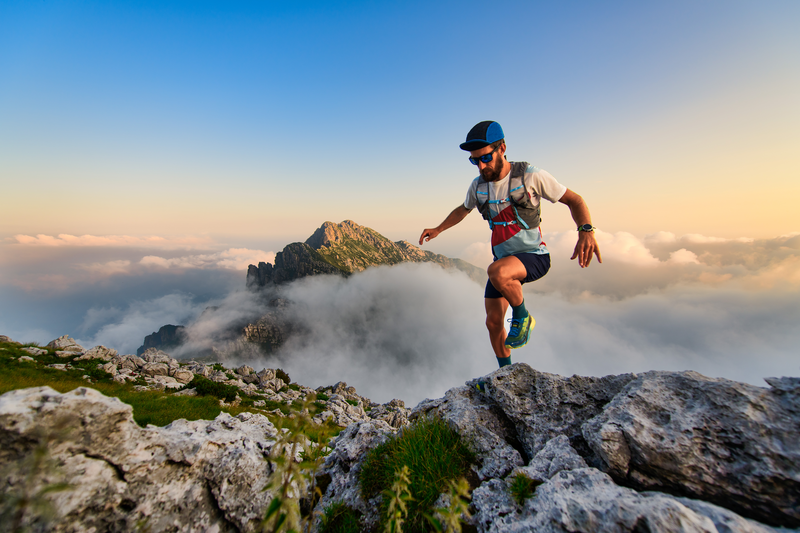
94% of researchers rate our articles as excellent or good
Learn more about the work of our research integrity team to safeguard the quality of each article we publish.
Find out more
REVIEW article
Front. Cell Dev. Biol. , 25 January 2024
Sec. Cancer Cell Biology
Volume 12 - 2024 | https://doi.org/10.3389/fcell.2024.1323348
This article is part of the Research Topic Catch Me If You Can: Cellular Plasticity in Tumor Progression and Drug Resistance. View all 7 articles
Gastric cancer (GC) is a tumor characterized by high incidence and mortality, with metastasis being the primary cause of poor prognosis. Extracellular vesicles (EVs) are an important intercellular communication medium. They contain bioactive substances such as proteins, nucleic acids, and lipids. EVs play a crucial biological role in the process of GC metastasis. Through mechanisms such as remodeling the tumor microenvironment (TME), immune suppression, promoting angiogenesis, and facilitating epithelial–mesenchymal transition (EMT) and mesothelial–mesenchymal transition (MMT), EVs promote invasion and metastasis in GC. Further exploration of the biological roles of EVs will contribute to our understanding of the mechanisms underlying GC metastasis and may provide novel targets and strategies for the diagnosis and treatment of GC. In this review, we summarize the mechanisms by which EVs influence GC metastasis from four aspects: remodeling the TME, modulating the immune system, influencing angiogenesis, and modulating the processes of EMT and MMT. Finally, we briefly summarized the organotropism of GC metastasis as well as the potential and limitations of EVs in GC.
Gastric cancer (GC) is one of the most prevalent tumors globally. According to the 2020 Global Cancer Epidemiology report, GC has the fifth highest incidence and the fourth highest mortality (Sung et al., 2021). Metastasis is the leading cause of death in GC patients, with more than 90% of GC-related deaths attributed to metastasis. Patients with GC metastasis often survive less than 1 year (Orditura et al., 2014). Tumor metastasis follows the theory of “seed” and “soil,” being influenced by both the characteristics of the primary tumor cells and the microenvironment of the metastatic site (Akhtar et al., 2019). The tumor microenvironment (TME) refers to the complex environment composed of cells, stroma, and molecular components surrounding the tumor, it plays an important role in the process of GC metastasis, promoting tumor cell dissemination through various mechanisms such as immune response modulation, extracellular matrix remodeling, and angiogenesis promotion (Tang et al., 2022). Epithelial-mesenchymal transition (EMT) refers to the process in which epithelial cells lose their epithelial characteristics and acquire enhanced mesenchymal features, resulting in a more invasive and migratory phenotype. Similarly, mesothelial-mesenchymal transition (MMT) refers to the process in which mesothelial cells undergo changes in cell morphology and acquire characteristics of mesenchymal cells. Both types of cellular transformation enhance the invasive and metastatic capabilities of tumor cells, thereby promoting tumor metastasis (Sandoval et al., 2013; Li et al., 2023a). Tumor cell metastasis is also influenced by the immune system. Under normal circumstances, tumor cells can be recognized and eliminated by the immune system, inhibiting tumor development. However, tumor cells can evade immune system attacks through various mechanisms, thereby promoting tumor metastasis (Becerril-Rico et al., 2021). Additionally, adequate nutrient supply is essential for tumor growth and metastasis. Neoangiogenesis provides a foundation for tumor growth and metastasis, while vascular leakage provides more pathways for tumor cell dissemination (Wang et al., 2022a). These factors interact with each other, ultimately promoting GC metastasis.
Extracellular vesicles (EVs) are membrane-enclosed vesicles released by cells, and they can be secreted by almost all cell types. Their diameters typically range from 30 to 5,000 nm. Generally, based on the size, origin, and functional characteristics of EVs, they can be classified into different subtypes, including exosomes, microvesicles, and apoptotic bodies (Kalluri and McAndrews, 2023; Pourali et al., 2023). EVs were discovered as early as the 1960s, but at that time, little was known about them (Bonucci, 1967; Wolf, 1967; Anderson, 1969). Subsequent research has shown that biological substances such as nucleic acids, proteins, and lipids are found in EVs; they can interact with recipient cells, transmit information, and alter the function and behavior of recipient cells, playing a crucial role in intercellular communication (Majood et al., 2022; Kalluri and McAndrews, 2023).
Numerous studies have reported a close association between EVs and GC metastasis. EVs exhibit a dual role in GC metastasis, as they can both promote and inhibit GC metastasis. This may depend on factors such as the cargos, origins, and characteristics of the recipient cells of EVs (Kalluri and McAndrews, 2023). EVs can influence GC metastasis through remodeling the TME, modulating the immune system, influencing angiogenesis, and modulating the processes of EMT and MMT (Gao et al., 2021a). Figure 1 and Table 1 summarize the relevant mechanisms by which EVs influence GC metastasis. Previous studies have summarized the role of EVs in promoting the formation of pre-metastatic niches in GC, whereas this review focuses on systematically and comprehensively summarizing the bidirectional regulatory role of EVs in GC metastasis from the above-mentioned aspects, expanding our understanding of the role of EVs in GC metastasis. The clinical translation of this knowledge will be helpful for the early treatment of GC.
FIGURE 1. Extracellular vesicles (EVs) influence gastric cancer (GC) metastasis. EVs can promote GC metastasis to sites such as the liver, lungs, lymph nodes, and peritoneum by remodeling the tumor microenvironment (TME), inhibiting the immune system, promoting angiogenesis, and facilitating epithelial–mesenchymal transition (EMT) and mesothelial–mesenchymal transition (MMT). Figure was created with BioRender.com.
The TME refers to the complex ecosystem composed of cells and the extracellular matrix surrounding the tumor. It includes mesenchymal stem cells (MSCs), immune cells, cancer-associated fibroblasts (CAFs), the extracellular matrix, and EVs, among others (Majood et al., 2022). The TME plays a crucial role in GC metastasis, where processes like angiogenesis, immune evasion, tumor-related inflammation, and matrix remodeling interact to promote the dissemination of GC cells (Yang et al., 2023). Numerous studies have reported that EVs modulate the TME through various pathways, influencing the invasive and migratory abilities of tumor cells and ultimately leading to tumor metastasis. Among these, EVs derived from CAFs and MSCs exhibit important regulatory effects on the TME. Figure 2 depicts the relevant mechanisms by which EVs remodel the TME to influence GC metastasis.
FIGURE 2. Extracellular vesicles (EVs) derived from cancer-associated fibroblasts (CAFs) and mesenchymal stem cells (MSCs) in the tumor microenvironment (TME) regulate gastric cancer (GC) metastasis through relevant mechanisms. Figure was created with BioRender.com.
CAFs are important components of the TME and can originate from various precursors, including normal fibroblasts, MSCs, and pericytes (Ning et al., 2018). CAFs possess multiple cellular functions, including extracellular matrix deposition, metabolic reprogramming, and chemoresistance, therefore, they play a significant role in tumor metastasis (LeBleu and Kalluri, 2018; Huang et al., 2023a). The oncogenic gene, miR-27a, can induce fibroblast reprogramming into CAFs, and is overexpressed in GC-derived EVs. Additionally, overexpression of miR-27a in CAFs can enhance the metastatic behavior of GC cells by downregulating CSRP2, thereby assisting tumor cells both in vitro and in vivo during migration and invasion, but its downstream mechanisms are still unclear (Wang et al., 2018). Human GC tissues and plasma significantly overexpress circ_0088300 and circ_0088300 from CAF-derived EVs sponged to miR-1305, leading to downregulation of the JAK/STAT pathway and facilitating tumor metastasis in GC cells (Shi et al., 2021). However, Qu et al. reported that CAF-derived EVs carrying the disheveled binding antagonist of beta catenin3 antisense1 were found to suppress the malignant transformation of GC cells, including migration and invasion, by targeting the miR-181a-5p/sirtuin 1 axis. Additionally, they increased the sensitivity of GC cells to oxaliplatin (Qu et al., 2023). Similarly, Xu et al. reported that EVs derived from CAFs carried miR-139, which suppressed the metastasis of GC by reducing the expression of matrix metalloproteinase 11, both in vivo and in vitro within the TME (Xu et al., 2019). In addition, EVs derived from CD9-positive CAFs significantly stimulated the metastasis of scirrhous-type GC cells, and CD9-positive GC patients had significantly lower 5-year survival, when compared with CD9-negative GC patients. However, the specific molecular mechanisms by which these EVs promote GC cell metastasis are not yet fully understood (Miki et al., 2018).
