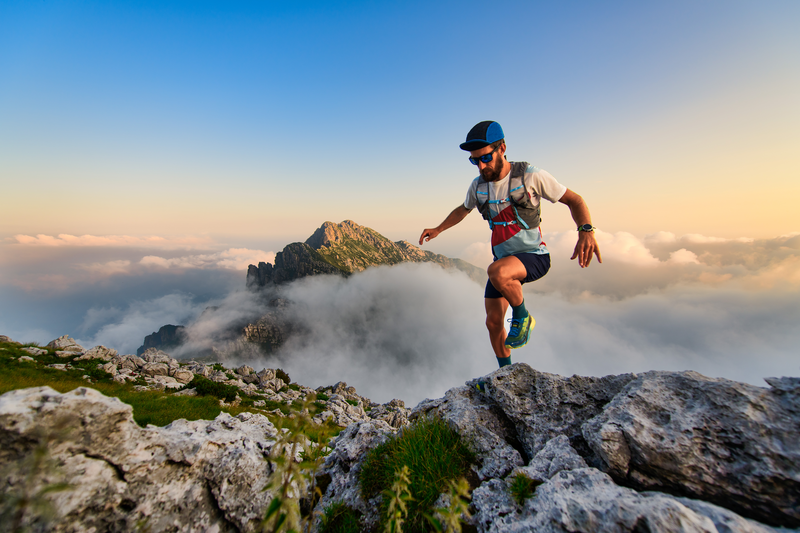
95% of researchers rate our articles as excellent or good
Learn more about the work of our research integrity team to safeguard the quality of each article we publish.
Find out more
EDITORIAL article
Front. Cell Dev. Biol. , 08 January 2024
Sec. Cell Growth and Division
Volume 11 - 2023 | https://doi.org/10.3389/fcell.2023.1359465
This article is part of the Research Topic Cytoskeletal Alterations in Aging and Disease View all 9 articles
Editorial on the Research Topic
Cytoskeletal alterations in aging and disease
The cytoskeleton of eukaryotic cells consists of microtubules, actin and intermediate filaments, which are highly interconnected and compartmentalized in polarized cells, such as neurons (Iwanski and Kapitein; Kevenaar and Hoogenraad, 2015; Leterrier et al., 2017). In particular, these filaments are much more than just pure structural elements and ensure various additional functions critical for neuronal development and maintenance (Iwanski and Kapitein; Dent and Baas, 2014; Coles and Bradke, 2015; Yuan et al., 2017). Not surprisingly, dysfunction of any of these components can cause neurodegenerative diseases, such as Alzheimer’s, Charcot-Marie-Tooth disease (CMT) or Hereditary spastic Paraplegia (HSP) (Martnez-Hernandez et al.; Morris and Brady; Bomont et al.; Costa and Sousa; Matamoros and Baas, 2016).
Microtubules, which are rod-like, polarized structures assembled from alpha/beta-tubulin dimers form the basis for organelle transport and modulate cell shape and behavior (Conde and Caceres, 2009; Guedes-Dias and Holzbaur, 2019). Interestingly, microtubules can be highly heterogeneous across cellular and subcellular compartments due to several factors: 1) cell-type specific expression of tubulin genes; 2) locally active enzymes catalyzing posttranslational modifications (PTMs); and 3) various flavors of Microtubule-Associated Proteins (MAPs) (Janke and Bulinski, 2011; Roll-Mecak, 2020; McKenna et al., 2023). However, how these different factors assemble and segregate in developing and mature neurons to drive specialized microtubule functions remains elusive (Chakraborti et al., 2016; Bodakuntla et al., 2021; Moutin et al., 2021; Atkins et al., 2023; Pero et al., 2023).
Recent innovative strategies to label or image specific microtubule subpopulations with high resolution led to novel concepts suggesting that the tubulin and MAP codes can cooperatively and locally drive microtubule lattice compaction or expansion, renewal, and rescue to tune microtubule structure and dynamics (Iwanski and Kapitein; Janke and Magiera, 2020). In addition, all cytoskeleton elements and associated proteins are heavily modified through PTMs, many of which are associated with neuronal development and degeneration. However, key questions remain: Do PTMs and MAPs have functional roles in driving the neuronal states, or are they simply indicators to reflect signaling changes associated with these different states, or both? To start answering these questions, three articles in this Research Topic illustrate the role of tubulin PTM crosstalk in AD progression (Martinez-Hernandez et al.), the impact of a major AD-related MAP, Tau, and its phosphorylation on axonal growth (Morris and Brady), and the effect of neurofilaments and their PTMs in neuronal homeostasis and neurodegenerative diseases (Kotaich et al.).
