- 1Molecular Biology and Viral Oncology Unit, Istituto Nazionale Tumori IRCCS Fondazione G. Pascale, Napoli, Italy
- 2Innovative Immunological Models Unit, Istituto Nazionale Tumori IRCCS Fondazione G. Pascale, Napoli, Italy
Telomerase activity and telomere elongation are essential conditions for the unlimited proliferation of neoplastic cells. Point mutations in the core promoter region of the telomerase reverse transcriptase (TERT) gene have been found to occur at high frequencies in several tumour types and considered a primary cause of telomerase reactivation in cancer cells. These mutations promote TERT gene expression by multiple mechanisms, including the generation of novel binding sites for nuclear transcription factors, displacement of negative regulators from DNA G-quadruplexes, recruitment of epigenetic activators and disruption of long-range interactions between TERT locus and telomeres. Furthermore, TERT promoter mutations cooperate with TPP1 promoter nucleotide changes to lengthen telomeres and with mutated BRAF and FGFR3 oncoproteins to enhance oncogenic signalling in cancer cells. TERT promoter mutations have been recognized as an early marker of tumour development or a major indicator of poor outcome and reduced patients survival in several cancer types. In this review, we summarize recent findings on the role of TERT promoter mutations, telomerase expression and telomeres elongation in cancer development, their clinical significance and therapeutic opportunities.
1 Introduction
Cancer cells acquire replicative immortality through a multistep process involving progressive accumulation of genetic and epigenetic changes, escape from replicative senescence and neoplastic transformation (Hanahan and Weinberg, 2011). Telomerase activity, commonly low or undetectable in normal somatic cells, is frequently reactivated in cancer cells causing elongation of telomeres at chromosomes ends and loss of the Hayflick limit (Shay and Wright, 2000; Jafri et al., 2016). Telomerase is a ribonucleoprotein complex formed by the RNA template component (TERC) and a tetrad of proteins including dyskerin, GAR1, NHP2 and NOP10 as well as the reverse transcriptase holoenzyme TERT (Figure 1), (Smith et al., 2020). Furthermore, a protein complex formed by shelterin, POT1, RAP1, TIN2, TPP1, TRF1 and TRF2 contributes to the maintenance of telomeres by protecting terminal tandem repeats from DNA damage response (Smith et al., 2020). The level of TERT, its subcellular localization and assembly with telomeres, represent the limiting factors of telomerase activity, since all other associated components are constitutively expressed in both normal and neoplastic cells (Cristofari and Lingner, 2006).
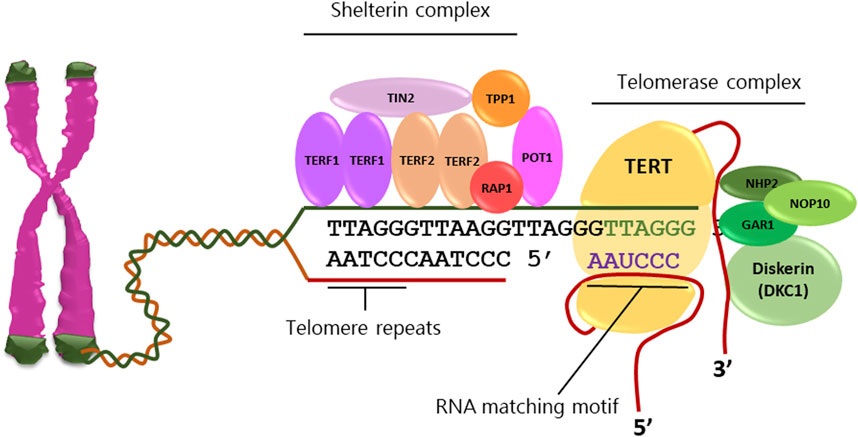
FIGURE 1. Telomeres are tandem repeats of nucleotide sequences (TTAGGG) protecting the chromosome ends from constitutive exposure to the DNA damage response, which are elongated by telomerase activity. Telomerase complex comprises the RNA template component (TERC), dyskerin, GAR1, NHP2, and NOP10 proteins as well as the reverse transcriptase holoenzyme TERT. The shelterin complex stabilizes telomeres through the direct binding of TERF1 and TERF2 homodimers as well as POT1 to telomeric sequences and stabilization by TIN2 and TPP1.
Telomerase activity is upregulated in neoplastic cells through multiple mechanisms including chromosomal rearrangements, amplification of TERT gene, generation of TERT structural variants, disruption of telomere position effect and activating mutations in TERT promoter (Zhao et al., 2009; Peifer et al., 2015; Barthel et al., 2017). Epigenetic factors causing DNA methylation and histone acetylation also contribute to abnormal regulation of TERT transcription (Dogan and Forsyth, 2021). In addition, virus-related cancers, which commonly require constitutive expression of viral oncoproteins to sustain cell transformation, are characterized by high levels of telomerase activity. In particular, oncoviral proteins, such as high-risk human papillomavirus (HPV) E6, Epstein-Barr virus (EBV) LMP1, Kaposi’s sarcoma-associated herpesvirus (HHV-8) LANA, hepatitis B virus (HBV) HBx, hepatitis C virus (HCV) core protein and human T-cell leukemia virus-1 (HTLV-1) Tax protein, have been demonstrated to contribute to the transcriptional activation of the TERT gene by interacting with negative regulators of TERT transcription (Tornesello et al., 2022a).
The TERT gene is located approximately one megabase from the end of the short arm of chromosome 5, and its core promoter is delimited by nucleotides located at positions −330 upstream and +37 downstream of the TERT ATG start site (Cong et al., 1999; Takakura et al., 1999). TERT gene expression is tightly controlled by several regulatory factors that interact with binding sites in the core promoter region, such as GC-box and E-box motifs (Ramlee et al., 2016; Yuan et al., 2019). These factors include among others E2F1 (Crowe et al., 2001), Ets2 (Xu et al., 2008), c-Myc (Wu et al., 1999), NF-kB (Sheng et al., 2006), NFX1-123 (Gewin et al., 2004), p27KIP1 (Kanzawa et al., 2003), Sp1 (Oh et al., 2001), STAT3 (Wang et al., 2011) and TGFβ/Snail axis (Yoo et al., 2015), which in normal cells are subjected to spatial and temporal transcription changes during development, cell renewal and differentiation processes. However, most of these factors are aberrantly produced in tumour cells and are responsible for the activation of oncogenic pathways through the uncontrolled de-repression of several genes involved in cell growth and division, including TERT (Liu et al., 2016; Lambert et al., 2018; Bushweller, 2019).
Activating mutations in the proximal promoter of the TERT gene were first reported in cutaneous melanoma by two independent studies, and have subsequently be shown to occur more frequently than any other carcinogenic mutation in different tumour types (Horn et al., 2013; Huang et al., 2013; Huang et al., 2015a). These single nucleotide changes are G>A transitions, mostly arising at positions −124 and −146 upstream of TERT ATG start site, which create de novo binding motifs for E-twenty-six transformation-specific (ETS) transcription factors (Bell et al., 2015; Liu et al., 2018; Mancini et al., 2018). Moreover, whole genome sequencing of 326 cell lines derived from different cancer types showed that 60 lineages harboured mutated TERT promoters with a mutation rate similar to that of the respective primary tumours (Huang et al., 2015b). Importantly, all of them showed that mRNA monoallelic expression is specifically transcribed by the TERT allele with mutated promoter. Only cancer cell lines presenting TERT promoter mutations exhibited the H3K4me2/3 active chromatin marks and recruitment of the GABPA/B1 transcription factor to the cognate binding sites. Therefore, these findings suggest that single nucleotide changes in the TERT promoter cause major epigenetic changes and monoallelic expression (Vogelstein et al., 2013; Huang et al., 2015b; Stern et al., 2015; Akincilar et al., 2016; Xu et al., 2018; Takeda et al., 2020).
Recent findings have shown that hotspot changes in the TERT promoter cooperate with mutations in the regulatory regions of other telomerase-related genes, such as the G>A transition in the promoter of the ACD gene, which encodes the TPP1 protein of the shelterin complex (Chun-On et al., 2022). Furthermore, specific mutations in the TERT promoter have been found to frequently coexist with BRAF V600E variation in melanoma and observed to affect the disease-free survival of cancer patients (Del Bia et al., 2020).
In this review, we summarize diverse molecular mechanisms involved in telomerase activation in cancer cells harbouring TERT promoter mutations, their relationship with disease outcome in patients with different cancer types as well as the importance of anticancer therapies targeting telomerase.
2 TERT promoter mutations and activation of telomerase expression
Mutations consisting of G>A transitions at positions −57, −124 and −146 in the core promoter region of the TERT gene were first reported to occur in nearly 70% of melanoma as well as in different cell lines derived from several cancer types (Horn et al., 2013; Huang et al., 2013). All of these mutations were found to induce increased transcriptional activity in reporter gene assays. A further study, including 1,230 cases of 60 different tumour types, demonstrated that tumours can be classified into types with low (<15%) and high (≥15%) frequencies of TERT promoter mutations (Killela et al., 2013; Vinagre et al., 2013). Tumours harbouring low rates of mutations included colon cancer, breast cancer, and pancreatic cancer. On the other hand, high rates of TERT promoter mutations were identified in some tumour types including glioblastoma, bladder cancer, cutaneous melanoma, hepatocellular carcinoma and squamous cell carcinoma (SCC) of the skin and oral mucosa (Huang et al., 2015a).
