- 1Biomedical Research, Novartis Pharma AG, Basel, Switzerland
- 2Department of Anesthesia, Critical Care & Pain Medicine, Beth Israel Deaconess Medical Center and Harvard Medical School, Boston, MA, United States
- 3Department of Immunology, University Medical Center Hamburg-Eppendorf, Hamburg, Germany
- 4Department of Life Science and Biotechnology, University of Ferrara, Ferrara, Italy
Extracellular ATP and its derivates mediate a signaling pathway that might be pharmacologically targeted to treat inflammatory conditions. Extracellular adenosine, the product of ATP hydrolysis by ectonucleotidase enzymes, plays a key role in halting inflammation while promoting immune tolerance. The rate-limiting ectoenzyme ENTPD1/CD39 and the ecto-5′-nucleotidase/CD73 are the prototype members of the ectonucleotidase family, being responsible for ATP degradation into immunosuppressive adenosine. The biological effects of adenosine are mediated via adenosine receptors, a family of G protein-coupled receptors largely expressed on immune cells where they modulate innate and adaptive immune responses. Inflammatory bowel disease (IBD) is a serious inflammatory condition of the gastrointestinal tract, associated with substantial morbidity and often refractory to currently available medications. IBD is linked to altered interactions between the gut microbiota and the immune system in genetically predisposed individuals. A wealth of studies conducted in patients and animal models highlighted the role of various adenosine receptors in the modulation of chronic inflammatory diseases like IBD. In this review, we will discuss the most recent findings on adenosine-mediated immune responses in different cell types, with a focus on IBD and its most common manifestations, Crohn’s disease and ulcerative colitis.
Introduction
Purinergic and adenosine signaling modulate innate and adaptive immune responses virtually in all tissues. Extracellular ATP is released as result of damage or pathological conditions, and rapidly accumulates in inflamed tissues where it is hydrolyzed into adenosine by different enzymes including ectonucleotidases, ectonucleotide pyrophosphatase/phosphodiesterase, alkaline phosphatase and adenylate kinase (Yegutkin and Boison, 2022). Prototype members of the ectonucleotidase family are ENTPD1/CD39, responsible for hydrolyzing ATP and ADP into AMP, and the ecto-5′-nucleotidase/CD73, which converts AMP into adenosine (Allard et al., 2017; Savio et al., 2020). Both ectonucleotidases along with adenosine limit inflammation and restore physiological homeostasis after inflammation-related injury. Additional cell membrane-expressed ectonucleotidases include NTPDase 3 and 8 that preferentially hydrolyze ATP and NTPDase 2 that hydrolyzes ATP only (Kukulski et al., 2005). Cell membrane-expressed ENTPDase 1, 2, 3 and 8 are also present in circulating microparticles in human plasma (Jiang et al., 2014). In the context of inflammatory bowel disease (IBD), a recent study showed that NTPDase8, the dominant enzyme responsible for nucleotide hydrolysis in the gut lumen, protects the intestine from inflammation (Salem et al., 2022). Extracellular nucleosides modulate cellular responses by entering target cells via nucleoside transport mechanisms, based on active transmembrane sodium gradient or facilitated-diffusion carriers (Thorn and Jarvis, 1996). The biological effects of adenosine are mediated also through adenosine receptors (AR), a family of G protein-coupled heptahelical transmembrane receptors, classified into four types: A1R, A2AR, A2BR, and A3R (Hasko et al., 2008). Adenosine receptors are largely expressed on immune cells and have been involved in the modulation of inflammatory conditions, including IBD (Thiel et al., 2003; Sitkovsky et al., 2008; Cronstein and Sitkovsky, 2017).
Ulcerative Colitis (UC) and Crohn’s disease (CD) are the most common manifestations of IBD, the incidence of which has increased significantly over the last decades (Ng et al., 2017). IBD has a relapsing/remitting course and often becomes refractory to current therapies. The condition likely results from altered interactions between the gut microbiota and the immune system and is characterized by an imbalance between effector and regulatory immune responses. Mounting evidence has pointed to a pivotal role of adenosine signaling in the modulation of innate and adaptive immunity, proposing it as a therapeutic target in chronic inflammation like in IBD (Vuerich et al., 2020).
Innate immune responses
Macrophages
Adenosine immunoregulatory effects on macrophages are supported by clinical and experimental evidence. In patients with ankylosing spondylitis, human M2-like macrophages display dysfunctional A2AR that fails to control MMP8 expression, this being associated with disease activity (Sadatpour et al., 2022). In a murine macrophage cell line, exposure to extracellular adenosine, decreases LPS-induced expression of the NADase/ADP-ribosyl-cyclase CD38, as well as the immunomodulatory molecule CD83, while boosting M2 markers and Th2 suppressive cytokines (Devi et al., 2023). In mouse peritoneal macrophages, adenosine suppresses LPS-induced IL-1β release, ROS and nitrite production (Ryzhov et al., 2008), while stimulation of A2BR boosts IL-10 production (Nemeth et al., 2005) and contributes to suppression of TNF-α release (Kreckler et al., 2006). In another study, A2BR simulation in LPS-activated murine macrophages favors upregulation of the pro-inflammatory cytokine IL-6 (Ryzhov et al., 2008), suggesting that the effects of A2BR activation might depend on the experimental setting. In murine peritoneal macrophages, activated A2AR suppresses LPS-induced TNF-α release (Kreckler et al., 2006) and promotes its own internalization and degradation by directly boosting lysosomal protease Cathepsin D activity. This latter mechanism is believed to be a strategy for modulating adenosine signaling in inflammatory processes (Skopal et al., 2022).
Dendritic cells
Adenosine regulates dendritic cells (DCs) upon activation of A2AR, A2BR and A1R. Human DCs tune their response to adenosine also by expressing adenosine deaminase (ADA) that scavenges the nucleoside (Desrosiers et al., 2007).
Exposure to adenosine during LPS-induced maturation, drives human monocyte-derived DC (mDC) differentiation towards an anti-inflammatory phenotype, thus impairing mDC ability to effectively prime CD8+ T-cells (Challier et al., 2013). Further, adenosine mediates the recruitment of immature human plasmacytoid dendritic cells (PDCs) to inflammatory sites, via A1R signaling. At the infection site, PDCs undergo maturation by decreasing A1R expression in favor of A2AR, which subsequently limits pro-inflammatory cytokine-release (Schnurr et al., 2004). In human immature DCs (iDCs) and mDCs, chronic A2AR stimulation enhances macropinocytotic activity and membrane expression of major histocompatibility complex class-I (MHC-I) and class-II (MHC-II) molecules. Additional evidence has shown that human DCs developed in the presence of adenosine display reduced ability to support T-helper 1 (Th1) polarization. In mDCs, adenosine stimulation inhibits TNF-α and IL-12 release while boosting IL-10 secretion. iDC allostimulatory capacity is reduced upon adenosine exposure (Panther et al., 2003), these data further supporting the immunoregulatory properties of this metabolite.
Akin to human DCs, murine DCs, differentiated in the presence of adenosine, display impaired allostimulatory capacity, and express increased levels of angiogenic and tolerogenic factors, associated with enhanced ability to promote tumors in vivo (Novitskiy et al., 2008). A2AR signaling limits maturation and inflammatory responses of re-oxygenated murine DCs after hypoxic exposure, critically limiting ischemia reperfusion injury (IRI) (Liu et al., 2015). A1R activation suppresses vesicular MHC-I cross-presentation in murine resting DCs (Chen et al., 2008).
The development of Th17-cells from CD4 naïve lymphocytes, has been linked to exposure to DCs-derived and A2BR-induced IL-6 (Wilson et al., 2011). In this regard, A2BR stimulation, drives differentiation of murine bone marrow-derived DCs into a CD11c(+)Gr-1(+)subset that promotes Th17 responses (Wilson et al., 2011). Genetic A2BR ablation or pharmacological blockade, significantly ameliorates murine autoimmune encephalomyelitis symptoms, by limiting adenosine-mediated IL-6 production by DCs along with Th17-cell differentiation (Wei et al., 2013).
Neutrophils
Neutrophil recruitment is driven by extracellular ATP and adenosine (Rubenich et al., 2021). Endothelium-derived adenosine prevents activated human neutrophils from damaging the microvasculature at inflammatory loci, by controlling their response to complement-coated beads (Kubersky et al., 1989). Adenosine produced by CD73 expressing human neutrophils, limits excessive endothelial paracellular permeability, this avoiding potentially deleterious disturbance in vascular function during inflammation (Lennon et al., 1998). Neutrophil-derived CD73 provides adenosine also in the intestinal lumen, triggering IL-6 release by epithelial cells, which leads to neutrophil activation and degranulation, an event that supports anti-microbial immune responses (Sitaraman et al., 2001).
