- 1Centre de Recherche du Centre Hospitalier de I’Université de Montréal (CRCHUM), Montréal, QC, Canada
- 2Department of Obstetrics and Gynaecology, Université de Montréal, Montréal, QC, Canada
- 3Department of Pathology and Cell Biology, Université de Montréal, Montréal, QC, Canada
Culture of oocytes and embryos in media under oil is a cornerstone of fertility treatment, and extensively employed in experimental investigation of early mammalian development. It has been noted anecdotally by some that certain small molecule inhibitors might lose activity in oil-covered culture systems, presumably by drug partitioning into the oil. Here we took a pseudo-pharmacological approach to appraise this formally using mouse oocytes and embryos. Using different culture dish designs with defined media:oil volume ratios, we show that the EC50 of the widely employed microtubule poison nocodazole shifts as a function of the media:oil ratio, such that nocodazole concentrations that prevent cell division in oil-free culture fail to in oil-covered media drops. Relatively subtle changes in culture dish design lead to measurable changes in EC50. This effect is not specific to one type of culture oil, and can be readily observed both in oocyte and embryo culture experiments. We subsequently applied a similar approach to a small panel of widely employed cell cycle-related inhibitors, finding that most lose activity in standard oil-covered oocyte/embryo culture systems. Our data suggest that loss of small molecule activity in oil-covered oocyte and embryo culture is a widespread phenomenon with potentially far-reaching implications for data reproducibility, and we recommend avoiding oil-covered culture for experiments employing inhibitors/drugs wherever possible.
Introduction
Oocyte and embryo culture in fertility clinics and research labs is routinely performed in plastic Petri dishes in drops of media under a covering layer of oil to prevent contamination and evaporation, often termed the ‘closed system’ (Gardner et al., 2014; Eskew and Jungheim, 2017; Gardner et al., 2019). Culture under oil allows dishes to be moved easily within the lab, permits longer-term manipulation out of the incubator such as for micromanipulation or imaging of live oocytes/embryos, and enables ex-vivo experimental access that has been fundamental in understanding early development. Typical dish setups in many labs involve multiple ∼20–50 µL drops of culture media under commercially-available mineral oils in plastic Petri dishes of diameter 3–5 cm, but many variations on this standard setup are used in different experimental contexts (discussed below). Extensive investigation and development takes place to ensure oils are embryo culture-safe, such as examination of the rate of media evaporation using different oils, and reducing the extent to which oils introduce pathogens or contaminants (Otsuki et al., 2009; Swain, 2018; Swain, 2019). Here we document a further hazard of culture oil with important consequences in oocyte and embryo studies.
The past few decades have seen major advances in our understanding of oogenesis and early embryogenesis owing to the ability to pair ex-vivo culture with specific experimental interventions such as conditional transgenics (Kudo et al., 2006; Sasaki et al., 2021), acute gene knockdown approaches (Stein et al., 2003; Homer et al., 2005), and use of small molecule inhibitors (“drugs”) to interrogate molecular mechanisms (Pratt et al., 1981; Longo and Chen, 1985). In this context it has occasionally been noted by some investigators that they avoided oil-covered culture when using some drugs that appear to lose activity under oil, presumably a result of the ability of the drug to partition into the oil. However, a formal appraisal of the effect of oil on drug activities has not to our knowledge been presented, and whether the phenomenon is limited to just a few niche drugs, or impacts diverse and commonly used inhibitors, is unclear.
Here we quantitatively assessed the impact of oil upon the effectiveness of several cell-cycle-related inhibitors that are widely used in oocyte and embryo research. Our results show that changing media:oil ratio profoundly impacts effective drug concentrations of many, and suggest that this is a widespread phenomenon. We argue that the impacts of this upon interpretation of some published data may be significant, and make proposals for experimental design and data reporting in such experiments.
Results
Media:oil ratios change the EC50 of nocodazole in oocyte maturation experiments
We reasoned that if oil reduces the effectiveness of inhibitors, then this should be quantitively demonstrable by altering the media:oil ratio in the culture dish. In this study we used a standardized series of dish setups, as illustrated in Figure 1, all employing the same 35 mm diameter plastic dish. At one extreme, we employed an oil-free culture dish wherein oocytes were cultured in 2 mL of media in a humidified incubator in the complete absence of oil. Our groups also included one 20 µL drop under 2 mL oil, and ten 20 µL drops under 2 mL oil (i.e., 1:100 and 1:10 media:oil ratio respectively), reminiscent of many standard culture systems. At the other extreme we included a setup in which 2 µL of media were placed under 2 mL of oil (1:1000 media:oil ratio). Low media:oil ratios such as this are often employed for live imaging experiments where it is important for oocytes/embryos to remain immobile, co-culture experiments, and also arise in micromanipulation studies where small media drops are used in oversized oil-filled dishes. A ratio of 1:4 comprising 500 µl of media under 2 mL of oil was included, 500 µl of media being approximately the biggest drop that can be made under 2 mL of oil in this dish type whilst retaining a full oil covering to prevent evaporation. Throughout the study we focused on well characterized inhibitors that are documented to prevent first polar body (PB1) extrusion (PBE) at the end of oocyte maturation. We first centered on nocodazole, a mitotic/meiotic spindle poison that causes spindle disassembly by buffering free tubulin, and thus at high concentrations prevents PBE by activating the Spindle Checkpoint (Kubiak et al., 1993; Duncan et al., 2009). Nocodazole is widely employed to explore cell cycle regulation in oocytes and embryos, and used routinely in micromanipulation studies to soften the cytoplasm for enucleation (Darbandi et al., 2017; Nakagawa and FitzHarris, 2017). Importantly, to our knowledge, nocodazole has not previously been noted to lose activity under oil.
