- Laboratory of Transcriptional Regulation, Institute of Molecular Genetics of the Czech Academy of Sciences, Prague, Czechia
The Pax6 gene is essential for eye and brain development across various animal species. Here, we investigate the function of Pax6 in the development of the anterior central nervous system (CNS) of the invertebrate chordate amphioxus using CRISPR/Cas9-induced genome editing. Specifically, we examined Pax6 mutants featuring a 6 bp deletion encompassing two invariant amino acids in the conserved paired domain, hypothesized to impair Pax6 DNA-binding capacity and gene regulatory functions. Although this mutation did not result in gross morphological changes in amphioxus larvae, it demonstrated a reduced ability to activate Pax6-responsive reporter gene, suggesting a hypomorphic effect. Expression analysis in mutant larvae revealed changes in gene expression within the anterior CNS, supporting the conserved role of Pax6 gene in brain regionalization across chordates. Additionally, our findings lend support to the hypothesis of a zona limitans intrathalamica (ZLI)-like region in amphioxus, suggesting evolutionary continuity in brain patterning mechanisms. ZLI region, found in both hemichordates and vertebrates, functions as a key signaling center and serves as a restrictive boundary between major thalamic regions.
Introduction
Pax6 is a member of the homeobox gene family, which also contains a DNA-binding paired-box motif originally identified in Drosophila (Bopp et al., 1986). The paired domains of Pax6 proteins exhibit a high degree of sequence conservation; vertebrate Pax6 proteins display nearly identical paired domains, whereas invertebrate Pax6 proteins show more than 90% sequence homology with their mouse Pax6 (Callaerts et al., 1997). Since its discovery in 1991 (Walther and Gruss, 1991), studies on Pax6 lead to the transformative thinking regarding the genetic programs orchestrating eye morphogenesis as well as the origin and evolution of diverse visual systems. The uncovering of the Pax6 gene as an essential factor in eye development within both mice (Hill et al., 1991; Grindley et al., 1995) and Drosophila (Quiring et al., 1994; Czerny et al., 1999) has given rise to the concept of a “master control gene for eye morphogenesis and evolution,” alongside the hypothesis of a monophyletic origin of eyes in metazoans (Gehring and Ikeo, 1999). This idea presented a stark contrast to the perspective originally posited by Salvini-Plawen and Mayr, which suggested a diverse, independent origin of photoreceptor organs across numerous species (von Salvini-Plawen and Mayr, 1977). The theory about a “master control gene,” has propelled a wave of scientific investigation into the expression and function of Pax6 across diverse animal species (Kozmik, 2005).
Pax6 is a typical pleiotropic transcription factor that has been implicated in diverse biological processes, and it is known to regulate expression of a broad range of molecules, including transcription factors, cell adhesion and cell signaling molecules, hormones, and structural proteins [reviewed in Simpson and Price (2002); Cvekl and Callaerts (2017)]. Pax6 function is not restricted to the visual system as it is also essential for the development of the central nervous system and endocrine glands of vertebrates and invertebrates. The expression patterns of Pax6 in the developing nervous systems of vertebrates, eyes included, show significant similarity (Carriere et al., 1993; Goulding et al., 1993; Grindley et al., 1995; Hirsch and Harris, 1997; Pan J. et al., 2023).
Heterozygous mice carrying the Small eye (Sey) Pax6 gene mutation (Hill et al., 1991), which involves a premature stop codon, display a range of eye deficits including aniridia, which is a condition also observed in humans, as well as lens size (Hogan et al., 1986; Hogan et al., 1988). These mice also exhibit abnormalities in the telencephalon, diencephalon, and metencephalon (Schmahl et al., 1993). Homozygous Sey mutants are not viable; the embryos exhibit profound brain and olfactory malformations (Grindley et al., 1995). Pax6 plays a role in establishing boundaries between regions of the central nervous system in the anteroposterior axis, at least in part, due to the regulation of homeobox-containing genes such as En1, Pax2, and Lhx1 (Mastick et al., 1997; Warren and Price, 1997; Matsunaga et al., 2000). The boundary between cortical and striatal regions of the telencephalon is dramatically altered in Sey mutants: radial glial fascicles do not form at the border, and the normal expression of R-cadherin and tenascin-C at the border is lost suggesting that Pax6 regulates boundary formation between developing forebrain regions (Stoykova et al., 1997). Paired domain is necessary for the regulation of neurogenesis, cell proliferation and patterning effects of Pax6, since these aspects are severely affected in the developing forebrain of the Pax6Aey18 mice with a deletion in the PD but intact homeodomain and transactivation domain (Haubst et al., 2004).
In Xenopus, mutations that result in truncated Pax6 proteins affect forebrain regionalization but do not completely eliminate eyes; rather, they lead to the formation of eye-like structures without lenses (Nakayama et al., 2015). It is hypothesized that an additional Pax6.2 gene may compensate for these phenotypic alterations. In medaka, mutations in the individual Pax6.1 or Pax6.2 genes do not completely eliminate eyes either (Pan K. et al., 2023; Pan J. et al., 2023). Despite the shift away from the “master control gene” concept, Pax6 central role in eye and brain development is undeniable, continuing to make it an intriguing subject for evolutionary studies.
The cephalochordate amphioxus, owing to its unique phylogenetic position as the presumed closest living relative to the common ancestor of chordates, serves as a pivotal model for exploring chordate evolution and vertebrate innovations. In amphioxus, Pax6 expression initiates during neurulation in the surface anterior ectoderm and neural plate. As neurulation progresses, strong expression becomes localized in the anterior ectoderm and the developing cerebral vesicle, the presumptive homolog of the vertebrate brain (Glardon et al., 1998). Previous studies, utilizing electron microscopy and gene expression analysis, have suggested that the brain’s anterior part corresponding to the frontal eye, may represent a potential homolog of the vertebrate paired eyes (Vopalensky et al., 2012; Pergner and Kozmik, 2017; Pergner et al., 2020). More recently, in light of new experimental evidence (Albuixech-Crespo et al., 2017) it has been proposed that the molecular signature of the frontal eye exhibits similarities to both the vertebrate retina and hypothalamus (Lacalli, 2022). Our research investigates the impact of a mutation in the most conserved region of the Pax6 gene on the anterior central nervous system of amphioxus.
Results
Targeted mutagenesis of Branchiostoma floridae Pax6 gene
To determine the functional role of Pax6 gene in amphioxus central nervous system development we analyzed mutants generated by CRISPR/Cas9 genome editing, methodology applied previously in amphioxus (Su et al., 2020). Targeting 5’end of the exon encoding the N-terminal half of paired domain produced an allele carrying a 6 bp deletion (Figure 1A) that was transmitted to F1 generation, and was designated Pax6ΔQL. Progeny of genetic crosses between Pax6ΔQL F1 (and F2) animals was genotyped to identify wild type, heterozygote, and homozygote embryos (Figure 1B). No significant morphological changes were observed in the homozygote mutant amphioxus larvae at 4 days of development (Figure 1C). The 6 bp deletion results in the elimination of the two evolutionarily conserved amino acids found in both bilaterian and cnidarian Pax proteins. Our analysis (Figure 1D) has shown that the respective QL amino acids are conserved in all nine human paralogues (PAX1-PAX9), in Drosophila Pax6, and even in the cnidarian PaxB that was previously characterized as a structural hybrid between a typical bilaterian Pax6 and Pax2 (Kozmik et al., 2003).
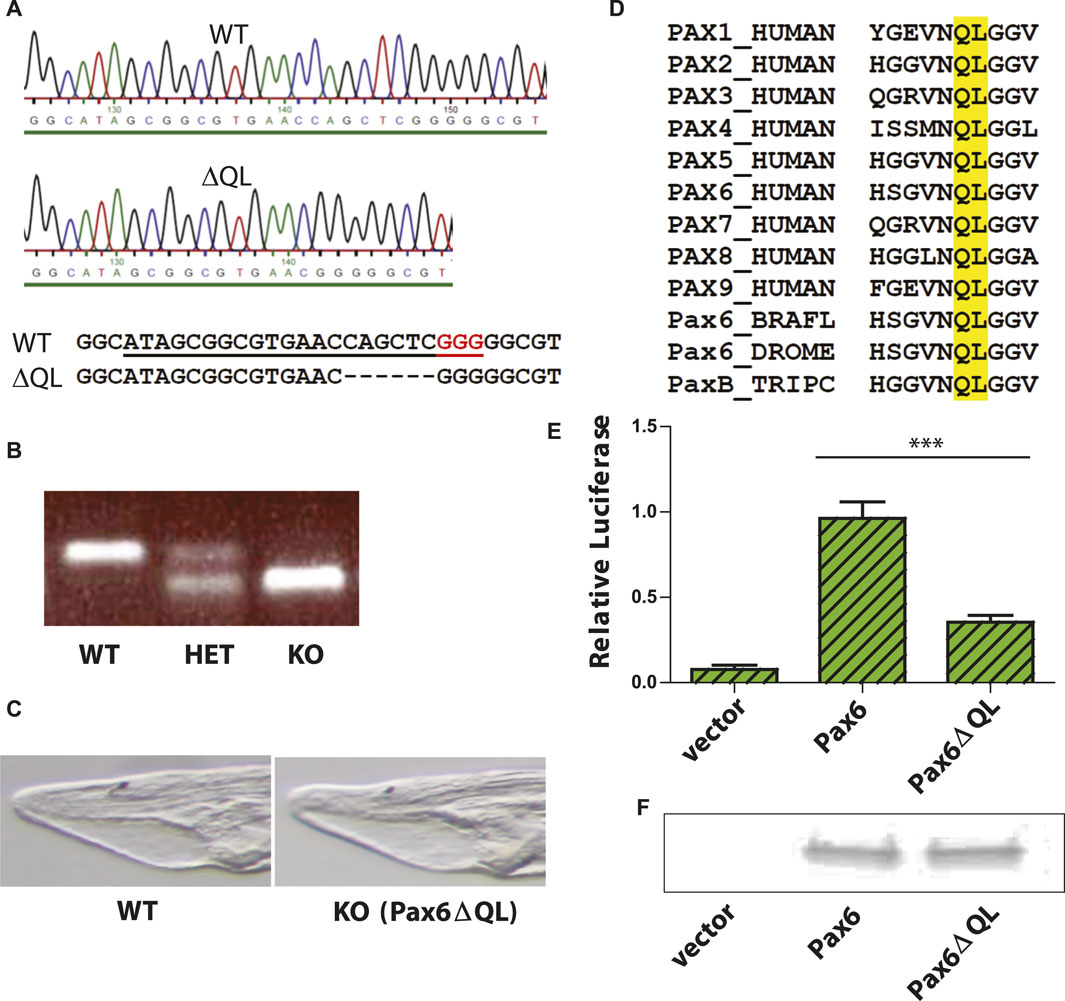
Figure 1. Targeted mutagenesis of Branchiostoma floridae Pax6 gene and characterization of the Pax6ΔQL mutant protein. (A) DNA sequencing results validating the 6 bp deletion in the Branchiostoma floridae Pax6 gene. sgRNA used for CRISPR/Cas9 genome editing is underlined and PAM sequence highlighted red. (B) A representative PCR based genotyping of amphioxus embryos carrying wild type and Pax6ΔQL alleles (C) Bright field images of the anterior part of the four-day-old wild type and KO (Pax6ΔQL homozygote mutant) larvae. (D) Amino acid sequence alignment of paired domain region for selected Pax genes. Amino acids QL deleted in mutant allele of Branchiostoma floridae Pax6 described here are highlighted by the yellow box. BRAFL, Branchiostoma floridae; DROME, Drosophila melanogaster; TRIPC, Tripedalia cystophora. (E) Reporter gene assays were performed by co-transfecting the indicated Pax6-encoding expression vector or empty expression vector with Pax6-responsive promoter construct. Triplicate assays were performed to obtain standard deviations and transfection efficiency was normalized by co-transfection of β-galactosidase expression plasmid. (F) Comparable levels of wild type Pax6 and mutant Pax6ΔQL proteins are detected in transfected cells by Western blotting.
