- 1Department of Pharmacy, Guangdong Provincial Key Laboratory of Major Obstetric Diseases, Guangdong Provincial Clinical Research Center for Obstetrics and Gynecology, The Third Affiliated Hospital, Guangzhou Medical University, Guangzhou, China
- 2International Institute for Translational Chinese Medicine, School of Pharmaceutical Science, Guangzhou University of Chinese Medicine, Guangzhou, China
- 3Department of Gastroenterology, The Second Affiliated Hospital of Guangzhou University of Chinese Medicine, Guangzhou, China
- 4Guangdong Provincial Key Laboratory of Clinical Research on Traditional Chinese Medicine Syndrome, Guangzhou, China
Colorectal cancer (CRC) is the third most common cancer and has ranked the third leading cause in cancerassociated death globally. Metastasis is the leading cause of death in colorectal cancer patients. The role of tumor microenvironment (TME) in colorectal cancer metastasis has received increasing attention. As the most abundant cell type in the TME of solid tumors, cancer-associated fibroblasts (CAFs) have been demonstrated to have multiple functions in advancing tumor growth and metastasis. They can remodel the extracellular matrix (ECM) architecture, promote epithelial-mesenchymal transition (EMT), and interact with cancer cells or other stromal cells by secreting growth factors, cytokines, chemokines, and exosomes, facilitating tumor cell invasion into TME and contributing to distant metastasis. This article aims to analyze the sources and heterogeneity of CAFs in CRC, as well as their role in invasion and metastasis, in order to provide new insights into the metastasis mechanism of CRC and its clinical applications.
1 Introduction
Colorectal cancer (CRC) is one of the most common malignant tumors of the digestive tract in the world. According to the global Cancer statistics in 2020, the new cases of CRC rank the third among malignant tumors (Sung et al., 2021). Metastasis is the primary cause of cancer-related mortality in CRC patients (Siegel et al., 2017). It is well known that tumor metastasis is a complex multi-stage, multi-step process that is closely influenced by the tumor microenvironment (TME). TME is a complex tissue environment composed of the extracellular matrix (ECM) and various types of stromal cells, such as cancer-associated fibroblasts (CAFs), macrophages, inflammatory cells and mesenchymal stem cells, and CAFs interacts with all other cells in the TME through direct cell contact and cytokine secretion, thereby stimulating tumor progression and ultimately metastasis. Therefore, CAF is considered a potential new target for cancer therapy (Sahai et al., 2020). CAFs are activated fibroblasts, a major component of TME, accounting for nearly 70% of cells in tumor tissue. These cells are highly heterogeneous in origin, coming from different tissues or cells, and currently lack specific biomarkers. Although more studies have been conducted, the source remains undetermined, and their functions differ greatly depending on their origin (Chen and Song, 2019). A large body of evidence favors the pro-tumorigenic ability of CAFs, which can directly promote tumor cell proliferation and invasion, indirectly affect the peripheral ECM, the vascular system, and modulate immune function to promote tumor progression (Biffi and Tuveson, 2021). However, it has also been recently suggested that CAFs also have tumor suppressive effects under certain circumstances (Wang et al., 2021). And research has shown that their existence is correlated with prognosis, and they are already under evaluation as a possible target for treatment (Chen et al., 2021b).
In view of the fact that metastasis is the main cause of CRC-related deaths and the key role of CAFs in metastasis, therefore, in this review, we focused on the origin and heterogeneity of CAFs in CRC, biomarkers, and elaborated their roles in CRC invasion and metastasis, with the aim of providing new ideas for the study of the metastatic mechanism and clinical application of CAFs in CRC.
2 Origin and heterogeneity of CAFs in CRC
2.1 Cellular origin of CAFs in CRC
Fibroblasts are a major cell type in the stroma, present in almost all organs and normal tissues, and contribute to inflammation and fibrosis during wound healing. CAFs are activated fibroblasts, which are the main components of TME. CAFs are distinct from normal fibroblasts in terms of structure and function. They are larger with serrated nuclei and branched cytoplasm, and possess greater proliferative, migratory and secretory abilities as well as increased metabolic activity (Glabman et al., 2022). It has been shown that CAFs can be derived from 1) fibroblasts, 2) mesenchymal stem cells, 3) epithelial-mesenchymal transition (EMT), 4) endothelial-mesenchymal transition (EndMT) of resident endothelial cells, and 5) transformation of adipocytes, pericytes, and smooth muscle cells (Rimal et al., 2022). Its highly heterogeneous cellular origin determines the heterogeneity of the CAFs population, which affects their phenotype and function. In CRC, CAFs are mainly derived from peripheral fibroblasts and bone marrow-derived mesenchymal stem cells (Figure 1). However, the underlying molecular mechanisms governing the differentiation of precursor cells into CAFs remain poorly understood.
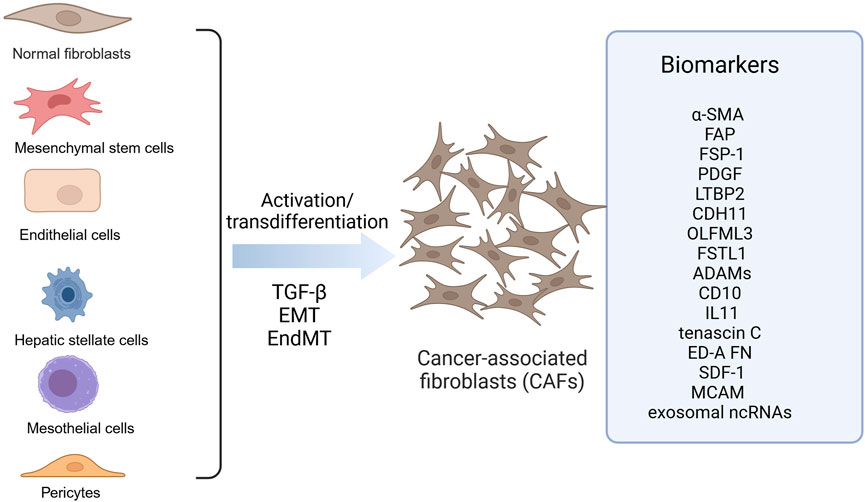
Figure 1. Origin of CAFs in colorectal cancer. CAFs in colorectal cancer are derived from diverse precursor cells through activation or transdifferentiation, including fibroblasts, mesenchymal stem cells, endothelial cells, hepatic stellate cells, mesothelial cells, pericytes. These CAFs express different biomarkers like a-SMA, FAP and FSP-1. ADAMS, A disintegrin and metalloproteinases; a-SMA, alpha-smooth muscle actin; CAFs, Cancer-associated fibroblasts; CDH11, Cadherin 11; ED-A FN, Fibronectin ED-A domain; FAP, Fibroblast activation protein α; FSP-1, Fibroblast specific protein-1; FSTL1, Follistatin-like 1; LTBP2, Latent TGF-b–binding protein 2; MCAM, Melanoma cell adhesion molecule; OLFML3, Olfactomedin-like 3; PDGF, Platelet-derived growth factor receptor; SDF-1, Stromal cell-derived factor 1. Image created with BioRender.com, with permission.
