- Institute of Biomedical Engineering, West China School of Basic Medical Sciences and Forensic Medicine, Sichuan University, Chengdu, China
The mineralization of the extracellular matrix (ECM) is an essential and crucial process for physiological bone formation and pathological calcification. The abnormal function of ECM mineralization contributes to the worldwide risk of developing mineralization-related diseases; for instance, vascular calcification is attributed to the hyperfunction of ECM mineralization, while osteoporosis is due to hypofunction. AnnexinA6 (AnxA6), a Ca2+-dependent phospholipid-binding protein, has been extensively reported as an essential target in mineralization-related diseases such as osteoporosis, osteoarthritis, atherosclerosis, osteosarcoma, and calcific aortic valve disease. To date, AnxA6, as the largest member of the Annexin family, has attracted much attention due to its significant contribution to matrix vesicles (MVs) production and release, MVs-ECM interaction, cytoplasmic Ca2+ influx, and maturation of hydroxyapatite, making it an essential target in ECM mineralization. In this review, we outlined the recent advancements in the role of AnxA6 in mineralization-related diseases and the potential mechanisms of AnxA6 under normal and mineralization-related pathological conditions. AnxA6 could promote ECM mineralization for bone regeneration in the manner described previously. Therefore, AnxA6 may be a potential osteogenic target for ECM mineralization.
Introduction
The extracellular matrix (ECM) mineralization is an orchestrated and fine-tuned biological process during which inorganic minerals are produced and deposited in ECM (Hasegawa et al., 2022). The formation of hydroxyapatite (HA), the core element of inorganic minerals, depends on the combined effects of surrounding calcium ions (Ca2+), inorganic phosphate (PO43−), as well as matrix vesicles (MVs) (Wuthier and Lipscomb, 2011; Bottini et al., 2018; Veschi et al., 2022). Annexins (Anxs) are Ca2+-dependent phospholipid-binding proteins that emerge as a multigene family with a conserved evolutionary origin and are widely distributed in animals and plants. Increasingly, investigations have shown that annexins regulate Ca2+ influx and intracellular Ca2+ concentrations (Gerke and Moss, 2002). Ca2+ and annexins are considered critical elements involved in mineralization and play central roles in the initiation and maturation of ECM mineralization (Balcerzak et al., 2003).
ECM mineralization involves the formation of calcium phosphate crystals from the combination of Ca2+ and PO43−, which are then deposited on the collagen of the ECM. Briefly, membrane transporters and enzymes, including ATP-dependent Ca2+ pumps, and annexins as previously reported (Benz et al., 1996; Hoyal et al., 1996; Naciff et al., 1996; Fleet et al., 1999), initially transported Ca2+ and PO43− in extracellular fluid into MVs to form hydroxyapatite crystals. Afterward, the crystals gradually grow in the MVs, penetrate through the membrane of the MVs, deposit on collagen fibers, and ultimately form mineralization nodules. Of note, the levels and locations of ECM mineralization vary according to different physiological and pathological conditions. Physiological mineralization is typically observed in bone, tooth, and epiphyseal plates. In contrast, pathological mineralization known as “ectopic calcification” occurs in the arteries, myocardium, joints, and brain, leading to atherosclerosis, osteoarthritis, and calcific aortic valve disease (Maré et al.t, 2020), and some genetic diseases (Hahn et al., 2015; Reiss et al., 2018; Sherwood, 2019). Therefore, it is of great clinical significance to investigate the regulatory mechanisms of ECM mineralization and uncover the potential therapeutic targets for related diseases.
AnnexinA6 (AnxA6), a major component of MVs, has been reported to regulate physiological ECM mineralization by participating in Ca2+ transport and forming mineralized nucleation sites (Davies et al., 2019; Veschi et al., 2020; Veschi et al., 2022). AnxA6 has a Ca2+ transport capacity that can mediate Ca2+ influx into artificial liposomes (Matsuda et al., 1997). Some evidence suggests that AnxA6 may have Ca2+ channel properties, which mediate Ca2+ influx into MVs (Kirsch et al., 2000). AnxA6 forms voltage-dependent Ca2+ channels when inserted into artificial phosphatidylserine bilayers (Benz et al., 1996). However, deterministic conclusions still need to be justified by many studies. Given the vital role of AnxA6 in ECM mineralization and osteogenesis (Bolean et al., 2020; Bozycki et al., 2021; Mroczek et al., 2022; Pei et al., 2022), we reviewed the biological function of AnxA6 in regulating physiological and pathological ECM mineralization. We illustrated the underlying mechanisms to provide novel perspectives for mineralization-associated studies and contribute to treating mineralization-related diseases.
The structure and biological functions of AnxA6
Annexin is a group of Ca2+-dependent multifunctional lipid-binding proteins that Creutz discovered in 1978 (Creutz et al., 1978). According to the expression in different species, Anx is generally categorized into five groups: A (vertebrates), B (invertebrates), C (in fungi and some groups of unicellular eukaryotes), D (plants), and E (protists) (Moss and Morgan, 2004). In vertebrates, 12 kinds of AnxA members, including A1-A11 and A13, have been found. AnxAs have been reported to exert diverse functions in human systems and play different roles in regulating the progression of many diseases. The functions and distributions of AnxAs and AnxAs-associated diseases in humans are highlighted and summarized in Table 1.
As the largest member of the AnxAs family, the molecular weight (MW) of AnxA6 is up to 68 kDa (Huber et al., 1990a). In contrast to other AnxAs family proteins with only four homeodomains (Huber et al., 1990b), AnxA6 has a highly conserved core containing eight homeodomains, which further influences the function of AnxA6, such as membrane crosslinking and fold stabilization at high Ca2+ (Boye et al., 2018) (Figure 1). AnxA6 consists of two domains connected by hinge-like loops, which are not found in other AnxAs (Cornely et al., 2011). The Ca2+ binding sites of AnxA6 are positioned in repeats 1, 2, 4, 5, 6, and 8 (Huber et al., 1990a). The repeated domains may be derived from the evolution of integrating repeats of the AnxA5 and AnxA10 genes (Enrich et al., 2011). Alternative splicing of the AnxA6 gene produces two varying isoforms, AnxA6-1 and AnxA6-2, with a similar molecular weight of approximately 35 kDa (Avila-Sakar et al., 1998). AnxA6-1, found in most mammalian tissues, shows higher hydrophobicity and negative surface charges, while AnxA6-2, discovered in some immortalized cell lines (González-Noriega et al., 2016), is more affinitive to Ca2+ (Cornely et al., 2011). As reported, AnxA6 plays a biological role in mediating membrane receptor binding (Cornely et al., 2011), endocellular transport (Rentero et al., 2018), cytoskeleton reconstitution (Alvarez-Guaita et al., 2015), and transportation processes (Alvarez-Guaita et al., 2020), as well as being involved in physiological or pathological processes that are closely associated with the advancement of various diseases. The roles of AnxA6 in diseases are shown in Table 2.
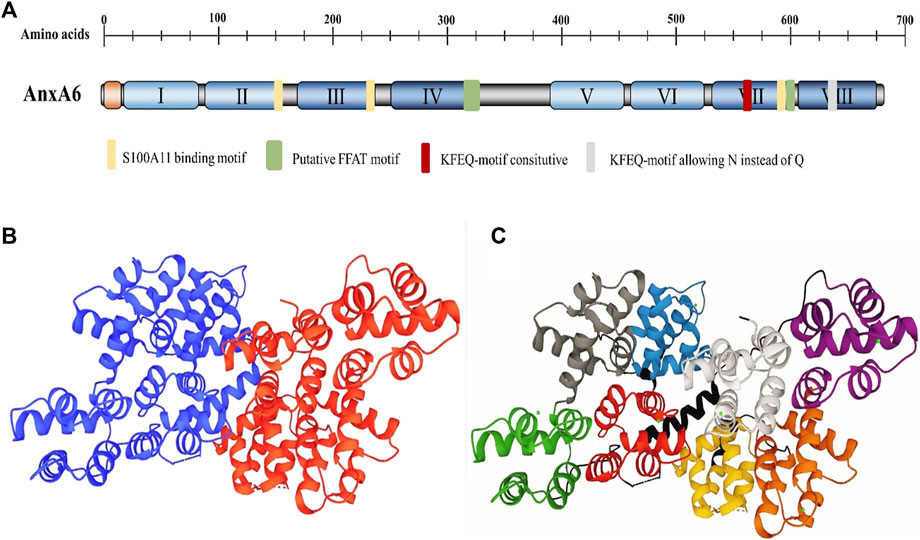
FIGURE 1. The structural organization of AnxA6. (A) Domain structure of AnxA6. The domain structure of AnxA6 is indicated. The N-terminal head domain (orange), C-terminal core with the AnxA6 repeats I–VIII (the only member within the Anx family), and length (in amino acids) are clarified. (B) The structure of AnxA6. AnxA6 consists of two lobes and a linker. The lobes are indicated by A (blue) and B (red). (C) Each lobe comprises four domains. The domain description and residue correspondence are as follows: Lobe A: N-terminal tail (1-19); domain I (green, 20-91); domain II (gray, 92-163); domain III (blue, 175-247); domain IV (red, 251-322). Interlobe linker: (black, 323-362). Lobe B: domain V (purple, 363-434); Domain VI (brown, 435-506); Domain VII (yellow, 521-595); Domain VIII (white, 599-670) (PDB file 1AVC).
However, despite these good insights, the need for an apparent phenotype in AnxA6 KO mice (Hawkins et al., 1999) has led to some questions about the role of AnxA6 in mineralization. AnxA6 is highly expressed in the skeleton, but no abnormalities of skeletal development have been found in AnxA6 KO mice or even in the double KO mice of AnxA6 and AnxA5 (Belluoccio et al., 2010; Grskovic et al., 2012). Still, Subsequent analysis of the skeletal phenotype of AnxA6 KO newborns shows a reduction in growth plate length and chondrocyte number, possibly due to reduced cartilage mineralization in the growth plate (Minashima et al., 2012). In addition, primary chondrocytes derived from AnxA6 KO mice show delayed terminal differentiation and reduced PKCα membrane translocation and activity, which may be one of the reasons for reduced MAPK signaling in chondrocytes (Minashima et al., 2012). In a study on osteoarthritis, cartilage destruction in knee joints was significantly reduced in AnxA6 KO mice, possibly due to reduced NFκB activity (Campbell et al., 2013). In contrast, in articular chondrocytes from control animals, AnxA6 attenuated cartilage degradation by interfering with the crosstalk between the Wnt/b-catenin signaling pathway and NFκB signaling, reducing catabolism, metabolism, and inflammatory responses in knee cartilage (Minashima and Kirsch, 2018). These studies on AnxA6 KO mice highlight the great therapeutic value of AnxA6 and the feasibility of AnxA6 for studying mineralization-related diseases.