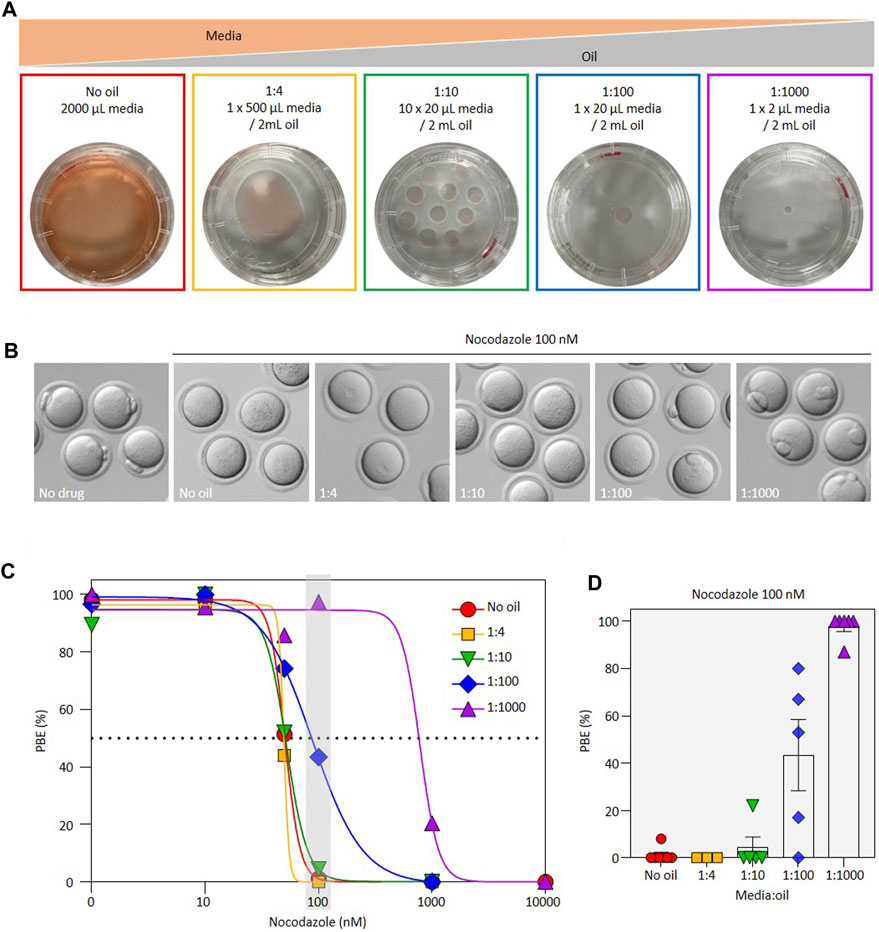
FIGURE 1. Effect of media:oil ratio on the EC50 of nocodazole during oocyte maturation. (A) Schematic representation of the experimental design to address impact of media:oil ratio on drug effects. Conditions were: no oil (2 mL media without oil); 1:4 (1 × 500 µL media covered with 2 mL oil); 1:10 (10 × 20 µL media covered with 2 mL oil); 1:100 (1 × 20 µL media covered with 2 mL oil) and 1:1000 (1 × 2 µL media covered with 2 mL oil). (B) Bright-field images illustrating different outcomes of PBE in oocytes incubated in 100 nM nocodazole for different media:oil ratios. (C) Dose-response curves for oocytes incubated in varying concentrations of nocodazole in different media:oil conditions. For this graph, each data point is an average of three to eight replicates, ∼10 oocytes per replicate, n = 1367 oocytes in total. Specific replicate numbers for Figure 1C are as follows for no oil, 1:4, 1:10, 1:100 and 1:1000 conditions, respectively. For 1 nM nocodazole, N = 4, 4, 4, 3, 3; 10 nM, N = 8, 4, 4, 4, 5; for 50 nM N = 8, 5, 5, 5, 4; for 100 nM, N = 8, 3, 5, 6, 7; for 1000 nM, N = 3, 4, 3, 6, 5; for 10,000 nM, N = 3, 0, 0, 0, 3 respectively. (D) Bar chart representation highlighting impact of different media:oil ratios for oocytes incubated in 100 nM nocodazole. Data from same experiments as (D); each data point is a replicate of ∼10 oocytes, n = 332 oocytes in total. Bars represent mean ± SEM.
Nocodazole is well established to prevent PBE during oocyte maturation by depolymerizing the spindle and therefore activating the spindle assembly checkpoint (Homer et al., 2005; Duncan et al., 2009; Illingworth et al., 2010). To establish an accurate EC50 for this effect, we initially cultured oocytes in a range of nocodazole concentrations in the absence of oil. We found that the EC50 for prevention of PBE in the complete absence of oil was 50.7nM, 100 nM nocodazole enforcing a 100% block to PBE. However, EC50 was substantially shifted in oil-covered culture (Figure 1). Notably, in the 1:100 media:oil dish setup, which reflects standard culture, the EC50 was shifted to 88.4nM, with 50% of oocytes extruding PB1. Strikingly, in the 1:1000 dish setup analogous to that used in many labs for live imaging, all oocytes extruded PB1 even at 100 nM nocodazole (EC50 of 785 nM). Thus, the EC50 of nocodazole for preventing PBE shifted more than tenfold across a range of media:oil ratios reflective of commonly used experimental setups, such that concentrations of nocodazole that prevent PBE in the absence of oil fail to do so in certain oil-covered dish setups.
