- 1Stazione Zoologica Anton Dohrn, Naples, Italy
- 2Developmental Biology Unit, European Molecular Biology Laboratory, Heidelberg, Germany
- 3VIB Center for Plant Systems Biology, Ghent, Belgium
- 4Department of Plant Biotechnology and Bioinformatics, Ghent University, Ghent, Belgium
Editorial on the Research Topic
Unicellular organisms as an evolutionary snapshot toward multicellularity
The emergence of multicellular organisms has been one of the major transitions during the evolutionary history of life on Earth (Smith and Szathmáry, 1995), with multicellularity evolving several times independently in the Tree of Life (Grosberg and Strathmann, 2007; Bonner, 2009; Niklas and Newman, 2013). Multicellularity can be simply defined as the result of cellular aggregation (Schirrmeister et al., 2013) or, if more stringent criteria are taken into account, as a coordinated behavior involving cell-to-cell interconnection, communication, cooperation, and differentiation (Kaiser, 2001; Wolpert and Szathmáry, 2002). For this reason, both complex organisms such as fungi, plants and animals, and, to some extent, simple microbial systems like colonial protists and biofilm-forming bacteria are considered multicellular (Grosberg and Strathmann, 2007; Hengge, 2020).
Unveiling the circumstances that caused unicellular organisms to evolve complex multicellularity and the molecular mechanisms that supported this transition is extremely challenging, considering that the first steps occurred more than 200 million years ago for plants, and 600 million years ago for animals (Herron et al., 2009; Ros-Rocher et al., 2021). Although researchers from various disciplines have studied this question for several decades, many unanswered questions remain about why unicellular organisms formed multicellular beings so frequently during evolution, which regulatory changes supported this profound change, and how organisms overcame individual self-interest in favor of altruistic or synergistic cooperation.
Typically, two changes are considered essential for the evolution of complex multicellularity: cell-cell adhesion and intercellular communication leading to coordinated action. These traits are common in unicellular species with a facultative multicellular life stage, such as green algae, fungi, slime molds, and choanoflagellates [reviewed in Brunet and King (2017)]. Indeed, many of these species show differentiation across their life cycle that, at some level, may be interpreted as developmental, although they do not involve communication among cells as sophisticated as that of multicellular organisms or cell death. Even obligate unicellular organisms can display traits that are linked to the emergence of multicellularity, such as intercellular communication, cell-cell adhesion, and differentiation into distinct developmental stages during their (a)sexual life cycles. These characteristics have been exploited in some unicellular groups, such as the yeast Saccharomyces cerevisiae, to explore the early phases of multicellularity through experimental evolution (Ratcliff et al., 2012).
Through this Research Topic, “Unicellular Organisms As An Evolutionary Snapshot Toward Multicellularity,” which consists of three Original Research articles and two Hypothesis and Theory articles (Figure 1), we wanted to emphasize the study of unicellular and simple multicellular organisms as an additional approach to understand the molecular mechanisms at the basis of multicellularity.
