- 1Department of Orthopaedics, Peking University Third Hospital, Beijing, China
- 2School of Medicine, Nankai University, Tianjin, China
- 3Department of Oncology, Liuzhou People’s Hospital Affiliated to Guangxi Medical University, Liuzhou, China
- 4School of Biomedical Engineering, Tsinghua University, Beijing, China
- 5Arthritis Clinical and Research Center, Peking University People’s Hospital, Beijing, China
- 6Shanxi Key Laboratory of Bone and Soft Tissue Injury Repair, Second Hospital of Shanxi Medical University, Taiyuan, Shanxi, China
Introduction: Our study aims to provide a comprehensive overview of mesenchymal stem cell-derived extracellular vesicles (MSC-EVs) in drug delivery research, focusing on the period between 2013 and 2023. Given the increasing global interest in this field, we utilized bibliometric tools to explore publication trends, key contributors, and thematic research clusters.
Methods: Data was collected from the Web of Science (WoS) database, and an in-depth bibliometric analysis was conducted using VOSviewer. The analysis encompassed bibliographic coupling, co-citation, co-authorship, and co-occurrence trends, offering a structured insight into global research activity. We also employed Citespace to further analyze thematic clusters in this domain.
Results: Our analysis revealed a total of 1,045 publications related to MSC-EVs in drug delivery over the past decade, showing a steady increase in research output. China led in publication count, H-index, prolific authors, and research funding, while the United States ranked highest in total citations, average citation counts, and H-index performance. Pharmaceutics emerged as the leading journal by publication volume, with the Journal of Controlled Release having the strongest total link strength. Top institutions driving research included Shanghai Jiao Tong University, Zhejiang University, and Harvard University. VOSviewer analysis identified four major research clusters: tissue engineering, cancer, neurological diseases, and targeted delivery. Citespace analysis refined this further into ten thematic areas, including differentiation, tissue regeneration, and drug resistance.
Discussion: This bibliometric assessment provides a holistic visualization of the research landscape for MSC-EVs in drug delivery, underlining the significant contributions of China and the United States. Our findings underscore the increasing global importance of MSC-EV research and highlight emerging themes that will likely guide future research directions. The insights from this study offer a foundational framework for identifying nascent frontiers in MSC-EV-based drug delivery.
1 Introduction
Mesenchymal stem cells (MSCs) are multipotent stromal cells that can differentiate into a variety of cell types, including osteoblasts (bone cells), chondrocytes (cartilage cells), adipocytes (fat cells), and myocytes (muscle cells) (Ala, 2023). They are found in various tissues such as bone marrow, adipose tissue, umbilical cord blood, and dental pulp (Wu et al., 2023; Shen et al., 2019; Ma et al., 2024; Kim et al., 2013). MSCs have gained significant attention in regenerative medicine and cell-based therapies due to their immunomodulatory properties, anti-inflammatory effects, and ability to promote tissue repair and regeneration (Porada and Almeida-Porada, 2010; Barry and Murphy, 2013). While MSCs hold considerable promise for tissue regeneration and repair applications, several limitations hinder their therapeutic efficacy. These challenges include poor MSC homing and engraftment at target sites, limited cell survival and retention post-transplantation, immune rejection, variability in potency and phenotype among donor sources, and concerns regarding long-term safety, such as tumorigenesis or ectopic tissue formation (Mansoor et al., 2019). Additionally, the complex interactions between MSCs and the host microenvironment can influence their functional properties and therapeutic outcomes, further complicating their clinical translation.
Increasing evidence suggests that the therapeutic effects of MSCs are largely mediated through paracrine mechanisms, and extracellular vesicles (EVs) (including exosomes, microvesicles, microparticles and apoptotic bodies) have been shown to playing a significant role in this process (Théry et al., 2018). These small membrane-bound vesicles secreted by MSCs contain a diverse cargo of bioactive molecules such as proteins, lipids, and nucleic acids, which exert therapeutic effects on target cells and tissues (Wu et al., 2021). EVs are seen as an alternative approach to stem cell therapy, offering numerous advantages such as enhanced stability, decreased manufacturing expenses, and improved convenience in sterilization and storage (Yuan et al., 2022). EVs facilitate intercellular communication and modulate various physiological processes including inflammation, immune response, angiogenesis, and tissue repair (Tan et al., 2024).
Additionally, MSC-derived EVs (MSC-EVs) are widely utilized as drug carriers in treating various diseases, offering several advantages including enhanced stability, prolonged circulation time, and reduced immunogenicity (Lin et al., 2022). Researchers generally use two methods to load therapeutic agents into extracellular vesicles (EVs): the direct method, where therapeutic agents are inserted into purified exosomes, and the indirect method, where parental cells are genetically modified or co-cultured with a therapeutic agent to produce engineered exosomes. Various therapeutic components, such as drugs, proteins, and RNA, are loaded into MSC-derived EVs, enhancing targeting effects to selectively reach target cells or tissues with minimal systemic side effects. Moreover, their exquisite biocompatibility and ability to cross biological barriers make them attractive candidates for targeted drug delivery. Recently, MSC-EVs have been widely used in bone fracture (Behera et al., 2021), osteoarthritis (Liu et al., 2018), osteoporosis (Yahao and Xinjia, 2021), spinal cord injury (Nakazaki et al., 2021), sciatic nerve injury (Bucan et al., 2019), intervertebral disc degeneration (Hu et al., 2021), ischemic stroke (Zhang et al., 2021), traumatic brain injury (Moore et al., 2019), Alzheimer’s disease (Chen et al., 2021), Parkinson’s disease (Huang et al., 2022) and etc., Nevertheless, there is inadequate research examining the quantitative and qualitative attributes of global trends and focal points in MSC-EVs for drug delivery. Therefore, it is imperative to assess the present state and trajectories of MSC-EVs in drug delivery, as this can anticipate promising areas of interest and avenues for advancement in this domain.
As the cornerstone of scientific inquiry, publications serve as a crucial barometer of research trends and contributions. Leveraging the characteristics of literature metrology and comprehensive literature databases, bibliometric analysis offers both qualitative and quantitative insights into the trajectory of research endeavors over time. Through this analytical approach, one can forecast the evolution of a specific field by examining the contributions of authors, journals, institutes, countries, and regions. Moreover, bibliometric analysis plays a pivotal role in informing the formulation of clinical policies and the establishment of guidelines. Currently, this viable methodology has been effectively employed in appraising research trends within nanomedicine (Wang et al., 2024), depressive disease (Liu et al., 2024), tumor (Song et al., 2023), and osteoarthritis (Yang Z. et al., 2022) and so on. While we conducted a prior examination of global research trends in EVs derived from stem cells spanning from 1991 to 2021, and Zhang et al. provided a review of current research on MSC-EVs from 2009 to 2021, neither study comprehensively nor promptly delved into the realm of MSC-EVs within the field of drug delivery (Zhang X. et al., 2022). The objective of our study was to assess the contemporary status and worldwide trends pertaining to the utilization of MSC-EVs in drug delivery.
2 Materials and methods
2.1 Search strategy and data collection
All publications sourced from Web of Science (WoS), encompassing Science Citation Index Expanded (SCIE), Arts and Humanities Citation Index, Social Sciences Citation Index, Conference Proceedings Citation Index-Science, Emerging Sources Citation Index, Conference Proceedings Citation Index-Social Science and Humanities, Current Chemical Reactions, and Index Chemicus, constituting a repository of over 12,000 international academic journals, represent one of the most comprehensive and authoritative database platforms for accessing global academic information (Lin et al., 2022). These publications underwent bibliometric analysis in accordance with our prior studies (Yang Z. et al., 2022; Yang et al., 2023a; Yang et al., 2023b).
All published papers were gathered from WoS, with the database’s expiration date set to 9 February 2024. The search criteria included the following terms: “mesenchymal stem cell” OR “mesenchymal stem cells” AND “extracellular vesicles” OR “exosomes” OR “microvesicles” OR “microparticles” OR “ectosomes” OR “oncosomes” OR “apoptotic bodies” AND “drug delivery,” limited to publications in English and categorized as either articles or reviews. The search period was defined as 1 January 2013, to 31 December 2023. Additionally, the detailed information regarding specific countries or regions was refined by indexing them within the WoS. The inclusion criteria stipulated that manuscripts must focus on the theme of MSC-EVs for drug delivery, be written in English, and categorized as either articles or reviews. Exclusion criteria included themes unrelated to MSC-EVs for drug delivery and article types such as briefings, news, and meeting abstracts. For in vivo studies, the search strategy was further refined by adding the term “AND in vivo” to the above search criteria. This resulted in a total of 286 published papers specifically focusing on the in vivo applications of MSC-EVs for drug delivery. The same inclusion and exclusion criteria were applied to ensure that only relevant articles or reviews were included in this subset of the analysis.
All publication records, comprising publication year, title, authors’ names, affiliations, nationalities, abstracts, keywords, and journal names, were extracted from the WoS database and saved as.txt files. These files were subsequently imported into Excel 2021. Coauthors (TZ and YM) independently reviewed and extracted data from these publications. Any discrepancies were resolved through discussion with experts to achieve consensus. Finally, all authors independently cleaned and analyzed the data using GraphPad Prism 8.
2.2 Bibliometric analysis and visualization
The intrinsic functions of WoSCC were utilized to characterize the fundamental features of eligible studies. Specifically, the publication frequency and citation counts were analyzed and visualized. GraphPad Prism 8 and Origin 8 were employed for the bibliometric analysis. Initially, the year was plotted on the x-axis and the number of documents published each year was plotted on the y-axis to examine the trend of publication output over time. Relative research interest (RRI), defined as the number of publications in a specific field per year relative to all field literature, was calculated (Shah et al., 2022). Utilizing a combination of R software, including Python, NumPy, SciPy, and Matplotlib, a world map was generated to visualize publication trends. Then a trend chart depicting the annual publication volume of the top ten countries is generated by using Origin 8 software. Additionally, the H-index, which denotes a researcher who has published H papers and has been cited at least H times, was computed to assess the impact of scientific research (Hu et al., 2022).
In this study, we employed VOSviewer software from Leiden University in the Netherlands to comprehensively visualize bibliometric networks of the publications. We conducted detailed analyses of bibliographic coupling, co-citation, and co-occurrence, and presented the results using VOSviewer. The key parameter settings mainly focused on determining the minimum number of documents, citations, or occurrences. Specifically, for bibliographic coupling analysis, we set the minimum number of documents for Country, Journal, Author, and Institution at more than 2. For co-citation analysis, we defined the minimum number of citations for Author, Reference, and Journal as more than 10. Regarding co-authorship analysis, we established the minimum number of documents for Country, Author, and Institution to be more than 2. Additionally, for co-occurrence analysis, keywords were identified as words appearing more than 2 times in titles/abstracts across all papers.
Furthermore, CiteSpace (6.2.R6) was employed to construct a dual-map overlay for journals, visualize diagrams of country/regional collaboration, institutional collaboration, and author collaboration, conduct cluster analysis of co-cited keywords, and identify references and keywords with intense citation bursts. This software, developed by Professor Chen C., was configured with the following parameters: link retaining factor (LRF = 3), look back years (LBY = 5), e for top N (e = 1), time span (2013–2023), years per slice (1), links (strength: Cosine, scope: Within slices), selection criteria (g-index: k = 10), and minimum duration (MD = 2 for keywords; MD = 5 for references).
The bibliometric analysis in this study was mainly conducted using VOSviewer and CiteSpace software. No custom code was used in this process. The parameters and settings applied in both tools are available upon request. Raw data and generated outputs can also be provided for further validation and reproducibility purposes.
3 Results
3.1 Trend of global publications
In the realm of global scholarly output, a comprehensive review of literature from 2013 to 2023 yielded 1,045 publications aligning with the established search parameters. Subsequent refinement of this corpus involved the exclusion of editorial materials (1), processing papers (1), letters (1), and corrections (1), along with the removal of two non-English studies. This meticulous process resulted in the identification of 1,039 pertinent studies, as depicted in Figure 1. Analyzing the temporal progression from 2013 to 2023, a consistent upward trajectory in global publication volume is delineated. The annual count of publications experienced a notable increase, escalating from 11 in 2013 to 268 in 2023. Particularly in the last 5 years (2019–2023), this ascending trend in publication volume has intensified, as illustrated in Figure 2A. Furthermore, the analysis of contributions by countries and regions revealed a diverse international involvement, with 73 countries/regions contributing to the literature in this field, as shown in Figure 2B. Among these, the top 10 countries/regions displayed a significant predominance in publication output. China led with a remarkable contribution of 445 papers, accounting for 43.8% of total publications, followed by the United States (207, 20.4%), Iran (96, 9.4%), Italy (63, 6.2%), South Korea (53, 5.2%), and India (51, 5%), as detailed in Figures 2C, D.