2.1.1 Fibroblasts
In CRC, fibroblasts are the predominant source of CAFs. Researches have demonstrated that the integrin α-v-β6 expressed by tumor cells is capable of activating latent forms of transforming growth factor (TGF)-β, a well-known stimulator that induces the differentiation of quiescent fibroblasts into CAFs in TME (Hawinkels et al., 2014). Another TGF superfamily member, Nodal, has recently been shown to be positively correlated with alpha-smooth muscle actin (α-SMA) expression in CRC tissues, which can be secreted by tumor cells and promotes the transformation of normal fibroblasts into CAFs through the activation of the TGF-β/Smad/Snail pathway, thereby promoting CRC cell proliferation (Li et al., 2019). Some evidence also suggests that Snail-positive fibroblasts have CAF properties, further supporting that Snail is an important regulator of CAF formation from fibroblasts. And snail is a downstream target gene of TGF-β that mediates the tumor-promoting effects of TGF-β (Moon et al., 2017), and this gene is also a key factor in the tumor-promoting effects of fibroblasts in CRC (Herrera et al., 2014), so it is reasonable to hypothesize that Nodal promotes the progression of CRC by mediating the formation of CAFs through the Snail signaling pathway. In addition to Nodal, interleukin (IL) 34 overexpression in CRC cells is also involved in the transition of normal fibroblasts to CAFs (Franze et al., 2020). Except for CRC cells, the tumor mesenchyme also has a key role in the differentiation of fibroblasts into CAFs. For example, increased expression of matrix metalloproteinase inhibitor-1 (TIMP-1) promotes the formation of CAFs of fibroblast origin in CRC (Gong et al., 2013). Additionally, Dickkopf-3 (DKK-3), which is located in the stroma, has been demonstrated to activate the wingless/integrated (Wnt) and yes-associated protein/transcriptional co-activator with PDZ-binding motif (YAP/TAZ) signaling pathway, thereby leading to the formation of CAFs in CRC (Ferrari et al., 2019). Furthermore, deletion in protein kinase Cζ matrix has been found to be strongly correlated with CAFs stimulating tumor progression (Kasashima et al., 2021). Therefore, both tumor cells and mesenchyme in TME can promote the transformation of fibroblasts into CAFs.
2.1.2 Mesenchymal stem cells (MSCs)
In addition to fibroblasts, MSCs derived from bone marrow have been extensively studied as another precursor of CAFs. These MSCs can migrate to the tumor stroma and differentiate into CAFs. It has been reported that CRC cells induce MSCs to differentiate into CAFs through cell-cell contact, which is mediated by Notch-Jagged1 and TGF-β/Smad signaling pathway (Peng et al., 2014). A recent study found that many α-SMA-positive CAFs in CRC arise from the proliferation of intestinal pericellular stromal cells (Lepr + cells), and Lepr is a well-established marker for perivascular mesenchymal cells, which support bone marrow hematopoietic stem cell maintenance (Kobayashi et al., 2022).
2.1.3 Endothelial cells (ECs)
ECs can be converted into CAF by EndMT process. One study showed that invasive CRC cells induce the conversion of endothelial cells into CAFs through the upregulation and phosphorylation of tubulin-β3, which was mainly dependent on TGF-β stimulation (Wawro et al., 2018).
2.1.4 Hepatic stellate cells
One study found that exosomes from CRC cells can stimulate hepatic stellate cells to become CAFs when liver metastasis of colon cancer occurs (C. Zhang et al., 2022). And a recent study also showed that CRC cells are able to interact with hepatic stellate cells to promote the secretion of stromal cell-derived factor 1 (SDF 1) and bind to C-X-C chemokine receptor type 4 (CXCR4) and induce the expression and secretion of TGF-β in CRC cells, which ultimately leads to the differentiation of hepatic stellate cells into CAFs, whereas the blockade of the CXCR4/TGF-β pathway inhibits the differentiation of hepatic CAFs and the metastasis of CRC to the liver (Tan et al., 2020).
2.1.5 Mesothelial cells (MCs)
In CRC with deep infiltration near the serosa and peritoneal metastases, a considerable proportion of CAFs are derived from MCs. MCs gradually lose their mesothelial cell markers through mesothelial-to-mesenchymal transition (MMT) and approache myofibroblast morphology (Demuytere et al., 2020). Another histological observational study showed that MCs in locally advanced primary colorectal cancer also differentiated into CAFs via MMT (Type II EMT) (Gordillo et al., 2020), and RNA sequencing analysis showed that TGF-β was associated with MMT (Rynne-Vidal et al., 2017); therefore, whether MCs also differentiate by activating TGF-β needs to be further investigated.
2.1.6 Pericytes
A study using single-cell RNA sequencing to analyze the transcriptome of primary tumors, matched liver metastases, and individual cells of peripheral blood from six patients with liver metastases from CRC revealed that contractile CAFs highly expressing pericyte-associated markers (e.g., RGS5 and CSPG4), largely were originate from pericytes (Che et al., 2021).
2.2 Biomarkers of CAFs in CRC
To identify and isolate CAFs from the fibroblast population throughout the body, markers such as α-SMA, fibroblast activation protein α (FAP), fibroblast specific protein-1 (FSP-1 or S100A4), vimentin, and platelet-derived growth factor receptor (PDGF) are commonly employed (Han et al., 2020; Nurmik et al., 2020). Despite the fact that CAFs originate from a variety of sources, they are not a unified group, but rather a subset of cells with varying spatial, phenotypic, and functional characteristics, thus, these biomarkers lack specificity. It has been proposed that a number of molecules may be used as biomarkers of CAFs in CRC. Proteomic analysis of normal fibroblasts and CAFs in colon tissue has revealed that latent TGF-b–binding protein 2 (LTBP2), cadherin 11 (CDH11), Olfactomedin-like 3 (OLFML3) and Follistatin-like 1 (FSTL1) may be used as biomarkers (Torres et al., 2013). Furthermore, increased expression of a disintegrin and metalloproteinases (ADAMs), such as ADAM9, ADAM10, ADAM12, and ADAM17, has been observed in CAFs found in colon tissues of CRC patients (Mochizuki et al., 2020). Additionally, CD10 (Zhu et al., 2016) and IL11 (Nishina et al., 2021) have also been suggested as potential markers of CAFs in CRC. In addition, a study of high-throughput differential secretome analysis of CAFs in colon cancer and non-cancer-activated bone marrow-derived MSC revealed that tenascin C, fibronectin ED-A domain and SDF-1 may also be biomarkers for CAFs in CRC (De Boeck et al., 2013). αSMA + CAFs express melanoma cell adhesion molecule (MCAM) derived from Lepr + cells, suggesting that MCAM can be used as a biomarker for αSMA + CAFs(Kobayashi et al., 2022). Interestingly, by performing the next-generation sequencing, a significant number of non-coding RNAs (ncRNAs) in exosomes were also found as potential biomarkers present in CAFs-derived exosomes (Herrera et al., 2018). It is worth noting that more studies are needed to validate whether these candidate molecules can be specific biomarkers for CAFs in CRC (Figure 1).
2.3 CAFs subtypes in CRC
The above biomarkers, such as α-SMA, FAP are commonly used to identify CAFs. However, labeling of CAFs is likely to be more complex, because it is now clear that CAFs are also characterized by increased heterogeneity (Koliaraki et al., 2017). CAFs may have multiple subtypes, which have different functions (Su et al., 2018). Recent progress in single-cell RNA-sequencing technologies has enabled detailed characterization of the complexity and heterogeneity of CAF subpopulations in multiple tumor types. Li et al. performed single-cell RNA sequencing of tumors and their microenvironments from samples derived from normal intestinal mucosa and CRC, and identified two distinct subtypes of CAFs, CAF-A and CAF-B. CAF-A cells express genes related to extracellular matrix remodeling and may be a separate subtype or an intermediate state between normal fibroblasts and CAF-B cells, while CAF-B cells express cytoskeletal genes and other known markers of fibroblasts (Li et al., 2017). In addition, Giguelay et al. identified two main subtypes of CAFs in patients with liver metastases from CRC, contractile CAFs (ctr-CAFs) and ECM remodeling/promoter angiogenesis CAFs (ECM-CAFs). And further analysis revealed that ECM remodeling/promoter angiogenesis CAFs were derived from intrinsic fibroblasts of the hepatic portal area (Giguelay et al., 2022). Chen LH et al. classified CAFs into three major subsets, including secretory CAFs, ECM-remodeling CAFs and contractile CAFs. Secretory CAFs highly express secretory proteins, such as various growth factors (e.g., Insulin-like growth factor 1(IGF1), Platelet-derived growth factor D (PDGFD), fibroblast growth factor (FGF) seven and Vascular endothelial growth factor B (VEGFB)) that mediate angiogenesis and cancer cell proliferation. The ECM-remodeling CAFs highly express ECM proteins (such as ECM collagens and fibronectin) and ECM proteases which alter ECM structure and assist tumor angiogenesis and metastasis. The contractile CAFs are enriched for genes involved in the regulation of cell contraction, suggesting some distinct phenotypes (Che et al., 2021). Chen SJ et al. identified five subtypes of CAFs from CRC animal model, then found that bifidobacterium adolescentis orchestrates CD143+ cancer-associated fibroblasts to suppress colorectal tumorigenesis (Chen et al., 2023). These studies revealed the diversity of CAFs in CRC and their complex functional properties, and that different subtypes of CAFs may originate from different precursor cells and perform different functions in TME, even have opposing capabilities such as tumor restraining vs tumor promoting (Table 1). Because of this, it is crucial to characterize CAFs as different subtypes based on their functional heterogeneity. In addition, while some studies have proposed potential precursor cells for specific CAFs subtype, their precise cellular identity remains elusive. Therefore, more studies need to continue to explore the origin, subtypes, and functions of CAFs in CRC and the relationship between them through the integration of cutting-edge techniques, including single-cell sequencing, spatial transcriptomics, lineage tracing method and advanced imaging techniques.