Many different embryo culture oils are commercially available and used by various investigators. Therefore, to determine whether this effect was specific to one type of embryo culture oil, we cultured oocytes in 100 nM nocodazole at different media:oil ratios using two different commercially available oils, one heavy oil and one light oil. Both oils are compatible with complete preimplantation embryo development in our lab (not shown). Notably, PBE occurred at lower media:oil ratios despite the presence of nocodazole, regardless of oil type (Figure 2). The extent to which nocodazole was inactivated was significantly less in Heavy oil compared to light (p < 0.0001, ANOVA with Sidak’s multiple comparison test at 1:1000 ratio), but nonetheless the inactivation of nocodazole was pronounced and significant in both oil types. Thus, media:oil ratios used commonly in standard experimental setups substantially impact the EC50 of nocodazole for preventing first PBE, and this is not specific to one type of oil.
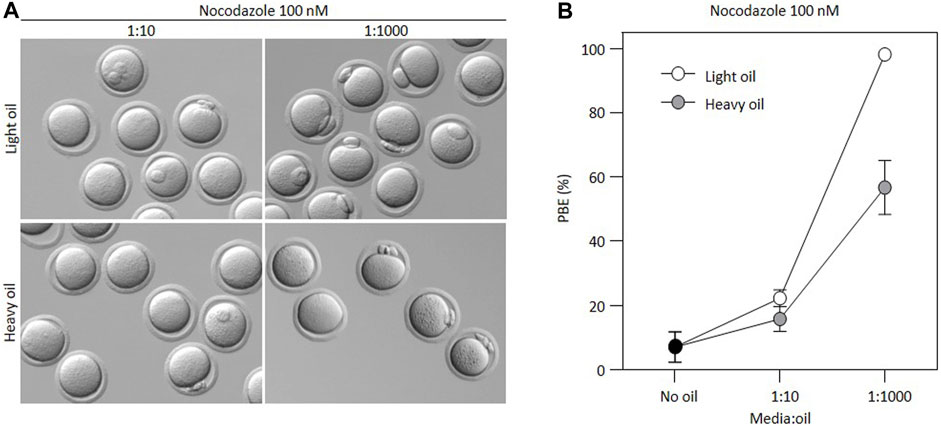
FIGURE 2. Loss of nocodazole activity is not specific to only one type of oil. (A) Bright-field images illustrating outcomes for oocytes incubated in 100 nM nocodazole for different media:oil ratios in light versus heavy mineral oil. (B) Graph representing changing PBE rate for oocytes incubated in 100 nM nocodazole in different media:oil conditions comparing two oil types (light vs. heavy mineral oil). For this graph, each data point is an average of four replicates, ∼10 oocytes per replicate, n = 223 oocytes in total. Bars represent mean ± SEM.
Media:oil ratio alters the EC50 of nocodazole during embryo development
To determine whether the same phenomenon could be observed in preimplantation embryos, we collected 2-cell embryos from mated females, and cultured them in the presence of nocodazole in similar dish setups as described above. Analogous to PBE, in the absence of oil, progression to the 4-cell stage was completely inhibited by 100 nM nocodazole, embryos instead arresting in M-phase of the 2-4 cell division, consistent with the activation of the spindle assembly checkpoint as expected (Figure 3). Notably however, some cells were able to divide in 100 nM nocodazole in a single 20 µL drop under oil (1:100), and only 6% of cells remained M-phase arrested in 2 µL drops under oil (1:1000). Most cells either divided to make normal 4 cell stage cells, or underwent chaotic cell divisions, suggesting low level spindle disruption that failed to activate SAC. Thus the loss of activity under oil of nocodazole that can prevent activation of the SAC in oocytes can also be observed in embryo culture experiments.
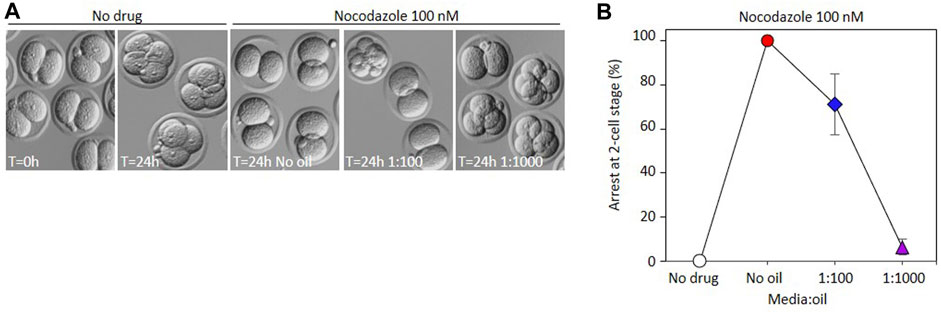
FIGURE 3. Impact of oil on the EC50 of nocodazole during embryo development. (A) Bright-field representative images illustrating appearance of embryos incubated in 100 nM nocodazole with different media:oil ratio conditions. Arrowheads indicate embryos characterized as chaotic. (B) Arrest at 2-cell stage was measured after embryos were incubated in 100 nM nocodazole in different media:oil ratios. Bars represent mean ± SEM. For B and C, each data point is an average mean of four replicates, 120 embryos in total.
