- 1Department of Otorhinolaryngology, The Second Affiliated Hospital, Hengyang Medical School, University of South China, Hengyang, China
- 2ENT Institute and Department of Otorhinolaryngology, Eye and ENT Hospital, State Key Laboratory of Medical Neurobiology and MOE Frontiers Center for Brain Science, Fudan University, Shanghai, China
- 3Institutes of Biomedical Sciences, Fudan University, Shanghai, China
- 4NHC Key Laboratory of Hearing Medicine, Fudan University, Shanghai, China
Aminoglycosides are commonly used for the treatment of life-threatening bacterial infections, however, aminoglycosides may cause irreversible hearing loss with a long-term clinical therapy. The mechanism and prevention of the ototoxicity of aminoglycosides are still limited although amounts of studies explored widely. Specifically, advancements in programmed cell death (PCD) provide more new perspectives. This review summarizes the general signal pathways in programmed cell death, including apoptosis, autophagy, and ferroptosis, as well as the mechanisms of aminoglycoside-induced ototoxicity. Additionally, novel interventions, especially gene therapy strategies, are also investigated for the prevention or treatment of aminoglycoside-induced hearing loss with prospective clinical applications.
1 Introduction
Hearing loss (HL) is the most common sensory impairment in human beings. Almost 466 million people worldwide currently suffer from HL, and this figure is estimated over 900 million by 2050 (Olusanya et al., 2019). Aging, noise, infections, genetic defects, and long-term use of ototoxic drugs are the main causes of HL. Up to now, more than 150 ototoxic drugs have been documented, resulting in functional impairment and/or cellular degeneration in the inner ear (Tanaka et al., 2019). Aminoglycosides are one of the most common anti-inflammatory therapies used for Gram-negative bacteria in clinical practice, they are often selected as the first-line agents to treat suspected or confirmed severe acute infections (Pogue et al., 2020), cystic fibrosis (Smyth and Bhatt, 2014), and multidrug-resistant tuberculosis (Dillard et al., 2021) owing to their low cost and high efficacy. Despite various types of aminoglycosides are widely used in the clinic, including streptomycin, gentamicin, amikacin, neomycin, and kanamycin, there is a high risk of ototoxicity contributing to the degeneration of auditory cells in the inner ear. A growing body of evidence indicates that the loss of sensory hair cell (HC)s is the main cochlear pathology underlying drug-induced hearing loss, and hair cells are special non-regenerative cells in the inner ear (Shu et al., 2019), converting sound-induced vibrations into electrochemical signals. Damage or loss of hair cells leads to permanent hearing impairment (Fettiplace and Hackney, 2006). Aminoglycoside-induced hearing loss is typically cumulatively dose-dependent. Clinical studies have shown that 20%–47% of patients suffered from hearing loss due to the side effects of aminoglycoside-induced ototoxicity. In general, aminoglycosides primarily induce high-frequency hearing loss above 8,000 Hz, and gradually affect hearing loss at low-frequency with the time past (Wu et al., 2001; Crundwell et al., 2016; Sacks et al., 2018). Although numerous experiments have been done to mitigate the ototoxicity of aminoglycosides, no drug has been licensed for patients to prevent ototoxicity.
Programmed cell death (PCD), an active and orderly cell death mode, is a universal phenomenon in the development of organisms (Nagata and Tanaka, 2017). Abnormal regulation of PCD is closely related to a series of human diseases, such as immune diseases (Günther et al., 2013), neuropsychiatric disorders (Margolis et al., 1994), and cancer (Carneiro and El-Deiry, 2020). Previous studies have reported that ototoxic drugs can cause programmed death of auditory hair cells, resulting in hair cell loss and hearing damage (Wu et al., 2020). For a better understanding of the mechanism of aminoglycoside-induced ototoxicity, it is necessary to investigate the different forms of hair cell programmed death, which may eventually provide new ideas for the prevention and treatment of drug-induced hearing loss. In this review, we collected the latest insights into protecting hair cells from the ototoxic effect of aminoglycosides by regulating PCD pathways.
2 The mechanisms of aminoglycoside ototoxicity
Over the past few decades, great progress has been made in elucidating the mechanisms of ototoxicity induced by aminoglycosides. Biological analysis has shown that the formation of oxidative free radicals and subsequent triggering of PCD plays a crucial role in hair cell death. Understanding the way aminoglycosides enter into hair cells and the role of free radicals, as well as how PCD contributes to irreversible HL is helpful to develop new therapeutic methods to protect hearing.
2.1 Trafficking of aminoglycosides into the sensory hair cells
To date, aminoglycosides are clinically available therapies for systematic or local administration to the inner ear. The endothelial cells of cochlear blood vessels are coupled together by tight junctions to form the primary blood–labyrinth barrier (BLB), separating the cochlear cells and fluids from the bloodstream (Nyberg et al., 2019). Aminoglycoside transmission in the inner ear, it is much easier to traverse into the BLB of the stria vascularis in comparison to the spiral ligament of the adjacent perilymphatic position (Dai and Steyger, 2008). Up to now, the mechanisms of the aminoglycosides passing through the BLB, i.e., endothelial cells, the vascular texture, and the marginal cells to the endolymph are still unclear (Koo et al., 2015). Kim et al. showed that megalin was a candidate carrier of the aminoglycosides in the stria vascularis in a study of an in vivo real-time tracking procedure, showing how the aminoglycosides transported across the blood–labyrinth barrier, and blockade of megalin by inhibitor cilastatin could prevent drug accumulation in the inner ear (Kim and Ricci, 2022) (Table 1). There are several pathways for aminoglycosides to permeate HCs from the endolymph. Ion channels transport, especially mechanoelectrical transducer (MET), plays a vital role in the uptake of aminoglycosides to HCs (Vu et al., 2013; Nilius and Szallasi, 2014). The MET channel is a nonspecific cation channel at the tip of hair cells stereociliary with high calcium permeability. Its narrowest portion is at least 1.25–1.5 nm in diameter, sufficient to allow aminoglycosides to enter hair cell cytoplasm (Farris et al., 2004; Alharazneh et al., 2011). Blocking MET channels (e.g., with ORC-13661, d-Tubocurarine, or UoS-7692) prevents aminoglycosides entry into hair cells and confers hair cell protection from aminoglycosides (Kirkwood et al., 2017; Kitcher et al., 2019; Kenyon et al., 2021). It is worth mentioning that long-term blocking of the MET channel would affect hair cell function and worsen hearing impairment. In addition to MET channels, other ion channels are also associated with the uptake of aminoglycosides into the HCs; for example, transient receptor potential (TRP) channels, which are highly expressed in hair cells and play a key role in aminoglycosides entering into hair cells (Myrdal and Steyger, 2005; Lee et al., 2013). The TRPA1 channel is a member of the TRP family, which is a non-selective cation channel activated by certain pungent compounds and lipid peroxidation (Balestrini et al., 2021). In the mammalian cochlea, blocked the MET channel, and activated TRPA1 channels promote the uptake of aminoglycoside. Therefore, it has been proposed that excessive oxidative stress activates TRPA1 channels to enhance the uptake of aminoglycosides by damaged hair cells (Stepanyan et al., 2011).
Endocytosis is another route by which aminoglycosides enter the apical membrane of hair cells, although there is no direct evidence that endocytosis is involved in aminoglycoside-induced cytotoxicity (Hashino and Shero, 1995). It is worth mentioning that Breglio et al. revealed that HSP70-dependent paracrine protection of hair cells is mediated by exosomes which are generated by the direct outward budding of the plasma membrane during endocytosis (Breglio et al., 2020). Targeting endocytosis is regarded as a strategy to prevent ototoxicity is still challenging, because it is not the primary mechanism of aminoglycoside-induced ototoxicity (Figure 1).
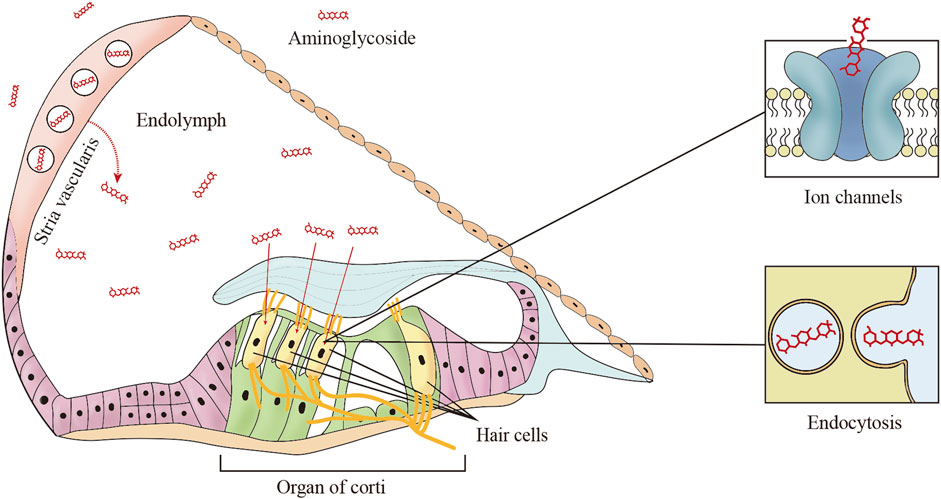
FIGURE 1. The main trafficking routes of aminoglycosides. In systemic administration, aminoglycosides are trafficked from cochlear capillaries into the endolymph, and then aminoglycosides enter the hair cells from the endolymph by two common mechanisms including ion channels and endocytosis.