More recently, a pan-cancer analysis of 10,336 cancer cases performed with a custom next-generation sequencing gene panel (MSK-IMPACT™, Memorial Sloan Kettering Cancer Center Integrated Mutation Profiling of Actionable Cancer Targets) provided relevant information regarding the distribution of TERT promoter variations in diverse tumour types and histological subtypes (Zehir et al., 2017). The data deposited in the cBioPortal database have been analysed for the distribution of TERT promoter mutations in cancer subtypes with at least 10 cases and results reported in Table 1. The high prevalence of TERT promoter mutations was confirmed in most tumour types such as glioma, bladder cancer, oral cavity carcinoma as well as melanoma and non-melanoma skin cancers (Zehir et al., 2017).
2.1 Transcription factors and activation of mutant TERT promoter
TERT promoter mutations have been shown to activate telomerase expression through the creation of de novo 5′-GGAA-3′ binding motifs that are recognized by transcription factors of the E-twenty-six (ETS) family, including the GA-binding proteins alpha and beta (GABPA and GABPB), ETS1 and ETS Variant Transcription Factor 1 (ETV1) (Bell et al., 2015; Sizemore et al., 2017). In particular, the GABPA/B tetramer selectively binds and activates the mutant TERT promoter, thus inducing overexpression of the TERT gene in several tumour types, including glioblastoma, melanoma, hepatocellular carcinoma and bladder cancer (Figure 2), (Bell et al., 2015). TERT promoter mutations are mostly heterozygous, but only the mutant alleles recruit GABPA/B and exhibit epigenetic changes such as active histone marks (H3K4me2/3 and H3K9ac) as well as modifications in the chromatin architecture (Stern et al., 2015).
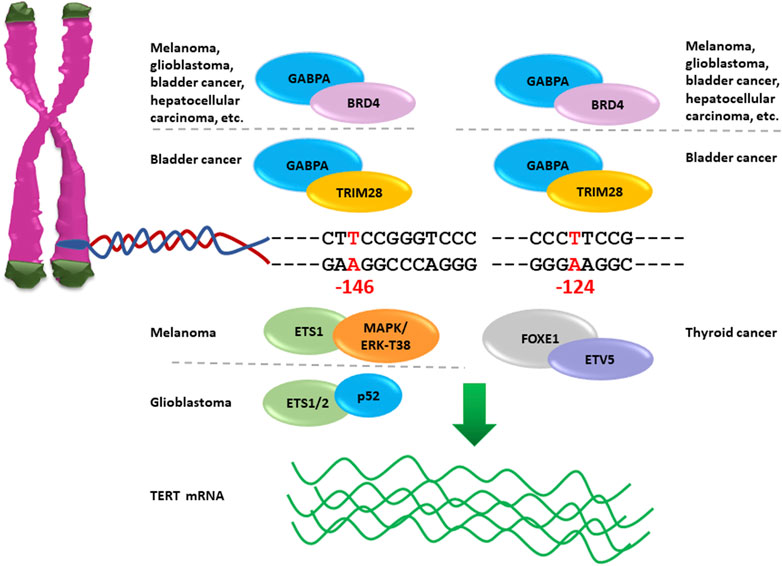
FIGURE 2. Nucleotide changes −124A and −146A in TERT promoter create novel binding sites for ETS factors reactivating telomerase expression. GABPA interacts with BRD4 and activates mutant TERT promoter −124A or −146A in several cancer types; NF-κB p52 recruits ETS1/2 to TERT promoter −146A causing reactivation of TERT gene expression in glioblastoma; the complex MAPK/ERK cause activation of TERT promoter −146A via the phosphorylation of ETS1 Thr38 amino acid in melanoma; FOXE1in combination with ETV5 activates TERT promoter −124A in thyroid cancer, and TRIM28 with GABPA is recruited on TERT promoter −124A and −146A to activate telomerase expression in bladder cancer.
Three-dimensional (3D) organization of the genome represent an important mechanism by which regulatory elements control epigenetic modifications and gene expression (Dekker et al., 2013; Kim and Shay, 2018). The binding of GABPA dimers to mutant TERT promoters play a key mechanism in the formation of long-range TERT enhancer-promoter interactions and 3D chromatin reorganization. The transcription factor GABPA enables the interaction of mutant TERT promoter with a distal region (T-INT1) located 260 kb upstream TERT ATG start site and facilitates epigenetic changes that drive TERT gene transcription (Akincilar et al., 2016). Reversal of TERT promoter mutations, or deletion of T-INT1 region abrogates the GABPA binding to TERT promoter, depletes H3K4Me3 and H3K9Ac marks and leads to TERT gene repression. Therefore, 3D-chromatin organization is crucial factor for the activation of mutant TERT promoters (Akincilar et al., 2016).
Duplication of GABP binding sites in TERT promoter have been shown to cause the increase of telomerase expression similarly to the hot spot nucleotide changes (Barger et al., 2022). Duplications of a wild type sequence within the TERT promoter region, that include an ETS motif, have been identified in seven cancer types. All duplications had similar length ranging from 21 to 25 base pairs and insertion site, they recruit the GABP tetramer and activate the telomerase expression similarly to the single nucleotide mutations. Such findings suggest a strong selection for the recruitment of GABP to activate TERT expression (Barger et al., 2022).
Moreover, the complex formed by ETS1 with p52 NF-κB, whose expression is specifically controlled by non-canonical NF-κB signalling, has been shown to bind selectively the mutant TERT promoter −146A thus promoting telomerase expression in glioblastoma and melanoma (Li et al., 2015; Vallarelli et al., 2016). The ETS1 activity is dependent on the phosphorylation of Thr38 residue mediated by ERK, which is a component of the mitogen-activated protein kinase (MAPK) cascade permanently activated in BRAF or NRAS mutated tumours. Of note, BRAF variations coexist with TERT promoter mutations both in melanoma and in thyroid cancer (Dubbink et al., 2014; Liu et al., 2014; Xing et al., 2014; Shi et al., 2015; Jin et al., 2016). Importantly, melanoma cells harbouring the BRAFV600E mutation are characterized by constitutive activation of the RAS-ERK signalling pathway, which promotes recruitment of ERK2/GABP or ERK2/ETS1 complexes on the mutant TERT promoter, phosphorylation of SP1, shift in HDAC1 occupancy, acetylation of histones H3K9, and reactivation of telomerase (Shi et al., 2015).
Recent studies have identified activating mutations in the promoter region of the ACD gene co-occurring with TERT promoter mutations in 5% of cutaneous melanoma (Chun-On et al., 2022). The ACD gene generates two independent transcripts encoding a long and a short form of tripeptidyl peptidase 1 (TPP1), named TPP1-L and TPP1-S, respectively, which are involved in telomerase recruitment to telomeres and DNA synthesis (Grill et al., 2019). The TPP1-L isoform differs from TPP1-S by an arginine-rich region of 86 amino acids at its N-terminus and both retain the ability to recruit telomerase, but only TPP1-L inhibits telomerase function while TPP1-S facilitates efficient telomere synthesis. Mutations in the ACD gene are localized at nucleotide positions such that they cause amino acid changes in TPP1-L and at the same time generate new binding sites for ETS transcription factors, such as ETV5, ETV4 or ETS1, in the region functioning as promoter for the expression of TPP1-S (Chun-On et al., 2022). Importantly, such mutations drive the overexpression of TPP1-S, which in the presence of mutant TERT promoter and increased TERT levels lead to synergistic telomere lengthening and cooperate in the indefinite proliferation of melanoma cells (Chun-On et al., 2022).
In papillary thyroid cancers the ETS variant 5 (ETV5) is abundantly expressed and able to activate mutant TERT promoter in GABP-negative thyroid cancer cells (Chun-On et al., 2022). In addition, the ETV5 has been shown to interact with FOXE1, which is a thyroid-specific transcription factor crucial for thyroid development and differentiation, and to bind the mutant TERT promoter −124A causing the reactivation of telomerase expression (Bullock et al., 2019). Moreover, the co-existence of BRAFV600E and TERT promoter mutations in papillary thyroid cancer has been shown to have a synergistic effect on poor clinical outcome, probably due to the BRAF-dependent upregulation of several ETS transcription factors, especially ETV1, ETV4 and ETV5, causing telomerase over-expression associated with increased cancer invasiveness and progression (Song et al., 2019a).
In bladder cancer, GABPA is involved in the recruitment of two transcriptional co-regulators of telomerase expression, namely, TRIM28 (tripartite motif containing 28) and TRIM24, to the mutant TERT promoter −124A (Agarwal et al., 2021). The transcription factor TRIM28 is an activator while TRIM24 is a repressor of TERT expression. Hyperactivation of TERT promoter −124A in bladder cancer cells is caused by phosphorylation of TRIM28, mediated by the mTORC1 (mammalian target of rapamycin complex 1), which abrogates the TRIM28/TRIM24 interaction leading to displacement of the repressor and causing significant upregulation of TERT expression (Agarwal et al., 2021).