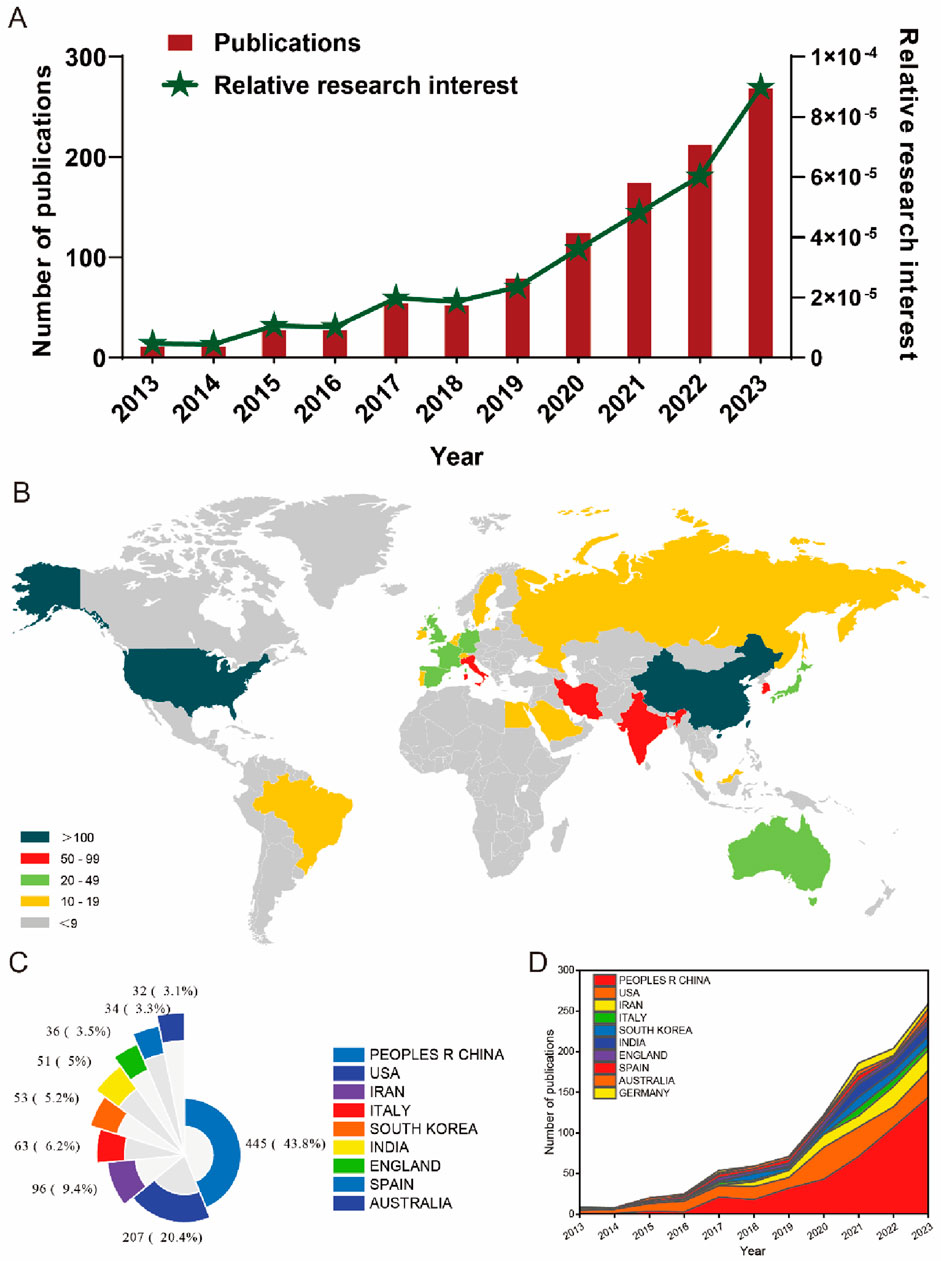
Figure 2. Global trends and the contributions of countries/regions to the research field of MSC-EVs for drug delivery from 2013 to 2023. (A) The yearly count of publications related to MSC-EVs for drug delivery from 2003 to 2023. (B) A world map illustrating the distribution of MSC-EVs for drug delivery from 2003 to 2023. The total (C) and yearly (D) number of publications in the top 10 most productive countries from 2013 to 2023 are analyzed.
3.2 Quality of publications of different countries/regions
In the context of total citation frequency analysis, publications originating from the United States demonstrated the highest aggregate citation count, amassing a total of 16,800 citations. China occupied the second position with an impressive citation tally of 15,489, succeeded by England with 4,118 citations, Iran with 3,028, and Italy with 2,329, as delineated in Figure 3A. Moreover, when examining the average citation frequency, publications from England emerged as the frontrunner with an average citation frequency of 114.39. The United States followed closely, ranking second with an average citation frequency of 81.16. Australia (50.06), Spain (30.15), and Germany (37.61) also featured prominently in this metric, as highlighted in Figure 3B. Additionally, in terms of the H-index, a key indicator of research impact, publications from China led with the highest H-index of 61. The United States followed with an H-index of 56, Iran with 31, Italy with 25, and South Korea with 22, underscoring the significant research impact of these countries in the domain, as illustrated in Figure 3C.
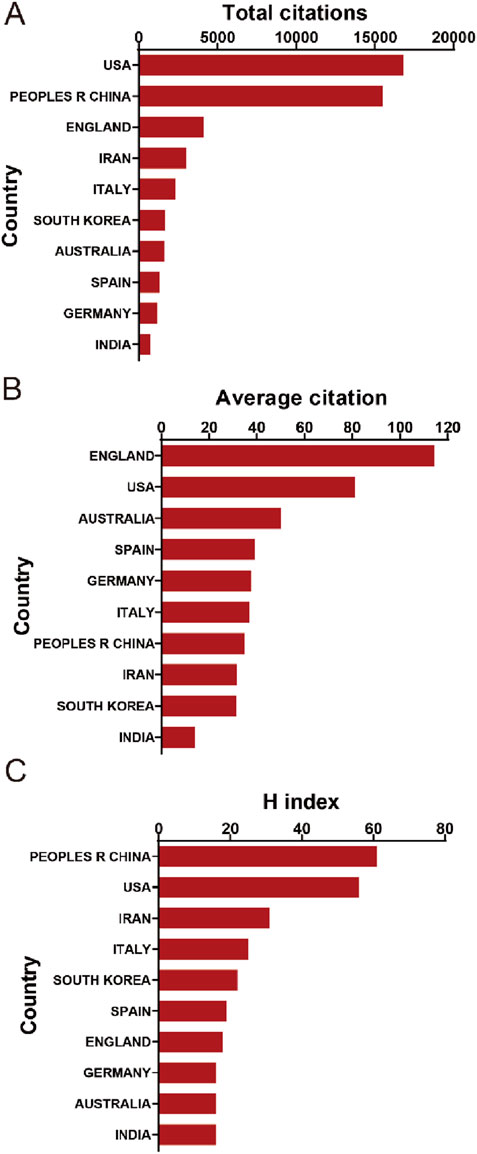
Figure 3. (A) The top 10 countries/regions in terms of total citations regarding MSC-EVs for drug delivery from 2013 to 2023. (B) The top 10 countries/regions in terms of the average citations per publication related to MSC-EVs for drug delivery from 2003 to 2023. (C) The top 10 countries/regions in terms of the publication H-index related to MSC-EVs for drug delivery from 2013 to 2023.
3.3 Analysis of country/regional and institution collaboration
In this comprehensive analysis, a global collaboration network was meticulously examined, focusing on publications with a minimum threshold of three documents per country. This scrutiny, conducted through VOS viewer and depicted in Figure 4A, involved publications from 42 countries. China emerged as a dominant force, showcasing the highest output area and robust international collaboration, as evidenced by its pronounced interactions with other nations. The five foremost countries, in terms of total link strength, were China (524,072 times), the United States (254,718 times), Iran (141,744 times), India (109,942 times), and South Korea (105,309 times).
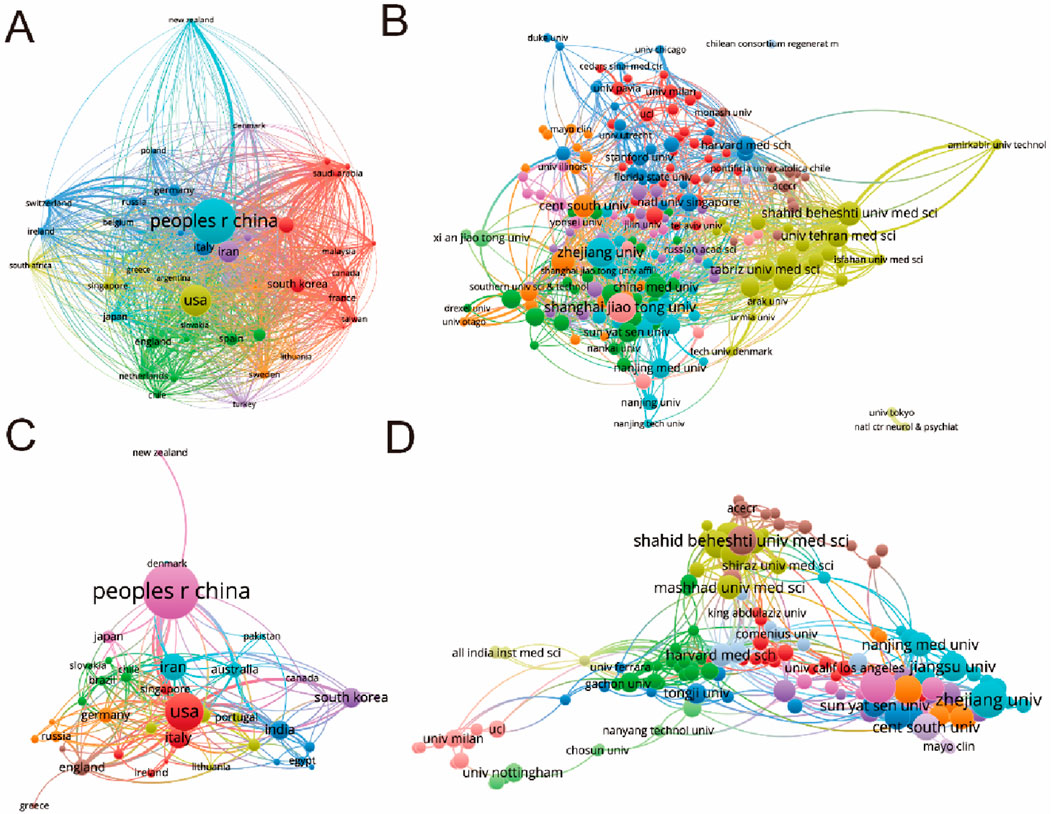
Figure 4. Mapping of countries/regions and institutions associated with MSC-EVs for drug delivery spanning from 2013 to 2023. (A) Examination of country/regional collaboration utilizing Vosviewer. (B) Analysis of institutional collaboration using Vosviewer. (C) Authorship-country collaboration analysis via Vosviewer. (D) Authorship-institution collaboration analysis via Vosviewer. Nodes represent countries/regions and institutions, varying in size based on the number of publications attributed to each. Connecting lines denote cooperation, with line thickness indicating collaboration strength; thicker lines denote closer cooperation.
Further analysis, delineated in Figure 4B, was directed towards academic institutions, with a focus on those with a minimum of three documents and a maximum of 25 organizations per document. This investigation encompassed 216 institutions, again employing VOS viewer for analytical insights. The institutions demonstrating the greatest total link strength were Zhejiang University (49,430 times), Shanghai Jiao Tong University (44,068 times), Sichuan University (43,342 times), Tehran University of Medical Sciences (39,876 times), and Jiangsu University (34,521 times).
Additionally, a comprehensive summary of funding sources for stem cell research in meniscal regeneration is presented in Table 1. The National Natural Science Foundation of China leads with support for 283 articles, followed by the United States Department of Health and Human Services (84 articles) and the National Institutes of Health (NIH), United States (83 articles). Notably, the top three funding sources corroborate the earlier findings, highlighting the significant contributions from both China and the United States in this research domain.
3.4 Co-authorship analysis of country, and institution
The co-authorship analysis, a critical component of understanding collaborative patterns in research, was conducted on a select group of countries and institutions. This analysis employed VOS viewer as the primary tool for visualization and assessment. Regarding the country-based co-authorship analysis, 32 countries, each contributing more than three papers, were selected for scrutiny. The results of this analysis are encapsulated in Figure 4C. The United States emerged as the leader in co-authorship strength, boasting a total link strength of 152 times. China followed with a total link strength of 93 times. Other notable countries included Iran (58 times), England (42 times), India (42 times), and Italy (also at 42 times), all demonstrating significant collaborative linkages in the research community. The institutional co-authorship analysis extended to 216 institutions, each with more than three documents to their credit. The findings, illustrated in Figure 4D, identified Shahid Beheshti University of Medical Sciences as having the highest total link strength at 49 times. Harvard Medical School followed closely with a strength of 46 times. Other institutions in the top five included Tehran University of Medical Sciences (44 times), Massachusetts General Hospital (42 times), and Shanghai Jiao Tong University (32 times). This comprehensive co-authorship analysis at both country and institutional levels underscores the interrelation and collaborative efforts in the field, highlighting key players and the strength of their scientific networks.
3.5 Analysis of authors collaboration
The investigation into authorship productivity, encompassing publications from 118 authors, revealed a remarkable level of output. Figure 5A illustrates these findings, with the most prolific authors being Qian, Hui (total link strength = 11,816 times), Shi, Hui (11,176 times), Xu, Wenrong (9,930 times), Liu, Yang (9,011 times), and Schiffelers, Raymond, M (8,835 times). This analysis highlights the individual contributions that have significantly shaped the research landscape in this field.
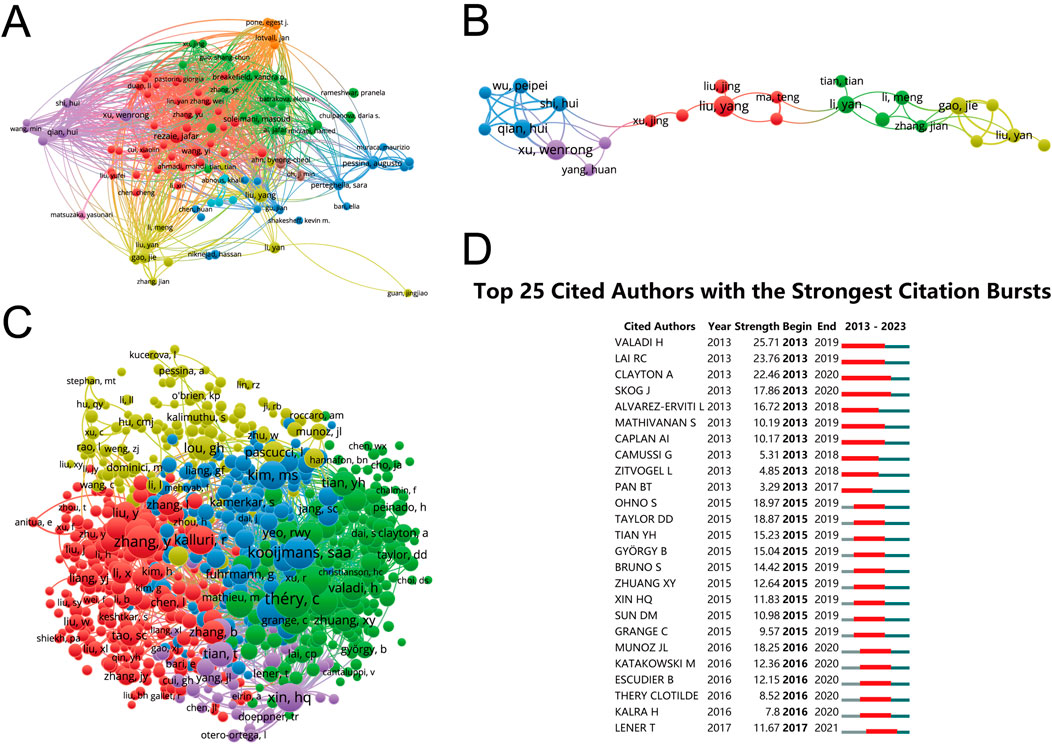
Figure 5. Network visualization of author collaboration analysis concerning MSC-EVs for drug delivery spanning from 2003 to 2023. (A) Author collaboration analysis conducted using Vosviewer. (B) Visualization diagram illustrating authorship-author analysis based on Vosviewer. (C) Network visualization diagram illustrating cocited-author analysis based on Vosviewer. (D) Identification of the top 25 cited authors exhibiting the most pronounced citation bursts in publications related to MSC-EVs for drug delivery. Author collaborations are depicted by nodes, with the size of each node scaling in accordance with the number of collaborations. Collaboration relationships are represented by connecting lines between nodes.
