestinal Cancer. Theranostics 10 (21), 9528–9543. doi:10.7150/thno.42971
Zhao, X., Liu, Y., and Yu, S. (2017). Long noncoding RNA AWPPH promotes hepatocellular carcinoma progression through YBX1 and serves as a prognostic biomarker. Biochim. Biophys. Acta Mol. Basis Dis. 1863 (7), 1805–1816. doi:10.1016/j.bbadis.2017.04.014
Zheng, X., Zhang, Y., Liu, Y., Fang, L., Li, L., Sun, J., et al. (2018). HIF-2α activated lncRNA NEAT1 promotes hepatocellular carcinoma cell invasion and metastasis by affecting the epithelial-mesenchymal transition. J. Cell Biochem. 119 (4), 3247–3256. doi:10.1002/jcb.26481
Zhong, F., Liu, S., Hu, D., and Chen, L. (2022). LncRNA AC099850.3 promotes hepatocellular carcinoma proliferation and invasion through PRR11/PI3K/AKT axis and is associated with patients prognosis. J. Cancer 13 (3), 1048–1060. doi:10.7150/jca.66092
Zhou, J., Zhi, X., Wang, L., Wang, W., Li, Z., Tang, J., et al. (2015). Linc00152 promotes proliferation in gastric cancer through the EGFR-dependent pathway. J. Exp. Clin. Cancer Res. 34, 135. doi:10.1186/s13046-015-0250-6
Keywords: lncRNAs, PI3K/Akt pathway, gastrointestinal tumors, expression features, mechanisms
Citation: Li P, Ma X and Gu X (2024) The essential roles of lncRNAs/PI3K/AKT axis in gastrointestinal tumors. Front. Cell Dev. Biol. 12:1442193. doi: 10.3389/fcell.2024.1442193
Received: 07 June 2024; Accepted: 25 July 2024;
Published: 05 August 2024.
Edited by:
Stefania Pizzimenti, University of Turin, ItalyReviewed by:
Jinghui Liang, Wistar Institute, United StatesCopyright © 2024 Li, Ma and Gu. This is an open-access article distributed under the terms of the Creative Commons Attribution License (CC BY). The use, distribution or reproduction in other forums is permitted, provided the original author(s) and the copyright owner(s) are credited and that the original publication in this journal is cited, in accordance with accepted academic practice. No use, distribution or reproduction is permitted which does not comply with these terms.
*Correspondence: Xinyu Gu, aGtkZ3V4eUAxNjMuY29t
Disclaimer: All claims expressed in this article are solely those of the authors and do not necessarily represent those of their affiliated organizations, or those of the publisher, the editors and the reviewers. Any product that may be evaluated in this article or claim that may be made by its manufacturer is not guaranteed or endorsed by the publisher.
Research integrity at Frontiers
Learn more about the work of our research integrity team to safeguard the quality of each article we publish.