Martnez-Hernandez et al. showed that PTMs, such as tubulin acetylation and tyrosination/detyrosination, influenced each other and could affect AD progression by altering microtubule dynamics. Morris and Brady showed that site-specific phosphorylation of tau may lead to tau conformational changes beneficial for normal neurite development. Both papers illustrated the unconventional roles of cytoskeletal PTMs that depend on neuronal states: tubulin PTMs, which are critical in normal development, can exacerbate AD progression when misregulated, while selective tau phosphorylation, which is indicated in AD, may promote axonal growth when physiologically regulated. These intriguing results are important for understanding neuronal cytoskeleton in health, disease and aging through multiple lenses focusing on different neuronal states. However, the underlying molecular mechanisms require further studies so that we may know how to regulate these PTMs, which can be a double-edged sword for neurons. In this context, the review by Kotaich et al. pointed out that the dynamicity of neurofilaments, which is based on their fine-tuned assembly/transport/degradation to sustain key structural and electrophysiological properties of the neurons, is critically influenced by PTMs (Yuan et al., 2017; Yuan and Nixon, 2023). These data shed new perspectives on the etiology and treatment of neurofilament-related neurodegenerative disorders (e.g., CMT or amyotrophic lateral sclerosis, (Rao and Nixon, 2003; Perrot and Eyer, 2009; Didonna and Opal, 2019; Laura et al., 2019; Stone et al., 2021).
Importantly, neurofilaments were shown to influence microtubule dynamics in neurons (Yadav et al., 2016). Such crosstalk may be established and regulated by PTMs and MAPs, leading to fine-tuning of neuronal morphology, cytoarchitecture, and physiology. This proposed mechanism may provide an additional step in untangling the complexity behind the cytoskeleton-mediated regulation of neuronal homeostasis and its contribution to aging and diseases. Following this idea, determining how cytoskeleton composition and axon morphology change during aging would be very informative (Kounakis and Tavernarakis, 2019; Kim et al., 2022). Interestingly, by examining sensory axons from healthy human skin biopsies, Metzner et al. reported increases in cytoskeleton composition in both sexes and larger axonal caliber in male during aging. They proposed that such changes may modify axonal function, thus, contributing to aging-related decrease in sensory perception or increased susceptibility to degeneration.
Another emerging theme from several articles concerns the key physiological roles of cytoskeleton-associated proteins incriminated in neurodegeneration or other aging-related disorders, such as the microtubule-regulatory proteins Gigaxonin (peripheral neuropathies; Kotaich et al.; Arribat et al., 2019), Tau (AD; Morris and Brady; Li et al., 2014), Spastin (HSP; Costa and Sousa; Yu et al., 2008; Brill et al., 2016; Jardin et al., 2018), or the F-actin binding protein Radaxin (Hearing loss; Hausrat et al.; Paglini et al., 1998) during neuronal development. This is particularly emphasized in the review from Costa and Sousa, summarizing the numerous studies demonstrating that tight control of microtubule dynamics and membrane trafficking by Spastin determined several key steps in neuronal circuit wiring (e.g., axonal outgrowth/branching, synapse elimination/maintenance). The authors further highlighted that fine tuning of Spastin expression and activity through post-transcriptional/-translational modifications and MAPs, is fundamental for both axonal development and homeostasis.
Altogether, these studies suggest cytoskeleton alterations as a major continuum between neuronal circuit development and dysfunction/degeneration and left us with open questions: How do mutations or PTMs in cytoskeleton-associated proteins with key developmental functions lead to late-onset neurodegeneration or aging-related disorders? Does a developmental component exist for these diseases? And if not, what molecular mechanisms compensate for their dysfunctions in neuronal development, and how are they lost during aging? Answering these questions will deepen our understanding of fundamental neurobiology and generate innovative therapies for neurodegenerative and other aging-related diseases.
MB: Writing–original draft, Writing–review and editing. CF: Writing–original draft, Writing–review and editing. YS: Writing–original draft, Writing–review and editing.
The author(s) declare financial support was received for the research, authorship, and/or publication of this article. A DFG research grant (project number: 450131873), the DGM foundation (no. Le3/1) and the DFG-funded Excellence Cluster SyNergy (EXC 2145—ID 390857198) to MSB. The ANR grant (ANR-20-CE16-0019), the Association Strümpell-Lorrain (ASL)-HSP France grants (AO 2019, AO 2022), the Tom Wahlig Foundation grant (2019) and the Association Française contre les Myopathies research grant (Grant 23695) to CF. NIH grants (P30AG062421 Developmental Project and AG072516), a Jack Satter Foundation grant, a Pape Adams ALS Transformative Scholar Award, and an AARG grant from the Alzheimer’s Association to YS.
The authors declare that the research was conducted in the absence of any commercial or financial relationships that could be construed as a potential conflict of interest.
