- 1Department of Biological Sciences, Middle East Technical University, Ankara, Türkiye
- 2Qatar University, QU Health, College of Medicine, Doha, Qatar
- 3Q3 Research Institute (QRI), Research and Policy Division, Ypsilanti, MI, United States
Metronomic chemotherapy (MCT), characterized by the continuous administration of chemotherapeutics at a lower dose without prolonged drug-free periods, has garnered significant attention over the last 2 decades. Extensive evidence from both pre-clinical and clinical settings indicates that MCT induces distinct biological effects than the standard Maximum Tolerated Dose (MTD) chemotherapy. The low toxicity profile, reduced likelihood of inducing acquired therapeutic resistance, and low cost of MCT render it an attractive chemotherapeutic regimen option. One of the most prominent aspects of MCT is its anti-angiogenesis effects. It has been shown to stimulate the expression of anti-angiogenic molecules, thereby inhibiting angiogenesis. In addition, MCT has been shown to decrease the regulatory T-cell population and promote anti-tumor immune response through inducing dendritic cell maturation and increasing the number of cytotoxic T-cells. Combination therapies utilizing MCT along with oncolytic virotherapy, radiotherapy or other chemotherapeutic regimens have been studied extensively. This review provides an overview of the current status of MCT research and the established mechanisms of action of MCT treatment and also offers insights into potential avenues of development for MCT in the future.
1 Metronomic chemotherapy: less is more
The principle of chemotherapeutic drugs is to inhibit cell proliferation or induce cell death through many different mechanisms (Shewach and Kuchta, 2009). The traditional regimen for chemotherapeutic administration has predominantly favored the Maximum Tolerated Dose (MTD) therapy approach for a long time (McConnell, 1989). MTD therapy utilizes the maximum dose that can be tolerated by the patient without inducing lethal consequences to the patient (Takimoto, 2009). Though it is aimed at maximizing tumor depletion in the patient, the cytotoxic burden of this treatment option in normal tissues brings out the need for prolonged rest periods between administrations (Xu et al., 2021). Side effects such as extensive damage to healthy tissues and neurotoxic properties are of great concern and greatly impair the quality of life of the patients (Ho et al., 2018). Although this administration route results in a serious decrease in the tumor volume initially, it is often accompanied by the development of therapeutic resistance and subsequent relapse in later stages (Kareva et al., 2015).
The history of MTD being the hallmark of chemotherapeutic treatment dates back to the 1970s when it was proven to be successful in treating pediatric acute lymphoblastic leukemia (Skipper et al., 1970). This was a rare case since the leukemic tumor clone could be fully eliminated. However, this is not the case in many other cancer subtypes, even in leukemias other than acute lymphoblastic leukemia. Many other cancer types require more than just the elimination of the tumor cells using very high MTD doses since there are also many other players contributing to tumorigenesis such as the tumor microenvironment (Wu and Dai, 2017), immune cells (Gun et al., 2019), different cancer subclones (Zhang et al., 2022), and epigenetic alterations (Ilango et al., 2020). Cancer is not a simple disease that can be explained by the mutations of the cancer cells by itself but rather it is a complex interplay revolving around many different aspects (Hanahan and Weinberg, 2011). The philosophy that “the more is better’’ in the case of cancer treatment is outdated, as it has been proven that this approach is not only insufficient but also could be even more harmful, in most cases (Scharovsky et al., 2009). Therefore, the need for the development of different chemotherapeutic administration regimens is of utmost importance.
A novel alternative concept of drug administration regimen named metronomic chemotherapy (MCT) has emerged in the last 2 decades. MCT refers to the continuous administration of low-dose chemotherapeutic agents within close intervals, without long rest periods (Cazzaniga et al., 2016). In the year 2000, Browder et al. published a study exploring the anti-angiogenic effects of varying doses of different cytotoxic drugs, primarily cyclophosphamide on a mouse model. They found that when the drug was given at a lower dose within 6-day intervals, it was more effective than the traditional MTD regimen in terms of tumor shrinkage and anti-angiogenesis properties (Browder et al., 2000). Klement et al. also published a study in the same year on the effects of the drugs Vinblastine and mAb DC-101 which were administered regularly in a mouse model at lower doses than MTD. They found that when these two drugs were given together in lower doses, namely, the MCT regimen, the tumors significantly regressed, anti-angiogenic effects were detected, no relapse period was observed afterward and the toxicity of the treatment was far less than that of MTD regimen (Klement et al., 2000). Later that year, Hanahan et al., used the term “metronomic chemotherapy’’, for the first time in a commentary based on the two studies mentioned above (Hanahan et al., 2000). Since then, it has been backed up by many other preclinical studies as well as clinical trials that MCT has different biological effects than MTD, and the many aspects of MCT have been delineated (Kamat et al., 2007; Schito et al., 2020; Mundo et al., 2022).
The main differences between conventional MTD chemotherapy and MCT lie in the temporal administration regimen and the given dosage of the chemotherapeutic drug. These differences are diagrammatically depicted in Figure 1. In conventional chemotherapy schedules, the standard of care MTD therapy involves administrating the maximum dose that can be tolerated by the patient without the induction of lethal outcomes (Scharovsky et al., 2020). Conversely, in MCT studies, dosages have consistently been reported at levels notably lower than the maximum tolerated dose (Mpekris et al., 2021; Romiti et al., 2017; Wysocki et al., 2022). Another significant difference between conventional MTD chemotherapy and MCT is their temporal administration routes. In conventional MTD chemotherapy, prolonged drug-free periods are often necessitated since the administration of high doses of the chemotherapeutics frequently leads to damage in normal tissues and requires recovery time (Scharovsky et al., 2020). In contrast, MCT employs lower doses, resulting in reduced damage to normal tissues, thereby the drugs can be administrated continuously or with minimal drug-free periods (Shi et al., 2019).
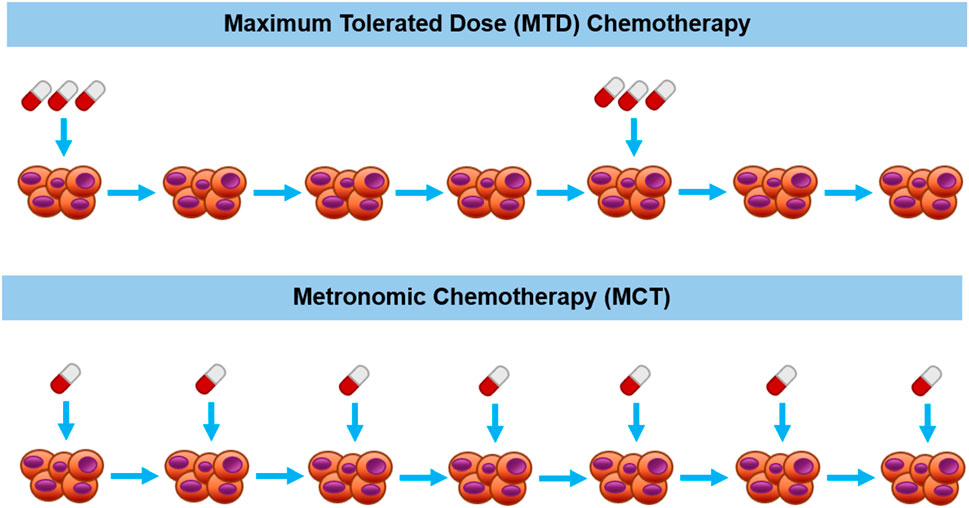
Figure 1. Diagrammatic description of maximum tolerated dose therapy (upper part) vs metronomic therapy (bottom part) for chemotherapeutic drug administration schedules.
There are a number of therapeutic options available for the treatment of different cancer types. Unfortunately, the opportunity to access them is not the same for people all over the world. According to the 2020 World Health Organization (WHO) report, cancer was responsible for approximately 10 million deaths across the world (World Health Organization, 2020). Among these 10 million cancer-associated deaths, around 70% were from low and middle-income countries (LMICs). Moreover, the incidence rates of cancers were lower in these countries despite the enormous death rate, indicating the necessity for improved monitoring of cancers. Cancer care is associated with an overwhelming demand for resources, infrastructure, and challenges for the national health systems (Fousseyni et al., 2011; Chalkidou et al., 2014) Patients from LMICs lack access to screening, early diagnosis, treatment facilities, pain-relief options, cancer-preventative vaccines, and many more (André et al., 2013). Furthermore, the escalating cost of novel therapeutics renders the clinical trials and biomedical research on the subject unsustainable and slows down scientific progress (Schrag, 2004). Under these conditions, considering the cost of treatment options to provide treatments as accessible as possible hold critical importance.
Considering cost-efficacy, MCT is a prominent therapeutic option (Bocci et al., 2005). Usually, generic, non-patented drugs are used in MCT applications. Many of these drugs can also be supplied in oral forms, which are easier to administer and require no hospital care during the administration process (André et al., 2013). The toxicity profile of MCT is profoundly less than that of other regimens, especially MTD (Benzekry and Hahnfeldt, 2013). This eliminates the need for costly hospital stays for the inevitable normal tissue recovery phase after the treatment (C). The minimal toxicity-inducing doses of MCT induce fewer adverse effects and decrease the cost of monitoring as well as supporting care (Munzone and Colleoni, 2015). All of the above-mentioned reasons make MCT a feasible potential therapeutic option in the fight against cancer.
A tremendous amount of data has been produced in cancer research, and the accumulation of data has continued over the years. One beneficial way to make use of this data is via the utilization of mathematical models of cancer growth and treatment (Araujo and McElwain, 2004; Tabassum et al., 2019). Using mechanistic insights and better understanding of cellular parameters provided by those mathematical models, both clinicians and biologists have a chance to develop better strategies to combat cancer (Majumder and Mukherjee, 2007; Gallasch et al., 2013). Mathematical modeling in cancer research dates back to the 20th century with the majority of these studies focusing on the tumor growth itself (Moolgavkar, 1991). The temporal administration of drugs has been an important area of interest within those efforts. For example, West and Newton designed an evolutionary growth/regression model to investigate the effects of chemotherapeutic dose scheduling (West and Newton, 2017). They compared the efficacy of MCT and MTD chemotherapeutic regimens on various tumors bearing different growth rates. They concluded that MCT strategies exceeded MTD in terms of a decrease in the total number of tumor cells, especially in the fast-growing tumors. Another study performed by Curtis et al., proposed a pharmacokinetic/pharmacodynamic model coupled with a vascularized tumor growth model to stimulate MTD and MCT regimens in lung cancer chemotherapy cases. They concluded that metronomic regimens were more advantageous than MTD regimens, and the combination of these two strategies did not improve the outcome (Curtis et al., 2018). Due to the heterogeneous nature of tumor populations, mathematical models of various kinds have been proven to favor low-dose employing regimens, such as MCT over MTD in this context (Ledzewicz and Schättler, 2014).
2 Metronomic chemotherapy: mechanisms of action
When MCT was first discovered, it was thought that the main function of this treatment was anti-angiogenesis (Colleoni et al., 2002; Perroud et al., 2013). As more evidence accumulated through both preclinical studies and clinical data, it was later revealed that MCT had not only provided anti-angiogenetic properties but also various other functions (Cazzaniga et al., 2016; Romiti et al., 2017). The aspects of metronomic chemotherapy highlighted in the review herein are summarized in Figure 2. In this section, important advantages and the main mechanisms of action of MCT will be discussed in detail, such as decreasing angiogenesis and increasing anti-tumor immune response.
2.1 Metronomic therapy induces less acquired therapeutic resistance
Intertumoral and intratumoral heterogeneity have been a hot topic of research in the past years (Liu et al., 2018; Bergmann et al., 2020; Yalcin et al., 2020; Grzywa et al., 2021). The tumor heterogeneity contributing to the presence of one or more cancer cell clones that are resistant to the chemotherapeutic agent of choice could be present prior to the treatment or it might emerge during the course of the treatment (Kelderman et al., 2014; Danisik, et al., 2023; Yalcin et al., 2023; Baygin et al., 2024). The heterogeneous nature of the tumor population contributes to the latter; acquired therapeutic resistance and poses a serious threat to the therapeutic outcomes (Foo and Michor, 2014; Acar et al., 2020). One of the key differences between MCT and MTD treatment regimens is the extent of their ability to form acquired therapeutic resistance since the temporal administration status of drugs is a key determinant of their resistance profile (Goldman et al., 2015; Kareva et al., 2015). As previously discussed, MTD administration generally results in increased acquired therapeutic resistance. The MTD therapy aims to eliminate all drug-sensitive cells through employing the highest possible dose of the cytotoxic agent, this in return creates a selective pressure leading to the selection of the most fit’’ drug-resistant clones in the tumor population (Foo and Michor, 2010; Vasan et al., 2019). Even though most of the drug-sensitive clones have been eliminated, this phenomenon drives the rapid recolonization of the tumor population through drug-resistant clones, hence relapses arise (Dagogo-Jack and Shaw, 2018). In the case of MCT, the observed effect of acquired therapeutic resistance is significantly lower than that of MTD (Scharovsky et al., 2020; Patwardhan et al., 2021). Typical MCT regimens employ much lower doses than MTD, and in return ensure the maintenance of the drug-resistant tumor population since the selective pressure is not as prominent (Bondarenko et al., 2021). A recent study performed by Mejia Peña et al. (2023), demonstrated that the administration of metronomic or MTD Paclitaxel regimens resulted in different resistant cancer cell populations with unique nuclear and phenotypic traits. The evidence they provided pointed toward the phenomenon that these two different resistant populations are primed differentially for the response they might give towards chemotherapy or induced metastasis stress (Meja Peña et al., 2023). In conclusion, metronomic chemotherapy is emerging as a prominent treatment strategy, offering the potential to mitigate acquired therapeutic resistance.