The co-authorship analysis, involving 118 authors with over 3 documents each, focused on the collaborative networks within the research community. As shown in Figure 5B, the top collaborators were identified based on their total link strength: Pessina, Augusto (20 times), Pascucci, Luisa (19 times), Qian, Hui (19 times), Shi, Hui (17 times), and Alessandri, Giulio (16 times). This analysis underscores the importance of collaborative endeavors in advancing research.
Co-citation analysis, which included 915 authors with a minimum of 20 documents, was conducted to understand the inter-relationships based on citation frequency. The findings in Figure 5C point to the most co-cited authors: Thery, C (total link strength = 23,454 times), Kooijmans, Saa (21,191 times), Haney, Mj (18,839 times), Alvarez-Erviti, I (16,181 times), and Kim, Ms (14,756 times). This aspect of the analysis sheds light on the influential works and authors within the field, reflecting their impact and relevance.
The citation burst analysis, summarized in Figure 5D, identified key publications that experienced a surge in citations over a specific period, indicating a spike in interest and relevance in the field. The study by Vakadi H exhibited the strongest citation burst (strength of 25.71) from 2013 to 2019, followed by Lai RC (23.76) and Clayton A (22.46). This analysis provides insights into the evolving trends and pivotal studies driving research focus over time.
3.6 Analysis of research areas and journals
In the realm of academic publishing, the 10 most prolific journals contributing to this study have been systematically chronicled in Table 2. An intricate dual-map overlay, delineating the journals associated with MSC-EVs for drug delivery, has been meticulously constructed and is presented in Figures 6A, B. Pharmaceutics leads the cohort with a commendable tally of 37 publications, followed closely by the Journal of Controlled Release with 35 publications, International Journal of Molecular Sciences with 34, Frontiers in Bioengineering and Biotechnology with 27, and International Journal of Nanomedicine contributing 19 articles.
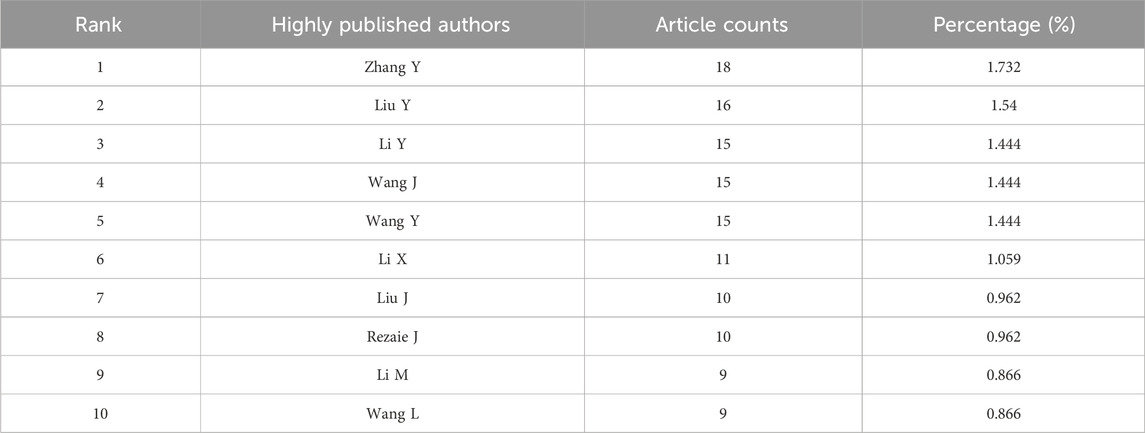
Table 2. The top 10 authors with the most publications on MSC-EVs for drug delivery from 2013 to 2023.
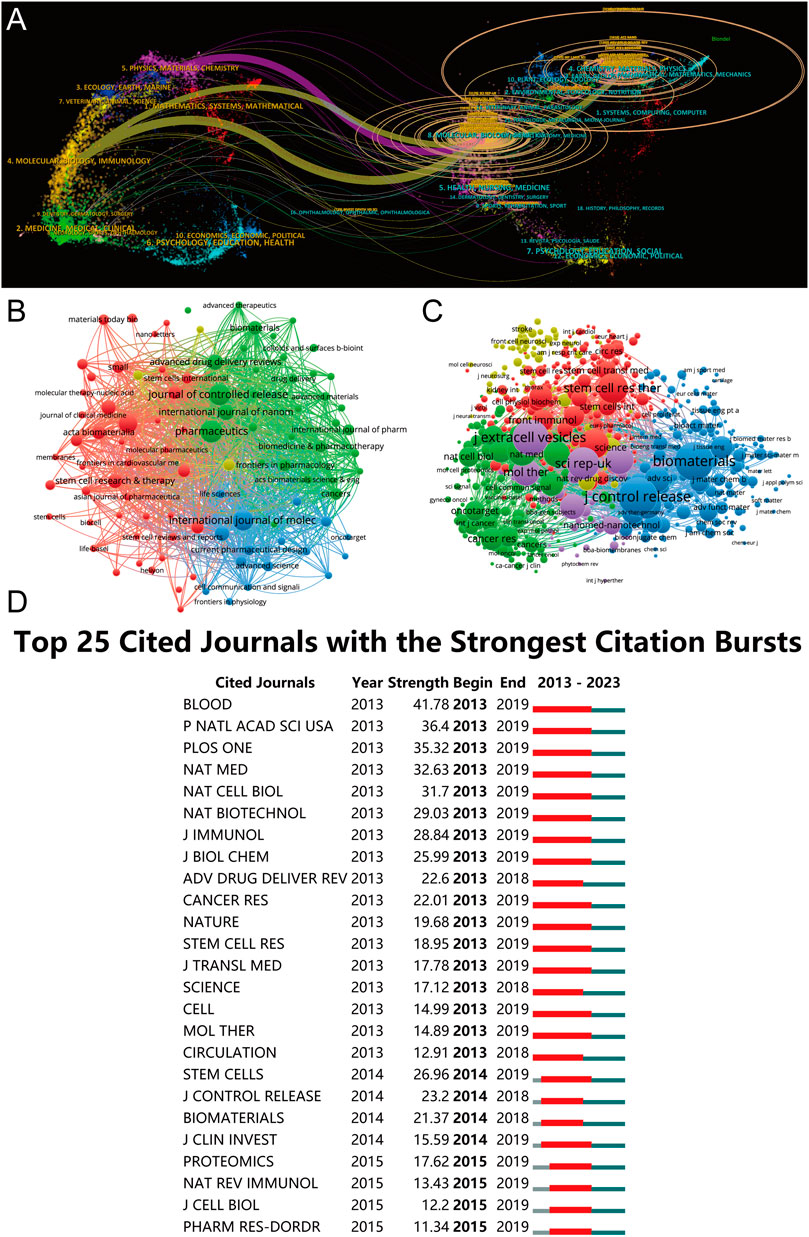
Figure 6. Articles published in various journals on MSC-EVs for drug delivery from 2013 to 2023. (A) Dual-map overlay depicting journals relevant to MSC-EVs for drug delivery. (B) Bibliographic analysis of journals utilizing VOSviewer. (C) Network map illustrating journals that were co-cited based on VOSviewer. (D) Identification of the top 25 cited journals exhibiting the most pronounced citation bursts in publications.
A thorough co-citation analysis of journals, employing VOSviewer and adhering to a criterion that excludes journals with fewer than 20 citations, yielded significant insights. As elucidated in Figure 6C, this analysis encapsulated 832 journals in total link strength. The forefront of this collection is dominated by five journals: the Journal of Controlled Release (3,986 citations), Biomaterials (2,934 citations), Journal of Extracellular Vesicles (2,536 citations), Scientific Reports (2,118 citations), and Stem Cell Research and Therapy (1,810 citations), each demonstrating substantial citation influence.
In the context of citation bursts over the past decade, CiteSpace analysis highlighted 25 journals, as depicted in Figure 6D. This analysis underscores the journals that garnered heightened scholarly attention within specific timeframes. Notably, the journal Blood exhibited the most pronounced citation burst, with an impressive strength of 41.78, spanning from 2013 to 2019.
Furthermore, a comprehensive enumeration of research orientations is collated in Table 3, utilizing VOSviewer for precision. The predominant research fields, as revealed, encompass pharmacology and pharmacy, materials science, science and technology in other topics, cell biology, and chemistry. These focal areas not only reflect the current research milieu but also hint at future trajectories and prospective avenues in the field.
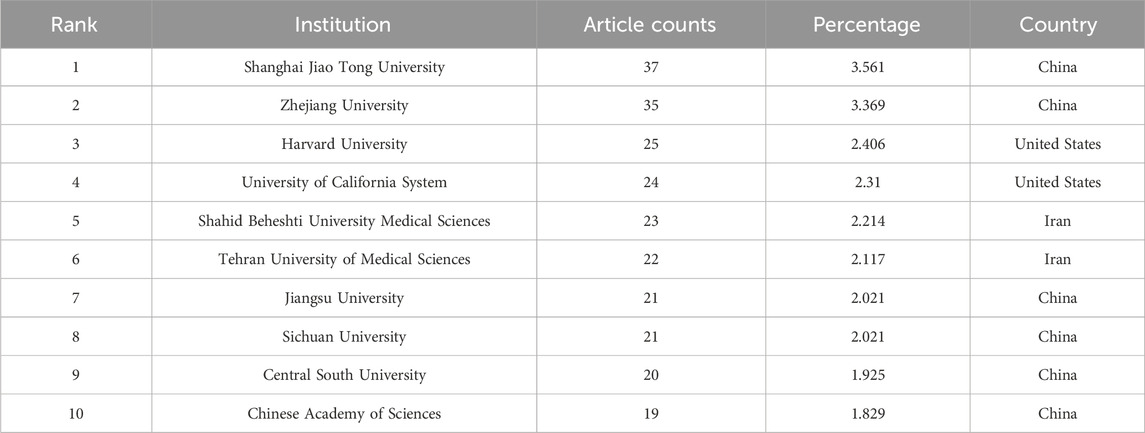
Table 3. The top 10 institutions published literature related to MSC-EVs for drug delivery from 2013 to 2023.
3.7 Analysis of references
In an effort to illuminate the landscape of seminal literature within the field, a sophisticated co-citation analysis was conducted using VOSviewer, as depicted in Figure 7A. This analysis, aimed at unraveling the intricate web of scholarly interrelations based on aggregate citation metrics, scrutinized 575 references, each surpassing the threshold of being cited in over 20 documents. The quintet of articles at the apex of this evaluation, distinguished bFy their formidable total link strength, includes Alvarez-Erviti L’s 2011 publication in “Nature Biotechnology” (291 times), Haney MJ’s 2015 work in “Journal of Controlled Release” (241 times), Théry C’s 2018 article in “Journal of Extracellular Vesicles” (212 times), Tian YH’s 2014 study in “Biomaterials” (191 times), and Kalluri R’s 2020 research in “Science” (also 191 times). Complementing this, the concept of ‘citation burst’, a critical indicator highlighting references that have sparked considerable interest over specific periods, was meticulously analyzed using CiteSpace. This analysis culminated in the identification of the most potent citation bursts, with Haney MJ’s study leading the pack, exhibiting a burst strength of 37.26 from 2016 to 2020, followed by Tian YH and Pascucci L, consolidating the understanding of trends and focal points in the domain’s research trajectory as summarized in Figure 7B.
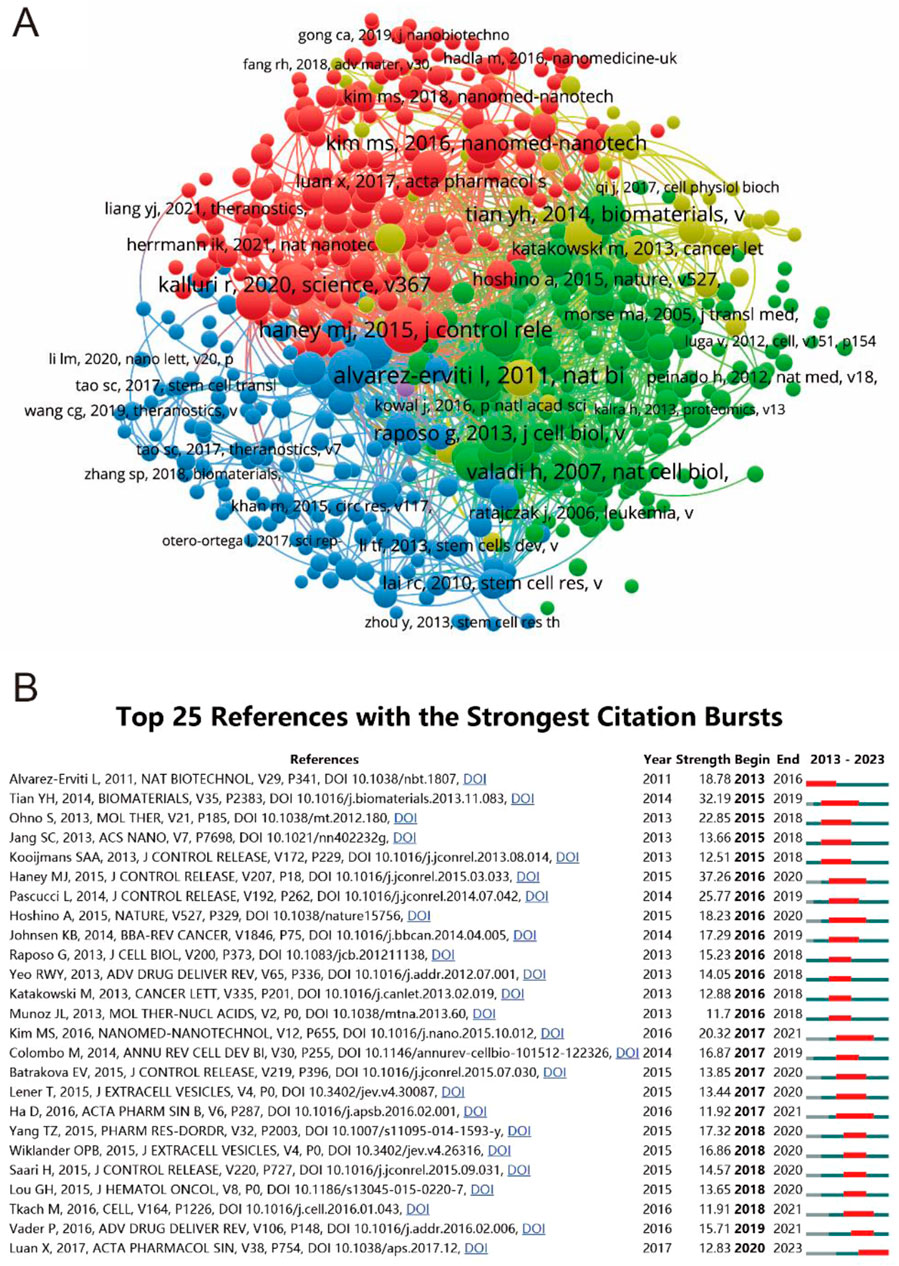
Figure 7. Mapping of references in studies on MSC-EVs for drug delivery spanning from 2013 to 2023. (A) Network visualization of reference analysis using VOSviewer. (B) Identification of the top 25 references exhibiting the most pronounced citation bursts in publications.