2.2 Free radical accumulation and programmed cell death
Aminoglycosides rapidly accumulate in the cytoplasm, mitochondria, and endoplasmic reticulum (ER) after entering the cell via the above channels. Aminoglycosides inhibit protein synthesis and increase mRNA misreading by interacting with ribosomes, leading to the accumulation of misfolded proteins and causing various cellular stresses. Excessive reactive oxygen species (ROS), produced by cellular metabolism or contact with foreign substances, have been detected in cochlear tissue immediately after cellular stresses (Navarro-Yepes et al., 2014). ROS is an important group of free radicals, which can cause direct damage or act as key mediator signaling molecules, resulting in a series of biological effects, including caspase3 activation, which promotes hair cell programmed death (O'Sullivan et al., 2017). Various forms of PCD lead to different degrees of biological changes in hair cells. When autophagy occurs, bilayer organelles called autophagosomes bring cytoplasmic products to the lysosomes for degradation. In contrast to autophagy, cells undergoing ferroptosis exhibit increased lipid peroxidation, shrunken mitochondria, and increased mitochondria membrane density (O'Sullivan et al., 2017). It is generally accepted that the most common form of programmed death induced by aminoglycosides in hair cells is apoptosis, which is characterized by cell shrinkage and chromatin agglutination. In preclinical models, antioxidants were used to reduce aminoglycoside-induced ROS production, suppress programmed cell death, and increase cell viability. Antioxidants applied in the study of ototoxicity of aminoglycosides, including D-methionine (Fox et al., 2016), Vitamin C (Gong et al., 2022), N-acetylcysteine (Aladag et al., 2016), quercetin (Hirose et al., 2016), galanin (Kim et al., 2016), fursultiamine (Kim et al., 2021), and salicylates (Sha et al., 2006).
3 Apoptosis pathways in aminoglycoside-induced ototoxicity
Apoptosis is a tightly controlled process by multiple genes. These genes are very conserved among species, such as the Bcl-2 family, caspase family, and tumor suppressor gene P53 (Igney and Krammer, 2002; Szegezdi et al., 2006). External and internal stimuli, in combination with extrinsic and intrinsic apoptosis apoptosis-related genes, can induce cell apoptosis. Bodmer et al. revealed that gentamicin does not cause apoptosis through the Fas receptor, which is the best-characterized member of the extrinsic pathway family and a receptor signal that must be activated during the extrinsic process of apoptosis. This suggests that hair cell apoptosis triggered by aminoglycosides may be initiated primarily through an intrinsic pathway in response to stress signals and excessive ROS (Bodmer et al., 2003) (Figure 2).
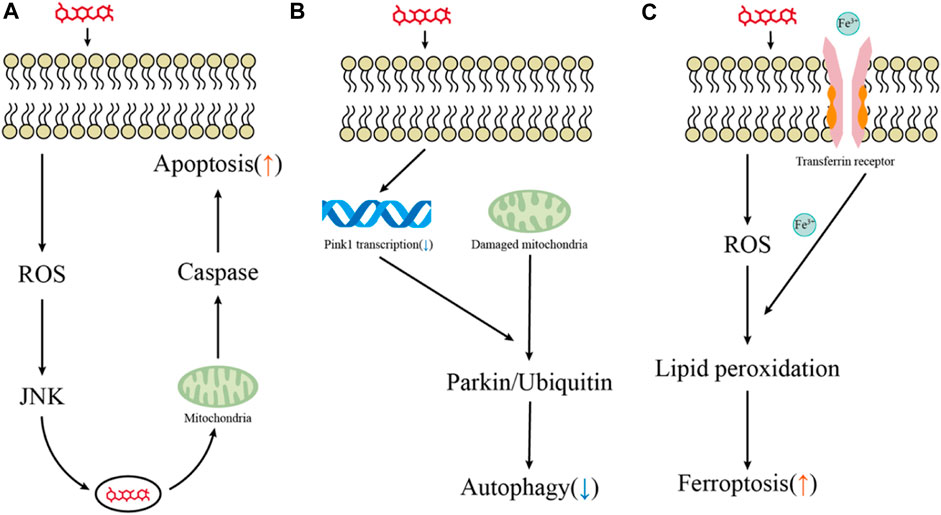
FIGURE 2. Key pathways for hair cell death induced by aminoglycosides. (A–C) Major pathways of aminoglycoside-induced hair cell apoptosis, autophagy, and ferroptosis.
3.1 Apoptosis mediated by caspase
Caspase activation is well known as a common intrinsic pathway for drug-induced hair cell apoptosis. Functionally, caspases involved in apoptosis have been subclassified into initiator (caspases 8, 9, and 10) and effector (caspases 3, 6, and 7) caspases, the initiator caspases are activated by proapoptotic signals and subsequently coordinate their activities to activate effector caspases (Brenner and Mak, 2009; McIlwain et al., 2013). Caspase-9 is considered the major initiator caspase that participates in aminoglycoside-induced hair cell death, which activates downstream caspase-3 and demolishes key structural proteins, ultimately leading to apoptotic of hair cells (McIlwain et al., 2013). It has been reported that caspase-9 and caspase-3 expression was elevated in both HEI-OC1 cells and cochlear exposed to aminoglycosides, and the use of caspase-9 specific inhibitor (z-LEHD-FMK) or general caspase inhibitor (z-VAD-FMK) was found to effectively reduce the expression of caspase and prevent HC death (Matsui et al., 2003; Okuda et al., 2005). Notably, caspase 8 was found to cooperate with RIP kinase to regulate a type of regulatory cell death—necroptosis. It was found to be involved in the ototoxicity of aminoglycosides in vivo (Ruhl et al., 2019), but, the mechanism of programmed necrosis in aminoglycoside-induced ototoxicity is poorly understood.
3.2 The c-jun NH2-terminal kinases (JNKs)
As a redox-sensitive factor, the c-jun NH2-terminal kinases (JNKs), an essential member of the mitogen-activated protein kinase superfamily, play a vital role in the cellular antioxidant self-defense system (Shen and Liu, 2006). Upon aminoglycoside insult, the JNK pathway is activated by phosphorylation and increases the expression of downstream target genes, including itself, to promote hair cell apoptosis (Ylikoski et al., 2002). At the molecular level, cyclin-dependent kinases (CDKs) regulate aminoglycoside-induced hair cell apoptosis by interfering with the JNKs pathway, inhibition of CDK2 activity by pharmaceutical or CDK2 knockout affects JNK signaling and improves resistance to the ototoxicity of gentamicin (Tao and Segil, 2022). Furthermore, small molecule drugs such as a peptide inhibitor (i.e., D-JNKI-1) (Eshraghi et al., 2007) and estradiol (Nakamagoe et al., 2010) were discovered to attenuate hair cell loss following aminoglycoside administration by interfering JNK pathway.
3.3 The role of nuclear factor erythroid 2- related factor 2 (Nrf2) in apoptosis
Nuclear factor erythroid 2-related factor 2 is a transcription factor encoded by the NFE2L2 gene (Sykiotis and Bohmann, 2010), it is localized in hair cells and supporting cells in the human Corti’s organ (Hosokawa et al., 2018). Nrf2 is an important antioxidant regulator in a series of chronic toxic lesions caused by oxidative stress. In recent years, studies on the relationship between Nrf2 transcription factors and hearing impairment have given people a new understanding of hair cell damage and provided new strategic targets for the development and reuse of new drugs (Cuadrado et al., 2019).
Nrf2 is regulated by upstream pathways, Baird et al. revealed that Sestrin-2 (Sesn2), a member of the antioxidant family, is involved in protecting hair cells against gentamicin by activating Nrf2 (Baird and Dinkova-Kostova, 2011). Appreciation that Nrf2 pathway activation provides hair cells protection from ototoxic drugs, the protective effect on hair cells seems to be heading in a new trend, that is, by stimulating the expression of Nrf2 and preventing hair cells from being damaged. Ebselen is a potent glutathione peroxidase (GPx) mimic and inducer with antioxidant and anti-inflammatory effects. It has been found to attenuate aminoglycoside-induced ototoxicity by activating the Nrf2 signaling pathway, increasing glutathione, and dramatically stimulating GPx1 transcription (Gu et al., 2021). Encouragingly, the safety and efficacy of ebselen for the prevention of noise-induced hearing loss are undergoing phase 2 clinical trials (Kil et al., 2017). Moreover, other drugs have also been found to protect hair cells from aminoglycosides by intervening in the NRF2 pathway, such as apigenin (Jia et al., 2022) and Heme oxygenase-1 (Yang et al., 2022).