We reasoned that elimination of two invariant amino acids of paired domain might compromise DNA binding ability of Pax6 and as a result diminish its ability to regulate target genes. This notion was further supported by the published structure of the Pax6 paired domain–DNA complex showing DNA contacts of the mutagenized amino acids with the phosphate backbone (Xu et al., 1999). To test the hypothesis we performed luciferase reporter gene assays using either wild type Pax6 or mutant Pax6ΔQL. Reporter gene assay revealed a strongly reduced capacity of the mutated protein to activate the Pax6 responsive promoter (Figure 1E). However, the observed residual activity of Pax6ΔQL as compared to the empty expression vector (Figure 1E) strongly suggests that the mutant allele generated by us here using CRISPR/Cas9 genome editing is hypomorhic. To exclude the possibility that the effect observed in reporter gene assays is not due to the reduced DNA binding of Pax6ΔQL as compared to the wild type protein but rather due to the reduced protein level we performed Western blotting following the transfection assay. As shown in Figure 1F, comparable levels of wild type Pax6 and mutant Pax6ΔQL proteins were detected in transfected cells.
Pax6 mutation affects the molecular organization and regionalization in the brain of amphioxus larvae
The results demonstrating the reduced activity of the mutated Pax6 protein encouraged us to closely examine the expression of marker genes in the region referred to as the frontal eye by Pergner et al. (2020), or as the retina and hypothalamus according to Lacalli (2022), as well as the proto-tectum and primary motor center, or dien-mesencephalon (suggested counterpart of vertebrate thalamus, pretectum, and midbrain). Up to now, it is not completely clear which concept should prevail, and for clarity, we will maintain the terminology proposed by Pergner et al. (2020).
We analyzed the expression of Six3/6, Otx, and Brn3 (Figure 2), which are found in photoreceptors of wild type larva (Figures 2A–2as3; Figures 2Bd–bd; Figures 2C–Cs1’ and 2c–cs1’). These genes showed no significant change in expression in these cells (Figure 4), indicating that the photoreceptors were likely unaffected. However, we did notice alterations in the expression of Six3/6, Brn1/2/4, Lhx3, and Pax6 in other regions of the frontal eye. Specifically, Six3/6 expression, seen at the boundary of the frontal eye with the proto-tectum in wild type larvae, was reduced in this region (Figures 2A–A’ and Figures 2a–a’; Figure 2As3 and 2as3; Figure 4). Conversely, Brn1/2/4 expression extended into the anterior frontal eye, affecting Row3, Row2, and even the photoreceptors (Figure 2D–ds2). Lhx3 expression was significantly diminished in Row4 but not in Row3 cells, and Pax6 showed reduced expression in Row4 and at the boundary of the frontal eye with the proto-tectum (Figures 3B–bs2; Figure 4). Notably, there were no marked changes in the expression of Otp and Lhx1 in Row4 (Figures 3C–cs2; Figure 3D–ds2). However, both these genes, along with Brn3, were downregulated in the proto-tectum (Figures 3C–cs2; Figures 3D–Dd, Figures 3d–dd; Figure 4). Additionally, Brn3 and Lhx1 expression was slightly elevated at the boundary of the frontal eye with the proto-tectum (Figures 2C–Cs1’ and 2c–cs1’; Figures 3D–ds2; Figure 4). Conversely, Pax6 and Otx expression was reduced in this area (Figures 3B–bs2; Figures 2B–Bs2 and 2b–bs2; Figure 4). Apart from Otp, several genes expressed in the primary motor center, including Lhx3, Brn3, and Six3/6, were downregulated (Figures 2A–as3; Figures 2C–cs2; Figures 3A–as2; Figure 4). In summary, our data suggest that the most significant changes due to the Pax6 mutation occur in the posterior frontal eye, proto-tectum, and primary motor center (Figure 5A).
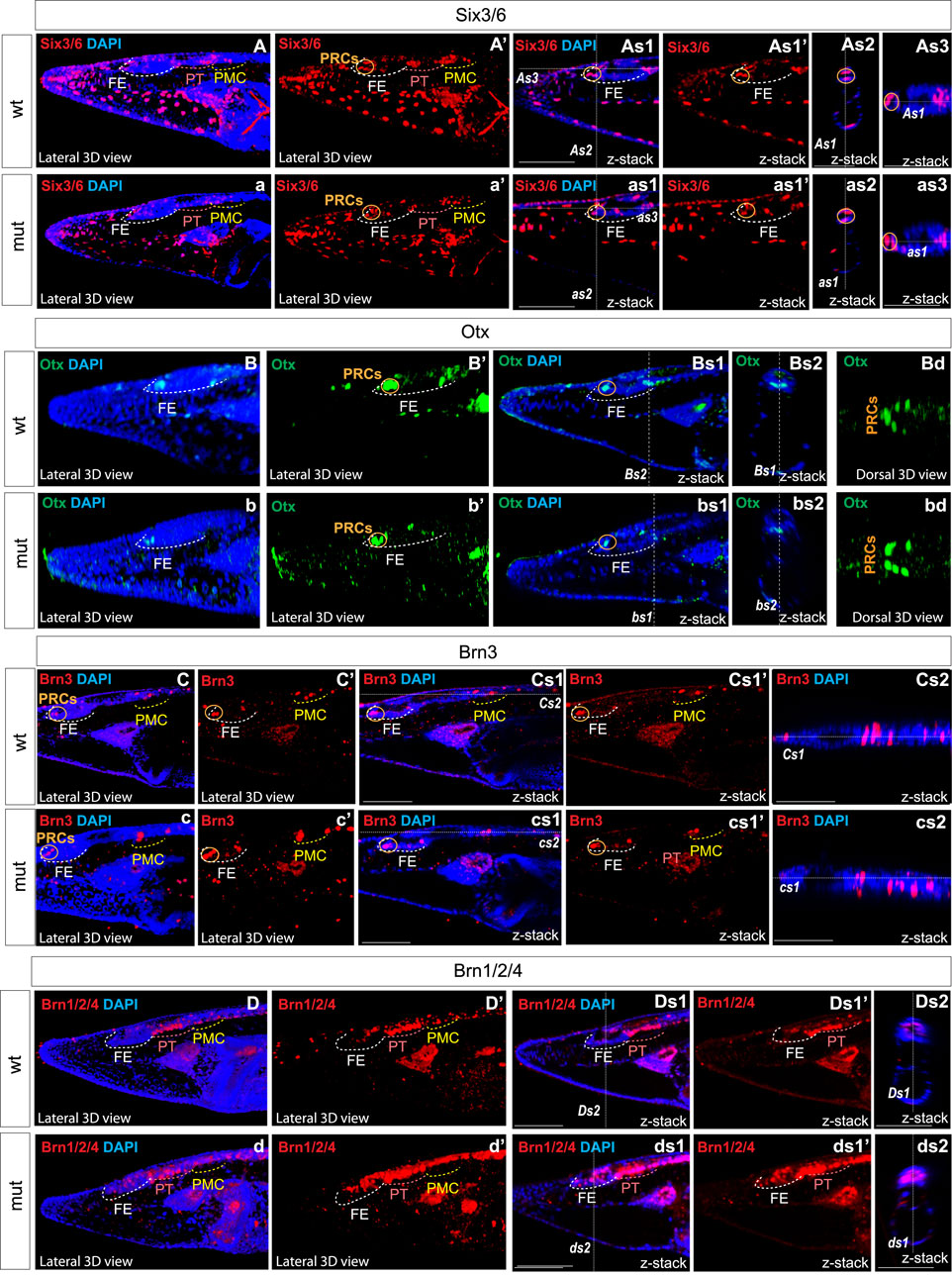
Figure 2. Expression of individual genes in amphioxus wild type (A)–As3, (B)–Bd, (C)–Cs2, (D)–Ds2 and Pax6ΔQL mutant embryos (a–as3, b–bd, c–cs2, d–ds2). Wt-wild type embryos. Mut – mutant embryos FE – frontal eye. PT – proto-tectum PMC – primary motor center. PRCs and an orange circle – photoreceptors. The positions of individual z-slices (As2, As1, As3, as1, as2, as3, Bs1, Bs2, bs1, bs2 Cs1, Cs2, cs1, cs2, Ds1, Ds2, ds1, ds2) from complete z-stacks are indicated with dashed lines (As2, As1, As3, as1, as2, as3, Bs1, Bs2, bs1, bs2 Cs1, Cs2, cs1, cs2, Ds1, Ds2, ds1, ds2).
