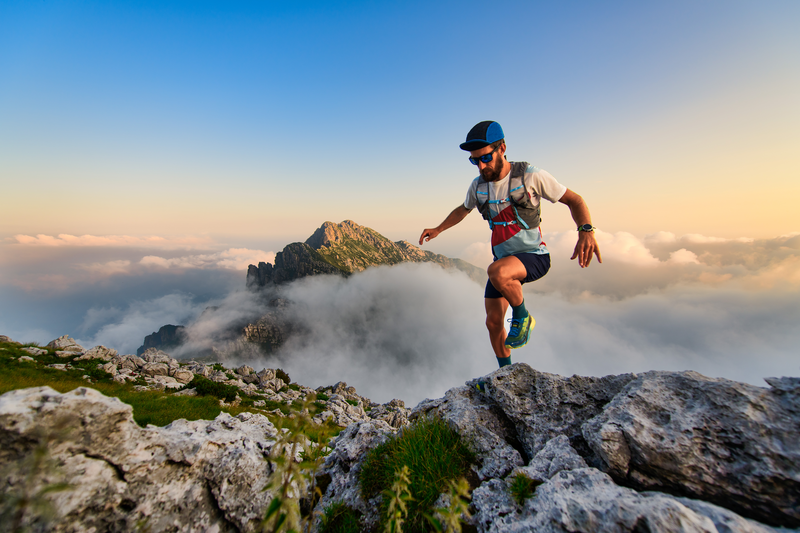
94% of researchers rate our articles as excellent or good
Learn more about the work of our research integrity team to safeguard the quality of each article we publish.
Find out more
REVIEW article
Front. Cell Dev. Biol. , 02 April 2024
Sec. Cell Growth and Division
Volume 12 - 2024 | https://doi.org/10.3389/fcell.2024.1343962
This article is part of the Research Topic Phosphorylation-Dependent Peptidyl-Prolyl Cis/Trans Isomerase PIN1 - Volume II View all 9 articles
Tauopathies are neurodegenerative diseases characterized by deposits of abnormal Tau protein in the brain. Conventional tauopathies are often defined by a limited number of Tau epitopes, notably neurofibrillary tangles, but emerging evidence suggests structural heterogeneity among tauopathies. The prolyl isomerase Pin1 isomerizes cis P-tau to inhibit the development of oligomers, tangles and neurodegeneration in multiple neurodegenerative diseases such as Alzheimer’s disease, traumatic brain injury, vascular contribution to cognitive impairment and dementia (VCID) and preeclampsia (PE). Thus, cis P-tau has emerged as an early etiological driver, blood marker and therapeutic target for multiple neurodegenerative diseases, with clinical trials ongoing. The discovery of cis P-tau and other tau pathologies in VCID and PE calls attention for simplistic classification of tauopathy in neurodegenerative diseases. These recent advances have revealed the exciting novel role of the Pin1-cis P-tau axis in the development and treatment of vascular contribution to cognitive impairment and dementia and preeclampsia.
VCID is featured with neurovascular insults, pathology reminiscent of Alzheimer’s disease (AD) and cognitive decline (Gorelick et al., 2011; Zlokovic, 2011; Iadecola, 2013; OBrien and Thomas, 2015; Snyder et al., 2015). The most common etiology of dementia includes mixed vascular and AD pathologies (Schneider et al., 2004; Schneider et al., 2007; Schneider et al., 2009; James et al., 2012). A critical pathological component of VCID is diffuse white matter lesions, which correlate with cognitive impairment (Pantoni and Garcia, 1997; Medana and Esiri, 2003; Esiri and Nagy, 2007). White matter primarily consists of axonal bundles ensheathed with myelin generated by mature oligodendrocytes, and plays an important role in passing signals between different areas of gray matter. Furthermore, cerebral endothelial cells may support neuronal and oligodendroglial function by secreting trophic factors (Guo et al., 2008; Arai and Lo, 2009). Although there are many potential clinical triggers, brain injury caused by lacunar and larger cerebral infarcts (Snowdon et al., 1997), other hypoperfusion and ischemia (Iadecola, 2004; Garcia-Alloza et al., 2011; Luitse et al., 2012; Rincon et al., 2014) are major vascular factors that contribute to the development of white matter dysfunction, chronic neurodegeneration and dementia (Zlokovic, 2011; Iadecola, 2013; OBrien and Thomas, 2015; Snyder et al., 2015). White matter tracts are especially vulnerable to vascular insults because of their location at the border between different vascular territories (De Reuck, 1971) and their vasculature is highly susceptible to risk factors (Brown and Thore, 2011). However, as compared with neurodegeneration in gray matter, white matter pathophysiology remains relatively understudied, and molecular and cellular mechanisms that connect vascular insults to white matter lesions and delayed neurodegeneration are incompletely understood.
Intercellular interaction among different cell types is critical to maintain white matter function (Itoh et al., 2015). However, under the pathological conditions, this trophic coupling among endothelial cells, oligodendrocytes and neurons may be disrupted, resulting in white matter dysfunction. Iadecola (Iadecola, 2013) has proposed that oxidative stress-induced endothelial dysfunction is likely an early event leading to white matter lesions. Endothelial dysfunction leads to reductions in resting cerebral blood flow (CBF) in the marginally perfused white matter and alterations in the permeability of the blood-brain barrier (BBB). Hypoperfusion and BBB disruption in turn lead to additional oxidative stress by inducing tissue hypoxia and extravasating plasma proteins. Tissue edema resulting from increased BBB permeability may exacerbate these alterations by compressing blood vessels and further reducing CBF, forming a vicious positive feedback cycle. Tissue hypoxia and oxidative stress subsequently activate inflammatory pathways, leading to production of cytokines and adhesion molecules in vascular cells, reactive astrocytes and activated microglia. Hypoxia, oxidative stress and inflammation damage the neurovascular units made of neuronal axons, oligodendrocytes and endothelial cells, and eventually lead to progressive degeneration in axons, myelin and endothelial cells in VCID (Zlokovic, 2011; Iadecola, 2013; OBrien and Thomas, 2015; Snyder et al., 2015).
Neurofibrillary tangles composed of hyperphosphorylated tau is a neuropathological hallmark of AD but not VCID (Mattson, 2004; Goedert and Spillantini, 2006; Roberson and Mucke, 2006; Ballatore et al., 2007; Spires-Jones et al., 2009). Pure VCID human brains were pathologically defined with absence of obvious tau tangle pathology (Gorelick et al., 2011; Zlokovic, 2011; Iadecola, 2013; OBrien and Thomas, 2015; Snyder et al., 2015). Although VCID is not conventionally viewed as a tauopathy, absence of some tau epitopes did not rule out the possibility that other pathogenic tau species could be involved. It has been recently reported that several tau epitopes are increased in VCID patients and mouse models with cerebral hypoperfusion (Castillo-Carranza et al., 2017; Faraco et al., 2019; Qiu et al., 2021; Karakaya et al., 2023; Wang et al., 2023). Importantly, targeting such tau epitopes prevents neuroinflammation, cognitive impairment and other behavioral dysfunctions in mice with cerebral hypoperfusion induced by surgery (Qiu et al., 2021) or high-salt diet (Faraco et al., 2019), revealing important contribution of tau pathologies to the development and progression of VCID.
PE is a hypertensive disease that occurs frequently in pregnant women and is often associated with cognitive impairment and dementia (Karrar and Hong, 2023). PE has an incidence rate of up to 8% in pregnancy complications, resulting in over 550,000 maternal and fetal deaths worldwide (Karrar and Hong, 2023). The pathogenesis of PE can be divided into two stages: placental abnormalities and maternal syndrome. Placental dysfunction is mainly influenced by genetic, maternal, and immune factors, leading to the generation of anti angiogenic factors such as soluble fms like tyrosine kinase 1 (sFlt-1), soluble endoglin (sEng), and other inflammatory mediators, thereby promoting the progression of PE. PE usually manifests as newly developed hypertension and proteinuria in late pregnancy, which can progress to multiple organ dysfunction, including chronic diseases such as liver, kidney, and brain (Phipps et al., 2019; Rana et al., 2019). Among the most serious prognostic diseases are the mild cognitive impairment and dementia in mothers and their offsprings (Tuovinen et al., 2012; Basit et al., 2018; Lu et al., 2019; Andolf et al., 2020). Tau is one of the candidate biomarkers for diagnosing and predicting PE complications (Bergman et al., 2022; Friis et al., 2022). It has been shown that phosphorylated tau is superior to total tau in predicting the cognitive ability of PE patients (Wang et al., 2023). However, these evidences are largely observational and do not reveal the molecular mechanisms and therapeutic potential of phosphorylated Tau. Recently, we revealed that cis conformation of phosphorylated Thr231 of Tau (cis P-tau) is a central circulating etiological driver in PE, and the cis P-tau specific monoclonal antibody (cis mAb) has potential for early PE diagnosis and treatment (Jash et al., 2023), suggesting that PE may be another non-conventional tauopathy. Below we review studies relevant to the discovery of cis P-tau, one of the major deleterious tau species that arises early and disrupts the function of the neurovascular unit to connect initial vascular insults to the development of white matter lesions, delayed neurodegeneration and neurologic defects in tauopathies such as VCID and PE.