MSCs are another important component of the TME, and play a crucial regulatory role in the occurrence and development of tumors, involving anti-cancer effects, regulation of angiogenesis, and anti-apoptosis functions (Kolf et al., 2007; Kidd et al., 2009; Ho et al., 2013; Li et al., 2020a). EVs have also been recently found to play a crucial role in tumor metastasis, particularly EVs derived from MSCs, which have gained increasing attention (Zhao et al., 2021; Zhu et al., 2022a; Qi et al., 2023). The overexpression of miR-221 is significantly associated with advanced tumor lymphatic metastasis (Liu et al., 2012), and a study reported that MSC-derived EVs delivered miR-221 to GC cells and promoted their metastasis (Wang et al., 2014). Several studies have also reported that MSC-derived EVs can effectively influence the TME and tumor metastasis through the delivery of miRNAs (Ma et al., 2017; Ji et al., 2023; Kimura et al., 2023). Gu et al. found that MSC-derived EVs induced EMT through the AKT pathway, thereby enhancing the metastasis of GC, however, the key cargoes within these EVs still need to be identified (Gu et al., 2016). Fatty acid oxidation (FAO) in stromal cell metabolic reprogramming plays an important role in tumor metastasis. EVs carrying CD44 derived from GC cells with lymph node metastasis increased FAO activity in Bone marrow-derived MSCs (BM-MSCs) by activating the ERK/PPARγ/CPT1A pathway, resulting in the secretion of IL-8 and STC1, and promoting lymph node metastasis (Huang et al., 2023b). In addition, Mao et al. reported that MSC-derived EVs carrying LINC01559 activated the PI3K/AKT pathway to enhance the migration of GC cells (Wang et al., 2020). EVs secreted by p53-deficient BM-MSCs can transport ubiquitin protein ligase E3 component n-recognin 2 to GC cells and p53 wild-type BM-MSCs, to reprogram cells in the TME and promote GC metastasis by activating the Wnt/β-catenin pathway, but its underlying mechanisms have not been elucidated yet (Mao et al., 2017). MSC-derived EVs can promote GC metastasis, and can also inhibit it. You et al. generated EVs-lipocalin-type prostaglandin D2 synthase (L-PGDS) by transfecting MSCs with an adenovirus encoding L-PGDS. EVs-L-PGDS inhibited the migration and invasion of GC cells and induced apoptosis (You et al., 2022).
The process of tumor metastasis is intricately linked to the body’s immune system. To metastasize, tumor cells must evade immune surveillance and escape from the killing mechanisms of the seeding organs (Fan et al., 2020). Immune cells such as macrophages, neutrophils, and T cells can recognize and eliminate tumor cells, preventing or delaying further tumor spread. Additionally, immune cells can produce cytokines and chemokines that regulate the TME and influence the invasive and metastatic abilities of tumor cells (Khan et al., 2023). EVs participate in cell communication by transporting and delivering bioactive substances such as nucleic acids and proteins, to regulate the function of immune cells and influence tumor metastasis (Joyce et al., 2016; Zhang et al., 2020). Figure 3 depicts the relevant mechanisms by which EVs modulate the immune system to influence GC metastasis.
FIGURE 3. Extracellular vesicles (EVs) can influence gastric cancer (GC) metastasis by modulating immune cells such as macrophages and T cells. Figure was created with BioRender.com.
Macrophages are one of the most important components of the immune system. They exist in two subtypes: M1, which inhibits tumor development, and M2, which promotes tumor development. M2 macrophages can alter the TME, promoting the invasion and migration of tumor cells and providing support for tumor cells metastasis (Chen et al., 2023a; Liu et al., 2023). Increasing evidence suggests that EVs can interact with macrophages, induce M2 polarization of macrophages, and promote the metastasis of GC (Ma et al., 2023a). A study reported that EVs derived from GC induced M2 polarization of macrophages through the STAT3 pathway; M2-polarized macrophages secrete IL-6 to promote the migration of GC cells, leading to peritoneal metastasis of GC, but the cargoes that play a key role in these EVs are still unclear (Ito et al., 2021). Li et al. reported that miR4435-2HG from GC-derived EVs promoted M2 polarization in macrophages through the Jagged1/Notch and JAK1/STAT3 axis, leading to the metastasis of GC (Li et al., 2022). In the study by Qiu et al., miR-519a-3p from GC-derived EVs was found to accumulate mainly in the liver, and EVs enriched with miR-519a-3p stimulated the MAPK/ERK pathway by targeting DUSP2, leading to M2 polarization of macrophages; M2 polarized macrophages accelerated liver metastasis of GC by promoting the formation of pre-metastatic niches and by inducing angiogenesis in the liver. Interestingly, phosphorylation of STAT3 has also been shown to be a key factor in macrophage M2 polarization. However, whether DUSP2 can also promote M2 polarization in macrophages by regulating STAT3 phosphorylation still requires further investigation (Qiu et al., 2022). Similarly, Gu et al. reported that miR-92a-3p from GC-derived EVs accumulated in the lungs. These EVs activated the ERK signaling pathway, induced immune-suppressive phenotypic differentiation of macrophages, increased PD-L1 expression, and promoted lung metastasis of GC. Furthermore, inhibition of the ERK signaling pathway with PD98059 significantly reduced PD-L1 expression in macrophages and inhibited the colonization of GC cells in the lungs (Gu et al., 2023). EVs can promote M2 polarization of macrophages, and can also inhibit M2 polarization. A previous study reported that hsa_circ_0017252 from GC-derived EVs effectively inhibited M2 polarization of macrophages, thereby suppressing the invasion and migration of GC cells (Song et al., 2022). In addition to EVs derived from GC, EVs derived from macrophages can also affect GC metastasis. A study reported that EVs derived from M2 macrophages enhanced the expression of PD-L1 through the P38MAPK pathway, leading to immune escape and promoting the metastasis of GC, however, the study failed to identify the key cargoes in EVs and did not perform more in-depth functional inhibition analysis (Wang et al., 2021a). Apolipoprotein E (ApoE) is a protein with high specificity found in EVs derived from M2-polarized macrophages. EVs secreted by M2 tumor-associated macrophages transfer functional ApoE to GC cells, resulting in activation of the PI3K-AKT signaling pathway and remodeling of the cell cytoskeleton to support migration, thereby promoting the metastasis of GC both in vitro and in vivo (Zheng et al., 2018).
In addition to macrophages, neutrophils and T lymphocytes are also important components of the immune system, and increasing evidence suggests that EVs can affect GC metastasis by acting on neutrophils and T lymphocytes. Transforming growth factor-β1 (TGF-β1) is an immunosuppressive cytokine produced by immune and tumor cells. Yen et al. reported that overexpression of TGF-β1 in EVs from GC patients was associated with lymph node metastasis, and further research has revealed that TGF-β1 in EVs converted naive T cells into FOXP3 Treg cells in vitro, allowing tumor cells to regulate immune surveillance, leading to lymph node metastasis of GC (Yen et al., 2017). EVs derived from GC cells are mainly absorbed by macrophages and NK cells in the lungs and implanted in the lungs, so these EVs can alter the gene expression and cytokine secretion levels of CD8 T cells, inducing apoptosis of CD8 T cells. Prolonged exposure to GC-derived EVs leads to the formation of an immunosuppressive TME in the lungs of mice, resulting in a reduction of CD8 T cells, which promotes lung metastasis of GC. Further research is needed to identify the key cargoes that play a role in these EVs (Liu et al., 2020). Zhang et al. reported that EVs derived from GC cells induced N2 polarization of neutrophils by the HMGB1/TLR4/NF-κB signaling pathway, promoting autophagy and tumor activation, and N2-polarized neutrophils, in turn, promoted the metastasis of GC (Zhang et al., 2018a). In addition, Lu et al. discovered the overexpression of miR-1246 in GC-derived EVs. Mouse experiments have shown that the overexpressed miR-1246 can promote lymphangiogenesis and lymph node metastasis in vivo. Further research revealed that miR-1246 promotes lymphangiogenesis and lymph node metastasis by inhibiting GSK3β to enhance PD-L1 expression and induce CD8+ T cell apoptosis (Lu et al., 2023).
EMT is considered a driving force for tumor cells metastasis, and is a significant contributor to cancer recurrence and metastasis (Zheng et al., 2020; Zhu et al., 2022b). The loss of epithelial cell markers (such as E-cadherin) and the acquisition of mesenchymal cell markers (such as N-cadherin and vimentin) are two key steps in EMT (Kimura et al., 2023). During EMT, tumor cells lose polarity of epithelial cells and acquire mesenchymal-like motility, which enhances their invasive and migratory abilities, which is considered as the initial stage of tumor metastasis (Maharati and Moghbeli, 2023). EVs can carry various bioactive molecules, including proteins, lipids, and nucleic acids, that can regulate EMT by modulating gene expression, activating related signaling pathways, and altering the TME, thereby influencing the metastatic ability of GC (Pan et al., 2017; Piao et al., 2021; Zhang et al., 2023a; Chen et al., 2023b). Figure 4 depicts the relevant mechanisms by which EVs modulate EMT to influence GC metastasis.
FIGURE 4. Extracellular vesicles (EVs) can influence gastric cancer (GC) metastasis by modulating the processes of epithelial–mesenchymal transition (EMT) and mesothelial–mesenchymal transition (MMT). Figure was created with BioRender.com.