AnxA6 participates in mineralization-related diseases
Osteoporosis (OP)
Osteoporosis is a common metabolic bone disease characterized by enhanced bone turnover, decreased bone mass, and susceptibility to fracture (Aibar-Almazán et al., 2022). Bone remodeling is a dynamic process during which the bone constantly experiences destruction and replacement. OP exists when the formation of new bone doesn’t follow the reduction of old bone. A case-control analysis in the Korean Women’s Cohort (3,570 subjects) has indicated that genetic variation of AnxA6 is significantly associated with OP (kim, 2011). The possible reasons are as follows: First, AnxA6 regulates osteoblast proliferation, differentiation, necrosis, and apoptosis (Kim et al., 2011); second, AnxA6 may interact with either phospholipids or type I collagen to induce the nucleation process in MVs-mediated mineralization (Veschi et al., 2020; Veschi et al., 2022). Accordingly, AnxA6 enhances the occurrence and progression of OP by exerting a significant influence on osteoblasts.
Osteoarthritis (OA)
Osteoarthritis is the most common joint disease, with more than 240 million people at risk worldwide (Katz et al., 2021). OA is generally represented by cartilage degeneration, bone remodeling, osteophyte generation, and joint dysfunction (Kraus et al., 2015). AnxA6 is reported to closely link with matrix vesicle-mediated mineralization of growth plate cartilage (Kirsch et al., 2000; Pfander et al., 2001). Notably, AnxA6 can be regarded as a marker in human osteoarthritic chondrocytes due to its high expression in OA cartilage, whereas low expression in healthy articular cartilage (Minashima et al., 2013). AnxA6 has been identified as a mediator of Ca2+ influx across membranes, leading to the induction of mineralization events in OA (Minashima et al., 2012). To test whether AnxA6 forms a Ca2+ channel in the plasma membrane, chondrocytes were treated with retinoic acid (RA) and antibodies specific to AnxA6. The anti–AnxA6 IgG fraction decreased the RA-mediated increase in the cytosolic calcium concentration by 65%, indicating that AnxA6 in the plasma membrane of growth plate chondrocytes and mediates Ca2+ influx (Wang and Kirsch, 2002). The underlying mechanisms of AnxA6-driven mineralization in OA probably depend on NF-κB and Wnt/β-catenin signaling pathways and their cross-talk (T. Minashima and Kirsch, 2018).
Atherosclerosis (AS)
Atherosclerosis is a chronic and complex inflammatory disease that can lead to life-threatening events, concentrated in most deaths worldwide (Libby, 2021). It is known that nidus calcification in atherosclerosis is widespread and enhanced with age (Hoffmann et al., 2003). Coronary artery calcium score, a measure of the total amount of calcification, is a positive biomarker of coronary plaque burden and offers prognostic information beyond that gained by conventional risk factor scoring (Pletcher et al., 2004). AnxA6 plays an essential role in the pathological calcification process of atherosclerosis, in which mature contractile vascular smooth muscle cells (VSMCs) withstand phenotypic transitions in response to pathological factors such as aging, oxidative stress, inflammation, and mechanical injury, leading to vascular ECM calcification (Gomez and Owens, 2012). Studies have revealed that vascular calcification is a strictly modulated process similar to bone mineralization (Shanahan et al., 2011). MVs-mediated mineralization is the primary pathological process that AnxA6 may participate in Reynolds et al. (2004) and New et al. (2013). That is to say, calcifying factors induce the secretion of MVs characterized by increased phosphatidyl serine and AnxA6 content, subsequently leading to vascular ECM calcification (Liberman and Marti, 2017). Meanwhile, an interesting study showed that AnxA6 was enriched in MVs derived from osteogenic medium-cultured smooth muscle cells (Rogers et al., 2020). Besides, several studies indicated that MVs from calcified smooth muscle cells had an increased AnxA6 content (Chen et al., 2008), as AnxA6 was also abundant at sites of vascular calcification in vivo (Kapustin et al., 2011).
Osteosarcoma (OS)
Osteosarcoma is the most common primary bone malignancy (Yang et al., 2020; Shoaib et al., 2022), characterized by osteolytic lesions radiographically (Kansara et al., 2014). A mineralized microenvironment is reported to induce osteogenic differentiation of mesenchymal stem cells, thus reducing OS progression (Rubio et al., 2014). Stimulation of cells for mineralization resulted in an upregulation of AnxA6 expression in OS Saos-2 cells, whereas its expression level significantly decreased upon inhibition of calcium channel activity. The existing evidence suggests that the membranous co-localization of AnxA6 and TNAP enhances submembrane mineralization (Bozycki et al., 2021). Additionally, AnxA6 is recruited to the membrane by co-localizing with cofilin-1 during MVs formation and participates in the mineralization process of OS Saos-2 cells (Thouverey et al., 2009). This approach can serve as a novel therapeutic intervention for osteoporosis by facilitating the process of mineralization.
Calcific aortic valve disease (CAVD)
Calcific aortic valve disease is a highly prevalent heart valve disease globally (Kraler et al., 2022). The pathophysiology of CAVD is complicated and influenced by various factors such as mechanical stress (Zhong et al., 2023), genetic factors (Iqbal et al., 2023), and inflammation (Broeders et al., 2022), but it shares similar mechanisms with physiological bone formation (Gollmann−Tepeköylü et al., 2023). The valvular interstitial cells (VICs) are the most plentiful type in the aortic valve and play a crucial role in CAVD development (Wu et al., 2017). VICs can transform into osteoblast-like cells, which cause osteogenic differentiation and calcification, consequently leading to the onset of CAVD. Previous research showed that 4-Octyl itaconate alleviated CAVD by ameliorating the osteogenic response of VICs (Peng et al., 2022). In addition, miR-22, as a promotor of the osteogenic differentiation of VICs, accelerated the process of CAVD (Yang et al., 2022). VIC-derived MVs (Cui et al., 2017) are critical in CAVD. Accordingly, AnxA6 was remarkably upregulated in calcified VIC-derived MVs in the calcified aortic valve compared with normal VICs. These data demonstrate the possible role of AnxA6 in the development of CAVD (Figure 2).
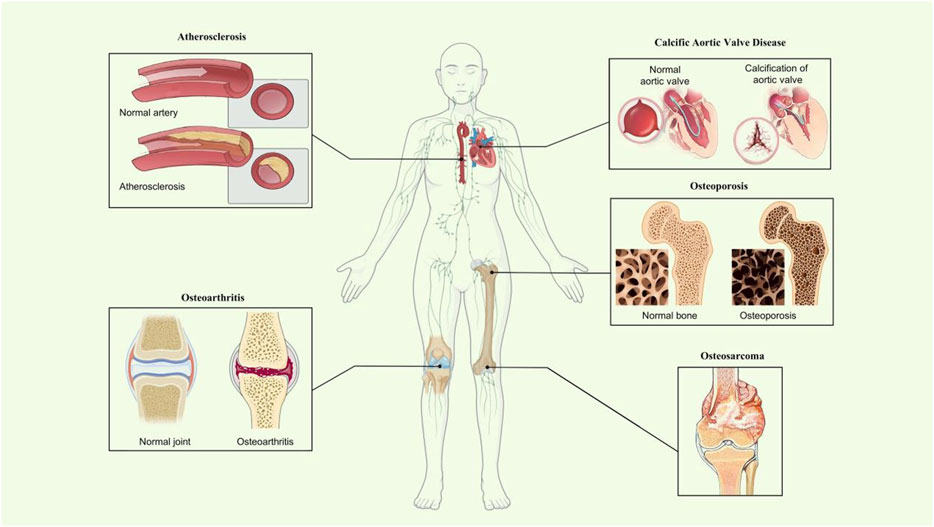
FIGURE 2. Roles of AnxA6 in mineralization-related disease. Schematic diagram showing what is known about AnxA6 in osteoporosis and osteoarthritis, atherosclerosis, osteosarcoma, and calcific aortic valve disease of mineralization-related diseases.
How does AnxA6 work during the physiological mineralization process?
AnxA6 promotes MVs generation and release
AnxA6 plays a crucial role in the generation and release of MVs. Studies have demonstrated that AnxA6 is enriched in MVs secreted by osteoblasts and hypertrophic chondrocytes in bone formation (Minashima et al., 2012). Previous studies indicated that elevated AnxA6 positively promoted the release of mineralization-competent MVs (Bozycki et al., 2021). The formation of MVs may be associated with the processes concerning AnxAs uncoupling from the cytoskeletal network (Wuthier and Lipscomb, 2011). Over the years, emerging evidence has shown that AnxA6 is involved in the formation of extracellular vesicles by mediating the fusion of early endosomes (Enrich et al., 2017), autophagosome/lysosome (Ghislat and Knecht, 2012), and late endosomes (LE)/lysosome (Futter and White, 2007). MVs are generally recognized to release from the cells by membrane budding, with complex regulatory mechanisms. Serving as an intracellular MVs biogenesis pathway, the effect of the mitochondria-lysosome axis has been previously identified (Iwayama et al., 2022). To date, AnxA6 has been reported to mediate the reprogramming of membrane-cytoskeleton interactions to upgrade membrane curvature, an initial condition for vesicle budding (Rentero et al., 2018). Furthermore, surface AnxA6 on the cell membrane interacts with proteins such as spectrin and dynamin, required for clathrin-coated vesicle budding and endocytic vesicle stripping of the plasma membrane (Grewal et al., 2000). AnxA6 is believed to be a key element in cell membrane fusion and budding events, which are essential for MVs generation and release.
AnxA6 promotes MVs binding to the ECM
After MVs are generated and secreted into the extracellular space, they will tightly anchor to the collagen in the ECM and initiate the secondary mineralization stage. The interaction between MVs and ECM mutually affected the extracellular accumulation and aggregation of calcified MVs (T.Li et al., 2022). Osteoblasts are generally responsible for synthesizing type I collagen-rich ECM, which is necessary for osteogenic mineralization (Bottini et al., 2018). In general, the linking role of AnxA6 should be analyzed from two aspects, including type I collagen and MVs membranes. Recently, AnxA6-loaded liposomes have been used to explore the role of AnxA6 in MVs-mediated mineralization, and the findings suggest that AnxA6 may exert its nucleation and mineralization abilities by necessary anchoring to type I collagen (Veschi et al., 2022). Other studies have reported similar findings (Chen et al., 2008). Take vascular calcification as an example; AnxA6 is enriched in calcified MVs and interacts with type I collagen to promote the mineralization processes (Kirsch et al., 2000). On the MVs membrane side, AnxA6 interacts with membranes by a lipid-related mechanism. In addition to perturbing cholesterol distribution (Swaggart et al., 2014), AnxA6 can bind to phosphatidylcholine on the MVs surface, which may contribute significantly to the interaction between MVs and collagen fibrils (Veschi et al., 2020).