3.4 Other apoptosis signals
Other pathways have been investigated for cellular apoptosis. The Bcl-2 protein family could be the main regulator that triggers caspase activation. From a functional point of view, Bcl-2-related proteins can inhibit or accelerate cell apoptosis, while the interaction between the two opposite proteins is a key factor in determining cell death (Willis et al., 2003). Studies have shown that overexpression of the anti-apoptotic Bcl-2 gene may have a certain inhibitory effect on hair cell apoptosis induced by aminoglycosides (Liu et al., 2007). Another apoptosis signal, transcription factor forkhead box O3 transcription factor (Foxo3), can regulate the expression of stress response proteins in the body and participate in the apoptosis of various tissues (Dansen and Burgering, 2008; Gargini et al., 2015). The role of the Foxo3 gene and Bcl-2 family genes in apoptosis was further confirmed by the discovery that Wnt/β-catenin signaling regulates Foxo3 and Bcl-2 expression, controls the content of ROS, inhibits apoptosis and protects HCs immune from neomycin injury (Liu et al., 2016). Interestingly, the Hippo/Yes-associated protein (YAP) signaling pathway, which plays a key role in the development and progression of cancer (Harvey and Tapon, 2007), may be involved in hair cell damage induced by aminoglycosides. Wang et al. regulated the Hippo/YAP signaling pathway in vitro against neomycin-induced HC loss by inhibiting cell apoptosis and decreasing ROS accumulation by using the YAP agonist XMU-MP-1 (XMU) successfully (Wang et al., 2022). Inhibition or activation of numerous signaling pathways should be considered when regulating apoptosis to better protect hair cells from ototoxic damage.
4 Autophagy pathways in aminoglycoside-induced ototoxicity
Substantial findings suggest that cellular death mediated via autophagy is essential in hearing loss. Autophagy is considered an orderly degradation and recycling mechanism, which decomposes unnecessary or dysfunctional cellular components to maintain the homeostasis of the body (Eskelinen, 2019). In the early stage of the disease, autophagy activation may facilitate the disease process delay, nevertheless, cells may over-activate autophagy leading to cell death as the prominent pathogenic factors appear (Wu et al., 2020). Thus, in the growth and maintenance of multicellular organisms, autophagy always keeps a delicate balance, and loss or overactivation of autophagy regulation could result in the occurrence of sickness (Levine and Kroemer, 2008; Narendra et al., 2009). Previous experiments proved that autophagy induction increases the survival of retinal ganglion cells (RGCs) (Rodríguez-Muela et al., 2012). However, the effect of the activation of autophagy on the ototoxicity of aminoglycosides remains controversial.
4.1 Autophagy activation protects hair cells from aminoglycoside-induced ototoxicity
Combined injection of kanamycin and furosemide can cause capillary detachment and reduce the autophagic flux for lysosome production, leading to the degeneration of hair cells and spiral ganglion neurons. After autophagy dysfunction was partially ameliorated with an MTOR inhibitor temsirolimus (CCI-779), lysosome defects were significantly relieved, oxidative stress levels were reduced, and the density of surviving spiral ganglion neurons and hair cells was significantly increased (Ye et al., 2019). He et al. stimulated the autophagy activity with rapamycin, a commonly used autophagy activator, and significantly reduced the ROS levels, apoptosis, and cell death after neomycin or gentamicin injury, and the viability of cochlear explants and HEI-OC1 cells was enhanced. In contrast, exposure to aminoglycosides resulted in reduced autophagy activity, increased ROS levels, apoptosis, and cell death following treatment with the autophagy inhibitor 3-methyladenine (3-MA) or knockdown of autophagy-related (ATG) proteins (He et al., 2017).
PINK1 is a serine/threonine kinase located in the mitochondria, while Parkin is an E3 ubiquitin ligase present in the cytoplasm. PINK1 recruits parkin to depolarized mitochondria, ubiquitinates the mitochondrial substrates, and drives autophagy (Lazarou et al., 2012; Goiran et al., 2018; Pickles et al., 2018). Yang et al. treated HEI-OC1 cells and mouse cochlear hair cells with 400 µM gentamicin and found that gentamicin exposure promoted the PINK1 degradation and parkin recruitment, enhancing autophagy activity. They interfered with PINK1 expression of HEI-OC1 by using specific PINK-siRNA and demonstrated that activation of PINK1 prevented gentamicin-induced damage by promoting spontaneous autophagic and inhibiting the increase of p53 in HEI-OC1 cells (Yang et al., 2018). The transcription of PINK1 is mainly regulated by ATF3 (activating transcription factor 3), XBP1 (X-box binding protein 1), and FOXO3 (forkhead box O3) (Bueno et al., 2018). By inducing ATF3 expression in HEI-OC1 cells and cochlear hair cells, neomycin repressed Pink1 transcription and decreased autophagic activity (Zhang et al., 2023). Accumulating evidence suggests that gene modulation or pharmacological intervention of autophagy may have therapeutic potential for aminoglycoside-induced hearing loss (Figure 2).
4.2 New perspective on the role of autophagy in ototoxicity of aminoglycosides
Some scholars have proposed that appropriate ROS after neomycin injury can promote autophagy, restore damaged cellular components, and maintain the stability of the internal environment. However, the role of autophagy in aminoglycoside ototoxicity is controversial, in a large, unbiased screen, Ryan and others found that autophagy is involved in the ototoxic injury of hair cells in a complex manner, they predicted that many complexes protect hair cells from aminoglycoside damage could inhibit autophagy, in contrast, some complexes could promote autophagy to reducing ototoxicity (Draf et al., 2021). Recently, Bruijn et al. reported that aminoglycosides could trigger the interaction of RIPOR2 in murine hair cells with GABARAP, an autophagy pathway that plays an important role in clearing and restoring dysfunctional cellular components (de Bruijn et al., 2020). Surprisingly, the reduction of RIPOR2 and GABARAP proteins completely inhibited aminoglycoside administration-induced hair cell death and subsequent hearing loss. In addition, disrupting the autophagy pathway by canceling PINK1 or Parkin expression could also protect hair cells from aminoglycoside-induced ototoxicity (Li et al., 2022). It would be interesting to explore the role of autophagy in aminoglycoside-induced ototoxicity, as both high and low levels of autophagy can cause damage to the hair cells, and the degree of autophagy requires exquisite control. As a possibility, the effects of autophagy on hair cell function and survival are multi-pathway regulated, and the level of autophagy needs to be precisely adjusted to protect hair cells from aminoglycosides.
5 Ferroptosis pathways in aminoglycoside-induced ototoxicity
Ferroptosis is a new type of iron-dependent programmed cell death, which is different from apoptosis, necrosis, and autophagy (Dixon et al., 2012; Galluzzi et al., 2018; Shen et al., 2018). Three essential hallmarks define ferroptosis: oxidation of polyunsaturated fatty acid-containing phospholipids, loss of lipid peroxide repair, and reduction in the accumulation of redox-active iron (Friedmann Angeli et al., 2014; Xie et al., 2016; Wu et al., 2021).
Iron has a great influence on the metabolism of cells and plays an important role in tissue damage, as it participates in the generation of highly reactive oxygen species. Two iron chelators, deferoxamine, and 2,3-dihydroxybenzoate could compete with gentamicin for “free” iron ions and reduce ototoxic damage to hair cells (Song and Schacht, 1996). Considered that “free” iron ions are crucial members in the occurrence of ferroptosis. Therefore, established iron chelates may be selected as promising therapeutic agents, reducing aminoglycoside-induced ototoxicity by inhibiting ferroptosis.
Increasing attention has been attracted to further research investigating the pathophysiological role of ferroptosis in the field of drug-induced hearing loss (Figure 2). Ferroptosis may coordinate with other types of cell death involved in drug-induced hair cell ototoxic damage, and recent studies have provided that activation of the autophagy pathway promotes ferroptosis by degrading ferritin (Hou et al., 2016). Mei et al. found that HEI-OC1 cells treated with cisplatin not only markedly augmented iron accumulation but reduced the activity of glutathione peroxidase 4 (GPX4), an intracellular antioxidant enzyme that inhibits the production of lipid peroxidation (Mei et al., 2020). Notably, treatment with the specific ferroptosis inhibitor ferrostatin-1 could effectively reduce cisplatin-induced ototoxicity by inhibiting lipid peroxide free radicals and improving the mitochondrial function of hair cells (Hu et al., 2020; Mei et al., 2020). Like cisplatin, ferroptosis could be induced in HEI-OC1 cells and neonatal mouse cochlear explants following aminoglycoside exposure. Pretreatment with selective ferroptosis inhibitor liproxstatin-1 (Lip-1) significantly alleviated the production of ROS and the destruction of mitochondrial membrane potential (ΔΨm) in the HEI-OC1 cells (Zheng et al., 2020).
Ferroptosis is a newly discovered hair cell programmed death associated with aminoglycoside-induced ototoxicity, and inhibition of ferroptosis successfully prevented hearing loss induced by cisplatin and aminoglycosides in vitro model. However, the role of ferroptosis in aminoglycoside-induced ototoxicity has not been studied in mammals in vivo, and the mechanisms of ferroptosis in regulating ototoxic hair cell death are unclear. We need more studies to understand the impact of ferroptosis on ototoxicity. Additionally, we need to extend this research to animals to promote ferroptosis inhibitors as possible ear protection drugs for patients.