Adenosine exposure can have antithetical effects on neutrophil function, depending on the adenosine receptor subtype involved. Rapid and beneficial effects of adenosine administration have been reported in COVID-19-related acute respiratory syndrome and pneumonia (Correale et al., 2020; Spiess et al., 2021), which are characterized by heightened neutrophil responses (Li et al., 2023). Upon stimulation of A1R, low adenosine concentrations promote neutrophil adherence to endothelium, chemotaxis towards the inflammation site and FcγR function. Higher adenosine levels, which may occur at sites of tissue damage, inhibit adherence, FcγR function, degranulation, and generation of toxic oxygen metabolites (Salmon and Cronstein, 1990; Cronstein et al., 1992; Visser et al., 2000).
In human and murine neutrophils, A2AR activation suppresses pro-inflammatory cytokine production (McColl et al., 2006) and activates the cyclooxygenase-2 pathway, leading to anti-inflammatory prostaglandin E2 release. This phenomenon could be part of an early regulatory mechanism that favors anti-inflammatory activities at inflamed sites (Cadieux et al., 2005).
In human neutrophils, A2AR expression and ligand affinity are tightly influenced by the surrounding environment. In vitro exposure of human neutrophils to LPS or Th1 cytokines boosts A2AR expression (Fortin et al., 2006). On the other hand, A2AR-ligand affinity is reduced in neutrophils isolated from sepsis patients, this explaining the limited regulatory effects of adenosine in this setting (Kreth et al., 2009). Moreover, A2AR stimulation in neutrophils isolated from murine models or patients with sepsis, fails to suppress cell death, slows aging, and promotes a N2 phenotype, when compared to cells obtained from controls (Lovaszi et al., 2022). In human polymorphonuclear leukocytes, exposure to pro-inflammatory conditions limits A2AR expression by boosting miRNA-214, miRNA-15 and miRNA-16.These miRNAs could serve as useful markers to identify patients more susceptible to severe inflammation (Heyn et al., 2012).
In a murine model of IRI, A2AR activation in neutrophils and CD4+ T-cells, improves animal conditions by limiting inflammation (Sharma et al., 2010). In LPS-induced acute lung injury, downregulation of neutrophil trafficking and responses is noted after A1R activation and is associated with beneficial effects (Ngamsri et al., 2010). Notably, the oxygen-induced iatrogenic exacerbation of acute lung injury is linked to the suppression of A2AR-mediated lung tissue-protecting pathway. Intratracheal injection of a selective A2AR agonist effectively restores the protective effects of endogenous adenosine on lungs (Thiel et al., 2005).
An immunoregulatory role has been noted for the low affinity A2BR that suppresses oxidase activity in murine neutrophils (van der Hoeven et al., 2011) in vitro and in an in vivo model of acute kidney injury, by curbing neutrophil-dependent TNF-α release (Grenz et al., 2012). Similar results have been obtained in murine models of peritonitis and peritonitis-related sepsis, where selective inhibition of CXCR4 and CXCR7 ameliorates disease, limiting neutrophil infiltration at the inflammatory site in an A2BR-mediated manner (Ngamsri et al., 2020).
Human and murine neutrophils can actively contribute to the metabolic control of the inflammatory milieu, by releasing ATP through connexin 43 hemichannels in a protein/phosphatase-A-dependent manner (Eltzschig et al., 2006).
A3R and P2Y2R play an important role in neutrophil chemotaxis. Experiments conducted in murine models of sepsis, revealed that both receptors are closely involved in neutrophil migration to the lungs, suggesting that pharmaceutical approaches targeting these receptors might help in controlling acute lung tissue injury in this condition (Inoue et al., 2008). Human neutrophil recruitment is also positively modulated by A3R (Butler et al., 2012).
NK/NKT-cells
In inflammatory conditions, a counteracting mechanism to prevent deleterious inflammatory responses, is represented by upregulation of adenosine signaling mediators in NK-cells.
In sickle cell disease (SDC), the widely disseminated microvascular IRI boosts A2AR expression in human and murine CD4+invariant-natural-killer-T (iNKT)-cells, via nuclear factor-kappaB (NF-κB)-signaling (Lin et al., 2013). A SCD phase-1 trial of the A2AR agonist regadenoson, resulted in decreased activation of iNKT-cells without significant side effects (Field et al., 2013). Ex vivo activated iNKT-cells from healthy donors, upregulate anti-inflammatory purinergic mediators like A2AR, CD39 and CD73 (Yu et al., 2018). iNKT-cells from SCD patients also express elevated levels of CD39 (Yu et al., 2018). In another study, CD39+ NK-cells were found to be induced by IL-15 and to display more effective cytotoxicity when compared to CD39− NK-cells. There is evidence that A2AR stimulation opposes IL-15-induced generation of human CD39+ NK-cells, by blocking IL-15 signaling (Kang et al., 2023). In the same cells, A2AR is also associated with IL-4 production, which is inhibited by receptor blockade. These data are further corroborated by the evidence that A2AR-deficient mice are characterized by a marked decrease in IL-4, IL-10 and TGF-β, while concomitantly displaying increase in IFN-γ (Nowak et al., 2010). Importantly, adenosine and its analogues have been found to limit NK cell lytic activity (Raskovalova et al., 2005). In murine models of liver IRI, the CD1d-dependent NKT-cell inflammatory response, is abrogated by A2AR activation (Lappas et al., 2006). Similarly, the NKT-cell-mediated liver injury associated to Concanavalin-A-induced acute hepatitis, is abolished by the A2AR agonist CGS21680 and exacerbated in A2AR-deficient mice (Subramanian et al., 2014). Adoptive transfer of murine iNKT and NK-cells with induced A2AR activation into SCD mice improves pulmonary function and prevents exacerbation of hypoxia-reoxygenation-induced pulmonary injury (Wallace and Linden, 2010).
Mast cells
Mast cells play an important role in asthma, where their activity is strongly boosted by adenosine. In ADA-deficient mice that naturally develop lung inflammation reminiscent of asthma, ADA enzyme therapy has beneficial effects, preventing accumulation of adenosine and mast cell degranulation (Zhong et al., 2001). In mouse, airway response to aerosolized adenosine largely depends on mast cells and A3R activation (Tilley et al., 2003).
A3R can activate human mast cells independently of IgE and triggers upregulation of growth factors, cytokines and chemokines, by coupling to the cellular target of receptor mimetic basic secretagogues Gi3 (Baram et al., 2010). The pro-inflammatory effects of adenosine, in murine mast cells, are phosphoinositide 3-kinase gamma (PI3Kgamma)-dependent (Laffargue et al., 2002). Data generated in human A3R-expressing mice, revealed that the human A3R response per se is not sufficient to activate PI3Kgamma-dependent signaling pathways, and to mediate adenosine effects (Yamano et al., 2005). The impact of adenosine on human mast cells also depends on A2BR that triggers release of Th2 cytokines, including IL-4 and IL-13, which, in turn, induce IgE synthesis by B lymphocytes (Ryzhov et al., 2004).
Th1/Tc1-cells
The effect of adenosine on murine Th1 and Tc1 responses has been largely investigated. Adenosine receptor activation inhibits type I cytokine secretion, especially IL-2, limiting expansion in vivo (Erdmann et al., 2005). In mouse, A2AR stimulation, can inhibit nonalcoholic steatohepatitis development by reducing Th17-cell expansion and IL-17-induced JNK-dependent lipotoxicity (Alchera et al., 2017). Inosine administration can also reduce inflammation and mortality in a model of endotoxemia in mice, through abrogation of pro-inflammatory Th1 cytokine production by macrophages and splenocytes. The effect is partially reversed by blockade of A1R and A2AR (Hasko et al., 2000). Further supporting these studies is the evidence that Leishmania infantum parasites stimulate A2AR signaling, limiting the development of Th1 adaptive immunity and favoring parasitic colonization (Lima et al., 2017).
Th17-cells
Adenosine signaling can trigger different responses in human and murine Th17-cells, these depending on the adenosine receptor involved.
In PBMCs from asthma patients, A2AR mRNA levels correlate positively with Treg- and negatively with Th17-cell markers and asthma severity. Accordingly, in a murine model of OVA-induced lung inflammation, pharmacological A2AR stimulation triggers Treg responses, while inhibiting Th17 lung infiltration (Wang et al., 2018). Low levels of CD39 and A2AR expression have been detected in Th17-cells and found to support inflammation in juvenile patients with autoimmune liver disease (Liberal et al., 2016).
A2BR is upregulated in DCs from MS patients, and its occupancy by adenosine triggers IL-6 secretion that promotes Th17-cell differentiation. In line with this, pharmacological A2BR blockade alleviates murine experimental autoimmune encephalomyelitis, via inhibition of the DCs/IL-6-Th17 axis (Wilson et al., 2011; Wei et al., 2013).
In Trichinella spiralis infection, A2AR upregulation accelerates post-infectious irritable bowel syndrome by promoting polarization of CD4+ T-cells into Th17 lymphocytes (Dong et al., 2022).