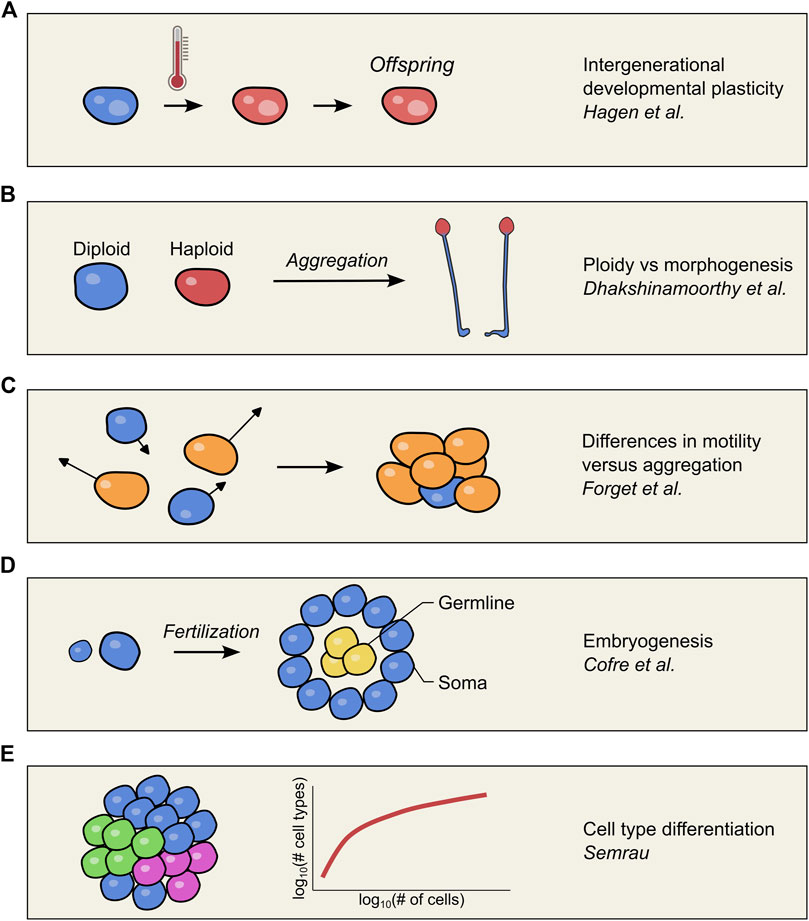
FIGURE 1. Schematic overview of the concepts investigated in each article of the Research Topic. (A) Somatic macronucleus development is affected by a heat shock in Paramecium, visualized by a red colored cell. Despite the lack of a heat shock treatment in the offspring, somatic development is still perturbed, suggesting epigenetic inheritance (Hagen et al.). (B) Haploid and diploid amoeboid cells were mixed to form a chimeric aggregate in the slime mold Dictyostelium discoideum. Cells showed a preferred localization based on their ploidy, with haploid cells differentiating into spores in the fruiting bodies (Dhakshinamoorthy and Singh). (C) Computer simulations explored how heterogeneity in the motility of free-living cells is reflected in the composition of the multicellular body after aggregation. The difference in motility between cells is shown through the length of arrows and the color of the corresponding cell (Forget et al.). (D) Cofre and Saalfeld discuss two crucial facets of embryogenesis: fertilization by unicellular gametes, and the separation of germline in yellow and the soma in blue. (E) The number of different cell types in a multicellular organism (colored cells) tends to scale allometrically with the number of cells in the organism (Semrau).
Environmental conditions may affect organismal development by stimulating long-term patterns of gene expression and inducing novel phenotypes, which can be hereditary via epigenetic mechanisms (Beldade et al., 2011). In this Research Topic, Hagen et al. exploited the process of germline to soma differentiation in the single-celled ciliate Paramecium tetraurelia to study developmental plasticity. After sexual reproduction, a polyploid, somatic macronucleus is formed from the germline micronucleus through endoreduplication and excision of internal elements. An elegant sequence-based readout system of excision profiles during somatic development not only revealed that environmental perturbation affected somatic development, but also that it was epigenetically inherited by the F1 population. This cross-generational transfer of ecological information implies that offspring cells can tune the development of a somatic nucleus to the conditions the parent experienced. This work establishes Paramecium as a new model system to study the molecular basis of developmental plasticity as an alternative to multicellular and long-lived organisms.
A cell’s ploidy triggers important phenomena in nature, such as sex determination in the Hymenoptera (Heimpel and de Boer, 2008) or the division of metabolic/cellular and reproductive labors in higher metazoans (Gavrilets, 2010; Goldsby et al., 2014). Dhakshinamoorthy and Singh showed that ploidy status influences cell fate commitment in chimeric aggregates of the amoeba Dictyostelium discoideum, where haploid cells were more prone to differentiate into spores. Their work helped to gain new perspectives on the evolution of germ-soma distinction, using D. discoideum as a model to study cell type differentiation in nascent multicellular organisms.