Impact of oil on diverse cell cycle inhibitors
Our pseudo-pharmacological analysis of the impact of media:oil ratios across a concentration range of nocodazole clearly demonstrates the impact of oil on the EC50 for preventing the completion of cell division, either in meiosis or mitosis. However, assembling the data presented in Figure 1D alone required 1367 mouse oocytes across dozens of experimental days. We therefore sought a simplified approach that could feasibly be used by labs to test the effect of oil on a given drug. We decided to first establish the concentration-dependency for PBE of a given drug in the complete absence of oil, and then use a minimum effective concentration to investigate the impact of different media:oil ratios. Although this approach does not allow formal appraisal of EC50 shift, it provides a quantifiable indication of the extent to which drug activity is lost under oil (Figure 4). We applied this approach to an additional five drugs that have been heavily employed in mouse oocyte studies: the proteasome inhibitor MG132 (Mailhes et al., 2002), the kinesin-5 inhibitors STLC and monastrol, the APC inhibitor APCin (Mihajlović et al., 2021), and the CDK1 inhibitor roscovitine. In all cases we added the drugs at the time of IBMX washout, as for nocodazole. APCin, MG132, STLC and monastrol are all compatible with GVBD but prevent PBE, similar to nocodazole. Roscovitine prevents GVBD, and thus our analyses were on the ability of oil to permit GVBD in the presence of roscovitine. Strikingly, as for nocodazole, the ability to prevent PBE was substantially reduced for MG132, STLC and monastrol. Oil potently prevented roscovitine from inhibiting GVBD, even the 1:4 media:oil dish setup permitting GVBD in ∼50% of cases. Thus, oil coverings potently inactivate a range of cell cycle related drugs.
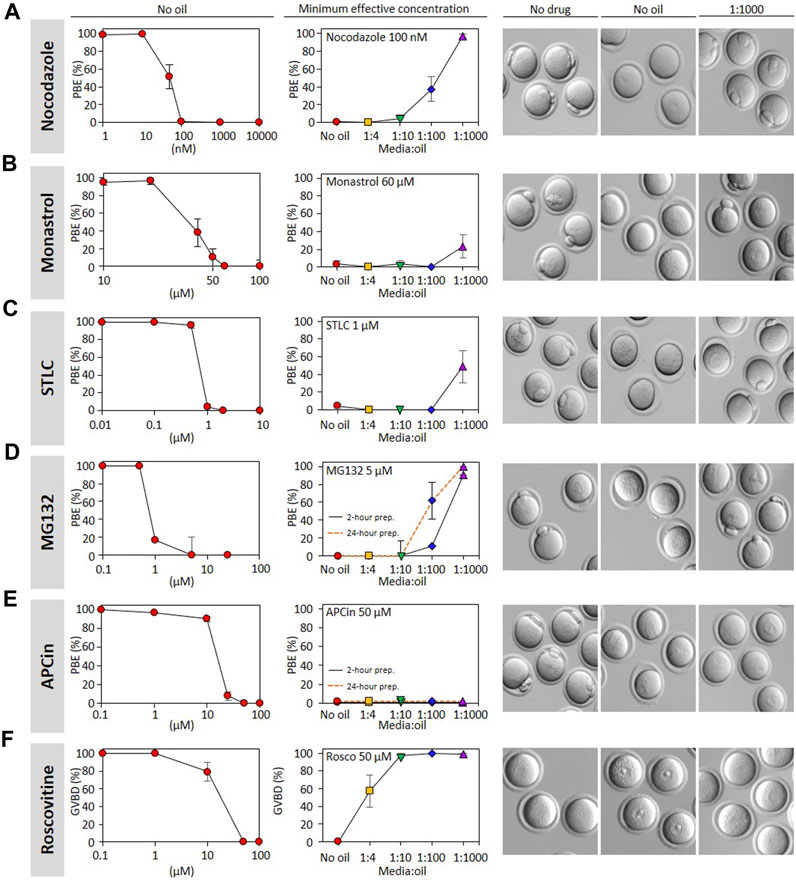
FIGURE 4. Oil covered culture affects the action of many inhibitors. Polar body extrusion (PBE) was assessed following incubation of GV oocytes with (A) nocodazole; (B) monastrol; (C) STLC; (D) MG132; (E) APCin. Germinal vesicle breakdown (GVBD) was evaluated with GV oocytes treated with (F) roscovitine. Oocytes were first incubated in varying concentrations of inhibitors without oil in order to determine the effective concentration of these drugs (i.e., concentration-response curves). In the central column, the lowest maximally effective concentration was used to analyze the impact of media:oil ratios. Dishes with media containing inhibitors ± oil were prepared and pre-equilibrated at 37°C with 5% CO2 for 2 h prior to oocytes being transferred to them, as elsewhere in the study, and for MG132 (D) and APCin (E), a parallel set of dishes were additionally pre-equilibrated for 24 h prior to oocytes incubation. On the right, bright-field images represent oocytes status when evaluating PBE and GVBD. Each data point is the mean of a minimum of three replicates, ∼10 oocytes/replicate, a total of 2245 oocytes throughout the figure. Bars represent mean ± SEM. Specific replicate numbers for Figure 4 are as follows. Nocodazole without oil => 1 nM, N = 4; 10 nM N = 8; 50 nM, N = 8; 100 nM, N = 8; 1,000 nM, N = 3; 10,000 nM, N = 3. Nocodazole 100 nM N = 8, 3, 5, 6, 7 for no oil, 1:4, 1:10, 1:100 and 1:1000, respectively. Monastrol without oil => 10 µM: N = 3; 20µM, N = 3; 40 μM, N = 3; 50 μM, N = 4; 60 µM, N = 4; 100 μM, N = 3. Monastrol 60 µM => N = 3 for each media:oil conditions. STLC without oil => 0.01 μM N = 3; 0.1 μM N = 3; 0.5 μM N = 3; 1 μM N = 5; 2 μM N = 3; 10 μM N = 3. STLC 1 µM=> N = 5, 3, 3, 3, 3 for no oil, 1:4, 1:10, 1:100 and 1:1000 conditions respectively. For MG132, N = 3 for all conditions. For APCin without oil => 0.1 µM, N = 3; 1µM, N = 4; 10 µM, N = 4; 25 μM, N = 4; 50 μM, N = 4; 100 μM N = 3. APCin 50 µM 2-h or 24-h conditioned dishes N = 3 for all media:oil conditions. Roscovitine without oil N = 3 for all concentrations. Roscovitine 50 μM N = 3, 3, 3, 3, 5 for no oil, 1:4, 1:10, 1:100 and 1:1000, respectively.