Th2-cells
In human and mouse, Th2 responses are driven upon activation of different adenosine receptors. In IL-33-activated murine bone-marrow-derived group 2 innate lymphoid cells, A2BR stimulation suppresses IL-13 and IL-5 levels while A2AR activation triggers IL-5 production without affecting IL-13 release (Csoka et al., 2018). In a cockroach allergen model of murine asthma-like pulmonary inflammation, systemic or myeloid A2BR deletion, has beneficial effects associated with decreased Th2-type airways responses, i.e., reduction of lung IL-4, IL-5, and IL-13 (Belikoff et al., 2012).
A1R removal in ADA-deficient mice, leads to enhanced pulmonary inflammation-related damage, associated with increased expression of the Th2 cytokines IL-4 and IL-13 in the lung (Sun et al., 2005).
Tregs
Human and murine Tregs express CD39 that initiates ATP/ADP hydrolysis to ultimately generate adenosine. Expression of A2AR by Tregs enables adenosine to act in an autocrine manner, favoring the maintenance of the Treg pool. A2AR stimulation further boosts CD39 and CD73 expression in Tregs isolated from septic mice (Bao et al., 2016). Notably, A2AR stimulation effectively counteracts Treg deficiency that drives autoimmunity in scurfy mice (He et al., 2017).
Adoptively transferred CD73-deficient or A2AR-deficient Tregs, fail to confer protection in a murine IRI model, while pharmacological A2AR stimulation augments the protective effect of wild-type and CD73-deficient Tregs (Kinsey et al., 2012). In OVA-induced lung inflammation, pharmacological A2AR activation promotes anti-inflammatory Treg responses (Wang et al., 2018). The same receptor mediates Treg homing to the site of inflammation in a murine model of experimental autoimmune uveoretinitis, and in cells from human uveitis patients, in vitro (Peters et al., 2023). In contrast, A2BR inhibits Treg cell infiltration in a murine heterotopic tracheal model of bronchiolitis obliterans (Zhao et al., 2010), postulating a different role of this receptor in this disease setting. Figure 1 represents adenosine receptor signaling in immune cells.
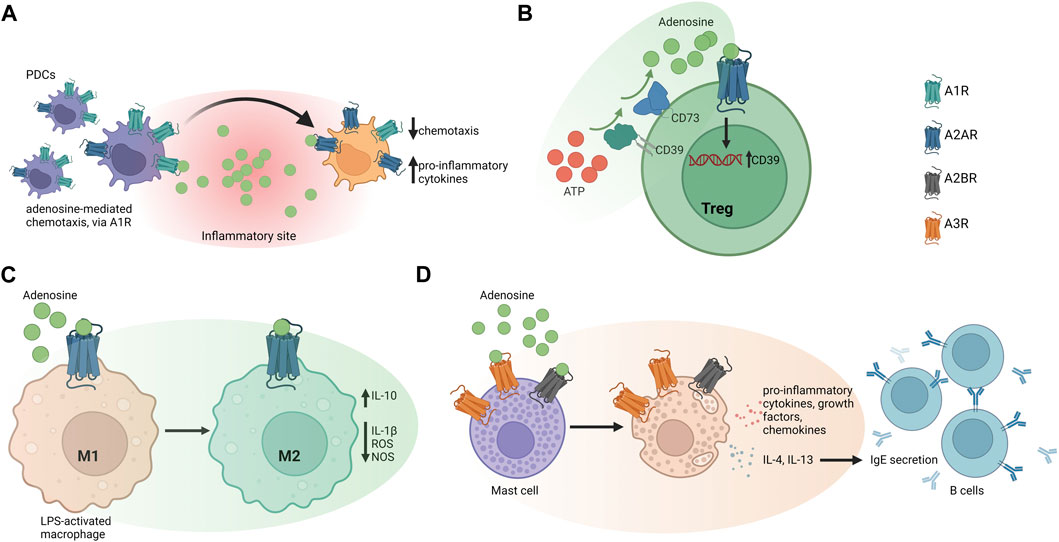
Figure 1. Adenosine receptor signaling in different immune cell types. (A) Adenosine mediates immature human plasmacytoid dendritic cells (PDCs) recruitment to inflammatory sites, via A1 receptor (A1R) signaling. At the infection site, PDCs undergo maturation by decreasing A1R expression, in favor of A2AR, which limits pro-inflammatory cytokine release. (B) Human and murine Tregs express CD39 that initiates ATP/ADP hydrolysis to ultimately produce adenosine. Expression of A2AR by Tregs enables adenosine to act in an autocrine manner that might favor the maintenance of the Treg-cell pool. (C) In mouse peritoneal macrophages, adenosine suppresses LPS-induced IL-1β release, ROS, and nitrite production. A2BR stimulation boosts IL-10 production and suppresses pro-inflammatory responses (D) A3R can activate human mast cells independently of IgE and triggers upregulation of growth factors, cytokines, and chemokines. The effect of adenosine on human mast cells also depends on A2BR, which promotes release of Th2 cytokines like IL-4 and IL-13 to induce IgE synthesis in B lymphocytes.
Supplementary Table S1 summarizes the effects of adenosine receptor activation in different cell types and disease models.
Adenosine signaling in IBD
Several studies support the beneficial effects of adenosine in IBD. Increasing evidence suggests that agonism or inhibition of adenosine receptors A2AR, A2BR and A3R might be exploited therapeutically (Vuerich et al., 2020).
A2AR
A2AR signaling is often impaired in IBD. In the colonic mucosa of UC patients, A2AR is post-transcriptionally downregulated by miR-16, this limiting anti-inflammatory inhibition of NF-κB signaling pathway (Tian et al., 2016).
The immunomodulatory role of A2AR in inflammation depends on its effect on T-cells, in which expression levels are subset-specific. A2AR expression is higher in CD4+ than CD8+ T-cells; however, upon T-cell activation, the receptor levels are mainly boosted in the latter (Koshiba et al., 1999). During CD8+ and CD4+ T-cell activation, A2AR signaling inhibits cytotoxicity and cytokine-release activity, while only marginally affecting proliferation (Ohta et al., 2009). A2AR stimulation favors also CD4+CD25highFoxP3+ Treg expansion, and this is associated with increased CTLA-4 levels and heightened suppression (Ohta et al., 2012). Blood and lamina propria of CD patients display reduced frequencies of suppressive CD39+ Th17-cells, an immunoregulatory Th17-cell population resistant to the effects of adenosine, as a result of heightened ADA and decreased A2AR levels (Longhi et al., 2014).
A2AR-deficient mice are more susceptible to tissue damage induced by sub-threshold doses of inflammatory stimuli (Ohta and Sitkovsky, 2001). These findings are corroborated by the evidence that administration of the A2AR selective agonist ATL-146e reduces intestinal mucosa inflammation by limiting leukocyte infiltration and pro-inflammatory cytokine release in murine experimental models of IBD (Odashima et al., 2005). Studies have shown that electroacupuncture inhibits visceral pain in IBD mice by boosting A2AR, A1R and A3R levels, while inhibiting A2BR in colon tissue (Hou et al., 2019). In murine models of chronic colitis, administration of ADA inhibitors results in beneficial effects through enhanced activation of A2AR and A3R (Antonioli et al., 2010). The immunosuppressive properties of A2AR are noted also in murine cancer models (Sitkovsky et al., 2008). Genetic deletion of A2AR in host animals favors rejection of immunogenic tumors and anti-cancer immunity is restored only upon administration of A2AR antagonists, or by silencing the receptor via siRNA pretreatment on T-cells (Ohta et al., 2006).
Despite robust evidence of an immunosuppressive role in cancer and experimental colitis models, a study showed that increased A2AR expression as a consequence of T. spiralis infection, promotes post-infectious irritable bowel syndrome by inducing Th17-cell polarization in mouse (Dong et al., 2022); this suggesting a different role of this receptor in specific disease settings.
A2AR inhibitory effects on colonic motility are enhanced in the presence of bowel inflammation, as noted when colonic longitudinal muscle preparations from healthy rats or with experimental colitis are used (Antonioli et al., 2006). Ex vivo treatment with a highly polar, perorally nonabsorbable A2AR selective agonist, significantly ameliorates acetylcholine-induced contractions in ileum/jejunum preparations from inflamed rats (El-Tayeb et al., 2011). In comparable settings, A2AR stimulation, or A2BR blockade, prevents inflammation-induced contractile disturbance (Michael et al., 2010).
In various experimental models of colitis in rats, administration of the A2AR agonist polydeoxyribonucleotide restores tissue structural integrity by reducing inflammatory cytokine expression, myeloperoxidase activity, and malondialdehyde. Accordingly, all the beneficial effects are abrogated by concomitant administration of A2AR antagonist DMPX (Pallio et al., 2016).
A2BR
The pro-inflammatory, low affinity A2BR is the predominant adenosine receptor expressed in the colon.