Forkhead box protein M1 (FOXM1) is an oncogene involved in regulating tumor growth and metastasis. A study reported that FOXM1-regulated long non-coding RNA (FRLnc1) was significantly upregulated in serum EVs of GC patients, and a significant association was found between FRLnc1 expression in EVs and GC metastasis. Further research revealed that FRLnc1 overexpression in EVs enhanced the activation of the ERK pathway, downregulated E-cadherin, and upregulated Slug and N-cadherin, promoting EMT in GC cells and facilitating GC metastasis. However, further in vivo experiments are needed to confirm this finding. In addition, the specific molecular mechanisms by which FRLnc1 functions in GC metastasis also need to be further studied (Zhang et al., 2021a). Zhao et al. reported that the levels of LINC00355 in plasma EVs of GC patients were significantly higher, when compared with healthy patients, and LINC00355 was found to promote the metastasis of GC cells. Further research revealed that EVs-derived LINC00355 recruited HDAC3 to inhibit the expression of tumor protein 53-induced nuclear protein 1, thereby promoting EMT and resulting in the metastasis of GC (Zhao et al., 2023). Hu et al. reported that EVs derived from malignant ascites of GC patients promoted EMT signaling in GC cells and in a mouse peritoneal tumor model, leading to peritoneal dissemination of the tumor. Moreover, in a mouse peritoneal tumor model, administration of malignant ascites-derived EVs resulted in a significantly reduced median survival, when compared with the control group, however, the molecular mechanisms by which EVs promote peritoneal metastasis of GC has still not been fully understood (Hu et al., 2019). Similarly, Yang et al. found a significant correlation between the elevated expression levels of miR-423-5p in serum EVs of GC patients and lymph node metastasis. Further research revealed that miR-423-5p can suppress the expression of the suppressor of fused protein (SUFU), thereby promoting EMT to facilitate lymph node metastasis in GC (Yang et al., 2018). Hypoxia is a typical characteristic of the TME, and a hypoxic TME leads to changes in tumor features such as angiogenesis, reprogramming of energy metabolism, and immune evasion, resulting in tumor progression (Bristow and Hill, 2008; Jain, 2014; Palazon et al., 2014). Xia et al. reported that in the hypoxic TME, hypoxia-inducible factors 1α increased the release of miR-301a-3p from GC-derived EVs, and treatment of GC cells with these EVs resulted in upregulation of mesenchymal cell markers (N-cadherin and vimentin) and downregulation of epithelial cell markers (E-cadherin), indicating that these EVs promoted tumor metastasis by inducing EMT in GC cells (Xia et al., 2020). An additional study reported that reducing GC-derived EVs altered the molecular mechanisms associated with EMT signaling pathway in GC cells, thereby reducing GC metastasis, particularly peritoneal metastasis, which provided a new direction for the treatment of GC patients (Shibamoto et al., 2022).
Not all EVs promote EMT and contribute to GC metastasis. Tripartite motif-containing 3 (TRIM3) is a key regulator of tumor cell development (Hatakeyama, 2011; Cambiaghi et al., 2012). Compared to healthy patients, GC patients have decreased levels of TRIM3 protein in serum EVs, and knockdown of TRIM3 in EVs from GC patients can alter the expression of EMT-related factors and promote GC metastasis. Conversely, in vivo studies have shown that overexpression of TRIM3 in EVs can inhibit EMT and suppress GC metastasis (Fu et al., 2018). Gastric intrinsic factor 1 (GKN1) is a gastric-specific tumor suppressor that maintains mucosal integrity and regulates cell differentiation (Yoon et al., 2014; Xing et al., 2015; Yoon et al., 2018). When GC cells were co-cultured with EVs rich in GKN1, the expression of E-cadherin in GC cells increased, while the expression of proteins such as N-cadherin significantly decreased, suggesting that GKN1 derived from EVs inhibited GC metastasis by suppressing EMT (Yoon et al., 2020). Studies have also shown that biologically active substances such as miR-486-5p and circ-ITCH delivered by EVs could inhibit the metastasis of GC by suppressing EMT (Wang et al., 2021b; Lin et al., 2021).
MMT refers to the process in which mesothelial cells acquire characteristics of mesenchymal cells, allowing them to gain increased invasiveness (Nakamura et al., 2015). The peritoneum is composed of a monolayer of flat mesothelial cells and a thin layer of submesothelial connective tissue, and the cohesive mesothelial layer, when undamaged, acts as the primary defense against tumor attachment (Mutsaers and Wilkosz, 2007). During MMT, as a consequence of the dissociation from each other within the monolayer, mesothelial cells lose their apical-basal polarity and undergo reorganization of their actin cytoskeleton. In addition, continuous submesothelial connective tissue is also disrupted (Yáñez-Mó et al., 2003; Rynne-Vidal et al., 2015). MMT typically occurs in the early stages of tumor cell peritoneal metastasis, through MMT, the peritoneum can form a pre-metastatic niche, which facilitates the adhesion and colonization of tumor cells (Sandoval et al., 2013). Several studies have therefore reported that EVs could mediate MMT and promote tumor metastasis (Zhu et al., 2020; Gao et al., 2021b; Pascual-Antón et al., 2021). Figure 4 depicts the relevant mechanisms by which EVs modulate MMT to influence GC metastasis.
Deng et al. reported that matrix metalloproteinase 2 in EVs could induce MMT and promote peritoneal metastasis of GC by activating the ERK pathway and increasing the expression of mesenchymal markers, such as vimentin and fibronectin in GC cells (Deng et al., 2017). Previous studies reported that miR-106a was significantly upregulated in GC and may be associated with GC development (Wang et al., 2013; Espinosa-Parrilla et al., 2014). Zhu et al. conducted related studies and found that miR-106a was overexpressed in GC-derived EVs. Stimulation with miR-106a suppressed the expression of Smad7, leading to increased expression of α-SMA and fibronectin in mesothelial cells, to promote MMT and facilitate peritoneal metastasis of GC (Zhu et al., 2022c). Li et al. also found that GC-derived EVs carrying miR-21-5p induced MMT in GC cells through the TGFβ/Smad pathway, resulting in peritoneal metastasis of GC (Li et al., 2018). An additional study reported that the long non-coding RNA small nucleolar RNA host gene 12 (SNHG12) promoted peritoneal metastasis of GC, and the levels of SNHG12 expression in EVs derived from GC patients with peritoneal metastasis were significantly elevated, when compared with those without peritoneal metastasis. GC-derived EVs could deliver SNHG12 to human peritoneal mesothelial cells, inducing MMT and further promoting peritoneal metastasis of GC, and further studies showed that SNHG12 promoted peritoneal metastasis through the miR-129-5p/E2F7/MAPK/ERK axis (Zhang et al., 2022a).
Tumor growth and metastasis rely on blood vessels to provide sufficient oxygen and nutrients. Neoangiogenesis provides a new blood supply to tumors, allowing tumor cells to obtain more oxygen and nutrients. It also provides a pathway for tumor cell metastasis using newly formed blood vessels; tumor cells can enter the bloodstream or lymphatic system and spread to distant organs and tissues (Nowosad et al., 2023; Yao and Zeng, 2023). Previous studies reported that EVs delivered various bioactive substances (such as miR-29, miR-10a-5p, circ29, and GRP78) to promote the expression of vascular endothelial growth factor (VEGF), thereby promoting angiogenesis (Zhang et al., 2016; Li et al., 2021; Zhu et al., 2022d; Iha et al., 2022). In addition, EVs increase vascular permeability, making it easier for tumor cells to cross the endothelial barrier of blood vessels to provide increased opportunities for tumor cell metastasis (Dou et al., 2021). Figure 5 depicts the relevant mechanisms by which EVs regulate angiogenesis to influence GC metastasis.
FIGURE 5. Extracellular vesicles (EVs) can influence gastric cancer (GC) metastasis by inducing angiogenesis. Figure was created with BioRender.com.
The expression of angiopoietin-2 (ANG2) is significantly increased in GC-derived EVs. ANG2 can activate the PI3K/AKT signaling pathway to regulate angiogenesis. Moreover, ANG2 is upregulated in GC omental metastatic samples, indicating that ANG2 may promote GC metastasis by regulating angiogenesis (Kalfon et al., 2022). The circ-RanGAP1 is overexpressed in the EVs from plasma of GC patients and GC tissues, and elevated expression of circ-RanGAP1 is strongly correlated with the prognosis of patients with GC. In addition, circ-RanGAP1 can induce VEGFA expression by sponging miR-877-3p, to promote angiogenesis and thereby facilitate GC metastasis. Further experimental and clinical studies are needed in the future to confirm whether treatments targeting circ-RanGAP1 can be applied in clinical settings (Lu et al., 2020). EVs enriched with circSHKBP1 are upregulated in GC patients, and the level of circSHKBP1 in EVs significantly decreases after GC surgery. CircSHKBP1 promotes VEGF secretion and induces its expression by sponging miR-582-3p, to promote angiogenesis, leading to lung metastasis of GC (Xie et al., 2020). The expression of miR-519a-3p in serum-derived EVs is significantly elevated in GC patients with liver metastasis (LM), when compared with those without LM. EVs derived from GC with overexpression of miR-519a-3p primarily accumulate in the liver, and induce M2 polarization of macrophages by targeting DUSP2 and activating the MAPK/ERK pathway, while M2-polarized macrophages accelerate GC-LM by inducing angiogenesis (Qiu et al., 2022). CircFCHO2 enhances the progression of GC by activating the JAK1/STAT3 signaling pathway by sponging miR-194-5p, and silencing circFCHO2 weakens angiogenesis and cancer stem cell characteristics in GC cells. In addition, in vivo studies have shown that silencing circFCHO2 inhibited lung metastasis of GC (Zhang et al., 2022b). A similar study reported that EVs containing hepatocyte growth factor siRNA administered through tail vein injection in mice effectively inhibited tumor angiogenesis (Zhang et al., 2018b). EVs promote angiogenesis and increase vascular permeability. Wang et al. reported that plasma levels of EVs in mice with GC and lung metastasis were significantly higher than those without lung metastasis, indicating that EVs may promote GC metastasis. Further studies showed that EVs activated endothelial cells and induced cytoskeletal reorganization through a dynamin-dependent pathway, disrupting the endothelial barrier and inducing vascular leakage, leading to lung metastasis of GC. However, the specific mechanisms of action of these EVs and their key cargoes are still unclear (Wang et al., 2022b).