2.2 Telomere proteins and activation of mutant TERT promoter
Proteins of the shelterin complex, besides protecting telomeres from unwanted DNA repair processes, have a major role in the formation of loops and regulation of telomerase expression. Indeed, telomeric factors such as TRF1, TRF2 and RAP1 have the ability to interact with non-telomeric binding sites, such as TERT promoter sequences (Mukherjee et al., 2019). Recent studies have demonstrated that TRF2 binds the TERT promoter G-quadruplex and recruits the polycomb-repressor EZH2/PRC2 complex causing H3K27 trimethylation and TERT promoter repression both in cancer and normal cells (Sharma et al., 2021). However, mutations in the TERT promoter cause destabilization of G-quadruplex structures and TRF2 displacement resulting in telomerase overexpression in glioblastoma multiforme cells. These results showed that the telomeric factor TRF2 is a negative regulator of telomerase activity, but its function is hampered by the presence of mutations in the TERT promoter region.
3 Temporal and spatial acquisition of TERT promoter mutations
Somatic mutations in the TERT promoter have been identified with variable frequencies in numerous cancer types (Sharma and Chowdhury, 2022). Highest rate of mutations have been detected in several tumours including among others glioma (>70%), melanoma and non-melanoma skin cancers (>70%), hepatocellular carcinoma (>60%), bladder cancer (>60%) as well as squamous cell carcinoma of the penis (>50%), conjunctiva (>45%) and oral cavity (>30%), (Huang et al., 2015a; Pezzuto et al., 2017; Annu et al., 2018; Starita et al., 2018; Giunco et al., 2021; Starita et al., 2022). Studies on the clonality of mutations in the TERT promoter have contributed to define their temporal appearance and the oncogenic consequences. Indeed, these mutations have been defined as early events in some tumours and late events in others, consistent with the possibility that they play multiple oncogenic roles in diverse cancer types (Lorbeer and Hockemeyer, 2020).
In glioblastoma, TERT promoter mutations are considered early genetic events of tumour development. Lee et al. (2018) by performing a comparison study on the presence of TERT promoter mutations in brain tissues from matched tumour-free sub-ventricular zone, tumour and normal tissue, identified TERT promoter mutations and other oncogenic mutations in non-tumour tissues. Single-cell sequencing of a glioblastoma and its single-cell-derived clonal cell populations demonstrated that all clones had both private mutations as well as shared mutations with the correspondent tumour-free sub-ventricular zone, according to the model of progressive accumulation of driver mutations in cancer cells.
Benign melanocytic nevi harbour trunk nucleotide changes, such as BRAF and NRAS mutations, before progressing to melanoma and accumulate nucleotide changes in TERT promoter at the transition from benign nevus to melanoma in situ (Damsky and Bosenberg, 2017; Colebatch et al., 2022). Recently, Ciba et al. reported that TERT promoter mutations elicit low levels of telomerase expression in the first stage of melanoma development, which correlate with cell proliferation and healing of short telomeres but not with telomeres elongation (Chiba et al., 2017). The continuous cell division and critically short telomeres promote the transition to a second stage of neoplastic transformation involving genomic instability, progressive increase in telomerase levels and overgrowth of transformed melanoma clones (Chiba et al., 2017). The coexistence of mutations in BRAF and NRAS genes as well as in TERT promoter are associated with poor disease-free survival in melanoma patients (Nagore et al., 2016). Particularly, nucleotide changes at positions −138_139 CC>TT in TERT promoter have been shown to correlate with poorer clinical outcome in stage I and stage II melanoma patients, mainly in association with BRAF and NRAS mutations (Andrés-Lencina et al., 2019).
In primary hepatocellular carcinoma, the TERT promoter mutation −124A is the most frequent genetic alteration in HCV-related and metabolic-related tumours and represents approximately 95% of all TERT promoter variations (Müller et al., 2020). Nucleotide changes in the TERT promoter are considered trunk mutations in liver carcinogenesis, being identified in a consistent fraction of dysplastic nodules and at increased frequency in hepatocellular carcinoma (Nault et al., 2013; Totoki et al., 2014; Takeda et al., 2020; Fredriksson et al., 2014; Nault et al., 2014). In addition, the genetic landscape of liver cancer, characterized by nodule-in-nodule feature for the presence of the inner hypervascular tissue surrounded by hypovascular transformed hepatocytes, showed that the two components shared many clonal genetic events, including TERT promoter mutations (Takeda et al., 2020). Moreover, Ningarhari et al. (2021) reported that nucleotide variations in the TERT promoter were more frequent in the subtype of hepatocellular carcinoma characterized by short telomeres, well-moderate differentiation, and low levels of alpha-fetoprotein, rare vascular invasion, chromosomal stability and lower aggressiveness compared to non-mutated tumours. Detection of TERT promoter mutations in circulating tumour DNA of patients with hepatocellular carcinoma is associated with shorter survival and proposed as useful biomarker of poor prognosis in patients with advanced liver cancers (Pezzuto et al., 2018; Ge et al., 2021; Hirai et al., 2021).
Recurrent mutations in the TERT promoter have been detected in over 60% of urothelial bladder cancers and represent the most frequent molecular alterations in all grades and stages of urothelial cancer (Günes et al., 2018). Such mutations represent early events in the transformation process since they are detected in the urinary exfoliate cells decades before the diagnosis of bladder cancer. Indeed, in the Golestan cohort followed for up to 14 years, Hosen et al. identified the presence of TERT promoter mutations in urine samples of 47% asymptomatic individuals, who subsequently developed primary bladder cancer, and in none of matched controls who did not develop bladder cancer (Hosen et al., 2020). Nucleotide changes in TERT promoter were detectable up to 10 years prior to bladder cancer diagnosis. Then, identification of TERT promoter mutations in urinary exfoliated cells has emerged as a promising biomarker for early diagnosis of bladder cancer.
Squamous cell carcinoma of the conjunctiva is a common cancer in equatorial Africa mainly associated with exposure to solar ultraviolet and infection with human immunodeficiency virus (HIV). The frequency of TERT promoter mutations in intraepithelial neoplasia grade 1-3 (CIN1-3) and squamous cell carcinoma of the conjunctiva was significantly higher in HIV-positive (between 32% and 46%) compared to HIV-negative patients (between 13% and 22%) (Starita et al., 2018). These findings showed that TERT promoter mutations are early events in conjunctival neoplasia and that the immunosuppression caused by HIV infection strongly contribute to the accumulation of UV-related mutations in conjunctival lesions (Starita et al., 2018).
Variable frequencies of TERT promoter mutations have been detected in diverse tumour types of the lower genital tract, associated or not with human papillomavirus infection. Two independent studies performed in India and in Italy showed that 20% of cervical squamous cell carcinoma harboured TERT promoter mutations independently from the HPV status (Vinothkumar et al., 2016; Annu et al., 2018). The consistent association of TERT promoter mutations with cervical carcinoma is also supported by the presence of the G to A transition at position −146 in the HPV16-positive SiHa cell line, which is derived from a squamous cell carcinoma of the cervix (Annunziata et al., 2018). Notably, TERT mRNA expression levels were much higher in SiHa cells than in squamous cell carcinoma-derived CaSki and C-4I cells, as well as adenocarcinoma-derived HeLa cells, despite comparable expression levels of HPV E6. These data demonstrate that TERT promoter mutations have a stronger effect than HPV E6 transactivation on TERT gene expression. However, the rarity of recurrent TERT promoter mutations in CIN1-3 and adenocarcinoma of the cervix suggested that this is a late event in the development of cervical cancer.
Squamous cell carcinoma of the vulva originates from two different etiopathogenic pathways, one associated with human papillomavirus (HPV) infection and the other in the setting of longstanding dermatosis, such as lichen sclerosus (van der Avoort et al., 2006; Del Pino et al., 2013). Overall, TERT promoter mutations are highly frequent in the carcinoma of the vulva (>50%) particularly in HPV-negative tumours were the mutation rate exceeds 90% and coexists with other genetic alterations such as mutations in TP53 (87%), alteration in CDKN2A (67%), and mutations in NOTCH1 and FAT1 (47% each) (Salama et al., 2022). It remain to be clarified whether TERT promoter mutations are early or late events in HPV-independent squamous cell carcinoma of the vulva.
Similarly to vulvar cancer, penile squamous cell carcinoma either develop through an HPV associated pathway or through other oncogenic mechanisms independent from the HPV infection (Iorga et al., 2020). The search for TERT promoter mutations in penile carcinoma showed an overall prevalence above 50%, which was significantly higher in HPV negative cases (>65%) (Kim et al., 2021; Starita et al., 2022). Mutations in TERT promoter co-exited with nucleotide changes in PIK3CA gene in several cases. However, the frequency of mutant alleles showed discordant values indicating that PIK3CA mutations appear in subclones of TERT promoter mutated cells (Starita et al., 2022). Thereby, it is possible to conclude that TERT promoter mutations in penile carcinoma precedes the occurrence of other oncogenic mutations.