There is evidence that A2BR depletion ameliorates the course of experimental colitis in different murine models (Kolachala V. L. et al., 2008). In DSS-induced colitis, administration of the A2BR antagonist ATL-801 prevents weight loss and suppresses the inflammatory infiltrate in the colonic mucosa (Kolachala V. et al., 2008). In a mouse model of IRI and in a cell model of acute hypoxia, A2BR antagonism improved the intestinal epithelial structure and barrier function, further supporting the pro-inflammatory role of A2BR activation (Yang et al., 2014).
Conversely, other studies provided evidence of a selective role for epithelial A2BR signaling in attenuating colonic inflammation in the context of DSS-induced colitis (Aherne et al., 2015). Similarly, additional investigations in the setting of DSS colitis in mice with partial genetic deficiency of netrin-1, a molecule implicated in the modulation of leukocyte trafficking, indicate a role for A2BR in mediating netrin-1 protective effects (Aherne et al., 2012). A2BR-mediated immunosuppression is critically involved in cancer immune escaping. A2BR pharmacological inactivation or genetic depletion prevents effector T-cell inhibition by the hypoxic tumor microenvironment, thus facilitating tumor rejection (Lukashev et al., 2007).
A3R
The Gi protein-associated A3R has been proposed having both anti- and pro-inflammatory properties. A study by Rybaczyk and coll showed a positive correlation between A3R downregulation and acute inflammatory score, disease chronicity and purine genes dysregulation, when considering colonic mucosal biopsies or PBMCs obtained from CD, UC or control subjects (Rybaczyk et al., 2009). In line with this, in colonic epithelial cells from UC patients, A3R activation mitigates pro-inflammatory cytokine production, by curbing NF-κB signaling (Ren et al., 2014; Ren et al., 2020). In another study on colonic epithelial cells from UC patients, A3R is downregulated and its expression levels inversely correlate with those of mir-206, a post-translational regulator associated with disease histological activity (Minacapelli et al., 2019) and linked to exacerbation of DSS colitis in mice (Wu et al., 2017). In mice with DSS-induced colitis downregulation of A3R is partially limited following miR-206-antagomir treatment (Wu et al., 2017).
Treatment of chronic colitis induced by 2,4,6-trinitrobenzene sulfonic acid using the A3R agonist N (6)-(3-iodobenzyl)-adenosine-5-N-methyluronamide (IB-MECA), further supports the protective role of this adenosine receptor. The treatment contains the colitis-induced upregulation of several pro-inflammatory genes including P2X1R, P2X4R, P2X7R, P2Y2R, and P2Y6R, downregulated P2X2R, P2Y1R, and P2Y4R, significantly reducing the inflammatory score and ameliorating disease course in animals (Guzman et al., 2006). Additional investigations showed that the A3R agonist AR170 contrasts inflammatory cell infiltration of the colon and decreases pro-inflammatory cytokine levels also in 2,4-dinitrobenzene sulfonic acid-induced colitis in rats (Antonioli et al., 2020). In contrast to the above studies, genetic deletion of A3R resulted in disrupted intestinal transit, conferring protection against DSS colitis (Ren et al., 2011). In line with these findings, Ochaion and coll reported upregulation of A3R levels in PBMCs from patients affected by rheumatoid arthritis, psoriasis, and CD. A3R upregulation was found to be related to NF-κB and CREB protein pathways (Ochaion et al., 2009). Figure 2 shows adenosine-related pathways in IBD.
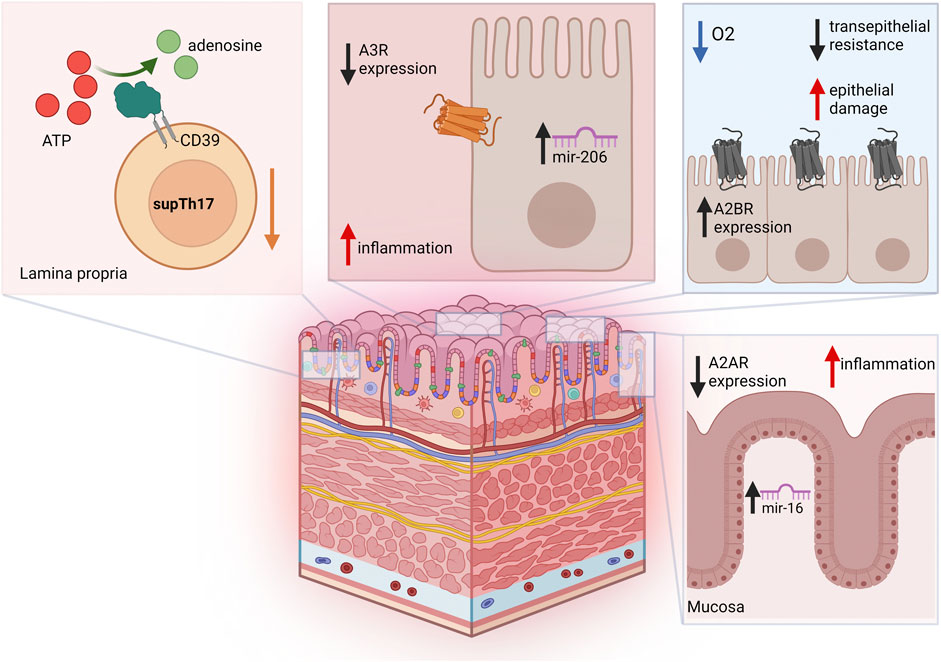
Figure 2. Adenosine-related pathways in IBD. Blood and lamina propria of Crohn’s disease patients are characterized by reduced frequencies of suppressive CD39+ Th17-cells (supTh17), an immunoregulatory Th17-cell population that is impaired in IBD. In colon epithelial cells from UC patients, A3R is downregulated, and its expression levels inversely correlate with mir-206. Increase in A2BR levels during intestinal IRI and acute hypoxia has been implicated in reduced transepithelial resistance and increased epithelial damage. In the colonic mucosa of UC patients, A2AR is post-transcriptionally downregulated by miR-16, this limiting A2AR anti-inflammatory effects.
Future perspectives and conclusions
We have briefly discussed how adenosine signaling modulates immune function and dictates outcomes in experimental and human IBD. How to fine-tuning adenosine-mediated immune responses to selectively halt inflammation at the sites of interest, while inducing homeostasis remains unclear. The development of purinergic signaling-based therapies, in combination with conventional treatments, could help promoting and maintaining immunotolerance in IBD and other immune-mediated chronic conditions.
Author contributions
MV: Writing–review and editing, Writing–original draft. DN: Data curation, Funding acquisition, Writing–original draft. DF: Conceptualization, Writing–review and editing. ML: Conceptualization, Writing–review and editing, Funding acquisition.
Funding
The author(s) declare that financial support was received for the research, authorship, and/or publication of this article. This work has been supported by the National Institutes of Health (R01 DK124408 to ML); the Crohn’s and Colitis Foundation (Litwin IBD Pioneers Award to ML); and the Boehringer Ingelheim Fonds MD fellowship (to DN).
Conflict of interest
Author MV was employed by Novartis Pharma AG.
The remaining authors declare that the research was conducted in the absence of any commercial or financial relationships that could be construed as a potential conflict of interest.
Publisher’s note
All claims expressed in this article are solely those of the authors and do not necessarily represent those of their affiliated organizations, or those of the publisher, the editors and the reviewers. Any product that may be evaluated in this article, or claim that may be made by its manufacturer, is not guaranteed or endorsed by the publisher.