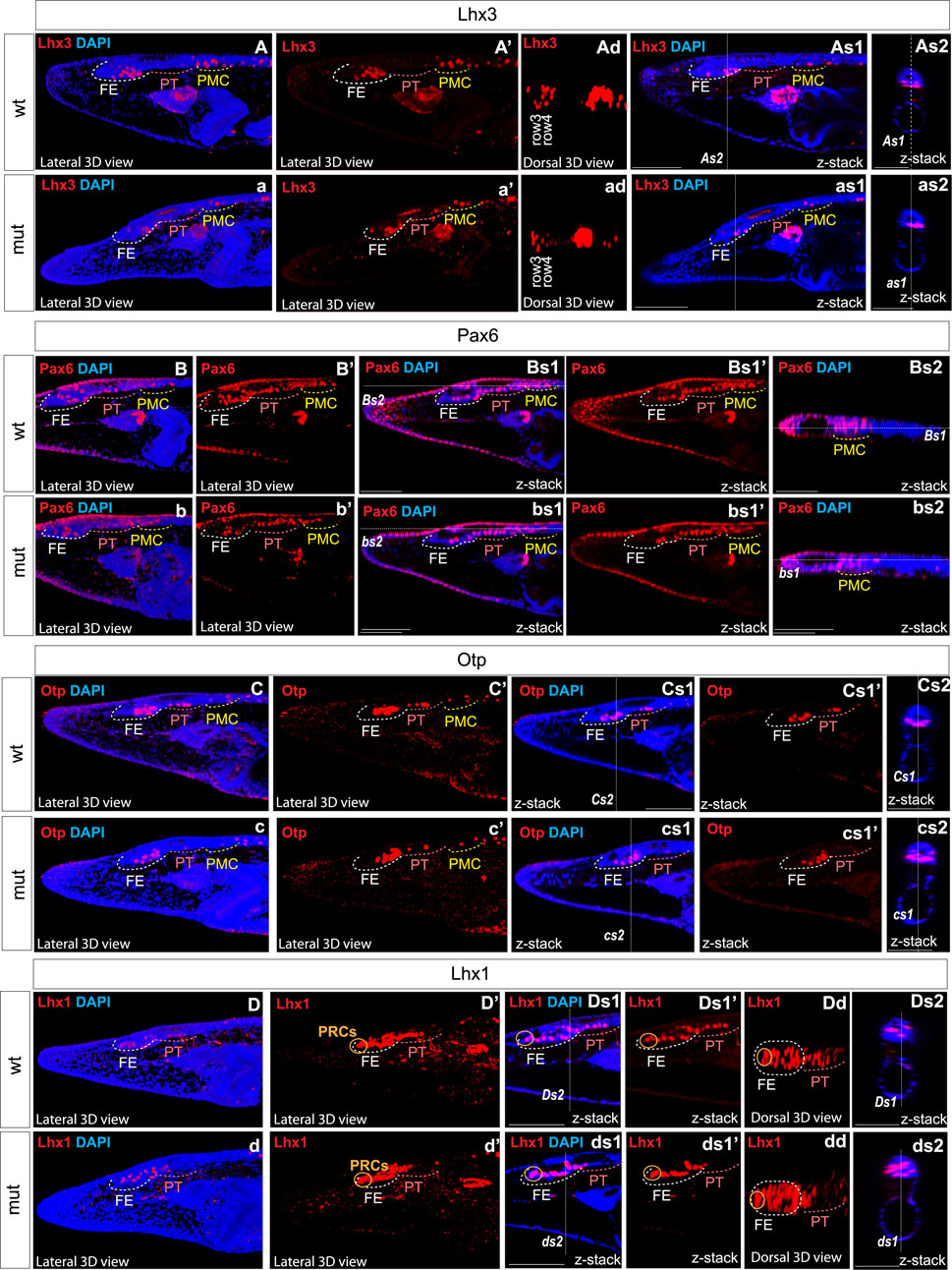
Figure 3. Expression of individual genes in amphioxus wild type (A)–As2, (B)–Bs2, (C)–Cs2, (D)–Ds2 and Pax6ΔQL mutant embryos (a–as3, b–bs2, c–cs2, d–ds2). Wt-wild type embryos. Mut – mutant embryos FE – frontal eye. PT – proto-tectum PMC – primary motor center. PRCs and an orange circle – photoreceptors. The positions of individual z-slices (As2, As1, as1, as2, Bs1, Bs2, bs1, bs2 Cs1, Cs2, cs1, cs2, Ds1, Ds2, ds1, ds2) from complete z-stacks are indicated with dashed lines (As2, As1, as1, as2, Bs1, Bs2, bs1, bs2 Cs1, Cs2, cs1, cs2, Ds1, Ds2, ds1, ds2).
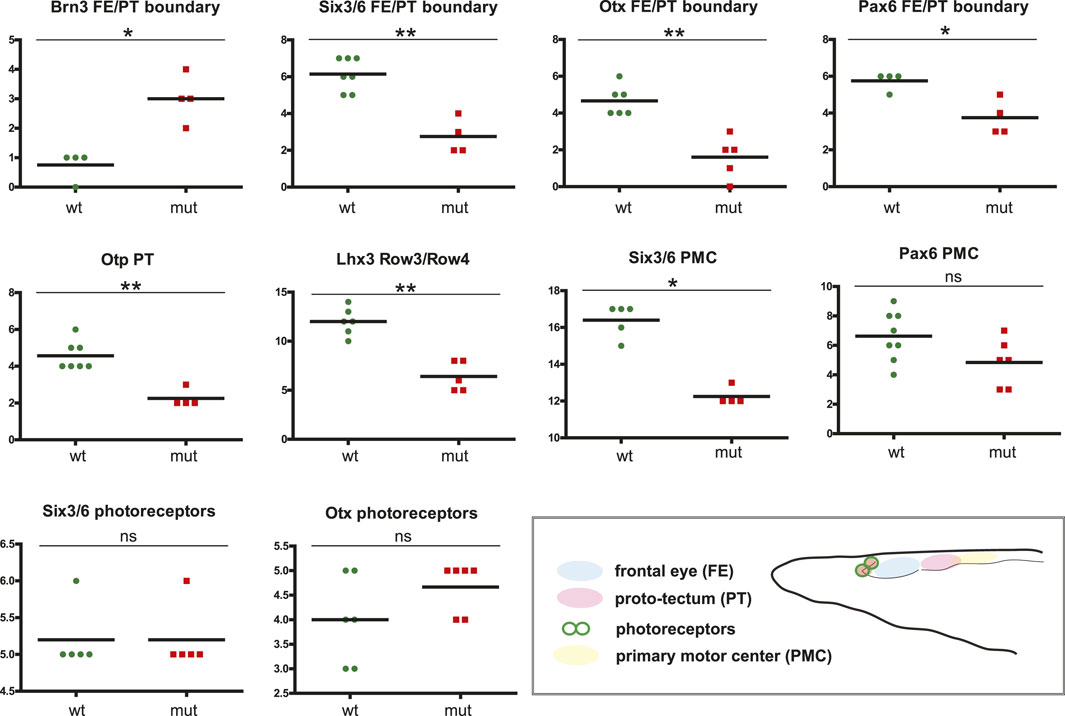
Figure 4. Difference in cell numbers between wild type and Pax6ΔQL mutant animals for the indicated regional markers. Mann-Whitney two tailed test was used for calculation of statistical significance. FE, frontal eye; PT, proto-tectum; PMC, primary motor center.