3.8 Co-occurrence analysis of keywords
The co-occurrence analysis undertaken in this study was pivotal in elucidating the prevailing research trajectories and focal areas in scientific inquiry. This analysis, instrumental in charting the course of research evolution, leveraged keywords that appeared more than 10 times in titles and abstracts across all papers. Analyzed through VOS viewer, as depicted in Figure 8A, a total of 741 keywords were discerned and primarily categorized into four distinct clusters: Cluster 1 embodies tissue engineering-related research (red), Cluster 2 encompasses cancer-related research (green), Cluster 3 covers neurological-related diseases research (yellow), and Cluster 4 pertains to targeted delivery (blue). This categorization not only highlights the salient research themes in MSC-EVs for drug delivery but also underscores the diversity and breadth of the field.
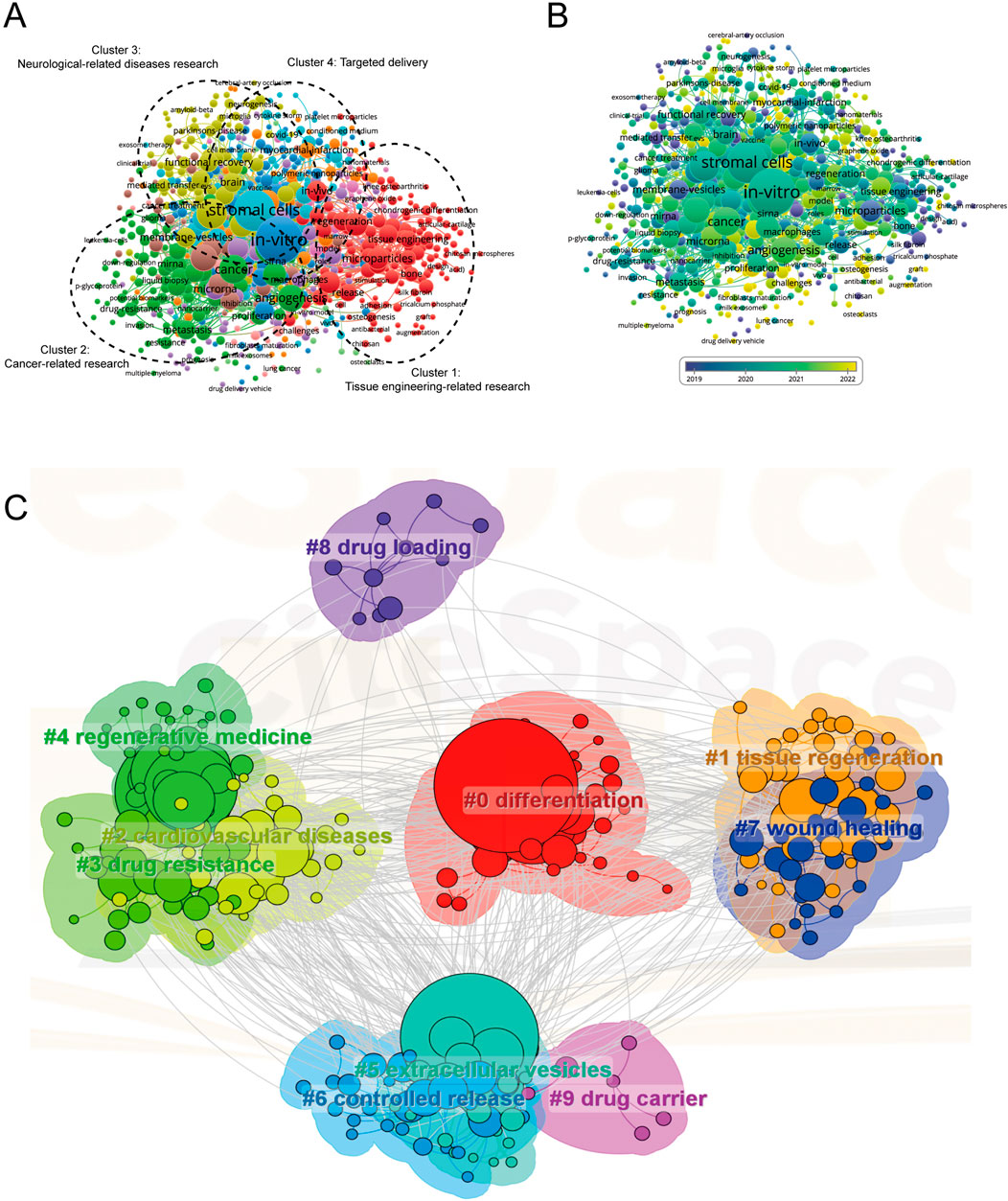
Figure 8. Mapping of keywords in studies on MSC-EVs for drug delivery spanning from 2013 to 2023. (A) Utilization of VOSviewer for network visualization of keywords, with point size representing frequency. Cluster 1 (red): research related to tissue engineering; Cluster 2 (green): cancer-related research; Cluster 3 (yellow): studies on neurological diseases; Cluster 4 (blue): research on targeted delivery. (B) Distribution of keywords based on mean frequency of appearance; yellow keywords appeared later than blue ones. (C) Visualization of keyword clustering from 2013 to 2023.
In the tissue engineering-related cluster, prominent keywords included regenerative medicine, biomaterials, and microparticles. The cancer-related research cluster predominantly featured keywords like angiogenesis, tumor microenvironment, and metastasis. The neurological-related diseases research cluster was characterized by keywords such as mesenchymal stromal cells, functional recovery, and drug-delivery vehicles. Lastly, the targeted delivery cluster was marked by keywords like cell-derived exosomes, stromal cells, and in-vitro. VOS viewer further color-coded the keywords based on their average occurrence in published papers (Figure 8B), with blue signifying earlier appearances and yellow indicating more recent emergence. This color coding revealed that the trends in the four clusters have remained relatively stable, suggesting a balanced and ongoing interest across these research domains in the foreseeable future. A network map, as shown in Figure 8C, was constructed to visualize these keyword clusters, with each node representing a significant keyword. The top ten keywords, in order of prominence, were #0 differentiation, #1 tissue regeneration, #2 cardiovascular diseases, #3 drug resistance, #4 regenerative medicine, #5 extracellular vesicles, #6 controlled release, #7 wound healing, #8 drug loading, #9 drug carrier.
The analysis extended to examining the transformation of keywords over the decade from 2013 to 2023, with a visualization in Figure 9A. This timeline revealed notable variations in keyword frequency over time, signifying shifts in research focus. Furthermore, using CiteSpace’s burst detection algorithm, with a minimum burst duration set at 5 years, the top 25 keywords with the strongest citation bursts were identified, as shown in Figure 9B. The blue lines in the graph represent the keyword timelines, with red segments indicating the burst duration. The keyword ‘biodistribution’ emerged as the most intense (strength = 6.17), closely followed by ‘progenitor cells’ (6.12) and ‘tumor-derived exosomes’ (5.69). Remarkably, ‘membrane vesicles’ had the longest burst duration, spanning 23 years from 1,013 to 2,020, reflecting its enduring relevance in the field.
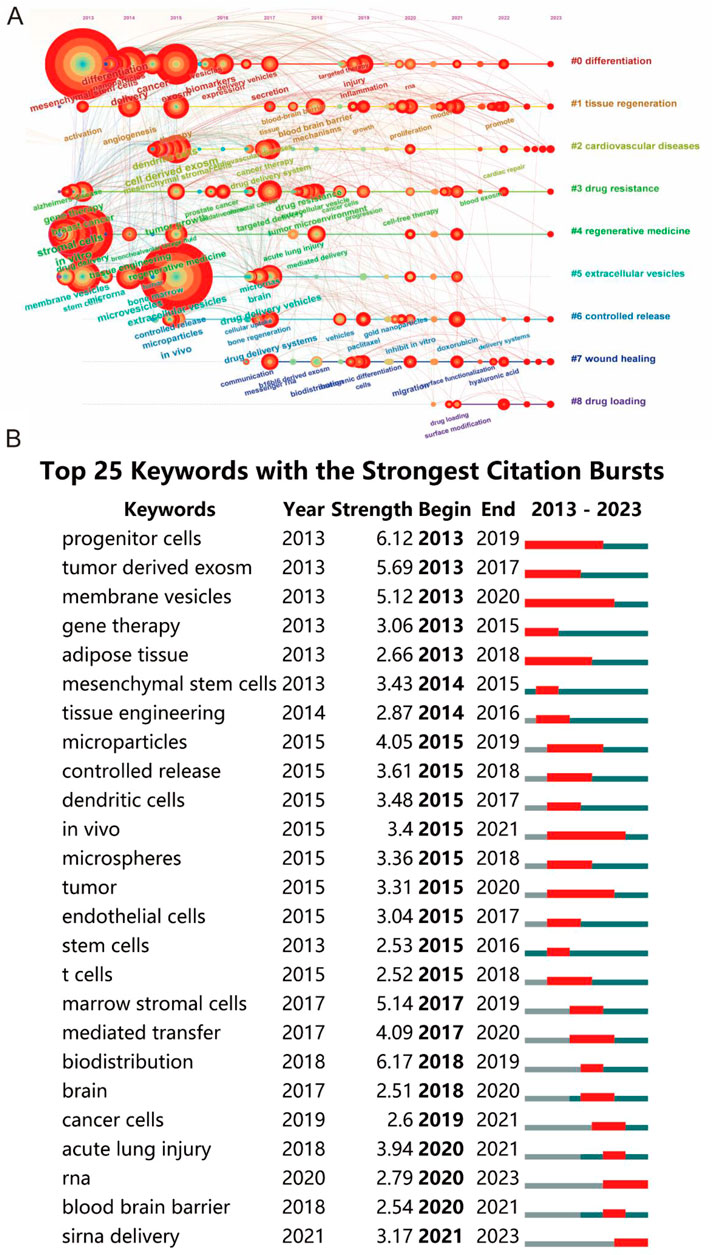
Figure 9. (A) Visualization of the timeline for keywords spanning from 2013 to 2023. (B) Identification of the top 25 keywords exhibiting the most pronounced citation bursts in publications.
3.9 Trends and hotpots of in vivo study on MSC-EVs delivery system
As the therapeutic applications of MSC-EVs continue to expand, understanding the key research trends and hotspots within in vivo studies is essential for guiding future investigations. A comprehensive analysis of the literature reveals critical areas of focus that are shaping the development of MSC-EV delivery systems for clinical use. In Figure 10A, the publication count and relative research interest indicate a significant upward trend in the number of studies on in vivo delivery of MSC-derived exosomes, particularly in the past 5 years. This rise reflects the growing recognition of MSC-derived exosomes as promising candidates for drug delivery and regenerative therapies. The corresponding increase in RRI further emphasizes the burgeoning interest within the scientific community. Figure 10B presents a co-occurrence network of keywords generated by VOSviewer. The network reveals key themes such as “exosome delivery,” “regenerative medicine,” “tumor microenvironment,” and “drug delivery systems.” These themes suggest that current research is heavily focused on leveraging MSC-derived exosomes for tissue repair, cancer treatment, and targeted therapeutic delivery. In Figure 10C, the CiteSpace clustering analysis identifies distinct research areas, including stem cell therapy, targeted delivery, and cancer therapy. This analysis illustrates the breadth of applications explored within MSC-derived exosome in vivo studies, ranging from regenerative medicine to oncology. Figure 10D highlights the top 20 keywords with the strongest citation bursts, indicating specific topics that have gained significant attention in recent years. Keywords like “tumor-derived exosomes,” “angiogenesis,” and “nanoparticle delivery” are among the strongest citation bursts, signaling the importance of targeted exosome delivery systems and their role in modifying the tumor microenvironment.