In contrast, it was noteworthy that 50 µM APCin retained the ability to prevent PBE even at low media:oil ratios. We wondered whether this indicated a slower loss of activity, and perhaps loss of activity might become evident over a longer time-course. We therefore examined APCin after a 24 h pre-incubation under oil, and found that the ability to prevent PBE was preserved (Figure 4, dashed curve). Thus, although nocodazole, MG132, STLC, monastrol, and roscovitine clearly lose activity under oil, we were unable to find evidence that APCin activity is lost, even after long-term media-oil contact.
Finally, we wondered whether drugs that lose activity under oil may do so even more profoundly after a 24 h incubation. To test this we examined the impact of a 24 h preincubation under oil upon MG132, one of the drugs that most profoundly lost activity even in our standard experimental setup (2 h preincubation under oil). We found that MG132 was even further inactivated by a 24 h incubation under oil, such that ∼60% of oocytes extruded PB1 in the 1:100 dish setup compared to only ∼10% in the standard 2 h experiment (all at 5 µM MG132) (Figure Fig4, dashed curve). This indicates that even the timing of dish setup (e.g., whether dishes are prepared the night before) affects drug EC50s.
To summarise, our analysis of a panel of 6 drugs suggests that although some specific drugs may retain their activity under oil, as exemplified by APCin, five of the six we tested are very clearly inactivated by a covering layer of oil, and that the extent of this inactivation can be dependent upon time spent under oil.
Discussion
Here we have used a conceptually simple experimental approach to demonstrate that the effective concentration of an array of commonly used cell cycle drugs is dramatically changed by culturing under oil. Although we have not formally measured the presence of drugs within oil after culture, and other possible explanations such as altered drug dynamics or availability in different culture conditions and drop sizes can be conceived, the simplest interpretation is that each drug partitions into the oil, and the oil acts as a sink. Some level of hydrophobicity is necessary for most drugs to enter cells. Moreover, LogP values that are provided with most inhibitors provide a broad indication of oil solubility based on water/octanol partitioning, and these values tend to support the notion that these drugs should partition into oil. Whilst our data suggest that the extent of drug partitioning into the oil might differ between oil types (Figure 2), the effect was nonetheless very pronounced both in heavy and light oils, and so we recommend that it be presumed that drugs are likely to partition into the oil regardless of oil type/brand employed, unless clearly demonstrated not to.
In some cases partial drug loss under oil may have little impact on broad experimental conclusions, particularly where supra-maximal concentrations are used to elicit well characterised effects. However, there are several types of conclusions that are more precarious. For example, ‘negative results’ in which a drug appears to have no impact upon a cellular process, or circumstances in which unexpectedly high concentrations are needed to elicit expected effects, may warrant revisiting. Moreover, interpretations of which molecular species are being inhibited by selective inhibitors at specific concentrations should be interpreted with extreme caution if oil-covered culture was employed. Instances in which different inhibitor concentrations were required between studies to achieve a given phenotype could be explained by different dish setups and even the timing of their preparation.