6 Gene therapy regulates programmed cell death induced by aminoglycosides
The past few decades witnessed the development of gene therapy, which focuses on providing effective gene interventions in different periods to treat or prevent diseases (Omichi et al., 2019; Zhang et al., 2020). Gene therapy candidates are probably the most complex drugs invented by mankind to date and may become one of the important means to treat intractable diseases (Maeder et al., 2019; Santiago-Fernández et al., 2019; Wu et al., 2019; Stadtmauer et al., 2020). Applying this technology to the clinic is still challenging, but the trends are encouraging in the future.
6.1 Overexpression of apoptotic genes
At present, it is possible to construct regulatory elements upstream of the target gene by artificial modification, so that the gene can be transcribed and translated under artificially controlled conditions to achieve the products of gene overexpression.
Several neurotrophic factors, especially brain-derived neurotrophic factor (BDNF) and neurotrophin-3 (NT-3) promote the growth, survival, and interconnectivity of hair cells (Ramekers et al., 2012). In animals exposed to kanamycin, infusion of NT-3 or BDNF into the perilymphatic space of the cochlea was found to prevent the loss of auditory hair cells (Ruan et al., 1999). Therefore, neurotrophin gene transfer into the inner ear may be a practical means to rescue ototoxin-exposed cochlear hair cells. Indeed, adenovirus-mediated neurotrophic factor overexpression in the inner ear demonstrated that hair cells and spiral ganglion neurons are protected from degeneration and death caused by aminoglycoside-induced ototoxicity (Kawamoto et al., 2003; Wise et al., 2010; Leake et al., 2020). Not only neurotrophins but also several apoptosis-related genes have been amplified by overexpression in vivo to observe if they can mitigate drug-induced ototoxicity. In the auditory system, overexpression of Bcl-2, an essential gene involved in hair cell apoptosis mentioned above, has been shown to protect against aminoglycoside-induce ototoxicity in vitro and in vivo mouse models (Pfannenstiel et al., 2009). Utilized adenovirus-mediated gene therapy overexpressing human catalase in the inner ear and demonstrated that overexpression of antioxidant genes could significantly protect the HC from ototoxic damage (Kawamoto et al., 2004). In preclinical studies, an increasing number of genes are amplified in mice by overexpression to prevent the occurrence and development of diseases. In the future, virus-mediated gene overexpression is expected to solve the disease problem in clinical practice.
6.2 Knockdown of apoptotic genes
CRISPR/Cas9 is a novel gene editing technology. Its simplicity, ease of use, and powerful gene editing capabilities have rapidly attracted substantial attention from scientists in different fields (Deltcheva et al., 2011; Cong et al., 2013). This system has been applied to the mouse model of human congenital deafness in preclinical studies multiple times and successfully corrected the gene mutation in the inner ear of mice to rescue their hearing (Gao et al., 2018; Xue et al., 2022). It is heartening to see the remarkable success in the prevention and treatment of acquired sensorineural hearing loss using CRISPR/Cas9 gene editing technology recently.
Inhibitor of apoptosis protein (IAP) prevents apoptosis by blocking canonical caspase-mediated apoptosis and JNK signaling pathways (Deveraux et al., 1999). In many cases, IAP family proteins can cross species barriers to inhibit apoptosis, this means that although the details of their regulation may differ, these proteins target a common mechanism of programmed cell death (Deveraux and Reed, 1999). X-linked inhibitor of apoptosis protein (XIAP) is one of the most effective IAP, it does not prevent caspase activation but inhibits them after they are activated, this post-activation inhibition occurs because XIAP binds to neo-epitopes that are exposed when caspases are activated by cleavage (Unsain et al., 2013). Sun et al. confirmed that XIAP was involved in HCs apoptosis. Injecting neomycin into high-expressing XIAP mice, they found that overexpression of XIAP effectively prevented the loss of HCs, especially in the apical turn (Sun et al., 2014). Whereas, XIAP in hair cells after exposure to aminoglycosides may be degraded by upstream pathways regulation. HtrA2 (High-temperature demand A2)/Omi is a nuclear-encoded protein found in the inner mitochondrial membrane space. In response to apoptotic stimuli, HtrA2 is released into the cytoplasm, cleaving XIAP, Apollon/BRUCE, and other proteins to promote apoptosis and maintain homeostasis (Goo et al., 2014). Our team tried to inhibit XIAP degradation to resist apoptosis by knocking down Htra2 with the application of the CRISPR-Cas9 system. We targeted editing of the Htra2 gene and demonstrated that knockout of the Htra2 gene by the adeno-associated virus (AAV)-mediated CRISPR/Cas9 system could effectively prevent aminoglycoside-induced ototoxic deafness in mice. The protection against neomycin exposure in vivo after pretreatment with the AAV-CRISPR/Cas9 system can last for a long time, even up to 8 weeks after the injection system. We also evaluated the safety of AAV-CRISPR/Cas9 systems, during the observation period of the study, no obvious off-target conditions were found, and there was no effect on the hearing of wild-type mice, indicating that the treatment system was safe (Gu et al., 2021). The CRISPR/CasRx system, a member of the CRISPR system type VI, provides an efficient and specific new method for RNA manipulation in both prokaryotes and eukaryotes (Shmakov et al., 2015; Abudayyeh et al., 2016; Smargon et al., 2017). After using the AAV-CasRx-gRNA system, we found that knocking out the Htra2 gene transcript could effectively reduce the loss of cochlear hair cells after neomycin exposure and attenuate hearing loss in mice. In addition, knockdown of the Htra2 gene significantly reduced the mRNA expression of Casp3 and Casp9 in cochlear hair cells after neomycin treatment (Guo et al., 2022). These results demonstrated that hair cell ototoxic damage can be effectively prevented by the specific knockout of a target gene at the DNA/RNA level. Recent clinical studies have shown that RNA interference therapy is an available option to target and suppress genes associated with cancer, and accelerate the development of cancer treatments in a novel way (Kara et al., 2022). What’s more, studies have been detecting the RNA interference (RNAi) as a way of selectively suppressing mutant alleles in animal models with genetic hearing loss (Shibata et al., 2016). Therefore, it is of great interest to investigate the treatment of RNA interference as a promising strategy for preventing drug-induced hearing loss in the future.
In most studies of protective therapy, drugs are administered intraperitoneally, subcutaneously, or intravenously. After entering the body, many small-molecule therapeutic drugs cannot play a role due to short plasma half-life or unable to reach inner ear cells through the blood–labyrinth barrier. Compared with drug administration, local injection of gene therapy agents into the inner ear owns several outstanding advantages (i) the therapeutic effect of gene therapy could achieve a long-term disease prevention and treatment, (ii) personalized gene therapy could achieve the curative effect based on the individual genetic status and treatment condition, (iii) gene therapy has the potential to correct defective genes for the treatment of diseases fundamentally. Further evaluation is necessary for the safety and application of gene therapy, we believe that shortly, gene therapy can be used as an accurate treatment for hearing loss, restoring hearing function and preventing the development of hearing loss in a way that conventional medicine cannot.
7 Conclusion
Ototoxic drugs enter hair cells via the endolymph and accumulate in organelles to produce excessive ROS. The accumulation of ROS will lead to the activation of the relevant target genes and subsequent cell death. As mentioned above, many methods have been tried to reduce the accumulation of drugs in the cochlea and affect the ototoxicity process to mitigate the loss of hair cells. This review focuses on drug and gene therapies to reduce the ototoxicity of aminoglycosides by regulating programmed cell death, including apoptosis, autophagy, and ferroptosis. In recent decades, the increasing understanding of apoptosis, autophagy, and ferroptosis has led to the development of clinical treatments for hearing loss. The occurrence and development of ototoxicity may involve multiple forms of hair cell death and related signaling pathways, audiologists have been trying to understand how these pathways map and integrate. To better protect hair cells from ototoxic drugs, we need to understand the pathogenesis of hearing loss, multi-target, and multi-pathway therapies should be considered according to the characteristics of programmed cell death. CRISPR/Cas9 gene editing technology, which allows precise editing of target genes, has set off a new wave of research and made remarkable achievements in the treatment of drug-induced deafness. In the future, the problem of drug ototoxicity will gradually dissipate with the continuous exploration of researchers.
Author contributions
LH: Writing–original draft. ZW: Writing–original draft. DW: Writing–original draft. ZG: Writing–original draft. SH: Writing–original draft. DS: Writing–review and editing. YS: Writing–review and editing.
Funding
The author(s) declare financial support was received for the research, authorship, and/or publication of this article. This work was supported by the National Key R&D Program of China (2020YFA0908201 and 2021YFA1101302), the National Natural Science Foundation of China (82225014, 82171148 and 82192864), the Shuguang Program of Shanghai Education Development Foundation and the Shanghai Municipal Education Commission (20SG08), Natural Science Foundation of Hunan Province (2021JJ70111), The Clinical Medical Technology Innovation Guidance Project of Department of Science and Technology of Hunan Provincial (2021SK51715).
Conflict of interest
The authors declare that the research was conducted in the absence of any commercial or financial relationships that could be construed as a potential conflict of interest.
