- 1Immune Signal Unit, Okinawa Institute of Science and Technology Graduate University, Okinawa, Japan
- 2Department of Molecular and Translational Medicine, Paul L. Foster School of Medicine, Texas Tech University Health Sciences Center El Paso, El Paso, TX, United States
- 3Department of Internal Medicine, Section of Cardiovascular Medicine, Section of Molecular Medicine, Wake Forest University School of Medicine, Winston-Salem, NC, United States
Editorial on the Research Topic
Oxidative stress link associated with mitochondrial bioenergetics: relevance in cell aging and age-related pathologies
Mitochondrial bioenergetics and its function in tissue homeostasis are vital for regulating energy processes. As cells age, mitochondrial functions are often disrupted by both intrinsic and extrinsic threats including oxidative stress (Paradies et al., 2017; Sharma et al., 2019). Although the steps involved in oxidative stress-led impaired mitochondrial bioenergetics are mechanistically conserved, involved factors/proteins may vary in different tissues and cell types (Bhatti et al., 2022; Kornicka-Garbowska et al., 2023). The regulation of these proteins in associated pathways is often compromised during aging (Paradies et al., 2010).
The original research published in this Research Topic includes two research articles on cardiomyocytes (Grün et al.; Zhang et al.) and one on human non-small cell lung cancer (NSCLC; Li et al.) along with a review article by Jagtap et al., collects new knowledge on mitochondrial function and shed light on the role of oxidative stress in altered mitochondrial bioenergetics and its relevance with aging-related pathologies. The aging-related decline in proteomic turnover, protein processing/folding, and quality control processes exaggerates the risk of oxidative stress to mitochondrial energetics (Fernandes et al., 2020; Gioran and Chondrogianni et al., 2020; Santiago et al., 2020), which potentiate progression of aging-related pathologies including cardiometabolic disorders, neurodegenerative diseases, and cancer (Dewanjee et al., 2022; Wang et al., 2022). The original research published in this Research Topic gathers new knowledge on these processes (proteomic turnover, protein processing/folding, quality control, proteotoxicity) and their oxidative stress/ROS link, impacting key cellular function in cardiomyocytes and cancer cells.
Grün et al. in an insightful original report, revealed that responses of immature/undifferentiated and differentiated cardiomyocytes to the inhibition of mitochondrial respiration are fundamentally different, which was reflected in their proliferation, survival, and stress responses (Figure 1; 1). Tissue homeostasis of cardiomyocytes is critical for heart development, maintenance, and function. The oxidative stress associated with ATP depletion or produced high levels of reactive oxygen species (ROS) is a feature of inhibited electron transport at the mitochondrial respiratory chain, which can be caused by selective inhibitors. Of note, while adult cardiomyocytes show high susceptibility, mouse embryonic cardiomyocytes are resistant to mitochondrial complex III inhibition. These cells were susceptible to inhibition of mitochondrial complex I, but not of complex III as no effect on cell proliferation and apoptosis was observed. In contrast, differentiated and contractile cardiomyocyte cells showed high susceptibility to complex III inhibition. In undifferentiated cells, complex III inhibition was found to induce different stress responses including unfolded protein response, heat shock response, and antioxidative defense. Interestingly, in these cells suppression of NRF2 (i.e., a transcription factor that regulates cellular antioxidant response) acquired susceptibility to complex III inhibition. These findings revealed that site specificity of the electron transport complex in the mitochondrial respiratory chain governs cell fate in immature cardiomyoblast. It reflects the fundamentally different stress tolerance and survival abilities of immature and mature cardiomyocytes, thus limited plasticity in the latter may restrict the application of cardioprotective therapies.
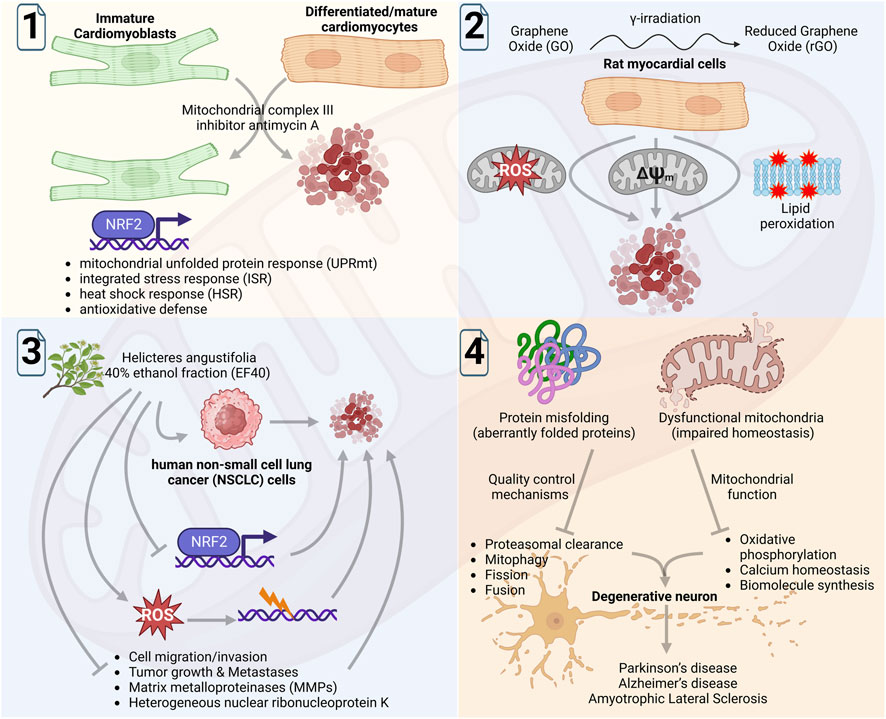
FIGURE 1. Schematic diagram showing summary of the findings by Grün et al. of mitochondrial complex III inhibitors on immature and differentiated cardiomyocytes (1); by Zhang et al. of investigating the cardiotoxic activity of graphene oxide (GO) and reduced GO (rGO) in rat myocardial cells (2); by Li et al. of analyzing the anticancer function of ROS induced by 40% ethanol fraction of Helicteres angustifolia L. in NSCLC cells (3); and by Jagtap et al., to comprehensively review the role of proteostasis in mitochondrial physiology and its function in the aging-related neurodegenerative conditions (4). The figure was Created with BioRender.com.