3 The role of CAFs in CRC invasion and metastasis
Comprehending the progression of events after cancer metastasis is of utmost importance, as it is often associated with a poor patient prognosis. The cancer metastasis cascade can be categorized into five steps: invasion, intravesation, circulation, extravasation, and colonization. Upon activation, CAFs can release a range of soluble factors that can enhance tumor invasion and metastasis (Linares et al., 2020), such as TGF-β, hepatocyte growth factor (HGF), epidermal growth factor (EGF), FGF and IL-6 cytokine (Owusu et al., 2017; Woolston et al., 2019; Zeng et al., 2017). These soluble factors can influence cancer invasiveness and metastasis by remodeling the ECM, regulating EMT in cancer cells, suppressing immunity, promoting angiogenesis, regulating cancer cell metabolism and metastasis-related signaling pathways (Figure 2).
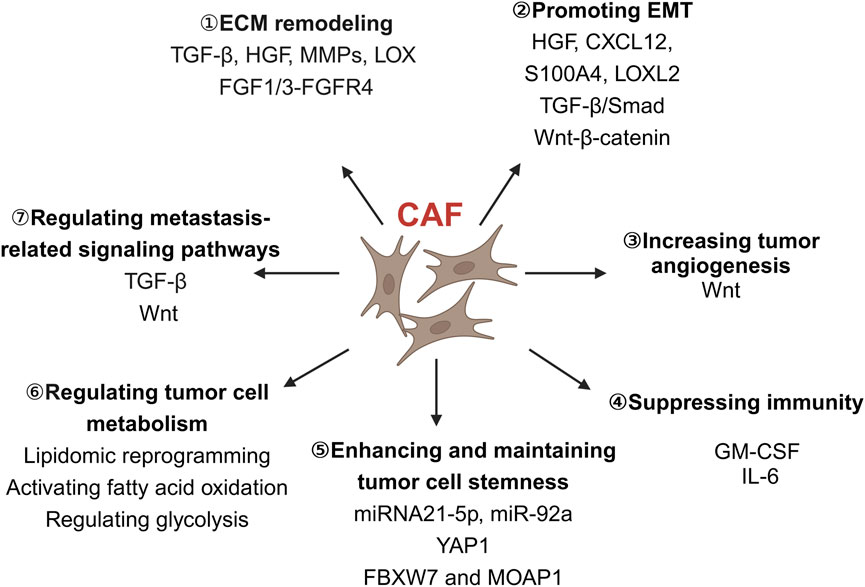
Figure 2. The role of CAFs in colorectal cancer invasion and metastasis. CAFs play a diverse role in colorectal cancer cell invasion and metastasis, including remodeling the ECM, promoting EMT, increasing tumor angiogenesis, suppressing immunity, enhancing and maintaining tumor cell stemness, regulating tumor cell metabolism and metastasis-related signaling pathways. CAFs, Cancer-associated fibroblasts; CXCL12, C-X-C chemokine ligand 12; ECM, Extracellular matrix; EMT, Epithelial-mesenchymal transition; FBXW7, F-Box and WD repeat domain containing 7; FGF, Fibroblast growth factor; GM-CSF, Granulocyte-macrophage colony-stimulating factor; HGF, Hepatocyte growth factor; IL-6, Interleukin-6; LOX, Lysyl oxidase; LOXL2, Lysyl oxidase-like 2; MMPs, Matrix metalloproteinases; MOAP1, Modulator of apoptosis 1; S100A4, S100 calcium-binding protein A4; TGF-β, Transforming growth factor-β; Wnt, Wingless/integrated; YAP1, Yes-associated protein 1. Image created with BioRender.com, with permission.
3.1 CAFs remodel the ECM
TME is composed of cellular and non-cellular components, the latter of which is referred to as the ECM. ECM is made up of structural proteins, collagen, proteoglycans, hyaluronic acid, and glycosaminoglycans, which provide structural and signaling support for cells and tissues. Research has demonstrated that the structure and function of the ECM can be altered by cells in the TME, which has a significant impact on cancer metastasis (Li et al., 2020). The fundamental elements for ECM remodeling are TGF-β, HGF, and particular interleukins and Matrix metalloproteinases (MMPs). Studies on CRC have revealed that MMPs have a considerable impact on the ECM composition. MMPs are a family of enzymes that can disintegrate the basement membrane and almost all ECM elements. They are critical for the degradation of ECM involved in tumor cell invasion, metastasis, and angiogenesis. MMP-7 is the only and smallest enzyme in the MMPs family that is specifically expressed by tumor cells. Studies have indicated that it is more important than other members in the MMPs family in terms of breaking down and degrading the ECM and basement membrane on the tumor surface, as well as aiding angiogenesis, hastening tumor cell invasion and metastasis. And it is significantly expressed in liver metastasis or lymph node of colon cancer (Bi et al., 2013). A study showed that CAFs can upregulate MMP-7 expression in colon cancer cells through the FGF1/3-FGFR4 signaling pathway, resulting in the progression of CRC (Bai et al., 2015). That is, CAFs can create passages in the ECM by breaking down the matrix to enable CRC cells to migrate from the original site and metastasize. Several studies have shown that the stiffness of the ECM is positively correlated with cancer invasion and metastasis. In CRC, stiffer ECM increases activin A secretion in stromal cells, which in turn induces invasion via the EMT-related protein Snail (Bauer et al., 2020). CAFs can both degrade the ECM to create pathways for cancer cell migration, and increase ECM stiffness to further promote cancer invasion and metastasis (Bauer et al., 2020; Gkretsi and Stylianopoulos, 2018; Kalli and Stylianopoulos, 2018). This two seemingly contradictory process may be linked to the diverse origins and heterogeneity of CAFs(Asif et al., 2021). Furthermore, activated stromal cells have the ability to produce lysyl oxidase (LOX), which is responsible for the formation of collagen cross-linking. This in turn increases matrix stiffness and cancer cell invasion (Pankova et al., 2016; Sahai et al., 2020) and it has been found that lysyl oxidase-like 2 (LOXL2) is overexpressed in CAFs of CRC, which could be used as a marker for predicting the prognosis of colon cancer patients (Torres et al., 2015). Targeting the LOX oxidase produced by CAFs has been proposed as a potential approach to combat cancer metastasis (Liu et al., 2019). Overall, By understanding and modulating the complex interactions between CAFs, ECM, and CRC cells, researchers can develop novel approaches to disrupt the supportive environment that fosters CRC progression and metastasis.