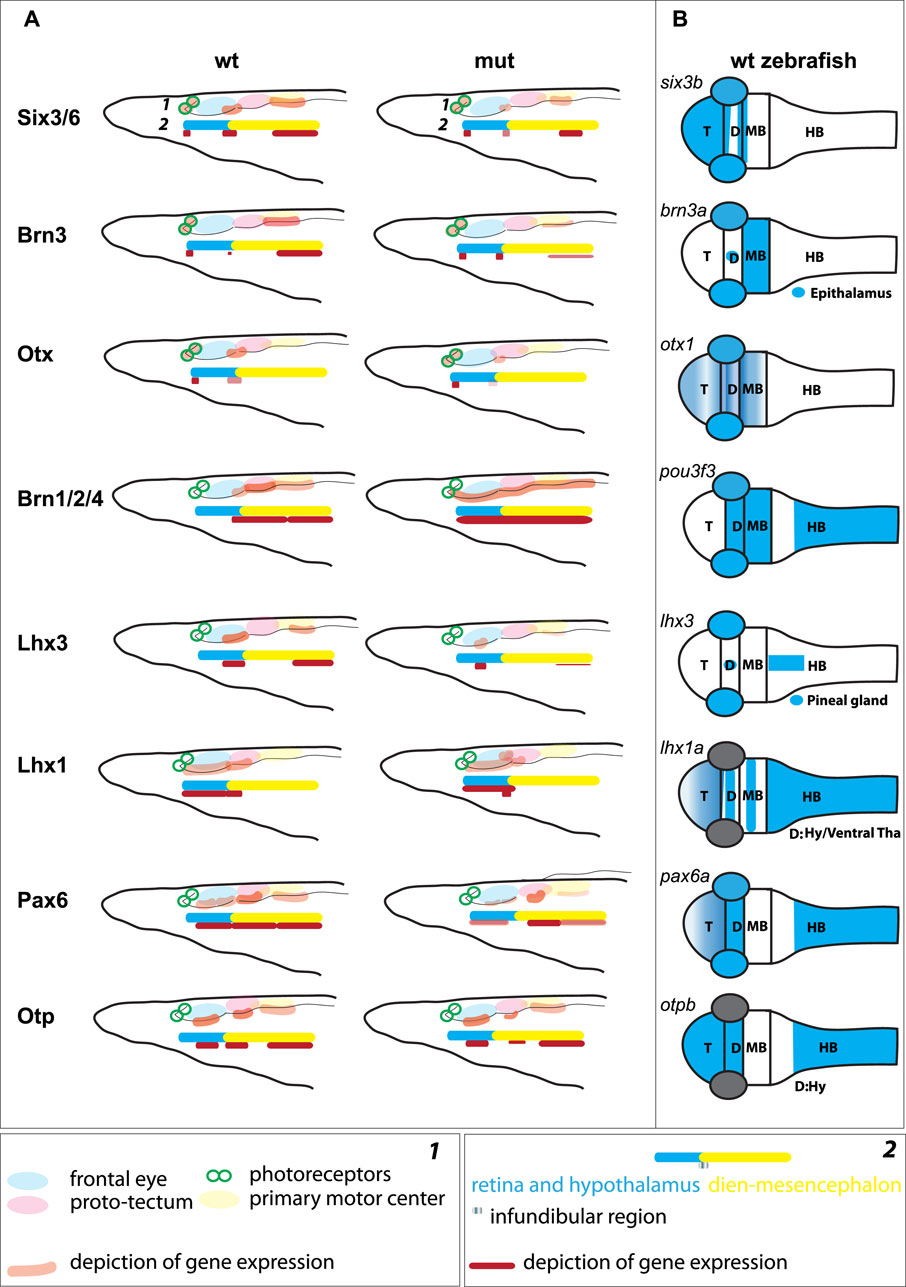
Figure 5. (A) Schematic illustration of gene expression in the anterior central nervous system of amphioxus, which is regionalized according to Pergner et al. (schematics 1) or Lacalli (schematics 2), for both wild type (wt) and Pax6ΔQL mutants (mut). (B) Schematic illustration of the orthologous gene expression (six3b (Kobayashi et al., 1998), brn3a (Halluin et al., 2016), otx1 (Scholpp et al., 2007), brn1/2/4 (pou3f3, http://zfin.org), lhx3 (Glasgow et al., 1997), lhx1a (Swanhart et al., 2010), pax6a (Scholpp et al., 2007), otpb (Del Giacco et al., 2006)) in the wild type pharyngula stage zebrafish. T – telencephalon; D –Diencephalon; MB – Midbrain; HB – Hindbrain; Hy – Hypothalamus; Tha – Thalamus.
Discussion
Genotype-phenotype correlation and hypomorphic mutations
The relationship between genotype and phenotype is generally intricate and multi-dimensional. Developmental pathways, behavioral changes, genetic networks, and gene expression patterns all contribute to the final phenotypic outcomes, often in ways that are not linear or directly correlated (Huang, 2012). Homology, a central concept in evolutionary biology, further complicates this relationship as homologous traits can arise from different genetic and developmental contexts (Tautz, 1998; Wray and Abouheif, 1998). Previous work underscores the importance of considering both genetic and epigenetic factors, as well as the potential for phenotypic plasticity and evolutionary innovation, in understanding the genotype-phenotype correlation (Wagner and Zhang, 2011). Finally, the nature of a particular mutation adds another level of complexity to a genetic study. For example, hypomorphic mutations typically produce a protein that retains some activity but is less effective than the wild-type (normal) version (Wilkie, 1994; St Johnston, 2002). This reduced functionality can lead to milder phenotypic effects compared to null mutations, which completely eliminate gene function (McLean et al., 2011). Hypomorphs are often useful in studying gene function because they can reveal the consequences of decreasing, but not entirely eliminating, the activity of a particular gene (Spradling et al., 1999).
Functional assays performed by us strongly argue that Pax6ΔQL protein maintains partial activity, and so the allele of Pax6 described here represents a hypomorphic mutation. We anticipate that the complete elimination of Pax6 gene in amphioxus would result in a more severe phenotype, especially in patterning and differentiation of the anterior neural tube. Since Pax6 is crucial for the differentiation of neural progenitor cells (Gotz and Huttner, 2005) its absence might result in a failure of these progenitor cells to properly differentiate, leading to a reduction or absence of specific neural cell types. In addition, Pax6 gene loss could lead to widespread defects in neural circuitry, affecting amphioxus sensory processing and motor functions. We found the hypomorphic Pax6 mutation to be highly informative and somewhat advantageous. In fact, it is plausible that a severe, more pleiotropic phenotype resulting from a complete loss-of-function allele of Pax6 would hinder the identification of the regional patterning defects described here.
Composite structure of the chordate brain
It has been argued that the central nervous system of chordates is intricately regionalized, characterized by a complex, gene-specific configuration of the rostral brain as defined by various studies (Holland, 2009; Vopalensky et al., 2012; Holland et al., 2013; Tosches and Arendt, 2013; Albuixech-Crespo et al., 2017; Pergner et al., 2020; Lacalli, 2022). At least two regions of the brain, the anterior and posterior, are recognized (Albuixech-Crespo et al., 2017; Holland, 2020; Lacalli, 2022). These regions are separated from each other by the junction that resembles zona limitans intrathalamica (ZLI), a feature molecularly defined in hemichordates and thought to correspond to the infundibular region located at the border between frontal eye and proto-tectum in amphioxus (Figure 5A) (Lacalli et al., 1994; Lacalli, 2022).
In four-day-old larvae of Branchiostoma floridae, we observed the presence of Six3/6 and Otx in putative photoreceptors, consistent with previous findings from two-day-old larvae of B. floridae (Vopalensky et al., 2012) and four-day-old larvae of Branchiostoma lanceolatum (Pergner et al., 2020). We did not detect Pax6 in the photoreceptors of our samples, consistent with similar observations in B. lanceolatum larvae (Pergner et al., 2020). However, this contrasts with the weak expression of Pax6 observed in photoreceptors of two-day-old larvae (Vopalensky et al., 2012), suggesting that the role for Pax6 is limited to early photoreceptor development. In amphioxus Pax6 mutants, the anterior frontal eye, including photoreceptors, appeared unaffected. No examinations of possible changes in the photoreceptors have been conducted in Xenopus and medaka Pax6 knockouts (Nakayama et al., 2015; Pan K. et al., 2023; Pan J. et al., 2023). In Xenopus Pax6 mutants, the retina is present but disorganized (Nakayama et al., 2015). In mice, retina-specific Pax6 gene ablation disrupts normal differentiation program leading to the complete absence of all mature retina neurons (Klimova and Kozmik, 2014).
A somewhat unexpected finding of our study is the conspicuous expression of Brn3 in ciliary photoreceptors, an apparent divergence from the situation in vertebrate retinas where Brn3 is typically absent from photoreceptors and is found in ganglion cells (Liu et al., 2000). A specialized subset of the vertebrate Brn3-positive retinal ganglion cells is intrinsically photosensitive due to the expression of the rhabdomeric type opsin (Opn4, melanopsin) (Berson et al., 2002; Hattar et al., 2002). The presence of Brn3 in amphioxus ciliary photoreceptors lends support to the hypothesis of a shared ancestral origin between photoreceptors and retina interneurons (Arendt, 2003; Arendt et al., 2016).
The notion of homologizing row 2, row 3, and row 4 of the amphioxus frontal eye with the interneuron organization of the vertebrate retina (Pergner et al., 2020) apparently warrants further investigation. The previously proposed homology appears less convincing due to the widespread presence of genes in these rows that are also found in other brain regions of vertebrates (Figure 5B). For example, we identified Otp expression in rows 3 and 4, the proto-tectum, and the primary motor center, a gene not typically expressed in the developing vertebrate eye but a marker of the vertebrate hypothalamus, crucial for neurosecretory cell differentiation (Wang and Lufkin, 2000; Fernandes et al., 2013). The presence of Otp expression in the frontal eye, which is believed to coincide with both the retina and hypothalamus, aligns with the brain regionalization scheme previously suggested (Albuixech-Crespo et al., 2017; Lacalli, 2022). Additionally, we discovered Pax6 expression in the proto-tectum, this contrasts with previous findings (Vopalensky et al., 2012; Pergner et al., 2020). In vertebrates, Pax6 shows weak expression in the mesencephalon only during early neurula stages, ceasing at the diencephalon-mesencephalon border in later stages (Callaerts et al., 1997; Nakayama et al., 2015; Albuixech-Crespo et al., 2017; Pan K. et al., 2023; Pan J. et al., 2023). However, the expression of Otp in the proto-tectum and primary motor center complicates the comparison of this region, called dien-mesencephalon, with the pretectum, thalamus and mesencephalon in vertebrates.