Multicellular organisms either arise through aggregation or mitotic cell division. During aggregation, heterogeneous sparse cells rapidly and transiently group to initiate a process of cell differentiation and division of labor to survive adverse environmental conditions (Márquez-Zacarías et al., 2021). Through computational simulation models, Forget et al. investigated how heterogeneity in the motility of adhesive particles affects the outcome of cell aggregation. The study was inspired by the life cycle of the prokaryote Myxococcus xantus and the eukaryote D. discoideum, two important model species for the transition from unicellular to multicellular through aggregation. For both species, circumstances like starvation and predation induce the formation of aggregates of genetically heterogeneous cells, which assume characteristics of labor division and differentiation (Vos and Velicer, 2009; Strassmann and Queller, 2011; Forget et al., 2021). In contrast to facultative multicellular organisms which use aggregation, obligate multicellular species undergo clonal cell division to form an embryo. The origin of the first embryo is discussed in this Research Topic by Cofre and Saalfeld, which focus on two crucial processes: the fusion of unicellular gametes, and the specification of the germline. In particular, the authors argue that the fusion between unicellular organisms led to the recruitment, through topological association in the main core of the first embryo, of independently pre-existing chromosomal domains, containing basic cancer and tumor suppressor genes. As a result, the co-regulation of these contrasting cellular processes and developmental pathways synergistically induced controlled high rates of cell proliferation. Hence, embryogenesis and cancer are considered to be two sides of the same coin, and tumor suppressors had to be simultaneously recruited to keep unbridled proliferation in check.
Finally, Semrau investigated the allometric relationship between the number of cells and the number of differentiated cell types that exist in a multicellular organism. The authors suggest an exciting new model where each new cell type that evolves in an organism is associated with a fitness cost and benefit.
The articles collected in this Research Topic use simple model systems and computer simulations to investigate several aspects of the evolution of multicellularity, ranging from embryogenesis and cellular aggregation to developmental plasticity and epigenetics. Together, they illustrate the power of using simple models systems to investigate complex evolutionary questions, leading to new insights that will contribute to shedding light on the transition from the microbial world to the plant and animal kingdom, and to stimulating future research in the field of multicellularity evolution.
Author contributions
PM: Conceptualization, Supervision, Writing–original draft, Writing–review and editing. AR: Conceptualization, Supervision, Writing–original draft, Writing–review and editing. GB: Conceptualization, Supervision, Writing–original draft, Writing–review and editing.
Funding
AR is a postdoctoral fellow supported by the Moore Foundation (Grant DIsCO 7978). GB is a postdoctoral fellow supported by Fonds Wetenschappelijk Onderzoek (FWO, 1228423N). PM was supported by a research fellowship funded by the grant PON PRIMA-Rafforzamento Del Capitale Umano, No. CIR01_00029.
Acknowledgments
We would like to thank all the authors who have contributed to this Research Topic and all the reviewers for their essential contribution to this Research Topic.
Conflict of interest
The authors declare that the research was conducted in the absence of any commercial or financial relationships that could be construed as a potential conflict of interest.
Publisher’s note
All claims expressed in this article are solely those of the authors and do not necessarily represent those of their affiliated organizations, or those of the publisher, the editors and the reviewers. Any product that may be evaluated in this article, or claim that may be made by its manufacturer, is not guaranteed or endorsed by the publisher.
References
Beldade, P., Mateus, A. R. A., and Keller, R. A. (2011). Evolution and molecular mechanisms of adaptive developmental plasticity. Mol. Ecol. 20, 1347–1363. doi:10.1111/j.1365-294X.2011.05016.x
Bonner, J. T. (2009). “First signals: the evolution of multicellular development,” in First signals (Princeton University Press).