Cis P-tau is featured with its Pro232 in a rare cis conformation, which likely affects the Thr231 phosphorylation and Tau function (Figure 1). Proline-directed Ser/Thr phosphorylation is a key signaling mechanism in cells (Blume-Jensen and Hunter, 2001; Pawson and Scott, 2005). Many pSer/Thr-Pro motifs exist in cis or trans conformations, and their conversion and function are regulated by the unique prolyl isomerase Pin1 (Lu et al., 1996; Ranganathan et al., 1997; Yaffe et al., 1997). Pin1 is tightly regulated (Wulf et al., 2001; Lu et al., 2002; Ryo et al., 2002; You et al., 2002; Hamdane et al., 2006; Lee et al., 2011) and its deregulation can contribute to various diseases, notably including AD, TBI, VCID, and PE (Nakamura et al., 2012; Kondo et al., 2015; Albayram et al., 2017; Albayram et al., 2019; Qiu et al., 2021; Jash et al., 2023). Pin1 acts on the pThr231-Pro motif (P-tau) in tau (Lu et al., 1999) to facilitate P-tau dephosphorylation by Protein phosphatase 2A (Lu et al., 1999), a trans-proline directed phosphatase (Zhou et al., 2000; Liou et al., 2003). Pin1 facilitated P-tau dephosphorylation leads to increased tau degradation; accordingly, various hyperphosphorylated tau, including P-tau, are resistant to degradation. Furthermore, Pin1 restores the physiological function of tau to promote microtubule assembly (Lu et al., 1999), a function of tau that is inhibited by hyperphosphorylation. In contrast, Pin1 has no effect on tau T231A mutant (Lu et al., 1999; Zhou et al., 2000; Liou et al., 2003; Lim et al., 2008), although Pin1 can bind and isomerize other motifs in vitro (Smet et al., 2004; Kimura et al., 2013; Eichner et al., 2016), suggesting that the primary Pin1 target site in Tau in vivo is pT231-Pro.
Genetic and pathological data have supported a critical role of Pin1 in neuroprotection. Pin1 −/− mice display age-dependent tau-related pathologies and neurodegeneration (Liou et al., 2003; Pastorino et al., 2006; Cancino et al., 2013), while moderate Pin1 overexpression prevents wild type tau pathology in mice (Lim et al., 2008). In humans, Pin1 can be inhibited by various mechanisms including downregulation/sequestration (Lu et al., 1999; Thorpe et al., 2001; Thorpe et al., 2004; Hamdane et al., 2006) Ser71 phosphorylation (Lee et al., 2011; Kim et al., 2014) and Cys113 oxidation (Sultana et al., 2006; Chen et al., 2015). For example, oxidative stress from the AD brain could oxidize Pin1 Cys113 and inactivate Pin1, leading to the loss of regulation on Tau and APP and increased neurofibrillary pathology (Lee et al., 2011; Kim et al., 2014). Furthermore, cerebral ischemic insults activate DAPK1, which phosphorylates Pin1 Ser71 and leads to Pin1 inhibition, cis P-tau induction, pathology, neuroinflammation and memory impairment (Sultana et al., 2006; Chen et al., 2015; Qiu et al., 2021). It is worth noting that the human PIN1 gene is located at 19p13.2, a new late onset AD locus distinct from ApoE4 (Wijsman et al., 2004). PIN1 SNPs that reduce Pin1 expression (Lu et al., 2009) are associated with increased risk for AD in an Italian cohort (Segat et al., 2007), although not in others (Nowotny et al., 2007; Ma et al., 2012) whereas a different SNP that prevents Pin1 suppression by the brain-selected AP4 is associated with delayed onset of AD (Ma et al., 2012). In addition, pT231-tau (P-tau), the Pin1 substrate, is induced at early stages of AD prior to tangle formation (Jicha et al., 1997; Augustinack et al., 2002; Luna-Munoz et al., 2005; Luna-Munoz et al., 2007). CSF P-tau is an early biomarker that correlates with cognitive decline (Buerger et al., 2002; Buerger et al., 2005), neocortical tangle accumulation (Buerger et al., 2006), hippocampal atrophy rate (Hampel et al., 2005), and predicts progression from mild cognitive impairment (MCI) to AD (Ewers et al., 2007; Blennow et al., 2010; Hampel et al., 2010). Thus, pT231-tau (P-tau) is likely an early disease driver in AD.
Pin1 facilitates the interconversion of cis and trans pT231-Pro motif in Tau, but it was unclear from the genetic and functional data whether cis P-tau or trans P-tau was pathogenic. To distinguish the functions of cis P-tau and trans P-tau, we generated cis and trans P-tau isomer-specific antibodies using an innovative approach leveraging the distinct structural properties of prolines (Nakamura et al., 2012; Kondo et al., 2015). We started by substituting proline232 with homoproline (Pip), which has a six-membered ring and leads to an increased proportion of cis peptide bond conformation (∼74%) (Table 1) (Nakamura et al., 2012). Using this peptide, we successfully produced antibodies that recognized P-tau with either trans or cis comformation. For further conformational specificity, we synthesized cis-locked or trans-locked peptides for selection and counter-selection of cis or trans P-tau specific antibodies. Peptides were locked in cis using pThr231-Dmp (5,5-dimethylproline) (∼96% in cis conformation) and locked in trans by pThr231-Ala (0% in cis), as validated by NMR (Table 1) (Nakamura et al., 2012). After selection and purification, the specificity of these cis- and trans-specific antibodies was confirmed by ELISA, demonstrating negligible cross-reactivity and high affinity for their respective cis and trans pT231-tau peptides. These antibodies did not react with an unphosphorylated Thr231-Pro tau peptide but showed strong reactivity to a wild-type pThr231-Pro tau peptide, highlighting their high phosphorylation and conformational specificity (Nakamura et al., 2012; Kondo et al., 2015).
Using these antibodies, we dissected the functions of cis P-tau and trans P-tau (Nakamura et al., 2012; Kondo et al., 2015). Cis, but not trans, P-tau loses its classical microtubule assembly function, confering resistance to dephosphorylation and degradation and also promoting its tendency to aggregation (Figure 2) (Nakamura et al., 2012). Cis to trans conversion does not occur spontaneously, but is significantly accelerated by Pin1 (Figure 2) (Nakamura et al., 2012). Thus, cis, but not trans, P-tau is likely the pathogenic form of P-tau, and antibodies targeting cis P-tau could have potential applications in both the diagnosis and treatment of various neurodegenerative diseases associated with P-tau (Nakamura et al., 2012). Below we summarize recent work highlighting the role of cis P-tau as an early, pathogenic conformer in AD, TBI, VCID, and PE, as well as the therapeutic potential of the monoclonal antibody targeting cis P-tau (cis mAb).
Figure 2. Mechanism of cis-trans isomerization of pT231-Tau (P-tau) in the regulation of microtubule stability and function. (A) Under physiological conditions, P-tau is predominantly in the physiological trans P-tau form, which binds to and stabilizes microtubules. (B) Under stress conditions, cis P-tau is induced, while the prolyl isomerase Pin1 binds and catalyzes the isomerization of the pathogenic cis P-tau to the physiological trans P-tau. In several neurodegenerative diseases such as TBI, VaD, PE, and AD, loss or inhibition of Pin1 leads to excessive accumulation of cis P-tau, which dissociates from microtubules, oligomerizes and eventually aggregates into neurofibrillary tangles, leading to destabilized microtubules.
Cis P-tau has emerged as an early pathogenic tau conformation in multiple neurodegenerative diseases since its initial discovery. First, we hypothesized that cis P-tau is pathogenic in AD, because pT231-Tau (P-tau) is induced at early stages of AD and Pin1 −/− mice conferred age-dependent tauopathy and neurodegeneration (Liou et al., 2003; Pastorino et al., 2006; Cancino et al., 2013). Indeed, in hTau mice, where endogenous mouse Tau is replaced with human Tau (Duff et al., 2000; Andorfer et al., 2003), cis P-tau is induced at 3-months of age, prior to formation of early tangles (Qiu et al., 2021). In patients, cis P-tau is induced early in MCI neurons and is specifically induced at the dystrophic neurites of degenerating neurons (Nakamura et al., 2012), correlating well with cognitive deficits as AD progresses (Davies et al., 1987; DeKosky and Scheff, 1990; Scheff et al., 1990; Terry et al., 1991; Masliah et al., 1992; Coleman and Yao, 2003; Thies and Mandelkow, 2007). Moreover, plasma cis P-tau is induced extremely early in AD patients (Ashton et al., 2021; Milà-Alomà et al., 2022; Shiravandi et al., 2022), being the first blood biomarker able to distinguish between Braak stage I/II in incipient AD from health controls (Ashton et al., 2021) and to reach the abnormal level with the lowest Aβ burden in pre-clinical AD patients (Milà-Alomà et al., 2022).