In the border region between the posterior frontal eye and the anterior proto-tectum, which likely corresponds to the infundibular region, we observed elevated expression of Otx. In zebrafish, Otx2 is expressed in the presumptive ZLI region at earlier stages and in the ZLI region at later stages, serving as one of the key factors in establishing this area (Scholpp et al., 2007). Contrary to the findings of Albuixech-Crespo et al. (2017), some researchers propose that the ZLI is present in the developing brain of amphioxus (Holland, 2020) and the infundibular region might represent this (Lacalli, 2022). Our findings lend support to this hypothesis. Otx, along with Wnt8, FoxA, Hh, and Ptch genes, is expressed in the ZLI-like region of hemichordates (Pani et al., 2012). We were interested in whether the expression of amphioxus Otx in the boundary between the posterior frontal eye and the anterior proto-tectum could be observed at earlier stages. We observed the expression of Otx and FoxA in this region at the tailbud neurula stage (Figure 6A a–f). Moreover, we detected elevated expression of Ptch specifically in this region (Figure 6A g–i). Ptch is a target gene which indicates where Hh signaling is active in amphioxus (Hu et al., 2017). These data suggest that Hh signaling operates in this region similarly to that in vertebrates and hemichordates. Our findings lend support to the hypothesis that a ZLI-like region is present in cephalochordate amphioxus (Figure 6B c).
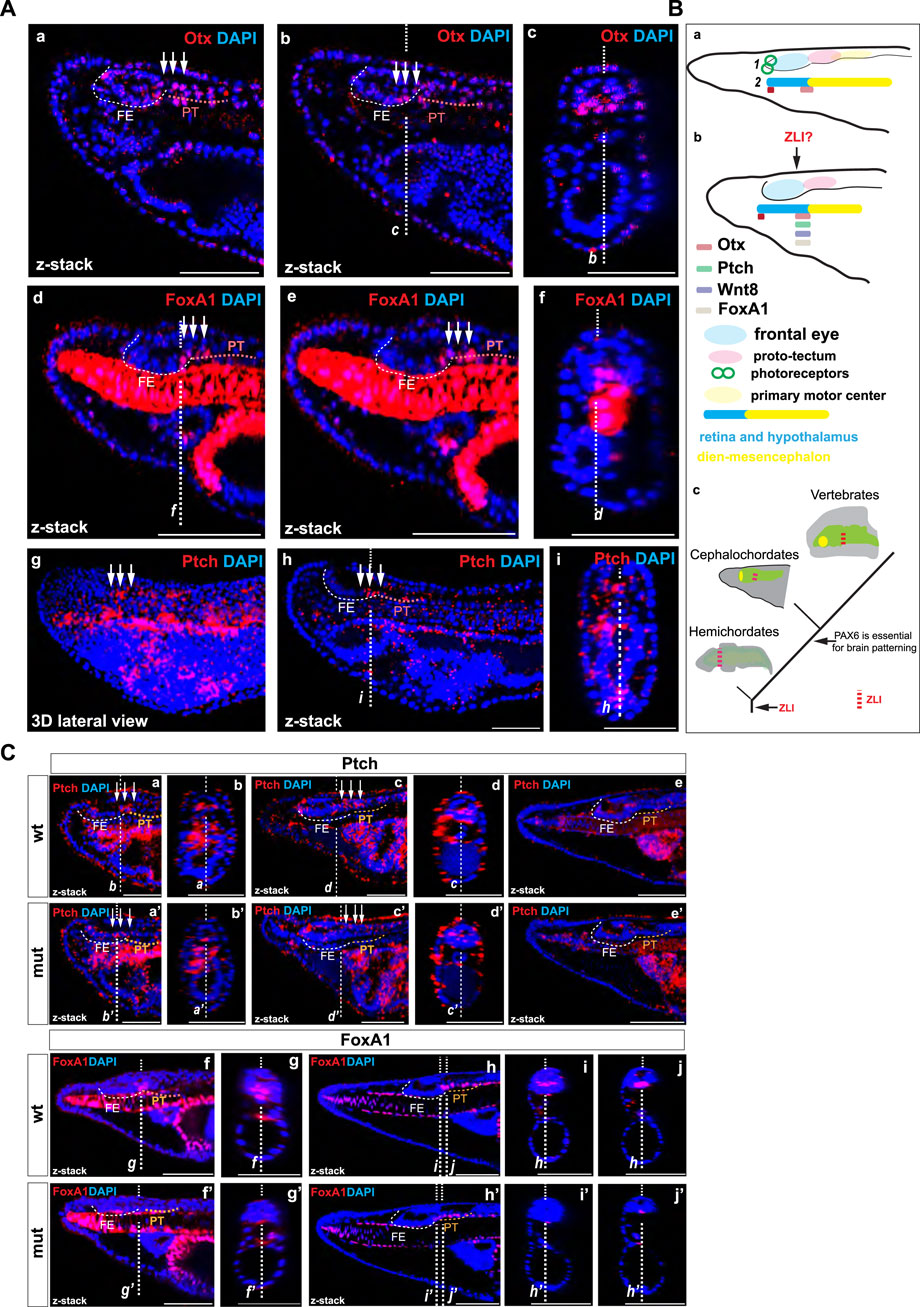
Figure 6. (A) The expression of Otx and FoxA1 proteins (a–c) and Ptch mRNA (d–e) in amphioxus tailbud neurula. The positions of individual z-slices (b–c, d, f, i–h) from complete zstacks are indicated with dashed lines (c–b, f, d, i–h). (B) Schematic illustration of gene expression in the anterior central nervous system of amphioxus, which is regionalized according to Pergner et al. (2020) (schematics 1) or Lacalli (schematics 2) for Otx at the amphioxus four-day-old larvae (a) and Otx, Ptch, FoxA, and Wnt8 (Schubert et al., 2000; Somorjai et al., 2018) at the tailbud neurula (b). Proposed scenario of brain patterning in deuterostomes (c). (C) The expression of Ptch mRNA in the wild type and Pax6ΔQL mutant animals at the T0 (a–b and a’–b’), two-day-old larva (c–d and c’–d’), and four-day-old larva (e and e’). The expression of FoxA1 protein in the wild type and Pax6ΔQL mutant animals at the two-day-old larva (c–d and c’–d’) and four-day-old larva (e and e’).
Conserved role of Pax6 in the brain regionalization
In our study, we examined amphioxus Pax6 mutants exhibiting a significant decrease in protein activity (measured as transcriptional output), apparently due to impaired binding of the paired domain to its DNA recognition site. In the case of heterozygous Pax6 mutant mice (Sey), which exhibit a noticeable phenotype (Hill et al., 1991), the concentration of functional Pax6 protein is reduced by half. Although not directly comparable, it can generally be stated that in both cases, the regulatory effect of Pax6 transcription factor on gene expression is partially impaired, though not completely abolished. The heterozygous mutation in mice leads to abnormal development of the central nervous system, affecting neuron growth and differentiation in the telencephalon, diencephalon, and metencephalon (Schmahl et al., 1993). In amphioxus mutants, we observed disorganization of gene expression patterns in various regions of the brain (Figure 5A).
In Xenopus, Pax6 mutant embryos demonstrate changes in the expression of marker genes responsible for telencephalon regionalization, and similar effects have been demonstrated in mice (van Heyningen and Williamson, 2002; Carney et al., 2009). It is suggested that Pax6 plays a crucial role in the regionalization of the telencephalon and diencephalon divisions (Grindley et al., 1997; Manuel and Price, 2005). In homozygote Pax6 mutant mouse embryos, the molecular patterning of the diencephalic regions is compromised, affecting the boundary between the mesencephalon and pretectum, and ZLI (Mastick et al., 1997; M Caballero et al., 2014). The molecular markers of the mesencephalon expand into pretectum and the identity of the pretectum is partially shifted towards that of the mesencephalon (Mastick et al., 1997). Additionally, the expression markers of the thalamus are downregulated, and genes normally confined to the ZLI are ectopically expressed in the surrounding regions (Warren and Price, 1997; Pratt et al., 2000; M Caballero et al., 2014).
In amphioxus, we observed distinct changes in the expression of individual genes in the posterior frontal eye, proto-tectum and in the primary motor center (the presumed counterparts of the vertebrate diencephalon/eyes, pretectum, and mesencephalon). Most of the examined genes that were expressed in these territories either lost their expression or were significantly downregulated, with the exception of Brn1/2/4, which expanded into the anterior region of the frontal eye. Additionally, we observed molecular disorganization in the border region between the frontal eye and the proto-tectum, which is presumed to be the ZLI-like region in amphioxus.
Interestingly, the pattern of molecular changes was different from the changes observed in the proto-tectum and primary motor center. Notably, the expression of Otx, which is required for the formation of the ZLI in zebrafish and the ZLI-like region in hemichordates, was severely diminished in the border region between the frontal eye and the proto-tectum in Pax6ΔQL mutants at the four-day-larva stage. Furthermore, Lhx1 expression expanded into the dorsal domain at the anterior border of this region, while its expression was reduced in the proto-tectum. In vertebrates, Lhx1 is expressed in the ZLI, ventral thalamus, and pretectum (Bachy et al., 2001). In Pax6 mutant mice, its expression expands into the dorsal thalamus but is reduced in the pretectum (Pratt et al., 2000). Additionally, in Pax6 mutant mice, the expression of Shh and Ptch is expanded in the regions around ZLI (M Caballero et al., 2014). In Pax6ΔQL mutants, the restricted elevated expression of Ptch disappears in the presumptive ZLI-like region at the T0 and two-day-old larva (Figure 6C a–d’). By the four-day-larva, we did not observe the expression in the border region between the frontal eye and the proto-tectum (Figure 6C e–e’). Furthermore, FoxA1, orthologous to FoxA expressed in the ZLI-like region of hemichordates (Pani et al., 2012), was severely diminished in the presumptive ZLI-like region of amphioxus Pax6ΔQL mutants at both the two-day-old and four-day-old larva stages (Figure 6C f–j’). Combined, these results further support our observation that the border between the frontal eye and the proto-tectum can be recognized as a distinct molecular entity and could thus be homologized to the vertebrate zona limitans intrathalamica (ZLI) (Holland, 2020; Lacalli, 2022).