Although here we have formally examined the loss of activity under oil of only 6 drugs, there are strong clues that many others behave similarly. Blegini and Schindler (Blengini et al., 2022) have noted that they avoid oil for Aurora-Kinase inhibitors. The Wassmann group noted that when using the MPS1/AurK inhibitor Reversine they supplemented the culture oil with drug (Gryaznova et al., 2021). Halet et al. used the PI3K inhibitor LY294002 in oil free conditions, noting a dramatic reduction in the required concentration to prevent preimplantation embryo development compared to other studies (Riley et al., 2005, Halet et al., 2008). Doubtless many other examples exist. Other drugs that we have anecdotally observed in our lab to lose activity under oil include the CENPE inhibitor GSK923295 (Vázquez-Diez et al., 2019), the APCcdh1 inhibitor Protame (Vázquez-Diez et al., 2019), and the myosin ATPase blocker Blebbistatin (Paim and FitzHarris, 2019). Nonetheless our data show that at least one small molecule inhibitor, APCin, did not partition into the oil, even after a 24 h incubation. Why this is the case is unclear, since LogP value for APCin and inferred polarity based on DMSO solubility would lead one to predict that APCin might do so. The case of APCin thus demonstrates that it is possible for a drug to remain in the media under oil, but this is the minority case and impossible to predict, and thus needs to be demonstrated empirically on a drug-by-drug basis if oil covering is to be used. Overall, while we believe the present study is the first to formally quantitate and highlight the effect, loss of drug activity under oil has certainly been noted by others, and is likely a widespread phenomenon.
To conclude, we advise caution when employing small molecule inhibitors, avoiding oil-covered culture wherever possible. Where the use of oil is unavoidable given the experimental context, in some live imaging studies, for example, thorough testing should be carried out to demonstrate that the media:oil ratio employed is far below the threshold where results are affected. Most importantly, detailed experimental information including exact media:oil ratios and timing of dish setup should be clearly reported when drugs are used under oil. As increasing importance is rightly placed upon data reproducibility, elimination of factors that inadvertently change experimental conditions in a manner that could critically alter results is paramount.
Materials and methods
Oocyte and embryo collection
Mouse oocytes were collected at the GV stage from the ovaries of CD1 females (Charles River Crl:CD1(ICR) 022) aged 6–12 weeks, after intraperitoneal injection of 5 IU pregnant mare serum gonadotropin (PMSG; Aviva system biotech OPPA01037). Oocytes were collected in M2 media containing 200 μM 3-isobutyl-I-methylxanthine (IBMX; Sigma I5879). Following collection oocytes were kept in M16 medium supplemented with IBMX to prevent GVBD (Wisent 311-630-QL) at 37C under 5% CO2, prior to transfer to experimental dishes (see below). Mouse two-cell embryos were collected from the oviducts of CD1 females aged 6–12 weeks, following intraperitoneal injection of 5 IU pregnant mare serum gonadotropin (∼90 h pre-collect) and 5 IU human chorionic gonadotropin (hCG; Sigma; ∼40–48 h pre-collect) and mating with BDF1 male mice (Jackson 100006). Embryos were collected in M2 media and cultured in KSOM (Wisent 003–026-XL) under 5% CO2 at 37 C. All animal experiments were performed in accordance with relevant CIPA (Comité institutionnel de protection des animaux - CHUM) guidelines and regulations under protocol IP22054GFs.
Small molecule treatments and analysis
Following collection oocytes were incubated in M16 + IBMX for 1–3 h to allow zona release (Tartia et al., 2009) and a final selection of healthy fully-grown oocytes was performed. To test the effect of oil on inhibitors, 35-mm plastic culture dishes (Sarstedt 83900) were prepared with different media:oil ratios as indicated in Figure 1 (“experimental dishes”). Importantly, experimental dishes were prepared exactly 2 h prior to the addition of oocytes/embryos for examination of the effect of drugs/oil in all cases, with the exception of Figures 4D, E which also included a group in which the dishes were assembled 24 h prior. Light oil (Sigma M8410) was used for all experiments, and heavy oil (Sigma 330760) was used in addition in Figure 2. The media:oil ratios used in experimental dishes were: no oil (2 mL media without oil); 1:4 (1 × 500 uL media covered with 2 mL oil); 1:10 (10 × 20 uL media covered with 2 mL oil); 1:100 (1 × 20 uL media covered with 2 mL oil) and 1:1000 (1 × 2 uL media covered with 2 mL oil)). For embryo experiments, two-cell embryos were collected and then transferred in inhibitors 2 h after collection, which was again exactly 2 h after dish setup.
Oocyte maturation was triggered by washing oocytes into IBMX-free media. Immediately thereafter, oocytes were transferred into the experimental dishes. Oocytes or embryos was washed through four drops of media with inhibitor in a similar setup (concentration, and media:oil ratio) as the final experimental dish, and then transferred to the experimental dish. On any given experimental day each investigator cultured an additional control group of oocytes or embryos incubated entirely without inhibitors—data was included in the final dataset from any experimental day only if >90% development (GVBD, PBE, or 2–4 cell division) was observed in this sentinel group. Inhibitors used in this project were: nocodazole (Calbiochem/Millipore 487928); monastrol (Calbiochem/Millipore 475879); S-Trityl-L-cysteine (STLC; Tocris 2191); MG132 (Calbiochem/Millipore 474790); APCin (Tocris 5747) and roscovitine (Sigma R7772). Effects of the treatment was assessed 16–18 h post transfer into inhibitors. Bright-field images were captured using a Leica M165C dissection scope equipped with a camera (Camera Opti-Vision 4K LITE- 8 MP Opti-Tech Scientific). Calculation of EC50 was performed in graphpad prism. Data were entered as XY table, where X = concentration and Y=PBE. A nonlinear regression analysis was performed and a dose response curve was fitted. EC50 was calculated by the software from the fitted curve.