TBI is another classical tauopathy when the timing of disease onset can be precisely modeled in mice (Kondo et al., 2015). cis P-tau is induced as early as 12 h after single severe closed head TBI in the mouse model (Kondo et al., 2015). After closed head TBI in mice and stress in vitro, neurons acutely produce cis P-tau notably at axons, which disrupts axonal microtubules and axonal transport, spreads to other neurons, and leads to apoptosis. In patients, cis P-tau can be induced as early as 8 h after motor vehicle injury (Albayram et al., 2017). Furthermore, we find robust cis P-tau in human chronic traumatic encephalopathy (CTE) brains (Kondo et al., 2015). Tangles are a neuropathological signature of CTE following sport and military TBI (Omalu et al., 2005; Blennow et al., 2012; Goldstein et al., 2012; DeKosky et al., 2013; McKee et al., 2013; Smith et al., 2013), but tau tangle pathology is not readily detectable acutely after closed head TBI in humans and mouse models (Smith et al., 2003; Yoshiyama et al., 2005; Flierl et al., 2009; Tran et al., 2011; Goldstein et al., 2012; Kane et al., 2012; Mannix et al., 2013; Ojo et al., 2013), although tau oligomers can be detected after open head severe TBI (Hawkins et al., 2013). Finally, cis P-tau in the CSF (Albayram et al., 2017) or P-tau in the plasma (Rubenstein et al., 2017) also correlate with TBI injury severity and clinical outcome in acute and chronic phases, with a 10- to 15-fold increase in plasma P-tau within 24 h after severe TBI (Rubenstein et al., 2017).
Discovery of cis P-tau at an early stage of AD and TBI, long before the presence of neurofibrillary tangles, suggested that absence of classical tangles does not exclude a neurodegenerative disease as a tauopathy. We next set out to ask if cis P-tau is implicated in VCID, because VCID pathology often co-occurs with AD in the late stage. We hypothesize that cis P-tau may be similarly induced at an early stage of VCID, prior to tangle pathology. Notably, cis P-tau is significantly induced in various cohorts of pure VCID patients prior to co-emergence of AD pathologies (Qiu et al., 2021). Furthermore, we set out to ask if cis P-tau is induced early in mice after bilateral common carotid artery stenosis (BCAS), an experimental approach widely used to induce chronic cerebral hypoperfusion and model VCID by partial occlusion of both common carotid arteries in mice (Shibata et al., 2004). The reduced blood flow to the brain leads to the vascular pathology and subsequently cognitive impairment observed in VCID, allowing for the study of its mechanisms and potential therapeutic interventions (Shibata et al., 2004; Shibata et al., 2007). We observed robust cis P-tau induction at 2 weeks after the BCAS sugery, an early time point prior to significant demyelination and cognitive decline (Qiu et al., 2021).
Finally, implication of cis P-tau in VCID, a non-conventional tauopathy, suggested that there might be a broader spectrum of neurodegenerative diseases driven by cis P-tau and other toxic Tau species. Indeed, cis P-tau and several other phosphorylated tau epitopes are significantly induced in PE placental tissue and serum as compared to that of normal pregnant women (Jash et al., 2023). Both in vivo and in vitro studies have shown that cis P-tau, sFlt-1, and sEng are induced in the primary human trophoblast in response to hypoxia and serum, as well as trophoblast invasion and interruption of intravascular activity in PE patients (Jash et al., 2023), suggesting that blood cis P-tau can be also an early biomarker for early and late-onset PE (Jash et al., 2023). Therefore, cis P-tau is an early tau conformation prior to oligomers and tangles in AD, TBI, VCID, and PE.
To explore the therapeutic potential of targeting cis and trans P-tau, we generated, screened and purified cis and trans P-tau monoclonal antibodies (cis mAb or trans mAb) with high affinity (Cis mAb: 0.27 nM, trans mAb: 42.1 nM) (Kondo et al., 2015), and evaluated their therapeutic potential in a range of TBI, AD-like, and VCID mouse models (Kondo et al., 2015; Albayram et al., 2017; Albayram et al., 2019; Qiu et al., 2021). We treated single-severe closed head TBI mice (54 g weight drop from 60 inches (Albayram et al., 2017)) with 200 µg cis mAb at 4 h after TBI, followed by weekly treatment until 2-month post-TBI and bi-weekly treatment (every other week) until 4-month TBI (Albayram et al., 2017). In parallel, we treated repetitive moderate closed head TBI mice (54 g weight drop from 34 inches per impact, and 7 impact over 9 days) with one dose after each impact, followed by weekly treatment until 2-month post-TBI and bi-weekly treatment (every other week) until 4-month TBI. In both TBI models, cis mAb blocks early cistauosis, prevents subsequent tau pathology, and restores TBI-related structural and functional outcomes (Kondo et al., 2015; Albayram et al., 2017; Albayram et al., 2019). Specifically, cis mAb reversed axonal and mitochondrial ultrastructural pathologies, cortical and hippocampal long-term potentiation impairment, risk-taking behavior, voiding dysfunction and working memory defects in TBI mouse models (Kondo et al., 2015; Albayram et al., 2017; Albayram et al., 2019). The cis mAb efficacy is consistent with the earlier evidence that Tau knockout prevents memory defects and axonopathy after repetitive TBI in mice (Cheng et al., 2014).
Second, in AD-like tauopathy hTau mice, which develops age-related tau pathologies and neurodegeneration, cis mAb (300 µg per mouse, intraperitoneally (i.p.) for the first 4 months, followed by every other week treatment) prevents formation of cis P-tau, tangles, neurodegeneration, demyelination and impairment in working memory and spatial learning/memory (Qiu et al., 2021). Furthermore, extended cis mAb treatment of 13-month-old hTau mice (300 µg per mouse, intraperitoneally (i.p.) weekly), which already developed tau pathologies and memory deficits, is sufficient to specifically eliminate cis P-tau, prevent further neuronal loss and rescue working memory impairment (Qiu et al., 2021). It is worth noting that cis mAb treatment does not reduce neurofibrillary tangles (Qiu et al., 2021), consistent with the hypothesis that soluble Tau, but not the insoluble Tau fibrils, is neurotoxic (Goedert et al., 2017; Hyman, 2023).
Third, cis mAb confers notable neuroprotection to the VCID mouse model (Qiu et al., 2021). Cis mAb treatment (300 µg per mouse, intraperitoneally (i.p.), every 3 days for four times, and then 150 µg per mouse every week afterwards) specifically eliminates cis P-tau (without perturbing total tau) in the BCAS mice, reducing neuroinflammation, demyelination and rescuing impaired hippocampal long-term potentiation (Qiu et al., 2021). Furthermore, cis mAb rescued the working memory impairment and chronically induced risk-taking behavior in BCAS mice in multiple behavioral assays (Qiu et al., 2021). In addition, BCAS induced diverse cortical cell type-specific transcriptomic changes, many of which resembled changes seen in AD patients, featuring alteration of pathways in myelination, axon/synapses function, microtubule related function and GTP signaling (Qiu et al., 2021). Strikingly, 85%–90% of the global alterations are recovered by cis mAb, and the extent of recovery in different cell types is correlated with the cell type-specific tau expression. This is consistent with the hypothesis that cis P-tau confers toxicity through prion-like propagation and requires endogenous Tau, thus the extent of cis P-tau toxicity correlates with endogenous Tau expression levels. Therefore, cis mAb is highly effective in eliminating cis P-tau, recovering pathology, behavior and cell type-specific transcriptome of BCAS mice.
Finally, depletion of cis P-tau in PE serum significantly inhibits the ability of PE serum to induce all PE-like pathological and clinical features in humanized tau mice during pregnancy (Jash et al., 2023). Serum from PE patients, but not healthy pregnant individuals, when injected (i.p.) into the pregnant hTau mice, leads to PE symptoms, including accumulation of protein aggregates in the junctional zone, elevated blood pressure, proteinuria, fetal growth restriction, and glomerular endotheliosis (Aoki et al., 2018). Notably, when the serum from PE patients were depleted for cis P-Tau by cis mAb, the PE related pathology and symptoms were largely rescued, and normal pregnancy was restored (Jash et al., 2023).Taken together, cis P-tau is an early driver of neurodegeneration and a potential therapeutic target in TBI, AD, VCID and PE. It is worth noting that cis mAb is the only Alzheimer’s clinical drug candidate that has potential for brain injury, VCID, and preeclampsia, which are among the best-known risk factors for dementia.