AnxA6 promotes calcium influx in cells and MVs
As is known, the levels of Ca2+ are a critical determinant for ECM mineralization (Murshed, 2018). AnxA6 has been proposed to facilitate the influx of Ca2+ into mineralized MVs, as previously mentioned (Benz et al., 1996; Kirsch et al., 2000), possibly depending on two specific functional domains: 1) Ca2+ and lipid binding domains (Montaville et al., 2002), which are responsible for Ca2+ transport to endosomes by binding AnxA6 to cholesterol (de Diego et al., 2002); 2) pH-sensitive domains, which regulate the ion channel activity by affecting the folding degree of AnxA6 under different pH conditions (Golczak et al., 2001a; Golczak et a;., 2001b) and providing the foundation for Ca2+ influx.
Two isoforms of AnxA6, AnxA6-1 and AnxA6-2, exert different functions for Ca2+ influx due to their different structures. Existing data suggest that AnxA6-2 has a greater affinity for Ca2+ (Kaetzel et al., 1994). AnxA6-2 can form a narrower region with better Ca2+ binding ability. Furthermore, AnxA6-2 has a more comprehensive pH response range and is sensitive to changes in Ca2+ and proton concentration (Strzelecka−Kiliszek et al., 2008). In addition to the two isoforms above, a 35-kDa fragment of AnxA6 is also present in MVs (Wu et al., 1993), which is responsive to collagenase and/or endogenous proteases (Mookhtiar and Van Wart, 1992; Chen and Golub, 2001) and can tightly bind to calcium ions. AnxA6 promotes Ca2+ influx due to its location in the outer lobe of bilayer structures (Kirsch et al., 2000). Besides its Ca2+ channel activity in cells such as osteogenic differentiated chondrocytes, AnxA6 also plays a crucial role in Ca2+ influx in MVs (Kirsch et al., 2000). AnxA6 was identified to regulate mineralization events of chondrocytes by interacting with Protein Kinase C (PKC) and subsequently regulate Ca2+ influx in MVs (Minashima et al., 2012). AnxA6 knockdown, on the other hand, inhibited chondrocyte terminal differentiation and calcium uptake capacity (Grewal et al., 2016), preventing internal Ca2+ influx in both cells and MVs (Minashima et al., 2012). In conclusion, AnxA6 likely promotes ECM mineralization by facilitating the influx of Ca2+ into mineralized MVs.
AnxA6 promotes nucleation core formation in MVs
AnxA6 is a major content protein of MVs and can also form nucleation sites upon binding to the MVs (Genge et al., 2007). SDS-PAGE characterization, Fourier-transform infrared, and NMR (Sauer and Wuthier, 1988; Genge et al., 1989; Genge et al., 1990; Wu et al., 1997) indicate that there are three crucial components in the nucleation core: 1) amorphous calcium phosphate (ACP); 2) phosphatidylserine-Ca2+-Pi complexes (PS-CPLX); 3) AnxAs, including AnxA6 (Wu et al., 1997).
How does AnxA6 contribute to nucleation core formation? First, the conformational variants of AnxA6 (Avila−Sakar et al., 2000) facilitate the nucleation of crystalline Ca-Pi. Second, AnxA6 promotes nucleation core formation due to its unique ability to bind to sphingolipids and cholesterol, which are abundant in membrane rafts (Babiychuk et al., 1999; Babiychuk and Draeger, 2000). Third, AnxA6 can further promote the accumulation of Ca2+ and stabilize the combination of Ca2+ and PS (Veschi et al., 2022), thus leading to hydroxyapatite formation. Finally, AnxA6 transfers from the inner surface of MVs to the outer surface and binds to phosphatidylcholine (PC) on the outer surface of MVs (Figure 3).
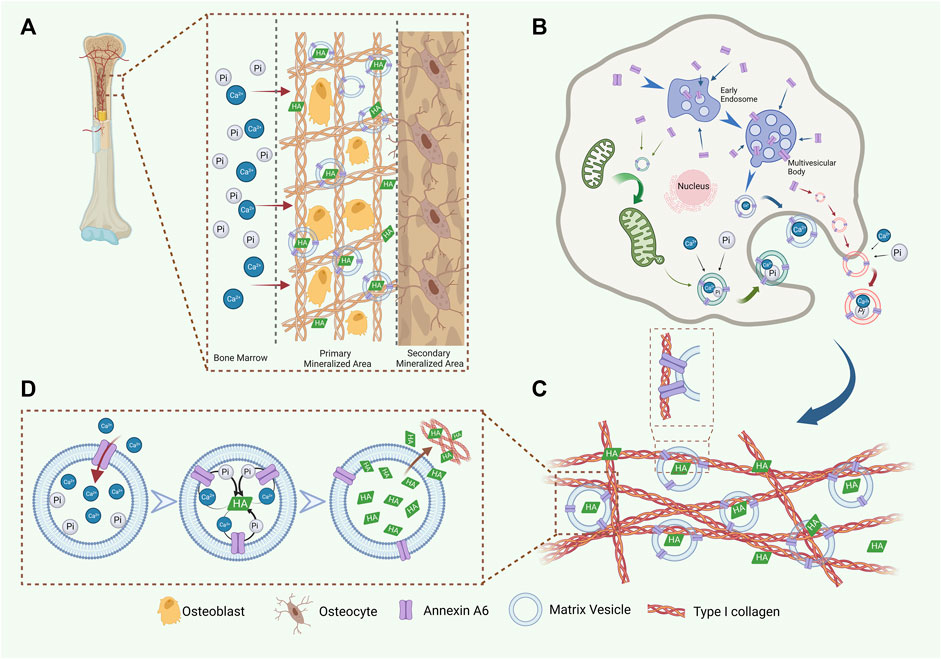
FIGURE 3. AnxA6 is involved in the formation, release, and Ca2+ influx of MVs. (A) A schematic representation of AnxA6 in ECM mineralization. (B) AnxA6 likely enhances the formation of MVs via three cooperative/redundant mechanisms include (i) MVs (red), which accumulate calcium (Ca2+) and phosphate (PO43−) ions extracellularly, bud from the plasma membrane; (ii) MVs (green), which transport amorphous calcium phosphate and ionic calcium stored in mitochondria to the ECM; (iii) MVs (blue), originated from multivesicular bodies (MVBs) in “exosome-like” biogenesis pathway. (C) AnxA6 mediates MVs’ tight binding to type I collagen in the ECM, which benefits ECM mineralization. (D) AnxA6 on the MVs membrane facilitates Ca2+ influx and the formation of HA within MVs. Subsequently, AnxA6 drains HA outside to promote ECM mineralization.
Conclusion and perspectives
In conclusion, AnxA6 has novel biological functions and potential therapeutic applications in the mineralization of extracellular matrix, which may provide promising AnxA6-based therapeutic strategies for mineralization-related diseases, pave a novel way for drug discovery, and pursue AnxA6-based therapeutic strategies for mineralization-related diseases. AnxA6 can create an enabling environment for hydroxyapatite formation by promoting Ca2+ influx. Additionally, as an essential component of MVs, AnxA6 promotes the attachment of MVs to ECM. However, there remain questions that need to be further addressed. AnxA6 has two isoforms, but the significance of these isoforms in mineralization-related progression has yet to be thoroughly investigated. Further studies are needed to better understand the interactions among AnxA6 subtypes and clarify the mechanisms of AnxA6-promoted ECM mineralization. A better understanding of these mechanisms may contribute to developing mineralization-related disease therapies. Moreover, to move such research forward, the translational capacity of AnxA6 should be confirmed through clinical trials in the future.
Author contributions
JY and XL designed the scope of the review and wrote the manuscript. JY, TP, GS, and PD performed the document searching and prepared the figures. XL guided the planning and critically amended the manuscript. All authors contributed to the article and approved the submitted version.
Funding
This study was supported by the National Natural Science Foundation of China (Nos 11932014 and 31971239) and Sichuan Science and Technology Program (Nos 2022NSFSC0765 and 2022ZYD0079).
Conflict of interest
The authors declare that the research was conducted in the absence of any commercial or financial relationships that could be construed as a potential conflict of interest.
Publisher’s note
All claims expressed in this article are solely those of the authors and do not necessarily represent those of their affiliated organizations, or those of the publisher, the editors and the reviewers. Any product that may be evaluated in this article, or claim that may be made by its manufacturer, is not guaranteed or endorsed by the publisher.
References
Aibar-Almazán, A., Voltes-Martínez, A., Castellote-Caballero, Y., Afanador-Restrepo, D. F., Carcelén-Fraile, M. D. C., and López-Ruiz, E. (2022). Current status of the diagnosis and management of osteoporosis. Int. J. Mol. Sci. 23 (16), 9465. doi:10.3390/ijms23169465
Alauddin, M., Salker, M. S., Umbach, A. T., Rajaxavier, J., Okumura, T., Singh, Y., et al. (2020). Annexin A7 regulates endometrial receptivity. Front. Cell. Dev. Biol. 8, 770. doi:10.3389/fcell.2020.00770
Alvarez-Guaita, A., Vilà de Muga, S., Owen, D. M., Williamson, D., Magenau, A., García-Melero, A., et al. (2015). Evidence for annexin A6-dependent plasma membrane remodelling of lipid domains. Br. J. Pharmacol. 172 (7), 1677–1690. doi:10.1111/bph.13022
Alvarez-Guaita, A., Blanco-Muñoz, P., Meneses-Salas, E., Wahba, M., Pollock, A. H., Jose, J., et al. (2020). Annexin A6 is critical to maintain glucose homeostasis and survival during liver regeneration in mice. Hepatol. Baltim. Md.) 72 (6), 2149–2164. doi:10.1002/hep.31232
Avila-Sakar, A. J., Creutz, C. E., and Kretsinger, R. H. (1998). Crystal structure of bovine annexin VI in a calcium-bound state. Biochimica Biophysica Acta 1387 (1-2), 103–116. doi:10.1016/s0167-4838(98)00111-3
Avila-Sakar, A. J., Kretsinger, R. H., and Creutz, C. E. (2000). Membrane-bound 3D structures reveal the intrinsic flexibility of annexin VI. J. Struct. Biol. 130 (1), 54–62. doi:10.1006/jsbi.2000.4246
Babiychuk, E. B., and Draeger, A. (2000). Annexins in cell membrane dynamics. Ca(2+)-regulated association of lipid microdomains. J. Cell. Biol. 150 (5), 1113–1124. doi:10.1083/jcb.150.5.1113
Babiychuk, E. B., Palstra, R. J., Schaller, J., Kämpfer, U., and Draeger, A. (1999). Annexin VI participates in the formation of a reversible, membrane-cytoskeleton complex in smooth muscle cells. J. Biol. Chem. 274 (49), 35191–35195. doi:10.1074/jbc.274.49.35191
Balcerzak, M., Hamade, E., Zhang, L., Pikula, S., Azzar, G., Radisson, J., et al. (2003). The roles of annexins and alkaline phosphatase in mineralization process. Acta Biochim. Pol. 50 (4), 1019–1038.