All claims expressed in this article are solely those of the authors and do not necessarily represent those of their affiliated organizations, or those of the publisher, the editors and the reviewers. Any product that may be evaluated in this article, or claim that may be made by its manufacturer, is not guaranteed or endorsed by the publisher.
Arribat, Y., Mysiak, K. S., Lescouzeres, L., Boizot, A., Ruiz, M., Rossel, M., et al. (2019). Sonic Hedgehog repression underlies gigaxonin mutation-induced motor deficits in giant axonal neuropathy. J. Clin. Investig. 129 (12), 5312–5326. doi:10.1172/JCI129788
Atkins, M., Nicol, X., and Fassier, C. (2023). Microtubule remodelling as a driving force of axon guidance and pruning. Semin. Cell. Dev. Biol. 140, 35–53. doi:10.1016/j.semcdb.2022.05.030
Bodakuntla, S., Janke, C., and Magiera, M. M. (2021). Tubulin polyglutamylation, a regulator of microtubule functions, can cause neurodegeneration. Neurosci. Lett. 746, 135656. doi:10.1016/j.neulet.2021.135656
Brill, M. S., Kleele, T., Ruschkies, L., Wang, M., Marahori, N. A., Reuter, M. S., et al. (2016). Branch-specific microtubule destabilization mediates axon branch loss during neuromuscular synapse elimination. Neuron 92 (4), 845–856. doi:10.1016/j.neuron.2016.09.049
Chakraborti, S., Natarajan, K., Curiel, J., Janke, C., and Liu, J. (2016). The emerging role of the tubulin code: from the tubulin molecule to neuronal function and disease. Cytoskelet. Hob. 73 (10), 521–550. doi:10.1002/cm.21290
Coles, C. H., and Bradke, F. (2015). Coordinating neuronal actin-microtubule dynamics. Curr. Biol. 25 (15), R677–R691. doi:10.1016/j.cub.2015.06.020
Conde, C., and Caceres, A. (2009). Microtubule assembly, organization and dynamics in axons and dendrites. Nat. Rev. Neurosci. 10 (5), 319–332. doi:10.1038/nrn2631
Dent, E. W., and Baas, P. W. (2014). Microtubules in neurons as information carriers. J. Neurochem. 129 (2), 235–239. doi:10.1111/jnc.12621
Didonna, A., and Opal, P. (2019). The role of neurofilament aggregation in neurodegeneration: lessons from rare inherited neurological disorders. Mol. Neurodegener. 14 (1), 19. doi:10.1186/s13024-019-0318-4
Guedes-Dias, P., and Holzbaur, E. L. F. (2019). Axonal transport: driving synaptic function. Science 366 (6462), eaaw9997. doi:10.1126/science.aaw9997
Janke, C., and Bulinski, J. C. (2011). Post-translational regulation of the microtubule cytoskeleton: mechanisms and functions. Nat. Rev. Mol. Cell. Biol. 12 (12), 773–786. doi:10.1038/nrm3227
Janke, C., and Magiera, M. M. (2020). The tubulin code and its role in controlling microtubule properties and functions. Nat. Rev. Mol. Cell. Biol. 21 (6), 307–326. doi:10.1038/s41580-020-0214-3
Jardin, N., Giudicelli, F., Ten Martin, D., Vitrac, A., De Gois, S., Allison, R., et al. (2018). BMP- and neuropilin 1-mediated motor axon navigation relies on spastin alternative translation. Development 145 (17), dev162701. doi:10.1242/dev.162701
Kevenaar, J. T., and Hoogenraad, C. C. (2015). The axonal cytoskeleton: from organization to function. Front. Mol. Neurosci. 8, 44. doi:10.3389/fnmol.2015.00044
Kim, Y. J., Cho, M. J., Yu, W. D., Kim, M. J., Kim, S. Y., and Lee, J. H. (2022). Links of cytoskeletal integrity with disease and aging. Cells 11 (18), 2896. doi:10.3390/cells11182896
Kounakis, K., and Tavernarakis, N. (2019). The cytoskeleton as a modulator of aging and neurodegeneration. Adv. Exp. Med. Biol. 1178, 227–245. doi:10.1007/978-3-030-25650-0_12
Laura, M., Pipis, M., Rossor, A. M., and Reilly, M. M. (2019). Charcot-Marie-Tooth disease and related disorders: an evolving landscape. Curr. Opin. Neurol. 32 (5), 641–650. doi:10.1097/WCO.0000000000000735
Leterrier, C., Dubey, P., and Roy, S. (2017). The nano-architecture of the axonal cytoskeleton. Nat. Rev. Neurosci. 18 (12), 713–726. doi:10.1038/nrn.2017.129
Matamoros, A. J., and Baas, P. W. (2016). Microtubules in health and degenerative disease of the nervous system. Brain Res. Bull. 126 (3), 217–225. doi:10.1016/j.brainresbull.2016.06.016