Zhang et al. in another original study, investigated the cardiotoxic activity of graphene oxide (GO) and reduced GO (rGO) that was found to be mediated by oxidative stress, lipid peroxidation, and mitochondrial dysfunction (Figure 1; 2). Using the rat myocardial cells, they examined the cardiotoxicity of GO and rGO (reduced by γ-irradiation and subsequently characterized by UV/visible light spectroscopy) in vitro and in vivo. The exposure of radiation absorbed dose gradually increased the rGO cytotoxicity that was linked with increasing ROS levels and declined mitochondrial membrane potential, causing mitochondrial dysfunction. Of note, mice treated with GO or rGO exhibited myocardial damage (histopathological changes in cardiac tissues) and altered activities of several enzymes/indicators associated with myocardial activity and lipid peroxidation, respectively. These data revealed evidence of GO and rGO cardiotoxicity to myocardial cells that was mediated by oxidative stress, lipid peroxidation, and mitochondrial dysfunction.
Although the oxidative stress produced by high ROS levels is central to the cardiotoxicity of myocardial cells, its lethality can be seen in cancer cells. In line with this, an original study by Li et al. provided evidence of the anticancer function of ROS, which was essentially promoted by NRF2 inhibition (Figure 1; 3). Using an in vitro model of human non-small cell lung cancer (NSCLC), Li et al. analyzed ethanol fractions of Helicteres angustifolia L. (Helicteres angustifolia, an herb used in folk medicine for its anticancer activity) root and showed that 40% ethanol fraction (EF40; consisted of several bioactive compounds) produced selective toxicity to NSCLC cells. Mechanistically, this bioactivity of EF40 was found to be linked with reduced expression of NRF2 that abundantly expresses across several cancers. Of note, EF40-led suppression of NRF2-dependent cellular defense response promoted the intracellular accumulation of ROS in the NSCLC cells. The biochemical analyses further explained the activity of EF40 in cell cycle arrest and apoptosis, promoted through the ROS-led DNA damage response. An evident decrease in matrix metalloproteinases (MMPs) and hnRNP-K expression levels in EF40-treated NSCLC cells further suggested its anti-migratory activity, which was corroborated by significantly reduced tumor growth and lung metastasis in an in vivo xenograft mice model. Conclusively, Li et al. provided direct evidence of the utility of ROS function in promoting anticancer activity that also reaffirmed the significance of NRF2-regulated defensive antioxidant response in cell survival.
Protein synthesis, protein processing and folding, and quality control mechanisms are critical cellular processes that are directly linked with mitochondrial homeostatic function, which progressively decline with aging. Although the role of protein homeostasis or ‘proteostasis’ in cellular function is understood, its significance in mitochondrial physiology under neurodegenerative conditions needs to be resolved. To this end, Jagtap et al. in a review report comprehensively discussed the new knowledge and developments in the stream (Figure 1; 4). The report carefully reviewed the impact of mitochondrial homeostasis disruption in different cellular processes, on other organelles, and its significance in neurodegenerative disease. It further assessed the impact of misfolded proteins on the physiological processes of mitochondria including fusion, fission, mitophagy, proteasomal clearance, and neurodegeneration. Although proteostasis enables mitochondria to recognize and respond to diverse cytotoxic stress signals that assist cells to adapt and survive, faulty processing may lead to mitochondria dysfunction and cell death. This review further underlined that the discovery of mitochondria and neurodegeneration-associated proteins is critical in understanding the disease and it could also help in designing targeted therapeutics.
Conclusively, the original research and review articles published under the present Research Topic shed light on the role of oxidative stress in altered mitochondrial bioenergetics and its outcome. It also discusses new knowledge on impaired mitochondrial bioenergetics and its relevance with age-related pathologies.