Brunet, T., and King, N. (2017). The origin of animal multicellularity and cell differentiation. Dev. Cell. 43, 124–140. doi:10.1016/j.devcel.2017.09.016
Forget, M., Adiba, S., and De Monte, S. (2021). Social conflicts in Dictyostelium discoideum: a matter of scales. Peer Community J. 1, e58. doi:10.24072/pcjournal.39
Gavrilets, S. (2010). Rapid transition towards the division of labor via evolution of developmental plasticity. PLOS Comput. Biol. 6, e1000805. doi:10.1371/journal.pcbi.1000805
Goldsby, H. J., Knoester, D. B., Ofria, C., and Kerr, B. (2014). The evolutionary origin of somatic cells under the dirty work Hypothesis. PLOS Biol. 12, e1001858. doi:10.1371/journal.pbio.1001858
Grosberg, R. K., and Strathmann, R. R. (2007). The evolution of multicellularity: a minor major transition? Annu. Rev. Ecol. Evol. Syst. 38, 621–654. doi:10.1146/annurev.ecolsys.36.102403.114735
Heimpel, G. E., and de Boer, J. G. (2008). Sex determination in the hymenoptera. Annu. Rev. Entomol. 53, 209–230. doi:10.1146/annurev.ento.53.103106.093441
Hengge, R. (2020). Linking bacterial growth, survival, and multicellularity - small signaling molecules as triggers and drivers. Curr. Opin. Microbiol. 55, 57–66. doi:10.1016/j.mib.2020.02.007
Herron, M. D., Hackett, J. D., Aylward, F. O., and Michod, R. E. (2009). Triassic origin and early radiation of multicellular volvocine algae. Proc. Natl. Acad. Sci. 106, 3254–3258. doi:10.1073/pnas.0811205106
Kaiser, D. (2001). Building a multicellular organism. Annu. Rev. Genet. 35, 103–123. doi:10.1146/annurev.genet.35.102401.090145
Márquez-Zacarías, P., Conlin, P. L., Tong, K., Pentz, J. T., and Ratcliff, W. C. (2021). Why have aggregative multicellular organisms stayed simple? Curr. Genet. 67, 871–876. doi:10.1007/s00294-021-01193-0
Niklas, K. J., and Newman, S. A. (2013). The origins of multicellular organisms. Evol. Dev. 15, 41–52. doi:10.1111/ede.12013
Ratcliff, W. C., Denison, R. F., Borrello, M., and Travisano, M. (2012). Experimental evolution of multicellularity. Proc. Natl. Acad. Sci. U. S. A. 109, 1595–1600. doi:10.1073/pnas.1115323109
Ros-Rocher, N., Pérez-Posada, A., Leger, M. M., and Ruiz-Trillo, I. (2021). The origin of animals: an ancestral reconstruction of the unicellular-to-multicellular transition. Open Biol. 11, 200359. doi:10.1098/rsob.200359
Schirrmeister, B. E., de Vos, J. M., Antonelli, A., and Bagheri, H. C. (2013). Evolution of multicellularity coincided with increased diversification of cyanobacteria and the Great Oxidation Event. Proc. Natl. Acad. Sci. 110, 1791–1796. doi:10.1073/pnas.1209927110
Smith, J. M., and Szathmáry, E. (1995). The major transitions in evolution. Oxford: Oxford University Press.
Strassmann, J. E., and Queller, D. C. (2011). Evolution of cooperation and control of cheating in a social microbe. Proc. Natl. Acad. Sci. U.S.A. 108, 10855–10862. doi:10.1073/pnas.1102451108
Vos, M., and Velicer, G. J. (2009). Social conflict in centimeter-and global-scale populations of the bacterium Myxococcus xanthus. Curr. Biol. 19, 1763–1767. doi:10.1016/j.cub.2009.08.061
Keywords: unicellular organism, evolution, multicellular organism, development, differentiation, cell cooperation
Citation: Marotta P, Ruggiero A and Bilcke G (2023) Editorial: Unicellular organisms as an evolutionary snapshot toward multicellularity. Front. Cell Dev. Biol. 11:1254636. doi: 10.3389/fcell.2023.1254636
Received: 07 July 2023; Accepted: 10 August 2023;
Published: 17 August 2023.
Edited by:
James A. Coffman, Mount Desert Island Biological Laboratory, United StatesReviewed by:
Pedro Martinez, University of Barcelona, SpainCopyright © 2023 Marotta, Ruggiero and Bilcke. This is an open-access article distributed under the terms of the Creative Commons Attribution License (CC BY). The use, distribution or reproduction in other forums is permitted, provided the original author(s) and the copyright owner(s) are credited and that the original publication in this journal is cited, in accordance with accepted academic practice. No use, distribution or reproduction is permitted which does not comply with these terms.
*Correspondence: Pina Marotta, cGluYW1hcm90dGE4MkBnbWFpbC5jb20=