3.2 CAFs promote EMT
Cancer metastasis involves a key step of ECM degradation, which is the acquisition of a mesenchymal phenotype. This is achieved through a process called EMT, where certain mesenchymal markers such as vimentin and fibronectin are upregulated, and epithelial junction proteins such as E-cadherin and occludin are downregulated. As a result, the intercellular adhesion junctions become weak, promoting the migration and invasion of tumor cells (Asif et al., 2021). Recent studies have indicated that CAFs can stimulate EMT of tumor cells which can facilitate distant metastasis. Normal human colon fibroblasts suppress EMT of colon cancer cells and Wnt-β-catenin signaling pathway activation, decreasing the migration and invasion capability of CRC cells. In contrast, CAFs are able to stimulate the secretion of Wnt4 and Wnt5 in CRC cells, thus activating the classical Wnt-β-catenin signaling pathway to induce EMT, which can result in the progression of CRC (Hu et al., 2019; Yang et al., 2020). Wanadi et al. demonstrated that CAFs can induce EMT of HT29 colon cancer cells through the secretion of HGF, thereby increasing their invasion and migration capabilities (Wanandi et al., 2021). In addition, Verification of other CAFs secreted factors involved in EMT has also been reported in CRC such as C-X-C chemokine ligand 12 (CXCL12), S100A4, and LOXL2 (Asif et al., 2021). Notably, CXCL12 (also known as SDF1) is a powerful activator of EMT which can induce this process via an over-activation of Wnt/β-catenin pathway via CXCR4/CXCL12 (Hu et al., 2014). And CXCR4/CXCL12 are believed to be potential therapeutic targets for CRC (Khare et al., 2021). S100A4 is a metastatic protein, also considered as an EMT-promoting protein, which is strongly associated with poor prognosis in CRC and promotes tumor cell progression and metastasis by down-regulating E-cadherin and modulating the EMT mesenchymal phenotype in epithelial cells (Fei et al., 2017). CAFs also stimulate EMT in colon cancer LOVO cells to promotes colon cancer metastasis through activating the focal adhesion kinase (FAK) signaling pathway by secreting LOXL2 (Xuefeng et al., 2020). Furthermore, CAFs can regulate EMT to promote metastasis by releasing exosomes, such as miRNA-181a, which can activate the TGF-β/Smad pathway and influence the EMT of colon cancer (Chao, 2020). Additionally, CAFs can directly transfer their own exosomes to CRC cells, which increases the level of miR-92a-3p in CRC cells. This can inhibit mitochondrial apoptosis and activate the Wnt/β-catenin pathway by suppressing F-Box and WD repeat domain containing 7 (FBXW7) and modulator of apoptosis 1 (MOAP1), thus promoting the EMT and metastasis of tumor cells (Hu et al., 2019). Similarly, exosomes miR-625-3p and LINC00659 also promote CRC cell migration, invasion, and EMT by inhibiting the Elav-like family member 2/WW domain-containing oxidoreductase (CELF2/WWOX) pathway and interacting directly with miR-342-3p, respectively (Zhang et al., 2022b; Zhou et al., 2021). In addition, IL-6 positively correlates with tumor TNM stage and is associated with depth of tumor infiltration and lymph node metastasis in CRC (Zeng et al., 2017). And it upregulates the expression of integrin β6 through the IL-6 receptor/signal transducer and activator of transcription (STAT)-3 signaling pathway, which promotes EMT and invasiveness of CRC cells (Sun et al., 2020). In summary, CAFs play a significant role in promoting CRC metastasis by inducing EMT through various molecular mechanisms. Understanding these processes could provide insights into potential therapeutic targets for CRC treatment.
3.3 CAFs increase tumor angiogenesis
Tumor cells are more proliferative than normal cells (De Palma et al., 2017). Therefore, tumor growth necessitates a heightened blood supply to meet the elevated demands for oxygen and nutrients. In human CRC, Wnt2 is preferentially upregulated in CAFs, which promotes metastasis and invasion. Wnt2 exhibits a pro-angiogenic role in the development of placental vasculature, stimulating angiogenesis within liver sinusoidal ECs and other ECs. In a comparative study between normal colon and CRC, CAFs were found to be the main producers of stromal Wnt2 in the stromal and epithelial compartments (Kramer et al., 2017). Wnt2 expression in CAFs resulted in the autocrine activation of canonical Wnt signaling and increased motility of fibroblasts, which positively affected the invasive and metastatic potential of CRC (Unterleuthner et al., 2020). Targeting Wnt2 or its signaling pathways could potentially be a promising strategy for CRC therapy.
3.4 CAFs suppress immunity
CAFs interact with most cells in the TME (Farhood et al., 2019), including various types of immune cells. Tumor-associated macrophages are the most abundant immune cells in the TME. Macrophages are usually classified into two groups: M1 and M2. The M1 phenotype is a pro-inflammatory cell type that has been shown to exhibit antitumor activity, while the M2 phenotype is an immunosuppressive cell type that promotes tumor progression. Schellerer et al. (2014) reported that CAFs express higher levels of intercellular adhesion molecule-1 (ICAM-1) and a higher affinity for attracting monocytes to infiltrate cancer tissues. Meiyun’research (Wang et al., 2020) further elucidates the role of CAFs in this process. They found that CAFs secrete exosomes rich in granulocyte-macrophage colony-stimulating factor (GM-CSF) and IL-6. These factors promote the differentiation of monocytes into M2 macrophages, and activate M2 macrophages to release chemokines and exosomes, thereby enhancing angiogenesis in CRC and promoting tumor metastasis. In conclusion, CAFs actively influence the TME by modulating the polarization of macrophages. This ultimately aids in the development and spread of CRC by stimulating angiogenesis and suppressing the immune system.
3.5 CAFs enhance and maintain tumor cell stemness
Cancer stem cells (CSCs) are closely related to tumor metastasis. CSCs are a class of tumor cells with the ability to self-renew and differentiate. Several studies have found that the tumor cell stemness is been increased by exosomes secreted from CAFs, which have been also found to be effectively taken up by tumor cells, thereby resulting in the promotion of proliferation, invasion, and metastasis of cancer cells (Chen et al., 2021a). For example, miRNA21-5p expressed in CAF-derived exosomes increases the proportion of CSCs and enhances the invasion and metastasis of cancer cells by regulating YAP1 protein. Similarly, exosomal miR-92a maintains the stemness of CRC cells and promotes their invasion and metastasis, and induces resistance to 5-FU/L-OHP by targeted inhibition of FBXW7 and MOAP1 (Hu et al., 2019). Therefore, It has been suggested that the treatment of cancer should not only focus on CSCs, but also inhibit the secretion of CAFs, in order to maximize the benefits for patients (Chen et al., 2021b).
3.6 CAFs regulate tumor cell metabolism
It has been recently discovered that CAFs can also regulate cancer cell metabolism (Li et al., 2021). Activated CAFs have been observed to promote metabolic switching in CRC cells, resulting in increased organ metastases as a result of glutamine depletion. Furthermore, CAFs undergo lipidomic reprogramming, resulting in increased production and secretion of fatty acids. These fatty acids are then taken up by CRC cells, enhancing their migratory capabilities (Gong et al., 2020). This process not only supports the growth of primary tumors but also contributes to the formation of distant metastases. CAFs also regulate the metabolism of CRC cells by activating fatty acid oxidation and regulating glycolysis (Peng et al., 2021). This shift in metabolism may give cancer cells the energy and resources they need to survive and proliferate, which can accelerate the disease’s progression. Excitingly, interventions targeting the metabolic activity of CAFs have shown promise in restraining CRC progression. For instance, the use of catechin to inhibit the aerobic glycolysis activity of CAFs has been found to inhibit the migration and invasion ability of colon cancer cell lines like HCT-116 and HT29 (Chen et al., 2022). These discoveries highlight the significant role of CAFs in shaping the metabolic landscape of CRC. Targeting the metabolic activities of CAFs presents promising avenues for therapeutic intervention in restraining CRC metastasis.