Publisher’s note
All claims expressed in this article are solely those of the authors and do not necessarily represent those of their affiliated organizations, or those of the publisher, the editors and the reviewers. Any product that may be evaluated in this article, or claim that may be made by its manufacturer, is not guaranteed or endorsed by the publisher.
References
Abudayyeh, O. O., Gootenberg, J. S., Konermann, S., Joung, J., Slaymaker, I. M., Cox, D. B., et al. (2016). C2c2 is a single-component programmable RNA-guided RNA-targeting CRISPR effector. Science 353 (6299), aaf5573. doi:10.1126/science.aaf5573
Aladag, I., Guven, M., and Songu, M. (2016). Prevention of gentamicin ototoxicity with N-acetylcysteine and vitamin A. J. Laryngol. Otol. 130 (5), 440–446. doi:10.1017/s0022215116000992
Alharazneh, A., Luk, L., Huth, M., Monfared, A., Steyger, P. S., Cheng, A. G., et al. (2011). Functional hair cell mechanotransducer channels are required for aminoglycoside ototoxicity. PLoS One 6 (7), e22347. doi:10.1371/journal.pone.0022347
Baird, L., and Dinkova-Kostova, A. T. (2011). The cytoprotective role of the Keap1-Nrf2 pathway. Arch. Toxicol. 85 (4), 241–272. doi:10.1007/s00204-011-0674-5
Balestrini, A., Joseph, V., Dourado, M., Reese, R. M., Shields, S. D., Rougé, L., et al. (2021). A TRPA1 inhibitor suppresses neurogenic inflammation and airway contraction for asthma treatment. J. Exp. Med. 218 (4), e20201637. doi:10.1084/jem.20201637
Bodmer, D., Brors, D., Pak, K., Bodmer, M., and Ryan, A. F. (2003). Gentamicin-induced hair cell death is not dependent on the apoptosis receptor Fas. Laryngoscope 113 (3), 452–455. doi:10.1097/00005537-200303000-00012
Breglio, A. M., May, L. A., Barzik, M., Welsh, N. C., Francis, S. P., Costain, T. Q., et al. (2020). Exosomes mediate sensory hair cell protection in the inner ear. J. Clin. Invest. 130 (5), 2657–2672. doi:10.1172/jci128867
Brenner, D., and Mak, T. W. (2009). Mitochondrial cell death effectors. Curr. Opin. Cell Biol. 21 (6), 871–877. doi:10.1016/j.ceb.2009.09.004
Bueno, M., Brands, J., Voltz, L., Fiedler, K., Mays, B., St Croix, C., et al. (2018). ATF3 represses PINK1 gene transcription in lung epithelial cells to control mitochondrial homeostasis. Aging Cell 17 (2), e12720. doi:10.1111/acel.12720
Carneiro, B. A., and El-Deiry, W. S. (2020). Targeting apoptosis in cancer therapy. Nat. Rev. Clin. Oncol. 17 (7), 395–417. doi:10.1038/s41571-020-0341-y
Cong, L., Ran, F. A., Cox, D., Lin, S., Barretto, R., Habib, N., et al. (2013). Multiplex genome engineering using CRISPR/Cas systems. Science 339 (6121), 819–823. doi:10.1126/science.1231143
Crundwell, G., Gomersall, P., and Baguley, D. M. (2016). Ototoxicity (cochleotoxicity) classifications: a review. Int. J. Audiol. 55 (2), 65–74. doi:10.3109/14992027.2015.1094188
Cuadrado, A., Rojo, A. I., Wells, G., Hayes, J. D., Cousin, S. P., Rumsey, W. L., et al. (2019). Therapeutic targeting of the NRF2 and KEAP1 partnership in chronic diseases. Nat. Rev. Drug Discov. 18 (4), 295–317. doi:10.1038/s41573-018-0008-x
Dai, C. F., and Steyger, P. S. (2008). A systemic gentamicin pathway across the stria vascularis. Hear Res. 235 (1-2), 114–124. doi:10.1016/j.heares.2007.10.010
Dansen, T. B., and Burgering, B. M. (2008). Unravelling the tumor-suppressive functions of FOXO proteins. Trends Cell Biol. 18 (9), 421–429. doi:10.1016/j.tcb.2008.07.004
de Bruijn, S. E., Smits, J. J., Liu, C., Lanting, C. P., Beynon, A. J., Blankevoort, J., et al. (2020). A RIPOR2 in-frame deletion is a frequent and highly penetrant cause of adult-onset hearing loss. J. Med. Genet. 58, 96–104. doi:10.1136/jmedgenet-2020-106863
Deltcheva, E., Chylinski, K., Sharma, C. M., Gonzales, K., Chao, Y., Pirzada, Z. A., et al. (2011). CRISPR RNA maturation by trans-encoded small RNA and host factor RNase III. Nature 471 (7340), 602–607. doi:10.1038/nature09886
Deveraux, Q. L., and Reed, J. C. (1999). IAP family proteins--suppressors of apoptosis. Genes Dev. 13 (3), 239–252. doi:10.1101/gad.13.3.239
Deveraux, Q. L., Stennicke, H. R., Salvesen, G. S., and Reed, J. C. (1999). Endogenous inhibitors of caspases. J. Clin. Immunol. 19 (6), 388–398. doi:10.1023/a:1020502800208
Dillard, L. K., Martinez, R. X., Perez, L. L., Fullerton, A. M., Chadha, S., and McMahon, C. M. (2021). Prevalence of aminoglycoside-induced hearing loss in drug-resistant tuberculosis patients: a systematic review. J. Infect. 83 (1), 27–36. doi:10.1016/j.jinf.2021.05.010
Dixon, S. J., Lemberg, K. M., Lamprecht, M. R., Skouta, R., Zaitsev, E. M., Gleason, C. E., et al. (2012). Ferroptosis: an iron-dependent form of nonapoptotic cell death. Cell 149 (5), 1060–1072. doi:10.1016/j.cell.2012.03.042
Draf, C., Wyrick, T., Chavez, E., Pak, K., Kurabi, A., Leichtle, A., et al. (2021). A screen of autophagy compounds implicates the proteasome in mammalian aminoglycoside-induced hair cell damage. Front. Cell Dev. Biol. 9, 762751. doi:10.3389/fcell.2021.762751
Eshraghi, A. A., Wang, J., Adil, E., He, J., Zine, A., Bublik, M., et al. (2007). Blocking c-Jun-N-terminal kinase signaling can prevent hearing loss induced by both electrode insertion trauma and neomycin ototoxicity. Hear Res. 226 (1-2), 168–177. doi:10.1016/j.heares.2006.09.008
Eskelinen, E. L. (2019). Autophagy: supporting cellular and organismal homeostasis by self-eating. Int. J. Biochem. Cell Biol. 111, 1–10. doi:10.1016/j.biocel.2019.03.010
Farris, H. E., LeBlanc, C. L., Goswami, J., and Ricci, A. J. (2004). Probing the pore of the auditory hair cell mechanotransducer channel in turtle. J. Physiol. 558 (Pt 3), 769–792. doi:10.1113/jphysiol.2004.061267
Fettiplace, R., and Hackney, C. M. (2006). The sensory and motor roles of auditory hair cells. Nat. Rev. Neurosci. 7 (1), 19–29. doi:10.1038/nrn1828
Fox, D. J., Cooper, M. D., Speil, C. A., Roberts, M. H., Yanik, S. C., Meech, R. P., et al. (2016). d-Methionine reduces tobramycin-induced ototoxicity without antimicrobial interference in animal models. J. Cyst. Fibros. 15 (4), 518–530. doi:10.1016/j.jcf.2015.06.005
Friedmann Angeli, J. P., Schneider, M., Proneth, B., Tyurina, Y. Y., Tyurin, V. A., Hammond, V. J., et al. (2014). Inactivation of the ferroptosis regulator Gpx4 triggers acute renal failure in mice. Nat. Cell Biol. 16 (12), 1180–1191. doi:10.1038/ncb3064
Galluzzi, L., Vitale, I., Aaronson, S. A., Abrams, J. M., Adam, D., Agostinis, P., et al. (2018). Molecular mechanisms of cell death: recommendations of the nomenclature committee on cell death 2018. Cell Death Differ. 25 (3), 486–541. doi:10.1038/s41418-017-0012-4
Gao, X., Tao, Y., Lamas, V., Huang, M., Yeh, W. H., Pan, B., et al. (2018). Treatment of autosomal dominant hearing loss by in vivo delivery of genome editing agents. Nature 553 (7687), 217–221. doi:10.1038/nature25164
Gargini, R., Cerliani, J. P., Escoll, M., Antón, I. M., and Wandosell, F. (2015). Cancer stem cell-like phenotype and survival are coordinately regulated by Akt/FoxO/Bim pathway. Stem Cells 33 (3), 646–660. doi:10.1002/stem.1904
Goiran, T., Duplan, E., Rouland, L., El Manaa, W., Lauritzen, I., Dunys, J., et al. (2018). Nuclear p53-mediated repression of autophagy involves PINK1 transcriptional down-regulation. Cell Death Differ. 25 (5), 873–884. doi:10.1038/s41418-017-0016-0