Belluoccio, D., Grskovic, I., Niehoff, A., Schlötzer-Schrehardt, U., Rosenbaum, S., Etich, J., et al. (2010). Deficiency of annexins A5 and A6 induces complex changes in the transcriptome of growth plate cartilage but does not inhibit the induction of mineralization. J. Bone Mineral Res. Official J. Am. Soc. Bone Mineral Res. 25 (1), 141–153. doi:10.1359/jbmr.090710
Benz, J., Bergner, A., Hofmann, A., Demange, P., Göttig, P., Liemann, S., et al. (1996). The structure of recombinant human annexin VI in crystals and membrane-bound. J. Mol. Biol. 260 (5), 638–643. doi:10.1006/jmbi.1996.0426
Bolean, M., Izzi, B., van Kerckhoven, S., Bottini, M., Ramos, A. P., Millán, J. L., et al. (2020). Matrix vesicle biomimetics harboring Annexin A5 and alkaline phosphatase bind to the native collagen matrix produced by mineralizing vascular smooth muscle cells. Biochimica Biophysica Acta General Subj. 1864 (8), 129629. doi:10.1016/j.bbagen.2020.129629
Bottini, M., Mebarek, S., Anderson, K. L., Strzelecka-Kiliszek, A., Bozycki, L., Simao, A. M. S., et al. (2018). Matrix vesicles from chondrocytes and osteoblasts: their biogenesis, properties, functions and biomimetic models. Biochim. Biophys. Acta Gen. Subj. 1862 (3), 532–546. doi:10.1016/j.bbagen.2017.11.005
Bouter, A., Gounou, C., Bérat, R., Tan, S., Gallois, B., Granier, T., et al. (2011). Annexin-A5 assembled into two-dimensional arrays promotes cell membrane repair. Nat. Commun. 2, 270. doi:10.1038/ncomms1270
Bouter, A., Carmeille, R., Gounou, C., Bouvet, F., Degrelle, S. A., Evain-Brion, D., et al. (2015). Review: annexin-A5 and cell membrane repair. Placenta 36 (1), S43–S49. doi:10.1016/j.placenta.2015.01.193
Boye, T. L., Maeda, K., Pezeshkian, W., Sønder, S. L., Haeger, S. C., Gerke, V., et al. (2017). Annexin A4 and A6 induce membrane curvature and constriction during cell membrane repair. Nat. Commun. 8 (1), 1623. doi:10.1038/s41467-017-01743-6
Boye, T. L., Jeppesen, J. C., Maeda, K., Pezeshkian, W., Solovyeva, V., Nylandsted, J., et al. (2018). Annexins induce curvature on free-edge membranes displaying distinct morphologies. Sci. Rep. 8 (1), 10309. doi:10.1038/s41598-018-28481-z
Bozycki, L., Mroczek, J., Bessueille, L., Mebarek, S., Buchet, R., Pikula, S., et al. (2021). Annexins A2, A6 and fetuin-A affect the process of mineralization in vesicles derived from human osteoblastic hFOB 1.19 and osteosarcoma saos-2 cells. Int. J. Mol. Sci. 22 (8), 3993. doi:10.3390/ijms22083993
Broeders, W., Bekkering, S., El Messaoudi, S., Joosten, L. A. B., van Royen, N., and Riksen, N. P. (2022). Innate immune cells in the pathophysiology of calcific aortic valve disease: lessons to be learned from atherosclerotic cardiovascular disease? Basic Res. Cardiol. 117 (1), 28. doi:10.1007/s00395-022-00935-6
Buzhynskyy, N., Golczak, M., Lai-Kee-Him, J., Lambert, O., Tessier, B., Gounou, C., et al. (2009). Annexin-A6 presents two modes of association with phospholipid membranes. A combined QCM-D, AFM and cryo-TEM study. J. Struct. Biol. 168 (1), 107–116. doi:10.1016/j.jsb.2009.03.007
Campbell, K. A., Minashima, T., Zhang, Y., Hadley, S., Lee, Y. J., Giovinazzo, J., et al. (2013). Annexin A6 interacts with p65 and stimulates NF-κB activity and catabolic events in articular chondrocytes. Arthritis Rheum. 65 (12), 3120–3129. doi:10.1002/art.38182
Cañas, F., Simonin, L., Couturaud, F., and Renaudineau, Y. (2015). Annexin A2 autoantibodies in thrombosis and autoimmune diseases. Thromb. Res. 135 (2), 226–230. doi:10.1016/j.thromres.2014.11.034
Chen, D., and Golub, E. E. (2001). Search for an endogenous collagenase in chicken endochondral bone matrix vesicles. Penn Dent. J. 101, 12–32. Retrieved from https://pubmed.ncbi.nlm.nih.gov/15484638.
Chen, N. X., O'Neill, K. D., Chen, X., and Moe, S. M. (2008). Annexin-mediated matrix vesicle calcification in vascular smooth muscle cells. J. Bone Mineral Res. 23 (11), 1798–1805. the Official Journal of the American Society For Bone and Mineral Research. doi:10.1359/jbmr.080604
Chen, J., Norling, L. V., Mesa, J. G., Silva, M. P., Burton, S. E., Reutelingsperger, C., et al. (2021). Annexin A1 attenuates cardiac diastolic dysfunction in mice with inflammatory arthritis. Proc. Natl. Acad. Sci. U. S. A. 118 (38), e2020385118. doi:10.1073/pnas.2020385118
Chen, X., Song, X., Li, J., Zhang, R., Yu, C., Zhou, Z., et al. (2023). Identification of HPCAL1 as a specific autophagy receptor involved in ferroptosis. Autophagy 19 (1), 54–74. doi:10.1080/15548627.2022.2059170
Clark, K. C., Nguyen, E. V., Niranjan, B., Wu, Y., Lim Kam Sian, T. C. C., Horvath, L. G., et al. (2023). Cell-type-specific signalling networks impacted by prostate epithelial-stromal intercellular communication. Cancers 15 (3), 699. doi:10.3390/cancers15030699
Cornely, R., Rentero, C., Enrich, C., Grewal, T., and Gaus, K. (2011). Annexin A6 is an organizer of membrane microdomains to regulate receptor localization and signalling. IUBMB Life 63 (11), 1009–1017. doi:10.1002/iub.540
Creutz, C. E., Pazoles, C. J., and Pollard, H. B. (1978). Identification and purification of an adrenal medullary protein (synexin) that causes calcium-dependent aggregation of isolated chromaffin granules. J. Biol. Chem. 253 (8), 2858–2866. doi:10.1016/s0021-9258(17)40901-x
Croissant, C., Gounou, C., Bouvet, F., Tan, S., and Bouter, A. (2020). Annexin-A6 in membrane repair of human skeletal muscle cell: A role in the cap subdomain. Cells 9 (7), 1742. doi:10.3390/cells9071742
Croissant, C., Carmeille, R., Brévart, C., and Bouter, A. (2021). Annexins and membrane repair dysfunctions in muscular dystrophies. Int. J. Mol. Sci. 22 (10), 5276. doi:10.3390/ijms22105276
Croissant, C., Gounou, C., Bouvet, F., Tan, S., and Bouter, A. (2022). Trafficking of annexins during membrane repair in human skeletal muscle cells. Membr. (Basel) 12 (2), 153. doi:10.3390/membranes12020153
Cui, L., Rashdan, N. A., Zhu, D., Milne, E. M., Ajuh, P., Milne, G., et al. (2017). End stage renal disease-induced hypercalcemia may promote aortic valve calcification via Annexin VI enrichment of valve interstitial cell derived-matrix vesicles. J. Cell. Physiology 232 (11), 2985–2995. doi:10.1002/jcp.25935
Davies, O. G., Cox, S. C., Azoidis, I., McGuinness, A. J. A., Cooke, M., Heaney, L. M., et al. (2019). Osteoblast-derived vesicle protein content is temporally regulated during osteogenesis: implications for regenerative therapies. Front. Bioeng. Biotechnol. 7, 92. doi:10.3389/fbioe.2019.00092
de Diego, I., Schwartz, F., Siegfried, H., Dauterstedt, P., Heeren, J., Beisiegel, U., et al. (2002). Cholesterol modulates the membrane binding and intracellular distribution of annexin 6. J. Biol. Chem. 277 (35), 32187–32194. doi:10.1074/jbc.M205499200
Demonbreun, A. R., Quattrocelli, M., Barefield, D. Y., Allen, M. V., Swanson, K. E., and McNally, E. M. (2016). An actin-dependent annexin complex mediates plasma membrane repair in muscle. J. Cell. Biol. 213 (6), 705–718. doi:10.1083/jcb.201512022
Demonbreun, A. R., Fallon, K. S., Oosterbaan, C. C., Bogdanovic, E., Warner, J. L., Sell, J. J., et al. (2019). Recombinant annexin A6 promotes membrane repair and protects against muscle injury. J. Clin. Investigation 129 (11), 4657–4670. doi:10.1172/jci128840
Demonbreun, A. R., Bogdanovic, E., Vaught, L. A., Reiser, N. L., Fallon, K. S., Long, A. M., et al. (2022). A conserved annexin A6-mediated membrane repair mechanism in muscle, heart, and nerve. JCI Insight 7 (14), e158107. doi:10.1172/jci.insight.158107
Du, R., Liu, B., Zhou, L., Wang, D., He, X., Xu, X., et al. (2018). Downregulation of annexin A3 inhibits tumor metastasis and decreases drug resistance in breast cancer. Cell. Death Dis. 9 (2), 126. doi:10.1038/s41419-017-0143-z
Enrich, C., Rentero, C., de Muga, S. V., Reverter, M., Mulay, V., Wood, P., et al. (2011). Annexin A6-Linking Ca(2+) signaling with cholesterol transport. Biochimica Biophysica Acta 1813 (5), 935–947. doi:10.1016/j.bbamcr.2010.09.015
Enrich, C., Rentero, C., and Grewal, T. (2017). Annexin A6 in the liver: from the endocytic compartment to cellular physiology. Biochim. Biophys. Acta Mol. Cell. Res. 1864 (6), 933–946. doi:10.1016/j.bbamcr.2016.10.017
Filipenko, N. R., MacLeod, T. J., Yoon, C.-S., and Waisman, D. M. (2004). Annexin A2 is a novel RNA-binding protein. J. Biol. Chem. 279 (10), 8723–8731. doi:10.1074/jbc.M311951200