With the introduction of Stephen Paget’s “seed and soil” theory, it has been recognized that tumor metastasis is not random but rather a selective process that targets specific organs (Akhtar et al., 2019). This phenomenon is known as organotropism in tumor metastasis. GC also exhibits organotropism during the metastatic process, where GC cells possess the ability to selectively migrate to certain organs such as the lungs, liver, and peritoneum (Urabe et al., 2021; He et al., 2023). This selective metastasis may be attributed to the affinity of EVs released by GC cells for specific recipient cells. Hoshino et al. discovered that EVs containing integrins can specifically bind to recipient cells, thereby forming pre-metastatic niches and promoting metastasis organotropism in GC (Hoshino et al., 2015). Additionally, Qiu et al. found that EVs rich in miR-519a-3p can induce M2-like polarization of macrophages through the MAPK/ERK pathway, and M2-like polarized macrophages facilitate the formation of pre-metastatic niches in the liver, thereby inducing liver-specific metastasis in GC (Qiu et al., 2022). Similarly, studies have indicated that EVs can promote metastasis organotropism of GC to organs such as the lungs and peritoneum (Li et al., 2018; Zhang et al., 2022a).
EVs serve as important intercellular communication mediators and have great potential in the field of oncology. EVs carry a diverse range of biomolecules that can not only influence tumor growth and metastasis but also serve as tumor markers for cancer diagnosis (Su et al., 2023). Analyzing biomarkers within EVs released by tumor cells therefore facilitates noninvasive tumor diagnosis and monitoring, compared to conventional diagnostic methods, it has the advantages of being rapid, cost-effective, and highly specific (Zhang et al., 2022c; Ma et al., 2023b). For example, the detection of urinary 3-gene expression levels can differentiate between high-grade and low-grade prostate cancer, as well as benign prostate diseases (McKiernan et al., 2016). Additionally, EVs can serve as carriers for drug or small interfering RNA (siRNA) delivery, they can be engineered to possess specific targeting and drug delivery functions. By encapsulating drugs or siRNA within EVs, their stability and bioavailability can be enhanced, while reducing their side effects (Kimura et al., 2023; Zeng et al., 2023). For example, Yu et al. discovered that encapsulating VEGFR2 siRNA within EVs for the treatment of lung metastasis in mice with osteosarcoma is a more efficient and less toxic therapeutic approach (Yu et al., 2023). Similarly, EVs have demonstrated great potential as efficient delivery vehicles for drugs such as paclitaxel and gemcitabine, highlighting their significant role in tumor therapy (Saari et al., 2015; Li et al., 2020b).
However, there are still some limitations in the current application of EVs in tumors. First, obtaining a sufficient quantity and quality of EV samples remains a challenge. The current sample sources primarily include body fluids such as saliva, urine, and blood, but the content of EVs in these fluids is low, and there are many nonspecific EVs present, limiting the accuracy and reliability of their applications (Zhang et al., 2022c). Furthermore, EVs are highly complex particles whose composition and function are influenced by various factors such as cell type, state, and the environment. Accurate identification, isolation, and purification of EVs remain challenging and require further development of technologies and methods (Wu et al., 2021). The emerging methods of EVs separation based on size and charge, as well as single EV analysis techniques in recent years, may help to address these issues (Ko et al., 2021; Zhang et al., 2023b; Chen et al., 2023c). Third, despite extensive studies on the roles of EVs in tumors, the specific mechanisms of action of EVs, especially the signaling pathways, are still not fully understood. In future studies, it is necessary to further explore the specific molecular mechanisms by which EVs affect GC metastasis (Wang et al., 2022b). Finally, although numerous studies have reported the mechanisms of EVs during tumor metastasis, current research is mainly limited to cell and animal experiments, how to apply these findings to the clinic will be one of the key focuses of future work (Kalluri and McAndrews, 2023).
This review focuses on summarizing the biological roles of EVs in GC metastasis from the following aspects. Firstly, EVs can affect the cell interactions and signal transduction in TME by releasing bioactive molecules, thus regulating the process of GC metastasis (Zhang et al., 2021b; Li et al., 2023b). Secondly, the molecules released by EVs, such as metastasis-related proteins and non-coding RNAs, can regulate the EMT and MMT of GC cells, enhance the metastatic ability of GC (Deng et al., 2017; Babaei et al., 2022; Ang et al., 2023). In addition, immune regulatory molecules contained in EVs can regulate tumor immune escape and immune suppression, thus affecting the immune recognition and clearance of GC cells and affecting their metastasis (Kiya et al., 2023; Yi et al., 2023). Finally, the bioactive molecules in EVs can regulate the expression of vascular growth factors, thereby affecting the formation of new blood vessels. The newly formed blood vessels not only increase the blood supply and nutrition of GC cells but also enhance their metastatic ability (Wang et al., 2022b; Qiu et al., 2022). It is also worth noting that tumor metastasis is a multi-step and complex process, and EVs often interact through previously mentioned multiple pathways to influence GC metastasis, so a thorough investigation of the mechanisms of EVs in GC metastasis is crucial for GC. Although a growing body of studies has revealed the biological roles of EVs in GC metastasis, challenges and limitations still exist. With the development of technologies and research, our understanding and application of EVs will continue to improve, providing more options and opportunities for diagnosing and treating GC.
YL: Writing–original draft, Writing–review and editing. SC: Writing–original draft, Writing–review and editing. C-DZ: Writing–review and editing. Y-SL: Writing–original draft, Writing–review and editing.
The author(s) declare financial support was received for the research, authorship, and/or publication of this article. This work was partly supported by Liaoning Provincial Natural Science Foundation (2023-MS-163).
The authors declare that the research was conducted in the absence of any commercial or financial relationships that could be construed as a potential conflict of interest.
All claims expressed in this article are solely those of the authors and do not necessarily represent those of their affiliated organizations, or those of the publisher, the editors and the reviewers. Any product that may be evaluated in this article, or claim that may be made by its manufacturer, is not guaranteed or endorsed by the publisher.
Akhtar, M., Haider, A., Rashid, S., and Al-Nabet, A. (2019). Paget's "seed and soil" theory of cancer metastasis: an idea whose time has come. Adv. anatomic pathology 26 (1), 69–74. doi:10.1097/PAP.0000000000000219
Anderson, H. C. (1969). Vesicles associated with calcification in the matrix of epiphyseal cartilage. J. Cell Biol. 41 (1), 59–72. doi:10.1083/jcb.41.1.59
Ang, H. L., Mohan, C. D., Shanmugam, M. K., Leong, H. C., Makvandi, P., Rangappa, K. S., et al. (2023). Mechanism of epithelial-mesenchymal transition in cancer and its regulation by natural compounds. Med. Res. Rev. 43 (4), 1141–1200. doi:10.1002/med.21948
Babaei, G., Asghari Vostakolaei, M., Rajabi Bazl, M., Gholizadeh-Ghaleh Aziz, S., Gholipour, E., and Nejati-Koshki, K. (2022). The role of exosomes in the molecular mechanisms of metastasis: focusing on EMT and cancer stem cells. Life Sci. 310, 121103. doi:10.1016/j.lfs.2022.121103
Becerril-Rico, J., Alvarado-Ortiz, E., Toledo-Guzmán, M. E., Pelayo, R., and Ortiz-Sánchez, E. (2021). The cross talk between gastric cancer stem cells and the immune microenvironment: a tumor-promoting factor. Stem Cell Res. Ther. 12 (1), 498. doi:10.1186/s13287-021-02562-9