2.2 The low toxicity profile of metronomic chemotherapy
The toxicity induced by chemotherapy is considered as one of the most important side effects (Livshits et al., 2014). The working mechanism of the chemotherapeutic drugs is to damage proliferating cells through inducing DNA damage, impairing mitosis and DNA damage repair mechanisms, and eventually leading to apoptosis (Kaufmann and Earnshaw, 2000; Hayashi and Karlseder, 2013). Importantly, these damages are not specific to the cancer cells but rather they affect all proliferating cells, including the normal ones (Toale et al., 2021). The cytotoxic burden of the treatment affects the administration intervals to allow sufficient time for the recovery of damaged non-cancerous tissues. MTD requires prolonged rest periods between the administrations since it utilizes highly cytotoxic doses. On the contrary, the MCT regimen has been shown to exhibit a much lower toxicity profile than MTD (Emmenegger et al., 2004; Simsek et al., 2019; Cazzaniga et al., 2022). The lower cytotoxic burden also means fewer side effects and an increased quality of life for the patients in general (Xu et al., 2021; Wysocki et al., 2022). This is especially advantageous for cancer patients in palliative care as the expectations of the treatment outcome are symptomatic relief and low toxicity (Patil et al., 2019; Harsh et al., 2023). In summary, the low toxicity profile of metronomic chemotherapy renders it an appealing and viable treatment option for cancer of many kinds.
2.3 Effects of metronomic chemotherapy on the immune system
The immune system plays a double-edged role in the initiation, progression and metastasis stages of cancers (Visser et al., 2006; NarendraBodduluru et al., 2013). Hanahan and Weinberg have categorized tumor-promoting inflammation and avoiding immune destruction as two of the hallmarks of cancer in their seminal 2011 paper (Hanahan and Weinberg, 2011). Among the many cells of the immune system, regulatory T cells (T-regs) are of great importance in the context of the tumor microenvironment (Wolf et al., 2015; Paluskievicz et al., 2019). T-regs are a specialized subset of helper T lymphocytes that bear the important role of modulating the immune response (Shevyrev and Tereshchenko, 2020). They express specific cell surface markers such as CD3, CD4, and CD25, as well as FoxP3 (Tanaka and Sakaguchi, 2019). They are important for building tolerance towards the host’s self-antigens, and they take part in key cellular processes including allergies, tumorigenesis, neoplasia and infections (Martins et al., 2012). Dysfunction of T-reg cells leads to an over-suppression of the immune response and it is correlated with a number of different diseases such as autoimmune disorders, allergy and cancer (Attias et al., 2019; Ohue and Nishikawa, 2019). Within the context of cancer, T-reg cell overexpression poses a serious problem as it results in the over-suppression of the effector anti-tumor immunity which may result in treatment unresponsiveness and tumor progression. Also, the assembling of T-reg cells in tumor sites as well as in the peripheral circulation leads to immune evasion of the tumors (Frydrychowicz et al., 2017). Furthermore, the anti-tumor immune response significantly weakens as the T-reg cells secrete cytokines (IL-10, TGF-β etc.) that suppress other types of immune cells such as helper and cytotoxic T cells, macrophages, natural killer cells that are responsible for anti-tumor responses (Ohue and Nishikawa, 2019). Although, it should be noted that the presence of T-reg cells is of great importance for numerous other vital functions, such as fighting autoimmunity, hence, the required T-reg population should be maintained (Wolf et al., 2015).
The administration of various chemotherapeutic agents has been reported to induce T-reg-decreasing effects. Banissi et al. has reported that a metronomic regimen of Temozolomide was the most successful among other treatment regimens in decreasing the T-reg population in a rat glioma model (Banissi et al., 2009). Another study performed by Shevchenko et al. investigated the effects of metronomic Gemcitabine administration on murine pancreatic cancer model Panc02. They concluded that the administration of the metronomic Gemcitabine depleted the T-regs, and it resulted in improved survival of the animal models (Shevchenko et al., 2013). In particular, the Treg-related effects of the metronomic administration of the drug Cyclophosphamide has been extensively studied in both animal models and clinical trials. Ge et al. reported that metronomic administration of Cyclophosphamide led to a selective and rapid decrease in the T-reg cell numbers of breast cancer patients and noted that this decrease was temporary (Ge et al., 2012). Generali et al. reported that Cyclophosphamide in combination with the aromatase inhibitor Letrozole resulted in a significant decrease in T-reg population in a cohort of 83 breast cancer patients (Generali et al., 2009) Ghiringhelli et al. has also shown that metronomic oral administration of Cyclophosphamide has led to a selective decrease in circulating T-reg cells, which positively correlated with the therapeutic outcomes of advanced cancer patients (Ghiringhelli et al., 2007).
Apart from decreasing the immune suppression caused by T-reg cells, MCT has been shown to have promising effects on enhancing the anti-tumor immune response as well. Cytotoxic T cells are a subset of immune cells that represent one of the most potent effectors in the immune response against cancer. They can be distinguished by their expression of the CD8 cell surface marker and serve as primary destroyers of pathogens as well as tumor cells (Raskov et al., 2021). Increase in the cytotoxic T-cell population was also observed in many MCT studies. Hermans et al. asserted that metronomic administration of Cyclophosphamide resulted in a considerably larger increase in the cytotoxic T-cell population compared to the MTD administration in a mouse model. They also observed that the MTD administration of Cyclophosphamide caused a dramatic decrease in the overall cytotoxic T-cell population after 28 days, which was not the case in the MCT administration of the same drug (Hermans et al., 2003). Likewise, He et al. reported that metronomic administration of Paclitaxel in combination with an anti-cancer vaccine has significantly increased the cytotoxic T-cell infiltration into the tumor tissue in a prostate cancer mouse model. In the same study, they also observed increased dendritic cell maturation, translating into enhanced anti-tumor immune response (He et al., 2011). Dendritic cells are another subset of immune cells that are responsible for activating the T-cell-mediated anti-tumor response through their antigen presenting properties (Wculek et al., 2020). Finally, additional studies have also supported the anti-tumor immune response-promoting effects of MCT (Ghiringhelli et al., 2007; Ge et al., 2012; Khan et al., 2020). Altogether, the presented data strongly indicate that metronomic chemotherapy not only diminishes the suppressive impact of regulatory T cell populations but also enhances the anti-tumor immune response, underscoring its potential as a pivotal element in cancer treatment strategies.
2.4 The anti-angiogenesis effects of metronomic chemotherapy
The cells in the human body require certain nutrients and oxygen to survive. To supply this demand, they must be located near blood vessels, the highways of the body, and new blood vessels should be recruited through processes called vasculogenesis and angiogenesis as the organism gets larger (Nishida et al., 2006). Angiogenesis refers to the formation of new capillaries through the pre-existing blood vessels. It is part of normal cellular processes such as wound healing and embryogenesis as well as pathological conditions such as atherosclerosis, tumorigenesis and rheumatoid arthritis (Rajabi and Mousa, 2017). In the case of tumors, the formation of new blood vessels is especially important for the tumor to grow to a certain size and for the metastasis process, moreover, it has been defined as one of the hallmarks of cancer (Hanahan and Weinberg, 2011). Pro-angiogenic and anti-angiogenic molecules are responsible for regulating this crucial cellular process. These molecules can be influenced by various events such as hypoglycemia, inflammatory response, and genetic changes, and they can originate from endothelial cells, blood, and cancer cells (Huang and Bao, 2004). Among the pro-angiogenic molecules, vascular endothelial growth factor (VEGF), fibroblast growth factor-2 (FGF-2), platelet-derived growth factor (PDGF), and angiopoietins have been characterized. On the other hand, thrombospondins TSP-1 and TSP-2, angiostatin, interleukins IL-1 β, IL-3, IL-6, IL-8, and tumor necrosis factor (TNF) α are crucial effector molecules of anti-angiogenesis (Carmeliet and Jain, 2000). Altogether, the balance between these two classes of molecules is of utmost significance for angiogenesis capacity and tumor control. If this balance is disrupted, favoring the pro-angiogenic side, the “angiogenic switch’’ occurs (Bergers and Benjamin, 2003). This results in circulating endothelial progenitors (CEP) recruitment which are able to promote angiogenesis. In addition, the angiogenic switch can induce genetic reprogramming in the cancer cells, consequently inducing new blood vessel formation, all of which favor tumor progression (Natale and Bocci, 2018).
The earliest studies on metronomic therapy have showcased the anti-angiogenesis effects of the treatment option (Browder et al., 2000; Klement et al., 2000). Since then, it has been assumed that the main anti-tumor effect of MCT was its anti-angiogenesis properties. This is postulated to be due to the characteristics of the tumor endothelial cells and the mechanism of action of the chemotherapeutic agents (Kerbel and Kamen, 2004). In the MCT regimens, low doses of the chemotherapeutic drugs are employed in a continuous manner. This ensures the presence of a certain level of the drug in the body for longer times. Chemotherapeutic drugs are not selective by nature; they act by disrupting the cellular proliferation of all dividing cells, including the endothelial cells found in growing blood vessels around the tumor sites (Soultati et al., 2012). Endothelial cells are relatively more genetically stable than cancer cells and do not develop drug resistance as easily (Hida et al., 2018). Therefore, the presence of chemotherapeutic drugs without long rest periods ensures the elimination of the dividing endothelial cells around the tumor, thus, translating into anti-angiogenic effects (Kerbel and Kamen, 2004). On the other hand, longer rest periods are needed in MTD regimens, thus, giving endothelial cells around the tumor more time to recover from the detrimental chemotherapeutic effects.
One of the pioneering studies on the anti-angiogenic effects of metronomic chemotherapy was performed in 2003 by Wang et al. They have demonstrated that when the drug Paclitaxel was administered at low doses (0.1–100 p.m.), it selectively inhibited the human endothelial cell proliferation in vitro. Whereas, when they administrated the same drug at concentrations 104–105 folds higher, they observed the inhibition of cells that are of non-endothelial origin (Wang et al., 2003). Perroud et al. has analyzed the serum levels of angiogenesis markers in breast cancer patients undergoing MCT treatment of Cyclophosphamide and Celecoxib. They have demonstrated that the ratio of VEGF/TSP-1 has decreased significantly during the course of the therapy. This suggests that there has been a shift towards the anti-angiogenic molecule TSP-1 during the treatment (Perroud et al., 2013). Similarly, Colleoni et al. has also looked at the serum VEGF levels of 63 breast cancer patients that received MCT treatment of Methotrexate and Cyclophosphamide. They displayed a decrease in VEGF levels, therefore, a decrease in angiogenesis after the MCT treatment (Colleoni et al., 2002). A recent study performed by Mundo et al., investigated the differences between angiogenesis markers on a mouse colorectal cancer model treated with Fluorouracil following either a MTD or MCT regimen. They have shown that the MTD regimen leads to a more prominent increase in the mean tissue oxygen saturation and oxyhemoglobin levels. In addition, the MTD group had a sustained decrease in VEGF expression. It was concluded that the MTD group displayed more evident blood vessel remodeling, which is correlated with angiogenesis (Mundo et al., 2022). To sum up, altering angiogenesis was the first effective mechanism of MCT to be identified, and until this day, a growing body of data confirm that MCT is responsible for decreasing angiogenesis (Pietras and Douglas, 2005; Biziota et al., 2017; Natale and Bocci, 2018).