Fleet, A., Ashworth, R., Kubista, H., Edwards, H., Bolsover, S., Mobbs, P., et al. (1999). Inhibition of EGF-dependent calcium influx by annexin VI is splice form-specific. Biochem. Biophys. Res. Commun. 260 (2), 540–546. doi:10.1006/bbrc.1999.0915
Foltz, S. J., Cui, Y. Y., Choo, H. J., and Hartzell, H. C. (2021). ANO5 ensures trafficking of annexins in wounded myofibers. J. Cell. Biol. 220 (3), e202007059. doi:10.1083/jcb.202007059
Foo, S. L., Yap, G., Cui, J., and Lim, L. H. K. (2019). Annexin-A1 - a blessing or a curse in cancer? Trends Mol. Med. 25 (4), 315–327. doi:10.1016/j.molmed.2019.02.004
Futter, C. E., and White, I. J. (2007). Annexins and endocytosis. Traffic (Copenhagen, Den. 8 (8), 951–958. doi:10.1111/j.1600-0854.2007.00590.x
Gao, Y., Zhang, H., Wang, J., Li, F., Li, X., Li, T., et al. (2023). Annexin A5 ameliorates traumatic brain injury-induced neuroinflammation and neuronal ferroptosis by modulating the NF-ĸB/HMGB1 and Nrf2/HO-1 pathways. Int. Immunopharmacol. 114, 109619. doi:10.1016/j.intimp.2022.109619
Garnier, B., Bouter, A., Gounou, C., Petry, K. G., and Brisson, A. R. (2009). Annexin A5-functionalized liposomes for targeting phosphatidylserine-exposing membranes. Bioconjug Chem. 20 (11), 2114–2122. doi:10.1021/bc9002579
Garrido-Gómez, T., Castillo-Marco, N., Cordero, T., and Simón, C. (2022). Decidualization resistance in the origin of preeclampsia. Am. J. Obstet. Gynecol. 226 (2), S886–s894. doi:10.1016/j.ajog.2020.09.039
Genge, B. R., Wu, L. N., and Wuthier, R. E. (1989). Identification of phospholipid-dependent calcium-binding proteins as constituents of matrix vesicles. J. Biol. Chem. 264 (18), 10917–10921. doi:10.1016/s0021-9258(18)81708-2
Genge, B. R., Wu, L. N., and Wuthier, R. E. (1990). Differential fractionation of matrix vesicle proteins. Further characterization of the acidic phospholipid-dependent Ca2(+)-binding proteins. J. Biol. Chem. 265 (8), 4703–4710. doi:10.1016/s0021-9258(19)39619-x
Genge, B. R., Wu, L. N., and Wuthier, R. E. (2007). In vitro modeling of matrix vesicle nucleation: synergistic stimulation of mineral formation by annexin A5 and phosphatidylserine. J. Biol. Chem. 282 (36), 26035–26045. doi:10.1074/jbc.M701057200
Gerke, V., and Moss, S. E. (2002). Annexins: from structure to function. Physiol. Rev. 82 (2), 331–371. doi:10.1152/physrev.00030.2001
Ghislat, G., and Knecht, E. (2012). New Ca(2+)-dependent regulators of autophagosome maturation. Commun. Integr. Biol. 5 (4), 308–311. doi:10.4161/cib.20076
Golczak, M., Kicinska, A., Bandorowicz-Pikula, J., Buchet, R., Szewczyk, A., and Pikula, S. (2001a). Acidic pH-induced folding of annexin VI is a prerequisite for its insertion into lipid bilayers and formation of ion channels by the protein molecules. FASEB J. Official Publ. Fed. Am. Soc. Exp. Biol. 15 (6), 1083–1085. doi:10.1096/fj.00-0523fje
Golczak, M., Kirilenko, A., Bandorowicz-Pikula, J., and Pikula, S. (2001b). Conformational states of annexin VI in solution induced by acidic pH. FEBS Lett. 496 (1), 49–54. doi:10.1016/s0014-5793(01)02402-4
Gollmann-Tepeköylü, C., Graber, M., Hirsch, J., Mair, S., Naschberger, A., Pölzl, L., et al. (2023). Toll-like receptor 3 mediates aortic stenosis through a conserved mechanism of calcification. Circulation 147 (20), 1518–1533. doi:10.1161/circulationaha.122.063481
Gomez, D., and Owens, G. K. (2012). Smooth muscle cell phenotypic switching in atherosclerosis. Cardiovasc. Res. 95 (2), 156–164. doi:10.1093/cvr/cvs115
González-Noriega, A., Michalak, C., Cervantes-Roldán, R., Gómez-Romero, V., and León-Del-Río, A. (2016). Two translation initiation codons direct the expression of annexin VI 64kDa and 68kDa isoforms. Mol. Genet. Metabolism 119 (4), 338–343. doi:10.1016/j.ymgme.2016.10.002
Gou, R., Zhu, L., Zheng, M., Guo, Q., Hu, Y., Li, X., et al. (2019). Annexin A8 can serve as potential prognostic biomarker and therapeutic target for ovarian cancer: based on the comprehensive analysis of annexins. J. Transl. Med. 17 (1), 275. doi:10.1186/s12967-019-2023-z
Gounou, C., Bouvet, F., Liet, B., Prouzet-Mauléon, V., d'Agata, L., Harté, E., et al. (2023). Annexin-A5 and annexin-A6 silencing prevents metastasis of breast cancer cells in zebrafish. Biol. Cell. 115 (6), e202200110. doi:10.1111/boc.202200110
Grewal, T., Heeren, J., Mewawala, D., Schnitgerhans, T., Wendt, D., Salomon, G., et al. (2000). Annexin VI stimulates endocytosis and is involved in the trafficking of low density lipoprotein to the prelysosomal compartment. J. Biol. Chem. 275 (43), 33806–33813. doi:10.1074/jbc.M002662200
Grewal, T., Wason, S. J., Enrich, C., and Rentero, C. (2016). Annexins - insights from knockout mice. Biol. Chem. 397 (10), 1031–1053. doi:10.1515/hsz-2016-0168
Grskovic, I., Kutsch, A., Frie, C., Groma, G., Stermann, J., Schlötzer-Schrehardt, U., et al. (2012). Depletion of annexin A5, annexin A6, and collagen X causes no gross changes in matrix vesicle-mediated mineralization, but lack of collagen X affects hematopoiesis and the Th1/Th2 response. J. Bone Mineral Res. Official J. Am. Soc. Bone Mineral Res. 27 (11), 2399–2412. doi:10.1002/jbmr.1682
Guo, C., Li, N., Dong, C., Wang, L., Li, Z., Liu, Q., et al. (2021). 33-kDa ANXA3 isoform contributes to hepatocarcinogenesis via modulating ERK, PI3K/Akt-HIF and intrinsic apoptosis pathways. J. Adv. Res. 30, 85–102. doi:10.1016/j.jare.2020.11.003
Hahn, D., Hodson, E. M., and Craig, J. C. (2015). Interventions for metabolic bone disease in children with chronic kidney disease. Cochrane Database Syst. Rev. 11, CD008327. doi:10.1002/14651858.CD008327.pub2
Hasegawa, T., Hongo, H., Yamamoto, T., Abe, M., Yoshino, H., Haraguchi-Kitakamae, M., et al. (2022). Matrix vesicle-mediated mineralization and osteocytic regulation of bone mineralization. Int. J. Mol. Sci. 23 (17), 9941. doi:10.3390/ijms23179941
Hawkins, T. E., Roes, J., Rees, D., Monkhouse, J., and Moss, S. E. (1999). Immunological development and cardiovascular function are normal in annexin VI null mutant mice. Mol. Cell. Biol. 19 (12), 8028–8032. doi:10.1128/mcb.19.12.8028
Heitzig, N., Brinkmann, B. F., Koerdt, S. N., Rosso, G., Shahin, V., and Rescher, U. (2017). Annexin A8 promotes VEGF-A driven endothelial cell sprouting. Cell. Adh Migr. 11 (3), 275–287. doi:10.1080/19336918.2016.1264559
Heitzig, N., Kühnl, A., Grill, D., Ludewig, K., Schloer, S., Galla, H. J., et al. (2018). Cooperative binding promotes demand-driven recruitment of AnxA8 to cholesterol-containing membranes. Biochim. Biophys. Acta Mol. Cell. Biol. Lipids 1863 (4), 349–358. doi:10.1016/j.bbalip.2018.01.001
Hoffmann, U., Brady, T. J., and Muller, J. (2003). Cardiology patient page. Use of new imaging techniques to screen for coronary artery disease. Circulation 108 (8), e50–e53. doi:10.1161/01.CIR.0000085363.88377.F2
Hoque, M., Elmaghrabi, Y. A., Köse, M., Beevi, S. S., Jose, J., Meneses-Salas, E., et al. (2020). Annexin A6 improves anti-migratory and anti-invasive properties of tyrosine kinase inhibitors in EGFR overexpressing human squamous epithelial cells. FEBS J. 287 (14), 2961–2978. doi:10.1111/febs.15186
Hoyal, C. R., Thomas, A. P., and Forman, H. J. (1996). Hydroperoxide-induced increases in intracellular calcium due to annexin VI translocation and inactivation of plasma membrane Ca2+-ATPase. J. Biol. Chem. 271 (46), 29205–29210. doi:10.1074/jbc.271.46.29205
Hua, K., Li, Y., Zhao, Q., Fan, L., Tan, B., and Gu, J. (2018). Downregulation of annexin A11 (ANXA11) inhibits cell proliferation, invasion, and migration via the AKT/GSK-3β pathway in gastric cancer. Med. Sci. Monit. 24, 149–160. doi:10.12659/msm.905372
Huber, R., Römisch, J., and Paques, E. P. (1990a). The crystal and molecular structure of human annexin V, an anticoagulant protein that binds to calcium and membranes. EMBO J. 9 (12), 3867–3874. doi:10.1002/j.1460-2075.1990.tb07605.x
Huber, R., Schneider, M., Mayr, I., Rmisch, J., and Paques, E. P. (1990b). The calcium binding sites in human annexin V by crystal structure analysis at 2.0 A resolution Implications for membrane binding and calcium channel activity. FEBS Lett. 275 (1-2), 15–21. doi:10.1016/0014-5793(90)81428-q
Iqbal, F., Schlotter, F., Becker-Greene, D., Lupieri, A., Goettsch, C., Hutcheson, J. D., et al. (2023). Sortilin enhances fibrosis and calcification in aortic valve disease by inducing interstitial cell heterogeneity. Eur. Heart J. 44 (10), 885–898. doi:10.1093/eurheartj/ehac818