Data availability statement
The raw data supporting the conclusion of this article will be made available by the authors, without undue reservation.
Ethics statement
The animal study was approved by Comité institutionnel de protection des animaux—Centre Hospitalier de l’Université de Montréal (CIPA) (protocol IP22054GFs—GF) under the aegis of the Canadian Council on Animal Care (CCAC). The study was conducted in accordance with the local legislation and institutional requirements.
Author contributions
GR: Conceptualization, Data curation, Formal Analysis, Investigation, Methodology, Validation, Writing–review and editing. SC: Conceptualization, Data curation, Formal Analysis, Investigation, Methodology, Validation, Writing–review and editing. ÉB: Conceptualization, Data curation, Formal Analysis, Investigation, Methodology, Validation, Writing–review and editing. KG: Conceptualization, Data curation, Formal Analysis, Investigation, Methodology, Validation, Writing–review and editing. FV: Conceptualization, Data curation, Formal Analysis, Investigation, Methodology, Writing–review and editing. AM: Conceptualization, Data curation, Formal Analysis, Methodology, Writing–review and editing. GF: Conceptualization, Data curation, Formal Analysis, Methodology, Writing–review and editing, Funding acquisition, Investigation, Project administration, Resources, Supervision, Validation, Writing–original draft.
Funding
The author(s) declare financial support was received for the research, authorship, and/or publication of this article. This research was supported by the grants from Canadian Institute for Health Research (CIHR), Foundation Jean-Louis Levesque, and Natural Sciences and Engineering Research Council of Canada (NSERC) to GF. EB received a scholarship from McGill University’s Centre for Research in Reproduction and Development (CRRD), and SC received a scholarship from Université de Montréal Centre de recherche en reproduction et fertilité (CRRF).
Acknowledgments
We thank François Proulx, Karen Schindler, and Guillaume Halet for valuable discussions and for critical reading of the manuscript. A version of this manuscript has been published as a preprint on the BioRxiv website (Rémillard Labrosse et al., 2023).
Conflict of interest
The authors declare that the research was conducted in the absence of any commercial or financial relationships that could be construed as a potential conflict of interest.
Publisher’s note
All claims expressed in this article are solely those of the authors and do not necessarily represent those of their affiliated organizations, or those of the publisher, the editors and the reviewers. Any product that may be evaluated in this article, or claim that may be made by its manufacturer, is not guaranteed or endorsed by the publisher.
References
Blengini, C. S., Ik Jung, G., Aboelenain, M., and Schindler, K. (2022). A field guide to Aurora kinase inhibitors: an oocyte perspective. Reproduction 164, V5–V7. doi:10.1530/REP-22-0292
Darbandi, S., Darbandi, M., Khorram Khorshid, H. R., Shirazi, A., Sadeghi, M. R., Agarwal, A., et al. (2017). Reconstruction of mammalian oocytes by germinal vesicle transfer: a systematic review. Int. J. Reprod. Biomed. 15, 601–612. doi:10.29252/ijrm.15.10.2
Duncan, F. E., Chiang, T., Schultz, R. M., and Lampson, M. A. (2009). Evidence that a defective spindle assembly checkpoint is not the primary cause of maternal age-associated aneuploidy in mouse eggs. Biol. Reprod. 81, 768–776. doi:10.1095/biolreprod.109.077909
Eskew, A. M., and Jungheim, E. S. (2017). A history of developments to improve in vitro fertilization. Mo Med. 114, 156–159.
Gardner, D. K., and Lane, M. (2014). “Chapter 6 - culture of viable mammalian embryos in vitro,” in Principles of cloning. Editors J. Cibelli, R. Lanza, K. H. S. Campbell, and M. D. West Second Edition (Academic Press), 63–84. doi:10.1016/B978-0-12-386541-0.00006-0
Gardner, D. K., and Truong, T. T. (2019). “Culture of the mouse preimplantation embryo,” in Comparative embryo culture: methods and protocols. Editor J. R. Herrick (Springer), 13–32. doi:10.1007/978-1-4939-9566-0_2
Gryaznova, Y., Keating, L., Touati, S. A., Cladière, D., El Yakoubi, W., Buffin, E., et al. (2021). Kinetochore individualization in meiosis I is required for centromeric cohesin removal in meiosis II. EMBO J. 40, e106797. doi:10.15252/embj.2020106797
Halet, G., Viard, P., and Carroll, J. (2008). Constitutive PtdIns(3,4,5)P3 synthesis promotes the development and survival of early mammalian embryos. Development 135, 425–429. doi:10.1242/dev.014894
Homer, H. A., McDougall, A., Levasseur, M., Yallop, K., Murdoch, A. P., and Herbert, M. (2005). Mad2 prevents aneuploidy and premature proteolysis of cyclin B and securin during meiosis I in mouse oocytes. Genes. Dev. 19, 202–207. doi:10.1101/gad.328105