3.7 CAFs regulate metastasis-related signaling pathways
Some soluble factor secreted by CAFs play crucial roles in regulating cancer metastasis by modulating specific signaling pathways. One such factor is TGF-β, a key member of the TGF superfamily. TGF-β is known to play a pivotal role in mediating the interaction between tumor cells and CAFs. Research suggests that TGF-β can induce the differentiation of normal fibroblasts into CAFs, and these CAFs, in turn, secrete more TGF-β to maintain and increase the activation of fibroblasts (Hawinkels et al., 2014). And the TGF-β can modulate the expression of various EMT markers, such as increased vimentin level and decreased E-cadherin expression, These changes facilitate the transition of tumor cells to a more invasive phenotype, promoting metastasis (Yu et al., 2014). Moreover, at metastatic sites, TGF-β-stimulated CAFs secrete IL-11, which activates the glycoprotein 130-signal transducer and activator of transcription 3 (GP130-STAT3) signaling pathway in tumor cells, this activation enhances the metastasis of CRC cells, ultimately promoting the organ colonization of metastatic CRC (Itatani et al., 2019). Another key factor is Wnt2, a protein secreted by CAFs. Wnt2 activates the classical Wnt pathway in fibroblasts, increases the migration and invasion of CRC cells, and acts in an autocrine manner to promote CRC (Kramer et al., 2017). To summarize, CAFs secrete TGF-β and Wnt2, which in turn trigger a cascade of signaling events that promote CRC cell invasion, migration, and EMT, ultimately leading to metastasis. Targeting these CAF-secreted factors and the metastasis-related signaling pathways could be a promising strategy for inhibiting CRC progression and metastasis.
4 Summary and perspectives
CRC is the third most common cause of cancer-related deaths worldwide (Biller and Schrag, 2021). Once metastasized, treatment strategies can be limited, and, if unresectable, outcomes in CRC patients are unfavorable. CAFs, as one of the important components of TME, are considered a crucial therapeutic target due to their involvement in the genesis and progression of tumors (Tauriello, 2023). At present, there have been a number of clinical trials evaluating the therapeutic effects of some drugs targeting biomarkers of CAFs for different cancers, but only two for CRC, and few of these drugs have provided promising results (Zhou and Zheng, 2024). As mentioned above, the origin of CAFs is very complex, resulting many subtypes with different biological functions in tumor progression, including remodeling the ECM, promoting EMT, increasing tumor angiogenesis, suppressing immunity, enhancing and maintaining tumor cell stemness, regulating tumor cell metabolism and metastasis-related signaling pathways. These processes in which CAFs participate in cancer metastasis are not independent and may occur simultaneously. These findings highlight the need for a more comprehensive understanding of the origin and role of CAFs in CRC.
The field faces a significant controversy surrounding the dual nature of CAFs, which typically exhibit tumor-promoting properties but can also exhibit potential tumor-suppressive functions. This dualism poses a substantial challenge for targeted therapy, as it necessitates the ability to selectively target the tumor-promoting CAFs without inadvertently disrupting their potential tumor-suppressive CAFs. Enhancing our understanding of CAF subtypes holds the key to overcoming this challenge more effectively.
While advances have been made in the study of CAFs, there are still several knowledge gaps that need to be addressed. The precise molecular pathways underlying the differentiation of CAFs from their diverse precursor cells have yet to be fully elucidated. Additionally, given high heterogeneity of CAFs, there is currently no single biomarker that is completely specific for identifying and isolating CAFs from tumor tissues, and identifying reliable biomarkers to distinguish different CAFs subtypes remains a critical challenge. Moreover, the correlation between the origins of CAFs in CRC and their impact on CAFs function is still uncertain. Addressing these gaps, which future research should focus on, is essential for enhancing our understanding of CAF roles and develop effective therapeutic strategies. Theoretically, CAF heterogeneity and their multifunctional roles should be deeperly understood. Technically, the development of more advanced in vivo models that closely mimic the human TME is crucial for elucidating the origin and function of CAFs. Such models will be invaluable for studying the dynamic interactions between CAFs and other components of the tumor stroma. Moreover, the development of highly specific biomarkers for different CAF subtypes is essential for the improvement targeted therapies.
In conclusion, CAFs, characterized by their high heterogeneity and diverse functions, play vital roles in the invasion and metastasis of CRC. As novel techniques like scRNA-seq continue to advance, the comprehensive characterisation of CAFs will become increasingly clear. This deeper understangding will pave the way for the development of new strategies specifically targeting CAFs, offering promising avenues for CRC treatment in the future.
Author contributions
JY: Conceptualization, Writing–original draft. WZ: Writing–original draft. SF: Writing–original draft. PY: Supervision, Writing–review and editing, Funding acquisition. SQ: Conceptualization, Funding acquisition, Writing–review and editing.
Funding
The author(s) declare financial support was received for the research, authorship, and/or publication of this article. This research was financially supported by the 2020 Guangdong Provincial Science and Technology Innovation Strategy Special Fund (Guangdong-Hong Kong-Macau Joint Lab, 2020B1212030006), Guangdong Provincial key research and development program (2020B1111100010), Guangzhou Science and Technology Planning Project (202201020288), The project of Guangdong Provincial Key Laboratory of Clinical Research on Traditional Chinese Medicine Syndrome (2023KT15492), Special Project of State Key Laboratory of Dampness Syndrome of Chinese Medicine (SZ2021ZZ13), Science and Technology Planning Project of Guangdong Province (2023B1212060063), National Natural Science Foundation of China (81971742).
Conflict of interest
The authors declare that the research was conducted in the absence of any commercial or financial relationships that could be construed as a potential conflict of interest.
Publisher’s note
All claims expressed in this article are solely those of the authors and do not necessarily represent those of their affiliated organizations, or those of the publisher, the editors and the reviewers. Any product that may be evaluated in this article, or claim that may be made by its manufacturer, is not guaranteed or endorsed by the publisher.