McKenna, E. D., Sarbanes, S. L., Cummings, S. W., and Roll-Mecak, A. (2023). The tubulin code, from molecules to health and disease. Annu. Rev. Cell. Dev. Biol. 39, 331–361. doi:10.1146/annurev-cellbio-030123-032748
Moutin, M. J., Bosc, C., Peris, L., and Andrieux, A. (2021). Tubulin post-translational modifications control neuronal development and functions. Dev. Neurobiol. 81 (3), 253–272. doi:10.1002/dneu.22774
Paglini, G., Kunda, P., Quiroga, S., Kosik, K., and Caceres, A. (1998). Suppression of radixin and moesin alters growth cone morphology, motility, and process formation in primary cultured neurons. J. Cell. Biol. 143 (2), 443–455. doi:10.1083/jcb.143.2.443
Pero, M. E., Chowdhury, F., and Bartolini, F. (2023). Role of tubulin post-translational modifications in peripheral neuropathy. Exp. Neurol. 360, 114274. doi:10.1016/j.expneurol.2022.114274
Perrot, R., and Eyer, J. (2009). Neuronal intermediate filaments and neurodegenerative disorders. Brain Res. Bull. 80 (4-5), 282–295. doi:10.1016/j.brainresbull.2009.06.004
Rao, M. V., and Nixon, R. A. (2003). Defective neurofilament transport in mouse models of amyotrophic lateral sclerosis: a review. Neurochem. Res. 28 (7), 1041–1047. doi:10.1023/a:1023259207015
Roll-Mecak, A. (2020). The tubulin code in microtubule dynamics and information encoding. Dev. Cell. 54 (1), 7–20. doi:10.1016/j.devcel.2020.06.008
Stone, E. J., Kolb, S. J., and Brown, A. (2021). A review and analysis of the clinical literature on Charcot-Marie-Tooth disease caused by mutations in neurofilament protein L. Cytoskelet. Hob. 78 (3), 97–110. doi:10.1002/cm.21676
Yadav, P., Selvaraj, B. T., Bender, F. L., Behringer, M., Moradi, M., Sivadasan, R., et al. (2016). Neurofilament depletion improves microtubule dynamics via modulation of Stat3/stathmin signaling. Acta Neuropathol. 132 (1), 93–110. doi:10.1007/s00401-016-1564-y
Yu, W., Qiang, L., Solowska, J. M., Karabay, A., Korulu, S., and Baas, P. W. (2008). The microtubule-severing proteins spastin and katanin participate differently in the formation of axonal branches. Mol. Biol. Cell. 19 (4), 1485–1498. doi:10.1091/mbc.e07-09-0878
Yuan, A., and Nixon, R. A. (2023). Posttranscriptional regulation of neurofilament proteins and tau in health and disease. Brain Res. Bull. 192, 115–127. doi:10.1016/j.brainresbull.2022.10.017
Keywords: microtubule, neurofilament (NF), microtubule associated proteins, cytoskeleton, actin, aging, disease, posttranslation modification
Citation: Brill MS, Fassier C and Song Y (2024) Editorial: Cytoskeletal alterations in aging and disease. Front. Cell Dev. Biol. 11:1359465. doi: 10.3389/fcell.2023.1359465
Received: 21 December 2023; Accepted: 22 December 2023;
Published: 08 January 2024.
Edited and reviewed by:
Philipp Kaldis, Lund University, SwedenCopyright © 2024 Brill, Fassier and Song. This is an open-access article distributed under the terms of the Creative Commons Attribution License (CC BY). The use, distribution or reproduction in other forums is permitted, provided the original author(s) and the copyright owner(s) are credited and that the original publication in this journal is cited, in accordance with accepted academic practice. No use, distribution or reproduction is permitted which does not comply with these terms.
*Correspondence: Monika S. Brill, bW9uaWthLmxlaXNjaG5lci1icmlsbEB0dW0uZGU=; Coralie Fassier, Y29yYWxpZS5mYXNzaWVyQGluc2VybS5mcg==; Yuyu Song, eXNvbmcxM0BtZ2guaGFydmFyZC5lZHU=
†These authors have contributed equally to this work
‡ORCID: Monika S. Brill, https://orcid.org/0000-0001-5422-9175; Coralie Fassier, https://orcid.org/0000-0003-3015-4281; Yuyu Song, https://orcid.org/0000-0002-9196-1610
Disclaimer: All claims expressed in this article are solely those of the authors and do not necessarily represent those of their affiliated organizations, or those of the publisher, the editors and the reviewers. Any product that may be evaluated in this article or claim that may be made by its manufacturer is not guaranteed or endorsed by the publisher.
Research integrity at Frontiers
Learn more about the work of our research integrity team to safeguard the quality of each article we publish.