Cortical injection of brain lysates or tau aggregates leads to progressive neurodegeneration in young WT mice (Clavaguera et al., 2009; Clavaguera et al., 2013; Clavaguera et al., 2014; Sanders et al., 2014; Kaufman et al., 2016). We next ask if purified cis P-tau is sufficient to induce neurodegeneration in neuronal culture and in wild-type animals. We purified cis P-tau from TBI mouse brains using cis mAb, and found that purified cis P-tau induced much higher neurotoxicity than recombinant Tau in neuronal culture. Furthermore, the cis P-tau induced neurotoxicity is blocked by cis mAb treatment or pan-caspase inhibitor, suggesting that cis P-tau induced neurotoxicity is caspase dependent and may be linked to neuronal apoptosis. Thus, purified cis P-tau, but not recombinant tau, induced neuronal apoptosis and could be blocked by cis mAb.
To test if purified cis P-tau is sufficient to induce progressive neurodegeneration and brain dysfunction in wild-type animals, we stereotaxically injected purified cis P-tau (or recombinant tau as a control) bilaterally into the cortex of 3-month-old WT mice, and evaluated the pathological and behavioral outcomes at 1 or 10 months after the injection. We observed progressive prion-like tau spreading, neuroinflammation and behavioral dysfunction. At 1 month post-injection, cis P-tau, but not recombinant tau control, induces apparent risk-taking behaviors reminiscent of TBI mice, as assayed by elevated plus maze and bright-light open field assays. At 10 months post-injection, cis P-tau induces a range of somatosensory motor deficits and working memory impairment in addition to the persistent apparent risk-taking behavior. Consistent with the behavioral alteration, cis P-tau appears to propagate across cortical regions and induce subsequent tau pathologies (e.g., tangles) and ultra-structural pathologies. Cis mAb treatment eliminated cis P-tau and prevented various pathological and behavioral changes. Finally, single-nucleus RNA-seq revealed that injection of purified cis P-tau induces a subset of cell type-specific transcriptomic changes in BCAS mice, recapitulating altered genes in myelination, axon/synapse function and microtubule related function. Strikingly, the cis P-tau induced transcriptomic changes in the excitatory neurons have significant overlap with recently reported excitatory neuronal changes in patients with early, but not late AD pathologies. The vast majority of these changes can be rescued by cis mAb, and injection of purified cis P-tau into Tau knock-out mice does not induce pathology and behavioral alternations, consistent with requirement of endogenous Tau for the prion-like propagation of cis P-tau.
Tauopathies are a series of neurodegenerative diseases characterized with neurofibrillary tangles or well-known Tau epitopes. Recent advances in cryo-EM structures of Tau fibrils enabled structurally based classification of Tauopathies, revealing distinct folds for different tauopathies (Fitzpatrick et al., 2017; Falcon et al., 2018a; Falcon et al., 2018b; Falcon et al., 2019; Zhang et al., 2020; Shi et al., 2021). However, it has been increasingly recognized that the toxic Tau species may be soluble but not aggregated (Goedert et al., 2017; Hyman, 2023). It is thus critical to evaluate which Tau epitopes may underlie the disease progression and to revisit how Tauopathies are defined. VCID and PE are two such examples. VCID was not conventionally considered as a tauopathy, largely due to the absence of neurofibrillary tangles in pure VCID patients with cognitive impairment. While most tauopathies are age-dependent and related to brain dysfunction, PE represents the first example where cis P-tau drives disease development outside of the brain and in a younger population represented by pregnant women. We and Faraco et al. have shown that absence of tangles does not preclude the pathogenic roles of other Tau PTMs such as pSer202, pThr205, P-tau (pThr231) and cis P-tau (Faraco et al., 2019; Qiu et al., 2021). Tau can be heavily modified by post-translational modifications, with >80 potential PTM sites, far beyond the known epitopes (Goedert, 2005; Goedert et al., 2017). The potential pathogenic role of cis P-tau in AD, TBI and non-conventional tauopathies (VCID and PE) reviewed here may suggest a broader spectrum of neurodegenerative diseases that could be driven by pathogenic Tau.
CQ: Conceptualization, Writing–original draft, Writing–review and editing. ZL: Writing–original draft, Writing–review and editing, Conceptualization. DL: Writing–review and editing. BD: Writing–review and editing. JS: Writing–review and editing. NK: Writing–original draft, Writing–review and editing. KL: Conceptualization, Funding acquisition, Supervision, Writing–original draft, Writing–review and editing. XZ: Conceptualization, Funding acquisition, Supervision, Writing–original draft, Writing–review and editing.
The author(s) declare that financial support was received for the research, authorship, and/or publication of this article. This work was supported the Canada Foundation for Innovation (CFI) grants (#43257 and #43822) and Canadian Institutes of Health Research (#502128; #506475 and #506500) to KL and XZ.
We thank the lab members of the corresponding authors for critical reading of the manuscript and for providing insightful suggestions.
XZ and KL are inventors of several patents on PIN1 and cis and trans stereo-specific antibody technology, as well as the scientific founders and former scientific advisors of and own equity in Pinteon. Their interests were reviewed and are managed by Western University in accordance with its conflict-of-interest policy.
The remaining authors declare that the research was conducted in the absence of any commercial or financial relationships that could be construed as a potential conflict of interest.
All claims expressed in this article are solely those of the authors and do not necessarily represent those of their affiliated organizations, or those of the publisher, the editors and the reviewers. Any product that may be evaluated in this article, or claim that may be made by its manufacturer, is not guaranteed or endorsed by the publisher.
Albayram, O., Kondo, A., Mannix, R., Smith, C., Li, C., Tsai, C. Y., et al. (2017). Cis P-tau is induced in clinical and preclinical brain injury and contributes to post-injury sequelae. Nat. Commun. 8, 1000. doi:10.1038/s41467-017-01068-4
Albayram, O., Maciver, B., Mathai, J., Verstegen, A., Baxley, S., Qiu, C., et al. (2019). Traumatic Brain Injury-related voiding dysfunction in mice is caused by damage to rostral pathways, altering inputs to the reflex pathways. Sci. Rep. 9, 8646–8712. doi:10.1038/s41598-019-45234-8
Andolf, E., Bladh, M., Möller, L., and Sydsjö, G. (2020). Prior placental bed disorders and later dementia: a retrospective Swedish register-based cohort study. BJOG Int. J. Obstetrics Gynaecol. 127, 1090–1099. doi:10.1111/1471-0528.16201
Andorfer, C., Kress, Y., Espinoza, M., De Silva, R., Tucker, K. L., Barde, Y. A., et al. (2003). Hyperphosphorylation and aggregation of tau in mice expressing normal human tau isoforms. J. Neurochem. 86, 582–590. doi:10.1046/j.1471-4159.2003.01879.x
Aoki, A., Nakashima, A., Kusabiraki, T., Ono, Y., Yoshino, O., Muto, M., et al. (2018). Trophoblast-specific conditional Atg7 knockout mice develop gestational hypertension. Am. J. pathology 188, 2474–2486. doi:10.1016/j.ajpath.2018.07.021
Arai, K., and Lo, E. H. (2009). An oligovascular niche: cerebral endothelial cells promote the survival and proliferation of oligodendrocyte precursor cells. J. Neurosci. 29, 4351–4355. doi:10.1523/JNEUROSCI.0035-09.2009