Gong, L., Chen, B., Chen, J., and Li, Y. (2022). Protective effects of vitamin C against neomycin-induced apoptosis in HEI-OC1 auditory cell. Neural. Plast. 2022, 1298692. doi:10.1155/2022/1298692
Goo, H. G., Rhim, H., and Kang, S. (2014). HtrA2/Omi influences the stability of LON protease 1 and prohibitin, proteins involved in mitochondrial homeostasis. Exp. Cell Res. 328 (2), 456–465. doi:10.1016/j.yexcr.2014.07.032
Gu, R., Longenecker, R. J., Homan, J., and Kil, J. (2021a). Ebselen attenuates tobramycin-induced ototoxicity in mice. J. Cyst. Fibros. 20 (2), 271–277. doi:10.1016/j.jcf.2020.02.014
Gu, X., Wang, D., Xu, Z., Wang, J., Guo, L., Chai, R., et al. (2021b). Prevention of acquired sensorineural hearing loss in mice by in vivoHtra2 gene editing. Genome Biol. 22 (1), 86. doi:10.1186/s13059-021-02311-4
Günther, C., Neumann, H., Neurath, M. F., and Becker, C. (2013). Apoptosis, necrosis and necroptosis: cell death regulation in the intestinal epithelium. Gut 62 (7), 1062–1071. doi:10.1136/gutjnl-2011-301364
Guo, Y., Han, L., Han, S., Tang, H., Wang, S., Cui, C., et al. (2022). Specific knockdown of Htra2 by CRISPR-CasRx prevents acquired sensorineural hearing loss in mice. Mol. Ther. Nucleic Acids 28, 643–655. doi:10.1016/j.omtn.2022.04.014
Harvey, K., and Tapon, N. (2007). The Salvador-Warts-Hippo pathway - an emerging tumour-suppressor network. Nat. Rev. Cancer 7 (3), 182–191. doi:10.1038/nrc2070
Hashino, E., and Shero, M. (1995). Endocytosis of aminoglycoside antibiotics in sensory hair cells. Brain Res. 704 (1), 135–140. doi:10.1016/0006-8993(95)01198-6
He, Z., Guo, L., Shu, Y., Fang, Q., Zhou, H., Liu, Y., et al. (2017). Autophagy protects auditory hair cells against neomycin-induced damage. Autophagy 13 (11), 1884–1904. doi:10.1080/15548627.2017.1359449
Hirose, Y., Sugahara, K., Kanagawa, E., Takemoto, Y., Hashimoto, M., and Yamashita, H. (2016). Quercetin protects against hair cell loss in the zebrafish lateral line and Guinea pig cochlea. Hear Res. 342, 80–85. doi:10.1016/j.heares.2016.10.001
Hosokawa, K., Hosokawa, S., Ishiyama, G., Ishiyama, A., and Lopez, I. A. (2018). Immunohistochemical localization of Nrf2 in the human cochlea. Brain Res. 1700, 1–8. doi:10.1016/j.brainres.2018.07.004
Hou, W., Xie, Y., Song, X., Sun, X., Lotze, M. T., Zeh, H. J., et al. (2016). Autophagy promotes ferroptosis by degradation of ferritin. Autophagy 12 (8), 1425–1428. doi:10.1080/15548627.2016.1187366
Hu, B., Liu, Y., Chen, X., Zhao, J., Han, J., Dong, H., et al. (2020). Ferrostatin-1 protects auditory hair cells from cisplatin-induced ototoxicity in vitro and in vivo. Biochem. Biophys. Res. Commun. 533 (4), 1442–1448. doi:10.1016/j.bbrc.2020.10.019
Igney, F. H., and Krammer, P. H. (2002). Death and anti-death: tumour resistance to apoptosis. Nat. Rev. Cancer 2 (4), 277–288. doi:10.1038/nrc776
Jia, G., Mao, H., Zhang, Y., Ni, Y., and Chen, Y. (2022). Apigenin alleviates neomycin-induced oxidative damage via the Nrf2 signaling pathway in cochlear hair cells. Front. Med. 16 (4), 637–650. doi:10.1007/s11684-021-0864-3
Kara, G., Calin, G. A., and Ozpolat, B. (2022). RNAi-based therapeutics and tumor targeted delivery in cancer. Adv. Drug Deliv. Rev. 182, 114113. doi:10.1016/j.addr.2022.114113
Kawamoto, K., Sha, S. H., Minoda, R., Izumikawa, M., Kuriyama, H., Schacht, J., et al. (2004). Antioxidant gene therapy can protect hearing and hair cells from ototoxicity. Mol. Ther. 9 (2), 173–181. doi:10.1016/j.ymthe.2003.11.020
Kawamoto, K., Yagi, M., Stöver, T., Kanzaki, S., and Raphael, Y. (2003). Hearing and hair cells are protected by adenoviral gene therapy with TGF-beta1 and GDNF. Mol. Ther. 7 (4), 484–492. doi:10.1016/s1525-0016(03)00058-3
Kenyon, E. J., Kirkwood, N. K., Kitcher, S. R., Goodyear, R. J., Derudas, M., Cantillon, D. M., et al. (2021). Identification of a series of hair-cell MET channel blockers that protect against aminoglycoside-induced ototoxicity. JCI Insight 6 (7), e145704. doi:10.1172/jci.insight.145704
Kil, J., Lobarinas, E., Spankovich, C., Griffiths, S. K., Antonelli, P. J., Lynch, E. D., et al. (2017). Safety and efficacy of ebselen for the prevention of noise-induced hearing loss: a randomised, double-blind, placebo-controlled, phase 2 trial. Lancet 390 (10098), 969–979. doi:10.1016/s0140-6736(17)31791-9
Kim, J., and Ricci, A. J. (2022). In vivo real-time imaging reveals megalin as the aminoglycoside gentamicin transporter into cochlea whose inhibition is otoprotective. Proc. Natl. Acad. Sci. U. S. A. 119 (9), e2117946119. doi:10.1073/pnas.2117946119
Kim, Y. R., Kim, M. A., Cho, H. J., Oh, S. K., Lee, I. K., Kim, U. K., et al. (2016). Galangin prevents aminoglycoside-induced ototoxicity by decreasing mitochondrial production of reactive oxygen species in mouse cochlear cultures. Toxicol. Lett. 245, 78–85. doi:10.1016/j.toxlet.2016.01.005
Kim, Y. R., Kwon, T. J., Kim, U. K., Lee, I. K., Lee, K. Y., and Baek, J. I. (2021). Fursultiamine prevents drug-induced ototoxicity by reducing accumulation of reactive oxygen species in mouse cochlea. Antioxidants (Basel) 10 (10), 1526. doi:10.3390/antiox10101526
Kirkwood, N. K., O'Reilly, M., Derudas, M., Kenyon, E. J., Huckvale, R., van Netten, S. M., et al. (2017). d-Tubocurarine and berbamine: alkaloids that are permeant blockers of the hair cell's mechano-electrical transducer channel and protect from aminoglycoside toxicity. Front. Cell Neurosci. 11, 262. doi:10.3389/fncel.2017.00262
Kitcher, S. R., Kirkwood, N. K., Camci, E. D., Wu, P., Gibson, R. M., Redila, V. A., et al. (2019). ORC-13661 protects sensory hair cells from aminoglycoside and cisplatin ototoxicity. JCI Insight 4 (15), e126764. doi:10.1172/jci.insight.126764
Koo, J. W., Quintanilla-Dieck, L., Jiang, M., Liu, J., Urdang, Z. D., Allensworth, J. J., et al. (2015). Endotoxemia-mediated inflammation potentiates aminoglycoside-induced ototoxicity. Sci. Transl. Med. 7 (298), 298ra118. doi:10.1126/scitranslmed.aac5546
Lazarou, M., Jin, S. M., Kane, L. A., and Youle, R. J. (2012). Role of PINK1 binding to the TOM complex and alternate intracellular membranes in recruitment and activation of the E3 ligase Parkin. Dev. Cell 22 (2), 320–333. doi:10.1016/j.devcel.2011.12.014
Leake, P. A., Akil, O., and Lang, H. (2020). Neurotrophin gene therapy to promote survival of spiral ganglion neurons after deafness. Hear Res. 394, 107955. doi:10.1016/j.heares.2020.107955
Lee, J. H., Park, C., Kim, S. J., Kim, H. J., Oh, G. S., Shen, A., et al. (2013). Different uptake of gentamicin through TRPV1 and TRPV4 channels determines cochlear hair cell vulnerability. Exp. Mol. Med. 45 (3), e12. doi:10.1038/emm.2013.25
Levine, B., and Kroemer, G. (2008). Autophagy in the pathogenesis of disease. Cell 132 (1), 27–42. doi:10.1016/j.cell.2007.12.018
Li, J., Liu, C., Müller, U., and Zhao, B. (2022). RIPOR2-mediated autophagy dysfunction is critical for aminoglycoside-induced hearing loss. Dev. Cell 57 (18), 2204–2220.e6. doi:10.1016/j.devcel.2022.08.011
Liu, L., Chen, Y., Qi, J., Zhang, Y., He, Y., Ni, W., et al. (2016). Wnt activation protects against neomycin-induced hair cell damage in the mouse cochlea. Cell Death Dis. 7 (3), e2136. doi:10.1038/cddis.2016.35
