- 1Department of Small Mammal, Reptile and Avian Diseases, University of Veterinary Medicine Hannover, Hanover, Germany
- 2Laboklin GmbH and Co., KG, Bad Kissingen, Germany
Salmonella are considered a part of the normal reptile gut microbiota, but have also been associated with disease in reptiles. Reptile-associated salmonellosis (RAS) can pose a serious health threat to humans, especially children, and an estimated 6% of human sporadic salmonellosis cases have been attributed to direct or indirect contact with reptiles, although the exact number is not known. Two literature searches were conducted for this review. The first evaluated reports of the prevalence of Salmonella in the intestinal tracts of healthy reptiles. Salmonella were most commonly detected in snakes (56.0% overall), followed by lizards (36.9%) and tortoises (34.2%), with lower detection rates reported for turtles (18.6%) and crocodilians (9%). Reptiles in captivity were significantly more likely to shed Salmonella than those sampled in the wild. The majority of Salmonella strains described in reptiles belonged to subspecies I (70.3%), followed by subspecies IIIb (29.7%) and subspecies II (19.6%). The second literature search focused on reports of RAS, revealing that the highest number of cases was associated with contact with turtles (35.3%), followed by lizards (27.1%) and snakes (20.0%). Reptiles associated with RAS therefore did not directly reflect prevalence of Salmonella reported in healthy representatives of a given reptile group. Clinical symptoms associated with RAS predominantly involved the gastrointestinal tract, but also included fever, central nervous symptoms, problems with circulation, respiratory symptoms and others. Disease caused by Salmonella in reptiles appears to be dependent on additional factors, including stress, inadequate husbandry and hygiene, and other infectious agents. While it has been suggested that reptile serovars may cause more severe disease than human-derived strains, and some data is available on invasiveness of individual strains in cell culture, limited information is available on potential mechanisms influencing invasiveness and immune evasion in reptiles and in RAS. Strategies to mitigate the spread of Salmonella through reptiles and to reduce RAS focus mostly on education and hygiene, and have often been met with some success, but additional efforts are needed. Many aspects regarding Salmonella in reptiles remain poorly understood, including the mechanisms by which Salmonella persist in reptile hosts without causing disease.
1 Introduction
The role of Salmonella in reptiles and their zoonotic potential has been discussed for decades, and this discussion has, in some cases, been controversial. Reptile-associated salmonellosis (RAS) has been considered a threat to public health since the 1970’s, and a wide range of restrictions and measures have been suggested and in some cases implemented over the years in different countries around the world in order to mitigate the associated risk. A large number of scientific studies have focused on the detection of Salmonella strains in reptiles as well as their role in both reptile and human health.
Salmonella are often considered a normal part of the reptile gut microbiome (Pasmans et al., 2021). However, studies on the prevalence of these bacteria in specific reptile species and populations have led to widely varying numbers (e.g., Maciel et al., 2010; Scheelings et al., 2011). In addition, Salmonella have regularly been associated with disease in individual reptiles (e.g., Clancy et al., 2016b) as well as in disease outbreaks in groups of animals (e.g., Ramsay et al., 2002; Sakaguchi et al., 2017).
In general, the Salmonella detection rate in reptiles is highly dependent on the sample type, sampling technique, time of sampling, and sample quality, as well as on the technique used for detection, complicating the comparison of various studies (e.g., Marin et al., 2013; Fagre et al., 2020). A wide variety of methods, including pre-enrichment in various media and the use of a variety of agar types and incubation temperatures have been used for the detection of Salmonella in reptiles. Only few studies cite specific guidelines, such as the ISO 6579-1:2017 for the isolation of Salmonella from reptile samples (Zając et al., 2021; Merkevičienė et al., 2022). PCR is also frequently used for the detection of Salmonella in reptiles (e.g., Sánchez-Jiménez et al., 2011; Fagre et al., 2020; Marenzoni, 2022; Vorbach et al., 2022).
Studies focusing on describing the intestinal microbiome in various reptiles using molecular methods rather than culture have not found Salmonella to be a major part of the microbiome (e.g., Keenan and Elsey, 2015; Jiang et al., 2017; Ahasan et al., 2018; Shang et al., 2023) and in many cases, Salmonella has only been found in limited amounts under specific circumstances in studies evaluating the gut microbiome of specific species. For example, Salmonella were only detected in a single green turtle (Chelonia mydas) after rehabilitation, but not in any animals tested pre-hospitalization, leading authors to hypothesize that living under human care could be involved in colonization of the intestine with Salmonella and possibly spread in the environment (Ahasan et al., 2018). Similarly, Jiang et al. found that the presence of Salmonella in the gut microbiome of captive crocodile lizards (Shinisaurus crocodilurus) was dependent on what they were fed (Jiang et al., 2017). Generally, the microbiome in reptiles is reported to be sensitive to environmental factors, such as temperature, with small changes leading to shifting and destabilization (Bestion et al., 2017; Zhang, 2022).
There are reports documenting that some reptiles are colonized with Salmonella very early in life, possibly through the eggshell (Holgersson et al., 2016) as well as during pregnancy and birth (Schroter et al., 2006) indicating that Salmonella spp. can contribute to the physiological microbiome early in life. Other modes of transmission include prey or environmental contamination.
The risk of spreading Salmonella spp. from reptiles to humans (RAS), especially from pet reptiles to exposed children, has been known for decades. However, the main reptile species reported transmitting Salmonella spp. have changed over the years and may vary among different age groups of affected humans (De Jong et al., 2005; Sauteur et al., 2013). Transmission may also depend on the countries or continents evaluated, as different pet reptiles may be popular in different areas (Bertrand et al., 2008; Valdez, 2021). Originally, mostly small turtles such as Trachemys spp. (formerly Pseudemys spp.) were reported as risk factors and potential transmitters, especially in young children (Williams, 1965; Altman et al., 1972; Bradley et al., 2001). However, in the USA, this led to a ban on selling turtles with shell diameters smaller than 4 inches in 1975 to prevent children from putting them in their mouths (Hatt et al., 2009). This ban led to a drastic reduction in the number of human salmonellosis cases in the USA (Bradley et al., 2001). In the last decades, a shift towards lizards as the most likely transmitters for RAS has been noted (Böhme et al., 2009; Pees et al., 2013; Whitten et al., 2015; Whiley et al., 2017; Kiebler et al., 2020). In particular, bearded dragons (Pogona spp.) have been reported as a source of salmonellosis globally, including in Germany (Böhme et al., 2009; Haase et al., 2011; Weiss et al., 2011; Pees et al., 2013; Whiley et al., 2017), the United Kingdom (Cooke et al., 2009), the Netherlands (Berendes et al., 2007), Australia (Moffatt et al., 2010) and the USA (Lang et al., 2007; Tabarani et al., 2010; Lowther et al., 2011). However, other reptile species such as chameleons (Willis et al., 2002), geckos, anoles, snakes (Whitten et al., 2015; Krishnasamy et al., 2018) and iguanas (Nowinski and Albert, 2000; Cellucci et al., 2010) have also been reported as sources of human infection. Salmonellosis associated with turtles is also still reported regularly around the world (Harris et al., 2010; Basler et al., 2015; Kuroki et al., 2015; Walters et al., 2016; Lane et al., 2019; Lane et al., 2019; Okabe et al., 2021; Waltenburg et al., 2022).
RAS is believed to account for at least 6% of all human salmonellosis cases, depending on region and age of the affected patients (Bradley et al., 2001; Wells et al., 2004; Meletiadis et al., 2022). It is usually a problem of children rather than adults (Ackman et al., 1995; Willis et al., 2002; Harris et al., 2010; Weiss et al., 2011; Lafuente et al., 2013; Whitten et al., 2015). Some studies also indicate that RAS occurs more often in younger children than salmonellosis of other origins and may also be associated with an increased hospitalization rate (Colomb-Cotinat et al., 2014; Sauteur et al., 2013; Murphy and Oshin, 2015). Especially young children are prone to severe manifestations with dehydration, sepsis, and meningitis which may be life-threatening (Hatt et al., 2009; Haase et al., 2011). One study also found younger children to be more prone to severe disease resulting in hospitalization (Cellucci et al., 2010). However, there are also multiple case reports describing RAS in adults (Stam et al., 2003; Brenneman et al., 2022; Castlemain and Castlemain, 2022) and a few years ago, a shift towards RAS cases in adults was reported (Mughini-Gras et al., 2016). Comorbidities are frequently reported in adults that develop bacteriaemia (Lane et al., 2019; Barry and Finn, 2022; Brenneman et al., 2022). Common clinical signs of RAS include gastrointestinal symptoms such as (bloody) diarrhea, vomitus, abdominal cramping as well as fever (Willis et al., 2002; Böhme et al., 2009; Harris et al., 2010; Weiss et al., 2011; Colomb-Cotinat et al., 2014). As mentioned above, invasive cases have been described and can encompass septicemia, meningitis, and bone or joint infection (Nowinski and Albert, 2000; Lang et al., 2007; Van Meervenne et al., 2009; Colomb-Cotinat et al., 2014; Sauteur et al., 2013; Murphy and Oshin, 2015).
This review aimed to collect end evaluate published data on Salmonella in reptiles, with a focus on their detection, interactions with their hosts, and relevance as a zoonotic pathogen. Special emphasis was placed on scientific papers published within the last 2 decades to provide a comprehensive overview and interpretation of the state of research, but also to determine gaps in our understanding of these bacteria in reptile hosts and necessary research steps for the future.
2 Salmonella as pathogens in reptiles
Despite the frequent detection of Salmonella in healthy reptiles and their possible role in the normal reptile microbiome, these bacteria have also been associated with disease in many cases. Disease outbreaks affecting multiple animals within a collection have also been described (Sakaguchi et al., 2017). However, the role of Salmonella as primary pathogens in reptiles is not always clear. Evaluation of their role in disease processes can be challenging. Several possible predisposing factors have been reported for the development of clinical salmonellosis in reptiles. These include stress (Clancy et al., 2016a; Bertolini et al., 2021), parasite infestations (Sting et al., 2013), viral disease (Sting et al., 2013), metabolic disease (Sting et al., 2013), and inappropriate husbandry (Bertolini et al., 2021). Snakes have been reported to have a higher risk of salmonellosis, followed by lizards, and crocodilians, with the lowest risk of disease reported for chelonians (Grupka et al., 2006; Clancy et al., 2016a). Snakes seem especially prone to clinical diseases caused by Salmonella ssp. IIIa and IIIb (Grupka et al., 2006). Clinical signs of a Salmonella-associated disease in reptiles are highly variable and often non-specific. Septicaemia (Orós et al., 1996; Mitchell and Shane, 2001; Téllez et al., 2002; Grupka et al., 2006; Köbölkuti et al., 2013) pneumonia (Orós et al., 1996; Mitchell and Shane, 2001; Schroff et al., 2010; Sakaguchi et al., 2017), enterocolitis (Sakaguchi et al., 2017), abscesses (Barboza et al., 2018; Hyeon et al., 2021), osteomyelitis, especially in snakes (Isaza et al., 2000; Ramsay et al., 2002; Grupka et al., 2006; Di Girolamo et al., 2014; Clancy et al., 2016b; Bertolini et al., 2021), dermatitis (Clancy et al., 2016a; Sakaguchi et al., 2017; Bertolini et al., 2021), splenitis (Sakaguchi et al., 2017; Bertolini et al., 2021), and hepatitis (Bertolini et al., 2021) have all been described in multiple cases. Clinical signs observed can include anorexia, lethargy, cachexia, paresis, dyspnoea, hypovolemic shock, and sudden death (Ramsay et al., 2002; Jacobson, 2007; Bölcskei et al., 2009; Gorman, 2010; Mayer and Donnelly, 2012; Silva-Hidalgo et al., 2014; Clancy et al., 2016a).
Pathological and histological changes associated with cases of reported salmonellosis include necrotizing inflammation in various tissues including e.g., the intestine, spleen, and lung in affected crocodilians (Sakaguchi et al., 2017), and renal granulomas in sea turtles (Work et al., 2019). Necrotizing and granulomatous inflammation have also characterized lesions associated with Salmonella in a wide variety of tissues in various snake species including the gastro-intestinal tract, liver, spleen, blood vessels, and myocardium (Pasmans et al., 2021). Snakes with osteomyelitis associated with Salmonella infections have been found to have diffuse heterophilic-granulomatous osteomyelitis and discrete heterophilic granulomas (Ramsay et al., 2002).
3 Systematic review
3.1 Salmonella detection in healthy reptiles
To evaluate the occurrence of Salmonella spp. in healthy reptiles throughout the world and among specific animal groups, a systematic literature review was conducted (modified PRISMA flow diagram see Supplementary Data Sheet S1) including the Pubmed query: [(salmonella) AND (reptile) AND (prevalence)] AND {[“2003/01/01” (Date—Publication): “3,000” (Date—Publication)]}. On 14 April 2023, this resulted in a total of 219 results published between 2003 and 2023. Inclusion criteria for further evaluation were: at least 10 individual animals tested, sample location (e.g., cloaca) provided, no clinical signs of disease reported, at least one enrichment procedure conducted, and identification of isolates at least to subspecies level. Reviews were excluded from further evaluation. This resulted in 77 studies that were further evaluated. A summary of the obtained data is given in Tables 1, 2. Data points used for the evaluation and reference to all studies included are provided in Supplementary Data Sheet S2. For the prevalence results, statistical analysis for significant differences was performed using the commercial software SPSS 28.0 (IBM, Armonck, United States) and the Mann-Whitney-U-Test. A significance was assumed with p ≤ 0.05.