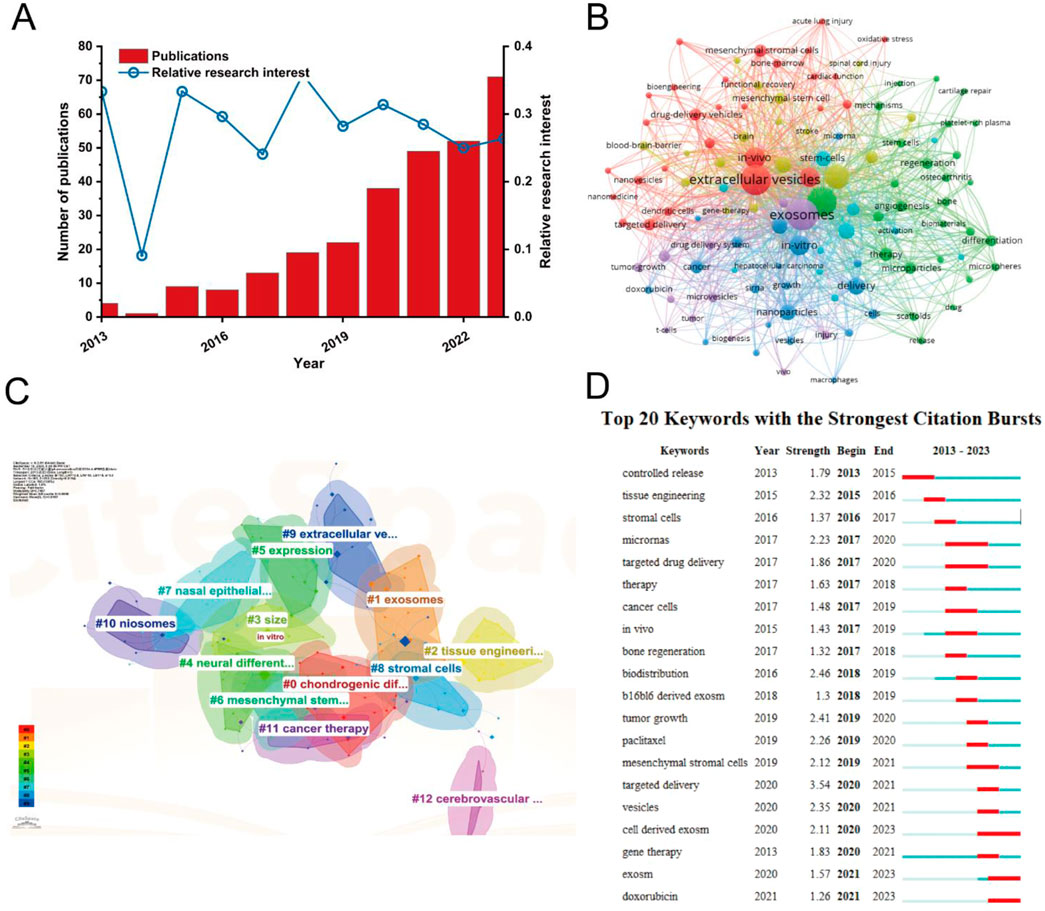
Figure 10. Analysis of in vivo studies on MSC-derived exosome delivery trends and research hotspots. (A) Publication count and relative research interest over time. (B) Co-occurrence network of keywords generated by VOSviewer. (C) Keyword clustering analysis using CiteSpace. (D) Top 20 keywords with the strongest citation bursts.
4 Discussions
4.1 Trend of global publications
MSCs have garnered significant attention in both basic and clinical research due to their potential in treating various diseases, as highlighted by Blau and Daley (Blau and Daley, 2019). However, challenges such as the complexities of long-term ex vivo culture, increased immunogenicity in differentiated cells, and limited therapeutic efficacy have been identified by Musiał-Wysocka et al. (Musiał-Wysocka et al., 2019), constraining their broader application. These limitations have catalyzed the exploration of alternative, cell-free therapies. Among these, EVs have emerged as a promising substitute. EVs, including diverse forms like exosomes, microvesicles, and apoptotic bodies, are cell-derived nanoparticles capable of mirroring certain cellular functions. Their advantages over traditional MSC therapies include comparable therapeutic effects, reduced immunogenicity, enhanced safety, minimal side effects, effective protection of therapeutic agents, simpler preservation, fewer ethical concerns, and the ability to traverse biological barriers, as detailed by Nguyen et al. (2021).
The field of EVs, particularly in the context of MSCs, has witnessed a surge in research activity. This is exemplified by the comprehensive reviews and studies conducted by various researchers. Wang et al. (2020) provided an insightful overview of global trends and developments in the exosome domain, a key component of EVs, from 1994 to 2017. Furthermore, Zhang X. et al. (2022) delved into MSC-EVs, uncovering a steep growth trend in research focused on their therapeutic effects and mechanisms. Their work also forecasts the future direction of this field, anticipating an increase in multidisciplinary integration and emphasis on senescence-related topics. MSCs, known for their multi-directional differentiation and self-renewal capabilities, are a subset of adult stem cells originating from the mesoderm (Li and Hua, 2017). Yet, a comprehensive global analysis and visualization of EVs derived not just from MSCs, but from a broader spectrum of stem cells, remains an area less explored.
To bridge this gap, our study employed bibliometric and visualized analysis techniques to encapsulate the current status and project future trends in the realm of stem cell-based EV research. Our findings reveal a marked increase in the volume of publications per year in this domain, alongside a significant rise in Research Relative Index (RRI) values, indicating a growing interest and investment in this field. Our analysis also showed that this research is a global endeavor, with contributions from approximately 73 countries and 1,479 institutions. Notably, China and the United States are leading this research front, collectively accounting for over half of the publications. Such a concentrated effort in MSC-derived EV research underscores its status as a burgeoning field of study. Given the increasing evidence of the paracrine effects of mesenchymal stem cells mediated through EV secretion (Baglio et al., 2012) and their observed intercellular communication capabilities under various conditions (Chang et al., 2018), it is reasonable to anticipate a continued escalation in detailed studies on stem cell-based EVs, guiding researchers towards high-caliber, innovative investigations.
4.2 Quality and status of global publications
To elucidate the academic impact and quality of research contributions from various countries in the field of EVs derived from MSCs, an in-depth analysis was conducted focusing on total citations and H-index, with results showcased in Figure 3. Despite China leading in publication volume with 445 papers, the United States, with 207 publications, boasts the highest total citation count of 16,800. The H-index comparison reveals a marginal disparity between China and the United States, signifying that both nations maintain a prominent research profile, with the United States garnering more extensive global attention. Intriguingly, England excels in average citations, surpassing the United States and Australia, suggesting the pivotal role of the United States in this specialized domain. Moreover, China’s ascendancy in both publication count and H-index, a significant leap from its fifth rank in 2019 (Zhang H. et al., 2022), underscores its growing global recognition in this research area. This balance of quantity and quality in Chinese publications may be attributed to recent shifts in the Chinese Academic Evaluation Systems (CAESs) towards a dual focus (Synnestvedt et al., 2005). Nevertheless, China still faces challenges in elevating the quality of its research output, a goal potentially achievable through CAES reforms and increased research funding (Xing et al., 2018).
An investigation into the journals associated with these publications, as depicted in Figure 6, reveals Pharmaceutics, Journal of Controlled Release, International Journal of Molecular Sciences, Frontiers in Bioengineering and Biotechnology, and International Journal of Nanomedicine as the leading publishers in this sphere. The close publication counts among these journals suggest they could be key venues for future research (Table 3). Furthermore, the significant contributions of leading institutes from the top 10 countries, particularly those in China and the United States, highlight the crucial role of premier research institutions in enhancing a country’s academic stature. The predominance of Chinese authors and institutions, notably Shanghai Jiao Tong University (Table 4), coupled with substantial funding from the National Natural Science Foundation of China (NSFC) (Table 1), emphasizes China’s pivotal role in this research area. These authors, listed in Table 5, could be focal points for accessing the latest advancements in the field.
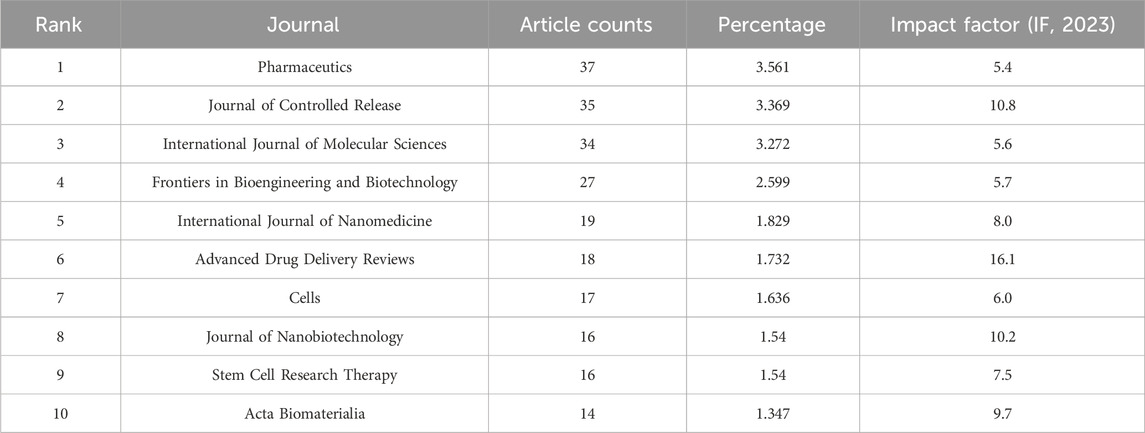
Table 5. The top 10 most productive journals related to MSC-EVs for drug delivery from 2013 to 2023.
Employing bibliometric research methods, this study establishes similarity relationships among publications across various parameters including institution, journal, country, and author. Figures 4–6 demonstrate China’s prominent position, with Shanghai Jiao Tong University and author Zhang Y being highly influential. The International Journal of Molecular Sciences emerges as a key journal in this domain. Additionally, co-citation analysis, examining publication impacts through total citation counts, reveals a high research frequency in pharmacology studies related to MSC-EVs, with the journal BLOOD potentially leading in citation frequency. Co-author analysis, aimed at identifying collaborative networks, indicates that authors, institutions, and countries with higher total link strength are more inclined towards collaboration. Based on these findings, the study offers insights and recommendations for future research, highlighting the active involvement of institutions like Shanghai Jiao Tong University and Zhejiang University from China, and Harvard University from the United States. The robust connections between these entities, primarily in China and the United States, may significantly influence research direction and quality enhancement in this burgeoning field.
4.3 Keyword trends and their relevance to MSC-EV drug delivery
In the burgeoning field of MSC-EVs, co-occurrence analysis has unveiled key research directions and prevailing themes. This analysis, grounded in the examination of keywords within the titles and abstracts of pertinent studies, culminated in the construction of a network map of occurrences. As delineated in Figure 8A, four predominant research trends emerged: tissue engineering, cancer research, neurological diseases, and targeted delivery. These trends not only resonate with the established understanding within the field but also offer clarity on potential avenues for future research endeavors.
In the tissue engineering cluster, MSC-EVs have emerged as powerful tools in regenerative medicine. By promoting tissue repair and regeneration, MSC-EVs have found applications in the development of tissue-engineered constructs. Keywords such as regenerative medicine, biomaterials, and microparticles underscore the diversity of approaches used to harness their therapeutic potential. For example, the integration of MSC-EVs with biomaterials has enhanced the delivery of these vesicles to damaged tissues, improving the effectiveness of cell-based therapies. Moreover, microparticles have provided innovative delivery mechanisms, allowing for the controlled release of MSC-EVs, thus prolonging their therapeutic effects. Such developments are especially relevant for regenerative applications in cartilage, bone, and skin, where the ability to accelerate tissue repair remains a priority in clinical settings.
The cancer research cluster highlights the dual role of MSC-EVs as both therapeutic agents and diagnostic biomarkers in oncology. Keywords such as angiogenesis, tumor microenvironment, and metastasis reflect the multifaceted nature of MSC-EV applications in cancer treatment. MSC-EVs have demonstrated their capacity to selectively target tumors and influence the tumor microenvironment, either inhibiting or promoting angiogenesis depending on the therapeutic context. Their ability to modulate the immune response and suppress tumor growth further solidifies their potential in oncology. Importantly, MSC-EVs are being explored for their role in preventing metastasis, by delivering anti-cancer agents directly to tumor cells, thus reducing the likelihood of secondary tumor formation. The capacity of MSC-EVs to carry tumor-specific proteins and nucleic acids also makes them promising candidates for early cancer detection and personalized therapies.
In the neurological diseases cluster, MSC-EVs are being explored as potential therapies for a range of central nervous system disorders, including neurodegenerative diseases, stroke, and traumatic brain injury. Keywords like mesenchymal stromal cells, functional recovery, and drug-delivery vehicles emphasize the regenerative potential of MSC-EVs in neural repair. Their ability to cross the blood-brain barrier—a significant challenge in neurological treatment—enhances their value in promoting functional recovery by reducing inflammation, stimulating neurogenesis, and improving the survival of neural cells. Studies have shown promising results in animal models, suggesting MSC-EVs could play a key role in developing therapies for stroke and neurodegenerative conditions. Furthermore, MSC-EVs are being engineered to deliver neuroprotective drugs and genes, potentially improving the precision and efficacy of treatments aimed at mitigating the effects of neurodegeneration.
Finally, the targeted delivery cluster underscores the innovations in MSC-EV-based delivery systems, where keywords such as cell-derived exosomes, stromal cells, and in-vitro reflect the ongoing efforts to enhance the specificity and efficiency of therapeutic delivery. MSC-EVs, particularly exosomes, have been widely recognized for their inherent targeting capabilities, driven by the surface proteins that allow them to home in on specific cell types. The ability to engineer these vesicles to carry bioactive molecules, including proteins and RNAs, positions them as ideal candidates for delivering therapeutic agents to precise locations in the body. Preclinical in-vitro studies have been instrumental in refining these delivery systems, providing insights into their uptake and therapeutic efficacy before transitioning to in-vivo and clinical trials. These advancements are critical in ensuring the safety and effectiveness of MSC-EV-based therapies for various conditions.
The centrality of specific keywords within the co-occurrence map underscores the need for advanced, high-quality research within these identified areas. Complementing this, an overlay visualization map, akin to the co-occurrence map but distinct in its color-coded representation, was developed, as shown in Figure 8C. The varied hues signify different scores, hinting at the likelihood of these themes evolving into focal topics of future research. Recent scholarly activities have seen a surge in studies involving various forms of EVs and mesenchymal stem cells, such as exosomes, microvesicles, and ectosomes, highlighting their increasing relevance (Shao et al., 2018; Lin et al., 2021; Phinney et al., 2015). Keyword burst detection further refined this analysis, identifying ‘progenitor cells’, ‘tumor-derived exosomes’, and ‘membrane vesicles’ as areas of intense research interest, as evident in Figures 9A, B.
4.4 Research frontiers and hotspots
The top 10 research and review articles with the most citations have been listed in Tables 6, 7. It shows that EVs have consistently been at the forefront of biomedical research, celebrated for their pivotal roles in a wide array of diseases. These nanoscale carriers, loaded with diverse biomolecules and encapsulated by phospholipid bilayers, are integral to the field of precision medicine, functioning as natural vehicles for the delivery of pharmaceutical agents (Wang et al., 2017). Recognized as a heterogeneous group of lipid-bound nanoparticles, EVs are involved in various (patho) physiological processes and are increasingly being explored for their potential to deliver therapeutic agents to specific cells or tissues. Their therapeutic applications are broad and include the alleviation of osteoarthritis (Chen L. et al., 2019; Khan et al., 2015; Tavakoli Dargani and Singla, 2019), mediation of cartilage repair, enhancement of cardiac function following myocardial infarction (Chen W. et al., 2019; Gao et al., 2020), and promotion of healing in pressure ulcers (Zhang et al., 2019; Luo et al., 2023; Tang et al., 2022).