Ashton, N. J., Pascoal, T. A., Karikari, T. K., Benedet, A. L., Lantero-Rodriguez, J., Brinkmalm, G., et al. (2021). Plasma p-tau231: a new biomarker for incipient Alzheimer’s disease pathology. Acta neuropathol. 141, 709–724. doi:10.1007/s00401-021-02275-6
Augustinack, J. C., Schneider, A., Mandelkow, E. M., and Hyman, B. T. (2002). Specific tau phosphorylation sites correlate with severity of neuronal cytopathology in Alzheimer's disease. Acta Neuropathol. 103, 26–35. doi:10.1007/s004010100423
Ballatore, C., Lee, V. M., and Trojanowski, J. Q. (2007). Tau-mediated neurodegeneration in Alzheimer's disease and related disorders. Nat. Rev. Neurosci. 8, 663–672. doi:10.1038/nrn2194
Basit, S., Wohlfahrt, J., and Boyd, H. A. (2018). Pre-eclampsia and risk of dementia later in life: nationwide cohort study. Bmj 363, k4109. doi:10.1136/bmj.k4109
Bergman, L., Hastie, R., Bokström-Rees, E., Zetterberg, H., Blennow, K., Schell, S., et al. (2022). Cerebral biomarkers in neurologic complications of preeclampsia. Am. J. Obstetrics Gynecol. 227, 298. e1–e298.e10. doi:10.1016/j.ajog.2022.02.036
Blennow, K., Hampel, H., Weiner, M., and Zetterberg, H. (2010). Cerebrospinal fluid and plasma biomarkers in Alzheimer disease. Nat. Rev. Neurol. 6, 131–144. doi:10.1038/nrneurol.2010.4
Blennow, K., Hardy, J., and Zetterberg, H. (2012). The neuropathology and neurobiology of traumatic brain injury. Neuron 76, 886–899. doi:10.1016/j.neuron.2012.11.021
Blume-Jensen, P., and Hunter, T. (2001). Oncogenic kinase signalling. Nature 411, 355–365. doi:10.1038/35077225
Brown, W. R., and Thore, C. R. (2011). Review: cerebral microvascular pathology in ageing and neurodegeneration. Neuropathology Appl. Neurobiol. 37, 56–74. doi:10.1111/j.1365-2990.2010.01139.x
Buerger, K., Ewers, M., Andreasen, N., Zinkowski, R., Ishiguro, K., Vanmechelen, E., et al. (2005). Phosphorylated tau predicts rate of cognitive decline in MCI subjects: a comparative CSF study. Neurology 65, 1502–1503. doi:10.1212/01.wnl.0000183284.92920.f2
Buerger, K., Ewers, M., Pirttila, T., Zinkowski, R., Alafuzoff, I., Teipel, S. J., et al. (2006). CSF phosphorylated tau protein correlates with neocortical neurofibrillary pathology in Alzheimer's disease. Brain a J. neurology 129, 3035–3041. doi:10.1093/brain/awl269
Buerger, K., Teipel, S. J., Zinkowski, R., Blennow, K., Arai, H., Engel, R., et al. (2002). CSF tau protein phosphorylated at threonine 231 correlates with cognitive decline in MCI subjects. Neurology 59, 627–629. doi:10.1212/wnl.59.4.627
Cancino, G. I., Miller, F. D., and Kaplan, D. R. (2013). p73 haploinsufficiency causes tau hyperphosphorylation and tau kinase dysregulation in mouse models of aging and Alzheimer's disease. Neurobiol. Aging 34, 387–399. doi:10.1016/j.neurobiolaging.2012.04.010
Castillo-Carranza, D. L., Nilson, A. N., Van Skike, C. E., Jahrling, J. B., Patel, K., Garach, P., et al. (2017). Cerebral microvascular accumulation of tau oligomers in Alzheimer’s disease and related tauopathies. Aging Dis. 8, 257–266. doi:10.14336/AD.2017.0112
Chen, C. H., Li, W., Sultana, R., You, M. H., Kondo, A., Shahpasand, K., et al. (2015). Pin1 cysteine-113 oxidation inhibits its catalytic activity and cellular function in Alzheimer's disease. Neurobiol. Dis. 76, 13–23. doi:10.1016/j.nbd.2014.12.027
Cheng, J. S., Craft, R., Yu, G. Q., Ho, K., Wang, X., Mohan, G., et al. (2014). Tau reduction diminishes spatial learning and memory deficits after mild repetitive traumatic brain injury in mice. PLoS One 9, e115765. doi:10.1371/journal.pone.0115765
Clavaguera, F., Akatsu, H., Fraser, G., Crowther, R. A., Frank, S., Hench, J., et al. (2013). Brain homogenates from human tauopathies induce tau inclusions in mouse brain. Proc. Natl. Acad. Sci. U. S. A. 110, 9535–9540. doi:10.1073/pnas.1301175110
Clavaguera, F., Bolmont, T., Crowther, R. A., Abramowski, D., Frank, S., Probst, A., et al. (2009). Transmission and spreading of tauopathy in transgenic mouse brain. Nat. Cell Biol. 11, 909–913. doi:10.1038/ncb1901
Clavaguera, F., Hench, J., Lavenir, I., Schweighauser, G., Frank, S., Goedert, M., et al. (2014). Peripheral administration of tau aggregates triggers intracerebral tauopathy in transgenic mice. Acta Neuropathol. 127, 299–301. doi:10.1007/s00401-013-1231-5
Coleman, P. D., and Yao, P. J. (2003). Synaptic slaughter in Alzheimer's disease. Neurobiol. Aging 24, 1023–1027. doi:10.1016/j.neurobiolaging.2003.09.001
Davies, C. A., Mann, D. M., Sumpter, P. Q., and Yates, P. O. (1987). A quantitative morphometric analysis of the neuronal and synaptic content of the frontal and temporal cortex in patients with Alzheimer's disease. J. Neurol. Sci. 78, 151–164. doi:10.1016/0022-510x(87)90057-8
DeKosky, S. T., Blennow, K., Ikonomovic, M. D., and Gandy, S. (2013). Acute and chronic traumatic encephalopathies: pathogenesis and biomarkers. Nat. Rev. Neurol. 9, 192–200. doi:10.1038/nrneurol.2013.36
DeKosky, S. T., and Scheff, S. W. (1990). Synapse loss in frontal cortex biopsies in Alzheimer's disease: correlation with cognitive severity. Ann. neurology 27, 457–464. doi:10.1002/ana.410270502
De Reuck, J. (1971). The human periventricular arterial blood supply and the anatomy of cerebral infarctions. Eur. Neurol. 5, 321–334. doi:10.1159/000114088
Duff, K., Knight, H., Refolo, L., Sanders, S., Yu, X., Picciano, M., et al. (2000). Characterization of pathology in transgenic mice over-expressing human genomic and cDNA tau transgenes. Neurobiol. Dis. 7, 87–98. doi:10.1006/nbdi.1999.0279
Eichner, T., Kutter, S., Labeikovsky, W., Buosi, V., and Kern, D. (2016). Molecular mechanism of pin1-tau recognition and catalysis. J. Mol. Biol. 428, 1760–1775. doi:10.1016/j.jmb.2016.03.009
Esiri, M. M., and Nagy, Z. (2007). Cerebrovascular disease and the expression. Vascular dementia: cerebrovascular mechanisms and clinical management, 275.
Ewers, M., Buerger, K., Teipel, S. J., Scheltens, P., Schroder, J., Zinkowski, R. P., et al. (2007). Multicenter assessment of CSF-phosphorylated tau for the prediction of conversion of MCI. Neurology 69, 2205–2212. doi:10.1212/01.wnl.0000286944.22262.ff
Falcon, B., Zhang, W., Murzin, A. G., Murshudov, G., Garringer, H. J., Vidal, R., et al. (2018b). Structures of filaments from Pick’s disease reveal a novel tau protein fold. Nature 561, 137–140. doi:10.1038/s41586-018-0454-y
Falcon, B., Zhang, W., Schweighauser, M., Murzin, A. G., Vidal, R., Garringer, H. J., et al. (2018a). Tau filaments from multiple cases of sporadic and inherited Alzheimer’s disease adopt a common fold. Acta neuropathol. 136, 699–708. doi:10.1007/s00401-018-1914-z
Falcon, B., Zivanov, J., Zhang, W., Murzin, A. G., Garringer, H. J., Vidal, R., et al. (2019). Novel tau filament fold in chronic traumatic encephalopathy encloses hydrophobic molecules. Nature 568, 420–423. doi:10.1038/s41586-019-1026-5
Faraco, G., Hochrainer, K., Segarra, S. G., Schaeffer, S., Santisteban, M. M., Menon, A., et al. (2019). Dietary salt promotes cognitive impairment through tau phosphorylation. Nature 574, 686–690. doi:10.1038/s41586-019-1688-z
Fitzpatrick, A. W., Falcon, B., He, S., Murzin, A. G., Murshudov, G., Garringer, H. J., et al. (2017). Cryo-EM structures of tau filaments from Alzheimer’s disease. Nature 547, 185–190. doi:10.1038/nature23002
Flierl, M. A., Stahel, P. F., Beauchamp, K. M., Morgan, S. J., Smith, W. R., and Shohami, E. (2009). Mouse closed head injury model induced by a weight-drop device. Nat. Protoc. 4, 1328–1337. doi:10.1038/nprot.2009.148