Bonucci, E. (1967). Fine structure of early cartilage calcification. J. Ultrastruct. Res. 20 (1), 33–50. doi:10.1016/s0022-5320(67)80034-0
Bristow, R. G., and Hill, R. P. (2008). Hypoxia and metabolism. Hypoxia, DNA repair and genetic instability. Nat. Rev. Cancer 8 (3), 180–192. doi:10.1038/nrc2344
Cambiaghi, V., Giuliani, V., Lombardi, S., Marinelli, C., Toffalorio, F., and Pelicci, P. G. (2012). TRIM proteins in cancer. Adv. Exp. Med. Biol. 770, 77–91. doi:10.1007/978-1-4614-5398-7_6
Chen, M., Lin, S., Zhou, C., Cui, D., Haick, H., and Tang, N. (2023c). From conventional to microfluidic: progress in extracellular vesicle separation and individual characterization. Adv. Healthc. Mater. 12 (8), e2202437. doi:10.1002/adhm.202202437
Chen, P., Liu, Z., Xiao, H., Yang, X., Li, T., Huang, W., et al. (2023b). Effect of tumor exosome-derived Lnc RNA HOTAIR on the growth and metastasis of gastric cancer. Clin. Transl. Oncol. 25, 3447–3459. doi:10.1007/s12094-023-03208-3
Chen, S., Saeed, A., Liu, Q., Jiang, Q., Xu, H., Xiao, G. G., et al. (2023a). Macrophages in immunoregulation and therapeutics. Signal Transduct. Target Ther. 8 (1), 207. doi:10.1038/s41392-023-01452-1
Deng, G., Qu, J. L., Zhang, Y., Che, X. F., Cheng, Y., Fan, Y. B., et al. (2017). Gastric cancer-derived exosomes promote peritoneal metastasis by destroying the mesothelial barrier. FEBS Lett. 591 (14), 2167–2179. doi:10.1002/1873-3468.12722
Dou, R., Liu, K., Yang, C., Zheng, J., Shi, D., Lin, X., et al. (2021). EMT-cancer cells-derived exosomal miR-27b-3p promotes circulating tumour cells-mediated metastasis by modulating vascular permeability in colorectal cancer. Clin. Transl. Med. 11 (12), e595. doi:10.1002/ctm2.595
Espinosa-Parrilla, Y., Muñoz, X., Bonet, C., Garcia, N., Venceslá, A., Yiannakouris, N., et al. (2014). Genetic association of gastric cancer with miRNA clusters including the cancer-related genes MIR29, MIR25, MIR93 and MIR106: results from the EPIC-EURGAST study. Int. J. cancer 135 (9), 2065–2076. doi:10.1002/ijc.28850
Fan, X., Jin, J., Yan, L., Liu, L., Li, Q., and Xu, Y. (2020). The impaired anti-tumoral effect of immune surveillance cells in the immune microenvironment of gastric cancer. Clin. Immunol. Orl. Fla) 219, 108551. doi:10.1016/j.clim.2020.108551
Fu, H., Yang, H., Zhang, X., Wang, B., Mao, J., Li, X., et al. (2018). Exosomal TRIM3 is a novel marker and therapy target for gastric cancer. J. Exp. Clin. cancer Res. CR 37 (1), 162. doi:10.1186/s13046-018-0825-0
Gao, J., Li, S., Xu, Q., Zhang, X., Huang, M., Dai, X., et al. (2021a). Exosomes promote pre-metastatic niche formation in gastric cancer. Front. Oncol. 11, 652378. doi:10.3389/fonc.2021.652378
Gao, L., Nie, X., Gou, R., Hu, Y., Dong, H., Li, X., et al. (2021b). Exosomal ANXA2 derived from ovarian cancer cells regulates epithelial-mesenchymal plasticity of human peritoneal mesothelial cells. J. Cell. Mol. Med. 25 (23), 10916–10929. doi:10.1111/jcmm.16983
Gu, H., Ji, R., Zhang, X., Wang, M., Zhu, W., Qian, H., et al. (2016). Exosomes derived from human mesenchymal stem cells promote gastric cancer cell growth and migration via the activation of the Akt pathway. Mol. Med. Rep. 14 (4), 3452–3458. doi:10.3892/mmr.2016.5625
Gu, J., Chu, X., Huo, Y. J., Liu, C. Y., Chen, Q. G., Hu, S. N., et al. (2023). Gastric cancer-derived exosomes facilitate pulmonary metastasis by activating ERK-mediated immunosuppressive macrophage polarization. J. Cell. Biochem. 124 (4), 557–572. doi:10.1002/jcb.30390
Hatakeyama, S. (2011). TRIM proteins and cancer. Nat. Rev. Cancer 11 (11), 792–804. doi:10.1038/nrc3139
He, K., Wang, Z., Luo, M., Li, B., Ding, N., Li, L., et al. (2023). Metastasis organotropism in colorectal cancer: advancing toward innovative therapies. J. Transl. Med. 21 (1), 612. doi:10.1186/s12967-023-04460-5
Ho, I. A., Toh, H. C., Ng, W. H., Teo, Y. L., Guo, C. M., Hui, K. M., et al. (2013). Human bone marrow-derived mesenchymal stem cells suppress human glioma growth through inhibition of angiogenesis. Stem cells Dayt. Ohio) 31 (1), 146–155. doi:10.1002/stem.1247
Hoshino, A., Costa-Silva, B., Shen, T. L., Rodrigues, G., Hashimoto, A., Tesic Mark, M., et al. (2015). Tumour exosome integrins determine organotropic metastasis. Nature 527 (7578), 329–335. doi:10.1038/nature15756
Hu, Y. T., Qi, C. S., Liu, X., Zhang, C., Gao, J., Wu, Y., et al. (2019). Malignant ascites-derived exosomes promote peritoneal tumor cell dissemination and reveal a distinct miRNA signature in advanced gastric cancer. Cancer Lett. 457, 142–150. doi:10.1016/j.canlet.2019.04.034
Huang, J., Tsang, W. Y., Li, Z. H., and Guan, X. Y. (2023a). The origin, differentiation, and functions of cancer-associated fibroblasts in gastrointestinal cancer. Cell. Mol. gastroenterology hepatology 16, 503–511. doi:10.1016/j.jcmgh.2023.07.001
Huang, J., Wang, X., Wen, J., Zhao, X., Wu, C., Wang, L., et al. (2023b). Gastric cancer cell-originated small extracellular vesicle induces metabolic reprogramming of BM-MSCs through ERK-PPARγ-CPT1A signaling to potentiate lymphatic metastasis. Cancer Cell Int. 23 (1), 87. doi:10.1186/s12935-023-02935-5
Iha, K., Sato, A., Tsai, H. Y., Sonoda, H., Watabe, S., Yoshimura, T., et al. (2022). Gastric cancer cell-derived exosomal GRP78 enhances angiogenesis upon stimulation of vascular endothelial cells. Curr. issues Mol. Biol. 44 (12), 6145–6157. doi:10.3390/cimb44120419
Ito, A., Kagawa, S., Sakamoto, S., Kuwada, K., Kajioka, H., Yoshimoto, M., et al. (2021). Extracellular vesicles shed from gastric cancer mediate protumor macrophage differentiation. BMC cancer 21 (1), 102. doi:10.1186/s12885-021-07816-6
Jain, R. K. (2014). Antiangiogenesis strategies revisited: from starving tumors to alleviating hypoxia. Cancer Cell 26 (5), 605–622. doi:10.1016/j.ccell.2014.10.006
Ji, R., Lin, J., Gu, H., Ma, J., Fu, M., and Zhang, X. (2023). Gastric cancer derived mesenchymal stem cells promote the migration of gastric cancer cells through miR-374a-5p. Curr. stem Cell Res. Ther. 18 (6), 853–863. doi:10.2174/1574888X18666221124145847
Joyce, D. P., Kerin, M. J., and Dwyer, R. M. (2016). Exosome-encapsulated microRNAs as circulating biomarkers for breast cancer. Int. J. cancer 139 (7), 1443–1448. doi:10.1002/ijc.30179
Kalfon, T., Loewenstein, S., Gerstenhaber, F., Leibou, S., Geller, H., Sher, O., et al. (2022). Gastric cancer-derived extracellular vesicles (EVs) promote angiogenesis via angiopoietin-2. Cancers 14 (12), 2953. doi:10.3390/cancers14122953
Kalluri, R., and McAndrews, K. M. (2023). The role of extracellular vesicles in cancer. Cell 186 (8), 1610–1626. doi:10.1016/j.cell.2023.03.010
Khan, S. U., Fatima, K., Malik, F., Kalkavan, H., and Wani, A. (2023). Cancer metastasis: molecular mechanisms and clinical perspectives. Pharmacol. Ther. 250, 108522. doi:10.1016/j.pharmthera.2023.108522
Kidd, S., Spaeth, E., Dembinski, J. L., Dietrich, M., Watson, K., Klopp, A., et al. (2009). Direct evidence of mesenchymal stem cell tropism for tumor and wounding microenvironments using in vivo bioluminescent imaging. Stem cells Dayt. Ohio) 27 (10), 2614–2623. doi:10.1002/stem.187
Kimura, Y., Ohzawa, H., Miyato, H., Kaneko, Y., Kuchimaru, T., Takahashi, R., et al. (2023). Intraperitoneal transfer of microRNA-29b-containing small extracellular vesicles can suppress peritoneal metastases of gastric cancer. Cancer Sci. 114 (7), 2939–2950. doi:10.1111/cas.15793
Kiya, Y., Yoshioka, Y., Nagakawa, Y., and Ochiya, T. (2023). Extracellular vesicles are important mediators that regulate tumor lymph node metastasis via the immune system. Int. J. Mol. Sci. 24 (2), 1362. doi:10.3390/ijms24021362
Ko, J., Wang, Y., Sheng, K., Weitz, D. A., and Weissleder, R. (2021). Sequencing-based protein analysis of single extracellular vesicles. ACS Nano 15 (3), 5631–5638. doi:10.1021/acsnano.1c00782
Kolf, C. M., Cho, E., and Tuan, R. S. (2007). Mesenchymal stromal cells. Biology of adult mesenchymal stem cells: regulation of niche, self-renewal and differentiation. Arthritis Res. Ther. 9 (1), 204. doi:10.1186/ar2116