Squamous cell carcinoma of head and neck comprises a heterogeneous group of tumours mainly localized in the oral cavity, larynx, oropharynx, hypopharynx and nasopharynx. According to a recent systematic review and meta-analysis including 1830 patients, the overall prevalence of TERT promoter mutations in head and neck cancer was 21% with significant differences according to cancer site (Boscolo-Rizzo et al., 2023). Indeed, TERT promoter mutations are frequently detected in tumours of the oral cavity (47%) and larynx/hypopharynx (12%), while they are rare in squamous cell carcinoma of the oropharynx (1%). Notably, TERT promoter mutation −124A is the most frequent mutation and is associated with higher risk of disease progression and death while the mutation −146A has no significant correlation with disease outcome. The search for the mutation −124A should be considered as a promising diagnostic and prognostic biomarker in patients with oral squamous cell carcinoma.
4 Therapeutic opportunities
Selective reactivation of the telomerase in the large majority of tumours, while remaining inhibited in normal cells, provides the opportunity to develop therapeutic approaches inhibiting telomerase activity (Arndt and MacKenzie, 2016). Several strategies have been devised to target different components of the telomerase molecular machinery such as TERT mRNA, TERT holoenzyme, components of the telomerase complex, TERT promoter, nuclear factors regulating TERT expression as well as mutant TERT promoters (Tornesello et al., 2022b). Most telomerase inhibitors might be effective to treat several cancer types, such as prostate, lung, breast, colorectal, and haematological malignancies, which rarely harbour TERT promoter mutations but display strong telomerase activity mainly depending on chromosomal rearrangements and TERT gene amplification (Dratwa et al., 2020). In addition, new compounds that selectively stabilize the G-quadruplex in mutant TERT promoters and inhibit telomerase expression have been evaluated in preclinical studies for their inhibitory effect on telomerase and killing of cancer cells (reviewed in (Song et al., 2019b; Tornesello et al., 2022b)).
Immunotherapy targeting telomerase also showed promising results in all cancer types with high telomerase activity (Mizukoshi and Kaneko, 2019). Specifically, the GV1001 peptide containing a 16 amino-acid (616–626; EARPALLTSRLRFIPK), located within the reverse transcriptase domain of the telomerase holoenzyme, was originally developed as an anti-cancer vaccine to treat several cancer types (Negrini et al., 2020). A Phase I/II study evaluating the safety, tolerability and immunogenicity in patients with pancreatic cancer demonstrated the induction of an immune response that correlated with prolonged survival (Bernhardt et al., 2006). The GV1001 peptide showed cell penetrating (CPP) properties which enabled the cytosolic delivery of macromolecules such as proteins, DNA and siRNA via extracellular heat shock protein 90 (eHSP90) and 70 (eHSP70) complexes (Kim et al., 2016). The CPP properties may contribute to enhance its anti-cancer immune response compared to other telomerase peptide-based vaccines. Importantly, the GV1001 CPP, besides eliciting immune response against telomerase, has an antiviral effect against hepatitis C virus (HCV) and human immunodeficiency virus type 1 (HIV-1) as well as inhibits HBV replication and hepatitis B surface antigen (HBsAg) secretion in a dose-dependent manner (Choi et al., 2020). A recent study showed the anti-cancer effect of GV1001 in non-small cell lung cancer cells consisting in the suppression of cell proliferation and invasion as well as inhibition of VEGF expression (Kim et al., 2022). Therefore, GV1001 has also an inhibitory effect on tumour-associated angiogenesis.
Other TERT-derived synthetic peptides, that are presented by the major histocompatibility complex (MHC) and induce specific CD8+ cytotoxic T lymphocytes (CTL), include the peptide I540 (540-548; ILAKFLHWL), that is the first TERT immunogenic peptide identified as an HLA-A*0201-restricted T-cell epitope and has been evaluated in phase III clinical trials for melanoma treatment (Li et al., 2007; Wenandy et al., 2008; Liu et al., 2010). In addition, TERT 988 (988–997; DLQVNSLQTV) has been shown to stimulate CTL specifically against lysed tumour cells of various histological types expressing HER-2/neu or TERT (Scardino et al., 2002). Peptide K973 (973-981; KLFGVLRLK) was used to generate specific CD8+ CTLs from HLA-A3+ cancer patients and healthy individuals. These CTLs were able to lyse diverse HLA-A3 tumour cell types expressing telomerase (Vonderheide et al., 2001). TERT 572 (572-580; RLFFYRKSV) is a vaccine containing the adjuvant Montanide ISA51 and has been evaluated in patients with chemotherapy-resistant solid tumors. Two doses of vaccine induced the development of TERT 572Y -specific CD8+ in more than 90% of the vaccinated patients (Mavroudis et al., 2006). UV1 peptide vaccine, which consists of three peptides [(peptide 725; TERT 691-705 (RTFVLRVRAQDPPPE), peptide 719-20; TERT 660-689 (ALFSVLNYERARRPGLLGASVLGLDDIHRA), peptide 728; TERT 651-665 (AERLTSRVKALFSVL)] and GM-CSF as adjuvant, has been used in combination with androgen deprivation therapy and radiotherapy to treat patients with androgene-sensitive metastatic prostate cancer in phase I/II trial (NCT01784913). Long-term monitoring showed that some patients maintained high immune responses without relaps (Lilleby et al., 2023). UV1 peptide vaccination was also evaluated in patients with non-small cell lung cancer (NSCLC) and found to induce specific T-cell responses in the 67% of patients non-HLA-typed with stage III/IV NSCLC and with no evidence of progression after prior treatments (Brunsvig et al., 2020).
5 Conclusion
The crucial role of telomerase activity and telomere maintenance in cell transformation and development of cancers has known since decades. Mechanisms of telomerase reactivation in cancer cells include complex molecular changes such as chromosomal translocations, TERT gene amplifications, TERT structural variants, transcription binding factors, TERT promoter mutations and spatial chromatin arrangements. Most of these genetic and epigenetic alterations drive strong telomerase activity in a manner that is specific to cancer histotypes. Paradigmatic is the observation that a subgroup of high-risk neuroblastoma patients having mostly unfavourable outcome were characterized by TERT rearrangements or by MYCN amplified tumours, both inducing massive transcriptional upregulation of the TERT gene (Peifer et al., 2015). Similarly, increased TERT gene copy number and upregulation of telomerase have been identified in breast cancer, thyroid carcinoma, and lung adenocarcinoma in association with poor prognosis (Gay-Bellile et al., 2017). Targeting telomerase, by using direct telomerase inhibitors and immunotherapies, might be effective in all these cancers expressing high levels of TERT gene.
Knowing the temporal occurrence of genetic and epigenetic changes in TERT locus during cancer growth is very important for the development of diagnostic and prognostic markers in diverse tumour types. The cancer driver effect of TERT promoter mutations in tumour development has been shown by a proof-of-concept study showing that correction of the −124A mutation to the wild type −124G blocked the binding of ETS transcription factors to the TERT promoter, reduced TERT gene transcription and telomerase expression, and induced senescence and proliferative arrest of glioblastoma cells (Li et al., 2020). Assessment of the mutated TERT promoter in fluid matrices of human body has already demonstrated its value for the monitoring of tumour diagnosis and recurrence.
In normal cells, TERT promoter is tightly controlled by numerous transcription factors, which bind to regulatory elements and control the silencing in somatic cells and the reactivation in stem cells. These factors include among others activators of TERT transcription, such as the oncogene c-MYC, Sp1, NF-κB, STAT family of proteins, AP-2, GSC, as well as repressors that downregulate TERT transcription, such as MAD1/2, p53, WT1, CTCF, and MZF-2 (Dratwa et al., 2020). The frequent overexpression of transcriptional activators in human cancers, and mutations of repressors (i.e., p53) lead to the enhanced expression of TERT gene and disruption of the fine tuning of telomerase function. As an example, small molecules that cause disruption of Myc/Max heterodimerization on TERT promoter and proteasome-mediated degradation of Myc have been shown to suppress cell proliferation in diverse cancer cell lines (Choi et al., 2017; Han et al., 2019).
Mutations in the core promoter of the TERT gene are exceptionally high in many cancer types and may represent specific targets for new therapies. Such mutations abrogate the repressive function of G4-structures in the TERT promoter by inducing conformational changes (Palumbo et al., 2009; Chaire et al., 2014; Song et al., 2019b). Recently, the screening of chemical libraries allowed to identify small molecules able to bind specifically TERT promoter, to redirect the misfolding of mutant promoter G-quadruplexes and to re-establish the silencing of TERT gene (Song et al., 2019b). Furthermore, the tetrameric complex GABPA/GABPB plays an important role in the regulation of TERT transcription since it selectively binds and activates the mutant but not the wild-type TERT promoter in glioblastoma (Bell et al., 2015). A strong inducer of GABPB expression is FOS and T-5224, which is a new small molecule inhibitor of FOS, selectively suppress TERT expression in thyroid cancer cells carrying TERT promoter mutations but not in wild-type TERT cells (Liu et al., 2021). Therapeutic strategies specifically directed to TERT promoter mutations will likely have an important clinical impact in many types of human cancers characterized by such mutations.
Author contributions
MT: Conceptualization, Data curation, Resources, Supervision, Writing–original draft, Writing–review and editing. AC: Writing–original draft. NS: Writing–original draft. SA: Writing–original draft. PB: Writing–review and editing. FT: Writing–review and editing. FB: Writing–review and editing. LB: Writing–review and editing. AT: Data curation, Writing–original draft, Writing–review and editing.