2.5 Cancer stem cell (CSC) targeting effects of metronomic chemotherapy
Cancer stem cells (CSCs) are a subpopulation of cells harboring cancer-initiating properties (Wysocki et al., 2022). The CSCs were first identified in 1994, when a subgroup of cancer cells was isolated through their CD34+ and CD38− surface marker expressions, in a study performed on a leukemia mouse model (Lapidot et al., 1994). They are also distinguished by expression signature of the transcription factors OCT4, Sox2, MYC, Nanog, and signaling pathways Wnt, Notch, JAK-STAT (Yang et al., 2020). Other biomarkers of CSC include expression of surface markers CD44, CD133, CD166 and CD90 (Leon et al., 2016). The CSCs are characterized by their self-renewal and cell differentiation capacities, thereby playing a fundamental role in various aspects of tumor malignancy. This subpopulation of cancer cells is especially important in the biological processes of tumor recurrence and metastasis, owing to their differentiation, senescence and self-renewing properties. Accordingly, CSCs pose a serious threat to the efficacy of cancer therapies. Several studies have shown that MCT led to a decrease in the proliferation and survival rates of CSCs (Folkins et al., 2007; Muñoz et al., 2021; Wysocki et al., 2022). In an in vitro study, MCT administration of Paclitaxel has been shown to reduce CSC population (Salem et al., 2020). In another study, the CSC population-depleting effects of MCT administration of Cyclophosphamide was shown in a xenograft model of pancreatic cancer (Vives et al., 2013). To conclude, beneficial effects of MCT on decreasing the CSC population, an important player in cancer progression, have been demonstrated by several studies.
3 The translation of MCT: from Petri dish to the clinic
The effects of various MCT regimens as first- and second-line treatments have been extensively studied in the clinic. Numerous ongoing phase II and III clinical trials persist in elucidating the diverse effects of metronomic chemotherapy on patients in real-world scenarios. Among these, the clinical studies concerning breast cancer patients are especially high (Montagna et al., 2017; Lago et al., 2022; Chai et al., 2023). In 2017, The International Consensus Guidelines for Advanced Breast Cancer (ABC) affirmed that MCT is a reasonable treatment for patients without the need for rapid tumor shrinkage. They also stated that so far, especially the low-dose oral Cyclophosphamide and Methotrexate MCT regimen had accumulated substantial clinical data and called for more randomized trials to better compare MCT with other standard regimens. The published statement garnered agreement from a consensus of 88% of the panelists (Cardoso et al., 2017). MCT is also a popular treatment option among clinical studies regarding other types of cancer, such as prostate cancer, ovarian cancer and non-small cell lung cancer (NSCLC). Table 1 provides a detailed overview of selected clinical studies conducted over the past decade, encompassing phase II, phase III, and retrospective studies across various cancer types. Overall survival (OS), progression free survival (PFS) and objective response rate (ORR%) properties were displayed to assess the efficacy of the therapy choice.
4 Combination therapy strategies regarding MCT
Combination therapeutic approaches have been widely used in cancer therapy. When compared with mono-therapy, the efficacy of the treatment is often increased synergistically or in an additive fashion when two or more therapeutic agents are used in combination (Matlock et al., 2017; Mokhtari et al., 2017). This phenomenon occurs primarily because approaches targeting distinct pathways are employed together in combination therapy. Consequently, the risk of developing acquired resistance to treatment and the subsequent progression of the tumor is reduced (Leary et al., 2018).
For a study design of anti-cancer combination therapy, the toxicity aspect is of great concern, since when more than one cytotoxic agent is being utilized it may result in an increased toxic burden on the patient (Delbado et al., 2004; Parhi et al., 2012). MCT is commonly used in combinational therapeutic approaches, primarily owing to its low toxicity profile and its capacity to minimize the acquired therapeutic resistance. There are many studies in which MCT was employed in combination with other anti-cancer therapies such as immunotherapy (Soriano et al., 2011), radiation therapy (Chung et al., 2017) and oncolytic virotherapy (Liikanen et al., 2013). There are also cases where MCT is coupled with an MTD treatment approach of another chemotherapeutic agent to strengthen the anti-tumor response without inducing adverse toxicity (Brems-Eskildsen et al., 2021).
Radiation therapy is a commonly used treatment modality for different cancer types. Approximately 50% of all cancer patients undergo radiation therapy as a part of their treatment regimen throughout the course of their illness (Baskar et al., 2012). A number of studies reported promising results upon the administration of combination therapy regimens employing radiation therapy along with MCT (Štěrba et al., 2002; Choi et al., 2008; Chung et al., 2017). It has been shown that the metronomic administration of four different drugs; namely, Celecoxib, Vinblastine, Cyclophosphamide, and Methotrexate coupled with radiotherapy afterward, resulted in favorable outcomes in 64 pediatric solid tumor-bearing patients (Ali and Mohamed, 2016). The disease response rate was found to be ∼76.6%, coupled with minimal toxicity and a 62% 1-year overall survival rate (Ali and Mohamed, 2016).). In another study, Salmaggi et al. investigated the effects of a combination of Carmustine wafers (which are implanted into the brain to release the drug Carmustine as the wafers dissolve), metronomic Temozolomide and radiotherapy regimen for the treatment of glioblastoma patients. They compared the efficacy of the treatment as compared to the Stupp protocol, a standard practice for the treatment of newly diagnosed glioblastoma patients (Salmaggi et al., 2013). They reported an increase in progression-free survival without the increased toxicity, as compared with the standard Stupp regimen. However, they also noted that the median survival rate increase in their newly-formed schedule was not as evident as in Stupp schedule, and therefore prospective comparative trials were recommended (Salmaggi et al., 2013). Collectively, combination therapeutic strategies employing radiation therapy along with metronomic chemotherapy have been shown to be effective in many studies.
Oncolytic virotherapy is a form of cancer therapy utilizing oncolytic viruses that specifically replicate and damage cancerous cells (Davis and Fang, 2005). It offers a multidirectional therapeutic platform since the viral vectors can be engineered to harbor transgenes that alter their cytotoxicity and immunostimulatory properties (Harrington et al., 2019). Combination therapies consisting of oncolytic virotherapy along with metronomic administration of chemotherapeutic drugs have been reported to have increased efficacy (Bramante et al., 2016; Toulmonde et al., 2022). Qiao et al. reported that the metronomic administration of a combination of Cyclophosphamide along with oncolytic reovirus resulted in greater antitumor efficacy and less toxicity when compared to single high or low-dose administrations (Qiao et al., 2008). Onimaru et al. also showed that the combination therapy involving metronomic administration of Gemcitabine along with engineered adenovirus resulted in increased cytotoxicity of cancer cells in pancreatic cancer mouse models. They have attributed this increase to the crosstalk mechanisms between the two components, allowing for enhanced viral entry and subsequent cancer cell cytotoxicity (Onimaru et al., 2010). A similar case of enhanced oncolytic adenovirus anti-tumor efficacy was reported when combined with low-dose Paclitaxel (Ingemarsdotter et al., 2015). Another study performed by Cheema et al. has also demonstrated that metronomic etopside induction enhanced the antitumor properties of oncolytic herpes simplex virus treatment and decreased the cytotoxic burden of the viral treatment on human glioblastoma stem cell xenografts (Cheema et al., 2011). Taken together, these data suggest that the combination of virotherapy with MCT is a promising strategy to achieve increased anti-tumor properties as well as decreased toxicity.
Cyclophosphamide is a widely used chemotherapeutic drug for the treatment of neoplasms, it is a nitrogen mustard and it induces anti-tumor effects through alkylation (Emadi et al., 2009; Voelcker et al., 2020). While relatively successful at eradicating the tumor cells, Cyclophosphamide is known to have a high cytotoxic profile, limiting its utilization (Haubitz, 2007). Rather than the highly cytotoxic MTD administration, MCT administration of Cyclophosphamide is extensively being utilized in many studies in combination with various other therapies such as vaccine administrations or immunotherapies (Soriano et al., 2011; Weir et al., 2014). It has been shown to help decrease the immune suppression caused by the tumor and strengthen the anti-immune responses (Hermans et al., 2003; Borch et al., 2016). It has been shown that low-dose metronomic application of Cyclophosphamide strengthens both the adaptive and innate anti-tumor immune responses when combined with a TLR9 agonist (Leong et al., 2019). In another study, Webb et al. proposed that a combination of anti-PD1 immunotherapy and metronomic CPA administration resulted in an increased survival rate among mouse models (Webb et al., 2022).
Lastly, drug repurposing is another phenomenon worth mentioning that helps salve the expenses of novel therapeutic drug research and makes MCT an attractive option. Drug repurposing is a remarkably cost-effective approach to utilize old drugs for novel purposes (Sleire et al., 2017; Correia et al., 2021). It enables faster clinical translation since the time-consuming testing of parameters such as toxicity, efficacy, and pharmacodynamic properties of the drug are already set. MCT offers a new possibility for the repurposing of old, non-cancer drugs. Muscarinic agonists are a great example of popular drug repurposing efforts in the context of MCT (Sales, 2018; Salem et al., 2020). In addition, since MTD therapy has been the cornerstone of chemotherapeutic treatment for a long time, most of the chemotherapeutic drugs were tested specifically on MTD regimen. MCT offers a promising novel low-cost option for the use of already existing chemotherapeutic drugs, as it has been proven by many studies that MCT and MTD regimens of the same drug induce different biological effects (Tongu et al., 2013; Fares et al., 2020).
5 More than chemotherapy: Metronomic photodynamic therapy
Photodynamic therapy (PDT) is a new field of cancer therapy. It is based on the principle of utilizing photosensitizer drugs which are administrated to the tumor area, and then irradiated when light is induced into the area (Agostinis et al., 2011). As the photosensitizer drug absorbs either visible or near-infrared light, a series of photochemical reactions are then triggered, releasing cytotoxic reactive oxygen species (ROS) byproducts and leading to cancer cell death through free radical-related DNA damage, oxidative stress, apoptosis, or necrosis (Wilson and Patterson, 2008; Kwiatkowski et al., 2018). PDT treatment has been a popular choice for the treatment of skin cancers owing to the feasibility of the treatment related to the positioning of the tumor, as well as brain cancers since modern therapies have been very limited (Quirk et al., 2015).
A downside of PDT was the emergence of treatment resistance due to the rapid consumption of oxygen that comes with using a high fluence rate. The concept of metronomic photodynamic therapy (mPDT) emerged around 2 decades ago, meeting the need for decreased treatment resistance and lower toxicity profile (Bisland et al., 2004). Metronomic photodynamic therapy is based on both the photosensitizer drug and the light source being metronomically applied at a low rate, continuously to maximize specifically tumor cell ablation and minimize damage to normal tissues. Especially in the case of brain cancer treatment, choosing a regimen inducing low toxicity to normal brain tissues is of utmost importance. In one of the earliest works within this research area, Davies and Wilson examined the feasibility of metronomic application of the photosensitizer 5-aminolevulinic acid induced protoporphyrin IX (ALA-PpIX) mediated photodynamic therapy in a rat-derived astrocytoma model. They were able to demonstrate a 4-day metronomic delivery, and reported that mPDT was the most successful delivery option among other PDT regimens. They also observed the ablation of the newly formed pre-bulk tumor population through metronomic application (Davies and Wilson, 2007). Other than brain tumors, the potential of mPDT has been demonstrated in various other types of cancers, such as colorectal cancer and cervical cancer (Shi et al., 2019; Dai et al., 2023) In a study by Caverzán et al., standard and metronomic regimens of conjugated polymer nanoparticles-based PDT was assessed on glioblastoma cell lines and xenograft mouse models. They reported that mPDT regimes induced increased cancer cell death, and lowered therapeutic resistance furthermore resulted in the polarization of one of the most important cancer-related immune cell subtypes, macrophages, towards an anti-tumoral phenotype (Caverzán et al., 2023).
There exists an additional challenge that needs to be overcome in the application of mPDT; delivery systems enabling the metronomic induction of the treatment. A growing interest has been towards the construction of wireless devices that adhere to the tissue of interest and ensure the induction of PDT in a metronomic manner (Lin et al., 2023). Another strategy is utilizing engineered microneedle devices for the delivery of photosensitizers in mPDT (Dai et al., 2023). The nature of this treatment option calls for interdisciplinary cooperation to help it advance. There is a need for optimization of the delivery systems used in metronomic photodynamic therapy.