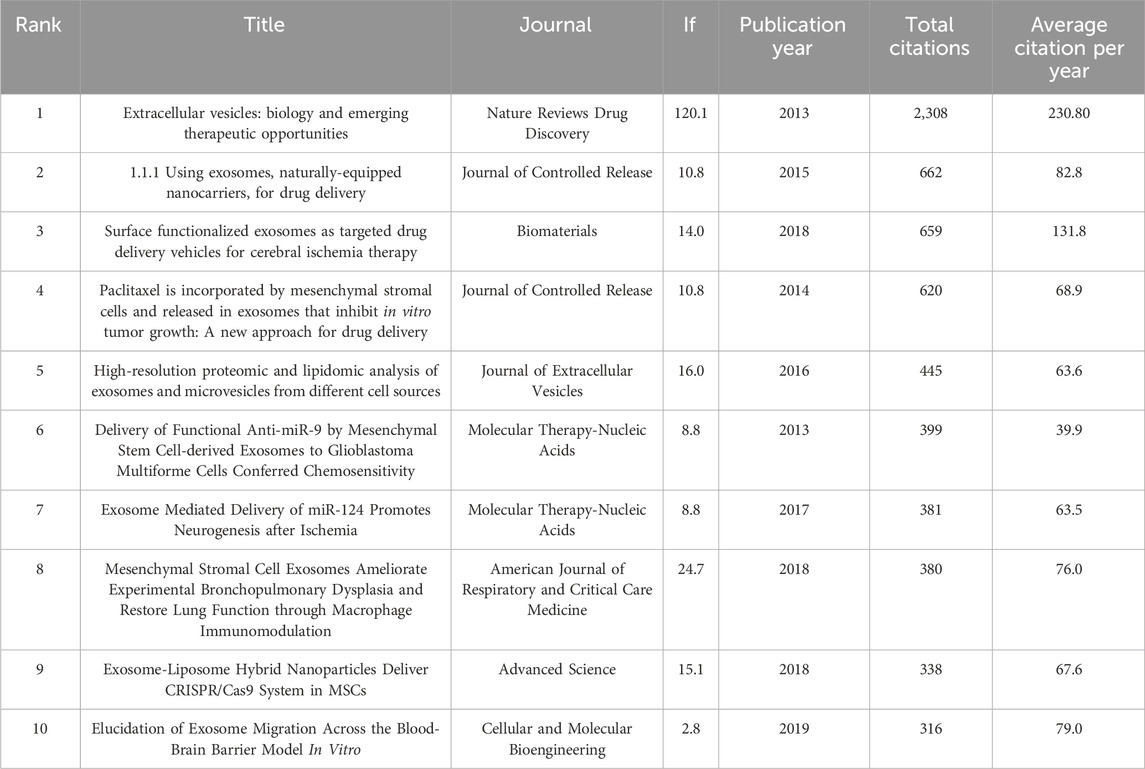
Table 6. The top 10 research articles with the most citations in the field of MSC-EVs for drug delivery from 2013 to 2023.
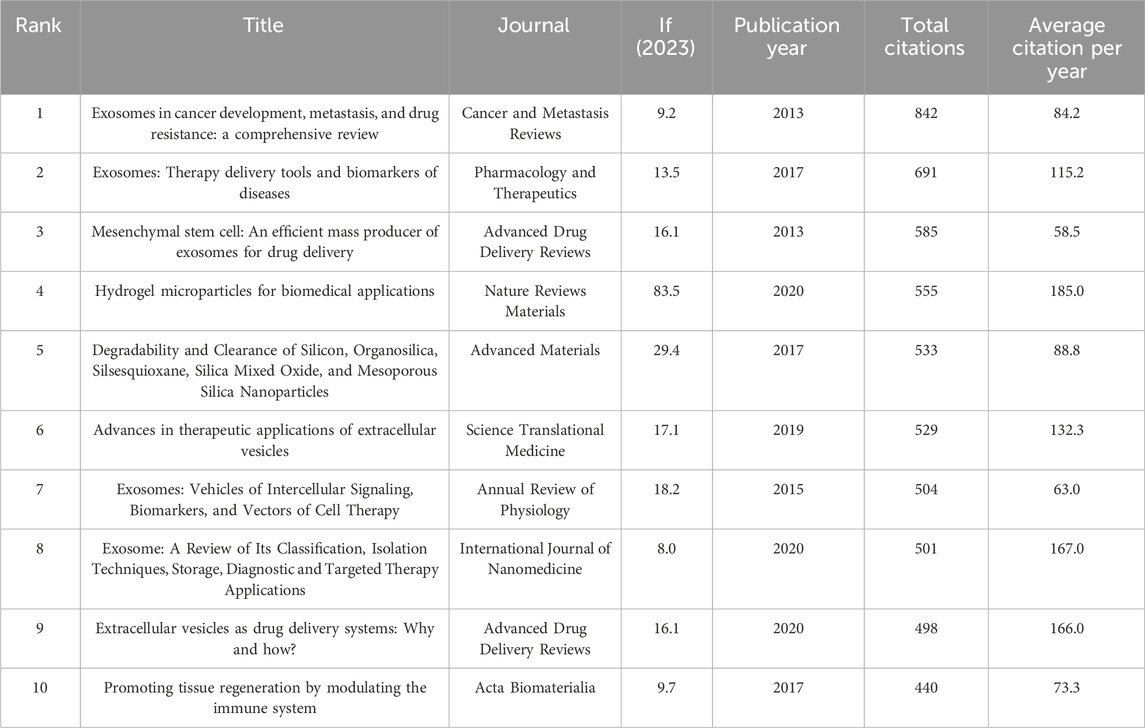
Table 7. The top 10 review articles with the most citations in the field of MSC-EVs for drug delivery from 2013 to 2023.
The intrinsic potential of EVs as natural delivery vehicles has spurred the development of bioengineering techniques aimed at maximizing their therapeutic efficacy (Evers et al., 2022). This enhancement can be achieved through two primary strategies: cargo engineering and surface engineering. By customizing the therapeutic payload of EVs or enhancing their selectivity for target cells, bioengineered EVs have the potential to become highly personalized and targeted therapeutic agents. Current research explores EVs as natural nanocarriers through artificial loading with a variety of therapeutic agents, including small molecules, drugs, proteins, and different RNA species such as small interfering RNA (siRNA) and microRNA (miRNA) (Table 8). Incorporating extrinsic cargo into EVs involves manipulating either the EVs themselves or the parental cells from which they originate. This can be done using two main methods: exogenous (direct) loading and endogenous (indirect) loading. Exogenous loading occurs after EV isolation and involves directly encapsulating the desired therapeutic cargo through processes such as co-incubation (Didiot et al., 2016; Sun et al., 2010), electroporation, sonication, freeze-thawing, extrusion, and permeation using detergent-based compounds (Didiot et al., 2016; Goh et al., 2017; Shtam et al., 2013; Haney et al., 2015). Endogenous loading, on the other hand, relies on the presence of the desired cargo within the producer cell, which then uses its cellular machinery to incorporate the cargo into the EVs naturally during their biogenesis.
However, EVs administered in vivo face the challenge of rapid clearance, primarily through uptake by cells in organs such as the liver, spleen, gastrointestinal tract, and lungs (Wiklander et al., 2015). The surface characteristics of EVs are crucial for their biodistribution, tissue tropism, and therapeutic efficacy. Modifying the EV surface can enhance their targeting to specific cell types, enable them to traverse various biological barriers, and extend their lifespan in vivo until they reach the target location (Jia et al., 2018; Kamerkar et al., 2017). Several strategies have been investigated to functionalize the surface of EVs, including genetic manipulation (engineering parental cells to produce EVs with transmembrane targeting moieties), chemical modification (anchoring targeting moieties to the surface of isolated EVs), and hybrid membrane engineering (conjugating natural EVs with synthetic liposome nanoparticles). Despite these advances, most methods for bioengineering the content and surface of MSC-derived EVs remain at the pre-clinical stage and face scalability challenges. Several obstacles hinder their therapeutic application, including inefficient production at clinical grade, lack of standardized methods for isolation, characterization, and quantification, inaccurate cargo characterization, pharmacokinetics issues, insufficient targeted delivery, limited drug loading efficiencies, EV heterogeneity, and safety profiles (Gowen et al., 2020; Meng et al., 2020). To facilitate the clinical translation of bioengineered MSC-EVs, the simple, cost-effective, and streamlined manufacturing process is urgently needed in the future (de Almeida Fuzeta et al., 2022; Herrmann et al., 2021).
The analysis of in vivo studies on MSC-derived exosome delivery (Figure 10) underscores several key research trends and hotspots. The sustained increase in publication volume and RRI reflects a growing interest in the clinical potential of MSC-derived exosomes. Keyword co-occurrence and clustering analysis reveal that much of the research is focused on therapeutic applications, particularly in regenerative medicine and cancer therapy. This is reinforced by the strong citation bursts for topics related to targeted delivery and the modulation of the tumor microenvironment, highlighting the potential of MSC-derived exosomes as both therapeutic agents and drug delivery vehicles. As the field continues to expand, in vivo studies will likely concentrate on optimizing the delivery efficiency and therapeutic efficacy of MSC-derived exosomes, particularly for cancer treatment and regenerative applications. The increasing focus on specific delivery mechanisms, such as nanoparticle-based systems and the targeting of tumor-derived exosomes, suggests that the next phase of research will aim to overcome current challenges related to bioavailability and precise targeting of therapeutic exosomes in vivo.
4.5 Limitations of this study
The current paper, while offering significant insights into the global research trends and interests in MSC-EVs for drug delivery, has limitations that merit careful consideration. A primary challenge lies in extracting detailed information about MSC-EVs, specifically their origins and intricate interrelationships within the context of drug delivery. This complexity poses a barrier to fully understanding the nuances and potential applications of MSC-EVs in this field. Furthermore, there is a potential risk of omitting relevant publications due to the constraints of database and language selection. The exclusion of major databases such as PubMed, Embase, Cochrane, and non-English language databases from the study might result in a skewed representation of the research landscape. This limitation could lead to an inadvertent oversight of pivotal studies and findings that are not indexed in the included databases or are published in languages other than English. Additionally, the reliance on citation counts as a measure of a paper’s impact can be problematic. Newly published papers, despite potentially being of high quality, may not have accumulated enough citations to be recognized in bibliometric analyses. This gap might create a disparity between the actual ongoing research activities and the trends observed through bibliometric methods. To address these limitations, it is crucial to broaden the scope of future research in this domain. This expansion would include incorporating the latest publications, regardless of their current citation count, and ensuring that non-English language papers are also considered. Such an inclusive approach would provide a more comprehensive and accurate reflection of the global research efforts and advancements in the field of MSC-EVs for drug delivery.
5 Conclusion
In conclusion, our comprehensive bibliometric and visualized study underscores the burgeoning interest and remarkable advancements in the field of MSC-EVs for drug delivery from 2013 to 2023. The analysis, spanning over a decade, reveals an ascending trajectory in global research efforts, with China and the United States leading in various key metrics, including publication count, H-index, total citations, and funding. The study identifies four major research clusters - tissue engineering, cancer, neurological diseases, and targeted delivery, further delineated into ten thematic areas such as differentiation, tissue regeneration, and drug resistance. These findings not only reflect the dynamic and evolving nature of MSC-EV research but also highlight its growing prominence in the scientific community. This study provides invaluable insights into the current state and potential future directions of MSC-EV research in drug delivery, offering a roadmap for researchers and policymakers to navigate this rapidly expanding field. As MSC-EVs continue to gain traction in therapeutic applications, their role in revolutionizing drug delivery systems becomes increasingly evident, paving the way for novel and more effective treatment modalities.
Data availability statement
The original contributions presented in the study are included in the article/supplementary material, further inquiries can be directed to the corresponding authors.
Author contributions
TZ: Writing–original draft, Writing–review and editing, Conceptualization, Software, Validation, Visualization. YM: Software, Visualization, Writing–original draft, Methodology. HD: Writing–review and editing. KL: Supervision, Conceptualization, Validation, Writing–original draft. FaZ: Data curation, Investigation, Methodology, Software, Writing–original draft. QL: Formal Analysis, Validation, Visualization, Writing–original draft. FC: Formal Analysis, Project administration, Supervision, Writing–review and editing. FeZ: Conceptualization, Funding acquisition, Project administration, Supervision, Writing–review and editing. ZY: Writing–original draft, Writing–review and editing.
Funding
The author(s) declare that financial support was received for the research, authorship, and/or publication of this article. This study was funded by grants from the National Natural Science Foundation of China (82272541).
Acknowledgments
We extend our gratitude to all the volunteers who participated in this study; their contributions were crucial to the success of our research.
Conflict of interest
The authors declare that the research was conducted in the absence of any commercial or financial relationships that could be construed as a potential conflict of interest.
Publisher’s note
All claims expressed in this article are solely those of the authors and do not necessarily represent those of their affiliated organizations, or those of the publisher, the editors and the reviewers. Any product that may be evaluated in this article, or claim that may be made by its manufacturer, is not guaranteed or endorsed by the publisher.