LeBleu, V. S., and Kalluri, R. (2018). A peek into cancer-associated fibroblasts: origins, functions and translational impact. Dis. models Mech. 11 (4), dmm029447. doi:10.1242/dmm.029447
Li, C., Chen, Z., Gao, J., Tang, T., Zhou, L., Zhang, G., et al. (2022). MIR4435-2HG in exosomes promotes gastric carcinogenesis by inducing M2 polarization in macrophages. Front. Oncol. 12, 1017745. doi:10.3389/fonc.2022.1017745
Li, D., Xia, L., Huang, P., Wang, Z., Guo, Q., Huang, C., et al. (2023a). Heterogeneity and plasticity of epithelial-mesenchymal transition (EMT) in cancer metastasis: focusing on partial EMT and regulatory mechanisms. Cell Prolif. 56 (6), e13423. doi:10.1111/cpr.13423
Li, J. N., Li, W., Cao, L. Q., Liu, N., and Zhang, K. (2020a). Efficacy of mesenchymal stem cells in the treatment of gastrointestinal malignancies. World J. Gastrointest. Oncol. 12 (4), 365–382. doi:10.4251/wjgo.v12.i4.365
Li, Q., Li, B., Li, Q., Wei, S., He, Z., Huang, X., et al. (2018). Exosomal miR-21-5p derived from gastric cancer promotes peritoneal metastasis via mesothelial-to-mesenchymal transition. Cell death Dis. 9 (9), 854. doi:10.1038/s41419-018-0928-8
Li, S., Dong, R., Kang, Z., Li, H., Wu, X., and Li, T. (2023b). Exosomes: another intercellular lipometabolic communication mediators in digestive system neoplasms? Cytokine and growth factor Rev. 73, 93–100. doi:10.1016/j.cytogfr.2023.06.005
Li, S., Li, J. L., Zhang, H. Y., Zhang, Y., Wang, X. Y., Yang, H. O., et al. (2021). Gastric cancer derived exosomes mediate the delivery of circRNA to promote angiogenesis by targeting miR-29a/VEGF axis in endothelial cells. Biochem. biophysical Res. Commun. 560, 37–44. doi:10.1016/j.bbrc.2021.04.099
Li, Y. J., Wu, J. Y., Wang, J. M., Hu, X. B., Cai, J. X., and Xiang, D. X. (2020b). Gemcitabine loaded autologous exosomes for effective and safe chemotherapy of pancreatic cancer. Acta Biomater. 101, 519–530. doi:10.1016/j.actbio.2019.10.022
Lin, X. M., Wang, Z. J., Lin, Y. X., and Chen, H. (2021). Decreased exosome-delivered miR-486-5p is responsible for the peritoneal metastasis of gastric cancer cells by promoting EMT progress. World J. Surg. Oncol. 19 (1), 312. doi:10.1186/s12957-021-02381-5
Liu, J., Wu, S., Zheng, X., Zheng, P., Fu, Y., Wu, C., et al. (2020). Immune suppressed tumor microenvironment by exosomes derived from gastric cancer cells via modulating immune functions. Sci. Rep. 10 (1), 14749. doi:10.1038/s41598-020-71573-y
Liu, K., Li, G., Fan, C., Diao, Y., Wu, B., and Li, J. (2012). Increased Expression of MicroRNA-221 in gastric cancer and its clinical significance. J. Int. Med. Res. 40 (2), 467–474. doi:10.1177/147323001204000208
Liu, K., Wang, H., Zhou, J., Zhu, S., Ma, M., Xiao, H., et al. (2023). HMGB1 in exosomes derived from gastric cancer cells induces M2-like macrophage polarization by inhibiting the NF-κB signaling pathway. Cell Biol. Int., doi:10.1002/cbin.12110
Lu, C., Xie, L., Qiu, S., Jiang, T., Wang, L., Chen, Z., et al. (2023). Small extracellular vesicles derived from Helicobacter pylori-infected gastric cancer cells induce lymphangiogenesis and lymphatic remodeling via transfer of miR-1246. Small 2023, e2308688. doi:10.1002/smll.202308688
Lu, J., Wang, Y. H., Yoon, C., Huang, X. Y., Xu, Y., Xie, J. W., et al. (2020). Circular RNA circ-RanGAP1 regulates VEGFA expression by targeting miR-877-3p to facilitate gastric cancer invasion and metastasis. Cancer Lett. 471, 38–48. doi:10.1016/j.canlet.2019.11.038
Ma, B., Wang, J., and Yusufu, P. (2023a). Tumor-derived exosome ElNF1-AS1 affects the progression of gastric cancer by promoting M2 polarization of macrophages. Environ. Toxicol. 38, 2228–2239. doi:10.1002/tox.23862
Ma, M., Chen, S., Liu, Z., Xie, H., Deng, H., Shang, S., et al. (2017). miRNA-221 of exosomes originating from bone marrow mesenchymal stem cells promotes oncogenic activity in gastric cancer. OncoTargets Ther. 10, 4161–4171. doi:10.2147/OTT.S143315
Ma, S., Zhou, M., Xu, Y., Gu, X., Zou, M., Abudushalamu, G., et al. (2023b). Clinical application and detection techniques of liquid biopsy in gastric cancer. Mol. cancer 22 (1), 7. doi:10.1186/s12943-023-01715-z
Maharati, A., and Moghbeli, M. (2023). PI3K/AKT signaling pathway as a critical regulator of epithelial-mesenchymal transition in colorectal tumor cells. Cell Commun. Signal. CCS 21 (1), 201. doi:10.1186/s12964-023-01225-x
Majood, M., Rawat, S., and Mohanty, S. (2022). Delineating the role of extracellular vesicles in cancer metastasis: a comprehensive review. Front. Immunol. 13, 966661. doi:10.3389/fimmu.2022.966661
Mao, J., Liang, Z., Zhang, B., Yang, H., Li, X., Fu, H., et al. (2017). UBR2 enriched in p53 deficient mouse bone marrow mesenchymal stem cell-exosome promoted gastric cancer progression via wnt/β-catenin pathway. Stem cells Dayt. Ohio) 35 (11), 2267–2279. doi:10.1002/stem.2702
McKiernan, J., Donovan, M. J., O'Neill, V., Bentink, S., Noerholm, M., Belzer, S., et al. (2016). A novel urine exosome gene expression assay to predict high-grade prostate cancer at initial biopsy. JAMA Oncol. 2 (7), 882–889. doi:10.1001/jamaoncol.2016.0097
Miki, Y., Yashiro, M., Okuno, T., Kitayama, K., Masuda, G., Hirakawa, K., et al. (2018). CD9-positive exosomes from cancer-associated fibroblasts stimulate the migration ability of scirrhous-type gastric cancer cells. Br. J. cancer 118 (6), 867–877. doi:10.1038/bjc.2017.487
Mutsaers, S. E., and Wilkosz, S. (2007). Structure and function of mesothelial cells. Cancer Treat. Res. 134, 1–19. doi:10.1007/978-0-387-48993-3_1
Nakamura, M., Ono, Y. J., Kanemura, M., Tanaka, T., Hayashi, M., Terai, Y., et al. (2015). Hepatocyte growth factor secreted by ovarian cancer cells stimulates peritoneal implantation via the mesothelial-mesenchymal transition of the peritoneum. Gynecol. Oncol. 139 (2), 345–354. doi:10.1016/j.ygyno.2015.08.010
Ning, X. F., Zhang, H. R., Wang, C., and Song, X. Q. (2018). Exosomes released by gastric cancer cells induce transition of pericytes into cancer-associated fibroblasts. Med. Sci. Monit. 24, 2350–2359. doi:10.12659/msm.906641
Nowosad, A., Marine, J. C., and Karras, P. (2023). Perivascular niches: critical hubs in cancer evolution. Trends cancer 9, 897–910. doi:10.1016/j.trecan.2023.06.010
Orditura, M., Galizia, G., Sforza, V., Gambardella, V., Fabozzi, A., Laterza, M. M., et al. (2014). Treatment of gastric cancer. World J. gastroenterology 20 (7), 1635–1649. doi:10.3748/wjg.v20.i7.1635
Palazon, A., Goldrath, A. W., Nizet, V., and Johnson, R. S. (2014). HIF transcription factors, inflammation, and immunity. Immunity 41 (4), 518–528. doi:10.1016/j.immuni.2014.09.008
Pan, L., Liang, W., Fu, M., Huang, Z. H., Li, X., Zhang, W., et al. (2017). Exosomes-mediated transfer of long noncoding RNA ZFAS1 promotes gastric cancer progression. J. cancer Res. Clin. Oncol. 143 (6), 991–1004. doi:10.1007/s00432-017-2361-2
Pascual-Antón, L., Cardeñes, B., Sainz de la Cuesta, R., González-Cortijo, L., López-Cabrera, M., Cabañas, C., et al. (2021). Mesothelial-to-Mesenchymal transition and exosomes in peritoneal metastasis of ovarian cancer. Int. J. Mol. Sci. 22 (21), 11496. doi:10.3390/ijms222111496
Piao, H. Y., Guo, S., Wang, Y., and Zhang, J. (2021). Exosome-transmitted lncRNA PCGEM1 promotes invasive and metastasis in gastric cancer by maintaining the stability of SNAI1. Clin. Transl. Oncol. official Publ. Fed. Span. Oncol. Soc. Natl. Cancer Inst. Mexico 23 (2), 246–256. doi:10.1007/s12094-020-02412-9
Pourali, G., Zafari, N., Fiuji, H., Batra, J., Nazari, E., Khazaei, M., et al. (2023). Extracellular vesicles: emerging mediators of cell communication in gastrointestinal cancers exhibiting metabolic abnormalities. Cytokine and growth factor Rev. 73, 101–113. doi:10.1016/j.cytogfr.2023.08.001
Qi, C., Shi, H., Fan, M., Chen, W., Yao, H., Jiang, C., et al. (2023). Microvesicles from bone marrow-derived mesenchymal stem cells promote Helicobacter pylori-associated gastric cancer progression by transferring thrombospondin-2. Cell Commun. Signal. CCS 21 (1), 274. doi:10.1186/s12964-023-01127-y