Liu, Y. H., Ke, X. M., Qin, Y., Gu, Z. P., and Xiao, S. F. (2007). Adeno-associated virus-mediated Bcl-xL prevents aminoglycoside-induced hearing loss in mice. Chin. Med. J. Engl. 120 (14), 1236–1240. doi:10.1097/00029330-200707020-00006
Maeder, M. L., Stefanidakis, M., Wilson, C. J., Baral, R., Barrera, L. A., Bounoutas, G. S., et al. (2019). Development of a gene-editing approach to restore vision loss in Leber congenital amaurosis type 10. Nat. Med. 25 (2), 229–233. doi:10.1038/s41591-018-0327-9
Margolis, R. L., Chuang, D. M., and Post, R. M. (1994). Programmed cell death: implications for neuropsychiatric disorders. Biol. Psychiatry 35 (12), 946–956. doi:10.1016/0006-3223(94)91241-6
Matsui, J. I., Haque, A., Huss, D., Messana, E. P., Alosi, J. A., Roberson, D. W., et al. (2003). Caspase inhibitors promote vestibular hair cell survival and function after aminoglycoside treatment in vivo. J. Neurosci. 23 (14), 6111–6122. doi:10.1523/jneurosci.23-14-06111.2003
McIlwain, D. R., Berger, T., and Mak, T. W. (2013). Caspase functions in cell death and disease. Cold Spring Harb. Perspect. Biol. 5 (4), a008656. doi:10.1101/cshperspect.a008656
Mei, H., Zhao, L., Li, W., Zheng, Z., Tang, D., Lu, X., et al. (2020). Inhibition of ferroptosis protects House Ear Institute-Organ of Corti 1 cells and cochlear hair cells from cisplatin-induced ototoxicity. J. Cell Mol. Med. 24 (20), 12065–12081. doi:10.1111/jcmm.15839
Myrdal, S. E., and Steyger, P. S. (2005). TRPV1 regulators mediate gentamicin penetration of cultured kidney cells. Hear Res. 204 (1-2), 170–182. doi:10.1016/j.heares.2005.02.005
Nagata, S., and Tanaka, M. (2017). Programmed cell death and the immune system. Nat. Rev. Immunol. 17 (5), 333–340. doi:10.1038/nri.2016.153
Nakamagoe, M., Tabuchi, K., Uemaetomari, I., Nishimura, B., and Hara, A. (2010). Estradiol protects the cochlea against gentamicin ototoxicity through inhibition of the JNK pathway. Hear Res. 261 (1-2), 67–74. doi:10.1016/j.heares.2010.01.004
Narendra, D., Tanaka, A., Suen, D. F., and Youle, R. J. (2009). Parkin-induced mitophagy in the pathogenesis of Parkinson disease. Autophagy 5 (5), 706–708. doi:10.4161/auto.5.5.8505
Navarro-Yepes, J., Burns, M., Anandhan, A., Khalimonchuk, O., del Razo, L. M., Quintanilla-Vega, B., et al. (2014). Oxidative stress, redox signaling, and autophagy: cell death versus survival. Antioxid. Redox Signal. 21 (1), 66–85. doi:10.1089/ars.2014.5837
Nilius, B., and Szallasi, A. (2014). Transient receptor potential channels as drug targets: from the science of basic research to the art of medicine. Pharmacol. Rev. 66 (3), 676–814. doi:10.1124/pr.113.008268
Nyberg, S., Abbott, N. J., Shi, X., Steyger, P. S., and Dabdoub, A. (2019). Delivery of therapeutics to the inner ear: the challenge of the blood-labyrinth barrier. Sci. Transl. Med. 11 (482), eaao0935. doi:10.1126/scitranslmed.aao0935
Okuda, T., Sugahara, K., Takemoto, T., Shimogori, H., and Yamashita, H. (2005). Inhibition of caspases alleviates gentamicin-induced cochlear damage in Guinea pigs. Auris Nasus Larynx 32 (1), 33–37. doi:10.1016/j.anl.2004.11.006
Olusanya, B. O., Davis, A. C., and Hoffman, H. J. (2019). Hearing loss: rising prevalence and impact. Bull. World Health Organ 97 (10), 646–646A. doi:10.2471/blt.19.224683
Omichi, R., Shibata, S. B., Morton, C. C., and Smith, R. J. H. (2019). Gene therapy for hearing loss. Hum. Mol. Genet. 28 (R1), R65–R79. doi:10.1093/hmg/ddz129
O'Sullivan, M. E., Perez, A., Lin, R., Sajjadi, A., Ricci, A. J., and Cheng, A. G. (2017). Towards the prevention of aminoglycoside-related hearing loss. Front. Cell Neurosci. 11, 325. doi:10.3389/fncel.2017.00325
Pfannenstiel, S. C., Praetorius, M., Plinkert, P. K., Brough, D. E., and Staecker, H. (2009). Bcl-2 gene therapy prevents aminoglycoside-induced degeneration of auditory and vestibular hair cells. Audiol. Neurootol. 14 (4), 254–266. doi:10.1159/000192953
Pickles, S., Vigié, P., and Youle, R. J. (2018). Mitophagy and quality control mechanisms in mitochondrial maintenance. Curr. Biol. 28 (4), R170–R185. doi:10.1016/j.cub.2018.01.004
Pogue, J. M., Kaye, K. S., Veve, M. P., Patel, T. S., Gerlach, A. T., Davis, S. L., et al. (2020). Ceftolozane/tazobactam vs polymyxin or aminoglycoside-based regimens for the treatment of drug-resistant pseudomonas aeruginosa. Clin. Infect. Dis. 71 (2), 304–310. doi:10.1093/cid/ciz816
Ramekers, D., Versnel, H., Grolman, W., and Klis, S. F. (2012). Neurotrophins and their role in the cochlea. Hear Res. 288 (1-2), 19–33. doi:10.1016/j.heares.2012.03.002
Rodríguez-Muela, N., Germain, F., Mariño, G., Fitze, P. S., and Boya, P. (2012). Autophagy promotes survival of retinal ganglion cells after optic nerve axotomy in mice. Cell Death Differ. 19 (1), 162–169. doi:10.1038/cdd.2011.88
Ruan, R. S., Leong, S. K., Mark, I., and Yeoh, K. H. (1999). Effects of BDNF and NT-3 on hair cell survival in Guinea pig cochlea damaged by kanamycin treatment. Neuroreport 10 (10), 2067–2071. doi:10.1097/00001756-199907130-00014
Ruhl, D., Du, T. T., Wagner, E. L., Choi, J. H., Li, S., Reed, R., et al. (2019). Necroptosis and apoptosis contribute to cisplatin and aminoglycoside ototoxicity. J. Neurosci. 39 (15), 2951–2964. doi:10.1523/jneurosci.1384-18.2019
Sacks, D., Baxter, B., Campbell, B. C. V., Carpenter, J. S., Cognard, C., Dippel, D., et al. (2018). Multisociety consensus quality improvement revised consensus statement for endovascular therapy of acute ischemic stroke. Int. J. Stroke 13 (6), 612–632. doi:10.1177/1747493018778713
Santiago-Fernández, O., Osorio, F. G., Quesada, V., Rodríguez, F., Basso, S., Maeso, D., et al. (2019). Development of a CRISPR/Cas9-based therapy for Hutchinson-Gilford progeria syndrome. Nat. Med. 25 (3), 423–426. doi:10.1038/s41591-018-0338-6
Sha, S. H., Qiu, J. H., and Schacht, J. (2006). Aspirin to prevent gentamicin-induced hearing loss. N. Engl. J. Med. 354 (17), 1856–1857. doi:10.1056/NEJMc053428
Shen, H. M., and Liu, Z. G. (2006). JNK signaling pathway is a key modulator in cell death mediated by reactive oxygen and nitrogen species. Free Radic. Biol. Med. 40 (6), 928–939. doi:10.1016/j.freeradbiomed.2005.10.056
Shen, Z., Song, J., Yung, B. C., Zhou, Z., Wu, A., and Chen, X. (2018). Emerging strategies of cancer therapy based on ferroptosis. Adv. Mat. 30 (12), e1704007. doi:10.1002/adma.201704007
Shibata, S. B., Ranum, P. T., Moteki, H., Pan, B., Goodwin, A. T., Goodman, S. S., et al. (2016). RNA interference prevents autosomal-dominant hearing loss. Am. J. Hum. Genet. 98 (6), 1101–1113. doi:10.1016/j.ajhg.2016.03.028
Shmakov, S., Abudayyeh, O. O., Makarova, K. S., Wolf, Y. I., Gootenberg, J. S., Semenova, E., et al. (2015). Discovery and functional characterization of diverse class 2 CRISPR-Cas systems. Mol. Cell 60 (3), 385–397. doi:10.1016/j.molcel.2015.10.008
Shu, Y., Li, W., Huang, M., Quan, Y. Z., Scheffer, D., Tian, C., et al. (2019). Renewed proliferation in adult mouse cochlea and regeneration of hair cells. Nat. Commun. 10 (1), 5530. doi:10.1038/s41467-019-13157-7