References
Abas, B. I., Demirbolat, G. M., and Cevik, O. (2022). Wharton jelly-derived mesenchymal stem cell exosomes induce apoptosis and suppress EMT signaling in cervical cancer cells as an effective drug carrier system of paclitaxel. PLoS One 17 (9), e0274607. doi:10.1371/journal.pone.0274607
Ala, M. (2023). The beneficial effects of mesenchymal stem cells and their exosomes on myocardial infarction and critical considerations for enhancing their efficacy. Ageing Res. Rev. 89, 101980. doi:10.1016/j.arr.2023.101980
Altanerova, U., Jakubechova, J., Benejova, K., Priscakova, P., Pesta, M., Pitule, P., et al. (2019). Prodrug suicide gene therapy for cancer targeted intracellular by mesenchymal stem cell exosomes. Int. J. Cancer 144 (4), 897–908. doi:10.1002/ijc.31792
Bagheri, E., Abnous, K., Farzad, S. A., Taghdisi, S. M., Ramezani, M., and Alibolandi, M. (2020). Targeted doxorubicin-loaded mesenchymal stem cells-derived exosomes as a versatile platform for fighting against colorectal cancer. Life Sci. 261, 118369. doi:10.1016/j.lfs.2020.118369
Baglio, S. R., Pegtel, D. M., and Baldini, N. (2012). Mesenchymal stem cell secreted vesicles provide novel opportunities in (stem) cell-free therapy. Front. Physiol. 3, 359. doi:10.3389/fphys.2012.00359
Banche Niclot, A. G. S., Marini, E., Ferrero, I., Barbero, F., Rosso, E., Fenoglio, I., et al. (2023). A new paclitaxel formulation based on secretome isolated from mesenchymal stem cells shows a significant cytotoxic effect on osteosarcoma cell lines. Pharmaceutics 15 (9), 2340. doi:10.3390/pharmaceutics15092340
Barry, F., and Murphy, M. (2013). Mesenchymal stem cells in joint disease and repair. Nat. Rev. Rheumatol. 9 (10), 584–594. doi:10.1038/nrrheum.2013.109
Behera, J., Kumar, A., Voor, M. J., and Tyagi, N. (2021). Exosomal lncRNA-H19 promotes osteogenesis and angiogenesis through mediating Angpt1/Tie2-NO signaling in CBS-heterozygous mice. Theranostics 11 (16), 7715–7734. doi:10.7150/thno.58410
Blau, H. M., and Daley, G. Q. (2019). Stem cells in the treatment of disease. N. Engl. J. Med. 380 (18), 1748–1760. doi:10.1056/NEJMra1716145
Bucan, V., Vaslaitis, D., Peck, C. T., Strauß, S., Vogt, P. M., and Radtke, C. (2019). Effect of exosomes from rat adipose-derived mesenchymal stem cells on neurite outgrowth and sciatic nerve regeneration after crush injury. Mol. Neurobiol. 56 (3), 1812–1824. doi:10.1007/s12035-018-1172-z
Chang, Y. H., Wu, K. C., Harn, H. J., Lin, S. Z., and Ding, D. C. (2018). Exosomes and stem cells in degenerative disease diagnosis and therapy. Cell Transpl. 27 (3), 349–363. doi:10.1177/0963689717723636
Chen, L., Mou, S., Li, F., Zeng, Y., Sun, Y., Horch, R. E., et al. (2019a). Self-assembled human adipose-derived stem cell-derived extracellular vesicle-functionalized biotin-doped polypyrrole titanium with long-term stability and potential osteoinductive ability. ACS Appl. Mater Interfaces 11 (49), 46183–46196. doi:10.1021/acsami.9b17015
Chen, W., Sun, Y., Gu, X., Hao, Y., Liu, X., Lin, J., et al. (2019b). Conditioned medium of mesenchymal stem cells delays osteoarthritis progression in a rat model by protecting subchondral bone, maintaining matrix homeostasis, and enhancing autophagy. J. Tissue Eng. Regen. Med. 13 (9), 1618–1628. doi:10.1002/term.2916
Chen, Y. A., Lu, C. H., Ke, C. C., Chiu, S. J., Jeng, F. S., Chang, C. W., et al. (2021). Mesenchymal stem cell-derived exosomes ameliorate alzheimer's disease pathology and improve cognitive deficits. Biomedicines 9 (6), 594. doi:10.3390/biomedicines9060594
Dashnyam, K., Lee, J. H., Mandakhbayar, N., Jin, G. Z., Lee, H. H., and Kim, H. W. (2018). Intra-articular biomaterials-assisted delivery to treat temporomandibular joint disorders. J. TISSUE Eng. 9, 2041731418776514. doi:10.1177/2041731418776514
de Almeida Fuzeta, M., Gonçalves, P. P., Fernandes-Platzgummer, A., Cabral, J. M. S., Bernardes, N., and da Silva, C. L. (2022). From promise to reality: bioengineering strategies to enhance the therapeutic potential of extracellular vesicles. Bioeng. (Basel) 9 (11), 675. doi:10.3390/bioengineering9110675
Deng, J., and Ke, H. (2023). Overcoming the resistance of hepatocellular carcinoma to PD-1/PD-L1 inhibitor and the resultant immunosuppression by CD38 siRNA-loaded extracellular vesicles. Oncoimmunology 12 (1), 2152635. doi:10.1080/2162402x.2022.2152635
Didiot, M. C., Hall, L. M., Coles, A. H., Haraszti, R. A., Godinho, B. M., Chase, K., et al. (2016). Exosome-mediated delivery of hydrophobically modified siRNA for huntingtin mRNA silencing. Mol. Ther. 24 (10), 1836–1847. doi:10.1038/mt.2016.126
Dubey, S., Chen, Z., Jiang, Y. J., Talis, A., Molotkov, A., Ali, A., et al. (2024). Small extracellular vesicles (sEVs)-based gene delivery platform for cell-specific CRISPR/Cas9 genome editing. Theranostics 14 (7), 2777–2793. doi:10.7150/thno.92133
Evers, M. J. W., van de Wakker, S. I., de Groot, E. M., de Jong, O. G., Gitz-François, J. J. J., Seinen, C. S., et al. (2022). Functional siRNA delivery by extracellular vesicle-liposome hybrid nanoparticles. Adv. Healthc. Mater 11 (5), e2101202. doi:10.1002/adhm.202101202
Gao, J., Zhang, G., Xu, K., Ma, D., Ren, L., Fan, J., et al. (2020). Bone marrow mesenchymal stem cells improve bone erosion in collagen-induced arthritis by inhibiting osteoclasia-related factors and differentiating into chondrocytes. Stem Cell Res. Ther. 11 (1), 171. doi:10.1186/s13287-020-01684-w
Goh, W. J., Lee, C. K., Zou, S., Woon, E. C., Czarny, B., and Pastorin, G. (2017). Doxorubicin-loaded cell-derived nanovesicles: an alternative targeted approach for anti-tumor therapy. Int. J. Nanomedicine 12, 2759–2767. doi:10.2147/IJN.S131786
Gowen, A., Shahjin, F., Chand, S., Odegaard, K. E., and Yelamanchili, S. V. (2020). Mesenchymal stem cell-derived extracellular vesicles: challenges in clinical applications. Front. Cell Dev. Biol. 8, 149. doi:10.3389/fcell.2020.00149
Haney, M. J., Klyachko, N. L., Zhao, Y., Gupta, R., Plotnikova, E. G., He, Z., et al. (2015). Exosomes as drug delivery vehicles for Parkinson's disease therapy. J. Control. release 207, 18–30. doi:10.1016/j.jconrel.2015.03.033
Hazrati, A., Malekpour, K., Soudi, S., and Hashemi, S. M. (2022). CRISPR/Cas9-engineered mesenchymal stromal/stem cells and their extracellular vesicles: a new approach to overcoming cell therapy limitations. Biomed. and Pharmacother. 156, 113943. doi:10.1016/j.biopha.2022.113943
Herrmann, I. K., Wood, M. J. A., and Fuhrmann, G. (2021). Extracellular vesicles as a next-generation drug delivery platform. Nat. Nanotechnol. 16 (7), 748–759. doi:10.1038/s41565-021-00931-2
Hinkelmann, S., Springwald, A. H., Schulze, S., Hempel, U., Mitrach, F., Wölk, C., et al. (2022). Mineralizing gelatin microparticles as cell carrier and drug delivery system for siRNA for bone tissue engineering. Pharmaceutics 14 (3), 548. doi:10.3390/pharmaceutics14030548
Hu, W., Chen, N., Yan, W., Pei, P., Wei, Y., and Zhan, X. (2022). Knowledge mapping of olfactory dysfunction: a bibliometric study. Front. Syst. Neurosci. 16, 904982. doi:10.3389/fnsys.2022.904982
Hu, Y., Tao, R., Wang, L., Chen, L., Lin, Z., Panayi, A. C., et al. (2021). Exosomes derived from bone mesenchymal stem cells alleviate compression-induced nucleus pulposus cell apoptosis by inhibiting oxidative stress. Oxid. Med. Cell Longev. 2021, 2310025. doi:10.1155/2021/2310025
Huang, D., Zhang, M., and Tan, Z. (2022). Bone marrow stem cell-exo-derived TSG-6 attenuates 1-methyl-4-phenylpyridinium+-induced neurotoxicity via the STAT3/miR-7/NEDD4/LRRK2 Axis. J. Neuropathol. Exp. Neurol. 81 (8), 621–634. doi:10.1093/jnen/nlac049
Jia, G., Han, Y., An, Y., Ding, Y., He, C., Wang, X., et al. (2018). NRP-1 targeted and cargo-loaded exosomes facilitate simultaneous imaging and therapy of glioma in vitro and in vivo. Biomaterials 178, 302–316. doi:10.1016/j.biomaterials.2018.06.029
Jiyah, A., Muhammad, S. A., Ibrahim, A., Bulama, I., Ibrahim, A., Abbas, A. Y., et al. (2024). Plant-derived extracellular vesicles as potential smart nano drug delivery systems for antioxidant vitamins C and E in Alzheimer's disease. J. Drug Deliv. Sci. Technol. 95, 105618. doi:10.1016/j.jddst.2024.105618
Kamerkar, S., LeBleu, V. S., Sugimoto, H., Yang, S., Ruivo, C. F., Melo, S. A., et al. (2017). Exosomes facilitate therapeutic targeting of oncogenic KRAS in pancreatic cancer. Nature 546 (7659), 498–503. doi:10.1038/nature22341
Khan, M., Nickoloff, E., Abramova, T., Johnson, J., Verma, S. K., Krishnamurthy, P., et al. (2015). Embryonic stem cell-derived exosomes promote endogenous repair mechanisms and enhance cardiac function following myocardial infarction. Circ. Res. 117 (1), 52–64. doi:10.1161/CIRCRESAHA.117.305990
Kim, H. S., Shin, T. H., Lee, B. C., Yu, K. R., Seo, Y., Lee, S., et al. (2013). Human umbilical cord blood mesenchymal stem cells reduce colitis in mice by activating NOD2 signaling to COX2. Gastroenterology 145 (6), 1392–1403. e1-8. doi:10.1053/j.gastro.2013.08.033
Li, L., Wang, Y., Xu, Y., Xu, J., Zhao, Y., Cheng, Z., et al. (2024). ROS-scavenging lipid-based liquid crystalline as a favorable stem cell extracellular vesicles delivery vector to promote wound healing. J. Control. Release 371, 298–312. doi:10.1016/j.jconrel.2024.05.048
Li, N., and Hua, J. (2017). Interactions between mesenchymal stem cells and the immune system. Cell Mol. Life Sci. 74 (13), 2345–2360. doi:10.1007/s00018-017-2473-5
Li, X., Xu, L., Nie, H. M., and Lei, L. (2021). Dexamethasone-loaded β-cyclodextrin for osteogenic induction of mesenchymal stem/progenitor cells and bone regeneration. J. Biomed. Mater. Res. PART A 109 (7), 1125–1135. doi:10.1002/jbm.a.37104
Lin, J., Wang, L., Lin, J., and Liu, Q. (2021). The role of extracellular vesicles in the pathogenesis, diagnosis, and treatment of osteoarthritis. Molecules 26 (16), 4987. doi:10.3390/molecules26164987
Lin, J., Yang, Z., Wang, L., Xing, D., and Lin, J. (2022). Global research trends in extracellular vesicles based on stem cells from 1991 to 2021: a bibliometric and visualized study. Front. Bioeng. Biotechnol. 10, 956058. doi:10.3389/fbioe.2022.956058
Liu, T., Li, T., Zheng, Y., Xu, X., Sun, R., Zhan, S., et al. (2022). Evaluating adipose-derived stem cell exosomes as miRNA drug delivery systems for the treatment of bladder cancer. Cancer Med. 11 (19), 3687–3699. doi:10.1002/cam4.4745
Liu, W., Liu, A., Li, X., Sun, Z., Sun, Z., Liu, Y., et al. (2023). Dual-engineered cartilage-targeting extracellular vesicles derived from mesenchymal stem cells enhance osteoarthritis treatment via miR-223/NLRP3/pyroptosis axis: toward a precision therapy. Bioact. Mater 30, 169–183. doi:10.1016/j.bioactmat.2023.06.012
Liu, Y., Lin, L., Zou, R., Wen, C., Wang, Z., and Lin, F. (2018). MSC-derived exosomes promote proliferation and inhibit apoptosis of chondrocytes via lncRNA-KLF3-AS1/miR-206/GIT1 axis in osteoarthritis. Cell Cycle 17 (21-22), 2411–2422. doi:10.1080/15384101.2018.1526603
Liu, Z., Zhou, Z., Ma, J., Dong, J., Sun, Y., Pang, M., et al. (2024). Major depressive disease research in BRICS: a bibliometric analysis of publications from 2003 to 2022. Asian J. Psychiatr. 92, 103900. doi:10.1016/j.ajp.2023.103900
Lopatina, T., Bruno, S., Tetta, C., Kalinina, N., Porta, M., and Camussi, G. (2014). Platelet-derived growth factor regulates the secretion of extracellular vesicles by adipose mesenchymal stem cells and enhances their angiogenic potential. Cell Commun. Signal 12, 26. doi:10.1186/1478-811X-12-26
Luo, L., Zhang, H., Zhang, S., Luo, C., Kan, X., Lv, J., et al. (2023). Extracellular vesicle-derived silk fibroin nanoparticles loaded with MFGE8 accelerate skin ulcer healing by targeting the vascular endothelial cells. J. Nanobiotechnology 21 (1), 455. doi:10.1186/s12951-023-02185-7
Luo, Q., Xian, P., Wang, T., Wu, S., Sun, T., Wang, W., et al. (2021). Antioxidant activity of mesenchymal stem cell-derived extracellular vesicles restores hippocampal neurons following seizure damage. Theranostics 11 (12), 5986–6005. doi:10.7150/thno.58632