In addition, the success of metronomic administration of photodynamic therapy underlines one critical point; that metronomic regimens do not have to be limited to chemotherapeutic uses only. The term ‘‘metronomic’’ refers to the type of temporal administration of the therapeutic agent used. As discussed above, the success of metronomic chemotherapy is mainly attributed to its low toxicity profile and its ability to decrease acquired therapeutic resistance. However, toxicity and resistance concerns are not limited to the use of chemotherapeutics, they are key factors to consider within the applications of other treatment options, too. Given that studies concerning metronomic chemotherapy and metronomic photodynamic therapy have both shown similar results in terms of their efficacy, metronomic therapy can be considered a promising temporal regimen that is not only limited to the chemotherapeutic administrations. This promising hypothesis calls for more studies concerning the use of metronomic administration in other therapeutic administrations also.
6 Limitations and challenges associated with MCT
As in all therapeutic efforts, MCT presents both merits and drawbacks. Recognizing cancer’s multifaceted and complex nature, the suitability of MCT may not be universal across all cases. Particularly in instances where the tumor burden is high, the MCT approach is usually not prioritized as it does not result in rapid shrinkage of the tumor. MCT may not be very suitable for aggressive tumor types also due to the aforementioned rationale. MCT is considered to be a minimally toxic and less resistance-inducing treatment approach, but it comes with the trade-off of the rapid and potent tumor shrinkage that MTD therapy is typically associated with. It is important to recognize the necessity of implementing precision medicine notion here by tailoring treatment strategies to the specific needs and characteristics of the individual medical cases, appreciating the nuances governing therapeutic decision-making by medical professionals.
Both the terms ‘‘metronomic therapy’’ and ‘‘metronomic chemotherapy’’ are used broadly, and there are no clear boundaries for their descriptions. In light of the success of metronomic PDT, we believe that metronomic therapy should be clearly defined as an umbrella term that refers to a certain administration regimen of cancer therapies of many kinds. Hence, metronomic therapy includes MCT, but it is not limited to chemotherapeutic administration alone. We believe there needs to be a clear distinction between the two terms in the literature.
Furthermore, administration of a chemotherapeutic drug at doses lower than MTD within short intervals is considered MCT, but different outcomes emerge when the drug is given under different parameters. The intervals between drug administrations, the dosage, are all among critical factors contributing greatly to the outcome of the treatment. In addition, as in many other therapeutic cases, when the drug is used in combination with another drug or therapy, the effects differ vastly. In a study performed in 2013, Tongu et al. reported that when Cetuximab was administered at 4-day intervals, it weakened the anti-tumor T cell response in mice models, whereas when the drug was administered at 8-day intervals along with Gemcitabine, an increased sensitivity of T-reg cells was observed (Tongu et al., 2013). This phenomenon highlights the need for the optimization of metronomic chemotherapeutic schedules and calls for more research to be performed to overcome this challenge.
Mathematical modeling is another powerful tool that aids in the optimization of MCT. Efforts made within this area help determine the parameters of MCT administration that give rise to the best outcomes without the need for trial-error and countless individual research efforts. Kweon et al., established a mathematical model to optimize the oral metronomic Doxorubicin regimen. Their model evaluated the relationships of several cases and determined the dose, frequency, formulation, and administration parameters that are optimal from both tumor suppression (pharmacodynamic) and cardiac toxicity (toxicodynamic) aspects of metronomic Doxorubicin administration (Kweon et al., 2022). We firmly believe that the mathematical modeling efforts to optimize metronomic chemotherapeutic administrations as such are key to the advancement of metronomic chemotherapy research in the upcoming era.
7 Conclusion and future perspectives
Considerable progress has been made in the treatment of cancer, each with its own set of successes and setbacks. Given the diverse and complex nature of cancer, it is clear that there is no one-size-fits-all treatment plan, which highlights the importance of precision medicine framework and the ongoing need for better therapeutic approaches. Although MTD chemotherapy has demonstrated efficacy in many conditions, there is increasing recognition that, in many cases, it may not only be ineffective but also have the potential to exacerbate the course of the disease when compared to MCT.
A collective amount of evidence from research over the past 2 decades has strengthened the rationale for MCT as a viable treatment option for a variety of cancer types. MCT’s unique benefit, its comparatively low cytotoxicity, opens the door for combinations with other treatment modalities. The efficacy of combining MCT with radiation therapy or immunotherapy indicates that these combinations should be taken into consideration as potential solutions. Furthermore, acquired therapeutic resistance can be efficiently managed with the metronomic administration of treatment modalities beyond standard chemotherapy, as exemplified by metronomic photodynamic therapy.
It is our opinion and firm belief that metronomic therapy must be viewed as an umbrella term and should not be limited to chemotherapeutic administrations (MCT) alone rather applicable to numerous regimens of cancer therapies of several types. Subsequently, this ought to be reflected in the literature.
In conclusion, MCT has many benefits, including cost-effectiveness, favorable toxicity and resistance profiles, and characteristics that support anti-tumor immune responses and anti-angiogenesis. These discoveries have opened up new therapeutic possibilities, which emphasize the significance of additional biological cancer research, clinical trials, and mathematical modeling approaches. These efforts are essential to thoroughly investigate and leverage on the possible advantages provided by MCT, impacting the course of cancer treatment in the future.
Author contributions
OB: Conceptualization, Writing–original draft, Writing–review and editing. SM: Conceptualization, Writing–review and editing. MQ: Conceptualization, Writing–review and editing. AA: Conceptualization, Supervision, Writing–original draft, Writing–review and editing.
Funding
The author(s) declare financial support was received for the research, authorship, and/or publication of this article. AA was supported by the International Fellowship for Outstanding Researchers Program administrated by The Scientific and Technological Research Council of Türkiye (TUBITAK).
Acknowledgments
The authors want to thank their respective institutions for their continued support. AA would like to acknowledge Republic of Türkiye The Council of Higher Education Research Universities Support Program (Grant number: ADEP-108-2022-11202).
Conflict of interest
The authors declare that the research was conducted in the absence of any commercial or financial relationships that could be construed as a potential conflict of interest.
Publisher’s note
All claims expressed in this article are solely those of the authors and do not necessarily represent those of their affiliated organizations, or those of the publisher, the editors and the reviewers. Any product that may be evaluated in this article, or claim that may be made by its manufacturer, is not guaranteed or endorsed by the publisher.
References
Acar, A., Nichol, D., Fernandez-Mateos, J., Cresswell, G. D., Barozzi, I., Hong, S. P., et al. (2020). Exploiting evolutionary steering to induce collateral drug sensitivity in cancer. Nat. Commun. 11 (1), 1923. doi:10.1038/s41467-020-15596-z
Agostinis, P., Berg, K., Cengel, K. A., Foster, T. H., Girotti, A. W., Gollnick, S. O., et al. (2011). Photodynamic therapy of cancer: an update. CA A Cancer J. Clin. 61 (4), 250–281. doi:10.3322/caac.20114
Ali, A. M., and Mohamed, I. E.-S. (2016). Metronomic chemotherapy and radiotherapy as salvage treatment in refractory or relapsed pediatric solid tumours. Curr. Oncol. 23 (3), e253–e259. doi:10.3747/co.23.2873
André, N., Banavali, S., Snihur, Y., and Pasquier, E. (2013). Has the time come for metronomics in low-income and middle-income countries? Lancet Oncol. 14, e239–e248. doi:10.1016/S1470-2045(13)70056-1
Araujo, R. P., and McElwain, D. L. S. (2004). A history of the study of solid tumour growth: the contribution of mathematical modelling. Bull. Math. Biol. 66, 1039–1091. doi:10.1016/j.bulm.2003.11.002
Asowed, M., Elander, N. O., Pettersson, L., Ekholm, M., and Papantoniou, D. (2023). Activity and safety of KEES - an oral multi-drug chemo-hormonal metronomic combination regimen in metastatic castration-resistant prostate cancer. BMC Cancer 23 (1), 309. doi:10.1186/s12885-023-10780-y
Attias, M., Al-Aubodah, T., and Piccirillo, C. A. (2019). Mechanisms of human FoxP3+ Treg cell development and function in health and disease. Clin. Exp. Immunol. 197, 36–51. doi:10.1111/cei.13290
Banissi, C., Ghiringhelli, F., Chen, L., and Carpentier, A. F. (2009). Treg depletion with a low-dose metronomic Temozolomide regimen in a rat glioma model. Cancer Immunol. Immunother. 58 (10), 1627–1634. doi:10.1007/s00262-009-0671-1
Baskar, R., Lee, K. A., Yeo, R., and Yeoh, K. W. (2012). Cancer and radiation therapy: current advances and future directions. Int. J. Med. Sci. 9, 193–199. doi:10.7150/ijms.3635
Baygin, R. C., Yilmaz, K. C., and Ahmet, A. (2024). Characterization of dabrafenib-induced drug insensitivity via cellular barcoding and collateral sensitivity to second-line therapeutics. Sci. Rep. 14 (1), 286. doi:10.1038/s41598-023-50443-3
Benzekry, S., and Hahnfeldt, P. (2013). Maximum tolerated dose versus metronomic scheduling in the treatment of metastatic cancers. J. Theor. Biol. 335, 235–244. doi:10.1016/j.jtbi.2013.06.036
Bergers, G., and Benjamin, L. E. (2003). Tumorigenesis and the angiogenic switch. Nat. Rev. Cancer 3, 401–410. doi:10.1038/nrc1093
Bergmann, N., Delbridge, C., Gempt, J., Feuchtinger, A., Walch, A., Schirmer, L., et al. (2020). The intratumoral heterogeneity reflects the intertumoral subtypes of glioblastoma multiforme: a regional immunohistochemistry analysis. Front. Oncol. 10, 494. doi:10.3389/fonc.2020.00494
Bhattacharyya, G. S., Malhotra, H., Parikh, P. M., Setty Govindbabu, K., Ranade, A., Biswas, G., et al. (2015). Phase II study of metronomic cyclophosphamide and Temozolomide in platinum resistance ovarian cancer. J. Clin. Oncol. 33 (15_Suppl. l), e16575. doi:10.1200/jco.2015.33.15_suppl.e16575
Bisland, S. K., Lilge, L., Lin, A., Rusnov, R., and Wilson, B. C. (2004). Metronomic photodynamic therapy as a new paradigm for photodynamic therapy: rationale and preclinical evaluation of technical feasibility for treating malignant brain tumors. Photochem. Photobiol. 80 (1), 22–30. doi:10.1562/2004-03-05-ra-100.1
Biziota, E., Mavroeidis, L., Hatzimichael, E., and Pappas, P. (2017). Metronomic chemotherapy: a potent macerator of cancer by inducing angiogenesis suppression and antitumor immune activation. Cancer Lett. 400, 243–251. doi:10.1016/j.canlet.2016.12.018
Bocci, G., Tuccori, M., Emmenegger, U., Liguori, V., Falcone, A., Kerbel, R. S., et al. (2005). Cyclophosphamide-methotrexate ‘metronomic’ chemotherapy for the palliative treatment of metastatic breast cancer. A comparative pharmacoeconomic evaluation. Ann. Oncol. 16 (8), 1243–1252. doi:10.1093/annonc/mdi240
Bondarenko, M., Le Grand, M., Shaked, Y., Raviv, Z., Chapuisat, G., Carrère, C., et al. (2021). Metronomic chemotherapy modulates clonal interactions to prevent drug resistance in non-small cell lung cancer. Cancers 13 (9), 2239. doi:10.3390/cancers13092239
Borch, T. H., Engell-Noerregaard, L., Zeeberg Iversen, T., Ellebaek, E., Met, Ö., Hansen, M., et al. (2016). MRNA-transfected dendritic cell vaccine in combination with metronomic cyclophosphamide as treatment for patients with advanced malignant melanoma. OncoImmunology 5 (9), e1207842. doi:10.1080/2162402X.2016.1207842
Bramante, S., Koski, A., Liikanen, I., Vassilev, L., Oksanen, M., Siurala, M., et al. (2016). Oncolytic virotherapy for treatment of breast cancer, including triple-negative breast cancer. OncoImmunology 5 (2), e1078057. doi:10.1080/2162402X.2015.1078057
Brems-Eskildsen, A. S., Linnet, S., Danø, H., Luczak, A., Vestlev, P. M., Hugger Jakobsen, E., et al. (2021). Metronomic treatment of vinorelbine with oral capecitabine is tolerable in the randomized phase 2 study XeNa including patients with HER2 non-amplified metastatic breast cancer. Acta Oncol. 60 (2), 157–164. doi:10.1080/0284186X.2020.1851045
Browder, T., Butterfield, C. E., Kräling, B. M., Shi, B., Marshall, B., O’Reilly, M. S., et al. (2000). Antiangiogenic scheduling of chemotherapy improves efficacy against experimental drug-resistant cancer. Cancer Res. 60 (7), 1878–1886.