In summary, our data suggest that the role of Pax6 gene in the brain patterning is conserved in the chordate lineage and support the hypothesis of the evolutionary continuity of the ZLI-like region in deuterostomes (Figure 6B c).
Materials and methods
Amphioxus husbandry
Amphioxus husbandry followed previously published protocols (Carvalho et al., 2017; Yong et al., 2019; Kozmikova and Kozmik, 2020). In brief, B. floridae adults were housed in seawater at a temperature of 28°C and were fed with algae daily. To induce spawning, the animals were transferred to a temperature of 18°C for at least 6 weeks before being exposed to a heat shock induced by elevating the temperature to 28°C for 24 h. Following in vitro fertilization at room temperature the embryos were raised at a temperature of 25°C.
Oligonucleotides
Oligonucleotides used for the generation of sgRNA and expression constructs, site-directed mutagenesis, and genotyping are shown in Supplementary Table S1.
Genome editing
Oligonucleotides zk1770A/B used to make sgRNA constructs were cloned into BsaI site of pDR274 (Hwang et al., 2013) (pDR274 was a gift from Keith Joung, Addgene plasmid # 42250). Cas9 mRNA was prepared using mMESSAGE mMACHINE T7 ULTRA Kit (Ambion) using plasmid pCS2-nCas9n (Jao et al., 2013) (pCS2-nCas9n was a gift from Wenbiao Chen, Addgene plasmid # 47929). The sgRNAs were transcribed using MEGAshortscript kit (Ambion). A mixture of Cas9 mRNA (100 ng/μL) and sgRNA (25 ng/μL) was injected into amphioxus eggs, eggs were fertilized, and the developing F0 embryos maintained at 25°C. The adult mature F0 animals were crossed with wild-type animals and the F1 progeny was assayed for mutations by DNA sequencing. Genetic crosses with Pax6ΔQL F1 heterozygotes were used to establish mutant line. Embryos of F2 or F3 generations were used for gene expression analysis. Amphioxus embryos were genotyped using primers zk2059/zk1989QL2/zk614 to distinguish wild type, heterozygotes, and homozygotes, respectively.
Reporter gene assays and Western blotting
Site-directed mutagenesis of B. floridae Pax6 cDNA cloned in pKW mammalian expression vector was performed by the Quick-Change kit (Stratagene) using primers zk 2027A/zk 2027B to generate Pax6ΔQL. The cell culture and transient cell transfection was performed as previously described (Klimova et al., 2015). Expression vectors encoding either wild type Pax6 or mutant Pax6ΔQL were co-transfected with Pax6-resposive reporter gene [-350GluLuc (Schwaninger et al., 1993)] and the β-galactosidase expression plasmid serving to normalize the transfection efficiency. Graph and statistical analysis of triplicate biological assays were generated in GraphPad Prism software. Western blotting was performed as previously described (Vopalensky et al., 2012).
In situ hybridization of amphioxus embryos
In situ hybridization followed the protocols described previously (Kozmikova et al., 2013). After being fixed overnight at 4°C with 4% PFA/MOPS solution [0.1M 3-(N-morpholino) propanesulfonic acid, 2 mM MgSO4, 1 mM EGTA, 0.5M NaCl, pH 7.5], the embryos were transferred to 70% ethanol with DEPC-treated water and stored at −20°C. To generate construct for Ptch in situ hybridization probe primers zk 1979C/D were used.
The color development was achieved through incubation in Vector blue solution from Vector Laboratories. Images of the embryos were captured using confocal microscopy. The embryos were mounted in glycerol on glass depression slides. Z-stack imaging was conducted using a Leica SP8 confocal microscopes, and analysed with FIJI image analysis software.
Immunohistochemistry of amphioxus embryos
Antibodies recognizing Pax6, Six3/6, Otx, Brn1/2/4, Brn3, FoxA, Lhx1, and Lhx3 were previously used (Vopalensky et al., 2012; Bozzo et al., 2017; Pergner et al., 2020). Antibody recognizing amphioxus Otp was prepared as described in Bozzo et al. (2017). To generate construct for over-expression of Otp protein fragment primers zk1361A/B were used. Embryos for immunohistochemistry were fixed and processed as described in detail before (Pergner et al., 2020). The embryos were imaged with Leica SP8 confocal microscope and processed with Fiji ImageJ analysis software. Cells positive for individual markers were counted in wild type and Pax6 mutant embryos. GraphPad Prism software was used to generate individual graphs and analyze statistical significance using Mann-Whitney two tailed test.
Data availability statement
The original contributions presented in the study are included in the article/Supplementary Material, further inquiries can be directed to the corresponding author.
Ethics statement
The manuscript presents research on animals that do not require ethical approval for their study.
Author contributions
ZK: Writing–review and editing, Conceptualization, Data curation, Formal Analysis, Funding acquisition, Investigation, Methodology, Resources, Validation, Visualization. IK: Writing–original draft, Writing–review and editing, Conceptualization, Data curation, Formal Analysis, Investigation, Methodology, Validation, Visualization.
Funding
The author(s) declare that financial support was received for the research, authorship, and/or publication of this article. This work was supported by Czechia Science Foundation grant 20-25377S awarded to ZK.
Acknowledgments
We thank Gaspar Jekely for the constructive criticism of an earlier version of the manuscript, Halyna Klymets for technical assistance, Anna Zitova and Veronika Noskova for animal husbandry, Walter Knepel for −350GluLuc, Keith Joung for pDR274 (Addgene plasmid # 42250), and Wenbiao Chen for pCS2-nCas9n (Addgene plasmid # 47929). We acknowledge the Light Microscopy Core Facility, IMG CAS, Prague, Czechia Republic, supported by MEYS (LM2018129, CZ.02.1.01/0.0/0.0/18_046/0016045) and RVO: 68378050-KAV-NPUI, for their support with the microscopy presented herein.
Conflict of interest
The authors declare that the research was conducted in the absence of any commercial or financial relationships that could be construed as a potential conflict of interest.
Publisher’s note
All claims expressed in this article are solely those of the authors and do not necessarily represent those of their affiliated organizations, or those of the publisher, the editors and the reviewers. Any product that may be evaluated in this article, or claim that may be made by its manufacturer, is not guaranteed or endorsed by the publisher.
Supplementary material
The Supplementary Material for this article can be found online at: https://www.frontiersin.org/articles/10.3389/fcell.2024.1431337/full#supplementary-material
References
Albuixech-Crespo, B., López-Blanch, L., Burguera, D., Maeso, I., Sánchez-Arrones, L., Moreno-Bravo, J. A., et al. (2017). Molecular regionalization of the developing amphioxus neural tube challenges major partitions of the vertebrate brain. PLoS Biol. 15 (4), e2001573. doi:10.1371/journal.pbio.2001573
Arendt, D. (2003). Evolution of eyes and photoreceptor cell types. Int. J. Dev. Biol. 47 (7-8), 563–571.