Ishikawa, A., Kuraoka, K., Zaitsu, J., Saito, A., Kuwai, T., Tazawa, H., et al. (2022a). Transcriptomic analysis of annexin A10 and chemosensitivity in gastric adenocarcinoma cells. Anticancer Res. 42 (4), 1707–1717. doi:10.21873/anticanres.15647
Ishikawa, A., Kuraoka, K., Zaitsu, J., Saito, A., Yamaguchi, A., Kuwai, T., et al. (2022b). High Annexin A10 expression is correlated with poor prognosis in pancreatic ductal adenocarcinoma. Histol. Histopathol. 37 (3), 243–250. doi:10.14670/hh-18-397
Iwayama, T., Bhongsatiern, P., Takedachi, M., and Murakami, S. (2022). Matrix vesicle-mediated mineralization and potential applications. J. Dent. Res. 101 (13), 1554–1562. doi:10.1177/00220345221103145
Jiang J, J., Zhan, X., Qu, H., Liang, T., Li, H., Chen, L., et al. (2022). Upregulated of ANXA3, SORL1, and neutrophils may Be key factors in the progressionof ankylosing spondylitis. Front. Immunol. 13, 861459. doi:10.3389/fimmu.2022.861459
Jiang Q, Q., Lin, J., Wei, Q., Li, C., Hou, Y., Cao, B., et al. (2022). Genetic analysis of and clinical characteristics associated with ANXA11 variants in a Chinese cohort with amyotrophic lateral sclerosis. Neurobiol. Dis. 175, 105907. doi:10.1016/j.nbd.2022.105907
Johari, M., Papadimas, G., Papadopoulos, C., Xirou, S., Kanavaki, A., Chrysanthou-Piterou, M., et al. (2022). Adult-onset dominant muscular dystrophy in Greek families caused by Annexin A11. Ann. Clin. Transl. Neurol. 9 (10), 1660–1667. doi:10.1002/acn3.51665
Jose, J., Hoque, M., Engel, J., Beevi, S. S., Wahba, M., Georgieva, M. I., et al. (2022). Annexin A6 and NPC1 regulate LDL-inducible cell migration and distribution of focal adhesions. Sci. Rep. 12 (1), 596. doi:10.1038/s41598-021-04584-y
Kaetzel, M. A., Pula, G., Campos, B., Uhrin, P., Horseman, N., and Dedman, J. R. (1994). Annexin VI isoforms are differentially expressed in mammalian tissues. Biochimica Biophysica Acta 1223 (3), 368–374. doi:10.1016/0167-4889(94)90097-3
Kang, T. H., Park, J. H., Yang, A., Park, H. J., Lee, S. E., Kim, Y. S., et al. (2020). Annexin A5 as an immune checkpoint inhibitor and tumor-homing molecule for cancer treatment. Nat. Commun. 11 (1), 1137. doi:10.1038/s41467-020-14821-z
Kansara, M., Teng, M. W., Smyth, M. J., and Thomas, D. M. (2014). Translational biology of osteosarcoma. Nat. Rev. Cancer 14 (11), 722–735. doi:10.1038/nrc3838
Kapustin, A. N., Davies, J. D., Reynolds, J. L., McNair, R., Jones, G. T., Sidibe, A., et al. (2011). Calcium regulates key components of vascular smooth muscle cell-derived matrix vesicles to enhance mineralization. Circ. Res. 109 (1), e1–e12. doi:10.1161/CIRCRESAHA.110.238808
Katz, J. N., Arant, K. R., and Loeser, R. F. (2021). Diagnosis and treatment of hip and knee osteoarthritis: A review. JAMA 325 (6), 568–578. doi:10.1001/jama.2020.22171
Kim, B.-Y., Yoon, H.-Y., Yun, S.-I., Woo, E.-R., Song, N.-K., Kim, H.-G., et al. (2011). In vitro and in vivo inhibition of glucocorticoid-induced osteoporosis by the hexane extract of Poncirus trifoliata. Phytotherapy Res. PTR 25 (7), 1000–1010. doi:10.1002/ptr.3373
Kirsch, T., Harrison, G., Golub, E. E., and Nah, H. D. (2000). The roles of annexins and types II and X collagen in matrix vesicle-mediated mineralization of growth plate cartilage. J. Biol. Chem. 275 (45), 35577–35583. doi:10.1074/jbc.M005648200
Korolkova, O. Y., Widatalla, S. E., Williams, S. D., Whalen, D. S., Beasley, H. K., Ochieng, J., et al. (2020). Diverse roles of annexin A6 in triple-negative breast cancer diagnosis, prognosis and EGFR-targeted therapies. Cells 9 (8), 1855. doi:10.3390/cells9081855
Kraler, S., Blaser, M. C., Aikawa, E., Camici, G. G., and Lüscher, T. F. (2022). Calcific aortic valve disease: from molecular and cellular mechanisms to medical therapy. Eur. Heart J. 43 (7), 683–697. doi:10.1093/eurheartj/ehab757
Kraus, V. B., Blanco, F. J., Englund, M., Karsdal, M. A., and Lohmander, L. S. (2015). Call for standardized definitions of osteoarthritis and risk stratification for clinical trials and clinical use. Osteoarthr. Cartil. 23 (8), 1233–1241. doi:10.1016/j.joca.2015.03.036
Leoni, G., Neumann, P.-A., Kamaly, N., Quiros, M., Nishio, H., Jones, H. R., et al. (2015). Annexin A1-containing extracellular vesicles and polymeric nanoparticles promote epithelial wound repair. J. Clin. Investigation 125 (3), 1215–1227. doi:10.1172/JCI76693
Li, F., Chung, H., Reddy, S. V., Lu, G., Kurihara, N., Zhao, A. Z., et al. (2005). Annexin II stimulates RANKL expression through MAPK. J. Bone Mineral Res. Official J. Am. Soc. Bone Mineral Res. 20 (7), 1161–1167. doi:10.1359/JBMR.050207
Li, X., Xia, Q., Mao, M., Zhou, H., Zheng, L., Wang, Y., et al. (2021). Annexin-A1 SUMOylation regulates microglial polarization after cerebral ischemia by modulating IKKα stability via selective autophagy. Sci. Adv. 7 (4), eabc5539. doi:10.1126/sciadv.abc5539
Li, T., Yu, H., Zhang, D., Feng, T., Miao, M., Li, J., et al. (2022). Matrix vesicles as a therapeutic target for vascular calcification. Front. Cell. Dev. Biol. 10, 825622. doi:10.3389/fcell.2022.825622
Liang, Y., Min, D., Fan, H., Liu, K., Tu, J., He, X., et al. (2023). Discovery of a first-in-class ANXA3 degrader for the treatment of triple-negative breast cancer. Acta Pharm. Sin. B 13 (4), 1686–1698. doi:10.1016/j.apsb.2022.11.023
Liao, Y. C., Fernandopulle, M. S., Wang, G., Choi, H., Hao, L., Drerup, C. M., et al. (2019). RNA granules hitchhike on lysosomes for long-distance transport, using annexin A11 as a molecular tether. Cell. 179 (1), 147–164. doi:10.1016/j.cell.2019.08.050
Libby, P. (2021). The changing landscape of atherosclerosis. Nature 592 (7855), 524–533. doi:10.1038/s41586-021-03392-8
Liberman, M., and Marti, L. C. (2017). Vascular calcification regulation by exosomes in the vascular wall. Adv. Exp. Med. Biol. 998, 151–160. doi:10.1007/978-981-10-4397-0_10
Lillebostad, P. A. G., Raasakka, A., Hjellbrekke, S. J., Patil, S., Røstbø, T., Hollås, H., et al. (2020). Structure of the ALS mutation target annexin A11 reveals a stabilising N-terminal segment. Biomolecules 10 (4), 660. doi:10.3390/biom10040660
Lin, L., and Hu, K. (2017). Tissue-type plasminogen activator modulates macrophage M2 to M1 phenotypic change through annexin A2-mediated NF-κB pathway. Oncotarget 8 (50), 88094–88103. doi:10.18632/oncotarget.21510
Lin, L., and Hu, K. (2022). Annexin A2 and kidney diseases. Front. Cell. Dev. Biol. 10, 974381. doi:10.3389/fcell.2022.974381
Lin, Q. S., Wang, W. X., Lin, Y. X., Lin, Z. Y., Yu, L. H., Kang, Y., et al. (2019). Annexin A7 induction of neuronal apoptosis via effect on glutamate release in a rat model of subarachnoid hemorrhage. J. Neurosurg. 132 (3), 777–787. doi:10.3171/2018.9.Jns182003
Liu, S., Li, X., Lin, Z., Su, L., Yan, S., Zhao, B., et al. (2018). SEC-induced activation of ANXA7 GTPase suppresses prostate cancer metastasis. Cancer Lett. 416, 11–23. doi:10.1016/j.canlet.2017.12.008
Liu, X., Yang, M., Guo, Y., and Lu, X. (2021). Annexin A10 is a novel prognostic biomarker of papillary thyroid cancer. Ir. J. Med. Sci. 190 (1), 59–65. doi:10.1007/s11845-020-02263-x
Lu, X., Hu, L., Mao, J., Zhang, S., Cai, Y., and Chen, W. (2023). Annexin A9 promotes cell proliferation by regulating the Wnt signaling pathway in colorectal cancer. Hum. Cell. 36, 1729–1740. doi:10.1007/s13577-023-00939-x
Lueck, K., Carr, A. F., Yu, L., Greenwood, J., and Moss, S. E. (2020). Annexin A8 regulates Wnt signaling to maintain the phenotypic plasticity of retinal pigment epithelial cells. Sci. Rep. 10 (1), 1256. doi:10.1038/s41598-020-58296-w
Ma, F., Li, X., Fang, H., Jin, Y., Sun, Q., and Li, X. (2020). Prognostic value of ANXA8 in gastric carcinoma. J. Cancer 11 (12), 3551–3558. doi:10.7150/jca.40010
Madureira, P. A., Surette, A. P., Phipps, K. D., Taboski, M. A., Miller, V. A., and Waisman, D. M. (2011). The role of the annexin A2 heterotetramer in vascular fibrinolysis. Blood 118 (18), 4789–4797. doi:10.1182/blood-2011-06-334672
Manke, M. C., Geue, S., Coman, C., Peng, B., Kollotzek, F., Münzer, P., et al. (2021). ANXA7 regulates platelet lipid metabolism and Ca(2+) release in arterial thrombosis. Circ. Res. 129 (4), 494–507. doi:10.1161/circresaha.121.319207
Maré, A. D., D'Haese, P. C., and Verhulst, A. (2020). The role of sclerostin in bone and ectopic calcification. Int. J. Mol. Sci. 21 (9), 3199. doi:10.3390/ijms21093199