Buda-Nowak, A., Kwinta, Ł., Potocki, P., Michałowska-Kaczmarczyk, A., Słowik, A., Konopka, K., et al. (2023). Metronomic chemo-endocrine therapy (FulVEC) as a salvage treatment for patients with advanced, treatment-refractory ER+/HER2-Breast cancer—a retrospective analysis of consecutive patients data. J. Clin. Med. 12 (4), 1350. doi:10.3390/jcm12041350
Caffo, O., Facchini, G., Biasco, E., Ferraù, F., Morelli, F., Donini, M., et al. (2019). Activity and safety of metronomic cyclophosphamide in the modern era of metastatic castration-resistant prostate cancer. Future Oncol. 15 (10), 1115–1123. doi:10.2217/fon-2018-0715
Calvani, N., Franco, M., Naglieri, E., Gnoni, A., Chiuri, V. E., Orlando, L., et al. (2019). Metronomic chemotherapy with cyclophosphamide plus low dose of corticosteroids in advanced castration-resistant prostate cancer across the era of taxanes and new hormonal drugs. Med. Oncol. 36 (9), 80. doi:10.1007/s12032-019-1304-y
Camerini, A., Puccetti, C., Donati, S., Valsuani, C., Petrella, M. C., Tartarelli, G., et al. (2015). Metronomic oral vinorelbine as first-line treatment in elderly patients with advanced non-small cell lung cancer: results of a phase II trial (MOVE trial). BMC Cancer 15 (1), 359. doi:10.1186/s12885-015-1354-2
Cardoso, F., Costa, A., Senkus, E., Aapro, M., André, F., Barrios, C. H., et al. (2017). 3rd ESO-ESMO international consensus Guidelines for advanced breast cancer (ABC 3). Ann. Oncol. 28 (1), 16–33. doi:10.1093/annonc/mdw544
Carmeliet, P., and Jain, R. K. (2000). Angiogenesis in cancer and other diseases. Nature 407, 249–257. doi:10.1038/35025220
Caverzán, M. D., Oliveda, P. M., Beaugé, L., Palacios, R. E., Chesta, C. A., and Ibarra, L. E. (2023). Metronomic photodynamic therapy with conjugated polymer nanoparticles in glioblastoma tumor microenvironment. Cells 12 (11), 1541. doi:10.3390/cells12111541
Cazzaniga, M. E., Capici, S., Cordani, N., Cogliati, V., Fulvia Pepe, F., Riva, F., et al. (2022). Metronomic chemotherapy for metastatic breast cancer treatment: clinical and preclinical data between lights and shadows. J. Clin. Med. 11, 4710. doi:10.3390/jcm11164710
Cazzaniga, M. E., Cortesi, L., Ferzi, A., Scaltriti, L., Cicchiello, F., Ciccarese, M., et al. (2016). Metronomic chemotherapy with oral vinorelbine (MVNR) and capecitabine (MCAPE) in advanced HER2-negative breast cancer patients: is it a way to optimize disease control? Final results of the VICTOR-2 study. Breast Cancer Res. Treat. 160 (3), 501–509. doi:10.1007/s10549-016-4009-3
Chai, Y., Liu, J., Jiang, M., He, M., Wang, Z., Ma, F., et al. (2023). A phase II study of a doublet metronomic chemotherapy regimen consisting of oral vinorelbine and capecitabine in Chinese women with HER2-negative metastatic breast cancer. Thorac. Cancer 14 (23), 2259–2268. doi:10.1111/1759-7714.15011
Chalkidou, K., Marquez, P., Dhillon, P. K., Teerawattananon, Y., Anothaisintawee, T., Gadelha, C. A. G., et al. (2014). Evidence-informed frameworks for cost-effective cancer care and prevention in low, middle, and high-income countries. Lancet Oncol. (13), 70547–70553. doi:10.1016/S1470-2045
Cheema, T. A., Kanai, R., Kim, G. W., Wakimoto, H., Passer, B., Rabkin, S. D., et al. (2011). Enhanced antitumor efficacy of low-dose etoposide with oncolytic herpes simplex virus in human glioblastoma stem cell xenografts. Clin. Cancer Res. 17 (23), 7383–7393. doi:10.1158/1078-0432.CCR-11-1762
Chen, Yu P., Liu, Xu, Zhou, Q., Yang, K.Yu, Jin, F., Zhu, X. D., et al. (2021). Metronomic capecitabine as adjuvant therapy in locoregionally advanced nasopharyngeal carcinoma: a multicentre, open-label, parallel-group, randomised, controlled, phase 3 trial. Lancet 398 (10297), 303–313. doi:10.1016/S0140-6736(21)01123-5
Choi, L.Mi R., Rood, B., Kamani, N., Fond, D.La, Packer, R. J., Santi, M. R., et al. (2008). Feasibility of metronomic maintenance chemotherapy following high-dose chemotherapy for malignant central nervous system tumors. Pediatr. Blood Cancer 50 (5), 970–975. doi:10.1002/pbc.21381
Chung, S. W., Kweon, S., Lee, B. S., Kim, G. C., Mahmud, F., Lee, H., et al. (2017). Radiotherapy-assisted tumor selective metronomic oral chemotherapy. Int. J. Cancer 141 (9), 1912–1920. doi:10.1002/ijc.30842
Colleoni, M., Rocca, A., Sandri, M. T., Zorzino, L., Masci, G., Nolè, F., et al. (2002). Low-dose oral methotrexate and cyclophosphamide in metastatic breast cancer: antitumor activity and correlation with vascular endothelial growth factor levels. Ann. Oncol. 13 (1), 73–80. doi:10.1093/annonc/mdf013
Correia, A. S., Gärtner, F., and Vale, N. (2021). Drug combination and repurposing for cancer therapy: the example of breast cancer. Heliyon 7, e05948. doi:10.1016/j.heliyon.2021.e05948
Curtis, L. T., van Berkel, V. H., and Frieboes, H. B. (2018). Pharmacokinetic/pharmacodynamic modeling of combination-chemotherapy for lung cancer. J. Theor. Biol. 448, 38–52. doi:10.1016/j.jtbi.2018.03.035
Dabkara, D., Ganguly, S., Biswas, B., and Ghosh, J. (2018). Metronomic therapy in metastatic castrate-resistant prostate cancer: experience from a tertiary cancer care center. Indian J. Cancer 55, 94–97. doi:10.4103/ijc.IJC_346_17
Dagogo-Jack, I., and Shaw, A. T. (2018). Tumour heterogeneity and resistance to cancer therapies. Nat. Rev. Clin. Oncol. 15, 81–94. doi:10.1038/nrclinonc.2017.166
Dai, J., Wei, S., Xu, J., Xue, H., Chen, Z., Wu, M., et al. (2023). Microneedle device delivering aggregation-induced emission photosensitizers for enhanced metronomic photodynamic therapy of cancer. ACS Appl. Mater. Interfaces 15 (13), 16526–16538. doi:10.1021/acsami.3c01682
Danisik, N., Celikbas Yilmaz, K., and Acar, A. (2023). Identification of collateral sensitivity and evolutionary landscape of chemotherapy-induced drug resistance using cellular barcoding technology. Front. Pharmacol. 14, 1178489. doi:10.3389/fphar.2023.1178489
Davies, N., and Wilson, B. C. (2007). Interstitial in vivo ALA-PpIX mediated metronomic photodynamic therapy (MPDT) using the CNS-1 astrocytoma with bioluminescence monitoring. Photodiagnosis Photodyn. Ther. 4 (3), 202–212. doi:10.1016/j.pdpdt.2007.06.002
Davis, J. J., and Fang, B. (2005). Oncolytic virotherapy for cancer treatment: challenges and solutions. J. Gene Med. 7, 1380–1389. doi:10.1002/jgm.800
Delbaldo, C., Michiels, S., Syz, N., Charles Soria, J., Le Chevalier, T., and Pignon, J. P. (2004). Benefits of adding a drug to a single-agent or a 2-agent chemotherapy regimen in advanced non-small-cell lung cancer: a meta-analysis. JAMA 292, 470–484. doi:10.1001/jama.292.4.470
Emadi, A., Jones, R. J., and Brodsky, R. A. (2009). Cyclophosphamide and cancer: golden anniversary. Nat. Rev. Clin. Oncol. 6, 638–647. doi:10.1038/nrclinonc.2009.146
Emmenegger, U., Man, S., Shaked, Y., Francia, G., Wong, J. W., Hicklin, D. J., et al. (2004). A comparative analysis of low-dose metronomic cyclophosphamide reveals absent or low-grade toxicity on tissues highly sensitive to the toxic effects of maximum tolerated dose regimens. Cancer Res. 64 (11), 3994–4000. doi:10.1158/0008-5472.CAN-04-0580
Fares, J. E., Paul, El T., Khalil, L. E., Atwani, R. W., Moukadem, H. A., Awada, A., et al. (2020). Metronomic chemotherapy for patients with metastatic breast cancer: review of effectiveness and potential use during pandemics. Cancer Treat. Rev. 89, 102066. doi:10.1016/j.ctrv.2020.102066
Folkins, C., Man, S., Xu, P., Shaked, Y., Hicklin, D. J., and Kerbel, R. S. (2007). Anticancer therapies combining antiangiogenic and tumor cell cytotoxic effects reduce the tumor stem-like cell fraction in glioma xenograft tumors. Cancer Res. 67 (8), 3560–3564. doi:10.1158/0008-5472.CAN-06-4238
Foo, J., and Michor, F. (2010). Evolution of resistance to anti-cancer therapy during general dosing schedules. J. Theor. Biol. 263 (2), 179–188. doi:10.1016/j.jtbi.2009.11.022
Foo, J., and Michor, F. (2014). Evolution of acquired resistance to anti-cancer therapy. J. Theor. Biol. 355, 10–20. doi:10.1016/j.jtbi.2014.02.025
Fousseyni, T., Diawara, M., Pasquier, E., and André, N. (2011). Children treated with metronomic chemotherapy in a low-income country: METRO-Mali-01. J. Pediatr. Hematology/Oncology 33 (1), 31–34. doi:10.1097/MPH.0b013e3182018ab8
Frydrychowicz, M., Boruczkowski, M., Kolecka-Bednarczyk, A., and Dworacki, G. (2017). The dual role of Treg in cancer. Scand. J. Immunol. 86, 436–443. doi:10.1111/sji.12615
Gallasch, R., Efremova, M., Charoentong, P., Hackl, H., and Trajanoski, Z. (2013). Mathematical models for translational and clinical oncology. J. Clin. Bioinforma. 3, 23. doi:10.1186/2043-9113-3-23
Ge, Y., Domschke, C., Stoiber, N., Schott, S., Heil, J., Rom, J., et al. (2012). Metronomic cyclophosphamide treatment in metastasized breast cancer patients: immunological effects and clinical outcome. Cancer Immunol. Immunother. 61 (3), 353–362. doi:10.1007/s00262-011-1106-3
Generali, D., Bates, G., Berruti, A., Brizzi, M. P., Campo, L., Bonardi, S., et al. (2009). Immunomodulation of FOXP3+ regulatory T cells by the aromatase inhibitor Letrozole in breast cancer patients. Clin. Cancer Res. 15 (3), 1046–1051. doi:10.1158/1078-0432.CCR-08-1507
Ghiringhelli, F., Menard, C., Puig, P. E., Ladoire, S., Roux, S., Martin, F., et al. (2007). Metronomic cyclophosphamide regimen selectively depletes CD4 +CD25+ regulatory T cells and restores T and NK effector functions in end stage cancer patients. Cancer Immunol. Immunother. 56 (5), 641–648. doi:10.1007/s00262-006-0225-8
Goldman, A., Majumder, B., Dhawan, A., Ravi, S., Goldman, D., Kohandel, M., et al. (2015). Temporally sequenced anticancer drugs overcome adaptive resistance by targeting a vulnerable chemotherapy-induced phenotypic transition. Nat. Commun. 6, 6139. doi:10.1038/ncomms7139
Granito, A., Marinelli, S., Terzi, E., Piscaglia, F., Renzulli, M., Venerandi, L., et al. (2015). Metronomic capecitabine as second-line treatment in hepatocellular carcinoma after sorafenib failure. Dig. Liver Dis. 47 (6), 518–522. doi:10.1016/j.dld.2015.03.010
Grzywa, T. M., Koppolu, A. A., Paskal, W., Klicka, K., Rydzanicz, M., Wejman, J., et al. (2021). Higher mutation burden in high proliferation compartments of heterogeneous melanoma tumors. Int. J. Mol. Sci. 22 (8), 3886. doi:10.3390/ijms22083886
Gun, S. Y., Lee, S. W. L., Lin Sieow, Je, and Wong, S. C. (2019). Targeting immune cells for cancer therapy. Redox Biol. 25, 101174. doi:10.1016/j.redox.2019.101174
Hall, M. R., Dehbi, H. M., Banerjee, S., Lord, R., Clamp, A., Ledermann, J. A., et al. (2020). A phase II randomised, placebo-controlled trial of low dose (metronomic) cyclophosphamide and nintedanib (BIBF1120) in advanced ovarian, fallopian tube or primary peritoneal cancer. Gynecol. Oncol. 159 (3), 692–698. doi:10.1016/j.ygyno.2020.09.048
Hanahan, D., Bergers, G., and Bergsland, E. (2000). Less is, more, regularly: metronomic dosing of cytotoxic drugs can target tumor angiogenesis in mice. J. Clin. Investigation 105, 1045–1047. doi:10.1172/JCI9872
Hanahan, D., and Weinberg, R. A. (2011). Hallmarks of cancer: the next generation. Cell 144, 646–674. doi:10.1016/j.cell.2011.02.013
Harrington, K., Freeman, D. J., Kelly, B., Harper, J., and Soria, J. C. (2019). Optimizing oncolytic virotherapy in cancer treatment. Nat. Rev. Drug Discov. 18, 689–706. doi:10.1038/s41573-019-0029-0
Harsh, K. K., Jakhar, S. L., Maheshwari, G., Nirban, R., Kumari, P., Beniwal, S. K., et al. (2023). Triple combination of palliative oral metronomic chemotherapy in recurrent and metastatic epithelial ovarian cancer: a retrospective study. Indian J. Cancer 60 (2), 167–172. doi:10.4103/ijc.IJC_143_20
Haubitz, M. (2007). Acute and long-term toxicity of cyclophosphamide. Transplantationsmedizin Organ Der Dtsch. Transplantationsgesellschaft 19 (2).