Illingworth, C., Pirmadjid, N., Serhal, P., Howe, K., and Fitzharris, G. (2010). MCAK regulates chromosome alignment but is not necessary for preventing aneuploidy in mouse oocyte meiosis I. Development 137, 2133–2138. doi:10.1242/dev.048306
Kubiak, J. Z., Weber, M., de Pennart, H., Winston, N. J., and Maro, B. (1993). The metaphase II arrest in mouse oocytes is controlled through microtubule-dependent destruction of cyclin B in the presence of CSF. EMBO J. 12, 3773–3778. doi:10.1002/j.1460-2075.1993.tb06055.x
Kudo, N. R., Wassmann, K., Anger, M., Schuh, M., Wirth, K. G., Xu, H., et al. (2006). Resolution of chiasmata in oocytes requires separase-mediated proteolysis. Cell 126, 135–146. doi:10.1016/j.cell.2006.05.033
Longo, F. J., and Chen, D.-Y. (1985). Development of cortical polarity in mouse eggs: involvement of the meiotic apparatus. Dev. Biol. 107, 382–394. doi:10.1016/0012-1606(85)90320-3
Mailhes, J. B., Hilliard, C., Lowery, M., and London, S. N. (2002). MG-132, an inhibitor of proteasomes and calpains, induced inhibition of oocyte maturation and aneuploidy in mouse oocytes. Cell and Chromosome 1, 2. doi:10.1186/1475-9268-1-2
Mihajlović, A. I., Haverfield, J., and FitzHarris, G. (2021). Distinct classes of lagging chromosome underpin age-related oocyte aneuploidy in mouse. Dev. Cell 56, 2273–2283.e3. doi:10.1016/j.devcel.2021.07.022
Nakagawa, S., and FitzHarris, G. (2017). Intrinsically defective microtubule dynamics contribute to age-related chromosome segregation errors in mouse oocyte meiosis-I. Curr. Biol. 27, 1040–1047. doi:10.1016/j.cub.2017.02.025
Otsuki, J., Nagai, Y., and Chiba, K. (2009). Damage of embryo development caused by peroxidized mineral oil and its association with albumin in culture. Fertil. Steril. 91, 1745–1749. doi:10.1016/j.fertnstert.2008.03.001
Paim, L. M. G., and FitzHarris, G. (2019). Tetraploidy causes chromosomal instability in acentriolar mouse embryos. Nat. Commun. 10, 4834. doi:10.1038/s41467-019-12772-8
Pratt, H. P., Chakraborty, J., and Surani, M. A. (1981). Molecular and morphological differentiation of the mouse blastocyst after manipulations of compaction with cytochalasin D. Cell 26, 279–292. doi:10.1016/0092-8674(81)90310-x
Rémillard Labrosse, G., Cohen, S., Boucher, É., Gagnon, K., Vasilev, F., Mihajlović, A., et al. (2023). Oocyte and embryo culture under oil profoundly alters effective concentrations of small molecule inhibitors. bioRxiv. doi:10.1101/2023.11.10.566607
Riley, J. K., Carayannopoulos, M. O., Wyman, A. H., Chi, M., Ratajczak, C. K., and Moley, K. H. (2005). The PI3K/Akt pathway is present and functional in the preimplantation mouse embryo. Dev. Biol. 284, 377–386. doi:10.1016/j.ydbio.2005.05.033
Sasaki, K., Takaoka, S., and Obata, Y. (2021). Oocyte-specific gene knockdown by intronic artificial microRNAs driven by Zp3 transcription in mice. J. Reprod. Dev. 67, 229–234. doi:10.1262/jrd.2020-146
Stein, P., Svoboda, P., and Schultz, R. M. (2003). Transgenic RNAi in mouse oocytes: a simple and fast approach to study gene function. Dev. Biol. 256, 187–193. doi:10.1016/s0012-1606(02)00122-7
Swain, J. E. (2018). Different mineral oils used for embryo culture microdrop overlay differentially impact media evaporation. Fertil. Steril. 109, e53. doi:10.1016/j.fertnstert.2018.02.101
Swain, J. E. (2019). Controversies in ART: considerations and risks for uninterrupted embryo culture. Reprod. Biomed. Online 39, 19–26. doi:10.1016/j.rbmo.2019.02.009
Tartia, A. P., Rudraraju, N., Richards, T., Hammer, M. A., Talbot, P., and Baltz, J. M. (2009). Cell volume regulation is initiated in mouse oocytes after ovulation. Development 136, 2247–2254. doi:10.1242/dev.036756
Keywords: oocyte, embryo, culture, artefact, inhibitor
Citation: Rémillard-Labrosse G, Cohen S, Boucher É, Gagnon K, Vasilev F, Mihajlović AI and FitzHarris G (2024) Oocyte and embryo culture under oil profoundly alters effective concentrations of small molecule inhibitors. Front. Cell Dev. Biol. 12:1337937. doi: 10.3389/fcell.2024.1337937
Received: 13 November 2023; Accepted: 19 February 2024;
Published: 08 March 2024.
Edited by:
Jason Knott, Michigan State University, United StatesReviewed by:
Zuzana Holubcová, Masaryk University, CzechiaNicole Camlin, University of Southern Mississippi, United States
Copyright © 2024 Rémillard-Labrosse, Cohen, Boucher, Gagnon, Vasilev, Mihajlović and FitzHarris. This is an open-access article distributed under the terms of the Creative Commons Attribution License (CC BY). The use, distribution or reproduction in other forums is permitted, provided the original author(s) and the copyright owner(s) are credited and that the original publication in this journal is cited, in accordance with accepted academic practice. No use, distribution or reproduction is permitted which does not comply with these terms.
*Correspondence: Greg FitzHarris, greg.fitzharris@umontreal.ca
†These authors have contributed equally to this work