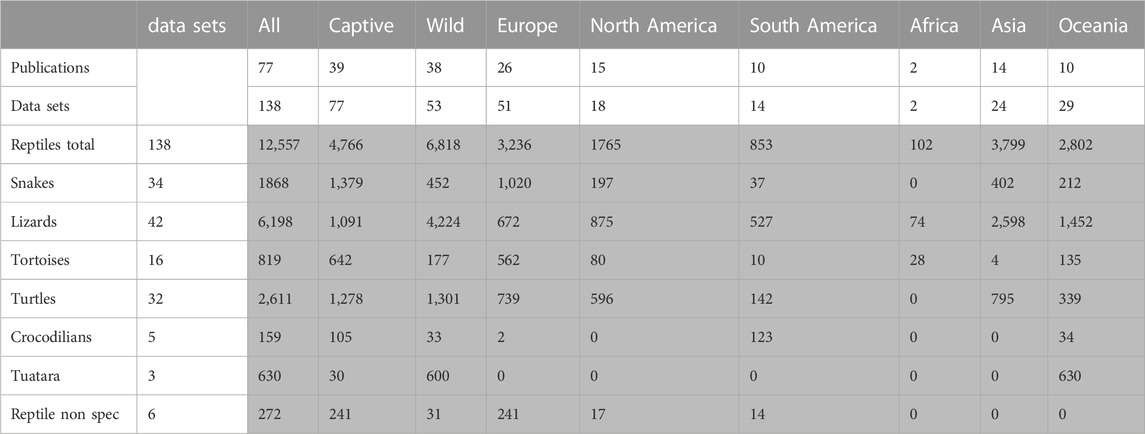
TABLE 1. Literature review of prevalence of Salmonella in healthy reptiles, number of studies and total number of animals included (grey) (reptile nonspec. = data could not be assigned to a specific reptile group). Data points used for the evaluation and references to all studies included are provided in Supplementary Data Sheet S2.
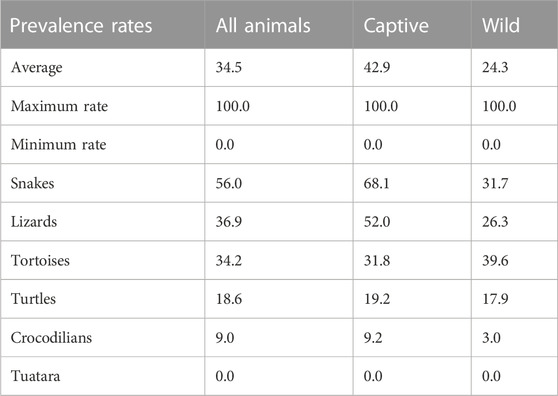
TABLE 2. Literature review of prevalence of Salmonella in healthy reptiles, reported Salmonella prevalences [% of animals].
Altogether, results from 12,557 individual reptiles were included. Prevalence studies were conducted on all continents except Antarctica, and many studies included reptiles from different groups or studies from both captive and wild animals. The data were evaluated for six different reptile groups (snakes, lizards, tortoises, turtles, crocodilians, tuatara) corresponding in part to taxonomic order (crocodilians, tuatara) or family (tortoises) and in part to lifestyle (turtle) or recognized groups within a joint order (snakes and lizards), as well as source (wild versus captive, or unclear). The data contained in the 77 publications were divided into 138 data sets: each for a single reptile group and source. These sets were the basis for all evaluations and calculations made here (Table 1). The methods used for species and serovar identification in the survey conducted here were also compared. Of all data evaluated, characterization of the Salmonella isolates was based on serological methods in 111 data sets. Molecular methods were used for characterization in 27 data sets. In 14 of these 27, both molecular and serological methods were used. The method used for identification was not clearly described in 14 data sets, either because no Salmonella were detected in the respective study, or due to reference to an external laboratory without explaining the method. Altogether, 86 data sets reported complete serovar identification, only a subset of the isolates were further characterized in 15 datasets, and identification was only reported to the subspecies level in 13 datasets. Details on the methods used in individual studies are reported in Supplementary Data Sheet S2. Statistical evaluation was only conducted for the reported prevalence depending on the source and the reptile group (crocodilians and tuataras were excluded due to the low number of data sets). A statistical evaluation of other results was deemed unreliable due to differences in the study designs.
Most frequently, reptile samples examined for Salmonella spp. are of cloacal or fecal origin, but studies on detection in tissue or environmental samples are also available. In our literature review, 73.9% of the samples analysed were cloacal swabs, while 37.7% were feces, and 15.9% were other types of samples. Comparing different studies regarding the time between collection and arrival of the samples in the laboratory, these ranged from less than 24 h (e.g., Corrente et al., 2017; Bjelland et al., 2020; Baling and Mitchell, 2021; Merkevičienė et al., 2022; Song et al., 2023) up to 72 h (e.g., Zając et al., 2021).
A mean Salmonella prevalence of 34.5% was calculated overall for all groups and studies. The prevalence reported in the studies varied between the reptile groups, with the highest rate of 56.0% in snakes, lower in turtles (18.6%) and the lowest in crocodilians (9.0%) and tuatara (0.0%), the latter two based on a limited number of data sets (Table 2; Figure 1). The differences in the reported prevalence were significant between snakes and lizards (p = 0.025), tortoises (p = 0.047), and turtles (p ≤ 0.001). Salmonella detection rates were also significantly lower in turtles than in lizards (p ≤ 0.001). Regarding the source of the reptile, a significantly (p = 0.002) higher mean Salmonella prevalence was noted for those reptiles living in captivity than for those sampled in the wild (Figure 2). A higher prevalence in captive animals could also be confirmed within the reptile groups except for tortoises, being significant for the group of snakes (p = 0.002) and lizards (p = 0.002). In general, the range of the reported prevalence was very broad and ranged between zero or close to zero and 100%. This broad range was found for all reptiles as well as for each group and source separately.
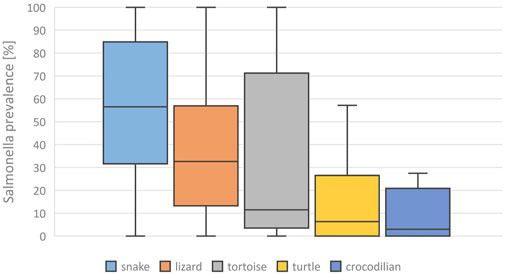
FIGURE 1. Evaluation of reported detection rates of Salmonella in healthy reptiles, from literature published between 2003 and 2023, based on 138 data sets (for each reptile group and source) extracted from 77 individual publications: Salmonella prevalences [%] reported for different reptile groups, Boxplots (median, 1. and 3. quartile, maximum reported detection rate).
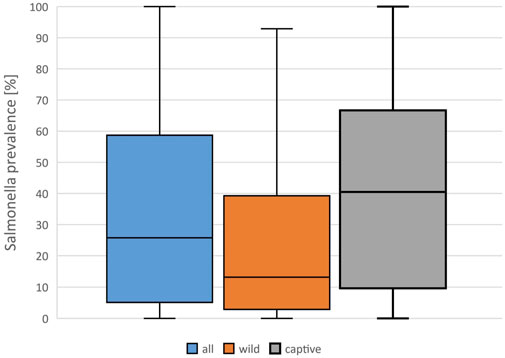
FIGURE 2. Evaluation of reported detection rates of Salmonella in healthy reptiles, from literature published between 2003 and 2023, based on 138 data sets (for each reptile group and source) extracted from 77 individual publications: Salmonella prevalences [%] reported in all data sets, and results for captive and wild reptiles, Boxplots (median, 1. and 3. quartile, maximum reported detection rate).
Besides the detection rate, the detected serovars also varied greatly between the different studies. By far the most frequently identified serovars in the analyzed studies belonged to subspecies I (70.3% reported rate per data sets), followed by subspecies IIIb (29.7%), and subspecies II (19.6%) (Figure 3). This dominance of subspecies I serovars was also seen for both captive and wild reptiles, although the detection rates for serovars from individual subspecies differed between wild and captive reptiles, and Salmonella ssp. V isolates were only reported from wild reptiles. For subspecies I, a broad range of serovars were reported in the evaluated publications, with the following serovars named most often (this list is not exhaustive): Abony, Amsterdam, Eastbourne, Fluntern, Halle, Heron, Java, Javiana, Kottbus, Newport, Oranienburg, Pomona, Poona, Potsdam, Rubislaw, Weltevreden.
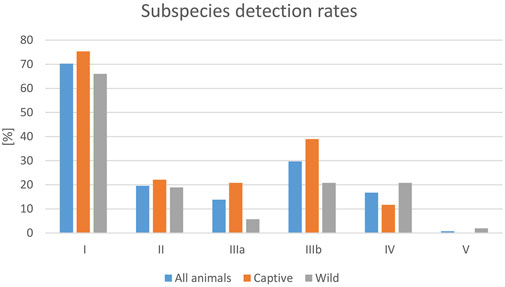
FIGURE 3. Evaluation of reported detection rates of Salmonella in healthy reptiles, from literature published between 2003 and 2023, based on 138 data sets (for each reptile group and source) extracted from 77 individual publications: frequencies of Salmonella subspecies reported [%].
3.2 Reptile-human transmission
To evaluate reports on reptile-associated salmonellosis (RAS) throughout the world with regard to occurrence, symptoms, and reptile species involved, a systematic literature review was conducted [modified PRISMA flow diagram see Supplementary Data Sheet S3) using the formula: Pubmed query: {[(salmonella) AND (reptile) AND [(human) or (zoonosis) or (RAS)]} AND {[“2003/01/01” (Date—Publication): “3,000” (Date—Publication)]} NOT (review)]. On 17 May 2023, this resulted in a total of 264 results published between 2003 and 2023. Inclusion criteria were a clear association of Salmonella spp. infection in humans and reptile contact, further classification of reptile contact and not just mentioned as a possible risk factor, no review article as well as text written in English, French, or German, resulting in 53 studies that were further evaluated. A summary of the obtained data is given in Table 3. Data points used for the evaluation and reference to all studies included are given in Supplementary Data Sheet S4. As for the prevalence survey, data was evaluated for six different reptile groups (snakes, lizards, tortoises, turtles, crocodilians, tuatara), as well as the source of the reptile (wild, pet, unknown). The data contained in the 53 publications were divided into 85 data sets: each for a single reptile group and source. Overall, 3,025 human cases were described. Studies were conducted mainly in Europe (47) and North America (32), and a few studies in Asia (3), Oceania (2), and Africa (1). The data was evaluated for five different reptile groups [snakes, lizards, tortoises, turtles, and reptile non-specific (for cases in which the information provided in the publication did not allow a clear identification of the reptile group involved)]; no infections associated with crocodilians or tuataras were reported in any of the evaluated studies. In 79 data sets, identification of the Salmonella isolates to the serovar level was confirmed, whereas in 6 studies, identification was incomplete. Most studies used molecular methods, including pulsed field gel electrophoresis (42) for isolate characterization, 34 used serologic methods (10 additionally to molecular methods). In 24 studies, identification methods were inconsistent or not clearly described (details in Supplementary Data Sheet S4). Reports on Salmonella subspecies and animal groups involved were only compared statistically between Europe and North America, as the number of studies on other continents was limited (SPSS 28.0 (IBM, Armonck, United States); Mann-Whitney-U-Test, significance assumed with p ≤ 0.05). As RAS is reported to affect especially young children, we calculated three age groups (less than 1 year, 1–4 years, and older than 4 years). The age reporting rates detected are shown in Figure 4.
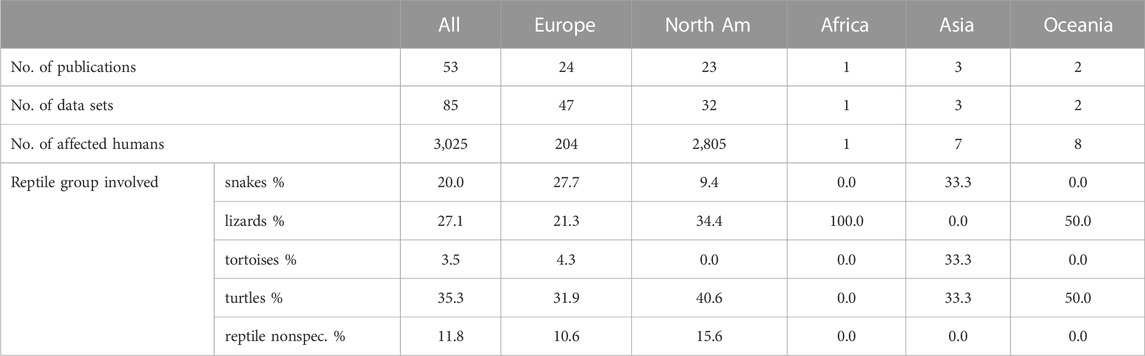
TABLE 3. Literature review of reptile-associated salmonellosis (RAS): Number of data sets, total number of humans involved, and frequency of reptile groups reported to be involved [% of data sets] (reptile nonspec. = reptile data could not be assigned to a specific reptile group). Data points used for the evaluation and references to all studies included are provided in Supplementary Data Sheet S4.