References
Asif, P. J., Longobardi, C., Hahne, M., and Medema, J. P. (2021). The role of cancer-associated fibroblasts in cancer invasion and metastasis. Cancers 13 (18), 4720. doi:10.3390/cancers13184720
Bai, Y. P., Shang, K., Chen, H., Ding, F., Wang, Z., Liang, C., et al. (2015). Fgf-1/-3/fgfr4 signaling in cancer-associated fibroblasts promotes tumor progression in colon cancer through erk and mmp-7. Cancer Sci. 106 (10), 1278–1287. doi:10.1111/cas.12745
Bauer, J., Emon, M., Staudacher, J. J., Thomas, A. L., Zessner-Spitzenberg, J., Mancinelli, G., et al. (2020). Increased stiffness of the tumor microenvironment in colon cancer stimulates cancer associated fibroblast-mediated prometastatic activin a signaling. Sci. Rep. 10 (1), 50. doi:10.1038/s41598-019-55687-6
Bi, Z., Dong, L. D., and Gu, X. M. (2013). Clinical significance of mmp-7 and pten expression in colorectal cancer. Hepatogastroenterology 60 (121), 32–36. doi:10.5754/hge12537
Biffi, G., and Tuveson, D. A. (2021). Diversity and biology of cancer-associated fibroblasts. Physiol. Rev. 101 (1), 147–176. doi:10.1152/physrev.00048.2019
Biller, L. H., and Schrag, D. (2021). Diagnosis and treatment of metastatic colorectal cancer: a review. JAMA-J. Am. Med. Assoc. 325 (7), 669–685. doi:10.1001/jama.2021.0106
Chao, G. (2020). Experimental study of tumor-associated fibroblast exosome mirna-181a affecting colon cancer proliferation and epithelial-mesenchymal transition through the tgf-β/smad pathway. Ph.D. dissertation. Tianjin: Tianjin Medical University. doi:10.27366/d.cnki.gtyku.2020.000153
Che, L. H., Liu, J. W., Huo, J. P., Luo, R., Xu, R. M., He, C., et al. (2021). A single-cell atlas of liver metastases of colorectal cancer reveals reprogramming of the tumor microenvironment in response to preoperative chemotherapy. Cell Discov. 7 (1), 80. doi:10.1038/s41421-021-00312-y
Chen, B., Sang, Y., Song, X., Zhang, D., Wang, L., Zhao, W., et al. (2021a). Exosomal mir-500a-5p derived from cancer-associated fibroblasts promotes breast cancer cell proliferation and metastasis through targeting usp28. Theranostics 11 (8), 3932–3947. doi:10.7150/thno.53412
Chen, S., Fan, L., Lin, Y., Qi, Y., Xu, C., Ge, Q., et al. (2023). Bifidobacterium adolescentis orchestrates cd143(+) cancer-associated fibroblasts to suppress colorectal tumorigenesis by wnt signaling-regulated gas1. Cancer Commun. 43 (9), 1027–1047. doi:10.1002/cac2.12469
Chen, S., Nishi, M., Morine, Y., Shimada, M., Tokunaga, T., Kashihara, H., et al. (2022). Epigallocatechin-3-gallate hinders metabolic coupling to suppress colorectal cancer malignancy through targeting aerobic glycolysis in cancer-associated fibroblasts. Int. J. Oncol. 60 (2), 19. doi:10.3892/ijo.2022.5309
Chen, X., and Song, E. (2019). Turning foes to friends: targeting cancer-associated fibroblasts. Nat. Rev. Drug Discov. 18 (2), 99–115. doi:10.1038/s41573-018-0004-1
Chen, Y., McAndrews, K. M., and Kalluri, R. (2021b). Clinical and therapeutic relevance of cancer-associated fibroblasts. Nat. Rev. Clin. Oncol. 18 (12), 792–804. doi:10.1038/s41571-021-00546-5
De Boeck, A., Hendrix, A., Maynard, D., Van Bockstal, M., Daniels, A., Pauwels, P., et al. (2013). Differential secretome analysis of cancer-associated fibroblasts and bone marrow-derived precursors to identify microenvironmental regulators of colon cancer progression. Proteomics 13 (2), 379–388. doi:10.1002/pmic.201200179
Demuytere, J., Ceelen, W., Van Dorpe, J., and Hoorens, A. (2020). The role of the peritoneal microenvironment in the pathogenesis of colorectal peritoneal carcinomatosis. Exp. Mol. Pathol. 115, 104442. doi:10.1016/j.yexmp.2020.104442
De Palma, M., Biziato, D., and Petrova, T. V. (2017). Microenvironmental regulation of tumour angiogenesis. Nat. Rev. Cancer. 17 (8), 457–474. doi:10.1038/nrc.2017.51
Farhood, B., Najafi, M., and Mortezaee, K. (2019). Cancer-associated fibroblasts: secretions, interactions, and therapy. J. Cell. Biochem. 120 (3), 2791–2800. doi:10.1002/jcb.27703
Fei, F., Qu, J., Zhang, M., Li, Y., and Zhang, S. (2017). S100a4 in cancer progression and metastasis: a systematic review. Oncotarget 8 (42), 73219–73239. doi:10.18632/oncotarget.18016
Ferrari, N., Ranftl, R., Chicherova, I., Slaven, N. D., Moeendarbary, E., Farrugia, A. J., et al. (2019). Dickkopf-3 links hsf1 and yap/taz signalling to control aggressive behaviours in cancer-associated fibroblasts. Nat. Commun. 10 (1), 130. doi:10.1038/s41467-018-07987-0
Franze, E., Di Grazia, A., Sica, G. S., Biancone, L., Laudisi, F., and Monteleone, G. (2020). Interleukin-34 enhances the tumor promoting function of colorectal cancer-associated fibroblasts. Cancers 12 (12), 3537. doi:10.3390/cancers12123537
Giguelay, A., Turtoi, E., Khelaf, L., Tosato, G., Dadi, I., Chastel, T., et al. (2022). The landscape of cancer-associated fibroblasts in colorectal cancer liver metastases. Theranostics 12 (17), 7624–7639. doi:10.7150/thno.72853
Gkretsi, V., and Stylianopoulos, T. (2018). Cell adhesion and matrix stiffness: coordinating cancer cell invasion and metastasis. Front. Oncol. 8, 145. doi:10.3389/fonc.2018.00145
Glabman, R. A., Choyke, P. L., and Sato, N. (2022). Cancer-associated fibroblasts: tumorigenicity and targeting for cancer therapy. Cancers 14 (16), 3906. doi:10.3390/cancers14163906
Gong, J., Lin, Y., Zhang, H., Liu, C., Cheng, Z., Yang, X., et al. (2020). Reprogramming of lipid metabolism in cancer-associated fibroblasts potentiates migration of colorectal cancer cells. Cell Death Dis. 11 (4), 267. doi:10.1038/s41419-020-2434-z
Gong, Y., Scott, E., Lu, R., Xu, Y., Oh, W. K., and Yu, Q. (2013). Timp-1 promotes accumulation of cancer associated fibroblasts and cancer progression. PLoS One 8 (10), e77366. doi:10.1371/journal.pone.0077366
Gordillo, C. H., Sandoval, P., Munoz-Hernandez, P., Pascual-Anton, L., Lopez-Cabrera, M., and Jimenez-Heffernan, J. A. (2020). Mesothelial-to-mesenchymal transition contributes to the generation of carcinoma-associated fibroblasts in locally advanced primary colorectal carcinomas. Cancers 12 (2), 499. doi:10.3390/cancers12020499
Han, C., Liu, T., and Yin, R. (2020). Biomarkers for cancer-associated fibroblasts. Biomark. Res. 8 (1), 64. doi:10.1186/s40364-020-00245-w
Hawinkels, L. J., Paauwe, M., Verspaget, H. W., Wiercinska, E., van der Zon, J. M., van der Ploeg, K., et al. (2014). Interaction with colon cancer cells hyperactivates TGF-β signaling in cancer-associated fibroblasts. Oncogene 33 (1), 97–107. doi:10.1038/onc.2012.536
Herrera, A., Herrera, M., Alba-Castellon, L., Silva, J., Garcia, V., Loubat-Casanovas, J., et al. (2014). Protumorigenic effects of snail-expression fibroblasts on colon cancer cells. Int. J. Cancer. 134 (12), 2984–2990. doi:10.1002/ijc.28613
Herrera, M., Llorens, C., Rodriguez, M., Herrera, A., Ramos, R., Gil, B., et al. (2018). Differential distribution and enrichment of non-coding rnas in exosomes from normal and cancer-associated fibroblasts in colorectal cancer. Mol. Cancer. 17 (1), 114. doi:10.1186/s12943-018-0863-4