Matsuda, R., Kaneko, N., and Horikawa, Y. (1997). Presence and comparison of Ca2+ transport activity of annexins I, II, V, and VI in large unilamellar vesicles. Biochem. Biophys. Res. Commun. 237 (3), 499–503. doi:10.1006/bbrc.1997.7177
McArthur, S., Juban, G., Gobbetti, T., Desgeorges, T., Theret, M., Gondin, J., et al. (2020). Annexin A1 drives macrophage skewing to accelerate muscle regeneration through AMPK activation. J. Clin. Investigation 130 (3), 1156–1167. doi:10.1172/jci124635
McCulloch, K. M., Yamakawa, I., Shifrin, D. A., McConnell, R. E., Foegeding, N. J., Singh, P. K., et al. (2019). An alternative N-terminal fold of the intestine-specific annexin A13a induces dimerization and regulates membrane-binding. J. Biol. Chem. 294 (10), 3454–3463. doi:10.1074/jbc.RA118.004571
Meng, H., Zhang, Y., An, S. T., and Chen, Y. (2019). Annexin A3 gene silencing promotes myocardial cell repair through activation of the PI3K/Akt signaling pathway in rats with acute myocardial infarction. J. Cell. Physiol. 234 (7), 10535–10546. doi:10.1002/jcp.27717
Meng, K., Lu, S., Yan, X., Sun, Y., Gao, J., Wang, Y., et al. (2020). Quantitative mitochondrial proteomics reveals ANXA7 as a crucial factor in mitophagy. J. Proteome Res. 19 (3), 1275–1284. doi:10.1021/acs.jproteome.9b00800
Middel, V., Zhou, L., Takamiya, M., Beil, T., Shahid, M., Roostalu, U., et al. (2016). Dysferlin-mediated phosphatidylserine sorting engages macrophages in sarcolemma repair. Nat. Commun. 7, 12875. doi:10.1038/ncomms12875
Minashima, T., and Kirsch, T. (2018). Annexin A6 regulates catabolic events in articular chondrocytes via the modulation of NF-κB and Wnt/ß-catenin signaling. PLoS One 13 (5), e0197690. doi:10.1371/journal.pone.0197690
Minashima, T., Small, W., Moss, S. E., and Kirsch, T. (2012). Intracellular modulation of signaling pathways by annexin A6 regulates terminal differentiation of chondrocytes. J. Biol. Chem. 287 (18), 14803–14815. doi:10.1074/jbc.M111.297861
Minashima, T., Campbell, K., and Kirsch, T. (2013). Annexins: novel therapeutic targets for the treatment of osteoarthritis? J. Am. Acad. Orthop. Surg. 21 (4), 256–257. doi:10.5435/JAAOS-21-04-256
Montaville, P., Neumann, J.-M., Russo-Marie, F., Ochsenbein, F., and Sanson, A. (2002). A new consensus sequence for phosphatidylserine recognition by annexins. J. Biol. Chem. 277 (27), 24684–24693. doi:10.1074/jbc.M109595200
Mookhtiar, K. A., and Van Wart, H. E. (1992). Clostridium histolyticum collagenases: A new look at some old enzymes. Matrix (Stuttgart, Ger. 1, 116–126. Retrieved from https://pubmed.ncbi.nlm.nih.gov/1336107.
Moss, S. E., and Morgan, R. O. (2004). The annexins. Genome Biol. 5 (4), 219. doi:10.1186/gb-2004-5-4-219
Mroczek, J., Pikula, S., Suski, S., Weremiejczyk, L., Biesaga, M., and Strzelecka-Kiliszek, A. (2022). Apigenin modulates AnxA6-and TNAP-mediated osteoblast mineralization. Int. J. Mol. Sci. 23 (21), 13179. doi:10.3390/ijms232113179
Murshed, M. (2018). Mechanism of bone mineralization. Cold Spring Harb. Perspect. Med. 8 (12), a031229. doi:10.1101/cshperspect.a031229
Naciff, J. M., Behbehani, M. M., Kaetzel, M. A., and Dedman, J. R. (1996). Annexin VI modulates Ca2+ and K+ conductances of spinal cord and dorsal root ganglion neurons. Am. J. Physiol. 271 (6), C2004–C2015. doi:10.1152/ajpcell.1996.271.6.C2004
Nahm, M., Lim, S. M., Kim, Y. E., Park, J., Noh, M. Y., Lee, S., et al. (2020). ANXA11 mutations in ALS cause dysregulation of calcium homeostasis and stress granule dynamics. Sci. Transl. Med. 12 (566), eaax3993. doi:10.1126/scitranslmed.aax3993
Nakayama, M., Miyagawa, H., Kuranami, Y., Tsunooka-Ota, M., Yamaguchi, Y., and Kojima-Aikawa, K. (2020). Annexin A4 inhibits sulfatide-induced activation of coagulation factor XII. J. Thromb. Haemost. 18 (6), 1357–1369. doi:10.1111/jth.14789
New, S. E. P., Goettsch, C., Aikawa, M., Marchini, J. F., Shibasaki, M., Yabusaki, K., et al. (2013). Macrophage-derived matrix vesicles: an alternative novel mechanism for microcalcification in atherosclerotic plaques. Circulation Res. 113 (1), 72–77. doi:10.1161/CIRCRESAHA.113.301036
Nguyen, M. K. L., Jose, J., Wahba, M., Bernaus-Esqué, M., Hoy, A. J., Enrich, C., et al. (2022). Linking late endosomal cholesterol with cancer progression and anticancer drug resistance. Int. J. Mol. Sci. 23 (13), 7206. doi:10.3390/ijms23137206
Niu, Y., Yang, X., Chen, Y., Jin, X., Xie, Y., Tang, Y., et al. (2019). Distinct prognostic values of Annexin family members expression in acute myeloid leukemia. Clin. Transl. Oncol. 21 (9), 1186–1196. Official Publication of the Federation of Spanish Oncology Societies and of the National Cancer Institute of Mexico. doi:10.1007/s12094-019-02045-7
Onuora, S. (2022). Annexin A1 could help prevent periprosthetic bone loss. Nat. Rev. Rheumatol. 18 (9), 494. doi:10.1038/s41584-022-00822-3
Ormesher, L., and Greer, I. A. (2016). ANXA5: A key to unlock the mystery of the spectrum of placental-mediated pregnancy complications? Women's Health (London, Engl. 12 (2), 159–161. doi:10.2217/whe-2015-0003
Pei, T., Su, G., Yang, J., Gao, W., Yang, X., Zhang, Y., et al. (2022). Fluid shear stress regulates osteogenic differentiation via AnnexinA6-mediated autophagy in mc3t3-E1 cells. Int. J. Mol. Sci. 23 (24), 15702. doi:10.3390/ijms232415702
Peng, B., Guo, C., Guan, H., Liu, S., and Sun, M.-Z. (2014). Annexin A5 as a potential marker in tumors. Clin. Chimica Acta; Int. J. Clin. Chem. 427, 42–48. doi:10.1016/j.cca.2013.09.048
Peng, X., Su, S., Zeng, J., Xie, K., Yang, X., Xian, G., et al. (2022). 4-Octyl itaconate suppresses the osteogenic response in aortic valvular interstitial cells via the Nrf2 pathway and alleviates aortic stenosis in mice with direct wire injury. Free Radic. Biol. Med. 188, 404–418. doi:10.1016/j.freeradbiomed.2022.06.246
Perretti, M., and D'Acquisto, F. (2009). Annexin A1 and glucocorticoids as effectors of the resolution of inflammation. Nat. Rev. Immunol. 9 (1), 62–70. doi:10.1038/nri2470
Pfander, D., Swoboda, B., and Kirsch, T. (2001). Expression of early and late differentiation markers (proliferating cell nuclear antigen, syndecan-3, annexin VI, and alkaline phosphatase) by human osteoarthritic chondrocytes. Am. J. Pathology 159 (5), 1777–1783. doi:10.1016/S0002-9440(10)63024-6
Pletcher, M. J., Tice, J. A., Pignone, M., and Browner, W. S. (2004). Using the coronary artery calcium score to predict coronary heart disease events: A systematic review and meta-analysis. Archives Intern. Med. 164 (12), 1285–1292. doi:10.1001/archinte.164.12.1285
Qi, H., Liu, S., Guo, C., Wang, J., Greenaway, F. T., and Sun, M. Z. (2015). Role of annexin A6 in cancer. Oncol. Lett. 10 (4), 1947–1952. doi:10.3892/ol.2015.3498
Reiss, A. B., Miyawaki, N., Moon, J., Kasselman, L. J., Voloshyna, I., D'Avino, R., et al. (2018). CKD, arterial calcification, atherosclerosis and bone health: inter-relationships and controversies. Atherosclerosis 278, 49–59. doi:10.1016/j.atherosclerosis.2018.08.046
Rentero, C., Blanco-Muñoz, P., Meneses-Salas, E., Grewal, T., and Enrich, C. (2018). Annexins-coordinators of cholesterol homeostasis in endocytic pathways. Int. J. Mol. Sci. 19 (5), 1444. doi:10.3390/ijms19051444
Reynolds, J. L., Joannides, A. J., Skepper, J. N., McNair, R., Schurgers, L. J., Proudfoot, D., et al. (2004). Human vascular smooth muscle cells undergo vesicle-mediated calcification in response to changes in extracellular calcium and phosphate concentrations: A potential mechanism for accelerated vascular calcification in ESRD. J. Am. Soc. Nephrol. JASN 15 (11), 2857–2867. doi:10.1097/01.ASN.0000141960.01035.28
Rogers, M. A., Buffolo, F., Schlotter, F., Atkins, S. K., Lee, L. H., Halu, A., et al. (2020). Annexin A1-dependent tethering promotes extracellular vesicle aggregation revealed with single-extracellular vesicle analysis. Sci. Adv. 6 (38), eabb1244. doi:10.1126/sciadv.abb1244
Rubio, R., Abarrategi, A., Garcia-Castro, J., Martinez-Cruzado, L., Suarez, C., Tornin, J., et al. (2014). Bone environment is essential for osteosarcoma development from transformed mesenchymal stem cells. Stem Cells Dayt. Ohio) 32 (5), 1136–1148. doi:10.1002/stem.1647