Hayashi, M. T., and Karlseder, J. (2013). DNA damage associated with mitosis and cytokinesis failure. Oncogene 32, 4593–4601. doi:10.1038/onc.2012.615
He, Q., Li, J., Yin, W., Song, Z., Zhang, Z., Yi, T., et al. (2011). Low-dose Paclitaxel enhances the anti-tumor efficacy of GM-CSF surface-modified whole-tumor-cell vaccine in mouse model of prostate cancer. Cancer Immunol. Immunother. 60 (5), 715–730. doi:10.1007/s00262-011-0988-4
Hermans, I. F., Wen Chong, T., Palmowski, M. J., Harris, A. L., and Vincenzo, C. (2003). Synergistic effect of metronomic dosing of cyclophosphamide combined with specific antitumor immunotherapy in a murine melanoma model. Cancer Res. 63 (23), 8408–8413.
Hida, K., Maishi, N., Annan, D. A., and Hida, Y. (2018). Contribution of tumor endothelial cells in cancer progression. Int. J. Mol. Sci. 19, 1272. doi:10.3390/ijms19051272
Ho, P. J., Gernaat, S. A. M., Hartman, M., and Verkooijen, H. M. (2018). Health-related quality of life in asian patients with breast cancer: a systematic review. BMJ Open 8, e020512. doi:10.1136/bmjopen-2017-020512
Huang, Z., and Bao, S. D. (2004). Roles of main pro- and anti-angiogenic factors in tumor angiogenesis. World J. Gastroenterology 10, 463–470. doi:10.3748/wjg.v10.i4.463
Ilango, S., Paital, B., Jayachandran, P., Padma, P. R., and Nirmaladevi, R. (2020). Epigenetic alterations in cancer. Front. Biosci. - Landmark 25, 1058–1109. doi:10.2741/4847
Ingemarsdotter, C. K., Tookman, L. A., Browne, A., Pirlo, K., Cutts, R., Chelela, C., et al. (2015). Paclitaxel resistance increases oncolytic adenovirus efficacy via upregulated CAR expression and dysfunctional cell cycle control. Mol. Oncol. 9 (4), 791–805. doi:10.1016/j.molonc.2014.12.007
Kamat, A. A., Kim, T. J., Landen, C. N., Lu, C., Han, L. Y., Lin, Y. G., et al. (2007). Metronomic chemotherapy enhances the efficacy of antivascular therapy in ovarian cancer. Cancer Res. 67 (1), 281–288. doi:10.1158/0008-5472.CAN-06-3282
Kareva, I., Waxman, D. J., and Klement, G. L. (2015). Metronomic chemotherapy: an attractive alternative to maximum tolerated dose therapy that can activate anti-tumor immunity and minimize therapeutic resistance. Cancer Lett. 358, 100–106. doi:10.1016/j.canlet.2014.12.039
Kaufmann, S. H., and Earnshaw, W. C. (2000). Induction of apoptosis by cancer chemotherapy. Exp. Cell Res. 256 (1), 42–49. doi:10.1006/excr.2000.4838
Kelderman, S., Schumacher, T. N. M., and Haanen, J. B. A. G. (2014). Acquired and intrinsic resistance in cancer immunotherapy. Mol. Oncol. 8, 1132–1139. doi:10.1016/j.molonc.2014.07.011
Kerbel, R. S., and Kamen, B. A. (2004). The anti-angiogenic basis of metronomic chemotherapy. Nat. Rev. Cancer 4, 423–436. doi:10.1038/nrc1369
Khan, K. A., Ponce de Léon, J. L., Benguigui, M., Xu, P., Chow, A., Cruz-Muñoz, W., et al. (2020). Immunostimulatory and anti-tumor metronomic cyclophosphamide regimens assessed in primary orthotopic and metastatic murine breast cancer. Npj Breast Cancer 6 (1), 29. doi:10.1038/s41523-020-0171-1
Klement, G., Baruchel, S., Rak, J., Man, S., Clark, K., Hicklin, D. J., et al. (2000). Continuous low-dose therapy with vinblastine and VEGF receptor-2 antibody induces sustained tumor regression without overt toxicity. J. Clin. Investigation 105 (8), R15–R24. doi:10.1172/JCI8829
Knipper, S., Mandel, P., Amsberg, G. v., Strölin, P., Graefen, M., and Steuber, T. (2019). Metronomische chemotherapie mit oralem cyclophosphamid. Der Urol. 58 (4), 410–417. doi:10.1007/s00120-018-0679-2
Krajnak, S., Decker, T., Schollenberger, L., Rosé, C., Ruckes, C., Fehm, T., et al. (2021). Phase II study of metronomic treatment with daily oral vinorelbine as first-line chemotherapy in patients with advanced/metastatic HR+/HER2− breast cancer resistant to endocrine therapy: VinoMetro—AGO-B-046. J. Cancer Res. Clin. Oncol. 147 (11), 3391–3400. doi:10.1007/s00432-021-03599-2
Krajnak, S., Krajnakova, J., Anic, K., Almstedt, K., Heimes, A. S., Linz, V. C., et al. (2023). Real-world experience of metronomic chemotherapy in metastatic breast cancer: results of a retrospective unicenter study. Breast Care 18 (2), 97–105. doi:10.1159/000528042
Krajnak, S., Schnatz, C., Almstedt, K., Brenner, W., Haertner, F., Heimes, A. S., et al. (2020). Low-dose metronomic chemotherapy as an efficient treatment option in metastatic breast cancer—results of an exploratory case–control study. Breast Cancer Res. Treat. 182 (2), 389–399. doi:10.1007/s10549-020-05711-5
Kweon, S., Jeong, Y. S., Chung, S. W., Lee, H., Lee, Ha K., Jin Park, S., et al. (2022). Metronomic dose-finding approach in oral chemotherapy by experimentally-driven integrative mathematical modeling: mathematical modeling for oral metronomic therapy of Doxorubicin. Biomaterials 286, 121584. doi:10.1016/j.biomaterials.2022.121584
Kwiatkowski, S., Knap, B., Przystupski, D., Saczko, J., Kędzierska, E., Knap-Czop, K., et al. (2018). Photodynamic therapy – mechanisms, photosensitizers and combinations. Biomed. Pharmacother. 106, 1098–1107. doi:10.1016/j.biopha.2018.07.049
Lago, D., Lambert Uwimana, A., Coens, C., Vuylsteke, P., Curigliano, G., Brouwers, B., et al. (2022). Health-related quality of life in older patients with HER2+ metastatic breast cancer: comparing pertuzumab plus trastuzumab with or without metronomic chemotherapy in a randomised open-label phase II clinical trial. J. Geriatric Oncol. 13 (5), 582–593. doi:10.1016/j.jgo.2022.01.009
Lapidot, T., Sirard, C., Vormoor, J., Murdoch, B., Hoang, T., Caceres-Cortes, J., et al. (1994). A cell initiating human acute myeloid leukaemia after transplantation into SCID mice. Nature 367 (6464), 645–648. doi:10.1038/367645a0
Leary, M., Heerboth, S., Lapinska, K., and Sarkar, S. (2018). Sensitization of drug resistant cancer cells: a matter of combination therapy. Cancers 10, 483. doi:10.3390/cancers10120483
Ledzewicz, U., and Schättler, H. (2014). A review of optimal chemotherapy protocols: from MTD towards metronomic therapy. Math. Model. Nat. Phenom. 9, 131-152. doi:10.1051/mmnp/20149409
Leon, G., MacDonagh, L., Finn, S. P., Cuffe, S., and Barr, M. P. (2016). Cancer stem cells in drug resistant lung cancer: targeting cell surface markers and signaling pathways. Pharmacol. Ther. 158, 71–90. doi:10.1016/j.pharmthera.2015.12.001
Leong, W.In, Ames, R. Y., Haverkamp, J. M., Torres, L., Kline, J., Bans, A., et al. (2019). Low-dose metronomic cyclophosphamide complements the actions of an intratumoral C-class CpG TLR9 agonist to potentiate innate immunity and drive potent T cell-mediated anti-tumor responses. Oncotarget 10 (68), 7220–7237. doi:10.18632/oncotarget.27322
Li, J. wei, Wen, jia Z., Ivanova, D., Jia, X. qing, Lei, Li, and Liu, G. yu (2019). Metronomic capecitabine combined with aromatase inhibitors for new chemoendocrine treatment of advanced breast cancer: a phase II clinical trial. Breast Cancer Res. Treat. 173 (2), 407–415. doi:10.1007/s10549-018-5024-3
Li, M., Lin, C., Lin, J., Chen, S., Weng, L., and He, Z. (2023). Efficacy of osimertinib continuation plus metronomic oral vinorelbine for EGFRmutant advanced NSCLC beyond limited progression on osimertinib. Anti-Cancer Agents Med. Chem. 23 (19), 2095–2101. doi:10.2174/1871520623666230803142758
Liikanen, I., Ahtiainen, L., Hirvinen, M. L., Bramante, S., Cerullo, V., Nokisalmi, P., et al. (2013). Oncolytic adenovirus with Temozolomide induces autophagy and antitumor immune responses in cancer patients. Mol. Ther. 21 (6), 1212–1223. doi:10.1038/mt.2013.51
Lin, T., Zou, P., Lin, R., Guan, H., Fang, Z., Chen, J., et al. (2023). A self-powered wireless detachable drug/light injector for metronomic photodynamic therapy in cancer treatment. Nano Energy 116, 108826. doi:10.1016/j.nanoen.2023.108826
Liu, C. T., Hsieh, M. C., Su, Yu Li, Hung, C. M., Pei, S. N., Liao, C. K., et al. (2021). Metronomic vinorelbine is an excellent and safe treatment for advanced breast cancer: a retrospective, observational study. J. Cancer 12 (17), 5355–5364. doi:10.7150/jca.60682
Liu, J., Dang, H., and Wang, X. W. (2018). The significance of intertumor and intratumor heterogeneity in liver cancer. Exp. Mol. Med. 50, e416. doi:10.1038/emm.2017.165
Livshits, Z., Rao, R. B., and Smith, S. W. (2014). An approach to chemotherapy-associated toxicity. Emerg. Med. Clin. N. Am. 32, 167–203. doi:10.1016/j.emc.2013.09.002
Majumder, D., and Mukherjee, A. (2007). Mathematical modelling of toxicity-related trade-offs in metronomic chemotherapy. IET Syst. Biol. 1, 298–305. doi:10.1049/iet-syb:20060085
Martins, S., Da, M., and Piccirillo, C. A. (2012). Functional stability of Foxp3+ regulatory T cells. Trends Mol. Med. 18, 454–462. doi:10.1016/j.molmed.2012.06.001
Matlock, K., Berlow, N., Keller, C., and Pal, R. (2017). Combination therapy design for maximizing sensitivity and minimizing toxicity. BMC Bioinforma. 18, 116. doi:10.1186/s12859-017-1523-1
McConnell, E. E. (1989). The maximum tolerated dose: the debate. Int. J. Toxicol. 8 (6), 1115–1120. doi:10.3109/10915818909018071
Mejia Peña, C., Skipper, T. A., Hsu, J., Schechter, I., Ghosh, D., and Dawson, M. R. (2023). Metronomic and single high-dose Paclitaxel treatments produce distinct heterogenous chemoresistant cancer cell populations. Sci. Rep. 13, 19232. doi:10.1038/s41598-023-46055-6
Mokhtari, R. B., Homayouni, T. S., Baluch, N., Morgatskaya, E., Kumar, S., Das, B., et al. (2017). Combination therapy in combating cancer. Oncotarget 8, 38022–38043. doi:10.18632/oncotarget.16723
Montagna, E., Palazzo, A., Maisonneuve, P., Cancello, G., Iorfida, M., Sciandivasci, A., et al. (2017). Safety and efficacy study of metronomic vinorelbine, cyclophosphamide plus capecitabine in metastatic breast cancer: a phase II trial. Cancer Lett. 400, 276–281. doi:10.1016/j.canlet.2017.01.027
Moolgavkar, S. H. (1991). Carcinogenesis models: an overview. Basic Life Sci. 58, 387–396. doi:10.1007/978-1-4684-7627-9_14
Mpekris, F., Voutouri, C., Panagi, M., Baish, J. W., Jain, R. K., Stylianopoulos, T., et al. (2022). Normalizing tumor microenvironment with nanomedicine and metronomic therapy to improve immunotherapy. J. Controll. Release 345, 190–199. doi:10.1016/j.jconrel.2022.03.008
Mundo, A. I., Muhammad, A., Kerlin, B., Nelson, C. E., and Muldoon, T. J. (2022). Longitudinal examination of perfusion and angiogenesis markers in primary colorectal tumors shows distinct signatures for metronomic and maximum-tolerated dose strategies. Neoplasia (United States) 32, 100825. doi:10.1016/j.neo.2022.100825