Funding
The author(s) declare financial support was received for the research, authorship, and/or publication of this article. This work was partly funded by grants from the Italian Ministry of Health Ricerca Corrente L1/10; Italian Ministry of Health Progetto Finalizzato (RF-2018-12366163); Italian Association for Cancer Research (AIRC-IG-2021-ID-26111).
Conflict of interest
The authors declare that the research was conducted in the absence of any commercial or financial relationships that could be construed as a potential conflict of interest.
The author(s) declared that they were an editorial board member of Frontiers, at the time of submission. This had no impact on the peer review process and the final decision.
Publisher’s note
All claims expressed in this article are solely those of the authors and do not necessarily represent those of their affiliated organizations, or those of the publisher, the editors and the reviewers. Any product that may be evaluated in this article, or claim that may be made by its manufacturer, is not guaranteed or endorsed by the publisher.
References
Agarwal, N., Rinaldetti, S., Cheikh, B. B., Zhou, Q., Hass, E. P., Jones, R. T., et al. (2021). TRIM28 is a transcriptional activator of the mutant TERT promoter in human bladder cancer. Proc. Natl. Acad. Sci. U. S. A. 118, e2102423118. doi:10.1073/pnas.2102423118
Akincilar, S. C., Khattar, E., Boon, P. L., Unal, B., Fullwood, M. J., and Tergaonkar, V. (2016). Long-range chromatin interactions drive mutant TERT promoter activation. Cancer Discov. 6, 1276–1291. doi:10.1158/2159-8290.CD-16-0177
Andrés-Lencina, J. J., Rachakonda, S., García-Casado, Z., Srinivas, N., Skorokhod, A., Requena, C., et al. (2019). TERT promoter mutation subtypes and survival in stage I and II melanoma patients. Int. J. Cancer 144, 1027–1036. doi:10.1002/ijc.31780
Annunziata, C., Pezzuto, F., Greggi, S., Ionna, F., Losito, S., Botti, G., et al. (2018). Distinct profiles of TERT promoter mutations and telomerase expression in head and neck cancer and cervical carcinoma. Int. J. Cancer 143, 1153–1161. doi:10.1002/ijc.31412
Arndt, G. M., and MacKenzie, K. L. (2016). New prospects for targeting telomerase beyond the telomere. Nat. Rev. Cancer 16, 508–524. doi:10.1038/nrc.2016.55
Barger, C. J., Suwala, A. K., Soczek, K. M., Wang, A. S., Kim, M. Y., Hong, C., et al. (2022). Conserved features of TERT promoter duplications reveal an activation mechanism that mimics hotspot mutations in cancer. Nat. Commun. 13, 5430. doi:10.1038/s41467-022-33099-x
Barthel, F. P., Wei, W., Tang, M., Martinez-Ledesma, E., Hu, X., Amin, S. B., et al. (2017). Systematic analysis of telomere length and somatic alterations in 31 cancer types. Nat. Genet. 49, 349–357. doi:10.1038/ng.3781
Bell, R. J., Rube, H. T., Kreig, A., Mancini, A., Fouse, S. D., Nagarajan, R. P., et al. (2015). Cancer. The transcription factor GABP selectively binds and activates the mutant TERT promoter in cancer. Science 348, 1036–1039. doi:10.1126/science.aab0015
Bernhardt, S. L., Gjertsen, M. K., Trachsel, S., Moller, M., Eriksen, J. A., Meo, M., et al. (2006). Telomerase peptide vaccination of patients with non-resectable pancreatic cancer: a dose escalating phase I/II study. Br. J. Cancer 95, 1474–1482. doi:10.1038/sj.bjc.6603437
Boscolo-Rizzo, P., Tirelli, G., Polesel, J., Sia, E., Phillips, V., Borsetto, D., et al. (2023). TERT promoter mutations in head and neck squamous cell carcinoma: a systematic review and meta-analysis on prevalence and prognostic significance. Oral Oncol. 140, 106398. doi:10.1016/j.oraloncology.2023.106398
Brunsvig, P. F., Guren, T. K., Nyakas, M., Steinfeldt-Reisse, C. H., Rasch, W., Kyte, J. A., et al. (2020). Long-term outcomes of a phase I study with UV1, a second generation telomerase based vaccine, in patients with advanced non-small cell lung cancer. Front. Immunol. 11, 572172. doi:10.3389/fimmu.2020.572172
Bullock, M., Lim, G., Zhu, Y., Aberg, H., Kurdyukov, S., and Clifton-Bligh, R. (2019). ETS factor ETV5 activates the mutant telomerase reverse transcriptase promoter in thyroid cancer. Thyroid 29, 1623–1633. doi:10.1089/thy.2018.0314
Bushweller, J. H. (2019). Targeting transcription factors in cancer - from undruggable to reality. Nat. Rev. Cancer 19, 611–624. doi:10.1038/s41568-019-0196-7
Chaires, J. B., Trent, J. O., Gray, R. D., Dean, W. L., Buscaglia, R., Thomas, S. D., et al. (2014). An improved model for the hTERT promoter quadruplex. PLoS One 9, e115580. doi:10.1371/journal.pone.0115580
Chiba, K., Lorbeer, F. K., Shain, A. H., McSwiggen, D. T., Schruf, E., Oh, A., et al. (2017). Mutations in the promoter of the telomerase gene TERT contribute to tumorigenesis by a two-step mechanism. Science 357, 1416–1420. doi:10.1126/science.aao0535
Choi, S. H., Mahankali, M., Lee, S. J., Hull, M., Petrassi, H. M., Chatterjee, A. K., et al. (2017). Targeted disruption of myc-max oncoprotein complex by a small molecule. ACS Chem. Biol. 12, 2715–2719. doi:10.1021/acschembio.7b00799
Choi, Y. M., Kim, H., Lee, S. A., Lee, S. Y., and Kim, B. J. (2020). A telomerase-derived peptide exerts an anti-hepatitis B virus effect via mitochondrial DNA stress-dependent type I interferon production. Front. Immunol. 11, 652. doi:10.3389/fimmu.2020.00652
Chun-On, P., Hinchie, A. M., Beale, H. C., Gil Silva, A. A., Rush, E., Sander, C., et al. (2022). TPP1 promoter mutations cooperate with TERT promoter mutations to lengthen telomeres in melanoma. Science 378, 664–668. doi:10.1126/science.abq0607
Colebatch, A. J., Paver, E. C., Vergara, I. A., Thompson, J. F., Long, G. V., Wilmott, J. S., et al. (2022). Elevated non-coding promoter mutations are associated with malignant transformation of melanocytic naevi to melanoma. Pathology 54, 533–540. doi:10.1016/j.pathol.2021.12.289
Cong, Y. S., Wen, J., and Bacchetti, S. (1999). The human telomerase catalytic subunit hTERT: organization of the gene and characterization of the promoter. Hum. Mol. Genet. 8, 137–142. doi:10.1093/hmg/8.1.137
Cristofari, G., and Lingner, J. (2006). Telomere length homeostasis requires that telomerase levels are limiting. EMBO J. 25, 565–574. doi:10.1038/sj.emboj.7600952
Crowe, D. L., Nguyen, D. C., Tsang, K. J., and Kyo, S. (2001). E2F-1 represses transcription of the human telomerase reverse transcriptase gene. Nucleic Acids Res. 29, 2789–2794. doi:10.1093/nar/29.13.2789
Damsky, W. E., and Bosenberg, M. (2017). Melanocytic nevi and melanoma: unraveling a complex relationship. Oncogene 36, 5771–5792. doi:10.1038/onc.2017.189
Dekker, J., Marti-Renom, M. A., and Mirny, L. A. (2013). Exploring the three-dimensional organization of genomes: interpreting chromatin interaction data. Nat. Rev. Genet. 14, 390–403. doi:10.1038/nrg3454
Del Bianco, P., Stagni, C., Giunco, S., Fabozzi, A., Elefanti, L., Pellegrini, S., et al. (2020). TERT promoter mutations differently correlate with the clinical outcome of MAPK inhibitor-treated melanoma patients. Cancers (Basel) 12, 946. doi:10.3390/cancers12040946
Del Pino, M., Rodriguez-Carunchio, L., and Ordi, J. (2013). Pathways of vulvar intraepithelial neoplasia and squamous cell carcinoma. Histopathology 62, 161–175. doi:10.1111/his.12034
Dogan, F., and Forsyth, N. R. (2021). Telomerase regulation: a role for epigenetics. Cancers (Basel) 13, 1213. doi:10.3390/cancers13061213
Dratwa, M., Wysoczanska, B., Lacina, P., Kubik, T., and Bogunia-Kubik, K. (2020). TERT-regulation and roles in cancer formation. Front. Immunol. 11, 589929. doi:10.3389/fimmu.2020.589929
Dubbink, H. J., Bakels, H., Post, E., Zwarthoff, E. C., and Verdijk, R. M. (2014). TERT promoter mutations and BRAF mutations are rare in sporadic, and TERT promoter mutations are absent in NF1-related malignant peripheral nerve sheath tumors. J. Neurooncol 120, 267–272. doi:10.1007/s11060-014-1553-8
Fredriksson, N. J., Ny, L., Nilsson, J. A., and Larsson, E. (2014). Systematic analysis of noncoding somatic mutations and gene expression alterations across 14 tumor types. Nat. Genet. 46, 1258–1263. doi:10.1038/ng.3141