Supplementary Material
The Supplementary Material for this article can be found online at: https://www.frontiersin.org/articles/10.3389/fcell.2024.1429736/full#supplementary-material
References
Aherne, C. M., Collins, C. B., Masterson, J. C., Tizzano, M., Boyle, T. A., Westrich, J. A., et al. (2012). Neuronal guidance molecule netrin-1 attenuates inflammatory cell trafficking during acute experimental colitis. Gut 61, 695–705. doi:10.1136/gutjnl-2011-300012
Aherne, C. M., Saeedi, B., Collins, C. B., Masterson, J. C., McNamee, E. N., Perrenoud, L., et al. (2015). Epithelial-specific A2B adenosine receptor signaling protects the colonic epithelial barrier during acute colitis. Mucosal Immunol. 8, 1324–1338. doi:10.1038/mi.2015.22
Alchera, E., Rolla, S., Imarisio, C., Bardina, V., Valente, G., Novelli, F., et al. (2017). Adenosine A2a receptor stimulation blocks development of nonalcoholic steatohepatitis in mice by multilevel inhibition of signals that cause immunolipotoxicity. Transl. Res. 182, 75–87. doi:10.1016/j.trsl.2016.11.009
Allard, B., Longhi, M. S., Robson, S. C., and Stagg, J. (2017). The ectonucleotidases CD39 and CD73: novel checkpoint inhibitor targets. Immunol. Rev. 276, 121–144. doi:10.1111/imr.12528
Antonioli, L., Fornai, M., Colucci, R., Awwad, O., Ghisu, N., Tuccori, M., et al. (2010). The blockade of adenosine deaminase ameliorates chronic experimental colitis through the recruitment of adenosine A2A and A3 receptors. J. Pharmacol. Exp. Ther. 335, 434–442. doi:10.1124/jpet.110.171223
Antonioli, L., Fornai, M., Colucci, R., Ghisu, N., Blandizzi, C., and Del Tacca, M. (2006). A2a receptors mediate inhibitory effects of adenosine on colonic motility in the presence of experimental colitis. Inflamm. Bowel Dis. 12, 117–122. doi:10.1097/01.MIB.0000198535.13822.a9
Antonioli, L., Lucarini, E., Lambertucci, C., Fornai, M., Pellegrini, C., Benvenuti, L., et al. (2020). The anti-inflammatory and pain-relieving effects of AR170, an adenosine A(3) receptor agonist, in a rat model of colitis. Cells 9, 1509. doi:10.3390/cells9061509
Bao, R., Shui, X., Hou, J., Li, J., Deng, X., Zhu, X., et al. (2016). Adenosine and the adenosine A2A receptor agonist, CGS21680, upregulate CD39 and CD73 expression through E2F-1 and CREB in regulatory T cells isolated from septic mice. Int. J. Mol. Med. 38, 969–975. doi:10.3892/ijmm.2016.2679
Baram, D., Dekel, O., Mekori, Y. A., and Sagi-Eisenberg, R. (2010). Activation of mast cells by trimeric G protein Gi3; coupling to the A3 adenosine receptor directly and upon T cell contact. J. Immunol. 184, 3677–3688. doi:10.4049/jimmunol.0901333
Belikoff, B. G., Vaickus, L. J., Sitkovsky, M., and Remick, D. G. (2012). A2B adenosine receptor expression by myeloid cells is proinflammatory in murine allergic-airway inflammation. J. Immunol. 189, 3707–3713. doi:10.4049/jimmunol.1201207
Butler, M., Sanmugalingam, D., Burton, V. J., Wilson, T., Pearson, R., Watson, R. P., et al. (2012). Impairment of adenosine A3 receptor activity disrupts neutrophil migratory capacity and impacts innate immune function in vivo. Eur. J. Immunol. 42, 3358–3368. doi:10.1002/eji.201242655
Cadieux, J. S., Leclerc, P., St-Onge, M., Dussault, A. A., Laflamme, C., Picard, S., et al. (2005). Potentiation of neutrophil cyclooxygenase-2 by adenosine: an early anti-inflammatory signal. J. Cell Sci. 118, 1437–1447. doi:10.1242/jcs.01737
Challier, J., Bruniquel, D., Sewell, A. K., and Laugel, B. (2013). Adenosine and cAMP signalling skew human dendritic cell differentiation towards a tolerogenic phenotype with defective CD8(+) T-cell priming capacity. Immunology 138, 402–410. doi:10.1111/imm.12053
Chen, L., Fredholm, B. B., and Jondal, M. (2008). Adenosine, through the A1 receptor, inhibits vesicular MHC class I cross-presentation by resting DC. Mol. Immunol. 45, 2247–2254. doi:10.1016/j.molimm.2007.11.016
Correale, P., Caracciolo, M., Bilotta, F., Conte, M., Cuzzola, M., Falcone, C., et al. (2020). Therapeutic effects of adenosine in high flow 21% oxygen aereosol in patients with Covid19-pneumonia. PLoS One 15, e0239692. doi:10.1371/journal.pone.0239692
Cronstein, B. N., Levin, R. I., Philips, M., Hirschhorn, R., Abramson, S. B., and Weissmann, G. (1992). Neutrophil adherence to endothelium is enhanced via adenosine A1 receptors and inhibited via adenosine A2 receptors. J. Immunol. 148, 2201–2206. doi:10.4049/jimmunol.148.7.2201
Cronstein, B. N., and Sitkovsky, M. (2017). Adenosine and adenosine receptors in the pathogenesis and treatment of rheumatic diseases. Nat. Rev. Rheumatol. 13, 41–51. doi:10.1038/nrrheum.2016.178
Csoka, B., Nemeth, Z. H., Duerr, C. U., Fritz, J. H., Pacher, P., and Hasko, G. (2018). Adenosine receptors differentially regulate type 2 cytokine production by IL-33-activated bone marrow cells, ILC2s, and macrophages. FASEB J. 32, 829–837. doi:10.1096/fj.201700770R
Desrosiers, M. D., Cembrola, K. M., Fakir, M. J., Stephens, L. A., Jama, F. M., Shameli, A., et al. (2007). Adenosine deamination sustains dendritic cell activation in inflammation. J. Immunol. 179, 1884–1892. doi:10.4049/jimmunol.179.3.1884
Devi, V. J., Radhika, A., and Biju, P. G. (2023). Adenosine receptor activation promotes macrophage class switching from LPS-induced acute inflammatory M1 to anti-inflammatory M2 phenotype. Immunobiology 228, 152362. doi:10.1016/j.imbio.2023.152362
Dong, L. W., Ma, Z. C., Fu, J., Huang, B. L., Liu, F. J., Sun, D., et al. (2022). Upregulated adenosine 2A receptor accelerates post-infectious irritable bowel syndrome by promoting CD4+ T cells’ T helper 17 polarization. World J. Gastroenterol. 28, 2955–2967. doi:10.3748/wjg.v28.i25.2955
El-Tayeb, A., Michael, S., Abdelrahman, A., Behrenswerth, A., Gollos, S., Nieber, K., et al. (2011). Development of polar adenosine A2A receptor agonists for inflammatory bowel disease: synergism with A2B antagonists. ACS Med. Chem. Lett. 2, 890–895. doi:10.1021/ml200189u
Eltzschig, H. K., Eckle, T., Mager, A., Kuper, N., Karcher, C., Weissmuller, T., et al. (2006). ATP release from activated neutrophils occurs via connexin 43 and modulates adenosine-dependent endothelial cell function. Circ. Res. 99, 1100–1108. doi:10.1161/01.RES.0000250174.31269.70
Erdmann, A. A., Gao, Z. G., Jung, U., Foley, J., Borenstein, T., Jacobson, K. A., et al. (2005). Activation of Th1 and Tc1 cell adenosine A2A receptors directly inhibits IL-2 secretion in vitro and IL-2-driven expansion in vivo. Blood 105, 4707–4714. doi:10.1182/blood-2004-04-1407
Field, J. J., Lin, G., Okam, M. M., Majerus, E., Keefer, J., Onyekwere, O., et al. (2013). Sickle cell vaso-occlusion causes activation of iNKT cells that is decreased by the adenosine A2A receptor agonist regadenoson. Blood 121, 3329–3334. doi:10.1182/blood-2012-11-465963
Fortin, A., Harbour, D., Fernandes, M., Borgeat, P., and Bourgoin, S. (2006). Differential expression of adenosine receptors in human neutrophils: up-regulation by specific Th1 cytokines and lipopolysaccharide. J. Leukoc. Biol. 79, 574–585. doi:10.1189/jlb.0505249
Grenz, A., Kim, J. H., Bauerle, J. D., Tak, E., Eltzschig, H. K., and Clambey, E. T. (2012). Adora2b adenosine receptor signaling protects during acute kidney injury via inhibition of neutrophil-dependent TNF-α release. J. Immunol. 189, 4566–4573. doi:10.4049/jimmunol.1201651
Guzman, J., Yu, J. G., Suntres, Z., Bozarov, A., Cooke, H., Javed, N., et al. (2006). ADOA3R as a therapeutic target in experimental colitis: proof by validated high-density oligonucleotide microarray analysis. Inflamm. Bowel Dis. 12, 766–789. doi:10.1097/00054725-200608000-00014
Hasko, G., Kuhel, D. G., Nemeth, Z. H., Mabley, J. G., Stachlewitz, R. F., Virag, L., et al. (2000). Inosine inhibits inflammatory cytokine production by a posttranscriptional mechanism and protects against endotoxin-induced shock. J. Immunol. 164, 1013–1019. doi:10.4049/jimmunol.164.2.1013
Hasko, G., Linden, J., Cronstein, B., and Pacher, P. (2008). Adenosine receptors: therapeutic aspects for inflammatory and immune diseases. Nat. Rev. Drug Discov. 7, 759–770. doi:10.1038/nrd2638
He, B., Hoang, T. K., Wang, T., Ferris, M., Taylor, C. M., Tian, X., et al. (2017). Resetting microbiota by Lactobacillus reuteri inhibits T reg deficiency-induced autoimmunity via adenosine A2A receptors. J. Exp. Med. 214, 107–123. doi:10.1084/jem.20160961
Heyn, J., Ledderose, C., Hinske, L. C., Limbeck, E., Mohnle, P., Lindner, H. A., et al. (2012). Adenosine A2A receptor upregulation in human PMNs is controlled by miRNA-214, miRNA-15, and miRNA-16. Shock 37, 156–163. doi:10.1097/SHK.0b013e31823f16bc
Hou, T., Xiang, H., Yu, L., Su, W., Shu, Y., Li, H., et al. (2019). Electroacupuncture inhibits visceral pain via adenosine receptors in mice with inflammatory bowel disease. Purinergic Signal 15, 193–204. doi:10.1007/s11302-019-09655-4
Inoue, Y., Chen, Y., Hirsh, M. I., Yip, L., and Junger, W. G. (2008). A3 and P2Y2 receptors control the recruitment of neutrophils to the lungs in a mouse model of sepsis. Shock 30, 173–177. doi:10.1097/shk.0b013e318160dad4
Jiang, Z. G., Wu, Y., Csizmadia, E., Feldbrugge, L., Enjyoji, K., Tigges, J., et al. (2014). Characterization of circulating microparticle-associated CD39 family ecto-nucleotidases in human plasma. Purinergic Signal 10, 611–618. doi:10.1007/s11302-014-9423-6
Kang, G., Zhao, X., Sun, J., Cheng, C., Wang, C., Tao, L., et al. (2023). A2AR limits IL-15-induced generation of CD39(+) NK cells with high cytotoxicity. Int. Immunopharmacol. 114, 109567. doi:10.1016/j.intimp.2022.109567
Kinsey, G. R., Huang, L., Jaworska, K., Khutsishvili, K., Becker, D. A., Ye, H., et al. (2012). Autocrine adenosine signaling promotes regulatory T cell-mediated renal protection. J. Am. Soc. Nephrol. 23, 1528–1537. doi:10.1681/ASN.2012010070
Kolachala, V., Ruble, B., Vijay-Kumar, M., Wang, L., Mwangi, S., Figler, H., et al. (2008b). Blockade of adenosine A2B receptors ameliorates murine colitis. Br. J. Pharmacol. 155, 127–137. doi:10.1038/bjp.2008.227
Kolachala, V. L., Vijay-Kumar, M., Dalmasso, G., Yang, D., Linden, J., Wang, L., et al. (2008a). A2B adenosine receptor gene deletion attenuates murine colitis. Gastroenterology 135, 861–870. doi:10.1053/j.gastro.2008.05.049
Koshiba, M., Rosin, D. L., Hayashi, N., Linden, J., and Sitkovsky, M. V. (1999). Patterns of A2A extracellular adenosine receptor expression in different functional subsets of human peripheral T cells. Flow cytometry studies with anti-A2A receptor monoclonal antibodies. Mol. Pharmacol. 55, 614–624.