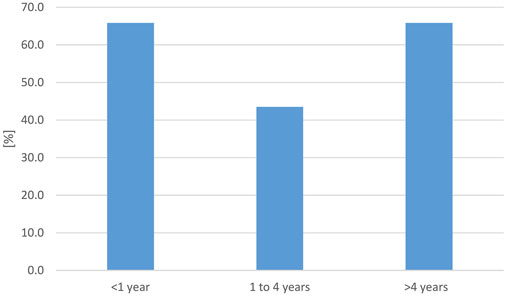
FIGURE 4. Evaluation of reported reptile-associated salmonellosis (RAS), from literature published between 2003 and 2023, based on 79 data sets (for each reptile group and source) extracted from 53 individual publications: percentage [%] of data sets that reported the respective age group to be affected by reptile-associated salmonellosis (RAS). Many reports included information on several affected age groups.
The majority of the reports included turtles (35.3%), lizards (27.1%), and snakes (20%), whereas only two studies in Europe and one study in Asia (3.5%) mentioned tortoises (Table 3). The reptile groups most frequently involved in RAS differed between Europe and North America (Figure 5), although the highest number of cases was reported to involve turtles on both continents. The difference in frequency of an association with contact with snakes was significant between these two continents (p = 0.035). The specific reptile species involved in RAS cases were often not provided. For those cases in which species was provided, the species mentioned most often were bearded dragons (Pogona vitticeps, 14 studies), followed by sliders (Trachema scripta spp., seven studies), green iguanas (Iguana iguana, six studies) and Chinese water dragons (Physignathus coincinus, five studies).
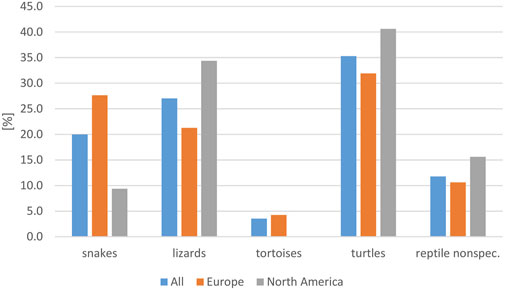
FIGURE 5. Evaluation of reported reptile-associated salmonellosis (RAS), from literature published between 2003 and 2023, based on 79 data sets (for each reptile group and source) extracted from 53 individual publications: frequency of reptile groups reported as the source of reptile-associated salmonellosis (RAS), for all data sets evaluated and for Europe and North America (reptile nonspec. = reptile data could not be assigned to a specific reptile group).
Although most studies on RAS were published in Europe, the number of individually affected humans was much higher in North America, due to the higher number of reported individuals per study. With respect to the isolated serovar in human cases, in 49.4% of all studies, the respective serovar was confirmed in a reptile. In 89.4% of all studies, a Salmonella subspecies I serovar was isolated, followed by a detection rate of 12.9% of subspecies IV and 10.6% of subspecies IIIa. The isolation rates are shown in Figure 6 for North America and Europe. Subspecies IIIa and IIIb were found to be significantly more often reported in RAS cases in Europe than in North America (p = 0.019 and p = 0.012). The subspecies I serovars most often reported included (this list is not exhaustive): Pomona (13/53), Poona (8/53), Apapa (5/53), Monschaui (4/53), Muenich (4/53), Oranienburg (4/53), Cotham (3/53), and Newport (3/53).
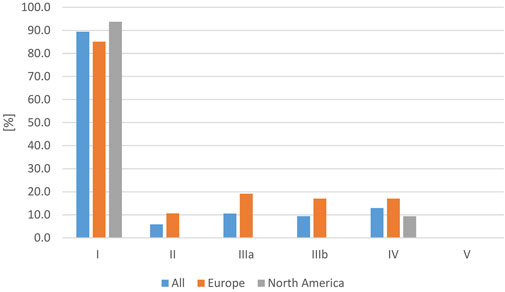
FIGURE 6. Evaluation of reported reptile-associated salmonellosis (RAS), from literature published between 2003 and 2023, based on 79 data sets (for each reptile group and source) extracted from 53 individual publications: frequency of Salmonella subspecies reported as a cause of reptile-associated salmonellosis (RAS) in all studies as well as only in Europe and only in North America in % of data sets in which each subspecies was reported [%].
Transmission of Salmonella between reptiles and humans can occur due to direct or indirect contact with infectious reptile-associated material. Indirect contact leading to RAS is frequently reported and may include living in the same home (Tabarani et al., 2010; Weiss et al., 2011; Colomb-Cotinat et al., 2014), contact with infected feeder mice (Harker et al., 2011; Cartwright et al., 2016; Kanagarajah et al., 2018; Marin et al., 2018; Vrbova et al., 2018), or contaminated surfaces, e.g., of reptile enclosures (Friedman et al., 1998; Hatt et al., 2009). This has even been described several weeks after the removal of the reptile (Weiss et al., 2011). Spread of RAS throughout the home is also possible, as reptile-associated Salmonella has even been found in vacuum cleaners (Whiley et al., 2017). Humans may also serve as vectors, e.g., an adult working in a pet reptile shop transmitting Salmonella to a child at home or a reptile owner transmitting Salmonella during a visit to another home (Weiss et al., 2011). In our literature review, about one-quarter of the publications (25.9%) included details on a direct infection, and one-quarter on indirect contamination (27.1%).
Described symptoms in affected humans were classified in the following categories: gastrointestinal symptoms (including diarrhea, vomiting, abdominal cramps, nausea), fever, respiratory symptoms, central nervous and/or circulatory symptoms (including meningitis, signs of sepsis, shock), and other symptoms. Symptoms in multiple categories were possible and gastrointestinal symptoms were most commonly reported (Figure 7).
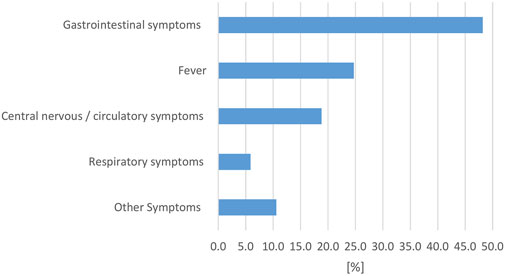
FIGURE 7. Evaluation of reported reptile-associated salmonellosis (RAS), from literature published between 2003 and 2023, based on 79 data sets (for each reptile group and source) extracted from 53 individual publications: percentage [%] of data sets that reported the respective clinical symptom complex in human cases with reptile-associated salmonellosis (RAS).
4 Discussion
Despite a plethora of publications and studies documenting Salmonella carriage in reptiles, there are still many open questions regarding the interaction between Salmonella, reptiles, the environment, the reptile immune system, and disease, as well as the factors influencing transmission between reptiles and humans and options for mitigating the risks associated with Salmonella in reptiles. These are questions that are of interest for human and veterinary medicine as well as for environmental health and conservation.
4.1 Salmonella in reptiles
The overview of recent (in the past 20 years) studies on the detection of Salmonella in healthy reptiles conducted for this review supports a wide range of previous work indicating both that these bacteria are common in the intestinal tract of reptiles and that their distribution can vary widely under various circumstances and in various environments and reptile species. Variations in samples and methods employed for Salmonella spp. detection are also known to influence detection rates and methods used for Salmonella detection in reptiles can vary widely.
The differences in Salmonella prevalence found in this literature search are similar to those reported previously in a number of individual studies. Specifically, lower detections rates in chelonians in comparison to snakes or lizards have been repeatedly reported (Geue and Löschner, 2002; Scheelings et al., 2011; Hydeskov et al., 2013; Lukac et al., 2015; Corrente et al., 2017; Bjelland et al., 2020; Cota et al., 2021; Zając et al., 2021). However, other studies have found a higher prevalence in chelonians (Ebani et al., 2005; Bertelloni et al., 2016). Differences in prevalence between wild-caught animals and captive collections have also been reported, with generally lower detection rates in wild animals (Geue and Löschner, 2002; Scheelings et al., 2011).
A lack of detection of Salmonella in a sample should always be considered as a snap shot, with limited value for a permanent assessment. Reptiles that carry Salmonella in their gastro-intestinal tracts have repeatedly been shown to shed these bacteria irregularly (Maciel et al., 2010; Goupil et al., 2012; McWhorter et al., 2021), and shedding is known to be influenced by a variety of factors including husbandry conditions (climate, hygiene, diet). For example, carnivores have been shown to be more prone to increased shedding, especially if rodents are fed (Scheelings et al., 2011). Stress has been shown to increase shedding in various reptiles (DuPonte et al., 1978; Smith et al., 2012; Marin et al., 2013). Beside these variations, almost all studies evaluated focused on the qualitative detection of Salmonella, but—in contrast to the food industry—not on a quantitative assessment of the number of bacteria shed by reptiles. Our findings indicate that shedding of Salmonella is influenced by both the reptile group and the reptile habitat, which can have implications for zoonotic risk assessment. Among the reptile groups, snakes were most often Salmonella positive, with significantly higher detection rates found in this group than in all other reptile groups evaluated. Interestingly, the shedding frequency for individual reptile groups did not correlate with their apparent importance in RAS–we address this discrepancy below.
The physiological role of Salmonella in reptiles is still unclear. Saprophytic relationships have been suggested (Jacobson, 2007; Baling and Mitchell, 2021). The pathogenesis of salmonellosis in reptiles is still not understood, but there are several extrinsic and intrinsic factors that are discussed to have a significance influence on the colonization as well as disease development in reptiles:
Following colonization of the gut, translocation across the gut wall and hematogenous spread throughout the body can occur and can lead to systemic disease (Wellehan and Gunkel, 2004). Pasmans et al. (2003) demonstrated the role of the reptile intestinal mucosal layer as the site for Salmonella colonization, but also as a protection against invasion of the underlying tissue structures and systemic spread. Detection in inner organs with no detectable inflammation or clinical signs has also been reported following oral administration of Salmonella isolates in healthy bearded dragons (P. vitticeps) (Pasmans et al., 2013; Renfert et al., 2021). In addition to the role of the intestinal barrier, other tissues have also been hypothesized to be possible entry ways for systemic salmonellosis. Lung tissue was found to be a possible entry site for Salmonella spp. infections, especially after initial viral damage to the epithelium. In snakes, Salmonella have been reported to be part of the oral cavity microbiota, but not a normal finding in the lower airways (Plenz et al., 2015). However, after experimental application of a ferlavirus (a virus targeting the lung epithelium cells) in corn snakes (Pantherophis guttatus), Salmonella spp. was found to be the dominant bacterium causing pneumonia (Pees et al., 2019). A further study demonstrated a massive immune response to this secondary infection including interstitial infiltration of heterophils and mononuclear cells (Starck et al., 2017). A case report on a Burmese python (Python molurus) suffering from pneumonia with subsequent development of endocarditis valvularis involving Salmonella indicates that lung infections may lead to hematogenous dissemination of Salmonella to internal organs (Schroff et al., 2010).
Several studies (Pasmans et al., 2002; Clancy et al., 2016a; Bertolini et al., 2021) have suggested that the disparity in shedding and clinical signs observed in Salmonella spp. infection between mammals and reptiles may be attributed to differences in their immune systems. In mice, the entry of Salmonella spp. into the intestinal wall through Peyer’s patches and M-like cells has been extensively documented. However, the presence of Peyer’s patches has not been observed in reptilian species, although M-cell-like structures have been identified in the esophagus of adult turtles (Pasmans et al., 2002). This absence of Peyer’s patches and M-like cells in reptiles could explain why Salmonella typically does not invade the intestinal wall in reptiles that carry these bacteria in their guts.
Temperature likely also influences the replication and distribution of Salmonella in ectothermic reptiles. Pasmans et al., 2002 demonstrated that intestinal infection with Salmonella Muenchen can occur in a turtle (Trachemy scripta ssp. scripta) when kept at a temperature of 37°C, which is similar to the core temperature of mammals. The ability of Salmonella to invade intestinal tissue and colonize internal organs at temperatures typical of homeothermic animals may be attributed to several factors. Firstly, higher temperatures promote increased bacterial growth and higher shedding numbers due to enhanced doubling rates. Additionally, elevated temperatures could disrupt the normal functioning of the intestinal barrier, leading to invasion (Pasmans et al., 2002). Temperature has also been hypothesized to influence intra-intestinal biofilm formation and regulation of Salmonella spp. in reptiles (Renfert et al., 2021). These poikilothermic animals, with their dependence on external sources of heat for temperature regulation and diurnal changes in body temperature, may exhibit differences in biofilm formation compared to homeothermic organisms, as no biofilm formation was reported at 37°C, but was found below specific temperature thresholds (30°C) (Gerstel and Romling, 2001; Speranza et al., 2011).
Differences in virulence factors of specific Salmonella serovars at different temperatures may contribute to increased invasiveness (Pasmans et al., 2002). The influence of body temperature on the induction of Salmonella pathogenicity islands I and HilA genes, which play a major role in the intestinal phase of infection in homeotherms, remains unknown. Individual reports have indicated that specific serovars may be more pathogenic for some reptiles than others (Bemis et al., 2007), but this has not been sufficiently studied to differentiate between effects specific to a bacterial strain and effects of host species, immune status, and environment.
4.2 Reptile-human transmission of Salmonella
The second survey conducted in this review aimed to provide an overview of recent (from the past 2 decades) reports on human salmonellosis associated with reptiles, RAS. Again, it is important to acknowledge the limitations of this survey, including the same constraints mentioned for the prevalence review. Another restriction is the limitation of published studies available almost exclusively to Europe and North America. This may be due to differences in attitudes toward publication of such cases. We consider it unlikely that the bias in numbers of publications represents a difference in inherent risk of RAS in different parts of the world, although cultural differences in keeping and treatment of pet reptiles could play a role.