Muñoz, R., Girotti, A., Hileeto, D., and Arias, F. J. (2021). Metronomic anti-cancer therapy: a multimodal therapy governed by the tumor microenvironment. Cancers 13, 5414. doi:10.3390/cancers13215414
Munzone, E., and Colleoni, M. (2015). Clinical overview of metronomic chemotherapy in breast cancer. Nat. Rev. Clin. Oncol. 12, 631–644. doi:10.1038/nrclinonc.2015.131
NarendraBodduluru, L., Reddy, K. E., Shantikumar, S., and Ramakrishna, S. (2013). Immune system: a double-edged sword in cancer. Inflamm. Res. doi:10.1007/s00011-013-0645-9
Nasr, K. E., Osman, M. A., Elkady, M. S., and Ellithy, M. A. (2015). Metronomic methotrexate and cyclophosphamide after carboplatin included adjuvant chemotherapy in triple negative breast cancer: a phase III study. Ann. Transl. Med. 3 (19), 284. doi:10.3978/j.issn.2305-5839.2015.11.14
Natale, G., and Bocci, G. (2018). Does metronomic chemotherapy induce tumor angiogenic dormancy? A review of available preclinical and clinical data. Cancer Lett. 432, 28–37. doi:10.1016/j.canlet.2018.06.002
Nishida, N., Yano, H., Nishida, T., Kamura, T., and Kojiro, M. (2006). Angiogenesis in cancer. Vasc. Health Risk Manag. 2, 213–219. doi:10.2147/vhrm.2006.2.3.213
Noronha, V., Patil, V. M., Menon, N. S., Joshi, A., Goud, S., More, S., et al. (2022). Oral metronomic chemotherapy after definitive chemoradiation in esophageal squamous cell carcinoma: a randomized clinical trial. Esophagus 19 (4), 670–682. doi:10.1007/s10388-022-00923-8
Ohue, Y., and Nishikawa, H. (2019). Regulatory T (Treg) cells in cancer: can Treg cells Be a new therapeutic target? Cancer Sci. 110, 2080–2089. doi:10.1111/cas.14069
Onimaru, M., Ohuchida, K., Nagai, E., Mizumoto, K., Egami, T., Cui, L., et al. (2010). Combination with low-dose gemcitabine and HTERT-promoter-dependent conditionally replicative adenovirus enhances cytotoxicity through their crosstalk mechanisms in pancreatic cancer. Cancer Lett. 294 (2), 178–186. doi:10.1016/j.canlet.2010.01.034
Orlando, L., Lorusso, V., Giotta, F., Di Maio, M., Schiavone, P., Fedele, P., et al. (2020). Metronomic oral chemotherapy with cyclophosphamide plus capecitabine combined with trastuzumab (hex) as first line therapy of HER-2 positive advanced breast cancer: a phase II trial of the gruppo oncologico italia meridionale (GOIM). Breast 53, 18–22. doi:10.1016/j.breast.2020.06.002
Paluskievicz, C. M., Cao, X., Abdi, R., Pan, Z., Liu, Y., and Bromberg, J. S. (2019). T regulatory cells and priming the suppressive tumor microenvironment. Front. Immunol. 10, 2453. doi:10.3389/fimmu.2019.02453
Parhi, P., Mohanty, C., and Sahoo, S. K. (2012). Nanotechnology-based combinational drug delivery: an emerging approach for cancer therapy. Drug Discov. Today 17, 1044–1052. doi:10.1016/j.drudis.2012.05.010
Pasqualini, C., Rubino, J., Brard, C., Cassard, L., André, N., Rondof, W., et al. (2021). Phase II and biomarker study of Programmed cell death protein 1 inhibitor nivolumab and metronomic cyclophosphamide in paediatric relapsed/refractory solid tumours: arm G of AcSé-ESMART, a trial of the European innovative therapies for children with cancer consortium. Eur. J. Cancer 150, 53–62. doi:10.1016/j.ejca.2021.03.032
Patil, V., Noronha, V., Dhumal, S. B., Joshi, A., Menon, N., Bhattacharjee, A., et al. (2020). Low-cost oral metronomic chemotherapy versus intravenous cisplatin in patients with recurrent, metastatic, inoperable head and neck carcinoma: an open-label, parallel-group, non-inferiority, randomised, phase 3 trial. Lancet Glob. Health 8 (9), e1213–e1222. doi:10.1016/S2214-109X(20)30275-8
Patil, V. M., Noronha, V., Joshi, A., Dhumal, S., Mahimkar, M., Bhattacharjee, A., et al. (2019). Phase I/II study of palliative triple metronomic chemotherapy in platinum-refractory/early-failure oral cancer. J. Clin. Oncol. 37 (32), 3032–3041. doi:10.1200/JCO.19.01076
Patwardhan, G. A., Marczyk, M., Wali, V. B., Stern, D. F., Pusztai, L., and Hatzis, C. (2021). Treatment scheduling effects on the evolution of drug resistance in heterogeneous cancer cell populations. Npj Breast Cancer 7 (1), 60. doi:10.1038/s41523-021-00270-4
Perroud, H. A., Rico, M. J., Alasino, C. M., Pezzotto, S. M., Rozados, V. R., and Graciela Scharovsky, O. (2013). Association between baseline VEGF/SVEGFR-2 and VEGF/TSP-1 ratios and response to metronomic chemotherapy using cyclophosphamide and Celecoxib in patients with advanced breast cancer. Indian J. Cancer 50, 115–121. doi:10.4103/0019-509X.117031
Peyrl, A., Chocholous, M., Sabel, M., Lassaletta, A., Sterba, J., Leblond, P., et al. (2023). Sustained survival benefit in recurrent medulloblastoma by a metronomic antiangiogenic regimen: a nonrandomized controlled trial. JAMA Oncol. 9, 1688–1695. doi:10.1001/jamaoncol.2023.4437
Pietras, K., and Douglas, H. (2005). A multitargeted, metronomic, and maximum-tolerated dose ‘chemo-switch’ regimen is antiangiogenic, producing objective responses and survival benefit in a mouse model of cancer. J. Clin. Oncol. 23 (5), 939–952. doi:10.1200/JCO.2005.07.093
Provencio, M., Majem, M., Guirado, M., Massuti, B., de las Peñas, R., Ortega, A. L., et al. (2021). Phase II clinical trial with metronomic oral vinorelbine and tri-weekly cisplatin as induction therapy, subsequently concomitant with radiotherapy (RT) in patients with locally advanced, unresectable, non-small cell lung cancer (NSCLC). Analysis of survival and value of CtDNA for patient selection. Lung Cancer 153, 25–34. doi:10.1016/j.lungcan.2021.01.005
Qiao, J., Wang, H., Kottke, T., White, C., Twigger, K., Diaz, R. M., et al. (2008). Cyclophosphamide facilitates antitumor efficacy against subcutaneous tumors following intravenous delivery of reovirus. Clin. Cancer Res. 14 (1), 259–269. doi:10.1158/1078-0432.CCR-07-1510
Quirk, B. J., Brandal, G., Donlon, S., Vera, J. C., Mang, T. S., Foy, A. B., et al. (2015). Photodynamic therapy (PDT) for malignant brain tumors - where do we stand? Photodiagnosis Photodyn. Ther. 12, 530–544. doi:10.1016/j.pdpdt.2015.04.009
Rajabi, M., and Mousa, S. A. (2017). The role of angiogenesis in cancer treatment. Biomedicines 5, 34. doi:10.3390/biomedicines5020034
Raskov, H., Orhan, A., Christensen, J. P., and Gögenur, I. (2021). Cytotoxic CD8+ T cells in cancer and cancer immunotherapy. Br. J. Cancer 124, 359–367. doi:10.1038/s41416-020-01048-4
Robison, N. J., Campigotto, F., Chi, S. N., Manley, P. E., Turner, C. D., Zimmerman, M. A., et al. (2014). A phase II trial of a multi-agent oral antiangiogenic (metronomic) regimen in children with recurrent or progressive cancer. Pediatr. Blood Cancer 61 (4), 636–642. doi:10.1002/pbc.24794
Romiti, A., Falcone, R., Roberto, M., and Marchetti, P. (2017). Current achievements and future perspectives of metronomic chemotherapy. Investig. New Drugs 35, 359–374. doi:10.1007/s10637-016-0408-x
Salem, A. R., Martínez Pulido, P., Sanchez, F., Sanchez, Y., Español, A. J., and Sales, M. E. (2020). Effect of low dose metronomic therapy on MCF-7 tumor cells growth and angiogenesis. Role of muscarinic acetylcholine receptors. Int. Immunopharmacol. 84, 106514. doi:10.1016/j.intimp.2020.106514
Sales, E. (2018). Breast cancer: metronomic therapy focused on muscarinic acetylcholine receptors. Integr. Cancer Sci. Ther. 5 (5). doi:10.15761/icst.1000291
Salmaggi, A., Milanesi, I., Silvani, A., Gaviani, P., Marchetti, M., Fariselli, L., et al. (2013). Prospective study of carmustine wafers in combination with 6-month metronomic Temozolomide and radiation therapy in newly diagnosed glioblastoma: preliminary results. J. Neurosurg. 118 (4), 821–829. doi:10.3171/2012.12.JNS111893
Scharovsky, O. G., José Rico, M., Mainetti, L. E., Perroud, H. A., and Rozados, V. R. (2020). Achievements and challenges in the use of metronomics for the treatment of breast cancer. Biochem. Pharmacol. 175, 113909. doi:10.1016/j.bcp.2020.113909
Scharovsky, O. G., Mainetti, L. E., and Rozados, V. R. (2009). Metronomic chemotherapy: changing the paradigm that more is better. Curr. Oncol. 16, 7–15. doi:10.3747/co.v16i2.420
Schito, L., Rey, S., Xu, P., Man, S., Cruz-Muñoz, W., and Kerbel, R. S. (2020). Metronomic chemotherapy offsets HIFα induction upon maximum-tolerated dose in metastatic cancers. EMBO Mol. Med. 12 (9), e11416. doi:10.15252/emmm.201911416
Schrag, D. (2004). The price tag on progress — chemotherapy for colorectal cancer. N. Engl. J. Med. 351 (4), 317–319. doi:10.1056/nejmp048143
Schwartzberg, L. S., Wang, G., Somer, B. G., Johnetta Blakely, L., Wheeler, B. M., Walker, M. S., et al. (2014). Phase II trial of fulvestrant with metronomic capecitabine for postmenopausal women with hormone receptor-positive, HER2-negative metastatic breast cancer. Clin. Breast Cancer 14 (1), 13–19. doi:10.1016/j.clbc.2013.09.003
Sharma, A., Malik, P., Khurana, S., Kumar, S., Bhatla, N., Ray, M., et al. (2019). Oral metronomic chemotherapy for recurrent and refractory epithelial ovarian cancer: a retrospective analysis. Indian J. Med. Res. 150 (6), 575–583. doi:10.4103/ijmr.IJMR_2030_18
Sharma, A., Singh, M., Chauhan, R., Singh Malik, P., Khurana, S., Mathur, S., et al. (2021). Pazopanib based oral metronomic therapy for platinum resistant/refractory epithelial ovarian cancer: a phase II, open label, randomized, controlled trial. Gynecol. Oncol. 162 (2), 382–388. doi:10.1016/j.ygyno.2021.05.025
Shevchenko, I., Karakhanova, S., Soltek, S., Link, J., Bayry, J., Werner, J., et al. (2013). Low-dose gemcitabine depletes regulatory T cells and improves survival in the orthotopic Panc02 model of pancreatic cancer. Int. J. Cancer 133 (1), 98–107. doi:10.1002/ijc.27990
Shevyrev, D., and Tereshchenko, V. (2020). Treg heterogeneity, function, and homeostasis. Front. Immunol. 10, 3100. doi:10.3389/fimmu.2019.03100
Shewach, D. S., and Kuchta, R. D. (2009). Introduction to cancer chemotherapeutics. Chem. Rev. 109, 2859–2861. doi:10.1021/cr900208x
Shi, X., Zhang, H., Jin, W., Liu, W., Yin, H., Li, Y., et al. (2019). Metronomic photodynamic therapy with 5-aminolevulinic acid induces apoptosis and autophagy in human SW837 colorectal cancer cells. J. Photochem. Photobiol. B Biol. 198, 111586. doi:10.1016/j.jphotobiol.2019.111586
Simsek, C., Esin, E., and Yalcin, S. (2019). Metronomic chemotherapy: a systematic review of the literature and clinical experience. J. Oncol. 2019, 5483791. doi:10.1155/2019/5483791
Skipper, H. E., Schabel, F. M., Mellett, L. B., Montgomery, J. A., Wilkoff, L. J., Lloyd, H. H., et al. (1970). Implications of biochemical, cytokinetic, pharmacologic, and toxicologic relationships in the design of optimal therapeutic schedules. Cancer Chemother. Rep. 54 (6), 431–450.