Gay-Bellile, M., Veronese, L., Combes, P., Eymard-Pierre, E., Kwiatkowski, F., Dauplat, M. M., et al. (2017). TERT promoter status and gene copy number gains: effect on TERT expression and association with prognosis in breast cancer. Oncotarget 8, 77540–77551. doi:10.18632/oncotarget.20560
Ge, Z., Helmijr, J. C. A., Jansen, M., Boor, P. P. C., Noordam, L., Peppelenbosch, M., et al. (2021). Detection of oncogenic mutations in paired circulating tumor DNA and circulating tumor cells in patients with hepatocellular carcinoma. Transl. Oncol. 14, 101073. doi:10.1016/j.tranon.2021.101073
Gewin, L., Myers, H., Kiyono, T., and Galloway, D. A. (2004). Identification of a novel telomerase repressor that interacts with the human papillomavirus type-16 E6/E6-AP complex. Genes Dev. 18, 2269–2282. doi:10.1101/gad.1214704
Giunco, S., Boscolo-Rizzo, P., Rampazzo, E., Tirelli, G., Alessandrini, L., Di Carlo, R., et al. (2021). TERT promoter mutations and rs2853669 polymorphism: useful markers for clinical outcome stratification of patients with oral cavity squamous cell carcinoma. Front. Oncol. 11, 782658. doi:10.3389/fonc.2021.782658
Grill, S., Bisht, K., Tesmer, V. M., Shami, A. N., Hammoud, S. S., and Nandakumar, J. (2019). Two separation-of-function isoforms of human TPP1 dictate telomerase regulation in somatic and germ cells. Cell Rep. 27, 3511–3521. doi:10.1016/j.celrep.2019.05.073
Günes, C., Wezel, F., Southgate, J., and Bolenz, C. (2018). Implications of TERT promoter mutations and telomerase activity in urothelial carcinogenesis. Nat. Rev. Urol. 15, 386–393. doi:10.1038/s41585-018-0001-5
Han, H., Jain, A. D., Truica, M. I., Izquierdo-Ferrer, J., Anker, J. F., Lysy, B., et al. (2019). Small-molecule MYC inhibitors suppress tumor growth and enhance immunotherapy. Cancer Cell 36, 483–497. doi:10.1016/j.ccell.2019.10.001
Hanahan, D., and Weinberg, R. A. (2011). Hallmarks of cancer: the next generation. Cell 144, 646–674. doi:10.1016/j.cell.2011.02.013
Hirai, M., Kinugasa, H., Nouso, K., Yamamoto, S., Terasawa, H., Onishi, Y., et al. (2021). Prediction of the prognosis of advanced hepatocellular carcinoma by TERT promoter mutations in circulating tumor DNA. J. Gastroenterol. Hepatol. 36, 1118–1125. doi:10.1111/jgh.15227
Horn, S., Figl, A., Rachakonda, P. S., Fischer, C., Sucker, A., Gast, A., et al. (2013). TERT promoter mutations in familial and sporadic melanoma. Science 339, 959–961. doi:10.1126/science.1230062
Hosen, M. I., Sheikh, M., Zvereva, M., Scelo, G., Forey, N., Durand, G., et al. (2020). Urinary TERT promoter mutations are detectable up to 10 years prior to clinical diagnosis of bladder cancer: evidence from the Golestan Cohort Study. EBioMedicine 53, 102643. doi:10.1016/j.ebiom.2020.102643
Huang, D. S., Wang, Z., He, X. J., Diplas, B. H., Yang, R., Killela, P. J., et al. (2015a). Recurrent TERT promoter mutations identified in a large-scale study of multiple tumour types are associated with increased TERT expression and telomerase activation. Eur. J. Cancer 51, 969–976. doi:10.1016/j.ejca.2015.03.010
Huang, F. W., Bielski, C. M., Rinne, M. L., Hahn, W. C., Sellers, W. R., Stegmeier, F., et al. (2015b). TERT promoter mutations and monoallelic activation of TERT in cancer. Oncogenesis 4, e176. doi:10.1038/oncsis.2015.39
Huang, F. W., Hodis, E., Xu, M. J., Kryukov, G. V., Chin, L., and Garraway, L. A. (2013). Highly recurrent TERT promoter mutations in human melanoma. Science 339, 957–959. doi:10.1126/science.1229259
Iorga, L., Dragos Marcu, R., Cristina Diaconu, C., Maria Alexandra Stanescu, A., Pantea Stoian, A., Liviu Dorel Mischianu, D., et al. (2020). Penile carcinoma and HPV infection (Review). Exp. Ther. Med. 20, 91–96. doi:10.3892/etm.2019.8181
Jafri, M. A., Ansari, S. A., Alqahtani, M. H., and Shay, J. W. (2016). Roles of telomeres and telomerase in cancer, and advances in telomerase-targeted therapies. Genome Med. 8, 69. doi:10.1186/s13073-016-0324-x
Jin, L., Chen, E., Dong, S., Cai, Y., Zhang, X., Zhou, Y., et al. (2016). BRAF and TERT promoter mutations in the aggressiveness of papillary thyroid carcinoma: a study of 653 patients. Oncotarget 7, 18346–18355. doi:10.18632/oncotarget.7811
Kanzawa, T., Komata, T., Kyo, S., Germano, I. M., Kondo, Y., and Kondo, S. (2003). Down-regulation of telomerase activity in malignant glioma cells by p27KIP1. Int. J. Oncol. 23, 1703–1708. doi:10.3892/ijo.23.6.1703
Killela, P. J., Reitman, Z. J., Jiao, Y., Bettegowda, C., Agrawal, N., Diaz, L. A., et al. (2013). TERT promoter mutations occur frequently in gliomas and a subset of tumors derived from cells with low rates of self-renewal. Proc. Natl. Acad. Sci. U. S. A. 110, 6021–6026. doi:10.1073/pnas.1303607110
Kim, H., Seo, E. H., Lee, S. H., and Kim, B. J. (2016). The telomerase-derived anticancer peptide vaccine GV1001 as an extracellular heat shock protein-mediated cell-penetrating peptide. Int. J. Mol. Sci. 17, 2054. doi:10.3390/ijms17122054
Kim, J. H., Cho, Y. R., Ahn, E. K., Kim, S., Han, S., Kim, S. J., et al. (2022). A novel telomerase-derived peptide GV1001-mediated inhibition of angiogenesis: regulation of VEGF/VEGFR-2 signaling pathways. Transl. Oncol. 26, 101546. doi:10.1016/j.tranon.2022.101546
Kim, S. K., Kim, J. H., Han, J. H., Cho, N. H., Kim, S. J., Kim, S. I., et al. (2021). TERT promoter mutations in penile squamous cell carcinoma: high frequency in non-HPV-related type and association with favorable clinicopathologic features. J. Cancer Res. Clin. Oncol. 147, 1125–1135. doi:10.1007/s00432-021-03514-9
Kim, W., and Shay, J. W. (2018). Long-range telomere regulation of gene expression: telomere looping and telomere position effect over long distances (TPE-OLD). Differentiation 99, 1–9. doi:10.1016/j.diff.2017.11.005
Lambert, M., Jambon, S., Depauw, S., and David-Cordonnier, M. H. (2018). Targeting transcription factors for cancer treatment. Molecules 23, 1479. doi:10.3390/molecules23061479
Lee, J. H., Lee, J. E., Kahng, J. Y., Kim, S. H., Park, J. S., Yoon, S. J., et al. (2018). Human glioblastoma arises from subventricular zone cells with low-level driver mutations. Nature 560, 243–247. doi:10.1038/s41586-018-0389-3
Li, H., Katik, I., and Liu, J. P. (2007). Uses of telomerase peptides in anti-tumor immune therapy. Methods Mol. Biol. 405, 61–86. doi:10.1007/978-1-60327-070-0_7
Li, X., Qian, X., Wang, B., Xia, Y., Zheng, Y., Du, L., et al. (2020). Programmable base editing of mutated TERT promoter inhibits brain tumour growth. Nat. Cell Biol. 22, 282–288. doi:10.1038/s41556-020-0471-6
Li, Y., Zhou, Q. L., Sun, W., Chandrasekharan, P., Cheng, H. S., Ying, Z., et al. (2015). Non-canonical NF-κB signalling and ETS1/2 cooperatively drive C250T mutant TERT promoter activation. Nat. Cell Biol. 17, 1327–1338. doi:10.1038/ncb3240
Lilleby, W., Seierstad, T., Inderberg, E. M., and Hole, K. H. (2023). Impact of human telomerase reverse transcriptase peptide vaccine combined with androgen deprivation therapy and radiotherapy in de novo metastatic prostate cancer: long-term clinical monitoring. Int. J. Cancer 152, 2166–2173. doi:10.1002/ijc.34448
Liu, J. P., Chen, W., Schwarer, A. P., and Li, H. (2010). Telomerase in cancer immunotherapy. Biochim. Biophys. Acta 1805, 35–42. doi:10.1016/j.bbcan.2009.09.001
Liu, R., Tan, J., Shen, X., Jiang, K., Wang, C., Zhu, G., et al. (2021). Therapeutic targeting of FOS in mutant TERT cancers through removing TERT suppression of apoptosis via regulating survivin and TRAIL-R2. Proc. Natl. Acad. Sci. U. S. A. 118, e2022779118. doi:10.1073/pnas.2022779118
Liu, R., Zhang, T., Zhu, G., and Xing, M. (2018). Regulation of mutant TERT by BRAF V600E/MAP kinase pathway through FOS/GABP in human cancer. Nat. Commun. 9, 579. doi:10.1038/s41467-018-03033-1
Liu, T., Yuan, X., and Xu, D. (2016). Cancer-specific telomerase reverse transcriptase (TERT) promoter mutations: biological and clinical implications. Genes (Basel) 7, 38. doi:10.3390/genes7070038