Hu, J. L., Wang, W., Lan, X. L., Zeng, Z. C., Liang, Y. S., Yan, Y. R., et al. (2019). Cafs secreted exosomes promote metastasis and chemotherapy resistance by enhancing cell stemness and epithelial-mesenchymal transition in colorectal cancer. Mol. Cancer. 18 (1), 91. doi:10.1186/s12943-019-1019-x
Hu, T. H., Yao, Y., Yu, S., Han, L. L., Wang, W. J., Guo, H., et al. (2014). SDF-1/CXCR4 promotes epithelial-mesenchymal transition and progression of colorectal cancer by activation of the Wnt/β-catenin signaling pathway. Cancer Lett. 354 (2), 417–426. doi:10.1016/j.canlet.2014.08.012
Itatani, Y., Kawada, K., and Sakai, Y. (2019). Transforming growth factor-β signaling pathway in colorectal cancer and its tumor microenvironment. Int. J. Mol. Sci. 20 (23), 5822. doi:10.3390/ijms20235822
Kalli, M., and Stylianopoulos, T. (2018). Defining the role of solid stress and matrix stiffness in cancer cell proliferation and metastasis. Front. Oncol. 8, 55. doi:10.3389/fonc.2018.00055
Kasashima, H., Duran, A., Martinez-Ordonez, A., Nakanishi, Y., Kinoshita, H., Linares, J. F., et al. (2021). Stromal sox2 upregulation promotes tumorigenesis through the generation of a sfrp1/2-expressing cancer-associated fibroblast population. Dev. Cell. 56 (1), 95–110.e10. doi:10.1016/j.devcel.2020.10.014
Khare, T., Bissonnette, M., and Khare, S. (2021). Cxcl12-cxcr4/cxcr7 axis in colorectal cancer: therapeutic target in preclinical and clinical studies. Int. J. Mol. Sci. 22 (14), 7371. doi:10.3390/ijms22147371
Kobayashi, H., Gieniec, K. A., Lannagan, T., Wang, T., Asai, N., Mizutani, Y., et al. (2022). The origin and contribution of cancer-associated fibroblasts in colorectal carcinogenesis. Gastroenterology 162 (3), 890–906. doi:10.1053/j.gastro.2021.11.037
Koliaraki, V., Pallangyo, C. K., Greten, F. R., and Kollias, G. (2017). Mesenchymal cells in colon cancer. Gastroenterology 152 (5), 964–979. doi:10.1053/j.gastro.2016.11.049
Kramer, N., Schmollerl, J., Unger, C., Nivarthi, H., Rudisch, A., Unterleuthner, D., et al. (2017). Autocrine wnt2 signaling in fibroblasts promotes colorectal cancer progression. Oncogene 36 (39), 5460–5472. doi:10.1038/onc.2017.144
Li, H., Courtois, E. T., Sengupta, D., Tan, Y., Chen, K. H., Goh, J., et al. (2017). Reference component analysis of single-cell transcriptomes elucidates cellular heterogeneity in human colorectal tumors. Nat. Genet. 49 (5), 708–718. doi:10.1038/ng.3818
Li, Z., Sun, C., and Qin, Z. (2021). Metabolic reprogramming of cancer-associated fibroblasts and its effect on cancer cell reprogramming. Theranostics 11 (17), 8322–8336. doi:10.7150/thno.62378
Li, Z., Zhang, J., Zhou, J., Lu, L., Wang, H., Zhang, G., et al. (2019). Nodal facilitates differentiation of fibroblasts to cancer-associated fibroblasts that support tumor growth in melanoma and colorectal cancer. Cells 8 (6), 538. doi:10.3390/cells8060538
Li, Z. L., Wang, Z. J., Wei, G. H., Yang, Y., and Wang, X. W. (2020). Changes in extracellular matrix in different stages of colorectal cancer and their effects on proliferation of cancer cells. World J. Gastrointest. Oncol. 12 (3), 267–275. doi:10.4251/wjgo.v12.i3.267
Linares, J., Marin-Jimenez, J. A., Badia-Ramentol, J., and Calon, A. (2020). Determinants and functions of cafs secretome during cancer progression and therapy. Front. Cell. Dev. Biol. 8, 621070. doi:10.3389/fcell.2020.621070
Liu, T., Zhou, L., Li, D., Andl, T., and Zhang, Y. (2019). Cancer-associated fibroblasts build and secure the tumor microenvironment. Front. Cell. Dev. Biol. 7, 60. doi:10.3389/fcell.2019.00060
Mochizuki, S., Ao, T., Sugiura, T., Yonemura, K., Shiraishi, T., Kajiwara, Y., et al. (2020). Expression and function of a disintegrin and metalloproteinases in cancer-associated fibroblasts of colorectal cancer. Digestion 101 (1), 18–24. doi:10.1159/000504087
Moon, H., Ju, H. L., Chung, S. I., Cho, K. J., Eun, J. W., Nam, S. W., et al. (2017). Transforming growth factor-β promotes liver tumorigenesis in Mice via up-regulation of snail. Gastroenterology 153 (5), 1378–1391. doi:10.1053/j.gastro.2017.07.014
Nishina, T., Deguchi, Y., Ohshima, D., Takeda, W., Ohtsuka, M., Shichino, S., et al. (2021). Interleukin-11-expressing fibroblasts have a unique gene signature correlated with poor prognosis of colorectal cancer. Nat. Commun. 12 (1), 2281. doi:10.1038/s41467-021-22450-3
Nurmik, M., Ullmann, P., Rodriguez, F., Haan, S., and Letellier, E. (2020). In search of definitions: cancer-associated fibroblasts and their markers. Int. J. Cancer. 146 (4), 895–905. doi:10.1002/ijc.32193
Owusu, B. Y., Galemmo, R., Janetka, J., and Klampfer, L. (2017). Hepatocyte growth factor, a key tumor-promoting factor in the tumor microenvironment. Cancers 9 (4), 35. doi:10.3390/cancers9040035
Pankova, D., Chen, Y., Terajima, M., Schliekelman, M. J., Baird, B. N., Fahrenholtz, M., et al. (2016). Cancer-associated fibroblasts induce a collagen cross-link switch in tumor stroma. Mol. Cancer Res. 14 (3), 287–295. doi:10.1158/1541-7786.MCR-15-0307
Peng, S., Chen, D., Cai, J., Yuan, Z., Huang, B., Li, Y., et al. (2021). Enhancing cancer-associated fibroblast fatty acid catabolism within a metabolically challenging tumor microenvironment drives colon cancer peritoneal metastasis. Mol. Oncol. 15 (5), 1391–1411. doi:10.1002/1878-0261.12917
Peng, Y., Li, Z., Yang, P., Newton, I. P., Ren, H., Zhang, L., et al. (2014). Direct contacts with colon cancer cells regulate the differentiation of bone marrow mesenchymal stem cells into tumor associated fibroblasts. Biochem. Biophys. Res. Commun. 451 (1), 68–73. doi:10.1016/j.bbrc.2014.07.074
Rimal, R., Desai, P., Daware, R., Hosseinnejad, A., Prakash, J., Lammers, T., et al. (2022). Cancer-associated fibroblasts: origin, function, imaging, and therapeutic targeting. Adv. Drug Deliv. Rev. 189, 114504. doi:10.1016/j.addr.2022.114504
Rynne-Vidal, A., Au-Yeung, C. L., Jimenez-Heffernan, J. A., Perez-Lozano, M. L., Cremades-Jimeno, L., Barcena, C., et al. (2017). Mesothelial-to-mesenchymal transition as a possible therapeutic target in peritoneal metastasis of ovarian cancer. J. Pathol. 242 (2), 140–151. doi:10.1002/path.4889
Sahai, E., Astsaturov, I., Cukierman, E., DeNardo, D. G., Egeblad, M., Evans, R. M., et al. (2020). A framework for advancing our understanding of cancer-associated fibroblasts. Nat. Rev. Cancer. 20 (3), 174–186. doi:10.1038/s41568-019-0238-1
Schellerer, V. S., Langheinrich, M., Hohenberger, W., Croner, R. S., Merkel, S., Rau, T. T., et al. (2014). Tumor-associated fibroblasts isolated from colorectal cancer tissues exhibit increased icam-1 expression and affinity for monocytes. Oncol. Rep. 31 (1), 255–261. doi:10.3892/or.2013.2860