Friis, T., Wikström, A.-K., Acurio, J., León, J., Zetterberg, H., Blennow, K., et al. (2022). Cerebral biomarkers and blood-brain barrier integrity in preeclampsia. Cells 11, 789. doi:10.3390/cells11050789
Garcia-Alloza, M., Gregory, J., Kuchibhotla, K. V., Fine, S., Wei, Y., Ayata, C., et al. (2011). Cerebrovascular lesions induce transient β-amyloid deposition. Brain a J. neurology 134, 3697–3707. doi:10.1093/brain/awr300
Goedert, M. (2005). Tau gene mutations and their effects. official J. Mov. Disord. Soc. 20, S45–S52. doi:10.1002/mds.20539
Goedert, M., Eisenberg, D. S., and Crowther, R. A. (2017). Propagation of tau aggregates and neurodegeneration. Annu. Rev. Neurosci. 40, 189–210. doi:10.1146/annurev-neuro-072116-031153
Goedert, M., and Spillantini, M. G. (2006). A century of Alzheimer's disease. Science 314, 777–781. doi:10.1126/science.1132814
Goldstein, L. E., Fisher, A. M., Tagge, C. A., Zhang, X. L., Velisek, L., Sullivan, J. A., et al. (2012). Chronic traumatic encephalopathy in blast-exposed military veterans and a blast neurotrauma mouse model. Sci. Transl. Med. 4, 134ra60. doi:10.1126/scitranslmed.3003716
Gorelick, P. B., Scuteri, A., Black, S. E., Decarli, C., Greenberg, S. M., Iadecola, C., et al. (2011). Intervention, S. Council on Cardiovascular, and Anesthesia, Vascular contributions to cognitive impairment and dementia: a statement for healthcare professionals from the american heart association/american stroke association. Stroke 42, 2672–2713. doi:10.1161/str.0b013e3182299496
Guo, S., Kim, W. J., Lok, J., Lee, S.-R., Besancon, E., Luo, B.-H., et al. (2008). Neuroprotection via matrix-trophic coupling between cerebral endothelial cells and neurons. Proc. Natl. Acad. Sci. 105, 7582–7587. doi:10.1073/pnas.0801105105
Hamdane, M., Dourlen, P., Bretteville, A., Sambo, A. V., Ferreira, S., Ando, K., et al. (2006). Pin1 allows for differential Tau dephosphorylation in neuronal cells. Mol. Cell Neurosci. 32, 155–160. doi:10.1016/j.mcn.2006.03.006
Hampel, H., Blennow, K., Shaw, L. M., Hoessler, Y. C., Zetterberg, H., and Trojanowski, J. Q. (2010). Total and phosphorylated tau protein as biological markers of Alzheimer's disease. Exp. Gerontol. 45, 30–40. doi:10.1016/j.exger.2009.10.010
Hampel, H., Burger, K., Pruessner, J. C., Zinkowski, R., DeBernardis, J., Kerkman, D., et al. (2005). Correlation of cerebrospinal fluid levels of tau protein phosphorylated at threonine 231 with rates of hippocampal atrophy in Alzheimer disease. Arch. Neurol. 62, 770–773. doi:10.1001/archneur.62.5.770
Hawkins, B. E., Krishnamurthy, S., Castillo-Carranza, D. L., Sengupta, U., Prough, D. S., Jackson, G. R., et al. (2013). Rapid accumulation of endogenous tau oligomers in a rat model of traumatic brain injury: possible link between traumatic brain injury and sporadic tauopathies. J. Biol. Chem. 288, 17042–17050. doi:10.1074/jbc.M113.472746
Hyman, B. (2023). All the Tau we cannot see. Annu. Rev. Med. 74, 503–514. doi:10.1146/annurev-med-042921-023749
Iadecola, C. (2004). Neurovascular regulation in the normal brain and in Alzheimer's disease. Nat. Rev. Neurosci. 5, 347–360. doi:10.1038/nrn1387
Iadecola, C. (2013). The pathobiology of vascular dementia. Neuron 80, 844–866. doi:10.1016/j.neuron.2013.10.008
Itoh, K., Maki, T., Lok, J., and Arai, K. (2015). Mechanisms of cell–cell interaction in oligodendrogenesis and remyelination after stroke. Brain Res. 1623, 135–149. doi:10.1016/j.brainres.2015.04.039
James, B. D., Bennett, D. A., Boyle, P. A., Leurgans, S., and Schneider, J. A. (2012). Dementia from Alzheimer disease and mixed pathologies in the oldest old. JAMA 307, 1798–1800. doi:10.1001/jama.2012.3556
Jash, S., Banerjee, S., Cheng, S., Wang, B., Qiu, C., Kondo, A., et al. (2023). Cis P-tau is a central circulating and placental etiologic driver and therapeutic target of preeclampsia. Nat. Commun. 14, 5414. doi:10.1038/s41467-023-41144-6
Jicha, G. A., Lane, E., Vincent, I., Otvos, L., Hoffmann, R., and Davies, P. (1997). A conformation- and phosphorylation-dependent antibody recognizing the paired helical filaments of Alzheimer's disease. J. Neurochem. 69, 2087–2095. doi:10.1046/j.1471-4159.1997.69052087.x
Kane, M. J., Angoa-Perez, M., Briggs, D. I., Viano, D. C., Kreipke, C. W., and Kuhn, D. M. (2012). A mouse model of human repetitive mild traumatic brain injury. J. Neurosci. methods 203, 41–49. doi:10.1016/j.jneumeth.2011.09.003
Karakaya, E., Berber, B., Kurtz, J., Edwards, J., Albayram, O., and Ergul, A. (2023). Tau hyperphosphorylation in brain microvasculature: relevance to vascular cognitive impairment and dementia (VCID). Physiology 38, 5731480. doi:10.1152/physiol.2023.38.s1.5731480
Karrar, S. A., and Hong, P. L. (2023). Preeclampsia, StatPearls. United States: StatPearls Publishing.
Kaufman, S. K., Sanders, D. W., Thomas, T. L., Ruchinskas, A. J., Vaquer-Alicea, J., Sharma, A. M., et al. (2016). Tau prion strains dictate patterns of cell pathology, progression rate, and regional vulnerability in vivo. Neuron 92, 796–812. doi:10.1016/j.neuron.2016.09.055
Kim, B. M., You, M. H., Chen, C. H., Lee, S., Hong, Y., Hong, Y., et al. (2014). Death-associated protein kinase 1 has a critical role in aberrant tau protein regulation and function. Cell Death Dis. 5, e1237. doi:10.1038/cddis.2014.216
Kimura, T., Tsutsumi, K., Taoka, M., Saito, T., Masuda-Suzukake, M., Ishiguro, K., et al. (2013). Isomerase Pin1 stimulates dephosphorylation of tau protein at cyclin-dependent kinase (Cdk5)-dependent Alzheimer phosphorylation sites. J. Biol. Chem. 288, 7968–7977. doi:10.1074/jbc.M112.433326
Kondo, A., Shahpasand, K., Mannix, R., Qiu, J., Moncaster, J., Chen, C. H., et al. (2015). Antibody against early driver of neurodegeneration cis P-tau blocks brain injury and tauopathy. Nature 523, 431–436. doi:10.1038/nature14658
Lee, T. H., Chen, C. H., Suizu, F., Huang, P., Schiene-Fischer, C., Daum, S., et al. (2011). Death-associated protein kinase 1 phosphorylates Pin1 and inhibits its prolyl isomerase activity and cellular function. Mol. Cell 42, 147–159. doi:10.1016/j.molcel.2011.03.005
Lim, J., Balastik, M., Lee, T. H., Liou, Y. C., Sun, A., Finn, G., et al. (2008). Pin1 has opposite effects on wild-type and P301L tau stability and tauopathy. J. Clin. Invest. 118, 1877–1889. doi:10.1172/JCI34308
Liou, Y. C., Sun, A., Ryo, A., Zhou, X. Z., Yu, Z. X., Huang, H. K., et al. (2003). Role of the prolyl isomerase Pin1 in protecting against age-dependent neurodegeneration. Nature 424, 556–561. doi:10.1038/nature01832
Lu, H. Q., Brown, M., Magee, L., Kenny, L., Phipps, E., Thadhani, R., et al. (2019). Lasting effects of intrauterine exposure to preeclampsia on offspring and the underlying mechanism. Am. J. perinatology Rep. 9, e275–e291. doi:10.1055/s-0039-1695004
Lu, J., Hu, Z., Wei, S., Wang, L. E., Liu, Z., El-Naggar, A. K., et al. (2009). A novel functional variant (-842G>C) in the PIN1 promoter contributes to decreased risk of squamous cell carcinoma of the head and neck by diminishing the promoter activity. Carcinogenesis 30, 1717–1721. doi:10.1093/carcin/bgp171
Lu, K. P., Hanes, S. D., and Hunter, T. (1996). A human peptidyl-prolyl isomerase essential for regulation of mitosis. Nature 380, 544–547. doi:10.1038/380544a0
Lu, P. J., Wulf, G., Zhou, X. Z., Davies, P., and Lu, K. P. (1999). The prolyl isomerase Pin1 restores the function of Alzheimer-associated phosphorylated tau protein. Nature 399, 784–788. doi:10.1038/21650
Lu, P. J., Zhou, X. Z., Liou, Y. C., Noel, J. P., and Lu, K. P. (2002). Critical role of WW domain phosphorylation in regulating phosphoserine binding activity and Pin1 function. J. Biol. Chem. 277, 2381–2384. doi:10.1074/jbc.C100228200