Sleire, L., Elisabeth Førde-Tislevoll, H., Anne Netland, I., Leiss, L., Sandvei Skeie, B., and Enger, P. Ø. (2017). Drug repurposing in cancer. Pharmacol. Res. 124, 74–91. doi:10.1016/j.phrs.2017.07.013
Soriano, J. L., Batista, N., Santiesteban, E., Lima, M., González, J., García, R., et al. (2011). Metronomic cyclophosphamide and methotrexate chemotherapy combined with 1E10 anti-idiotype vaccine in metastatic breast cancer. Int. J. Breast Cancer 2011, 710292. doi:10.4061/2011/710292
Soultati, A., Mountzios, G., Avgerinou, C., Papaxoinis, G., Pectasides, D., Athanassios Dimopoulos, M., et al. (2012). Endothelial vascular toxicity from chemotherapeutic agents: preclinical evidence and clinical implications. Cancer Treat. Rev. 38, 473–483. doi:10.1016/j.ctrv.2011.09.002
Štěrba, J., Pavelka, Z., and Šlampa, P. (2002). Concomitant radiotherapy and metronomic Temozolomide in pediatric high-risk brain tumors. Neoplasma 49 (2), 117–120.
Tabassum, S., Rosli, N. B., and Mazalan, M. S. A. B. (2019). Mathematical modeling of cancer growth process: a review. J. Phys. Conf. Ser. 1366, 012018. doi:10.1088/1742-6596/1366/1/012018
Takimoto, C. H. (2009). Maximum tolerated dose: clinical endpoint for a bygone era? Target. Oncol. 4, 143–147. doi:10.1007/s11523-009-0108-y
Tanaka, A., and Sakaguchi, S. (2019). Targeting Treg cells in cancer immunotherapy. Eur. J. Immunol. 49, 1140–1146. doi:10.1002/eji.201847659
Toale, K. M., Johnson, T. N., Ma, M. Q., and Vu, N. H. (2021). “Chemotherapy toxicities,” in Oncologic emergency medicine: principles and practice (Berlin, Germany: Springer). doi:10.1007/978-3-030-67123-5_48
Tongu, M., Harashima, N., Monma, H., Inao, T., Yamada, T., Kawauchi, H., et al. (2013). Metronomic chemotherapy with low-dose cyclophosphamide plus gemcitabine can induce anti-tumor T cell immunity in vivo. Cancer Immunol. Immunother. 62 (2), 383–391. doi:10.1007/s00262-012-1343-0
Toulmonde, M., Cousin, S., Kind, M., Guegan, J. P., Bessede, A., Le Loarer, F., et al. (2022). Randomized phase 2 trial of intravenous oncolytic virus JX-594 combined with low-dose cyclophosphamide in patients with advanced soft-tissue sarcoma. J. Hematol. Oncol. 15, 149. doi:10.1186/s13045-022-01370-9
Vasan, N., Baselga, J., and Hyman, D. M. (2019). A view on drug resistance in cancer. Nature 575, 299–309. doi:10.1038/s41586-019-1730-1
Visser, K. E.De, Eichten, A., and Coussens, L. M. (2006). Paradoxical roles of the immune system during cancer development. Nat. Rev. Cancer 6, 24–37. doi:10.1038/nrc1782
Vives, M., Ginestà, M. M., Gracova, K., Graupera, M., Casanovas, O., Capellà, G., et al. (2013). Metronomic chemotherapy following the maximum tolerated dose is an effective anti-tumour therapy affecting angiogenesis, tumour dissemination and cancer stem cells. Int. J. Cancer 133 (10), 2464–2472. doi:10.1002/ijc.28259
Voelcker, G. (2020). The mechanism of action of cyclophosphamide and its consequences for the development of a new generation of oxazaphosphorine cytostatics. Sci. Pharm. 88, 42. doi:10.3390/scipharm88040042
Wang, J., Lou, P., Lesniewski, R., and Henkin, J. (2003). Paclitaxel at ultra low concentrations inhibits angiogenesis without affecting cellular microtubule assembly. Anti-Cancer Drugs 14 (1), 13–19. doi:10.1097/00001813-200301000-00003
Wang, Z., Liu, J., Ma, F., Wang, J., Luo, Y., Fan, Y., et al. (2021). Safety and efficacy study of oral metronomic vinorelbine combined with trastuzumab (MNH) in HER2-positive metastatic breast cancer: a phase II trial. Breast Cancer Res. Treat. 188 (2), 441–447. doi:10.1007/s10549-021-06216-5
Wculek, S. K., Cueto, F. J., Mujal, A. M., Melero, I., Krummel, M. F., and Sancho, D. (2020). Dendritic cells in cancer immunology and immunotherapy. Nat. Rev. Immunol. 20, 7–24. doi:10.1038/s41577-019-0210-z
Webb, E. R., Moreno-Vincente, J., Easton, A., Lanati, S., Taylor, M., James, S., et al. (2022). Cyclophosphamide depletes tumor infiltrating T regulatory cells and combined with anti-PD-1 therapy improves survival in murine neuroblastoma. IScience 25 (9), 104995. doi:10.1016/j.isci.2022.104995
Weir, G. M., Hrytsenko, O., Stanford, M. M., Berinstein, N. L., Karkada, M., Liwski, R. S., et al. (2014). Metronomic cyclophosphamide enhances HPV16E7 peptide vaccine induced antigen-specific and cytotoxic T-cell mediated antitumor immune response. OncoImmunology 3 (8), e953407. doi:10.4161/21624011.2014.953407
West, J., and Newton, P. K. (2017). Chemotherapeutic dose scheduling based on tumor growth rates provides a case for low-dose metronomic high-entropy therapies. Cancer Res. 77 (23), 6717–6728. doi:10.1158/0008-5472.CAN-17-1120
WHO (2020) WHO report on cancer: setting priorities, investing wisely and providing care for all. Switzerland: Ganeva.
Wilson, B. C., and Patterson, M. S. (2008). The physics, biophysics and technology of photodynamic therapy. Phys. Med. Biol. 53, R61–R109. doi:10.1088/0031-9155/53/9/R01
Wolf, D., Sopper, S., Pircher, A., Gastl, G., and Wolf, A. M. (2015). Treg(s) in cancer: friends or foe? J. Cell. Physiology 230, 2598–2605. doi:10.1002/jcp.25016
Wu, T., and Dai, Y. (2017). Tumor microenvironment and therapeutic response. Cancer Lett. 387, 61–68. doi:10.1016/j.canlet.2016.01.043
Wysocki, P. J., Łobacz, M., Potocki, P., Kwinta, Ł., Michałowska-Kaczmarczyk, A., Słowik, A., et al. (2023). Metronomic chemotherapy based on topotecan or topotecan and cyclophosphamide combination (CyTo) in advanced, pretreated ovarian cancer. Cancers 15 (4), 1067. doi:10.3390/cancers15041067
Wysocki, P. J., Lubas, M. T., and Wysocka, M. L. (2022). Metronomic chemotherapy in prostate cancer. J. Clin. Med. 11, 2853. doi:10.3390/jcm11102853
Xu, B., Sun, T., Wang, S., and Lin, Y. (2021). Metronomic therapy in advanced breast cancer and NSCLC: vinorelbine as a paradigm of recent progress. Expert Rev. Anticancer Ther. 21, 71–79. doi:10.1080/14737140.2021.1835478
Yalcin, G. D., Danisik, N., Baygin, R. C., and Acar, A. (2020). Systems biology and experimental model systems of cancer. J. Personalized Med. 10 (4), 180. doi:10.3390/jpm10040180
Yalcin, G. D., Yilmaz, K. C., Dilber, T., and Acar, A. (2023). Investigation of evolutionary dynamics for drug resistance in 3D spheroid model system using cellular barcoding technology. PLoS ONE 18 (9 September), e0291942. doi:10.1371/journal.pone.0291942
Yang, L., Shi, P., Zhao, G., Xu, J., Wen, P., Zhang, J., et al. (2020). Targeting cancer stem cell pathways for cancer therapy. Signal Transduct. Target. Ther. 5, 8. doi:10.1038/s41392-020-0110-5
Yoshimoto, M., Takao, S., Hirata, M., Okamoto, Y., Yamashita, S., Kawaguchi, Y., et al. (2012). Metronomic oral combination chemotherapy with capecitabine and cyclophosphamide: a phase II study in patients with HER2-negative metastatic breast cancer. Cancer Chemother. Pharmacol. 70 (2), 331–338. doi:10.1007/s00280-012-1826-x
Zhang, A., Miao, K., Sun, H., and Deng, C. X. (2022). Tumor heterogeneity reshapes the tumor microenvironment to influence drug resistance. Int. J. Biol. Sci. 18, 3019–3033. doi:10.7150/ijbs.72534
Zsiros, E., Lynam, S., Attwood, K. M., Wang, C., Chilakapati, S., Gomez, E. C., et al. (2021). Efficacy and safety of pembrolizumab in combination with bevacizumab and oral metronomic cyclophosphamide in the treatment of recurrent ovarian cancer: a phase 2 nonrandomized clinical trial. JAMA Oncol. 7, 78–85. doi:10.1001/jamaoncol.2020.5945
Keywords: cancer, metronomic chemotherapy, therapeutic resistance, combination therapy, photodynamic therapy
Citation: Basar OY, Mohammed S, Qoronfleh MW and Acar A (2024) Optimizing cancer therapy: a review of the multifaceted effects of metronomic chemotherapy. Front. Cell Dev. Biol. 12:1369597. doi: 10.3389/fcell.2024.1369597
Received: 12 January 2024; Accepted: 29 April 2024;
Published: 15 May 2024.
Edited by:
Eswar Shankar, The Ohio State University, United StatesReviewed by:
Balaji Chandrasekaran, Texas A and M University, United StatesRadha Vaddavalli, The Ohio State University, United States
Copyright © 2024 Basar, Mohammed, Qoronfleh and Acar. This is an open-access article distributed under the terms of the Creative Commons Attribution License (CC BY). The use, distribution or reproduction in other forums is permitted, provided the original author(s) and the copyright owner(s) are credited and that the original publication in this journal is cited, in accordance with accepted academic practice. No use, distribution or reproduction is permitted which does not comply with these terms.
*Correspondence: Ahmet Acar, acara@metu.edu.tr; M. Walid Qoronfleh, walidq@yahoo.com; Sawsan Mohammed, sawsan@qu.edu.qa