Siegel, R. L., Miller, K. D., Fedewa, S. A., Ahnen, D. J., Meester, R., Barzi, A., et al. (2017). Colorectal cancer statistics, 2017. CA-Cancer J. Clin. 67 (3), 177–193. doi:10.3322/caac.21395
Su, S., Chen, J., Yao, H., Liu, J., Yu, S., Lao, L., et al. (2018). Cd10(+)gpr77(+) cancer-associated fibroblasts promote cancer formation and chemoresistance by sustaining cancer stemness. Cell 172 (4), 841–856.e16. doi:10.1016/j.cell.2018.01.009
Sun, Q., Shang, Y., Sun, F., Dong, X., Niu, J., and Li, F. (2020). Interleukin-6 promotes epithelial-mesenchymal transition and cell invasion through integrin β6 upregulation in colorectal cancer. Oxidative Med. Cell. Longev. 2020, 8032187. doi:10.1155/2020/8032187
Sung, H., Ferlay, J., Siegel, R. L., Laversanne, M., Soerjomataram, I., Jemal, A., et al. (2021). Global cancer statistics 2020: globocan estimates of incidence and mortality worldwide for 36 cancers in 185 countries. CA-Cancer J. Clin. 71 (3), 209–249. doi:10.3322/caac.21660
Tan, H. X., Gong, W. Z., Zhou, K., Xiao, Z. G., Hou, F. T., Huang, T., et al. (2020). CXCR4/TGF-β1 mediated hepatic stellate cells differentiation into carcinoma-associated fibroblasts and promoted liver metastasis of colon cancer. Cancer Biol. Ther. 21 (3), 258–268. doi:10.1080/15384047.2019.1685157
Tauriello, D. (2023). Targeting cafs to improve anti-pd-1 checkpoint immunotherapy. Cancer Res. 83 (5), 655–656. doi:10.1158/0008-5472.CAN-22-3677
Torres, S., Bartolome, R. A., Mendes, M., Barderas, R., Fernandez-Acenero, M. J., Pelaez-Garcia, A., et al. (2013). Proteome profiling of cancer-associated fibroblasts identifies novel proinflammatory signatures and prognostic markers for colorectal cancer. Clin. Cancer Res. 19 (21), 6006–6019. doi:10.1158/1078-0432.CCR-13-1130
Torres, S., Garcia-Palmero, I., Herrera, M., Bartolome, R. A., Pena, C., Fernandez-Acenero, M. J., et al. (2015). Loxl2 is highly expressed in cancer-associated fibroblasts and associates to poor colon cancer survival. Clin. Cancer Res. 21 (21), 4892–4902. doi:10.1158/1078-0432.CCR-14-3096
Unterleuthner, D., Neuhold, P., Schwarz, K., Janker, L., Neuditschko, B., Nivarthi, H., et al. (2020). Cancer-associated fibroblast-derived wnt2 increases tumor angiogenesis in colon cancer. Angiogenesis 23 (2), 159–177. doi:10.1007/s10456-019-09688-8
Wanandi, S. I., Hilbertina, N., Siregar, N. C., Abdullah, M., and Jeo, W. S. (2021). Cancer-associated fibroblast (caf) secretomes-induced epithelial-mesenchymal transition on ht-29 colorectal carcinoma cells associated with hepatocyte growth factor (hgf) signalling. J. Pak. Med. Assoc. 71 (2), S18–S24.
Wang, M., Su, Z., and Amoah, B. P. (2020). Crosstalk among colon cancer-derived exosomes, fibroblast-derived exosomes, and macrophage phenotypes in colon cancer metastasis. Int. Immunopharmacol. 81, 106298. doi:10.1016/j.intimp.2020.106298
Wang, Z., Yang, Q., Tan, Y., Tang, Y., Ye, J., Yuan, B., et al. (2021). Cancer-associated fibroblasts suppress cancer development: the other side of the coin. Front. Cell. Dev. Biol. 9, 613534. doi:10.3389/fcell.2021.613534
Wawro, M. E., Chojnacka, K., Wieczorek-Szukala, K., Sobierajska, K., and Niewiarowska, J. (2018). Invasive colon cancer cells induce transdifferentiation of endothelium to cancer-associated fibroblasts through microtubules enriched in tubulin-β3. Int. J. Mol. Sci. 20 (1), 53. doi:10.3390/ijms20010053
Woolston, A., Khan, K., Spain, G., Barber, L. J., Griffiths, B., Gonzalez-Exposito, R., et al. (2019). Genomic and transcriptomic determinants of therapy resistance and immune landscape evolution during anti-egfr treatment in colorectal cancer. Cancer Cell 36 (1), 35–50. doi:10.1016/j.ccell.2019.05.013
Xuefeng, X., Hou, M. X., Yang, Z. W., Agudamu, A., Wang, F., Su, X. L., et al. (2020). Epithelial-mesenchymal transition and metastasis of colon cancer cells induced by the fak pathway in cancer-associated fibroblasts. J. Int. Med. Res. 48 (6), 300060520931242. doi:10.1177/0300060520931242
Yang, D., Li, Q., Shang, R., Yao, L., Wu, L., Zhang, M., et al. (2020). WNT4 secreted by tumor tissues promotes tumor progression in colorectal cancer by activation of the Wnt/β-catenin signalling pathway. J. Exp. Clin. Cancer Res. 39 (1), 251. doi:10.1186/s13046-020-01774-w
Yu, Y., Xiao, C. H., Tan, L. D., Wang, Q. S., Li, X. Q., and Feng, Y. M. (2014). Cancer-associated fibroblasts induce epithelial-mesenchymal transition of breast cancer cells through paracrine TGF-β signalling. Br. J. Cancer. 110 (3), 724–732. doi:10.1038/bjc.2013.768
Zeng, J., Tang, Z. H., Liu, S., and Guo, S. S. (2017). Clinicopathological significance of overexpression of interleukin-6 in colorectal cancer. World J. Gastroenterol. 23 (10), 1780–1786. doi:10.3748/wjg.v23.i10.1780
Zhang, C., Wang, X. Y., Zhang, P., He, T. C., Han, J. H., Zhang, R., et al. (2022a). Cancer-derived exosomal hspc111 promotes colorectal cancer liver metastasis by reprogramming lipid metabolism in cancer-associated fibroblasts. Cell Death Dis. 13 (1), 57. doi:10.1038/s41419-022-04506-4
Zhang, Y., Yin, C., Wei, C., Xia, S., Qiao, Z., Zhang, X. W., et al. (2022b). Exosomal mir-625-3p secreted by cancer-associated fibroblasts in colorectal cancer promotes emt and chemotherapeutic resistance by blocking the celf2/wwox pathway. Pharmacol. Res. 186, 106534. doi:10.1016/j.phrs.2022.106534
Zhou, D., and Zheng, L. (2024). Recent advances in cancer-associated fibroblast: biomarkers, signaling pathways, and therapeutic opportunities. Chin. Med. J. 137 (6), 638–650. doi:10.1097/CM9.0000000000003031
Zhou, L., Li, J., Tang, Y., and Yang, M. (2021). Exosomal lncrna linc00659 transferred from cancer-associated fibroblasts promotes colorectal cancer cell progression via mir-342-3p/anxa2 axis. J. Transl. Med. 19 (1), 8. doi:10.1186/s12967-020-02648-7
Keywords: cancer-associated fibroblasts, colorectal cancer, heterogeneity, invasion, metastasis
Citation: Yin J, Zhu W, Feng S, Yan P and Qin S (2024) The role of cancer-associated fibroblasts in the invasion and metastasis of colorectal cancer. Front. Cell Dev. Biol. 12:1375543. doi: 10.3389/fcell.2024.1375543
Received: 24 January 2024; Accepted: 11 July 2024;
Published: 30 July 2024.
Edited by:
Pulin Che, University of Alabama at Birmingham, United StatesReviewed by:
Yingpu Li, Harbin Medical University Cancer Hospital, ChinaGeorge Cai, University of Alabama at Birmingham, United States
Copyright © 2024 Yin, Zhu, Feng, Yan and Qin. This is an open-access article distributed under the terms of the Creative Commons Attribution License (CC BY). The use, distribution or reproduction in other forums is permitted, provided the original author(s) and the copyright owner(s) are credited and that the original publication in this journal is cited, in accordance with accepted academic practice. No use, distribution or reproduction is permitted which does not comply with these terms.
*Correspondence: Pengke Yan, gysyypk@126.com; Shumin Qin, shuminqin@gzucm.edu.cn