Arendt, D., Musser, J. M., Baker, C. V. H., Bergman, A., Cepko, C., Erwin, D. H., et al. (2016). The origin and evolution of cell types. Nat. Rev. Genet. 17 (12), 744–757. doi:10.1038/nrg.2016.127
Bachy, I., Vernier, P., and Retaux, S. (2001). The LIM-homeodomain gene family in the developing Xenopus brain: conservation and divergences with the mouse related to the evolution of the forebrain. J. Neurosci. 21 (19), 7620–7629. doi:10.1523/JNEUROSCI.21-19-07620.2001
Berson, D. M., Dunn, F. A., and Takao, M. (2002). Phototransduction by retinal ganglion cells that set the circadian clock. Science 295 (5557), 1070–1073. doi:10.1126/science.1067262
Bopp, D., Burri, M., Baumgartner, S., Frigerio, G., and Noll, M. (1986). Conservation of a large protein domain in the segmentation gene paired and in functionally related genes of Drosophila. Cell. 47 (6), 1033–1040. doi:10.1016/0092-8674(86)90818-4
Bozzo, M., Pergner, J., Kozmik, Z., and Kozmikova, I. (2017). Novel polyclonal antibodies as a useful tool for expression studies in amphioxus embryos. Int. J. Dev. Biol. 61 (10-11-12), 793–800. doi:10.1387/ijdb.170259ik
Callaerts, P., Halder, G., and Gehring, W. (1997). PAX6 in development and evolution. Annu. Rev. Neurosci. 20, 483–532. doi:10.1146/annurev.neuro.20.1.483
Carney, R. S., Cocas, L. A., Hirata, T., Mansfield, K., and Corbin, J. G. (2009). Differential regulation of telencephalic pallial-subpallial boundary patterning by Pax6 and Gsh2. Cereb. Cortex 19 (4), 745–759. doi:10.1093/cercor/bhn123
Carriere, C., Plaza, S., Martin, P., Quatannens, B., Bailly, M., Stehelin, D., et al. (1993). Characterization of quail Pax-6 (Pax-QNR) proteins expressed in the neuroretina. Mol. Cell. Biol. 13 (12), 7257–7266. doi:10.1128/mcb.13.12.7257
Carvalho, J. E., Lahaye, F., and Schubert, M. (2017). Keeping amphioxus in the laboratory: an update on available husbandry methods. Int. J. Dev. Biol. 61 (10-11-12), 773–783. doi:10.1387/ijdb.170192ms
Cvekl, A., and Callaerts, P. (2017). PAX6: 25th anniversary and more to learn. Exp. Eye Res. 156, 10–21. doi:10.1016/j.exer.2016.04.017
Czerny, T., Halder, G., Kloter, U., Souabni, A., Gehring, W. J., and Busslinger, M. (1999). Twin of eyeless, a second Pax-6 gene of Drosophila, acts upstream of eyeless in the control of eye development. Mol. Cell. 3 (3), 297–307. doi:10.1016/s1097-2765(00)80457-8
Del Giacco, L., Sordino, P., Pistocchi, A., Andreakis, N., Tarallo, R., Di Benedetto, B., et al. (2006). Differential regulation of the zebrafish orthopedia1 gene during fate determination of diencephalic neurons. BMC Dev. Biol. 6 (1), 50. doi:10.1186/1471-213X-6-50
Fernandes, A. M., Beddows, E., Filippi, A., and Driever, W. (2013). Orthopedia transcription factor otpa and otpb paralogous genes function during dopaminergic and neuroendocrine cell specification in larval zebrafish. PLoS One 8 (9), e75002. doi:10.1371/journal.pone.0075002
Gehring, W. J., and Ikeo, K. (1999). Pax 6: mastering eye morphogenesis and eye evolution. Trends Genet. 15 (9), 371–377. doi:10.1016/s0168-9525(99)01776-x
Glardon, S., Holland, L. Z., Gehring, W. J., and Holland, N. D. (1998). Isolation and developmental expression of the amphioxus Pax-6 gene (AmphiPax-6): insights into eye and photoreceptor evolution. Development 125 (14), 2701–2710. doi:10.1242/dev.125.14.2701
Glasgow, E., Karavanov, A. A., and Dawid, I. B. (1997). Neuronal and neuroendocrine expression oflim3,a LIM class homeobox gene, is altered in mutant zebrafish with axial signaling defects. Dev. Biol. 192 (2), 405–419. doi:10.1006/dbio.1997.8761
Gotz, M., and Huttner, W. B. (2005). The cell biology of neurogenesis. Nat. Rev. Mol. Cell. Biol. 6 (10), 777–788. doi:10.1038/nrm1739
Goulding, M. D., Lumsden, A., and Gruss, P. (1993). Signals from the notochord and floor plate regulate the region-specific expression of two Pax genes in the developing spinal cord. Development 117 (3), 1001–1016. doi:10.1242/dev.117.3.1001
Grindley, J. C., Davidson, D. R., and Hill, R. E. (1995). The role of Pax-6 in eye and nasal development. Development 121 (5), 1433–1442. doi:10.1242/dev.121.5.1433
Grindley, J. C., Hargett, L. K., Hill, R. E., Ross, A., and Hogan, B. L. M. (1997). Disruption of PAX6 function in mice homozygous for the Pax6Sey-1Neu mutation produces abnormalities in the early development and regionalization of the diencephalon. Mech. Dev. 64 (1), 111–126. doi:10.1016/S0925-4773(97)00055-5
Halluin, C., Madelaine, R., Naye, F., Peers, B., Roussigné, M., and Blader, P. (2016). Habenular neurogenesis in zebrafish is regulated by a hedgehog, Pax6 proneural gene cascade. PLoS One 11 (7), e0158210. doi:10.1371/journal.pone.0158210
Hattar, S., Liao, H. W., Takao, M., Berson, D. M., and Yau, K. W. (2002). Melanopsin-containing retinal ganglion cells: architecture, projections, and intrinsic photosensitivity. Science 295 (5557), 1065–1070. doi:10.1126/science.1069609
Haubst, N., Berger, J., Radjendirane, V., Graw, J., Favor, J., Saunders, G. F., et al. (2004). Molecular dissection of Pax6 function: the specific roles of the paired domain and homeodomain in brain development. Development 131 (24), 6131–6140. doi:10.1242/dev.01524
Hill, R. E., Favor, J., Hogan, B. L., Ton, C. C., Saunders, G. F., Hanson, I. M., et al. (1991). Mouse small eye results from mutations in a paired-like homeobox-containing gene. Nature 354 (6354), 522–525. doi:10.1038/354522a0
Hirsch, N., and Harris, W. A. (1997). Xenopus Pax-6 and retinal development. J. Neurobiol. 32 (1), 45–61. doi:10.1002/(sici)1097-4695(199701)32:1<45::aid-neu5>3.0.co;2-e
Hogan, B. L., Hirst, E. M., Horsburgh, G., and Hetherington, C. M. (1988). Small eye (Sey): a mouse model for the genetic analysis of craniofacial abnormalities. Development 103 (Suppl. l), 115–119. doi:10.1242/dev.103.Supplement.115
Hogan, B. L., Horsburgh, G., Cohen, J., Hetherington, C. M., Fisher, G., and Lyon, M. F. (1986). Small eyes (Sey): a homozygous lethal mutation on chromosome 2 which affects the differentiation of both lens and nasal placodes in the mouse. J. Embryol. Exp. Morphol. 97, 95–110. doi:10.1242/dev.97.1.95
Holland, L. Z. (2009). Chordate roots of the vertebrate nervous system: expanding the molecular toolkit. Nat. Rev. Neurosci. 10 (10), 736–746. doi:10.1038/nrn2703
Holland, L. Z. (2020). “"chapter 4 - invertebrate origins of vertebrate nervous systems,” in Evolutionary neuroscience. Editor J. H. Kaas (London: Academic Press), 51–73.
Holland, L. Z., Carvalho, J. E., Escriva, H., Laudet, V., Schubert, M., Shimeld, S. M., et al. (2013). Evolution of bilaterian central nervous systems: a single origin? Evodevo 4 (1), 27. doi:10.1186/2041-9139-4-27
Hu, G., Li, G., Wang, H., and Wang, Y. (2017). Hedgehog participates in the establishment of left-right asymmetry during amphioxus development by controlling Cerberus expression. Development 144 (24), 4694–4703. doi:10.1242/dev.157172
Huang, S. (2012). The molecular and mathematical basis of Waddington's epigenetic landscape: a framework for post-Darwinian biology? Bioessays 34 (2), 149–157. doi:10.1002/bies.201100031
Hwang, W. Y., Fu, Y., Reyon, D., Maeder, M. L., Tsai, S. Q., Sander, J. D., et al. (2013). Efficient genome editing in zebrafish using a CRISPR-Cas system. Nat. Biotechnol. 31 (3), 227–229. doi:10.1038/nbt.2501
Jao, L. E., Wente, S. R., and Chen, W. (2013). Efficient multiplex biallelic zebrafish genome editing using a CRISPR nuclease system. Proc. Natl. Acad. Sci. U. S. A. 110 (34), 13904–13909. doi:10.1073/pnas.1308335110
Klimova, L., Antosova, B., Kuzelova, A., Strnad, H., and Kozmik, Z. (2015). Onecut1 and Onecut2 transcription factors operate downstream of Pax6 to regulate horizontal cell development. Dev. Biol. 402 (1), 48–60. doi:10.1016/j.ydbio.2015.02.023
Klimova, L., and Kozmik, Z. (2014). Stage-dependent requirement of neuroretinal Pax6 for lens and retina development. Development 141 (6), 1292–1302. doi:10.1242/dev.098822
Kobayashi, M., Toyama, R., Takeda, H., Dawid, I. B., and Kawakami, K. (1998). Overexpression of the forebrain-specific homeobox gene six3 induces rostral forebrain enlargement in zebrafish. Development 125 (15), 2973–2982. doi:10.1242/dev.125.15.2973
Kozmik, Z. (2005). Pax genes in eye development and evolution. Curr. Opin. Genet. Dev. 15 (4), 430–438. doi:10.1016/j.gde.2005.05.001
Kozmik, Z., Daube, M., Frei, E., Norman, B., Kos, L., Dishaw, L. J., et al. (2003). Role of Pax genes in eye evolution: a cnidarian PaxB gene uniting Pax2 and Pax6 functions. Dev. Cell. 5 (5), 773–785. doi:10.1016/s1534-5807(03)00325-3
Kozmikova, I., Candiani, S., Fabian, P., Gurska, D., and Kozmik, Z. (2013). Essential role of Bmp signaling and its positive feedback loop in the early cell fate evolution of chordates. Dev. Biol. 382 (2), 538–554. doi:10.1016/j.ydbio.2013.07.021
Kozmikova, I., and Kozmik, Z. (2020). Wnt/β-catenin signaling is an evolutionarily conserved determinant of chordate dorsal organizer. Elife 9, e56817. doi:10.7554/eLife.56817
Lacalli, T. (2022). An evolutionary perspective on chordate brain organization and function: insights from amphioxus, and the problem of sentience. Philos. Trans. R. Soc. Lond B Biol. Sci. 377 (1844), 20200520. doi:10.1098/rstb.2020.0520