Kreckler, L. M., Wan, T. C., Ge, Z. D., and Auchampach, J. A. (2006). Adenosine inhibits tumor necrosis factor-alpha release from mouse peritoneal macrophages via A2A and A2B but not the A3 adenosine receptor. J. Pharmacol. Exp. Ther. 317, 172–180. doi:10.1124/jpet.105.096016
Kreth, S., Kaufmann, I., Ledderose, C., Luchting, B., and Thiel, M. (2009). Reduced ligand affinity leads to an impaired function of the adenosine A2A receptor of human granulocytes in sepsis. J. Cell Mol. Med. 13, 985–994. doi:10.1111/j.1582-4934.2008.00530.x
Kubersky, S. M., Hirschhorn, R., Broekman, M. J., and Cronstein, B. N. (1989). Occupancy of adenosine receptors on human neutrophils inhibits respiratory burst stimulated by ingestion of complement-coated particles and occupancy of chemoattractant but not Fc receptors. Inflammation 13, 591–599. doi:10.1007/BF00916765
Kukulski, F., Levesque, S. A., Lavoie, E. G., Lecka, J., Bigonnesse, F., Knowles, A. F., et al. (2005). Comparative hydrolysis of P2 receptor agonists by NTPDases 1, 2, 3 and 8. Purinergic Signal 1, 193–204. doi:10.1007/s11302-005-6217-x
Laffargue, M., Calvez, R., Finan, P., Trifilieff, A., Barbier, M., Altruda, F., et al. (2002). Phosphoinositide 3-kinase gamma is an essential amplifier of mast cell function. Immunity 16, 441–451. doi:10.1016/s1074-7613(02)00282-0
Lappas, C. M., Day, Y. J., Marshall, M. A., Engelhard, V. H., and Linden, J. (2006). Adenosine A2A receptor activation reduces hepatic ischemia reperfusion injury by inhibiting CD1d-dependent NKT cell activation. J. Exp. Med. 203, 2639–2648. doi:10.1084/jem.20061097
Lennon, P. F., Taylor, C. T., Stahl, G. L., and Colgan, S. P. (1998). Neutrophil-derived 5'-adenosine monophosphate promotes endothelial barrier function via CD73-mediated conversion to adenosine and endothelial A2B receptor activation. J. Exp. Med. 188, 1433–1443. doi:10.1084/jem.188.8.1433
Li, J., Zhang, K., Zhang, Y., Gu, Z., and Huang, C. (2023). Neutrophils in COVID-19: recent insights and advances. Virol. J. 20, 169. doi:10.1186/s12985-023-02116-w
Liberal, R., Grant, C. R., Ma, Y., Csizmadia, E., Jiang, Z. G., Heneghan, M. A., et al. (2016). CD39 mediated regulation of Th17-cell effector function is impaired in juvenile autoimmune liver disease. J. Autoimmun. 72, 102–112. doi:10.1016/j.jaut.2016.05.005
Lima, M. H. F., Sacramento, L. A., Quirino, G. F. S., Ferreira, M. D., Benevides, L., Santana, A. K. M., et al. (2017). Leishmania infantum parasites subvert the host inflammatory response through the adenosine A2(A) receptor to promote the establishment of infection. Front. Immunol. 8, 815. doi:10.3389/fimmu.2017.00815
Lin, G., Field, J. J., Yu, J. C., Ken, R., Neuberg, D., Nathan, D. G., et al. (2013). NF-κB is activated in CD4+ iNKT cells by sickle cell disease and mediates rapid induction of adenosine A2A receptors. PLoS One 8, e74664. doi:10.1371/journal.pone.0074664
Liu, C., Shang, Q., Bai, Y., Guo, C., Zhu, F., Zhang, L., et al. (2015). Adenosine A2A receptor, a potential valuable target for controlling reoxygenated DCs-triggered inflammation. Mol. Immunol. 63, 559–565. doi:10.1016/j.molimm.2014.10.012
Longhi, M. S., Moss, A., Bai, A., Wu, Y., Huang, H., Cheifetz, A., et al. (2014). Characterization of human CD39+ Th17 cells with suppressor activity and modulation in inflammatory bowel disease. PLoS One 9, e87956. doi:10.1371/journal.pone.0087956
Lovaszi, M., Nemeth, Z. H., Pacher, P., Gause, W. C., Wagener, G., and Hasko, G. (2022). A(2A) adenosine receptor activation prevents neutrophil aging and promotes polarization from N1 towards N2 phenotype. Purinergic Signal 18, 345–358. doi:10.1007/s11302-022-09884-0
Lukashev, D., Sitkovsky, M., and Ohta, A. (2007). From "Hellstrom Paradox" to anti-adenosinergic cancer immunotherapy. Purinergic Signal 3, 129–134. doi:10.1007/s11302-006-9044-9
McColl, S. R., St-Onge, M., Dussault, A. A., Laflamme, C., Bouchard, L., Boulanger, J., et al. (2006). Immunomodulatory impact of the A2A adenosine receptor on the profile of chemokines produced by neutrophils. FASEB J. 20, 187–189. doi:10.1096/fj.05-4804fje
Michael, S., Warstat, C., Michel, F., Yan, L., Muller, C. E., and Nieber, K. (2010). Adenosine A(2A) agonist and A(2B) antagonist mediate an inhibition of inflammation-induced contractile disturbance of a rat gastrointestinal preparation. Purinergic Signal 6, 117–124. doi:10.1007/s11302-009-9174-y
Minacapelli, C. D., Bajpai, M., Geng, X., Van Gurp, J., Poplin, E., Amenta, P. S., et al. (2019). miR-206 as a biomarker for response to mesalamine treatment in ulcerative colitis. Inflamm. Bowel Dis. 25, 78–84. doi:10.1093/ibd/izy279
Nemeth, Z. H., Lutz, C. S., Csoka, B., Deitch, E. A., Leibovich, S. J., Gause, W. C., et al. (2005). Adenosine augments IL-10 production by macrophages through an A2B receptor-mediated posttranscriptional mechanism. J. Immunol. 175, 8260–8270. doi:10.4049/jimmunol.175.12.8260
Ng, S. C., Shi, H. Y., Hamidi, N., Underwood, F. E., Tang, W., Benchimol, E. I., et al. (2017). Worldwide incidence and prevalence of inflammatory bowel disease in the 21st century: a systematic review of population-based studies. Lancet 390, 2769–2778. doi:10.1016/S0140-6736(17)32448-0
Ngamsri, K. C., Jans, C., Putri, R. A., Schindler, K., Gamper-Tsigaras, J., Eggstein, C., et al. (2020). Inhibition of CXCR4 and CXCR7 is protective in acute peritoneal inflammation. Front. Immunol. 11, 407. doi:10.3389/fimmu.2020.00407
Ngamsri, K. C., Wagner, R., Vollmer, I., Stark, S., and Reutershan, J. (2010). Adenosine receptor A1 regulates polymorphonuclear cell trafficking and microvascular permeability in lipopolysaccharide-induced lung injury. J. Immunol. 185, 4374–4384. doi:10.4049/jimmunol.1000433
Novitskiy, S. V., Ryzhov, S., Zaynagetdinov, R., Goldstein, A. E., Huang, Y., Tikhomirov, O. Y., et al. (2008). Adenosine receptors in regulation of dendritic cell differentiation and function. Blood 112, 1822–1831. doi:10.1182/blood-2008-02-136325
Nowak, M., Lynch, L., Yue, S., Ohta, A., Sitkovsky, M., Balk, S. P., et al. (2010). The A2aR adenosine receptor controls cytokine production in iNKT cells. Eur. J. Immunol. 40, 682–687. doi:10.1002/eji.200939897
Ochaion, A., Bar-Yehuda, S., Cohen, S., Barer, F., Patoka, R., Amital, H., et al. (2009). The anti-inflammatory target A(3) adenosine receptor is over-expressed in rheumatoid arthritis, psoriasis and Crohn's disease. Cell Immunol. 258, 115–122. doi:10.1016/j.cellimm.2009.03.020