A consistent finding across the reports was that turtles were the reptile group most frequently named as a source of RAS, followed by lizards and, notably, snakes. The involvement of lizards and snakes varied, with lizards more often associated with RAS in North America. Interestingly, tortoises did not play a significant role in the reports, being identified as a source in only 3.5% of the cases. This is noteworthy considering the popularity of tortoises as pets, and the finding in the review of Salmonella prevalence that they frequently carry Salmonella (35% compared to 18% in turtles). Several factors likely contribute to these discrepancies:
Firstly, the study data do not directly reflect the popularity of specific reptile groups as pets, and therefore the possible exposure to humans. It is difficult to obtain reliable data on popularities of reptile species as pets. However, a study using google trends for analysis of changes in the reptile pet trade found that bearded dragons are currently by far the most popular reptiles traded, especially in the USA and Europe, whereas red-eared sliders (a common turtle species) have declined in popularity (Valdez, 2021). This is relevant for the association between bearded dragons and RAS, since this was the species most often identified in recent reports on RAS. The relevance of possible changes in the trade of turtles cannot be estimated at this point.
Secondly, the level of direct contact between reptiles and owners varies depending on the reptile species as well as on the owner. It is possible that the probability of close contact between owners and pet reptiles and especially between children and pet reptiles may vary depending on the reptile species, with lower average levels of contact with snakes than turtles and lizards.
Thirdly, for Salmonella transmission, the direct environment may play an important role, and most turtle species are kept in water, which may well serve as a vector (wet hands, wet material). Water—especially if hygiene measures are poor—can be contaminated with reptile-derived Salmonella (Mermin et al., 2004; Corrente et al., 2017; Waltenburg et al., 2022).
The differences noted in detection rates between wild reptiles and reptiles in captivity could—as mentioned above—also be due to differences in conditions, with dietary, stress and crowding-related factors likely causing the higher detection rates found in reptiles in captivity. Given the high dependency of ectothermic organisms on their environment, and natural living situations probably reflecting more physiological conditions than those in captivity, this could contribute to a stronger immune system and reduced stress (DuPonte et al., 1978; Geue and Löschner, 2002; Dickinson et al., 2005; Scheelings et al., 2011; Cota et al., 2021).
In general, the most important source of salmonellosis in humans is contaminated food (Bradley et al., 2001; Faulder et al., 2017; Meletiadis et al., 2022). Contaminated food can play a role in RAS, but direct consumption of Salmonella-contaminated reptile meat leading to human infection has also been described. Marine turtles (O’Grady and Krause, 1999), lizards (Ríos et al., 2022), terrapins, and crocodilians (Madsen, 1996; Magnino et al., 2009; López-Quintana et al., 2015; Draper et al., 2017) are all used as a source of food and their meat can be a source of salmonellosis, as can food contaminated with excretions of reptiles. The latter was reported for example, after a Thanksgiving dinner prepared by a bearded dragon owner (Lowther et al., 2011).
Age may also influence the risk for RAS. A study investigating the development of RAS in children concluded that the age of the children and the reptile group are significant risk factors, with children at a median age of 0.17 years affected more than the group with a median age of 2.0 years, and turtles being significantly more involved than other reptile groups (Sauteur et al., 2013). Another recent study, however, reports a shift of RAS cases to adulthood, while cases in children decreased (Mughini-Gras et al., 2016). Those and other studies (Pees et al., 2013) also conclude that hygienic insufficiencies are relevant risk factors when keeping reptiles.
Our literature survey confirmed the importance of subspecies I strains (almost 90%) for zoonotic risk. The prevalence study also confirmed subspecies I as the predominant Salmonella subspecies found in the reptile gastrointestinal tract, but to a lesser degree (about 70%). The zoonotic potential of reptile-derived Salmonella strains was examined on a cellular basis using Caco-2 cells to test for invasiveness. Pasmans et al. (Pasmans et al., 2005) found that saurian isolates belonging to subspecies I invaded human colon cells, Caco-2-cells, to a higher extent than isolates belonging to other subspecies, and to a higher extent than corresponding human serotypes. This was also confirmed by a more recent study on pet reptiles in Beijing, in which more than 50% of the Salmonella isolated were found to be more cytotoxic to Caco-2-cells than a defined standard strain (Salmonella Typhimurium 1,344) (Song et al., 2023). Additionally, those authors found that highly virulent strains most often belong to subspecies I and IIIb, and lizards seemed to have the highest prevalence with higher cytotoxicity followed by turtles and snakes. Among the tested isolates, strain 1101PV5, belonging to the serovar Pomona, showed the highest pathogenicity on the Caco-2-cells. McWorhter et al. (2021) tested the invasiveness of different Salmonella strains isolated from both captive and wild reptiles in human Caco-2 and mouse macrophage (J774A.1) cell lines, yielding diverse results. All isolates demonstrated invasiveness in both cell lines, with subspecies I and IV strains exhibiting the highest degree of invasiveness, while subspecies IIIa and IIIb strains displayed the lowest. The assessment of invasiveness in macrophages was undertaken due to its importance in within-host survival and replication. All isolates exhibited lower invasiveness in J774.1A macrophages compared to Caco-2 cells. The presence of virulence genes in isolates from reptiles was also examined by PCR (Pasmans et al., 2005), and no significant differences were found in the distribution of the virulence genes agfA, shdA, sopE, pefA, and spvR between subspecies I serovars from reptiles and the corresponding serovars from humans. Also, the presence of pathogenicity islands with virulence genes such as invA and spiC were found in strains isolated from reptiles (Bruce et al., 2018). However, it must be noted that having one or more virulence genes does not confer pathogenicity on a strain unless the strain acquires the appropriate virulence gene combination to cause disease in a particular host species (Whiley et al., 2017; Xia et al., 2020; Meletiadis et al., 2022).
In the authors’ opinion, the risk of RAS should be effectively communicated, and risk reduction can be most efficiently achieved with public education and the improvement of animal health. Despite the existence of numerous case reports, reviews, and studies on RAS, the risk of developing RAS does not seem to be fully understood within the population of reptile owners as poorly informed owners and inadequate hygiene standards are still observed (Corrente et al., 2017). A recent evaluation of online platforms selling turtles in the USA revealed that turtles, including small turtles <4 inches in diameter, which are banned from sale in many US states, appear to be readily available for purchase. These websites also did not provide adequate information about Salmonella or federal regulations (Montague et al., 2022). Therefore, further education efforts are necessary to address this gap in knowledge. The responsibility for establishing and implementing prevention strategies for RAS falls not only on the reptile owners but also on veterinarians and regulatory bodies to ensure that adequate information and guidelines are accessible and widely disseminated.
4.3 Prevention and treatment
Considering the well-documented risk of RAS, preventive measures should be implemented to mitigate the zoonotic risk of Salmonella in reptiles. Various options have been reported over the decades, and their implementation should prioritize both animal and owner welfare. While restrictions on the trade and keeping of reptile may have a measurable effect on RAS prevalence, such measures also carry the risk of stimulating black-market trading, compromising control, and, even worse, impacting overall animal health by limiting access to veterinary care and equipment for husbandry as well as reducing exchange of information on high quality husbandry (Harris et al., 2010; Montague et al., 2022). Moreover, targeted measures focusing on individual species or reptile groups do not appear to be promising, given the high shedding rates reported across captive reptile groups and species. Implementing such regulations also raises legal concerns in many countries. As pointed out by Pasmans et al. (Pasmans et al., 2017), the risks posed by reptiles as pets should be seen in context, with higher risks for infection or injury in general posed by more traditional pets, and higher risks for human salmonellosis specifically posed e.g., through food.
Numerous approaches have been investigated and implemented to mitigate the occurrence of RAS in the human population. These strategies encompass a wide range of interventions, including the prohibition of keeping certain reptile groups in captivity, public education campaigns, and various methods for assessing and reducing Salmonella contamination in reptiles.
4.3.1 Education
Educational initiatives have been identified as crucial in the prevention of RAS (De Jong et al., 2005; Whiley et al., 2017; Meletiadis et al., 2022). Recent studies have shed light on behaviours and knowledge gaps among reptile owners. For instance, research by Meletiadis et al. (2022) revealed that some owners allow their reptiles access to the kitchen and/or bathroom, and handwashing hygiene after handling these animals was found to be suboptimal, with only 69% of owners consistently washing their hands after contact. Notably, in one study only a single family out of 13 with children affected by RAS was aware of the risk before the illness occurred (Colomb-Cotinat et al., 2014). Another study revealed similarly low numbers with 14% of affected RAS patients aware that reptiles may carry Salmonella spp. (Bosch et al., 2016). Furthermore, while some owners claimed to be aware of potential risks, they were unable to name any specific hazard (Corrente et al., 2017). However, higher levels of risk awareness have also been reported, with approximately 50%–60% of patients with RAS confirming awareness of the potential risk of RAS in one study (Whitten et al., 2015). Awareness of RAS may also depend on the species owned, as in one study owners of lizards and snakes were reported to have a higher awareness of the risk of RAS compared to turtle owners (Waltenburg et al., 2022). Studies have documented the effectiveness of educational programs targeting reptile owners in reducing numbers of RAS cases (De Jong et al., 2005).
Various approaches can be employed to reach different audiences and contribute to the prevention of RAS. Educational materials should encompass a range of essential information, including hygiene measures such as appropriate hand washing routines. It is crucial to prevent reptiles from freely roaming in rooms inhabited by people and to prohibit their presence in areas such as kitchens, bathrooms, or food preparation areas (Bradley et al., 2001; Warwick et al., 2001).
4.3.2 Reptile bans
In the United States, the implementation of a ban on small turtles with shell diameters smaller than 4 inches in 1975 is estimated to have averted approximately 100.000 cases of RAS annually (Vora et al., 2012). However, the impacts of subsequent regulations within the USA, for example, in relation to childcare centers, have been found to be highly dependent on the respective state (Vora et al., 2012). In Sweden, import restrictions on reptiles were enforced from 1970 until 1994. These regulations prohibited the trade of turtles with shell diameters less than 10 cm and required reptiles to be certified as Salmonella-free prior to importation. The effectiveness of these measures in preventing RAS was generally acknowledged (De Jong et al., 2005). The removal of these importation restrictions in 1994 resulted in an initial rise in RAS cases in the country by up to 4.5%–11.6% in the following years. Subsequently, an education program was launched in 1997, which was associated with a significant reduction in RAS cases (De Jong et al., 2005).
4.3.3 Antibiotic treatments
Since reptiles have been shown to be able to carry multi-drug resistant Salmonella spp. (D’Aoust et al., 1990; Marin et al., 2020; Marin et al., 2022), treatment can generally be challenging. Treatment of healthy animals is not recommended (Bradley et al., 2001; Pasmans et al., 2008; Romero, 2016) and is unlikely to lead to permanently Salmonella-free animals (Mitchell 2011; Pasmans et al., 2008; Romero 2016). Mitchell (2011) recommended removal instead of treatment for Salmonella-positive tested animals used for educational programs. Depending on the clinical case, the use of different antibiotic agents has been described in reptiles for the treatment of salmonellosis. Antibiotics frequently reported in the treatment of salmonellosis in reptiles include ceftazidime (Schroff et al., 2010; Barboza et al., 2018), enrofloxacin (Ramsay et al., 2002; Barboza et al., 2018), and amikacin (Clancy et al., 2016a), as well as ampicillin or piperacillin (Ramsay et al., 2002; Barboza et al., 2018). Antibiotic agents should generally be chosen according to microbial susceptibility results (Clancy et al., 2016a).
A study by Marin et al., 2022 reported antibiotic resistance in all the Salmonella strains of reptile origin tested, 72% of which were multidrug-resistant strains. The most frequently observed resistance pattern was the combination of gentamicin-colistin and gentamicin-colistin-ampicillin. The high resistance against gentamicin is suspected to be a result of the indiscriminate use of aminoglycosides by pet reptile breeders. Gentamicin is commonly used in the USA as a prophylactic Salmonella treatment in eggs to reduce bacterial loads (Siebeling et al., 1984; Mitchell et al., 2007; Marin et al., 2022). Some studies have focused exclusively on the production of Salmonella-free reptiles, and various treatment methods have been evaluated, including antimicrobials such as oxytetracycline, gentamicin or chloramphenicol in water or directly on the eggs (Mitchell et al., 2007). However, although some of these methods resulted in the reduction of infection and/or shedding rates in hatchling turtles, their excessive use also increased antibiotic resistance (Mitchell et al., 2007). Other substances, such as sodium hypochlorite and polyhexamethylene biguanides (Mitchell et al., 2007) or chlorhexidine gluconate (Cota et al., 2021) also lead to some degree of reduction in Salmonella carriage of hatchlings. However, in general, efforts to eliminate Salmonella spp. in reptiles have been unsuccessful (Pasmans et al., 2021).
Not only has the efficacy of antibiotic eradication shown limited success, but the utilization of certain antimicrobial agents, such as 3rd and 4th generation cephalosporins, fluoroquinolones, and other antimicrobials classified by the World Health Organization (WHO) as “Highest Priority Critically Important Antimicrobials (HPCIA),” in veterinary medicine has been subject to critical discussion. This concern is further compounded by the escalating issue of antimicrobial resistance. Consequently, antibiotic treatment for clinically ill animals should rely on guidelines, such as the consensus statement (Weese et al., 2015) from the American College of Veterinary Internal Medicine (ACVIM). Recommendations on the use of antimicrobials in exotic animals, including reptiles, have also been published (Broens and Van Geijlswijk, 2018; Perry and Mitchell, 2019; Hedley et al., 2021; Divers and Burgess, 2023).