Qiu, S., Xie, L., Lu, C., Gu, C., Xia, Y., Lv, J., et al. (2022). Gastric cancer-derived exosomal miR-519a-3p promotes liver metastasis by inducing intrahepatic M2-like macrophage-mediated angiogenesis. J. Exp. Clin. cancer Res. CR 41 (1), 296. doi:10.1186/s13046-022-02499-8
Qu, X., Liu, B., Wang, L., Liu, L., Zhao, W., Liu, C., et al. (2023). Loss of cancer-associated fibroblast-derived exosomal DACT3-AS1 promotes malignant transformation and ferroptosis-mediated oxaliplatin resistance in gastric cancer. Drug Resist. Updat. Rev. Comment. Antimicrob. anticancer Chemother. 68, 100936. doi:10.1016/j.drup.2023.100936
Rynne-Vidal, A., Jiménez-Heffernan, J. A., Fernández-Chacón, C., López-Cabrera, M., and Sandoval, P. (2015). The mesothelial origin of carcinoma associated-fibroblasts in peritoneal metastasis. Cancers 7 (4), 1994–2011. doi:10.3390/cancers7040872
Saari, H., Lázaro-Ibáñez, E., Viitala, T., Vuorimaa-Laukkanen, E., Siljander, P., and Yliperttula, M. (2015). Microvesicle- and exosome-mediated drug delivery enhances the cytotoxicity of Paclitaxel in autologous prostate cancer cells. J. Control. release official J. Control. Release Soc. 220 (Pt B), 727–737. doi:10.1016/j.jconrel.2015.09.031
Sandoval, P., Jiménez-Heffernan, J. A., Rynne-Vidal, Á., Pérez-Lozano, M. L., Gilsanz, Á., Ruiz-Carpio, V., et al. (2013). Carcinoma-associated fibroblasts derive from mesothelial cells via mesothelial-to-mesenchymal transition in peritoneal metastasis. J. pathology 231 (4), 517–531. doi:10.1002/path.4281
Shi, H., Huang, S., Qin, M., Xue, X., Guo, X., Jiang, L., et al. (2021). Exosomal circ_0088300 derived from cancer-associated fibroblasts acts as a miR-1305 sponge and promotes gastric carcinoma cell tumorigenesis. Front. Cell Dev. Biol. 9, 676319. doi:10.3389/fcell.2021.676319
Shi, L., Wang, Z., Geng, X., Zhang, Y., and Xue, Z. (2020). Exosomal miRNA-34 from cancer-associated fibroblasts inhibits growth and invasion of gastric cancer cells in vitro and in vivo. Aging 12 (9), 8549–8564. doi:10.18632/aging.103157
Shibamoto, J., Arita, T., Konishi, H., Kataoka, S., Furuke, H., Takaki, W., et al. (2022). Removal of small extracellular vesicles inhibits the progression of peritoneal dissemination in gastric cancer. Gastric cancer official J. Int. Gastric Cancer Assoc. Jpn. Gastric Cancer Assoc. 25 (4), 712–725. doi:10.1007/s10120-022-01293-x
Song, J., Xu, X. L., He, S. S., Wang, N., Bai, Y. J., Li, B., et al. (2022). Exosomal hsa_circ_0017252 attenuates the development of gastric cancer via inhibiting macrophage M2 polarization. Hum. Cell 35 (5), 1499–1511. doi:10.1007/s13577-022-00739-9
Su, H., Ren, W., and Zhang, D. (2023). Research progress on exosomal proteins as diagnostic markers of gastric cancer (review article). Clin. Exp. Med. 23 (2), 203–218. doi:10.1007/s10238-022-00793-5
Sung, H., Ferlay, J., Siegel, R. L., Laversanne, M., Soerjomataram, I., Jemal, A., et al. (2021). Global cancer statistics 2020: GLOBOCAN estimates of incidence and mortality worldwide for 36 cancers in 185 countries. CA Cancer J. Clin. 71 (3), 209–249. doi:10.3322/caac.21660
Tang, D., Liu, S., Shen, H., Deng, G., and Zeng, S. (2022). Extracellular vesicles promote the formation of pre-metastasis niche in gastric cancer. Front. Immunol. 13, 813015. doi:10.3389/fimmu.2022.813015
Urabe, F., Patil, K., Ramm, G. A., Ochiya, T., and Soekmadji, C. (2021). Extracellular vesicles in the development of organ-specific metastasis. J. Extracell. vesicles 10 (9), e12125. doi:10.1002/jev2.12125
Wang, J. Y., Guan, X. W., Zhang, Y., Ge, S. H., Zhang, L., Li, H. L., et al. (2018). Exosomal miR-27a derived from gastric cancer cells regulates the transformation of fibroblasts into cancer-associated fibroblasts. Cell. Physiology Biochem. 49 (3), 869–883. doi:10.1159/000493218
Wang, L., Bo, X., Yi, X., Xiao, X., Zheng, Q., Ma, L., et al. (2020). Exosome-transferred LINC01559 promotes the progression of gastric cancer via PI3K/AKT signaling pathway. Cell death Dis. 11 (9), 723. doi:10.1038/s41419-020-02810-5
Wang, M., Cai, W., Yang, A. J., Wang, C. Y., Zhang, C. L., Liu, W., et al. (2022b). Gastric cancer cell-derived extracellular vesicles disrupt endothelial integrity and promote metastasis. Cancer Lett. 545, 215827. doi:10.1016/j.canlet.2022.215827
Wang, M., Zhao, C., Shi, H., Zhang, B., Zhang, L., Zhang, X., et al. (2014). Deregulated microRNAs in gastric cancer tissue-derived mesenchymal stem cells: novel biomarkers and a mechanism for gastric cancer. Br. J. cancer 110 (5), 1199–1210. doi:10.1038/bjc.2014.14
Wang, X., Huang, D., Wu, J., Li, Z., Yi, X., and Zhong, T. (2022a). The biological effect of small extracellular vesicles on colorectal cancer metastasis. Cells 11 (24), 4071. doi:10.3390/cells11244071
Wang, Y., Wang, H. T., Zheng, R. Q., Wu, P. P., Sun, Z. X., Chen, J. Y., et al. (2021b). Circular RNA ITCH suppresses metastasis of gastric cancer via regulating miR-199a-5p/Klotho axis. Cell cycleGeorget. Tex) 20 (5-6), 522–536. doi:10.1080/15384101.2021.1878327
Wang, Y. S., Shang, K., Zhang, N. G., Zhao, J., and Cao, B. W. (2021a). Tumor-associated macrophage-derived exosomes promote the progression of gastric cancer by regulating the P38MAPK signaling pathway and the immune checkpoint PD-L1. Cancer Biotherapy Radiopharm., doi:10.1089/cbr.2021.0218
Wang, Z., Liu, M., Zhu, H., Zhang, W., He, S., Hu, C., et al. (2013). miR-106a is frequently upregulated in gastric cancer and inhibits the extrinsic apoptotic pathway by targeting FAS. Mol. Carcinog. 52 (8), 634–646. doi:10.1002/mc.21899
Wolf, P. (1967). The nature and significance of platelet products in human plasma. Br. J. Haematol. 13 (3), 269–288. doi:10.1111/j.1365-2141.1967.tb08741.x
Wu, H., Fu, M., Liu, J., Chong, W., Fang, Z., Du, F., et al. (2021). The role and application of small extracellular vesicles in gastric cancer. Mol. cancer 20 (1), 71. doi:10.1186/s12943-021-01365-z
Xia, X., Wang, S., Ni, B., Xing, S., Cao, H., Zhang, Z., et al. (2020). Hypoxic gastric cancer-derived exosomes promote progression and metastasis via MiR-301a-3p/PHD3/HIF-1α positive feedback loop. Oncogene 39 (39), 6231–6244. doi:10.1038/s41388-020-01425-6
Xie, M., Yu, T., Jing, X., Ma, L., Fan, Y., Yang, F., et al. (2020). Exosomal circSHKBP1 promotes gastric cancer progression via regulating the miR-582-3p/HUR/VEGF axis and suppressing HSP90 degradation. Mol. cancer 19 (1), 112. doi:10.1186/s12943-020-01208-3
Xing, R., Cui, J. T., Xia, N., and Lu, Y. Y. (2015). GKN1 inhibits cell invasion in gastric cancer by inactivating the NF-kappaB pathway. Discov. Med. 19 (103), 65–71.
Xu, G. F., Zhang, B., Ye, J. H., Cao, S. L., Shi, J. J., Zhao, Y., et al. (2019). Exosomal miRNA-139 in cancer-associated fibroblasts inhibits gastric cancer progression by repressing MMP11 expression. Int. J. Biol. Sci. 15 (11), 2320–2329. doi:10.7150/ijbs.33750
Yáñez-Mó, M., Lara-Pezzi, E., Selgas, R., Ramírez-Huesca, M., Domínguez-Jiménez, C., Jiménez-Heffernan, J. A., et al. (2003). Peritoneal dialysis and epithelial-to-mesenchymal transition of mesothelial cells. N. Engl. J. Med. 348 (5), 403–413. doi:10.1056/NEJMoa020809
Yang, H., Fu, H., Wang, B., Zhang, X., Mao, J., Li, X., et al. (2018). Exosomal miR-423-5p targets SUFU to promote cancer growth and metastasis and serves as a novel marker for gastric cancer. Mol. Carcinog. 57 (9), 1223–1236. doi:10.1002/mc.22838
Yang, P., Yang, W., Wei, Z., Li, Y., Yang, Y., and Wang, J. (2023). Novel targets for gastric cancer: the tumor microenvironment (TME), N6-methyladenosine (m6A), pyroptosis, autophagy, ferroptosis and cuproptosis. Biomed. Pharmacother. = Biomedecine Pharmacother. 163, 114883. doi:10.1016/j.biopha.2023.114883
Yao, X., and Zeng, Y. (2023). Tumour associated endothelial cells: origin, characteristics and role in metastasis and anti-angiogenic resistance. Front. physiology 14, 1199225. doi:10.3389/fphys.2023.1199225
Yen, E. Y., Miaw, S. C., Yu, J. S., and Lai, I. R. (2017). Exosomal TGF-β1 is correlated with lymphatic metastasis of gastric cancers. Am. J. cancer Res. 7 (11), 2199–2208.