Lacalli, T. C., Holland, N., and West, J. (1994). Landmarks in the anterior central nervous system of amphioxus larvae. Philosophical Trans. R. Soc. Lond. Ser. B Biol. Sci. 344 (1308), 165–185. doi:10.1098/rstb.1994.0059
Liu, W., Khare, S. L., Liang, X., Peters, M. A., Liu, X., Cepko, C. L., et al. (2000). All Brn3 genes can promote retinal ganglion cell differentiation in the chick. Development 127 (15), 3237–3247. doi:10.1242/dev.127.15.3237
Manuel, M., and Price, D. J. (2005). Role of Pax6 in forebrain regionalization. Brain Res. Bull. 66 (4), 387–393. doi:10.1016/j.brainresbull.2005.02.006
Mastick, G. S., Davis, N. M., Andrews, G. L., and Easter Jr, S. S. (1997). Pax-6 functions in boundary formation and axon guidance in the embryonic mouse forebrain. Development 124 (10), 1985–1997. doi:10.1242/dev.124.10.1985
Matsunaga, E., Araki, I., and Nakamura, H. (2000). Pax6 defines the di-mesencephalic boundary by repressing En1 and Pax2. Development 127 (11), 2357–2365. doi:10.1242/dev.127.11.2357
M Caballero, I., Manuel, M., Molinek, M., Quintana-Urzainqui, I., Mi, D., Shimogori, T., et al. (2014). Cell-autonomous repression of Shh by transcription factor Pax6 regulates diencephalic patterning by controlling the central diencephalic organizer. Cell. Rep. 8, 1405–1418. doi:10.1016/j.celrep.2014.07.051
McLean, C. Y., Reno, P. L., Pollen, A. A., Bassan, A. I., Capellini, T. D., Guenther, C., et al. (2011). Human-specific loss of regulatory DNA and the evolution of human-specific traits. Nature 471 (7337), 216–219. doi:10.1038/nature09774
Nakayama, T., Fisher, M., Nakajima, K., Odeleye, A. O., Zimmerman, K. B., Fish, M. B., et al. (2015). Xenopus pax6 mutants affect eye development and other organ systems, and have phenotypic similarities to human aniridia patients. Dev. Biol. 408 (2), 328–344. doi:10.1016/j.ydbio.2015.02.012
Pan, Q., Lu, K., Luo, J., Jiang, Y., Xia, B., Chen, L., et al. (2023a). Japanese medaka Olpax6.1 mutant as a potential model for spondylo-ocular syndrome. Funct. Integr. Genomics 23 (2), 168. doi:10.1007/s10142-023-01090-4
Pan, Q., Luo, J., Jiang, Y., Wang, Z., Lu, K., and Chen, T. (2023b). Medaka (Oryzias latipes) Olpax6.2 acquires maternal inheritance and germ cells expression, but functionally degenerate in the eye. Gene 872, 147439. doi:10.1016/j.gene.2023.147439
Pani, A. M., Mullarkey, E. E., Aronowicz, J., Assimacopoulos, S., Grove, E. A., and Lowe, C. J. (2012). Ancient deuterostome origins of vertebrate brain signalling centres. Nature 483 (7389), 289–294. doi:10.1038/nature10838
Pergner, J., and Kozmik, Z. (2017). Amphioxus photoreceptors - insights into the evolution of vertebrate opsins, vision and circadian rhythmicity. Int. J. Dev. Biol. 61 (10-11-12), 665–681. doi:10.1387/ijdb.170230zk
Pergner, J., Vavrova, A., Kozmikova, I., and Kozmik, Z. (2020). Molecular fingerprint of amphioxus frontal eye illuminates the evolution of homologous cell types in the chordate retina. Front. Cell. Dev. Biol. 8, 705. doi:10.3389/fcell.2020.00705
Pratt, T., Vitalis, T., Warren, N., Edgar, J. M., Mason, J. O., and Price, D. J. (2000). A role for Pax6 in the normal development of dorsal thalamus and its cortical connections. Development 127 (23), 5167–5178. doi:10.1242/dev.127.23.5167
Quiring, R., Walldorf, U., Kloter, U., and Gehring, W. J. (1994). Homology of the eyeless gene of Drosophila to the Small eye gene in mice and Aniridia in humans. Science 265 (5173), 785–789. doi:10.1126/science.7914031
Schmahl, W., Knoedlseder, M., Favor, J., and Davidson, D. (1993). Defects of neuronal migration and the pathogenesis of cortical malformations are associated with Small eye (Sey) in the mouse, a point mutation at the Pax-6-locus. Acta Neuropathol. 86 (2), 126–135. doi:10.1007/bf00334879
Scholpp, S., Foucher, I., Staudt, N., Peukert, D., Lumsden, A., and Houart, C. (2007). Otx1l, Otx2 and Irx1b establish and position the ZLI in the diencephalon. Development 134 (17), 3167–3176. doi:10.1242/dev.001461
Schubert, M., Holland, L. Z., Panopoulou, G. D., Lehrach, H., and Holland, N. D. (2000). Characterization of amphioxus AmphiWnt8: insights into the evolution of patterning of the embryonic dorsoventral axis. Evol. Dev. 2 (2), 85–92. doi:10.1046/j.1525-142x.2000.00047.x
Schwaninger, M., Blume, R., Oetjen, E., Lux, G., and Knepel, W. (1993). Inhibition of cAMP-responsive element-mediated gene transcription by cyclosporin A and FK506 after membrane depolarization. J. Biol. Chem. 268 (31), 23111–23115. doi:10.1016/s0021-9258(19)49433-7
Simpson, T. I., and Price, D. J. (2002). Pax6; a pleiotropic player in development. Bioessays 24 (11), 1041–1051. doi:10.1002/bies.10174
Somorjai, I. M. L., Marti-Solans, J., Diaz-Gracia, M., Nishida, H., Imai, K. S., Escriva, H., et al. (2018). Wnt evolution and function shuffling in liberal and conservative chordate genomes. Genome Biol. 19 (1), 98. doi:10.1186/s13059-018-1468-3
Spradling, A. C., Stern, D., Beaton, A., Rhem, E. J., Laverty, T., Mozden, N., et al. (1999). The Berkeley Drosophila Genome Project gene disruption project: single P-element insertions mutating 25% of vital Drosophila genes. Genetics 153 (1), 135–177. doi:10.1093/genetics/153.1.135
St Johnston, D. (2002). The art and design of genetic screens: Drosophila melanogaster. Nat. Rev. Genet. 3 (3), 176–188. doi:10.1038/nrg751
Stoykova, A., Gotz, M., Gruss, P., and Price, J. (1997). Pax6-dependent regulation of adhesive patterning, R-cadherin expression and boundary formation in developing forebrain. Development 124 (19), 3765–3777. doi:10.1242/dev.124.19.3765
Su, L., Shi, C., Huang, X., Wang, Y., and Li, G. (2020). Application of CRISPR/Cas9 nuclease in amphioxus genome editing. Genes. (Basel) 11 (11), 1311. doi:10.3390/genes11111311
Swanhart, L., Takahashi, N., Jackson, R., Gibson, G., Watkins, S., Dawid, I., et al. (2010). Characterization of an lhx1a transgenic reporter in zebrafish. Int. J. Dev. Biol. 54 (4), 731–736. doi:10.1387/ijdb.092969ls
Tautz, D. (1998). Evolutionary biology. Debatable homologies. Nature 395 (6697), 17, 19–19. doi:10.1038/25604
Tosches, M. A., and Arendt, D. (2013). The bilaterian forebrain: an evolutionary chimaera. Curr. Opin. Neurobiol. 23 (6), 1080–1089. doi:10.1016/j.conb.2013.09.005
van Heyningen, V., and Williamson, K. A. (2002). PAX6 in sensory development. Hum. Mol. Genet. 11 (10), 1161–1167. doi:10.1093/hmg/11.10.1161
von Salvini-Plawen, L., and Mayr, E. (1977). On the evolution of photorecptors and eyes. USA: Plenum Press.
Vopalensky, P., Pergner, J., Liegertova, M., Benito-Gutierrez, E., Arendt, D., and Kozmik, Z. (2012). Molecular analysis of the amphioxus frontal eye unravels the evolutionary origin of the retina and pigment cells of the vertebrate eye. Proc. Natl. Acad. Sci. U. S. A. 109 (38), 15383–15388. doi:10.1073/pnas.1207580109
Wagner, G. P., and Zhang, J. (2011). The pleiotropic structure of the genotype-phenotype map: the evolvability of complex organisms. Nat. Rev. Genet. 12 (3), 204–213. doi:10.1038/nrg2949
Walther, C., and Gruss, P. (1991). Pax-6, a murine paired box gene, is expressed in the developing CNS. Development 113 (4), 1435–1449. doi:10.1242/dev.113.4.1435
Wang, W., and Lufkin, T. (2000). The murine Otp homeobox gene plays an essential role in the specification of neuronal cell lineages in the developing hypothalamus. Dev. Biol. 227 (2), 432–449. doi:10.1006/dbio.2000.9902
Warren, N., and Price, D. J. (1997). Roles of Pax-6 in murine diencephalic development. Development 124 (8), 1573–1582. doi:10.1242/dev.124.8.1573
Wilkie, A. O. (1994). The molecular basis of genetic dominance. J. Med. Genet. 31 (2), 89–98. doi:10.1136/jmg.31.2.89
Wray, G. A., and Abouheif, E. (1998). When is homology not homology? Curr. Opin. Genet. Dev. 8 (6), 675–680. doi:10.1016/s0959-437x(98)80036-1
Xu, H. E., Rould, M. A., Xu, W., Epstein, J. A., Maas, R. L., and Pabo, C. O. (1999). Crystal structure of the human Pax6 paired domain-DNA complex reveals specific roles for the linker region and carboxy-terminal subdomain in DNA binding. Genes. Dev. 13 (10), 1263–1275. doi:10.1101/gad.13.10.1263
Keywords: evolution, brain, eye, amphioxus, chordates, genome editing, pax6
Citation: Kozmik Z and Kozmikova I (2024) Ancestral role of Pax6 in chordate brain regionalization. Front. Cell Dev. Biol. 12:1431337. doi: 10.3389/fcell.2024.1431337
Received: 11 May 2024; Accepted: 10 July 2024;
Published: 25 July 2024.
Edited by:
Michael Schubert, UMR7009 Laboratoire de Biologie du Développement de Villefranche sur Mer, FranceReviewed by:
Guang Li, Xiamen University, ChinaJian Sun, University of Delaware, United States
David McClay, Duke University, United States
Nicholas Holland, University of California, San Diego, United States
Copyright © 2024 Kozmik and Kozmikova. This is an open-access article distributed under the terms of the Creative Commons Attribution License (CC BY). The use, distribution or reproduction in other forums is permitted, provided the original author(s) and the copyright owner(s) are credited and that the original publication in this journal is cited, in accordance with accepted academic practice. No use, distribution or reproduction is permitted which does not comply with these terms.
*Correspondence: Iryna Kozmikova, kozmikova@img.cas.cz