Odashima, M., Bamias, G., Rivera-Nieves, J., Linden, J., Nast, C. C., Moskaluk, C. A., et al. (2005). Activation of A2A adenosine receptor attenuates intestinal inflammation in animal models of inflammatory bowel disease. Gastroenterology 129, 26–33. doi:10.1053/j.gastro.2005.05.032
Ohta, A., Gorelik, E., Prasad, S. J., Ronchese, F., Lukashev, D., Wong, M. K., et al. (2006). A2A adenosine receptor protects tumors from antitumor T cells. Proc. Natl. Acad. Sci. U. S. A. 103, 13132–13137. doi:10.1073/pnas.0605251103
Ohta, A., Kini, R., Ohta, A., Subramanian, M., Madasu, M., and Sitkovsky, M. (2012). The development and immunosuppressive functions of CD4(+) CD25(+) FoxP3(+) regulatory T cells are under influence of the adenosine-A2A adenosine receptor pathway. Front. Immunol. 3, 190. doi:10.3389/fimmu.2012.00190
Ohta, A., Ohta, A., Madasu, M., Kini, R., Subramanian, M., Goel, N., et al. (2009). A2A adenosine receptor may allow expansion of T cells lacking effector functions in extracellular adenosine-rich microenvironments. J. Immunol. 183, 5487–5493. doi:10.4049/jimmunol.0901247
Ohta, A., and Sitkovsky, M. (2001). Role of G-protein-coupled adenosine receptors in downregulation of inflammation and protection from tissue damage. Nature 414, 916–920. doi:10.1038/414916a
Pallio, G., Bitto, A., Pizzino, G., Galfo, F., Irrera, N., Squadrito, F., et al. (2016). Adenosine receptor stimulation by polydeoxyribonucleotide improves tissue repair and symptomology in experimental colitis. Front. Pharmacol. 7, 273. doi:10.3389/fphar.2016.00273
Panther, E., Corinti, S., Idzko, M., Herouy, Y., Napp, M., la Sala, A., et al. (2003). Adenosine affects expression of membrane molecules, cytokine and chemokine release, and the T-cell stimulatory capacity of human dendritic cells. Blood 101, 3985–3990. doi:10.1182/blood-2002-07-2113
Peters, K., McDonald, T., Muhammad, F., Walsh, M., Drenen, K., Montieth, A., et al. (2023). A2Ar-dependent PD-1+ and TIGIT+ Treg cells have distinct homing requirements to suppress autoimmune uveitis in mice. Mucosal Immunol. 16, 422–431. doi:10.1016/j.mucimm.2023.04.005
Raskovalova, T., Huang, X., Sitkovsky, M., Zacharia, L. C., Jackson, E. K., and Gorelik, E. (2005). Gs protein-coupled adenosine receptor signaling and lytic function of activated NK cells. J. Immunol. 175, 4383–4391. doi:10.4049/jimmunol.175.7.4383
Ren, T., Grants, I., Alhaj, M., McKiernan, M., Jacobson, M., Hassanain, H. H., et al. (2011). Impact of disrupting adenosine A₃ receptors (A₃⁻/⁻ AR) on colonic motility or progression of colitis in the mouse. Inflamm. Bowel Dis. 17, 1698–1713. doi:10.1002/ibd.21553
Ren, T., Qiu, Y., Wu, W., Feng, X., Ye, S., Wang, Z., et al. (2014). Activation of adenosine A3 receptor alleviates TNF-α-induced inflammation through inhibition of the NF-κB signaling pathway in human colonic epithelial cells. Mediat. Inflamm. 2014, 818251. doi:10.1155/2014/818251
Ren, T. H., Lv, M. M., An, X. M., Leung, W. K., and Seto, W. K. (2020). Activation of adenosine A3 receptor inhibits inflammatory cytokine production in colonic mucosa of patients with ulcerative colitis by down-regulating the nuclear factor-kappa B signaling. J. Dig. Dis. 21, 38–45. doi:10.1111/1751-2980.12831
Rubenich, D. S., de Souza, P. O., Omizzollo, N., Lenz, G. S., Sevigny, J., and Braganhol, E. (2021). Neutrophils: fast and furious-the nucleotide pathway. Purinergic Signal 17, 371–383. doi:10.1007/s11302-021-09786-7
Rybaczyk, L., Rozmiarek, A., Circle, K., Grants, I., Needleman, B., Wunderlich, J. E., et al. (2009). New bioinformatics approach to analyze gene expressions and signaling pathways reveals unique purine gene dysregulation profiles that distinguish between CD and UC. Inflamm. Bowel Dis. 15, 971–984. doi:10.1002/ibd.20893
Ryzhov, S., Goldstein, A. E., Matafonov, A., Zeng, D., Biaggioni, I., and Feoktistov, I. (2004). Adenosine-activated mast cells induce IgE synthesis by B lymphocytes: an A2B-mediated process involving Th2 cytokines IL-4 and IL-13 with implications for asthma. J. Immunol. 172, 7726–7733. doi:10.4049/jimmunol.172.12.7726
Ryzhov, S., Zaynagetdinov, R., Goldstein, A. E., Novitskiy, S. V., Blackburn, M. R., Biaggioni, I., et al. (2008). Effect of A2B adenosine receptor gene ablation on adenosine-dependent regulation of proinflammatory cytokines. J. Pharmacol. Exp. Ther. 324, 694–700. doi:10.1124/jpet.107.131540
Sadatpour, O., Ebrahimi, M. T., Akhtari, M., Ahmadzadeh, N., Vojdanian, M., Jamshidi, A., et al. (2022). A(2A) adenosine receptor agonist reduced MMP8 expression in healthy M2-like macrophages but not in macrophages from ankylosing spondylitis patients. BMC Musculoskelet. Disord. 23, 908. doi:10.1186/s12891-022-05846-0
Salem, M., Lecka, J., Pelletier, J., Gomes Marconato, D., Dumas, A., Vallieres, L., et al. (2022). NTPDase8 protects mice from intestinal inflammation by limiting P2Y(6) receptor activation: identification of a new pathway of inflammation for the potential treatment of IBD. Gut 71, 43–54. doi:10.1136/gutjnl-2020-320937
Salmon, J. E., and Cronstein, B. N. (1990). Fc gamma receptor-mediated functions in neutrophils are modulated by adenosine receptor occupancy. A1 receptors are stimulatory and A2 receptors are inhibitory. J. Immunol. 145, 2235–2240. doi:10.4049/jimmunol.145.7.2235
Savio, L. E. B., Robson, S. C., and Longhi, M. S. (2020). Ectonucleotidase modulation of lymphocyte function in gut and liver. Front. Cell Dev. Biol. 8, 621760. doi:10.3389/fcell.2020.621760
Schnurr, M., Toy, T., Shin, A., Hartmann, G., Rothenfusser, S., Soellner, J., et al. (2004). Role of adenosine receptors in regulating chemotaxis and cytokine production of plasmacytoid dendritic cells. Blood 103, 1391–1397. doi:10.1182/blood-2003-06-1959
Sharma, A. K., Laubach, V. E., Ramos, S. I., Zhao, Y., Stukenborg, G., Linden, J., et al. (2010). Adenosine A2A receptor activation on CD4+ T lymphocytes and neutrophils attenuates lung ischemia-reperfusion injury. J. Thorac. Cardiovasc Surg. 139, 474–482. doi:10.1016/j.jtcvs.2009.08.033
Sitaraman, S. V., Merlin, D., Wang, L., Wong, M., Gewirtz, A. T., Si-Tahar, M., et al. (2001). Neutrophil-epithelial crosstalk at the intestinal lumenal surface mediated by reciprocal secretion of adenosine and IL-6. J. Clin. Invest. 107, 861–869. doi:10.1172/JCI11783
Sitkovsky, M., Lukashev, D., Deaglio, S., Dwyer, K., Robson, S. C., and Ohta, A. (2008). Adenosine A2A receptor antagonists: blockade of adenosinergic effects and T regulatory cells. Br. J. Pharmacol. 153 (Suppl. 1), S457–S464. doi:10.1038/bjp.2008.23