Liu, X., Qu, S., Liu, R., Sheng, C., Shi, X., Zhu, G., et al. (2014). TERT promoter mutations and their association with BRAF V600E mutation and aggressive clinicopathological characteristics of thyroid cancer. J. Clin. Endocrinol. Metab. 99, E1130–E1136. doi:10.1210/jc.2013-4048
Lorbeer, F. K., and Hockemeyer, D. (2020). TERT promoter mutations and telomeres during tumorigenesis. Curr. Opin. Genet. Dev. 60, 56–62. doi:10.1016/j.gde.2020.02.001
Mancini, A., Xavier-Magalhaes, A., Woods, W. S., Nguyen, K. T., Amen, A. M., Hayes, J. L., et al. (2018). Disruption of the β1L isoform of GABP reverses glioblastoma replicative immortality in a TERT promoter mutation-dependent manner. Cancer Cell 34, 513–528 e8. doi:10.1016/j.ccell.2018.08.003
Mavroudis, D., Bolonakis, I., Cornet, S., Myllaki, G., Kanellou, P., Kotsakis, A., et al. (2006). A phase I study of the optimized cryptic peptide TERT(572y) in patients with advanced malignancies. Oncology 70, 306–314. doi:10.1159/000096252
Mizukoshi, E., and Kaneko, S. (2019). Telomerase-Targeted cancer immunotherapy. Int. J. Mol. Sci. 20, 1823. doi:10.3390/ijms20081823
Mukherjee, A. K., Sharma, S., Bagri, S., Kutum, R., Kumar, P., Hussain, A., et al. (2019). Telomere repeat-binding factor 2 binds extensively to extra-telomeric G-quadruplexes and regulates the epigenetic status of several gene promoters. J. Biol. Chem. 294, 17709–17722. doi:10.1074/jbc.RA119.008687
Müller, M., Bird, T. G., and Nault, J. C. (2020). The landscape of gene mutations in cirrhosis and hepatocellular carcinoma. J. Hepatol. 72, 990–1002. doi:10.1016/j.jhep.2020.01.019
Nagore, E., Heidenreich, B., Rachakonda, S., Garcia-Casado, Z., Requena, C., Soriano, V., et al. (2016). TERT promoter mutations in melanoma survival. Int. J. Cancer 139, 75–84. doi:10.1002/ijc.30042
Nault, J. C., Calderaro, J., Di Tommaso, L., Balabaud, C., Zafrani, E. S., Bioulac-Sage, P., et al. (2014). Telomerase reverse transcriptase promoter mutation is an early somatic genetic alteration in the transformation of premalignant nodules in hepatocellular carcinoma on cirrhosis. Hepatology 60, 1983–1992. doi:10.1002/hep.27372
Nault, J. C., Mallet, M., Pilati, C., Calderaro, J., Bioulac-Sage, P., Laurent, C., et al. (2013). High frequency of telomerase reverse-transcriptase promoter somatic mutations in hepatocellular carcinoma and preneoplastic lesions. Nat. Commun. 4, 2218. doi:10.1038/ncomms3218
Negrini, S., De Palma, R., and Filaci, G. (2020). Anti-cancer immunotherapies targeting telomerase. Cancers (Basel) 12, 2260. doi:10.3390/cancers12082260
Ningarhari, M., Caruso, S., Hirsch, T. Z., Bayard, Q., Franconi, A., Vedie, A. L., et al. (2021). Telomere length is key to hepatocellular carcinoma diversity and telomerase addiction is an actionable therapeutic target. J. Hepatol. 74, 1155–1166. doi:10.1016/j.jhep.2020.11.052
Oh, S. T., Kyo, S., and Laimins, L. A. (2001). Telomerase activation by human papillomavirus type 16 E6 protein: induction of human telomerase reverse transcriptase expression through Myc and GC-rich Sp1 binding sites. J. Virol. 75, 5559–5566. doi:10.1128/JVI.75.12.5559-5566.2001
Palumbo, S. L., Ebbinghaus, S. W., and Hurley, L. H. (2009). Formation of a unique end-to-end stacked pair of G-quadruplexes in the hTERT core promoter with implications for inhibition of telomerase by G-quadruplex-interactive ligands. J. Am. Chem. Soc. 131, 10878–10891. doi:10.1021/ja902281d
Peifer, M., Hertwig, F., Roels, F., Dreidax, D., Gartlgruber, M., Menon, R., et al. (2015). Telomerase activation by genomic rearrangements in high-risk neuroblastoma. Nature 526, 700–704. doi:10.1038/nature14980
Pezzuto, F., Buonaguro, L., Buonaguro, F. M., and Tornesello, M. L. (2017). Frequency and geographic distribution of TERT promoter mutations in primary hepatocellular carcinoma. Infect. Agent Cancer 12, 27. doi:10.1186/s13027-017-0138-5
Pezzuto, F., Buonaguro, L., Buonaguro, F. M., and Tornesello, M. L. (2018). The role of circulating free DNA and MicroRNA in non-invasive diagnosis of HBV- and HCV-related hepatocellular carcinoma. Int. J. Mol. Sci. 19, 1007. doi:10.3390/ijms19041007
Ramlee, M. K., Wang, J., Toh, W. X., and Li, S. (2016). Transcription regulation of the human telomerase reverse transcriptase (hTERT) gene. Genes (Basel) 7, 50. doi:10.3390/genes7080050
Salama, A. M., Momeni-Boroujeni, A., Vanderbilt, C., Ladanyi, M., and Soslow, R. (2022). Molecular landscape of vulvovaginal squamous cell carcinoma: new insights into molecular mechanisms of HPV-associated and HPV-independent squamous cell carcinoma. Mod. Pathol. 35, 274–282. doi:10.1038/s41379-021-00942-3
Scardino, A., Gross, D. A., Alves, P., Schultze, J. L., Graff-Dubois, S., Faure, O., et al. (2002). HER-2/neu and hTERT cryptic epitopes as novel targets for broad spectrum tumor immunotherapy. J. Immunol. 168, 5900–5906. doi:10.4049/jimmunol.168.11.5900
Sharma, S., and Chowdhury, S. (2022). Emerging mechanisms of telomerase reactivation in cancer. Trends Cancer 8, 632–641. doi:10.1016/j.trecan.2022.03.005
Sharma, S., Mukherjee, A. K., Roy, S. S., Bagri, S., Lier, S., Verma, M., et al. (2021). Human telomerase is directly regulated by non-telomeric TRF2-G-quadruplex interaction. Cell Rep. 35, 109154. doi:10.1016/j.celrep.2021.109154
Shay, J. W., and Wright, W. E. (2000). Hayflick, his limit, and cellular ageing. Nat. Rev. Mol. Cell Biol. 1, 72–76. doi:10.1038/35036093
Sheng, W. Y., Chen, Y. R., and Wang, T. C. (2006). A major role of PKC theta and NFkappaB in the regulation of hTERT in human T lymphocytes. FEBS Lett. 580, 6819–6824. doi:10.1016/j.febslet.2006.11.044
Shi, X., Liu, R., Qu, S., Zhu, G., Bishop, J., Liu, X., et al. (2015). Association of TERT promoter mutation 1,295,228 C>T with BRAF V600E mutation, older patient age, and distant metastasis in anaplastic thyroid cancer. J. Clin. Endocrinol. Metab. 100, E632–E637. doi:10.1210/jc.2014-3606
Sizemore, G. M., Pitarresi, J. R., Balakrishnan, S., and Ostrowski, M. C. (2017). The ETS family of oncogenic transcription factors in solid tumours. Nat. Rev. Cancer 17, 337–351. doi:10.1038/nrc.2017.20
Smith, E. M., Pendlebury, D. F., and Nandakumar, J. (2020). Structural biology of telomeres and telomerase. Cell Mol. Life Sci. 77, 61–79. doi:10.1007/s00018-019-03369-x
Song, J. H., Kang, H. J., Luevano, L. A., Gokhale, V., Wu, K., Pandey, R., et al. (2019b). Small-molecule-targeting hairpin loop of hTERT promoter G-quadruplex induces cancer cell death. Cell Chem. Biol. 26, 1110–1121. doi:10.1016/j.chembiol.2019.04.009
Song, Y. S., Yoo, S. K., Kim, H. H., Jung, G., Oh, A. R., Cha, J. Y., et al. (2019a). Interaction of BRAF-induced ETS factors with mutant TERT promoter in papillary thyroid cancer. Endocr. Relat. Cancer 26, 629–641. doi:10.1530/ERC-17-0562
Starita, N., Buonaguro, L., Buonaguro, F. M., and Tornesello, M. L. (2018). Telomerase promoter mutations in human immunodeficiency virus-related conjunctiva neoplasia. J. Transl. Med. 16, 77. doi:10.1186/s12967-018-1456-0
Starita, N., Pezzuto, F., Sarno, S., Losito, N. S., Perdonà, S., Buonaguro, L., et al. (2022). Mutations in the telomerase reverse transcriptase promoter and PIK3CA gene are common events in penile squamous cell carcinoma of Italian and Ugandan patients. Int. J. Cancer 150, 1879–1888. doi:10.1002/ijc.33990
Stern, J. L., Theodorescu, D., Vogelstein, B., Papadopoulos, N., and Cech, T. R. (2015). Mutation of the TERT promoter, switch to active chromatin, and monoallelic TERT expression in multiple cancers. Genes Dev. 29, 2219–2224. doi:10.1101/gad.269498.115
Takakura, M., Kyo, S., Kanaya, T., Hirano, H., Takeda, J., Yutsudo, M., et al. (1999). Cloning of human telomerase catalytic subunit (hTERT) gene promoter and identification of proximal core promoter sequences essential for transcriptional activation in immortalized and cancer cells. Cancer Res. 59, 551–557.