4.3.4 Use of phages or probiotics
Bacteriophages have demonstrated effectiveness in moderating bacterial populations in numerous veterinary settings (Filho et al., 2007; Hong, 2013; Cortés et al., 2015; Fadlallah et al., 2015; Wernicki et al., 2017; Gigante and Atterbury, 2019). Several studies have investigated the potential of bacteriophages to reduce Salmonella shedding in different animal species, demonstrating some efficacy (Berchieri et al., 1991; Filho et al., 2007; Lim et al., 2012; Adhikari et al., 2017). However, very few studies are currently available describing the use of bacteriophages for the reduction of Salmonella in the gut of reptiles. One study demonstrated the ability of a specific phage to replicate in Salmonella spp. in the intestines of bearded dragons and thereby to decrease the number of Salmonella shed in the feces (Renfert et al., 2021). Another study successfully used a phage to reduce the numbers of Salmonella spp. associated with reptile skin (Kwon et al., 2021).
Probiotics have also been suggested to influence reptile health and gut microbiota. Positive effects have been reported, e.g., in turtles, probiotics were associated with an increase in body weight and enhanced shell mineralization (Rawski et al., 2016). However, existing studies on the use of pro- or prebiotics to decrease the shedding of Salmonella spp. have not been successful (Holz and Middleton, 2002; 2005).
4.3.5 Optimization of reptile health
Another important measure that should be emphasized to reduce the risk of RAS is the improvement of overall reptile health. Striving to recreate natural conditions is not only a fundamental goal for herpetologists but also a key factor in promoting healthy reptiles and supporting a functional immune system (Pasmans et al., 2008). Welfare assessment methods have been described, with a focus on behavioural criteria being particularly relevant (Warwick et al., 2013; Bashaw et al., 2016; Moszuti et al., 2017; Benn et al., 2019; Whittaker et al., 2021). Although the connection between behavioural enrichment, the immune system, and Salmonella has not yet been studied, multiple studies have indicated a relationship between stress, disease, and shedding of Salmonella in reptiles (Sting et al., 2013; Clancy et al., 2016b; Bertolini et al., 2021).
4.4 Conclusion: a need for further research and education
The data summarized in this review support the assessment of Salmonella in reptiles as a common finding and a zoonotic risk, but also illustrate the limited knowledge of physiologic mechanisms and pathogenic processes. Although the reviews were able to summarize and interpret important data, there are still many aspects of Salmonella in reptiles that require further study. Exact mechanisms of shedding, interaction, and invasiveness of Salmonella are still not well understood. Little is also known about the specific virulence of reptile-associated serovars in humans. Studies investigating the cytotoxicity of Salmonella serovars isolated from reptiles have provided initial insights into their pathogenic potential. However, further research is necessary before definitive conclusions can be drawn and comprehensive risk assessments can be conducted.
Therefore, there is a need to initiate further research on
• The role of Salmonella in the normal gut microbiome of reptiles
• Factors influencing invasion and pathogenicity of Salmonella in reptiles
• Factors influencing and methods for reducing shedding of Salmonella in reptiles
• The role of specific strains and their genomic makeup in epidemiology, shedding, and pathogenicity of Salmonella for reptiles and humans
• Understanding the role of humans in increasing Salmonella prevalence in reptiles
To control the zoonotic risk of Salmonella in reptiles, it is essential to
• Improve the general health status of reptiles by providing a physiologic, healthy environment
• Improve hygienic standards for reptiles kept in family homes
• Stimulate education programs focusing on the understanding of needs and risks when keeping exotic animals
Author contributions
MP and RM conceived and planned the review. RM and MB conducted the survey on Salmonella prevalence. MP and NS conducted the survey on reptile-associated salmonellosis. MP conducted statistical analysis of the survey data. All authors contributed to the article and approved the submitted version.
Funding
This Open Access publication was funded by the Deutsche Forschungsgemeinschaft (DFG, German Research Foundation)—491094227 “Open Access Publication Funding” and the University of Veterinary Medicine Hannover, Foundation.
Conflict of interest
Authors MB and RM were employed by Laboklin GmbH and Co., KG. Authors MB and RM are employed by a commercial laboratory offering diagnostic testing for veterinarians. This employment did not influence data collection, interpretation, or publication preparation.
The remaining authors declare that the research was conducted in the absence of any commercial or financial relationships that could be construed as a potential conflict of interest.
Publisher’s note
All claims expressed in this article are solely those of the authors and do not necessarily represent those of their affiliated organizations, or those of the publisher, the editors and the reviewers. Any product that may be evaluated in this article, or claim that may be made by its manufacturer, is not guaranteed or endorsed by the publisher.
Supplementary material
The Supplementary Material for this article can be found online at: https://www.frontiersin.org/articles/10.3389/fcell.2023.1251036/full#supplementary-material
SUPPLEMENTARY DATA SHEET S1 | Prevalence of Salmonella in healthy reptiles survey (2002–2023). Prisma Chart flow.
SUPPLEMENTARY DATA SHEET S2 | Prevalence of Salmonella in healthy reptiles survey (2002–2023). Data evaluation details, references see list in Supplementary Data Sheet S3.
SUPPLEMENTARY DATA SHEET S3 | Prevalence of Salmonella in healthy reptiles survey (2002–2023). References.
SUPPLEMENTARY DATA SHEET S4 | Reptile associated Salmonellosis Survey (2002–2023). Prisma Chart flow.
SUPPLEMENTARY DATA SHEET S5 | Reptile associated Salmonllosis Survey (2002–2023). Data evaluation details, references see list in Supplementary Data Sheet S6.
SUPPLEMENTARY DATA SHEET S6 | Reptile associated Salmonellosis Survey (2002–2023). References.
References
Ackman, D. M., Drabkin, P., Birkhead, G., and Cieslak, P. (1995). Reptile-associated salmonellosis in New York state. Pediatr. Infect. Dis. J. 14 (11), 955–959. doi:10.1097/00006454-199511000-00006
Adhikari, P. A., Cosby, D. E., Cox, N. A., Lee, J. H., and Kim, W. K. (2017). Effect of dietary bacteriophage supplementation on internal organs, fecal excretion, and ileal immune response in laying hens challenged by Salmonella Enteritidis. Poult. Sci. 96 (9), 3264–3271. doi:10.3382/ps/pex109
Ahasan, Md. S., Waltzek, T. B., Huerlimann, R., and Ariel, E. (2018). Comparative analysis of gut bacterial communities of green turtles (Chelonia mydas) pre-hospitalization and post-rehabilitation by high-throughput sequencing of bacterial 16S rRNA gene. Microbiol. Res. 207, 91–99. doi:10.1016/j.micres.2017.11.010
Altman, R., Gorman, J. C., Bernhardt, L. L., and Goldfield, M. (1972). Turtle-associated salmonellosis. II. The relationship of pet turtles to salmonellosis in children in New Jersey. Am. J. Epidemiol. 95 (6), 518–520. doi:10.1093/oxfordjournals.aje.a121419
Baling, M., and Mitchell, C. (2021). Prevalence of Salmonella spp. in translocated wild reptiles and effect of duration of quarantine on their body condition. N. Z. Veterinary J. 69 (3), 174–179. doi:10.1080/00480169.2021.1890647
Barboza, T., Beaufrère, H., and Chalmers, H. (2018). Epipterygoid bone Salmonella abscess in a savannah monitor (Varanus exanthematicus). J. Herpetological Med. Surg. 28 (1), 29. doi:10.5818/17-04-106.1
Barry, K., and Finn, A. (2022). Salmonella Typhimurium as a causative agent of spontaneous bacterial peritonitis. BMJ Case Rep. 15 (4), e249550. doi:10.1136/bcr-2022-249550
Bashaw, M. J., Gibson, M. D., Schowe, D. M., and Kucher, A. S. (2016). Does enrichment improve reptile welfare? Leopard geckos (Eublepharis macularius) respond to five types of environmental enrichment. Appl. Animal Behav. Sci. 184, 150–160. doi:10.1016/j.applanim.2016.08.003
Basler, C., Bottichio, L., Higa, J., Prado, B., Wong, M., and Bosch, S. (2015). Multistate outbreak of human Salmonella Poona infections associated with pet turtle exposure — United States, 2014. MMWR. Morb. Mortal. Wkly. Rep. 64 (29), 804. doi:10.15585/mmwr.mm6429a7
Bemis, D. A., Grupka, L. M., Liamthong, S., Folland, D. W., Sykes, J. M., and Ramsay, E. C. (2007). Clonal relatedness of Salmonella isolates associated with invasive infections in captive and wild-caught rattlesnakes. Veterinary Microbiol. 120 (3–4), 300–307. doi:10.1016/j.vetmic.2006.10.028
Benn, A., McLelland, D., and Whittaker, A. (2019). A Review of welfare assessment methods in reptiles, and preliminary application of the welfare quality® protocol to the pygmy blue-tongue skink, Tiliqua adelaidensis, using animal-based measures. Animals 9 (1), 27. doi:10.3390/ani9010027
Berchieri, Aj., Lovell, M. A., and Barrow, P. A. (1991). The activity in the chicken alimentary tract of bacteriophages lytic for Salmonella Typhimurium. Res. Microbiol. 142 (5), 541–549. doi:10.1016/0923-2508(91)90187-f
Berendes, T. D., Keijman, J. M. G., te Velde, L. F., and Oostenbroek, R. J. (2007). Splenic abscesses caused by a reptile-associated Salmonella infection. Dig. Surg. 24 (5), 397–399. doi:10.1159/000107718
Bertelloni, F., Chemaly, M., Cerri, D., Le Gall, F., and Ebani, V. V. (2016). Salmonella infection in healthy pet reptiles: bacteriological isolation and study of some pathogenic characters. Acta Microbiol. Immunol. Hung. 63 (2), 203–216. doi:10.1556/030.63.2016.2.5
Bertolini, M., Schwertz, C. I., Vielmo, A., Piva, M. M., Bilhalva, L. C., Pavarini, S. P., et al. (2021). Pathological and microbiological findings in fatal cases of salmonellosis in captive bothrops snakes in Southern Brazil. J. Comp. Pathology 186, 7–12. doi:10.1016/j.jcpa.2021.04.011
Bertrand, S., Rimhanen-Finne, R., Weill, F. X., Rabsch, W., Thornton, L., Perevoscikovs, J., et al. (2008). Salmonella infections associated with reptiles: the current situation in Europe. Eurosurveillance 13 (24), 18902. doi:10.2807/ese.13.24.18902-en
Bestion, E., Jacob, S., Zinger, L., Di Gesu, L., Richard, M., White, J., et al. (2017). Climate warming reduces gut microbiota diversity in a vertebrate ectotherm. Nat. Ecol. Evol. 1 (6), 161–163. doi:10.1038/s41559-017-0161
Bjelland, A. M., Sandvik, L. M., Skarstein, M. M., Svendal, L., and Debenham, J. J. (2020). Prevalence of Salmonella serovars isolated from reptiles in Norwegian zoos. Acta Veterinaria Scand. 62 (1), 3. doi:10.1186/s13028-020-0502-0
Böhme, H., Fruth, A., and Rabsch, W. (2009). Reptile-associated salmonellosis in infants in Germany. Klin. Pädiatrie 221 (02), 60–64. doi:10.1055/s-0028-1112156
Bölcskei, A. M., Gál, J., Jacsó, O., Jánosi, K., and Adrián, E. (2009). Foleyella furcata (Linstow, 1889) infection and chronic inflammation in the ovaries caused by Salmonella Uzaramo in imported Senegal chameleon (Chamaeleo senegalensis). Case report. Magy. Állatorvosok Lapja 131 (2), 120–124.
Bosch, S., Tauxe, R. V., and Behravesh, C. B. (2016). Turtle-associated salmonellosis, United States, 2006-2014. Emerg. Infect. Dis. 22 (7), 1149–1155. doi:10.3201/eid2207.150685
Bradley, T., Angulo, F., and Mitchell, M. (2001). Public health education on Salmonella spp. and reptiles. J. Am. Veterinary Med. Assoc. 219 (6), 754–755. doi:10.2460/javma.2001.219.754
Brenneman, E., Keil, E., Advani, S. D., Campbell, K. B., and Wrenn, R. (2022). A turtle disaster: salmonella enteritidis cardiovascular implantable electronic device infection. Open Forum Infect. Dis. 9 (12), ofac668. doi:10.1093/ofid/ofac668
Broens, E. M., and Van Geijlswijk, I. M. (2018). Prudent use of antimicrobials in exotic animal medicine. Veterinary Clin. N. Am. Exot. Animal Pract. 21 (2), 341–353. doi:10.1016/j.cvex.2018.01.014
Bruce, H. L., Barrow, P. A., and Rycroft, A. N. (2018). Zoonotic potential of Salmonella enterica carried by pet tortoises. Veterinary Rec. 182 (5), 141. doi:10.1136/vr.104457
Cartwright, E. J., Nguyen, T., Melluso, C., Ayers, T., Lane, C., Hodges, A., et al. (2016). A multistate investigation of antibiotic-resistant Salmonella enterica serotype I 4,[5],12:i:- Infections as part of an international outbreak associated with frozen feeder rodents. Zoonoses Public Health 63 (1), 62–71. doi:10.1111/zph.12205
Castlemain, B. M., and Castlemain, B. D. (2022). Case report: post-salmonellosis abscess positive for Salmonella Oranienburg. BMC Infect. Dis. 22 (1), 337. doi:10.1186/s12879-022-07217-5
Cellucci, T., Seabrook, J., Chagla, Y., Bannister, S., and Salvadori, M. (2010). A 10-year retrospective review of Salmonella infections at the children’s hospital in London, Ontario. Can. J. Infect. Dis. Med. Microbiol. 21 (2), 78–82. doi:10.1155/2010/968960
Clancy, M. M., Davis, M., Valitutto, M. T., Nelson, K., and Sykes, J. M. (2016a). Salmonella infection and carriage in reptiles in a zoological collection. J. Am. Veterinary Med. Assoc. 248 (9), 1050–1059. doi:10.2460/javma.248.9.1050
Clancy, M. M., Newton, A. L., and Sykes, J. M. (2016b). Management of osteomyelitis caused by Salmonella enterica subsp. houtenae in a Taylor's cantil (Agkristrodon bilineatus taylori) using amikacin delivered via osmotic pump. J. Zoo Wildl. Med. 47 (2), 691–694. doi:10.1638/2015-0207.1
Colomb-Cotinat, M., Le Hello, S., Rosières, X., Lailler, R., Weill, F. X., Jourdan-Da Silva, N., et al. (2014). Salmonelloses chez des jeunes enfants et exposition aux reptiles domestiques: investigation en France métropolitaine en 2012. Bull. Épidémiologique Hebd 2014, 1–2.