Skopal, A., Keki, T., Toth, P. A., Csoka, B., Koscso, B., Nemeth, Z. H., et al. (2022). Cathepsin D interacts with adenosine A(2A) receptors in mouse macrophages to modulate cell surface localization and inflammatory signaling. J. Biol. Chem. 298, 101888. doi:10.1016/j.jbc.2022.101888
Spiess, B. D., Sitkovsky, M., Correale, P., Gravenstein, N., Garvan, C., Morey, T. E., et al. (2021). Case report: can inhaled adenosine attenuate COVID-19? Front. Pharmacol. 12, 676577. doi:10.3389/fphar.2021.676577
Subramanian, M., Kini, R., Madasu, M., Ohta, A., Nowak, M., Exley, M., et al. (2014). Extracellular adenosine controls NKT-cell-dependent hepatitis induction. Eur. J. Immunol. 44, 1119–1129. doi:10.1002/eji.201343866
Sun, C. X., Young, H. W., Molina, J. G., Volmer, J. B., Schnermann, J., and Blackburn, M. R. (2005). A protective role for the A1 adenosine receptor in adenosine-dependent pulmonary injury. J. Clin. Invest. 115, 35–43. doi:10.1172/JCI22656
Thiel, M., Caldwell, C. C., and Sitkovsky, M. V. (2003). The critical role of adenosine A2A receptors in downregulation of inflammation and immunity in the pathogenesis of infectious diseases. Microbes Infect. 5, 515–526. doi:10.1016/s1286-4579(03)00068-6
Thiel, M., Chouker, A., Ohta, A., Jackson, E., Caldwell, C., Smith, P., et al. (2005). Oxygenation inhibits the physiological tissue-protecting mechanism and thereby exacerbates acute inflammatory lung injury. PLoS Biol. 3, e174. doi:10.1371/journal.pbio.0030174
Thorn, J. A., and Jarvis, S. M. (1996). Adenosine transporters. Gen. Pharmacol. 27, 613–620. doi:10.1016/0306-3623(95)02053-5
Tian, T., Zhou, Y., Feng, X., Ye, S., Wang, H., Wu, W., et al. (2016). MicroRNA-16 is putatively involved in the NF-κB pathway regulation in ulcerative colitis through adenosine A2a receptor (A2aAR) mRNA targeting. Sci. Rep. 6, 30824. doi:10.1038/srep30824
Tilley, S. L., Tsai, M., Williams, C. M., Wang, Z. S., Erikson, C. J., Galli, S. J., et al. (2003). Identification of A3 receptor- and mast cell-dependent and -independent components of adenosine-mediated airway responsiveness in mice. J. Immunol. 171, 331–337. doi:10.4049/jimmunol.171.1.331
van der Hoeven, D., Wan, T. C., Gizewski, E. T., Kreckler, L. M., Maas, J. E., Van Orman, J., et al. (2011). A role for the low-affinity A2B adenosine receptor in regulating superoxide generation by murine neutrophils. J. Pharmacol. Exp. Ther. 338, 1004–1012. doi:10.1124/jpet.111.181792
Visser, S. S., Theron, A. J., Ramafi, G., Ker, J. A., and Anderson, R. (2000). Apparent involvement of the A(2A) subtype adenosine receptor in the anti-inflammatory interactions of CGS 21680, cyclopentyladenosine, and IB-MECA with human neutrophils. Biochem. Pharmacol. 60, 993–999. doi:10.1016/s0006-2952(00)00414-7
Vuerich, M., Mukherjee, S., Robson, S. C., and Longhi, M. S. (2020). Control of gut inflammation by modulation of purinergic signaling. Front. Immunol. 11, 1882. doi:10.3389/fimmu.2020.01882
Wallace, K. L., and Linden, J. (2010). Adenosine A2A receptors induced on iNKT and NK cells reduce pulmonary inflammation and injury in mice with sickle cell disease. Blood 116, 5010–5020. doi:10.1182/blood-2010-06-290643
Wang, L., Wan, H., Tang, W., Ni, Y., Hou, X., Pan, L., et al. (2018). Critical roles of adenosine A2A receptor in regulating the balance of Treg/Th17 cells in allergic asthma. Clin. Respir. J. 12, 149–157. doi:10.1111/crj.12503
Wei, W., Du, C., Lv, J., Zhao, G., Li, Z., Wu, Z., et al. (2013). Blocking A2B adenosine receptor alleviates pathogenesis of experimental autoimmune encephalomyelitis via inhibition of IL-6 production and Th17 differentiation. J. Immunol. 190, 138–146. doi:10.4049/jimmunol.1103721
Wilson, J. M., Kurtz, C. C., Black, S. G., Ross, W. G., Alam, M. S., Linden, J., et al. (2011). The A2B adenosine receptor promotes Th17 differentiation via stimulation of dendritic cell IL-6. J. Immunol. 186, 6746–6752. doi:10.4049/jimmunol.1100117
Wu, W., He, Y., Feng, X., Ye, S., Wang, H., Tan, W., et al. (2017). MicroRNA-206 is involved in the pathogenesis of ulcerative colitis via regulation of adenosine A3 receptor. Oncotarget 8, 705–721. doi:10.18632/oncotarget.13525
Yamano, K., Inoue, M., Masaki, S., Saki, M., Ichimura, M., and Satoh, M. (2005). Human adenosine A(3) receptor leads to intracellular Ca(2+) mobilization but is insufficient to activate the signaling pathway via phosphoinositide 3-kinase gamma in mice. Biochem. Pharmacol. 70, 1487–1496. doi:10.1016/j.bcp.2005.08.003
Yang, Y., Qiu, Y., Wang, W., Xiao, W., Liang, H., Zhang, C., et al. (2014). Adenosine A2B receptor modulates intestinal barrier function under hypoxic and ischemia/reperfusion conditions. Int. J. Clin. Exp. Pathol. 7, 2006–2018.
Yegutkin, G. G., and Boison, D. (2022). ATP and adenosine metabolism in cancer: exploitation for therapeutic gain. Pharmacol. Rev. 74, 797–822. doi:10.1124/pharmrev.121.000528
Yu, J. C., Lin, G., Field, J. J., and Linden, J. (2018). Induction of antiinflammatory purinergic signaling in activated human iNKT cells. JCI Insight 3, e91954. doi:10.1172/jci.insight.91954
Zhao, Y., LaPar, D. J., Steidle, J., Emaminia, A., Kron, I. L., Ailawadi, G., et al. (2010). Adenosine signaling via the adenosine 2B receptor is involved in bronchiolitis obliterans development. J. Heart Lung Transpl. 29, 1405–1414. doi:10.1016/j.healun.2010.07.005
Keywords: adenosine, inflammatory bowel disease, inflammation, ATP, adenosine receptors
Citation: Vuerich M, Nguyen DH, Ferrari D and Longhi MS (2024) Adenosine-mediated immune responses in inflammatory bowel disease. Front. Cell Dev. Biol. 12:1429736. doi: 10.3389/fcell.2024.1429736
Received: 08 May 2024; Accepted: 16 July 2024;
Published: 12 August 2024.
Edited by:
Lei Shi, Lanzhou University, ChinaReviewed by:
Mireia Martin-Satue, University of Barcelona, SpainStephen Hatfield, Northeastern University, United States
Copyright © 2024 Vuerich, Nguyen, Ferrari and Longhi. This is an open-access article distributed under the terms of the Creative Commons Attribution License (CC BY). The use, distribution or reproduction in other forums is permitted, provided the original author(s) and the copyright owner(s) are credited and that the original publication in this journal is cited, in accordance with accepted academic practice. No use, distribution or reproduction is permitted which does not comply with these terms.
*Correspondence: Davide Ferrari, ZGF2aWRlLmZlcnJhcmlAdW5pZmUuaXQ=; Maria Serena Longhi, bWxvbmdoaUBiaWRtYy5oYXJ2YXJkLmVkdQ==