Smargon, A. A., Cox, D. B. T., Pyzocha, N. K., Zheng, K., Slaymaker, I. M., Gootenberg, J. S., et al. (2017). Cas13b is a type VI-B CRISPR-associated RNA-guided RNase differentially regulated by accessory proteins Csx27 and Csx28. Mol. Cell 65 (4), 618–630. doi:10.1016/j.molcel.2016.12.023
Smyth, A. R., and Bhatt, J. (2014). Once-daily versus multiple-daily dosing with intravenous aminoglycosides for cystic fibrosis. Cochrane Database Syst. Rev. 2, Cd002009. doi:10.1002/14651858.CD002009.pub5
Song, B. B., and Schacht, J. (1996). Variable efficacy of radical scavengers and iron chelators to attenuate gentamicin ototoxicity in Guinea pig in vivo. Hear Res. 94 (1-2), 87–93. doi:10.1016/0378-5955(96)00003-2
Stadtmauer, E. A., Fraietta, J. A., Davis, M. M., Cohen, A. D., Weber, K. L., Lancaster, E., et al. (2020). CRISPR-engineered T cells in patients with refractory cancer. Science 367 (6481), eaba7365. doi:10.1126/science.aba7365
Stepanyan, R. S., Indzhykulian, A. A., Vélez-Ortega, A. C., Boger, E. T., Steyger, P. S., Friedman, T. B., et al. (2011). TRPA1-mediated accumulation of aminoglycosides in mouse cochlear outer hair cells. J. Assoc. Res. Otolaryngol. 12 (6), 729–740. doi:10.1007/s10162-011-0288-x
Sun, S., Sun, M., Zhang, Y., Cheng, C., Waqas, M., Yu, H., et al. (2014). In vivo overexpression of X-linked inhibitor of apoptosis protein protects against neomycin-induced hair cell loss in the apical turn of the cochlea during the ototoxic-sensitive period. Front. Cell Neurosci. 8, 248. doi:10.3389/fncel.2014.00248
Sykiotis, G. P., and Bohmann, D. (2010). Stress-activated cap'n'collar transcription factors in aging and human disease. Sci. Signal. 3 (112), re3. doi:10.1126/scisignal.3112re3
Szegezdi, E., Logue, S. E., Gorman, A. M., and Samali, A. (2006). Mediators of endoplasmic reticulum stress-induced apoptosis. EMBO Rep. 7 (9), 880–885. doi:10.1038/sj.embor.7400779
Tanaka, M., Hasegawa, S., Nakao, S., Shimada, K., Mukai, R., Matsumoto, K., et al. (2019). Analysis of drug-induced hearing loss by using a spontaneous reporting system database. PLoS One 14 (10), e0217951. doi:10.1371/journal.pone.0217951
Tao, L., and Segil, N. (2022). CDK2 regulates aminoglycoside-induced hair cell death through modulating c-Jun activity: inhibiting CDK2 to preserve hearing. Front. Mol. Neurosci. 15, 1013383. doi:10.3389/fnmol.2022.1013383
Unsain, N., Higgins, J. M., Parker, K. N., Johnstone, A. D., and Barker, P. A. (2013). XIAP regulates caspase activity in degenerating axons. Cell Rep. 4 (4), 751–763. doi:10.1016/j.celrep.2013.07.015
Vu, A. A., Nadaraja, G. S., Huth, M. E., Luk, L., Kim, J., Chai, R., et al. (2013). Integrity and regeneration of mechanotransduction machinery regulate aminoglycoside entry and sensory cell death. PLoS One 8 (1), e54794. doi:10.1371/journal.pone.0054794
Wang, M., Dong, Y., Gao, S., Zhong, Z., Cheng, C., Qiang, R., et al. (2022). Hippo/YAP signaling pathway protects against neomycin-induced hair cell damage in the mouse cochlea. Cell Mol. Life Sci. 79 (2), 79. doi:10.1007/s00018-021-04029-9
Willis, S., Day, C. L., Hinds, M. G., and Huang, D. C. (2003). The Bcl-2-regulated apoptotic pathway. J. Cell Sci. 116 (Pt 20), 4053–4056. doi:10.1242/jcs.00754
Wise, A. K., Hume, C. R., Flynn, B. O., Jeelall, Y. S., Suhr, C. L., Sgro, B. E., et al. (2010). Effects of localized neurotrophin gene expression on spiral ganglion neuron resprouting in the deafened cochlea. Mol. Ther. 18 (6), 1111–1122. doi:10.1038/mt.2010.28
Wu, J., Ye, J., Kong, W., Zhang, S., and Zheng, Y. (2020). Programmed cell death pathways in hearing loss: a review of apoptosis, autophagy and programmed necrosis. Cell Prolif. 53 (11), e12915. doi:10.1111/cpr.12915
Wu, W. J., Sha, S. H., McLaren, J. D., Kawamoto, K., Raphael, Y., and Schacht, J. (2001). Aminoglycoside ototoxicity in adult CBA, C57BL and BALB mice and the Sprague-Dawley rat. Hear Res. 158 (1-2), 165–178. doi:10.1016/s0378-5955(01)00303-3
Wu, X., Li, Y., Zhang, S., and Zhou, X. (2021). Ferroptosis as a novel therapeutic target for cardiovascular disease. Theranostics 11 (7), 3052–3059. doi:10.7150/thno.54113
Wu, Y., Zeng, J., Roscoe, B. P., Liu, P., Yao, Q., Lazzarotto, C. R., et al. (2019). Highly efficient therapeutic gene editing of human hematopoietic stem cells. Nat. Med. 25 (5), 776–783. doi:10.1038/s41591-019-0401-y
Xie, Y., Hou, W., Song, X., Yu, Y., Huang, J., Sun, X., et al. (2016). Ferroptosis: process and function. Cell Death Differ. 23 (3), 369–379. doi:10.1038/cdd.2015.158
Xue, Y., Hu, X., Wang, D., Li, D., Li, Y., Wang, F., et al. (2022). Gene editing in a Myo6 semi-dominant mouse model rescues auditory function. Mol. Ther. 30 (1), 105–118. doi:10.1016/j.ymthe.2021.06.015
Yang, Q., Zhou, Y., Yin, H., Li, H., Zhou, M., Sun, G., et al. (2018). PINK1 protects against gentamicin-induced sensory hair cell damage: possible relation to induction of autophagy and inhibition of p53 signal pathway. Front. Mol. Neurosci. 11, 403. doi:10.3389/fnmol.2018.00403
Yang, Y., Chen, X., Tian, K., Tian, C., Chen, L., Mi, W., et al. (2022). Heme oxygenase-1 protects hair cells from gentamicin-induced death. Front. Cell Neurosci. 16, 783346. doi:10.3389/fncel.2022.783346
Ye, B., Wang, Q., Hu, H., Shen, Y., Fan, C., Chen, P., et al. (2019). Restoring autophagic flux attenuates cochlear spiral ganglion neuron degeneration by promoting TFEB nuclear translocation via inhibiting MTOR. Autophagy 15 (6), 998–1016. doi:10.1080/15548627.2019.1569926
Ylikoski, J., Xing-Qun, L., Virkkala, J., and Pirvola, U. (2002). Blockade of c-Jun N-terminal kinase pathway attenuates gentamicin-induced cochlear and vestibular hair cell death. Hear Res. 163 (1-2), 71–81. doi:10.1016/s0378-5955(01)00380-x
Zhang, Y., Fang, Q., Wang, H., Qi, J., Sun, S., Liao, M., et al. (2023). Increased mitophagy protects cochlear hair cells from aminoglycoside-induced damage. Autophagy 19 (1), 75–91. doi:10.1080/15548627.2022.2062872
Zhang, Z., Wang, J., Li, C., Xue, W., Xing, Y., and Liu, F. (2020). Gene therapy development in hearing research in China. Gene Ther. 27 (7-8), 349–359. doi:10.1038/s41434-020-0177-1
Keywords: hair cell, ototoxicity, aminoglycosides, programmed cell death, gene therapy
Citation: Han L, Wang Z, Wang D, Gao Z, Hu S, Shi D and Shu Y (2024) Mechanisms and otoprotective strategies of programmed cell death on aminoglycoside-induced ototoxicity. Front. Cell Dev. Biol. 11:1305433. doi: 10.3389/fcell.2023.1305433
Received: 01 October 2023; Accepted: 14 December 2023;
Published: 08 January 2024.
Edited by:
Yinan Gong, University of Pittsburgh, United StatesCopyright © 2024 Han, Wang, Wang, Gao, Hu, Shi and Shu. This is an open-access article distributed under the terms of the Creative Commons Attribution License (CC BY). The use, distribution or reproduction in other forums is permitted, provided the original author(s) and the copyright owner(s) are credited and that the original publication in this journal is cited, in accordance with accepted academic practice. No use, distribution or reproduction is permitted which does not comply with these terms.
*Correspondence: Dazhi Shi, MjA3MDg0OTVAcXEuY29t; Yilai Shu, eWlsYWlfc2h1QGZ1ZGFuLmVkdS5jbg==
†These authors have contributed equally to this work and share first authorship