Cooke, F., De, P., Maguire, C., Guha, S., Pickard, D., Farrington, M., et al. (2009). First report of human infection with Salmonella enterica serovar Apapa resulting from exposure to a pet lizard. J. Clin. Microbiol. 47 (8), 2672–2674. doi:10.1128/JCM.02475-08
Corrente, M., Sangiorgio, G., Grandolfo, E., Bodnar, L., Catella, C., Trotta, A., et al. (2017). Risk for zoonotic Salmonella transmission from pet reptiles: A survey on knowledge, attitudes and practices of reptile-owners related to reptile husbandry. Prev. Veterinary Med. 146, 73–78. doi:10.1016/j.prevetmed.2017.07.014
Cortés, P., Spricigo, D. A., Bardina, C., and Llagostera, M. (2015). Remarkable diversity of Salmonella bacteriophages in swine and poultry. FEMS Microbiol. Lett. 362 (2), 1–7. doi:10.1093/femsle/fnu034
Cota, J. B., Carvalho, A. C., Dias, I., Reisinho, A., Bernardo, F., and Oliveira, M. (2021). Salmonella spp. in pet reptiles in Portugal: prevalence and chlorhexidine gluconate antimicrobial efficacy. Antibiotics 10 (3), 324. doi:10.3390/antibiotics10030324
D’Aoust, J. Y., Daley, E., Crozier, M., and Sewell, A. M. (1990). Pet turtles: A continuing international threat to public health. Am. J. Epidemiol. 132 (2), 233–238. doi:10.1093/oxfordjournals.aje.a115652
De Jong, B., Andersson, Y., and Ekdahl, K. (2005). Effect of regulation and education on reptile-associated salmonellosis. Emerg. Infect. Dis. 11 (3), 398–403. doi:10.3201/eid1103.040694
Di Girolamo, N., Selleri, P., Nardini, G., Corlazzoli, D., Fonti, P., Rossier, C., et al. (2014). Computed tomography guided bone biopsies for evaluation of proliferative vertebral lesions in two boa constrictors (Boa constrictor imperator). J. Zoo Wildl. Med. 45 (4), 973–978. doi:10.1638/2014-0049.1
Dickinson, V. M., Schumacher, I. M., Jarchow, J. L., Duck, T., and Schwalbe, C. R. (2005). Mycoplasmosis in free-ranging desert tortoises in Utah and Arizona. J. Wildl. Dis. 41 (4), 839–842. doi:10.7589/0090-3558-41.4.839
Divers, S. J., and Burgess, B. A. (2023). ARAV antimicrobial stewardship policy. J. Herpetological Med. Surg. 33 (1). doi:10.5818/JHMS.33.1.61
Draper, A. D. K., James, C. L., Pascall, J. E., Shield, K. J., Langrell, J., and Hogg, A. (2017). An outbreak of Salmonella Muenchen after consuming sea turtle. North. Territ. 2017, 41.
DuPonte, Mw, Rm, N., and Em, C. (1978). Activation of latent Salmonella and Arizona organisms by dehydration of red-eared turtles, Pseudemys scripta-elegans. Am. J. Veterinary Res. 39 (3), 529–530.
Ebani, V. V., Cerri, D., Fratini, F., Meille, N., Valentini, P., and Andreani, E. (2005). Salmonella enterica isolates from faeces of domestic reptiles and a study of their antimicrobial in vitro sensitivity. Res. Veterinary Sci. 78 (2), 117–121. doi:10.1016/j.rvsc.2004.08.002
Fadlallah, A., Chelala, E., and Legeais, J.-M. (2015). Corneal infection therapy with topical bacteriophage administration. Open Ophthalmol. J. 9, 167–168. doi:10.2174/1874364101509010167
Fagre, A., Kl, P., Ms, J., Ps, M., and Ba, B. (2020). Comparison of detection methods for Salmonella enterica shedding among reptilian patients at a veterinary teaching hospital. J. Veterinary Diagnostic Investigation 32 (1), 118–123. doi:10.1177/1040638719886542
Faulder, K. E., Simmonds, K., and Robinson, J. L. (2017). The epidemiology of childhood Salmonella infections in Alberta, Canada. Foodborne Pathogens Dis. 14 (6), 364–369. doi:10.1089/fpd.2016.2259
Filho, R. L., Higgins, J. P., Higgins, S. E., Gaona, G., Wolfenden, A. D., Tellez, G., et al. (2007). Ability of bacteriophages isolated from different sources to reduce Salmonella enterica serovar Enteritidis in vitro and in vivo. Poult. Sci. 86 (9), 1904–1909. doi:10.1093/ps/86.9.1904
Friedman, C. R., Torigian, C., Shillam, P. J., Hoffman, R. E., Heltze, D., Beebe, J. L., et al. (1998). An outbreak of salmonellosis among children attending a reptile exhibit at a zoo. J. Pediatr. 132 (5), 802–807. doi:10.1016/S0022-3476(98)70307-5
Gerstel, U., and Romling, U. (2001). Oxygen tension and nutrient starvation are major signals that regulate agfD promoter activity and expression of the multicellular morphotype in Salmonella Typhimurium. Environ. Microbiol. 3 (10), 638–648. doi:10.1046/j.1462-2920.2001.00235.x
Geue, L., and Löschner, U. (2002). Salmonella enterica in reptiles of German and Austrian origin. Veterinary Microbiol. 84 (1–2), 79–91. doi:10.1016/S0378-1135(01)00437-0
Gigante, A., and Atterbury, R. J. (2019). Veterinary use of bacteriophage therapy in intensively-reared livestock. Virology J. 16 (1), 155. doi:10.1186/s12985-019-1260-3
Gorman, C. (2010). Spinal osteopathy in a boa constrictor. U. K. Vet. Companion Anim. 15 (1), 42–44. doi:10.1111/j.2044-3862.2010.tb00438.x
Goupil, B. A., Trent, A. M., Bender, J., Olsen, K. E., Morningstar, B. R., and Wünschmann, A. (2012). A longitudinal study of Salmonella from snakes used in a public outreach program. J. Zoo Wildl. Med. 43 (4), 836–841. doi:10.1638/2011-0281R1.1
Grupka, L. M., Ramsay, E. C., and Bemis, D. A. (2006). Salmonella surveillance in a collection of rattlesnakes (Crotallus spp.). J. Zoo Wildl. Med. 37 (3), 306–312. doi:10.1638/05-059.1
Haase, R., Beier, T., Bernstädt, M., Merkel, N., and Bartnicki, J. (2011). Neugeborenensepsis durch Salmonella Apapa nach Reptilienkontakt im Haushalt. Z. für Geburtshilfe Neonatologie 215 (02), 86–88. doi:10.1055/s-0031-1275308
Harker, K. S., Lane, C., De Pinna, E., and Adak, G. K. (2011). An outbreak of Salmonella Typhimurium DT191a associated with reptile feeder mice. Epidemiol. Infect. 139 (8), 1254–1261. doi:10.1017/S0950268810002281
Harris, J. R., Neil, K. P., Behravesh, C. B., Sotir, M. J., and Angulo, F. J. (2010). Recent multistate outbreaks of human Salmonella infections acquired from turtles: A continuing public health challenge. Clin. Infect. Dis. 50 (4), 554–559. doi:10.1086/649932
Hatt, J.-M., Fruth, A., and Rabsch, W. (2009). Information update on reptile-associated salmonellosis. Tierärztliche Prax. Ausg. Kleintiere Heimtiere 37 (3), 188–193. doi:10.1055/s-0038-1622784
Hedley, J., Whitehead, M. L., Munns, C., Pellett, S., Abou-Zahr, T., Calvo Carrasco, D., et al. (2021). Antibiotic stewardship for reptiles. J. Small Animal Pract. 62 (10), 829–839. doi:10.1111/jsap.13402
Holgersson, M. C. N., Nichols, W. A., Paitz, R. T., and Bowden, R. M. (2016). How important is the eggshell as a source for initial acquisition of Salmonella in hatchling turtles? J. Exp. Zoology Part A Ecol. Genet. Physiology 325 (2), 142–148. doi:10.1002/jez.2004
Holz, P. H., and Middleton, D. R. (2005). The effect of feeding a prebiotic on Salmonella excretion in carpet pythons, morelia and scrub pythons, Morelia amethystina. J. Herpetological Med. Surg. 15 (1), 4–6. doi:10.5818/1529-9651.15.1.4
Holz, P. H., and Middleton, D. R. (2002). The effect of probiotic feeding on Salmonella excretion in carpet pythons, Morelia spilota. J. Herpetological Med. Surg. 12 (3), 5–7. doi:10.5818/1529-9651.12.3.5
Hong, S. S., Jeong, J., Lee, J., Kim, S., Min, W., and Myung, H. (2013). Therapeutic effects of bacteriophages against Salmonella Gallinarum infection in chickens. J. Microbiol. Biotechnol. 23 (10), 1478–1483. doi:10.4014/jmb.1304.04067
Hydeskov, H. B., Guardabassi, L., Aalbaek, B., Olsen, K. E. P., Nielsen, S. S., and Bertelsen, M. F. (2013). Salmonella prevalence among reptiles in a zoo education setting: salmonella in a zoo education setting. Zoonoses Public Health 60 (4), 291–295. doi:10.1111/j.1863-2378.2012.01521.x
Hyeon, J.-Y., Helal, Z. H., Polkowski, R., Vyhnal, K., Mishra, N., Kim, J., et al. (2021). Genomic features of Salmonella enterica subspecies houtenae serotype 45:g,z51:- isolated from multiple abdominal abscesses of an African fat-tailed gecko, United States, 2020. Antibiotics 10 (11), 1322. doi:10.3390/antibiotics10111322
Isaza, R., Garner, M., and Jacobson, E. (2000). Proliferative osteoarthritis and osteoarthrosis in 15 snakes. J. Zoo Wildl. Med. 31 (1), 20–27. doi:10.1638/1042-7260(2000)031[0020:POAOIS]2.0.CO;2
Jacobson, E. R. (2007). “Viruses and viral diseases of reptiles,” in Infectious diseases and Pathology of reptiles. Editor E. R. Jacobson (Boca Raton: CRC Press), 395–460.