Author contributions
RK: Conceptualization, Visualization, Writing–original draft, Writing–review and editing. SK: Conceptualization, Validation, Writing–review and editing. DT: Conceptualization, Validation, Visualization, Writing–review and editing.
Funding
DT acknowledges research funding from National Institute of Diabetes and Digestive and Kidney Diseases grant number R00DK120876, R00DK120876-04S1, Harold S. Geneen Charitable Trust Awards Program, and Wake Startup Funds. SK acknowledges research funding from National Institute of Aging grant number K99/R00AG065645 and TTUHSC EP MTM Startup Funds.
Acknowledgments
We extend our thanks to all contributing authors and reviewer editors for their valuable contributions and comments to the success of this Research Topic. The summary figure in the editorial was Created with BioRender.com.
Conflict of interest
The authors declare that the research was conducted in the absence of any commercial or financial relationships that could be construed as a potential conflict of interest.
Publisher’s note
All claims expressed in this article are solely those of the authors and do not necessarily represent those of their affiliated organizations, or those of the publisher, the editors and the reviewers. Any product that may be evaluated in this article, or claim that may be made by its manufacturer, is not guaranteed or endorsed by the publisher.
References
Bhatti, G. K., Gupta, A., Pahwa, P., Khullar, N., Singh, S., Navik, U., et al. (2022). Targeting mitochondrial bioenergetics as a promising therapeutic strategy in metabolic and neurodegenerative diseases. Biomed. J. 45, 733–748. doi:10.1016/j.bj.2022.05.002
Dewanjee, S., Chakraborty, P., Bhattacharya, H., Chacko, L., Singh, B., Chaudhary, A., et al. (2022). Altered glucose metabolism in alzheimer's disease: role of mitochondrial dysfunction and oxidative stress. Free Radic. Biol. Med. 193, 134–157. doi:10.1016/j.freeradbiomed.2022.09.032
Fernandes, V., Choudhary, M., Kumar, A., and Singh, S. B. (2020). Proteotoxicity and mitochondrial dynamics in aging diabetic brain. Pharmacol. Res. 159, 104948. doi:10.1016/j.phrs.2020.104948
Gioran, A., and Chondrogianni, N. (2020). Mitochondria (cross)talk with proteostatic mechanisms: focusing on ageing and neurodegenerative diseases. Mech. ageing Dev. 190, 111324. doi:10.1016/j.mad.2020.111324
Kornicka-Garbowska, K., Bourebaba, L., Röcken, M., and Marycz, K. (2023). Correction: inhibition of protein tyrosine phosphatase improves mitochondrial bioenergetics and dynamics, reduces oxidative stress, and enhances adipogenic differentiation potential in metabolically impaired progenitor stem cells. Cell Commun. Signal. CCS 21, 12. doi:10.1186/s12964-023-01055-x
Paradies, G., Paradies, V., Ruggiero, F. M., and Petrosillo, G. (2017). Mitochondrial bioenergetics decay in aging: beneficial effect of melatonin. Cell. Mol. life Sci. CMLS 74, 3897–3911. doi:10.1007/s00018-017-2619-5
Paradies, G., Petrosillo, G., Paradies, V., and Ruggiero, F. M. (2010). Oxidative stress, mitochondrial bioenergetics, and cardiolipin in aging. Free Radic. Biol. Med. 48, 1286–1295. doi:10.1016/j.freeradbiomed.2010.02.020
Santiago, A. M., Gonçalves, D. L., and Morano, K. A. (2020). Mechanisms of sensing and response to proteotoxic stress. Exp. Cell Res. 395, 112240. doi:10.1016/j.yexcr.2020.112240
Sharma, A., Smith, H. J., Yao, P., and Mair, W. B. (2019). Causal roles of mitochondrial dynamics in longevity and healthy aging. EMBO Rep. 20, e48395. doi:10.15252/embr.201948395
Keywords: mitochondria, oxidative stress, bioenergetics, aging, age-related pathologies, neurodegenerative disease, cancer
Citation: Kalra RS, Kumar S and Tomar D (2023) Editorial: Oxidative stress link associated with mitochondrial bioenergetics: relevance in cell aging and age-related pathologies. Front. Cell Dev. Biol. 11:1273420. doi: 10.3389/fcell.2023.1273420
Received: 06 August 2023; Accepted: 30 August 2023;
Published: 05 September 2023.
Edited and reviewed by:
Ramani Ramchandran, Medical College of Wisconsin, United StatesCopyright © 2023 Kalra, Kumar and Tomar. This is an open-access article distributed under the terms of the Creative Commons Attribution License (CC BY). The use, distribution or reproduction in other forums is permitted, provided the original author(s) and the copyright owner(s) are credited and that the original publication in this journal is cited, in accordance with accepted academic practice. No use, distribution or reproduction is permitted which does not comply with these terms.
*Correspondence: Rajkumar Singh Kalra, rajkumar.singh@oist.jp; Subodh Kumar, subodh.kumar@ttuhsc.edu; Dhanendra Tomar, dtomar@wakehealth.edu