Luitse, M. J., Biessels, G. J., Rutten, G. E., and Kappelle, L. J. (2012). Diabetes, hyperglycaemia, and acute ischaemic stroke. Lancet Neurology 11, 261–271. doi:10.1016/S1474-4422(12)70005-4
Luna-Munoz, J., Chavez-Macias, L., Garcia-Sierra, F., and Mena, R. (2007). Earliest stages of tau conformational changes are related to the appearance of a sequence of specific phospho-dependent tau epitopes in Alzheimer's disease. J. Alzheimers Dis. 12, 365–375. doi:10.3233/jad-2007-12410
Luna-Munoz, J., Garcia-Sierra, F., Falcon, V., Menendez, I., Chavez-Macias, L., and Mena, R. (2005). Regional conformational change involving phosphorylation of tau protein at the Thr231, precedes the structural change detected by Alz-50 antibody in Alzheimer's disease. J. Alzheimers Dis. 8, 29–41. doi:10.3233/jad-2005-8104
Ma, S. L., Tang, N. L., Tam, C. W., Lui, V. W., Lam, L. C., Chiu, H. F., et al. (2012). A PIN1 polymorphism that prevents its suppression by AP4 associates with delayed onset of Alzheimer's disease. Neurobiol. Aging 33, 804–813. doi:10.1016/j.neurobiolaging.2010.05.018
Mannix, R., Meehan, W. P., Mandeville, J., Grant, P. E., Gray, T., Berglass, J., et al. (2013). Clinical correlates in an experimental model of repetitive mild brain injury. Ann. neurology 74, 65–75. doi:10.1002/ana.23858
Masliah, E., Ellisman, M., Carragher, B., Mallory, M., Young, S., Hansen, L., et al. (1992). Three-dimensional analysis of the relationship between synaptic pathology and neuropil threads in Alzheimer disease. J. Neuropathol. Exp. Neurol. 51, 404–414. doi:10.1097/00005072-199207000-00003
Mattson, M. P. (2004). Pathways towards and away from Alzheimer's disease. Nature 430, 631–639. doi:10.1038/nature02621
McKee, A. C., Stern, R. A., Nowinski, C. J., Stein, T. D., Alvarez, V. E., Daneshvar, D. H., et al. (2013). The spectrum of disease in chronic traumatic encephalopathy. Brain a J. neurology 136, 43–64. doi:10.1093/brain/aws307
Medana, I., and Esiri, M. (2003). Axonal damage: a key predictor of outcome in human CNS diseases. Brain a J. neurology 126, 515–530. doi:10.1093/brain/awg061
Milà-Alomà, M., Ashton, N. J., Shekari, M., Salvadó, G., Ortiz-Romero, P., Montoliu-Gaya, L., et al. (2022). Plasma p-tau231 and p-tau217 as state markers of amyloid-β pathology in preclinical Alzheimer’s disease. Nat. Med. 28, 1797–1801. doi:10.1038/s41591-022-01925-w
Nakamura, K., Greenwood, A., Binder, L., Bigio, E. H., Denial, S., Nicholson, L., et al. (2012). Proline isomer-specific antibodies reveal the early pathogenic tau conformation in Alzheimer's disease. Cell 149, 232–244. doi:10.1016/j.cell.2012.02.016
Nowotny, P., Bertelsen, S., Hinrichs, A. L., Kauwe, J. S., Mayo, K., Jacquart, S., et al. (2007). Association studies between common variants in prolyl isomerase Pin1 and the risk for late-onset Alzheimer's disease. Neurosci. Lett. 419, 15–17. doi:10.1016/j.neulet.2007.03.071
OBrien, J. T., and Thomas, A. (2015). Vascular dementia. Lancet 386, 1698–1706. doi:10.1016/S0140-6736(15)00463-8
Ojo, J. O., Mouzon, B., Greenberg, M. B., Bachmeier, C., Mullan, M., and Crawford, F. (2013). Repetitive mild traumatic brain injury augments tau pathology and glial activation in aged hTau mice. J. Neuropathol. Exp. Neurol. 72, 137–151. doi:10.1097/NEN.0b013e3182814cdf
Omalu, B. I., DeKosky, S. T., Minster, R. L., Kamboh, M. I., Hamilton, R. L., and Wecht, C. H. (2005). Chronic traumatic encephalopathy in a national football league player. Neurosurgery 57, 128–134. doi:10.1227/01.neu.0000163407.92769.ed
Pantoni, L., and Garcia, J. H. (1997). Pathogenesis of leukoaraiosis: a review. Stroke 28, 652–659. doi:10.1161/01.str.28.3.652
Pastorino, L., Sun, A., Lu, P. J., Zhou, X. Z., Balastik, M., Finn, G., et al. (2006). The prolyl isomerase Pin1 regulates amyloid precursor protein processing and amyloid-beta production. Nature 440, 528–534. doi:10.1038/nature04543
Pawson, T., and Scott, J. D. (2005). Protein phosphorylation in signaling--50 years and counting. Trends Biochem. Sci. 30, 286–290. doi:10.1016/j.tibs.2005.04.013
Phipps, E. A., Thadhani, R., Benzing, T., and Karumanchi, S. A. (2019). Pre-eclampsia: pathogenesis, novel diagnostics and therapies. Nat. Rev. Nephrol. 15, 275–289. doi:10.1038/s41581-019-0119-6
Qiu, C., Albayram, O., Kondo, A., Wang, B., Kim, N., Arai, K., et al. (2021). Cis P-tau underlies vascular contribution to cognitive impairment and dementia and can be effectively targeted by immunotherapy in mice. Sci. Transl. Med. 13, eaaz7615. doi:10.1126/scitranslmed.aaz7615
Rana, S., Lemoine, E., Granger, J. P., and Karumanchi, S. A. (2019). Preeclampsia: pathophysiology, challenges, and perspectives. Circulation Res. 124, 1094–1112. doi:10.1161/CIRCRESAHA.118.313276
Ranganathan, R., Lu, K. P., Hunter, T., and Noel, J. P. (1997). Structural and functional analysis of the mitotic rotamase Pin1 suggests substrate recognition is phosphorylation dependent. Cell 89, 875–886. doi:10.1016/s0092-8674(00)80273-1
Rincon, F., Kang, J., Maltenfort, M., Vibbert, M., Urtecho, J., Athar, M. K., et al. (2014). Association between hyperoxia and mortality after stroke: a multicenter cohort study. Crit. care Med. 42, 387–396. doi:10.1097/CCM.0b013e3182a27732
Roberson, E. D., and Mucke, L. (2006). 100 years and counting: prospects for defeating Alzheimer's disease. Science 314, 781–784. doi:10.1126/science.1132813
Rubenstein, R., Chang, B., Yue, J. K., Chiu, A., Winkler, E. A., Puccio, A. M., et al. (2017). Comparing plasma phospho tau, total tau, and phospho tau–total tau ratio as acute and chronic traumatic brain injury biomarkers. JAMA neurol. 74, 1063–1072. doi:10.1001/jamaneurol.2017.0655
Ryo, A., Liou, Y. C., Wulf, G., Nakamura, N., Lee, S. W., and Lu, K. P. (2002). PIN1 is an E2F target gene essential for Neu/Ras-induced transformation of mammary epithelial cells. Mol. Cell Biol. 22, 5281–5295. doi:10.1128/mcb.22.15.5281-5295.2002
Sanders, D. W., Kaufman, S. K., DeVos, S. L., Sharma, A. M., Mirbaha, H., Li, A., et al. (2014). Distinct tau prion strains propagate in cells and mice and define different tauopathies. Neuron 82, 1271–1288. doi:10.1016/j.neuron.2014.04.047
Scheff, S. W., DeKosky, S. T., and Price, D. A. (1990). Quantitative assessment of cortical synaptic density in Alzheimer's disease. Neurobiol. Aging 11, 29–37. doi:10.1016/0197-4580(90)90059-9
Schneider, J. A., Arvanitakis, Z., Bang, W., and Bennett, D. A. (2007). Mixed brain pathologies account for most dementia cases in community-dwelling older persons. Neurology 69, 2197–2204. doi:10.1212/01.wnl.0000271090.28148.24
Schneider, J. A., Arvanitakis, Z., Leurgans, S. E., and Bennett, D. A. (2009). The neuropathology of probable Alzheimer disease and mild cognitive impairment. Ann. neurology 66, 200–208. doi:10.1002/ana.21706
Schneider, J. A., Wilson, R. S., Bienias, J. L., Evans, D. A., and Bennett, D. A. (2004). Cerebral infarctions and the likelihood of dementia from Alzheimer disease pathology. Neurology 62, 1148–1155. doi:10.1212/01.wnl.0000118211.78503.f5
Segat, L., Pontillo, A., Annoni, G., Trabattoni, D., Vergani, C., Clerici, M., et al. (2007). PIN1 promoter polymorphisms are associated with Alzheimer's disease. Neurobiol. Aging 28, 69–74. doi:10.1016/j.neurobiolaging.2005.11.009
Shi, Y., Zhang, W., Yang, Y., Murzin, A. G., Falcon, B., Kotecha, A., et al. (2021). Structure-based classification of tauopathies. Nature 598, 359–363. doi:10.1038/s41586-021-03911-7
Shibata, M., Ohtani, R., Ihara, M., and Tomimoto, H. (2004). White matter lesions and glial activation in a novel mouse model of chronic cerebral hypoperfusion. Stroke 35, 2598–2603. doi:10.1161/01.STR.0000143725.19053.60