Ma, T., Chen, S., Wang, J., Liang, S., Chen, M., Liu, Q., et al. (2024). Enhanced osteolysis targeted therapy through fusion of exosomes derived from M2 macrophages and bone marrow mesenchymal stem cells: modulating macrophage polarization. Small 20 (7), e2303506. doi:10.1002/smll.202303506
Mansoor, H., Ong, H. S., Riau, A. K., Stanzel, T. P., Mehta, J. S., and Yam, G. H. (2019). Current trends and future perspective of mesenchymal stem cells and exosomes in corneal diseases. Int. J. Mol. Sci. 20 (12), 2853. doi:10.3390/ijms20122853
Meng, W., He, C., Hao, Y., Wang, L., Li, L., and Zhu, G. (2020). Prospects and challenges of extracellular vesicle-based drug delivery system: considering cell source. Drug Deliv. 27 (1), 585–598. doi:10.1080/10717544.2020.1748758
Moore, T. L., Bowley, B. G. E., Pessina, M. A., Calderazzo, S. M., Medalla, M., Go, V., et al. (2019). Mesenchymal derived exosomes enhance recovery of motor function in a monkey model of cortical injury. Restor. Neurol. Neurosci. 37 (4), 347–362. doi:10.3233/RNN-190910
Musiał-Wysocka, A., Kot, M., and Majka, M. (2019). The pros and cons of mesenchymal stem cell-based therapies. Cell Transpl. 28 (7), 801–812. doi:10.1177/0963689719837897
Nakazaki, M., Morita, T., Lankford, K. L., Askenase, P. W., and Kocsis, J. D. (2021). Small extracellular vesicles released by infused mesenchymal stromal cells target M2 macrophages and promote TGF-β upregulation, microvascular stabilization and functional recovery in a rodent model of severe spinal cord injury. J. Extracell. Vesicles 10 (11), e12137. doi:10.1002/jev2.12137
Naseri, Z., Oskuee, R. K., Jaafari, M. R., and Forouzandeh Moghadam, M. (2018). Exosome-mediated delivery of functionally active miRNA-142-3p inhibitor reduces tumorigenicity of breast cancer in vitro and in vivo. Int. J. Nanomedicine 13, 7727–7747. doi:10.2147/IJN.S182384
Nguyen, T. H., Duong, C. M., Nguyen, X. H., and Than, U. T. T. (2021). Mesenchymal stem cell-derived extracellular vesicles for osteoarthritis treatment: extracellular matrix protection, chondrocyte and osteocyte physiology, pain and inflammation management. Cells 10 (11), 2887. doi:10.3390/cells10112887
Pascucci, L., Coccè, V., Bonomi, A., Ami, D., Ceccarelli, P., Ciusani, E., et al. (2014). Paclitaxel is incorporated by mesenchymal stromal cells and released in exosomes that inhibit in vitro tumor growth: a new approach for drug delivery. J. Control Release 192, 262–270. doi:10.1016/j.jconrel.2014.07.042
Phinney, D. G., Di Giuseppe, M., Njah, J., Sala, E., Shiva, S., St Croix, C. M., et al. (2015). Mesenchymal stem cells use extracellular vesicles to outsource mitophagy and shuttle microRNAs. Nat. Commun. 6, 8472. doi:10.1038/ncomms9472
Porada, C. D., and Almeida-Porada, G. (2010). Mesenchymal stem cells as therapeutics and vehicles for gene and drug delivery. Adv. Drug Deliv. Rev. 62 (12), 1156–1166. doi:10.1016/j.addr.2010.08.010
Schultz, I., Vollmers, F., Lühmann, T., Rybak, J.-C., Wittmann, R., Stank, K., et al. (2015). Pulmonary insulin-like growth factor I delivery from trehalose and silk-fibroin microparticles. ACS Biomaterials Sci. and Eng. 1 (2), 119–129. doi:10.1021/ab500101c
Shah, M. W., Ahmad, T., Khan, M., Muhammad, S., and Sun, G. (2022). Global research on vitamin D and coronavirus disease 2019: a bibliometric and visualized study. Med. Baltim. 101 (27), e29768. doi:10.1097/MD.0000000000029768
Shao, H., Im, H., Castro, C. M., Breakefield, X., Weissleder, R., and Lee, H. (2018). New technologies for analysis of extracellular vesicles. Chem. Rev. 118 (4), 1917–1950. doi:10.1021/acs.chemrev.7b00534
Shen, W. C., Lai, Y. C., Li, L. H., Liao, K., Lai, H. C., Kao, S. Y., et al. (2019). Methylation and PTEN activation in dental pulp mesenchymal stem cells promotes osteogenesis and reduces oncogenesis. Nat. Commun. 10 (1), 2226. doi:10.1038/s41467-019-10197-x
Shtam, T. A., Kovalev, R. A., Varfolomeeva, E. Y., Makarov, E. M., Kil, Y. V., and Filatov, M. V. (2013). Exosomes are natural carriers of exogenous siRNA to human cells in vitro. Cell Commun. Signal 11, 88. doi:10.1186/1478-811X-11-88
Song, L., Liang, X., Zhu, M., Su, Q., and Li, F. (2023). Knowledge mapping of immunotherapy in cervical carcinoma: a bibliometric analysis (2000-2023). Front. Immunol. 14, 1328103. doi:10.3389/fimmu.2023.1328103
Sun, D., Zhuang, X., Xiang, X., Liu, Y., Zhang, S., Liu, C., et al. (2010). A novel nanoparticle drug delivery system: the anti-inflammatory activity of curcumin is enhanced when encapsulated in exosomes. Mol. Ther. 18 (9), 1606–1614. doi:10.1038/mt.2010.105
Sun, X., Song, W., Teng, L., Huang, Y., Liu, J., Peng, Y., et al. (2023). MiRNA 24-3p-rich exosomes functionalized DEGMA-modified hyaluronic acid hydrogels for corneal epithelial healing. Bioact. Mater 25, 640–656. doi:10.1016/j.bioactmat.2022.07.011
Synnestvedt, M. B., Chen, C., and Holmes, J. H. (2005). CiteSpace II: visualization and knowledge discovery in bibliographic databases. AMIA Annu. Symp. Proc. 2005, 724–728.
Tan, F., Li, X., Wang, Z., Li, J., Shahzad, K., and Zheng, J. (2024). Clinical applications of stem cell-derived exosomes. Signal Transduct. Target Ther. 9 (1), 17. doi:10.1038/s41392-023-01704-0
Tang, J., Cui, X., Zhang, Z., Xu, Y., Guo, J., Soliman, B. G., et al. (2022). Injection-free delivery of MSC-derived extracellular vesicles for myocardial infarction therapeutics. Adv. Healthc. Mater 11 (5), e2100312. doi:10.1002/adhm.202100312
Tavakoli Dargani, Z., and Singla, D. K. (2019). Embryonic stem cell-derived exosomes inhibit doxorubicin-induced TLR4-NLRP3-mediated cell death-pyroptosis. Am. J. Physiol. Heart Circ. Physiol. 317 (2), H460–h471. doi:10.1152/ajpheart.00056.2019
Théry, C., Witwer, K. W., Aikawa, E., Alcaraz, M. J., Anderson, J. D., Andriantsitohaina, R., et al. (2018). Minimal information for studies of extracellular vesicles 2018 (MISEV2018): a position statement of the International Society for Extracellular Vesicles and update of the MISEV2014 guidelines. J. Extracell. Vesicles 7 (1), 1535750. doi:10.1080/20013078.2018.1535750
Wang, C., Zhang, Y., Zhang, Y., and Li, B. (2024). A bibliometric analysis of gastric cancer liver metastases: advances in mechanisms of occurrence and treatment options. Int. J. Surg. 110, 2288–2299. doi:10.1097/js9.0000000000001068
Wang, J., Li, M., Jin, L., Guo, P., Zhang, Z., Zhanghuang, C., et al. (2022). Exosome mimetics derived from bone marrow mesenchymal stem cells deliver doxorubicin to osteosarcoma in vitro and in vivo. Drug Deliv. 29 (1), 3291–3303. doi:10.1080/10717544.2022.2141921
Wang, N., Chen, C., Yang, D., Liao, Q., Luo, H., Wang, X., et al. (2017). Mesenchymal stem cells-derived extracellular vesicles, via miR-210, improve infarcted cardiac function by promotion of angiogenesis. Biochim. Biophys. Acta Mol. Basis Dis. 1863 (8), 2085–2092. doi:10.1016/j.bbadis.2017.02.023
Wang, X., Shah, F. A., Vazirisani, F., Johansson, A., Palmquist, A., Omar, O., et al. (2020). Exosomes influence the behavior of human mesenchymal stem cells on titanium surfaces. Biomaterials 230, 119571. doi:10.1016/j.biomaterials.2019.119571
Wiklander, O. P., Nordin, J. Z., O'Loughlin, A., Gustafsson, Y., Corso, G., Mäger, I., et al. (2015). Extracellular vesicle in vivo biodistribution is determined by cell source, route of administration and targeting. J. Extracell. Vesicles 4, 26316. doi:10.3402/jev.v4.26316
Wu, P., Zhang, B., Ocansey, D. K. W., Xu, W., and Qian, H. (2021). Extracellular vesicles: a bright star of nanomedicine. Biomaterials 269, 120467. doi:10.1016/j.biomaterials.2020.120467
Wu, Q., Fu, X., Li, X., Li, J., Han, W., and Wang, Y. (2023). Modification of adipose mesenchymal stem cells-derived small extracellular vesicles with fibrin-targeting peptide CREKA for enhanced bone repair. Bioact. Mater 20, 208–220. doi:10.1016/j.bioactmat.2022.05.031
Xing, D., Zhao, Y., Dong, S., and Lin, J. (2018). Global research trends in stem cells for osteoarthritis: a bibliometric and visualized study. Int. J. Rheum. Dis. 21 (7), 1372–1384. doi:10.1111/1756-185X.13327
Yahao, G., and Xinjia, W. (2021). The role and mechanism of exosomes from umbilical cord mesenchymal stem cells in inducing osteogenesis and preventing osteoporosis. Cell Transpl. 30, 9636897211057465. doi:10.1177/09636897211057465
Yang, C., Guan, Z., Pang, X., Tan, Z., Yang, X., Li, X., et al. (2022b). Desialylated mesenchymal stem cells-derived extracellular vesicles loaded with doxorubicin for targeted inhibition of hepatocellular carcinoma. Cells 11 (17), 2642. doi:10.3390/cells11172642
Yang, Z., Fan, Z., Wang, D., Li, H., He, Z., Xing, D., et al. (2023b). Bibliometric and visualization analysis of stem cell therapy for meniscal regeneration from 2012 to 2022. Front. Bioeng. Biotechnol. 11, 1107209. doi:10.3389/fbioe.2023.1107209
Yang, Z., Li, J., Deng, H., Li, H., Zhao, T., Gao, T., et al. (2023a). Visualization and bibliometric analysis of 3D printing in cartilage regeneration. Front. Bioeng. Biotechnol. 11, 1214715. doi:10.3389/fbioe.2023.1214715
Yang, Z., Lin, J., Li, H., He, Z., Wang, K., Lei, L., et al. (2022a). Bibliometric and visualization analysis of macrophages associated with osteoarthritis from 1991 to 2021. Front. Immunol. 13, 1013498. doi:10.3389/fimmu.2022.1013498
Ye, Y., Zhang, X., Xie, F., Xu, B., Xie, P., Yang, T., et al. (2020). An engineered exosome for delivering sgRNA: cas9 ribonucleoprotein complex and genome editing in recipient cells. Biomaterials Sci. 8 (10), 2966–2976. doi:10.1039/d0bm00427h
Yuan, X., Sun, L., Jeske, R., Nkosi, D., York, S. B., Liu, Y., et al. (2022). Engineering extracellular vesicles by three-dimensional dynamic culture of human mesenchymal stem cells. J. Extracell. Vesicles 11 (6), e12235. doi:10.1002/jev2.12235
Zhang, H., Li, C., Wen, D., Li, R., Lu, S., Xu, R., et al. (2022b). Melatonin improves the quality of maternally aged oocytes by maintaining intercellular communication and antioxidant metabolite supply. Redox Biol. 49, 102215. doi:10.1016/j.redox.2021.102215
Zhang, X., Lu, Y., Wu, S., Zhang, S., Li, S., and Tan, J. (2022a). An overview of current research on mesenchymal stem cell-derived extracellular vesicles: a bibliometric analysis from 2009 to 2021. Front. Bioeng. Biotechnol. 10, 910812. doi:10.3389/fbioe.2022.910812
Zhang, Y., Xu, J., Liu, S., Lim, M., Zhao, S., Cui, K., et al. (2019). Embryonic stem cell-derived extracellular vesicles enhance the therapeutic effect of mesenchymal stem cells. Theranostics 9 (23), 6976–6990. doi:10.7150/thno.35305
Zhang, Z., Zou, X., Zhang, R., Xie, Y., Feng, Z., Li, F., et al. (2021). Human umbilical cord mesenchymal stem cell-derived exosomal miR-146a-5p reduces microglial-mediated neuroinflammation via suppression of the IRAK1/TRAF6 signaling pathway after ischemic stroke. Aging (Albany NY) 13 (2), 3060–3079. doi:10.18632/aging.202466
Keywords: research hotspots and trends, mesenchymal stem cell, extracellular vesicles, bibliometric, visualization
Citation: Zhao T, Mu Y, Deng H, Liang K, Zhou F, Lin Q, Cao F, Zhou F and Yang Z (2024) Research hotspots and trends of mesenchymal stem cell-derived extracellular vesicles for drug delivery: a bibliometric and visualization analysis from 2013 to 2023. Front. Cell Dev. Biol. 12:1412363. doi: 10.3389/fcell.2024.1412363
Received: 04 April 2024; Accepted: 14 October 2024;
Published: 30 October 2024.
Edited by:
Michele Iafisco, National Research Council (CNR), ItalyReviewed by:
Xun Sun, Tianjin Hospital, ChinaXuegang Yuan, University of California, Los Angeles, United States
Copyright © 2024 Zhao, Mu, Deng, Liang, Zhou, Lin, Cao, Zhou and Yang. This is an open-access article distributed under the terms of the Creative Commons Attribution License (CC BY). The use, distribution or reproduction in other forums is permitted, provided the original author(s) and the copyright owner(s) are credited and that the original publication in this journal is cited, in accordance with accepted academic practice. No use, distribution or reproduction is permitted which does not comply with these terms.
*Correspondence: Zhen Yang, emhlbnlhbmdfbmt1QDE2My5jb20=; Feifei Zhou, b3J0aG96aG91QDE2My5jb20=; Fuyang Cao, ZHJjYW8wNjAzQDE2My5jb20=
†These authors have contributed equally to this work and share first authorship