Jiang, H.-Y., Ma, J.-E., Li, J., Zhang, X.-J., Li, L.-M., He, N., et al. (2017). Diets alter the gut microbiome of crocodile lizards. Front. Microbiol. 8, 2073. doi:10.3389/fmicb.2017.02073
Kanagarajah, S., Waldram, A., Dolan, G., Jenkins, C., Ashton, P. M., Carrion Martin, A. I., et al. (2018). Whole genome sequencing reveals an outbreak of Salmonella Enteritidis associated with reptile feeder mice in the United Kingdom, 2012-2015. Food Microbiol. 71, 32–38. doi:10.1016/j.fm.2017.04.005
Keenan, S. W., and Elsey, R. M. (2015). The good, the bad, and the unknown: microbial symbioses of the American alligator. Integr. Comp. Biol. 55 (6), 972–985. doi:10.1093/icb/icv006
Kiebler, C. A., Bottichio, L., Simmons, L., Basler, C., Klos, R., Gurfield, N., et al. (2020). Outbreak of human infections with uncommon Salmonella serotypes linked to pet bearded dragons, 2012–2014. Zoonoses Public Health 67 (4), 425–434. doi:10.1111/zph.12701
Köbölkuti, L. B., Spinu, M., Szakács, A., Tenk, M., Kelemen, A., and Czirják, G. Á. (2013). Salmonella septicaemia in a smooth snake. Veterinary Rec. 172 (17), 457–458. doi:10.1136/vr.f2580
Krishnasamy, V., Stevenson, L., Koski, L., Kellis, M., Schroeder, B., Sundararajan, M., et al. (2018). Notes from the field: investigation of an outbreak of Salmonella paratyphi B variant L(+) tartrate + (Java) associated with ball python exposure — United States, 2017. MMWR. Morb. Mortal. Wkly. Rep. 67 (19), 562–563. doi:10.15585/mmwr.mm6719a7
Kuroki, T., Ito, K., Ishihara, T., Furukawa, I., Kaneko, A., Suzuki, Y., et al. (2015). Turtle-associated Salmonella infections in kanagawa, Japan. Jpn. J. Infect. Dis. 68 (4), 333–337. doi:10.7883/yoken.JJID.2014.490
Kwon, J., Kim, S. G., Kim, H. J., Giri, S. S., Kim, S. W., Lee, S. B., et al. (2021). Bacteriophage as an alternative to prevent reptile-associated Salmonella transmission. Zoonoses Public Health 68 (2), 131–143. doi:10.1111/zph.12804
Lafuente, S., Bellido, J. B., Moraga, F. A., Herrera, S., Yagüe, A., Montalvo, T., et al. (2013). Salmonella Paratyphi B and Salmonella Litchfield outbreaks associated with pet turtle exposure in Spain. Enfermedades Infecc. Microbiol. Clínica 31 (1), 32–35. doi:10.1016/j.eimc.2012.05.013
Lane, T., Wakwaya, Y., and Graney, B. (2019). Tortoise and the air: salmonella pneumonia presenting with adult respiratory distress syndrome in a patient with liver disease. B59. Bacterial infection case reports, Presented at the American Thoracic Society 2019 International Conference, May 17-22, 2019 - Dallas, TX,. May 17-22, 2019, American. American Thoracic Society, A3722. doi:10.1164/ajrccm-conference.2019.199.1_MeetingAbstracts.A3722
Lang, B. Y., Varman, M., Reindel, R., and Hasley, B. P. (2007). Salmonella Gaminara osteomyelitis and septic arthritis in an infant with exposure to bearded dragon. Infect. Dis. Clin. Pract. 15 (5), 348–350. doi:10.1097/IPC.0b013e3180325a6d
Lim, T.-H., Kim, M.-S., Lee, D.-H., Lee, Y.-N., Park, J.-K., Youn, H.-N., et al. (2012). Use of bacteriophage for biological control of Salmonella Enteritidis infection in chicken. Res. Veterinary Sci. 93 (3), 1173–1178. doi:10.1016/j.rvsc.2012.06.004
López-Quintana, B., Rivas-González, P., Toro-Rueda, C., and Enríquez-Crego, A. (2015). Infección por Salmonella enterica subespecie Salamae en un paciente ecuatoguineano consumidor de carne de tortuga. Enfermedades Infecc. Microbiol. Clínica 33 (6), 430–431. doi:10.1016/j.eimc.2014.09.012
Lowther, S. A., Medus, C., Scheftel, J., Leano, F., Jawahir, S., and Smith, K. (2011). Foodborne outbreak of Salmonella subspecies IV infections associated with contamination from bearded dragons: foodborne outbreak of reptile-associated salmonellosis. Zoonoses Public Health 58 (8), 560–566. doi:10.1111/j.1863-2378.2011.01403.x
Lukac, M., Pedersen, K., and Prukner-Radovcic, E. (2015). Prevalence of Salmonella in captive reptiles from Croatia. J. Zoo Wildl. Med. 46 (2), 234–240. doi:10.1638/2014-0098R1.1
Maciel, B. M., Argôlo Filho, R. C., Nogueira, S. S. C., Dias, J. C. T., and Rezende, R. P. (2010). High prevalence of Salmonella in tegu lizards (Tupinambis merianae), and susceptibility of the serotypes to antibiotics: prevalence of Salmonella in tegus. Zoonoses Public Health 57 (7–8), e26–e32. doi:10.1111/j.1863-2378.2009.01283.x
Madsen, M. (1996). Prevalence and serovar distribution of Salmonella in fresh and frozen meat from captive Nile crocodiles (Crocodylus niloticus). Int. J. Food Microbiol. 29 (1), 111–118. doi:10.1016/0168-1605(95)00020-8
Magnino, S., Colin, P., Dei-Cas, E., Madsen, M., McLauchlin, J., Nockler, K., et al. (2009). Biological risks associated with consumption of reptile products. Int. J. Food Microbiol. 134 (3), 163–175. doi:10.1016/j.ijfoodmicro.2009.07.001
Marenzoni, M. L., Stefanetti, V., Del Rossi, E., Zicavo, A., Scuota, S., Origgi, F. C., et al. (2022). Detection of testudinid alphaherpesvirus, Chlamydia spp., Mycoplasma spp., and Salmonella spp. in free-ranging and rescued Italian Testudo hermanni hermanni. Veterinaria Ital. 58 (1), 25–34. doi:10.12834/VetIt.1915.13833.1
Marin, C., Ingresa-Capaccioni, S., González-Bodi, S., Marco-Jiménez, F., and Vega, S. (2013). Free-Living turtles are a reservoir for Salmonella but not for Campylobacter. PLOS ONE 8 (8), e72350. doi:10.1371/journal.pone.0072350
Marin, C., Lorenzo-Rebenaque, L., Laso, O., Villora-Gonzalez, J., and Vega, S. (2020). Pet reptiles: A potential source of transmission of multidrug-resistant Salmonella. Front. Veterinary Sci. 7, 613718. doi:10.3389/fvets.2020.613718
Marin, C., Martelli, F., Rabie, A., and Davies, R. (2018). Commercial frozen mice used by owners to feed reptiles are highly externally contaminated with Salmonella Enteritidis PT8. Vector-Borne Zoonotic Dis. 18 (9), 453–457. doi:10.1089/vbz.2018.2295
Marin, C., Martín-Maldonado, B., Cerdà-Cuéllar, M., Sevilla-Navarro, S., Lorenzo-Rebenaque, L., Montoro-Dasi, L., et al. (2022). Antimicrobial resistant Salmonella in chelonians: assessing its potential risk in zoological institutions in Spain. Veterinary Sci. 9 (6), 264. doi:10.3390/vetsci9060264
Mayer, J., and Donnelly, T. M. (2012). Clinical veterinary advisor: birds and exotic pets. 2nd Edn. St. Louis: Elsevier.
McWhorter, A., Owens, J., Valcanis, M., Olds, L., Myers, C., Smith, I., et al. (2021). In vitro invasiveness and antimicrobial resistance of Salmonella enterica subspecies isolated from wild and captive reptiles. Zoonoses Public Health 68 (5), 402–412. doi:10.1111/zph.12820
Meletiadis, A., Biolatti, C., Mugetti, D., Zaccaria, T., Cipriani, R., Pitti, M., et al. (2022). Surveys on exposure to reptile-associated salmonellosis (RAS) in the piedmont region—Italy. Animals 12 (7), 906. doi:10.3390/ani12070906
Merkevičienė, L., Butrimaitė-Ambrozevičienė, Č., Paškevičius, G., Pikūnienė, A., Virgailis, M., Dailidavičienė, J., et al. (2022). Serological variety and antimicrobial resistance in Salmonella Isolated from Reptiles. Biology 11 (6), 836. doi:10.3390/biology11060836
Mermin, J., Hutwagner, L., Vugia, D., Shallow, S., Daily, P., Bender, J., et al. (2004). Reptiles, amphibians, and human Salmonella infection: A population-based, case-control study. Clin. Infect. Dis. official Publ. Infect. Dis. Soc. Am. 38 (3), S253–S261. doi:10.1086/381594
Mitchell, M. A., and Shane, S. M. (2001). Salmonella in reptiles. Seminars Avian Exot. Pet Med. 10 (1), 25–35. doi:10.1053/saep.2001.19798
Mitchell, M. A., Adamson, T. W., Singleton, C. B., Roundtree, M. K., Bauer, R. W., and Acierno, M. J. (2007). Evaluation of a combination of sodium hypochlorite and polyhexamethylene biguanide as an egg wash for red-eared slider turtles (Trachemys scripta elegans) to suppress or eliminate Salmonella organisms on egg surfaces and in hatchlings. Am. J. Veterinary Res. 68 (2), 158–164. doi:10.2460/ajvr.68.2.158
Mitchell, M. A. (2011). Zoonotic diseases associated with reptiles and amphibians: an update. Veterinary Clin. Exot. Anim. Pract. 14 (3), 439–456. doi:10.1016/j.cvex.2011.05.005
Moffatt, C. R. M., Lafferty, A. R., Khan, S., Krsteski, R., Valcanis, M., Powling, J., et al. (2010). Salmonella Rubislaw gastroenteritis linked to a pet lizard. Med. J. Aust. 193 (1), 54–55. doi:10.5694/j.1326-5377.2010.tb03743.x
Montague, L. E., Marcotrigiano, J. M., Keane, N. E., Marquardt, H. E., Sevin, J. A., and Karraker, N. E. (2022). Online sale of small turtles circumvents public health regulations in the United States. PLOS ONE 17 (12), e0278443. doi:10.1371/journal.pone.0278443
Moszuti, S. A., Wilkinson, A., and Burman, O. H. (2017). Response to novelty as an indicator of reptile welfare. Appl. Animal Behav. Sci. 193, 98–103. doi:10.1016/j.applanim.2017.03.018
Mughini-Gras, L., Heck, M., and van Pelt, W. (2016). Increase in reptile-associated human salmonellosis and shift toward adulthood in the age groups at risk, The Netherlands, 1985 to 2014. Eurosurveillance 21 (34), 30324. doi:10.2807/1560-7917.ES.2016.21.34.30324
Murphy, D., and Oshin, F. (2015). Reptile-associated salmonellosis in children aged under 5 years in south west england. Archives Dis. Child. 100 (4), 364–365. doi:10.1136/archdischild-2014-306134
Nowinski, R. J., and Albert, M. C. (2000). Salmonella osteomyelitis secondary to iguana exposure: A case report. Clin. Orthop. Relat. Res. 372, 250–253. doi:10.1097/00003086-200003000-00027
O’Grady, K. A., and Krause, V. (1999). An outbreak of salmonellosis linked to a marine turtle. Southeast Asian J. Trop. Med. Public Health 30 (2), 324–327.
Okabe, H., Murai, H., Takano, S., and Kanno, S. (2021). Turtle-associated salmonellosis due to Salmonella litchfield in a 57-day-old infant: A case report. J. Rural Med. 16 (4), 286–288. doi:10.2185/jrm.2021-007
Orós, J., Ramirez, A. S., Poveda, J. B., Rodriguez, J. L., and Fernández, A. (1996). Systemic mycosis caused by Penicillium griseofulvum in a Seychelles giant tortoise (Megalochelys gigantea). Veterinary Rec. 139, 295–296. doi:10.1136/vr.139.12.295
Pasmans, H., Blahak, S., Martel, A., and Pantchev, N. (2008). Introducing reptiles into a captive collection: the role of the veterinarian. Veterinary J. Lond. Engl. 175 (1), 53–68. doi:10.1016/j.tvjl.2006.12.009
Pasmans, F., Martel, A., and Jacobson, E. R. (2021). “Bacterial diseases of reptiles,” in Infectious diseases and Pathology of reptiles. Editors E. R. Jacobson, and M. M. Garner (Boca Raton, FL, USA: CRC Press), 2nd Edition, 705–748.
Pasmans, F., Bogaerts, S., Braeckman, J., Cunningham, A. A., Hellebuyck, T., Griffiths, R. A., et al. (2017). Future of keeping pet reptiles and amphibians: towards integrating animal welfare, human health and environmental sustainability. Veterinary Rec. 181, 450. doi:10.1136/vr.104296
Pasmans, F., Boyer, F., and Hasebrouck, F. (2013). “Salmonella infections in exotic pets,” in Salmonella in domestic animals. Editors P. A. Barrow, and U. Methner (Oxfordshire, UK: CABI Publishing), 337–350.
Pasmans, F., De, H., Dewulf, J., and Haesebrouck, F. (2002). Pathogenesis of infections with Salmonella enterica subsp. enterica serovar Muenchen in the turtle Trachemys scripta scripta. Veterinary Microbiol. 87 (4), 315–325. doi:10.1016/s0378-1135(02)00081-0
Pasmans, F., Martel, A., Boyen, F., Vandekerchove, D., Wybo, I., Immerseel, F., et al. (2005). Characterization of Salmonella isolates from captive lizards. Veterinary Microbiol. 110 (3–4), 285–291. doi:10.1016/j.vetmic.2005.07.008
Pasmans, F., Van Immerseel, F., Van den Broeck, W., Bottreau, E., Velge, P., Ducatelle, R., et al. (2003). Interactions of Salmonella enterica subsp. enterica serovar Muenchen with intestinal explants of the turtle Trachemys scripta scripta. J. Comp. Pathology 128 (2–3), 119–126. doi:10.1053/jcpa.2002.0614
Pees, M., Rabsch, W., Plenz, B., Fruth, A., Prager, R., Simon, S., et al. (2013). Evidence for the transmission of Salmonella from reptiles to children in Germany. Eurosurveillance 18 (46), 20634. doi:10.2807/1560-7917.ES2013.18.46.20634
Pees, M., Schmidt, V., Papp, T., Gellért, A., Abbas, M., Starck, J. M., et al. (2019). Three genetically distinct ferlaviruses have varying effects on infected corn snakes (Pantherophis guttatus). PLOS ONE 14 (6), e0217164. doi:10.1371/journal.pone.0217164
Perry, S. M., and Mitchell, M. A. (2019). “Antibiotic therapy,” in Hrsg. Mader's reptile and amphibian medicine and surgery. Editors S. J. Divers, and S. J. Stahl (St Louis, Missouri: Elsevier), 1139–1159.