Takeda, H., Takai, A., Kumagai, K., Iguchi, E., Arasawa, S., Eso, Y., et al. (2020). Multiregional whole-genome sequencing of hepatocellular carcinoma with nodule-in-nodule appearance reveals stepwise cancer evolution. J. Pathol. 252, 398–410. doi:10.1002/path.5533
Tornesello, M. L., Cerasuolo, A., Starita, N., Tornesello, A. L., Bonelli, P., Tuccillo, F. M., et al. (2022a). The molecular interplay between human oncoviruses and telomerase in cancer development. Cancers (Basel) 14, 5257. doi:10.3390/cancers14215257
Tornesello, M. L., Tornesello, A. L., Starita, N., Cerasuolo, A., Izzo, F., Buonaguro, L., et al. (2022b). Telomerase: a good target in hepatocellular carcinoma? An overview of relevant preclinical data. Expert Opin. Ther. Targets 26, 767–780. doi:10.1080/14728222.2022.2147062
Totoki, Y., Tatsuno, K., Covington, K. R., Ueda, H., Creighton, C. J., Kato, M., et al. (2014). Trans-ancestry mutational landscape of hepatocellular carcinoma genomes. Nat. Genet. 46, 1267–1273. doi:10.1038/ng.3126
Vallarelli, A. F., Rachakonda, P. S., Andre, J., Heidenreich, B., Riffaud, L., Bensussan, A., et al. (2016). TERT promoter mutations in melanoma render TERT expression dependent on MAPK pathway activation. Oncotarget 7, 53127–53136. doi:10.18632/oncotarget.10634
van der Avoort, I. A., Shirango, H., Hoevenaars, B. M., Grefte, J. M., de Hullu, J. A., de Wilde, P. C., et al. (2006). Vulvar squamous cell carcinoma is a multifactorial disease following two separate and independent pathways. Int. J. Gynecol. Pathol. 25, 22–29. doi:10.1097/01.pgp.0000177646.38266.6a
Vinagre, J., Almeida, A., Populo, H., Batista, R., Lyra, J., Pinto, V., et al. (2013). Frequency of TERT promoter mutations in human cancers. Nat. Commun. 4, 2185. doi:10.1038/ncomms3185
Vinothkumar, V., Arunkumar, G., Revathidevi, S., Arun, K., Manikandan, M., Rao, A. K., et al. (2016). TERT promoter hot spot mutations are frequent in Indian cervical and oral squamous cell carcinomas. Tumour Biol. 37, 7907–7913. doi:10.1007/s13277-015-4694-2
Vogelstein, B., Papadopoulos, N., Velculescu, V. E., Zhou, S., Diaz, L. A., and Kinzler, K. W. (2013). Cancer genome landscapes. Science 339, 1546–1558. doi:10.1126/science.1235122
Vonderheide, R. H., Anderson, K. S., Hahn, W. C., Butler, M. O., Schultze, J. L., and Nadler, L. M. (2001). Characterization of HLA-A3-restricted cytotoxic T lymphocytes reactive against the widely expressed tumor antigen telomerase. Clin. Cancer Res. 7, 3343–3348.
Wang, X. H., Liu, B. R., Qu, B., Xing, H., Gao, S. L., Yin, J. M., et al. (2011). Silencing STAT3 may inhibit cell growth through regulating signaling pathway, telomerase, cell cycle, apoptosis and angiogenesis in hepatocellular carcinoma: potential uses for gene therapy. Neoplasma 58, 158–171. doi:10.4149/neo_2011_02_158
Wenandy, L., Sørensen, R. B., Sengeløv, L., Svane, I. M., thor Straten, P., and Andersen, M. H. (2008). The immunogenicity of the hTERT540-548 peptide in cancer. Clin. Cancer Res. 14, 4–7. doi:10.1158/1078-0432.CCR-07-4590
Wu, K. J., Grandori, C., Amacker, M., Simon-Vermot, N., Polack, A., Lingner, J., et al. (1999). Direct activation of TERT transcription by c-MYC. Nat. Genet. 21, 220–224. doi:10.1038/6010
Xing, M., Liu, R., Liu, X., Murugan, A. K., Zhu, G., Zeiger, M. A., et al. (2014). BRAF V600E and TERT promoter mutations cooperatively identify the most aggressive papillary thyroid cancer with highest recurrence. J. Clin. Oncol. 32, 2718–2726. doi:10.1200/JCO.2014.55.5094
Xu, D., Dwyer, J., Li, H., Duan, W., and Liu, J. P. (2008). Ets2 maintains hTERT gene expression and breast cancer cell proliferation by interacting with c-Myc. J. Biol. Chem. 283, 23567–23580. doi:10.1074/jbc.M800790200
Xu, X., Li, Y., Bharath, S. R., Ozturk, M. B., Bowler, M. W., Loo, B. Z. L., et al. (2018). Structural basis for reactivating the mutant TERT promoter by cooperative binding of p52 and ETS1. Nat. Commun. 9, 3183. doi:10.1038/s41467-018-05644-0
Yoo, Y. S., Park, S., Gwak, J., Ju, B. G., and Oh, S. (2015). Involvement of transcription repressor Snail in the regulation of human telomerase reverse transcriptase (hTERT) by transforming growth factor-β. Biochem. Biophys. Res. Commun. 465, 131–136. doi:10.1016/j.bbrc.2015.07.146
Yuan, X., Larsson, C., and Xu, D. (2019). Mechanisms underlying the activation of TERT transcription and telomerase activity in human cancer: old actors and new players. Oncogene 38, 6172–6183. doi:10.1038/s41388-019-0872-9
Zehir, A., Benayed, R., Shah, R. H., Syed, A., Middha, S., Kim, H. R., et al. (2017). Mutational landscape of metastatic cancer revealed from prospective clinical sequencing of 10,000 patients. Nat. Med. 23, 703–713. doi:10.1038/nm.4333
Zhao, Y., Wang, S., Popova, E. Y., Grigoryev, S. A., and Zhu, J. (2009). Rearrangement of upstream sequences of the hTERT gene during cellular immortalization. Genes Chromosom. Cancer 48, 963–974. doi:10.1002/gcc.20698
Glossary
Keywords: telomerase, tumour, telomeres, TERT promoter mutations, TRF2, G-quadruplex, TERT inhibitors, TERT peptides
Citation: Tornesello ML, Cerasuolo A, Starita N, Amiranda S, Bonelli P, Tuccillo FM, Buonaguro FM, Buonaguro L and Tornesello AL (2023) Reactivation of telomerase reverse transcriptase expression in cancer: the role of TERT promoter mutations. Front. Cell Dev. Biol. 11:1286683. doi: 10.3389/fcell.2023.1286683
Received: 31 August 2023; Accepted: 02 November 2023;
Published: 15 November 2023.
Edited by:
Andrew Liss, Massachusetts General Hospital and Harvard Medical School, United StatesReviewed by:
Andrew T. Ludlow, University of Michigan, United StatesJiyue Zhu, Washington State University Health Sciences Spokane, United States
Copyright © 2023 Tornesello, Cerasuolo, Starita, Amiranda, Bonelli, Tuccillo, Buonaguro, Buonaguro and Tornesello. This is an open-access article distributed under the terms of the Creative Commons Attribution License (CC BY). The use, distribution or reproduction in other forums is permitted, provided the original author(s) and the copyright owner(s) are credited and that the original publication in this journal is cited, in accordance with accepted academic practice. No use, distribution or reproduction is permitted which does not comply with these terms.
*Correspondence: Maria Lina Tornesello, bS50b3JuZXNlbGxvQGlzdGl0dXRvdHVtb3JpLm5hLml0