Shibata, M., Yamasaki, N., Miyakawa, T., Kalaria, R. N., Fujita, Y., Ohtani, R., et al. (2007). Selective impairment of working memory in a mouse model of chronic cerebral hypoperfusion. Stroke 38, 2826–2832. doi:10.1161/STROKEAHA.107.490151
Shiravandi, A., Yari, F., Tofigh, N., Kazemi Ashtiani, M., Shahpasand, K., Ghanian, M.-H., et al. (2022). Earlier detection of Alzheimer’s disease based on a novel biomarker cis P-tau by a label-free electrochemical immunosensor. Biosensors 12, 879. doi:10.3390/bios12100879
Smet, C., Sambo, A. V., Wieruszeski, J. M., Leroy, A., Landrieu, I., Buee, L., et al. (2004). The peptidyl prolyl cis/trans-isomerase Pin1 recognizes the phospho-Thr212-Pro213 site on Tau. Biochemistry 43, 2032–2040. doi:10.1021/bi035479x
Smith, C., Graham, D. I., Murray, L. S., and Nicoll, J. A. (2003). Tau immunohistochemistry in acute brain injury. Neuropathology Appl. Neurobiol. 29, 496–502. doi:10.1046/j.1365-2990.2003.00488.x
Smith, D. H., Johnson, V. E., and Stewart, W. (2013). Chronic neuropathologies of single and repetitive TBI: substrates of dementia? Nat. Rev. Neurol. 9, 211–221. doi:10.1038/nrneurol.2013.29
Snowdon, D. A., Greiner, L. H., Mortimer, J. A., Riley, K. P., Greiner, P. A., and Markesbery, W. R. (1997). Brain infarction and the clinical expression of Alzheimer disease: the Nun Study. Jama 277, 813–817. doi:10.1001/jama.277.10.813
Snyder, H. M., Corriveau, R. A., Craft, S., Faber, J. E., Greenberg, S. M., Knopman, D., et al. (2015). Vascular contributions to cognitive impairment and dementia including Alzheimer's disease. Alzheimers Dement. 11, 710–717. doi:10.1016/j.jalz.2014.10.008
Spires-Jones, T. L., Stoothoff, W. H., de Calignon, A., Jones, P. B., and Hyman, B. T. (2009). Tau pathophysiology in neurodegeneration: a tangled issue. Trends Neurosci. 32, 150–159. doi:10.1016/j.tins.2008.11.007
Sultana, R., Boyd-Kimball, D., Poon, H. F., Cai, J., Pierce, W. M., Klein, J. B., et al. (2006). Oxidative modification and down-regulation of Pin1 in Alzheimer's disease hippocampus: a redox proteomics analysis. Neurobiol. Aging 27, 918–925. doi:10.1016/j.neurobiolaging.2005.05.005
Terry, R. D., Masliah, E., Salmon, D. P., Butters, N., DeTeresa, R., Hill, R., et al. (1991). Physical basis of cognitive alterations in Alzheimer's disease: synapse loss is the major correlate of cognitive impairment. Ann. neurology 30, 572–580. doi:10.1002/ana.410300410
Thies, E., and Mandelkow, E. M. (2007). Missorting of tau in neurons causes degeneration of synapses that can be rescued by the kinase MARK2/Par-1. J. Neurosci. 27, 2896–2907. doi:10.1523/JNEUROSCI.4674-06.2007
Thorpe, J. R., Morley, S. J., and Rulten, S. L. (2001). Utilizing the peptidyl-prolyl cis-trans isomerase pin1 as a probe of its phosphorylated target proteins. Examples of binding to nuclear proteins in a human kidney cell line and to tau in Alzheimer's diseased brain. J. Histochem Cytochem 49, 97–108. doi:10.1177/002215540104900110
Thorpe, J. R., Mosaheb, S., Hashemzadeh-Bonehi, L., Cairns, N. J., Kay, J. E., Morley, S. J., et al. (2004). Shortfalls in the peptidyl-prolyl cis-trans isomerase protein Pin1 in neurons are associated with frontotemporal dementias. Neurobiol. Dis. 17, 237–249. doi:10.1016/j.nbd.2004.07.008
Tran, H. T., LaFerla, F. M., Holtzman, D. M., and Brody, D. L. (2011). Controlled cortical impact traumatic brain injury in 3xTg-AD mice causes acute intra-axonal amyloid-β accumulation and independently accelerates the development of tau abnormalities. J. Neurosci. 31, 9513–9525. doi:10.1523/JNEUROSCI.0858-11.2011
Tuovinen, S., Räikkönen, K., Kajantie, E., Henriksson, M., Leskinen, J. T., Pesonen, A.-K., et al. (2012). Hypertensive disorders in pregnancy and cognitive decline in the offspring up to old age. Neurology 79, 1578–1582. doi:10.1212/WNL.0b013e31826e2606
Wang, R., Lu, K. P., and Zhou, X. Z. (2023). Function and regulation of cis P-tau in the pathogenesis and treatment of conventional and nonconventional tauopathies. J. Neurochem. 166, 904–914. doi:10.1111/jnc.15909
Wijsman, E. M., Daw, E. W., Yu, C. E., Payami, H., Steinbart, E. J., Nochlin, D., et al. (2004). Evidence for a novel late-onset Alzheimer disease locus on chromosome 19p13.2. Am. J. Hum. Genet. 75, 398–409. doi:10.1086/423393
Wulf, G. M., Ryo, A., Wulf, G. G., Lee, S. W., Niu, T., Lu, K. P., et al. (2001). Pin1 is overexpressed in breast cancer and cooperates with Ras signaling in increasing the transcriptional activity of c-Jun towards cyclin D1. EMBO J. 20, 3459–3472. doi:10.1093/emboj/20.13.3459
Yaffe, M. B., Schutkowski, M., Shen, M., Zhou, X. Z., Stukenberg, P. T., Rahfeld, J.-U., et al. (1997). Sequence-specific and phosphorylation-dependent proline isomerization: a potential mitotic regulatory mechanism. Science 278, 1957–1960. doi:10.1126/science.278.5345.1957
Yoshiyama, Y., Uryu, K., Higuchi, M., Longhi, L., Hoover, R., Fujimoto, S., et al. (2005). Enhanced neurofibrillary tangle formation, cerebral atrophy, and cognitive deficits induced by repetitive mild brain injury in a transgenic tauopathy mouse model. J. neurotrauma 22, 1134–1141. doi:10.1089/neu.2005.22.1134
You, H., Zheng, H., Murray, S. A., Yu, Q., Uchida, T., Fan, D., et al. (2002). IGF-1 induces Pin1 expression in promoting cell cycle S-phase entry. J. Cell Biochem. 84, 211–216. doi:10.1002/jcb.10037
Zhang, W., Tarutani, A., Newell, K. L., Murzin, A. G., Matsubara, T., Falcon, B., et al. (2020). Novel tau filament fold in corticobasal degeneration. Nature 580, 283–287. doi:10.1038/s41586-020-2043-0
Zhou, X. Z., Kops, O., Werner, A., Lu, P. J., Shen, M., Stoller, G., et al. (2000). Pin1-dependent prolyl isomerization regulates dephosphorylation of Cdc25C and tau proteins. Mol. Cell 6, 873–883. doi:10.1016/s1097-2765(05)00083-3
Keywords: Pin1, tau, tauopathies, cistauosis, vascular dementia, Alzheimer’s disease, stroke, preemclampsia
Citation: Qiu C, Li Z, Leigh DA, Duan B, Stucky JE, Kim N, Xie G, Lu KP and Zhou XZ (2024) The role of the Pin1-cis P-tau axis in the development and treatment of vascular contribution to cognitive impairment and dementia and preeclampsia. Front. Cell Dev. Biol. 12:1343962. doi: 10.3389/fcell.2024.1343962
Received: 24 November 2023; Accepted: 14 March 2024;
Published: 02 April 2024.
Edited by:
Giovanni Levi, Centre National de la Recherche Scientifique (CNRS), FranceReviewed by:
Laura Beth McIntire, NewYork-Presbyterian, United StatesCopyright © 2024 Qiu, Li, Leigh, Duan, Stucky, Kim, Xie, Lu and Zhou. This is an open-access article distributed under the terms of the Creative Commons Attribution License (CC BY). The use, distribution or reproduction in other forums is permitted, provided the original author(s) and the copyright owner(s) are credited and that the original publication in this journal is cited, in accordance with accepted academic practice. No use, distribution or reproduction is permitted which does not comply with these terms.
*Correspondence: Xiao Zhen Zhou, eHpob3U2NTlAdXdvLmNh; Kun Ping Lu, a2x1OTJAdXdvLmNh
†These authors have contributed equally to this work
Disclaimer: All claims expressed in this article are solely those of the authors and do not necessarily represent those of their affiliated organizations, or those of the publisher, the editors and the reviewers. Any product that may be evaluated in this article or claim that may be made by its manufacturer is not guaranteed or endorsed by the publisher.
Research integrity at Frontiers
Learn more about the work of our research integrity team to safeguard the quality of each article we publish.