Plenz, B., Schmidt, V., Grosse-Herrenthey, A., Krüger, M., and Pees, M. (2015). Characterisation of the aerobic bacterial flora of boid snakes: application of MALDI-TOF mass spectrometry. Veterinary Rec. 176 (11), 285. doi:10.1136/vr.102580
Ramsay, E. C., Daniel, G. B., Tryon, B. W., Merryman, J. I., Morris, P. J., and Bemis, D. A. (2002). Osteomyelitis associated with Salmonella enterica SS arizonae in a colony of ridgenose rattlesnakes (Crotalus willardi). J. Zoo Wildl. Med. 33 (4), 301–310. doi:10.1638/1042-7260(2002)033[0301:OAWSES]2.0.CO;2
Rawski, M., Kierończyk, B., Długosz, J., Świątkiewicz, S., and Józefiak, D. (2016). Dietary probiotics affect gastrointestinal microbiota, histological structure and shell mineralization in turtles. PLOS ONE 11 (2), e0147859. doi:10.1371/journal.pone.0147859
Renfert, K., Rabsch, W., Fruth, A., Marschang, R. E., Speck, S., and Pees, M. (2021). Influence of Salmonella specific bacteriophages (O1; s16) on the shedding of naturally occurring Salmonella and an orally applied Salmonella Eastbourne strain in bearded dragons (Pogona vitticeps). Veterinary Med. Sci. 7 (2), 534–547. doi:10.1002/vms3.388
Ríos, R., Flores, B., Mora-Sánchez, B., Torres, D., Sheleby-Elías, J., Jirón, W., et al. (2022). Isolation of Salmonella spp. from black spiny-tailed iguana (Ctenosaura similis) meat commercialised in markets of León city, Nicaragua. Veterinary Med. Sci. 8 (2), 695–699. doi:10.1002/vms3.654
Romero, S. B. (2016). The prevalence and antimicrobial resistance of Salmonella species isolated from captive reptiles at ljubljana zoo. Slovenian Veterinary Res. 53, 43–48.
Sakaguchi, K., Nevarez, J. G., and Del Piero, F. (2017). Salmonella enterica serovar Pomona infection in farmed juvenile American alligators (Alligator mississippiensis). Veterinary Pathol. 54 (2), 316–319. doi:10.1177/0300985816677149
Sánchez-Jiménez, M. M., Rincón-Ruiz, P. A., Duque, S., Giraldo, M. A., Ramírez-Monroy, D. M., Jaramillo, G., et al. (2011). Salmonella enterica in semi-aquatic turtles in Colombia. J. Infect. Dev. Ctries. 5 (05), 361–364. doi:10.3855/jidc.1126
Sauteur, P. M. M., Relly, C., Hug, M., Wittenbrink, M. M., and Berger, C. (2013). Risk factors for invasive reptile-associated salmonellosis in children. Vector-Borne Zoonotic Dis. 13 (6), 419–421. doi:10.1089/vbz.2012.1133
Scheelings, T. F., Lightfoot, D., and Holz, P. (2011). Prevalence of Salmonella in Australian reptiles. J. Wildl. Dis. 47 (1), 1–11. doi:10.7589/0090-3558-47.1.1
Schroff, S., Schmidt, V., Kiefer, I., Krautwald-Junghanns, M.-E., and Pees, M. (2010). Ultrasonographic diagnosis of an endocarditis valvularis in a Burmese python (Python molurus bivittatus) with pneumonia. J. Zoo Wildl. Med. 41 (4), 721–724. doi:10.1638/2009-0240.1
Schroter, M., Speicher, A., Hofmann, J., and Roggentin, P. (2006). Analysis of the transmission of Salmonella spp. through generations of pet snakes. Environ. Microbiol. 8 (3), 556–559. doi:10.1111/j.1462-2920.2005.00934.x
Shang, Y., Zhong, H., Liu, G., Wang, X., Wu, X., Wei, Q., et al. (2023). Characteristics of microbiota in different segments of the digestive tract of Lycodon rufozonatus. Animals 13 (4), 731. doi:10.3390/ani13040731
Siebeling, R. J., Caruso, D., and Neuman, S. (1984). Eradication of Salmonella and Arizona species from turtle hatchlings produced from eggs treated on commercial turtle farms. Appl. Environ. Microbiol. 47 (4), 658–662. doi:10.1128/aem.47.4.658-662.1984
Silva-Hidalgo, G., López-Valenzuela, M., Juárez-Barranco, F., Montiel-Vázquez, E., and Valenzuela-Sánchez, B. (2014). Salmonella serovars and antimicrobial resistance in strains isolated from wild animals in captivity in Sinaloa, Mexico. Graduate Sch. Veterinary Med. Hokkaido Univ. 62, 129–134. doi:10.14943/jjvr.62.3.129
Smith, K. F., Yabsley, M. J., Sanchez, S., Casey, C. L., Behrens, M. D., and Hernandez, S. M. (2012). Salmonella Isolates from wild-caught Tokay geckos (Gekko gecko) imported to the U.S. from Indonesia. Vector-Borne Zoonotic Dis. 12 (7), 575–582. doi:10.1089/vbz.2011.0899
Song, D., He, X., Chi, Y., Zhang, Z., Shuai, J., Wang, H., et al. (2023). Cytotoxicity and antimicrobial resistance of Salmonella enterica subspecies Isolated from raised reptiles in Beijing, China. Animals 13 (2), 315. doi:10.3390/ani13020315
Speranza, B., Corbo, M. R., and Sinigaglia, M. (2011). Effects of nutritional and environmental conditions on Salmonella sp. biofilm formation. J. Food Sci. 76 (1), M12–M16. doi:10.1111/j.1750-3841.2010.01936.x
Stam, F., Romkens, T., Hekker, T., and Smulders, Y. (2003). Turtle-associated human salmonellosis. Clin. Infect. Dis. 37 (11), e167–e169. doi:10.1086/379612
Starck, J. M., Neul, A., Schmidt, V., Kolb, T., Franz-Guess, S., Balcecean, D., et al. (2017). Morphology and morphometry of the lung in corn snakes (Pantherophis guttatus) infected with three different strains of ferlavirus. J. Comp. pathology 156 (4), 419–435. doi:10.1016/j.jcpa.2017.02.001
Sting, R., Ackermann, D., Blazey, B., Rabsch, W., and Szabo, I. (2013). Salmonella infections in reptiles - prevalence, serovar spectrum and impact on animal health. Berl. Münchener Tierärztliche Wochenschr. 126, 202–208. doi:10.2376/0005-9366-126-202
Tabarani, C., Bennett, N., Kiska, D., Riddell, S., Botash, A., and Domachowske, J. (2010). Empyema of preexisting subdural hemorrhage caused by a rare Salmonella species after exposure to bearded dragons in a foster home. J. Pediatr. 156 (2), 322–323. doi:10.1016/j.jpeds.2009.07.050
Téllez, S., Briones, V., González, S., Vela, A. I., Blanco, M. M., Ballesteros, C., et al. (2002). Salmonella septicaemia in a beauty snake (Elaphe taeniura taeniura). Veterinary Rec. 151 (1), 28–29. doi:10.1136/vr.151.1.28
Valdez, J. W. (2021). Using google trends to determine current, past, and future trends in the reptile pet trade. Animals 11 (3), 676. doi:10.3390/ani11030676
Van Meervenne, E., Botteldoorn, N., Lokietek, S., Vatlet, M., Cupa, A., Naranjo, M., et al. (2009). turtle-associated Salmonella septicaemia and meningitis in a 2-month-old baby. J. Med. Microbiol. 58 (10), 1379–1381. doi:10.1099/jmm.0.012146-0
Vora, N. M., Smith, K. M., Machalaba, C. C., and Karesh, W. B. (2012). Reptile- and amphibian-associated salmonellosis in childcare centers, United States. Emerg. Infect. Dis. 18 (12), 2092–2094. doi:10.3201/eid1812.120784
Vorbach, B. S., Clayton, L. A., Roosenburg, W. M., Norton, T. M., Adamovicz, L., Hadfield, C. A., et al. (2022). Prevalence of multiple reptilian pathogens in the oropharyngeal mucosa, cloacal mucosa and blood of diamondback terrapin (Malaclemys terrapin). J. Wildl. Dis. 58 (4), 782–790. doi:10.7589/JWD-D-21-00107
Vrbova, L., Sivanantharajah, S., Walton, R., Whitfield, Y., Lee, C., Picard, I., et al. (2018). Outbreak of Salmonella Typhimurium associated with feeder rodents. Zoonoses Public Health 65 (4), 386–394. doi:10.1111/zph.12442
Waltenburg, M. A., Perez, A., Salah, Z., Karp, B. E., Whichard, J., Tolar, B., et al. (2022). Multistate reptile- and amphibian-associated salmonellosis outbreaks in humans, United States, 2009-2018. Zoonoses Public Health 69 (8), 925–937. doi:10.1111/zph.12990
Walters, M. S., Simmons, L., Anderson, T. C., DeMent, J., Van Zile, K., Matthias, L. P., et al. (2016). Outbreaks of salmonellosis from small turtles. Pediatrics 137 (1), e20151735. doi:10.1542/peds.2015-1735
Warwick, C., Arena, P., Lindley, S., Jessop, M., and Steedman, C. (2013). Assessing reptile welfare using behavioural criteria. Practice 35 (3), 123–131. doi:10.1136/inp.f1197
Warwick, C., Lambiris, A. J. L., Westwood, D., and Steedman, C. (2001). Reptile-related salmonellosis. J. R. Soc. Med. 94 (3), 124–126. doi:10.1177/014107680109400306
Weese, J. S., Giguère, S., Guardabassi, L., Morley, P. S., Papich, M., Ricciuto, D. R., et al. (2015). ACVIM consensus statement on therapeutic antimicrobial use in animals and antimicrobial resistance. J. Veterinary Intern. Med. 29 (2), 487–498. doi:10.1111/jvim.12562
Weiss, B., Rabsch, W., Prager, R., Tietze, E., Koch, J., Mutschmann, F., et al. (2011). Babies and bearded dragons: sudden increase in reptile-associated Salmonella enterica serovar Tennessee infections, Germany 2008. Vector-Borne Zoonotic Dis. 11 (9), 1299–1301. doi:10.1089/vbz.2010.0239
Wellehan, J. F. X., and Gunkel, C. I. (2004). Emergent diseases in reptiles. Seminars Avian Exot. Pet Med. 13 (3), 160–174. doi:10.1053/j.saep.2004.03.006
Wells, E. V., Boulton, M., Hall, W., and Bidol, S. A. (2004). Reptile-associated salmonellosis in preschool-aged children in Michigan, January 2001-June 2003. Clin. Infect. Dis. 39 (5), 687–691. doi:10.1086/423002
Wernicki, A., Nowaczek, A., and Urban-Chmiel, R. (2017). Bacteriophage therapy to combat bacterial infections in poultry. Virology J. 14 (1), 179. doi:10.1186/s12985-017-0849-7
Whiley, H., Gardner, M. G., and Ross, K. (2017). A review of Salmonella and squamates (lizards, snakes and amphisbians): implications for public health. Pathogens 6 (3), 38. doi:10.3390/pathogens6030038
Whittaker, A. L., Golder-Dewar, B., Triggs, J. L., Sherwen, S. L., and McLelland, D. J. (2021). Identification of animal-based welfare indicators in captive reptiles: A delphi consultation survey. Animals 11 (7), 2010. doi:10.3390/ani11072010
Whitten, T., Bender, J. B., Smith, K., Leano, F., and Scheftel, J. (2015). Reptile-associated salmonellosis in Minnesota, 1996–2011. Zoonoses Public Health 62 (3), 199–208. doi:10.1111/zph.12140
Williams, L. P., and Helsdon, H. L. (1965). Pet turtles as a cause of human salmonellosis. JAMA J. Am. Med. Assoc. 192 (5), 347–351. doi:10.1001/jama.1965.03080180005001
Willis, C., Wilson, T., Greenwood, M., and Ward, L. (2002). Pet reptiles associated with a case of salmonellosis in an infant were carrying multiple strains of Salmonella. J. Clin. Microbiol. 40 (12), 4802–4803. doi:10.1128/jcm.40.12.4802-4803.2002
Work, T. M., Dagenais, J., Stacy, B. A., Ladner, J. T., Lorch, J. M., Balazs, G. H., et al. (2019). A novel host-adapted strain of Salmonella Typhimurium causes renal disease in olive ridley turtles (Lepidochelys olivacea) in the Pacific. Sci. Rep. 9 (1), 9313. doi:10.1038/s41598-019-45752-5
Xia, Y., Li, H., and Shen, Y. (2020). Antimicrobial drug resistance in Salmonella Enteritidis isolated from edible snakes with pneumonia and its pathogenicity in chickens. Front. Veterinary Sci. 7, 463. doi:10.3389/fvets.2020.00463
Zając, M., Skarżyńska, M., Lalak, A., Kwit, R., Śmiałowska-Węglińska, A., Pasim, P., et al. (2021). Salmonella in captive reptiles and their environment—Can we tame the dragon? Microorganisms 9 (5), 1012. doi:10.3390/microorganisms9051012
Keywords: Salmonella, reptile, zoonosis, prevalence, interactions
Citation: Pees M, Brockmann M, Steiner N and Marschang RE (2023) Salmonella in reptiles: a review of occurrence, interactions, shedding and risk factors for human infections. Front. Cell Dev. Biol. 11:1251036. doi: 10.3389/fcell.2023.1251036
Received: 30 June 2023; Accepted: 11 September 2023;
Published: 26 September 2023.
Edited by:
Frank Edlich, University of Leipzig, GermanyReviewed by:
Ricardo Lucena, Federal University of Paraíba, BrazilAna Fernández-Bravo, University of Rovira i Virgili, Spain
Copyright © 2023 Pees, Brockmann, Steiner and Marschang. This is an open-access article distributed under the terms of the Creative Commons Attribution License (CC BY). The use, distribution or reproduction in other forums is permitted, provided the original author(s) and the copyright owner(s) are credited and that the original publication in this journal is cited, in accordance with accepted academic practice. No use, distribution or reproduction is permitted which does not comply with these terms.
*Correspondence: Michael Pees, michael.pees@tiho-hannover.de