Yi, Q., Yue, J., Liu, Y., Shi, H., Sun, W., Feng, J., et al. (2023). Recent advances of exosomal circRNAs in cancer and their potential clinical applications. J. Transl. Med. 21 (1), 516. doi:10.1186/s12967-023-04348-4
Yoon, J. H., Ashktorab, H., Smoot, D. T., Nam, S. W., Hur, H., and Park, W. S. (2020). Uptake and tumor-suppressive pathways of exosome-associated GKN1 protein in gastric epithelial cells. Gastric cancer official J. Int. Gastric Cancer Assoc. Jpn. Gastric Cancer Assoc. 23 (5), 848–862. doi:10.1007/s10120-020-01068-2
Yoon, J. H., Choi, W. S., Kim, O., and Park, W. S. (2014). The role of gastrokine 1 in gastric cancer. J. gastric cancer 14 (3), 147–155. doi:10.5230/jgc.2014.14.3.147
Yoon, J. H., Ham, I. H., Kim, O., Ashktorab, H., Smoot, D. T., Nam, S. W., et al. (2018). Gastrokine 1 protein is a potential theragnostic target for gastric cancer. Gastric cancer official J. Int. Gastric Cancer Assoc. Jpn. Gastric Cancer Assoc. 21 (6), 956–967. doi:10.1007/s10120-018-0828-8
You, B., Jin, C., Zhang, J., Xu, M., Xu, W., Sun, Z., et al. (2022). MSC-derived extracellular vesicle-delivered L-PGDS inhibit gastric cancer progression by suppressing cancer cell stemness and STAT3 phosphorylation. Stem cells Int. 2022, 9668239. doi:10.1155/2022/9668239
Yu, L., Fan, G., Wang, Q., Zhu, Y., Zhu, H., Chang, J., et al. (2023). In vivo self-assembly and delivery of VEGFR2 siRNA-encapsulated small extracellular vesicles for lung metastatic osteosarcoma therapy. Cell death Dis. 14 (9), 626. doi:10.1038/s41419-023-06159-3
Zeng, W., Wen, Z., Chen, H., and Duan, Y. (2023). Exosomes as carriers for drug delivery in cancer therapy. Pharm. Res. 40 (4), 873–887. doi:10.1007/s11095-022-03224-y
Zhang, F., Guo, C., Cao, X., Yan, Y., Zhang, J., and Lv, S. (2022a). Gastric cancer cell-derived extracellular vesicles elevate E2F7 expression and activate the MAPK/ERK signaling to promote peritoneal metastasis through the delivery of SNHG12. Cell death Discov. 8 (1), 164. doi:10.1038/s41420-022-00925-6
Zhang, H., Bai, M., Deng, T., Liu, R., Wang, X., Qu, Y., et al. (2016). Cell-derived microvesicles mediate the delivery of miR-29a/c to suppress angiogenesis in gastric carcinoma. Cancer Lett. 375 (2), 331–339. doi:10.1016/j.canlet.2016.03.026
Zhang, H., Yang, M., Wu, X., Li, Q., Li, X., Zhao, Y., et al. (2021b). The distinct roles of exosomes in tumor-stroma crosstalk within gastric tumor microenvironment. Pharmacol. Res. 171, 105785. doi:10.1016/j.phrs.2021.105785
Zhang, H. Y., Wang, Y., Bai, M., Wang, J. Y., Zhu, K. G., Liu, R., et al. (2018b). Exosomes serve as nanoparticles to suppress tumor growth and angiogenesis in gastric cancer by delivering hepatocyte growth factor siRNA. Cancer Sci. 109 (3), 629–641. doi:10.1111/cas.13488
Zhang, J., Wu, J., Wang, G., He, L., Zheng, Z., Wu, M., et al. (2023b). Extracellular vesicles: techniques and biomedical applications related to single vesicle analysis. ACS Nano 17 (18), 17668–17698. doi:10.1021/acsnano.3c03172
Zhang, X., Shi, H., Yuan, X., Jiang, P., Qian, H., and Xu, W. (2018a). Tumor-derived exosomes induce N2 polarization of neutrophils to promote gastric cancer cell migration. Mol. cancer 17 (1), 146. doi:10.1186/s12943-018-0898-6
Zhang, Y., Bi, J., Huang, J., Tang, Y., Du, S., and Li, P. (2020). Exosome: a review of its classification, isolation techniques, storage, diagnostic and targeted therapy applications. Int. J. nanomedicine 15, 6917–6934. doi:10.2147/IJN.S264498
Zhang, Y., Chen, L., Ye, X., Wu, Z., Zhang, Z., Sun, B., et al. (2021a). Expression and mechanism of exosome-mediated A FOXM1 related long noncoding RNA in gastric cancer. J. nanobiotechnology 19 (1), 133. doi:10.1186/s12951-021-00873-w
Zhang, Y., Guo, S., Mao, T., Guo, J., Zhang, Q., Tian, Z., et al. (2023a). Tumor-derived exosomal LINC01480 upregulates VCAM1 expression by acting as a competitive endogenous RNA of miR-204-5p to promote gastric cancer progression. ACS biomaterials Sci. Eng. 10, 550–562. doi:10.1021/acsbiomaterials.3c00394
Zhang, Z., Sun, C. Y., Zheng, Y., and Gong, Y. Y. (2022b). circFCHO2 promotes gastric cancer progression by activating the JAK1/STAT3 pathway via sponging miR-194-5p. Cell cycleGeorget. Tex) 21 (20), 2145–2164. doi:10.1080/15384101.2022.2087280
Zhang, Z., Wu, H., Chong, W., Shang, L., Jing, C., and Li, L. (2022c). Liquid biopsy in gastric cancer: predictive and prognostic biomarkers. Cell death Dis. 13 (10), 903. doi:10.1038/s41419-022-05350-2
Zhao, L. X., Zhang, K., Shen, B. B., and Li, J. N. (2021). Mesenchymal stem cell-derived exosomes for gastrointestinal cancer. World J. Gastrointest. Oncol. 13 (12), 1981–1996. doi:10.4251/wjgo.v13.i12.1981
Zhao, W., Zhang, Y., Zhang, W., Sun, Y., Zheng, B., Wang, J., et al. (2023). Exosomal LINC00355 promotes the malignant progression of gastric cancer through histone deacetylase HDAC3-mediated TP53INP1 transcriptional inhibition. Life Sci. 315, 121387. doi:10.1016/j.lfs.2023.121387
Zheng, P., Luo, Q., Wang, W., Li, J., Wang, T., Wang, P., et al. (2018). Tumor-associated macrophages-derived exosomes promote the migration of gastric cancer cells by transfer of functional Apolipoprotein E. Cell death Dis. 9 (4), 434. doi:10.1038/s41419-018-0465-5
Zheng, P. M., Gao, H. J., Li, J. M., Zhang, P., and Li, G. (2020). Effect of exosome-derived miR-223 from macrophages on the metastasis of gastric cancer cells. Zhonghua yi xue za zhi 100 (22), 1750–1755. doi:10.3760/cma.j.cn112137-20200425-01309
Zhu, J. X., Du, S. S., Zhang, J. F., Huang, G. Z., Dong, L. J., Ren, E. B., et al. (2022d). microRNA-10a-5p from gastric cancer cell-derived exosomes enhances viability and migration of human umbilical vein endothelial cells by targeting zinc finger MYND-type containing 11. Bioengineered 13 (1), 496–507. doi:10.1080/21655979.2021.2009962
Zhu, L. Y., Zhang, S. S., Chen, S. D., Wu, H. J., Jiang, M. J., and Liu, A. Q. (2022b). Exosomal miR-552-5p promotes tumorigenesis and disease progression via the PTEN/TOB1 axis in gastric cancer. J. Cancer 13 (3), 890–905. doi:10.7150/jca.66903
Zhu, M., Zhang, N., He, S., and Lu, X. (2020). Exosomal miR-106a derived from gastric cancer promotes peritoneal metastasis via direct regulation of Smad7. Cell cycleGeorget. Tex 19 (10), 1200–1221. doi:10.1080/15384101.2020.1749467
Zhu, M., Zhang, N., Ma, J., and He, S. (2022c). Integration of exosomal miR-106a and mesothelial cells facilitates gastric cancer peritoneal dissemination. Cell. Signal. 91, 110230. doi:10.1016/j.cellsig.2021.110230
Zhu, T. Y., Hu, Z. H., Wang, Z. Y., Ding, H. X., Li, R. X., Wang, J. T., et al. (2022a). microRNA-301b-3p from mesenchymal stem cells-derived extracellular vesicles inhibits TXNIP to promote multidrug resistance of gastric cancer cells. Cell Biol. Toxicol. 39, 1923–1937. doi:10.1007/s10565-021-09675-0
Keywords: gastric cancer, extracellular vesicles, metastasis, tumor microenvironment, epithelial-mesenchymal transition
Citation: Lei Y, Cai S, Zhang C-D and Li Y-S (2024) The biological role of extracellular vesicles in gastric cancer metastasis. Front. Cell Dev. Biol. 12:1323348. doi: 10.3389/fcell.2024.1323348
Received: 20 October 2023; Accepted: 15 January 2024;
Published: 25 January 2024.
Edited by:
Anthoula Lazaris, McGill University Health Center, CanadaReviewed by:
Luciana N. S. Andrade, University of São Paulo, BrazilCopyright © 2024 Lei, Cai, Zhang and Li. This is an open-access article distributed under the terms of the Creative Commons Attribution License (CC BY). The use, distribution or reproduction in other forums is permitted, provided the original author(s) and the copyright owner(s) are credited and that the original publication in this journal is cited, in accordance with accepted academic practice. No use, distribution or reproduction is permitted which does not comply with these terms.
*Correspondence: Yong-Shuang Li, bGl5b25nc2h1YW5nQGNtdS5lZHUuY24=
†These authors share first authorship
Disclaimer: All claims expressed in this article are solely those of the authors and do not necessarily represent those of their affiliated organizations, or those of the publisher, the editors and the reviewers. Any product that may be evaluated in this article or claim that may be made by its manufacturer is not guaranteed or endorsed by the publisher.
Research integrity at Frontiers
Learn more about the work of our research integrity team to safeguard the quality of each article we publish.