Salom, C., Álvarez-Teijeiro, S., Fernández, M. P., Morgan, R. O., Allonca, E., Vallina, A., et al. (2019). Frequent alteration of annexin A9 and A10 in HPV-negative head and neck squamous cell carcinomas: correlation with the histopathological differentiation grade. J. Clin. Med. 8 (2), 229. doi:10.3390/jcm8020229
Sauer, G. R., and Wuthier, R. E. (1988). Fourier transform infrared characterization of mineral phases formed during induction of mineralization by collagenase-released matrix vesicles in vitro. J. Biol. Chem. 263 (27), 13718–13724. doi:10.1016/s0021-9258(18)68300-0
Schloer, S., Pajonczyk, D., and Rescher, U. (2018). Annexins in translational research: hidden treasures to Be found. Int. J. Mol. Sci. 19 (6), 1781. doi:10.3390/ijms19061781
Shanahan, C. M., Crouthamel, M. H., Kapustin, A., and Giachelli, C. M. (2011). Arterial calcification in chronic kidney disease: key roles for calcium and phosphate. Circulation Res. 109 (6), 697–711. doi:10.1161/CIRCRESAHA.110.234914
Shao, Y. Y., Kuo, H. Y., Jeng, Y. M., Wu, Y. M., Wang, H. P., Hsu, C., et al. (2022). Association of annexin A10 expression with poor prognosis of intrahepatic cholangiocarcinoma. BMC Cancer 22 (1), 219. doi:10.1186/s12885-022-09288-8
Sherwood, J. (2019). Osteoarthritis year in review 2018: biology. Osteoarthr. Cartil. 27 (3), 365–370. doi:10.1016/j.joca.2018.10.005
Shimada, A., Ideno, H., Arai, Y., Komatsu, K., Wada, S., Yamashita, T., et al. (2018). Annexin A5 involvement in bone overgrowth at the enthesis. J. Bone Mineral Res. Official J. Am. Soc. Bone Mineral Res. 33 (8), 1532–1543. doi:10.1002/jbmr.3453
Shoaib, Z., Fan, T. M., and Irudayaraj, J. M. K. (2022). Osteosarcoma mechanobiology and therapeutic targets. Br. J. Pharmacol. 179 (2), 201–217. doi:10.1111/bph.15713
Smith, D. L., Evans, C. A., Pierce, A., Gaskell, S. J., and Whetton, A. D. (2002). Changes in the proteome associated with the action of Bcr-Abl tyrosine kinase are not related to transcriptional regulation. Mol. Cell. Proteomics MCP 1 (11), 876–884. doi:10.1074/mcp.m200035-mcp200
Smith, B. N., Topp, S. D., Fallini, C., Shibata, H., Chen, H. J., Troakes, C., et al. (2017). Mutations in the vesicular trafficking protein annexin A11 are associated with amyotrophic lateral sclerosis. Sci. Transl. Med. 9 (388), eaad9157. doi:10.1126/scitranslmed.aad9157
Solito, E., McArthur, S., Christian, H., Gavins, F., Buckingham, J. C., and Gillies, G. E. (2008). Annexin A1 in the brain--undiscovered roles? Trends Pharmacol. Sci. 29 (3), 135–142. doi:10.1016/j.tips.2007.12.003
Stogbauer, F., Weigert, J., Neumeier, M., Wanninger, J., Sporrer, D., Weber, M., et al. (2009). Annexin A6 is highly abundant in monocytes of obese and type 2 diabetic individuals and is downregulated by adiponectin in vitro. Exp. Mol. Med. 41 (7), 501–507. doi:10.3858/emm.2009.41.7.055
Strzelecka-Kiliszek, A., Buszewska, M. E., Podszywalow-Bartnicka, P., Pikula, S., Otulak, K., Buchet, R., et al. (2008). Calcium- and pH-dependent localization of annexin A6 isoforms in Balb/3T3 fibroblasts reflecting their potential participation in vesicular transport. J. Cell. Biochem. 104 (2), 418–434. doi:10.1002/jcb.21632
Sun, L., Xu, Q., Zhang, W., Jiao, C., Wu, H., and Chen, X. (2019). The involvement of spinal annexin A10/NF-κB/MMP-9 pathway in the development of neuropathic pain in rats. BMC Neurosci. 20 (1), 28. doi:10.1186/s12868-019-0513-9
Sun, X., Shu, Y., Xu, M., Jiang, J., Wang, L., Wang, J., et al. (2020). ANXA6 suppresses the tumorigenesis of cervical cancer through autophagy induction. Clin. Transl. Med. 10 (6), e208. doi:10.1002/ctm2.208
Swaggart, K. A., Demonbreun, A. R., Vo, A. H., Swanson, K. E., Kim, E. Y., Fahrenbach, J. P., et al. (2014). Annexin A6 modifies muscular dystrophy by mediating sarcolemmal repair. Proc. Natl. Acad. Sci. U. S. A. 111 (16), 6004–6009. doi:10.1073/pnas.1324242111
Tan, S. H., Young, D., Chen, Y., Kuo, H. C., Srinivasan, A., Dobi, A., et al. (2021). Prognostic features of Annexin A2 expression in prostate cancer. Pathology 53 (2), 205–213. doi:10.1016/j.pathol.2020.07.006
Thouverey, C., Strzelecka-Kiliszek, A., Balcerzak, M., Buchet, R., and Pikula, S. (2009). Matrix vesicles originate from apical membrane microvilli of mineralizing osteoblast-like Saos-2 cells. J. Cell. Biochem. 106 (1), 127–138. doi:10.1002/jcb.21992
Tong, M., Che, N., Zhou, L., Luk, S. T., Kau, P. W., Chai, S., et al. (2018). Efficacy of annexin A3 blockade in sensitizing hepatocellular carcinoma to sorafenib and regorafenib. J. Hepatol. 69 (4), 826–839. doi:10.1016/j.jhep.2018.05.034
Trilla-Fuertes, L., Gámez-Pozo, A., Prado-Vázquez, G., Zapater-Moros, A., Díaz-Almirón, M., Fortes, C., et al. (2019). Melanoma proteomics suggests functional differences related to mutational status. Sci. Rep. 9 (1), 7217. doi:10.1038/s41598-019-43512-z
Veschi, E. A., Bolean, M., Strzelecka-Kiliszek, A., Bandorowicz-Pikula, J., Pikula, S., Granjon, T., et al. (2020). Localization of annexin A6 in matrix vesicles during physiological mineralization. Int. J. Mol. Sci. 21 (4), 1367. doi:10.3390/ijms21041367
Veschi, E. A., Bolean, M., da Silva Andrilli, L. H., Sebinelli, H. G., Strzelecka-Kiliszek, A., Bandorowicz-Pikula, J., et al. (2022). Mineralization profile of annexin A6-harbouring proteoliposomes: shedding light on the role of annexin A6 on matrix vesicle-mediated mineralization. Int. J. Mol. Sci. 23 (16), 8945. doi:10.3390/ijms23168945
Vicic, N., Guo, X., Chan, D., Flanagan, J. G., Sigal, I. A., and Sivak, J. M. (2022). Evidence of an Annexin A4 mediated plasma membrane repair response to biomechanical strain associated with glaucoma pathogenesis. J. Cell. Physiol. 237 (9), 3687–3702. doi:10.1002/jcp.30834
Wan, Y. C. E., Liu, J., Zhu, L., Kang, T. Z. E., Zhu, X., Lis, J., et al. (2020). The H2BG53D oncohistone directly upregulates ANXA3 transcription and enhances cell migration in pancreatic ductal adenocarcinoma. Signal Transduct. Target Ther. 5 (1), 106. doi:10.1038/s41392-020-00219-2
Wang, W., and Kirsch, T. (2002). Retinoic acid stimulates annexin-mediated growth plate chondrocyte mineralization. J. Cell. Biol. 157 (6), 1061–1069. doi:10.1083/jcb.200203014
Wang, K., Zhang, T., Lei, Y., Li, X., Jiang, J., Lan, J., et al. (2018). Identification of ANXA2 (annexin A2) as a specific bleomycin target to induce pulmonary fibrosis by impeding TFEB-mediated autophagic flux. Autophagy 14 (2), 269–282. doi:10.1080/15548627.2017.1409405
Wang, Y., Wang, C., Yang, Q., and Cheng, Y. L. (2019). ANXA3 silencing ameliorates intracranial aneurysm via inhibition of the JNK signaling pathway. Mol. Ther. Nucleic Acids 17, 540–550. doi:10.1016/j.omtn.2019.06.005
Wang, H., Chen, F., Hu, A., Liang, H., Liang, Y., Seetharamu, N., et al. (2023). Harmine loaded Au@MSNs@PEG@Asp6 nano-composites for treatment of spinal metastasis from lung adenocarcinoma by targeting ANXA9 in vivo experiment. Transl. Lung Cancer Res. 12 (5), 1062–1077. doi:10.21037/tlcr-23-191
Wei, T., and Zhu, X. (2021). Knockdown of ANXA10 inhibits proliferation and promotes apoptosis of papillary thyroid carcinoma cells by down-regulating TSG101 thereby inactivating the MAPK/ERK signaling pathway. J. Bioenerg. Biomembr. 53 (4), 429–440. doi:10.1007/s10863-021-09902-7
Wei, B., Guo, C., Liu, S., and Sun, M. Z. (2015). Annexin A4 and cancer. Clin. Chim. Acta 447, 72–78. doi:10.1016/j.cca.2015.05.016
Woodward, A., Faria, G. N. F., and Harrison, R. G. (2022). Annexin A5 as a targeting agent for cancer treatment. Cancer Lett. 547, 215857. doi:10.1016/j.canlet.2022.215857
Wu, L. N., Yoshimori, T., Genge, B. R., Sauer, G. R., Kirsch, T., Ishikawa, Y., et al. (1993). Characterization of the nucleational core complex responsible for mineral induction by growth plate cartilage matrix vesicles. J. Biol. Chem. 268 (33), 25084–25094. doi:10.1016/s0021-9258(19)74574-8
Wu, L. N., Genge, B. R., Dunkelberger, D. G., LeGeros, R. Z., Concannon, B., and Wuthier, R. E. (1997). Physicochemical characterization of the nucleational core of matrix vesicles. J. Biol. Chem. 272 (7), 4404–4411. doi:10.1074/jbc.272.7.4404
Wu, B., Wang, Y., Xiao, F., Butcher, J. T., Yutzey, K. E., and Zhou, B. (2017). Developmental mechanisms of aortic valve malformation and disease. Annu. Rev. Physiol. 79, 21–41. doi:10.1146/annurev-physiol-022516-034001
Wu, L., Liu, C., Chang, D. Y., Zhan, R., Sun, J., Cui, S. H., et al. (2021). Annexin A1 alleviates kidney injury by promoting the resolution of inflammation in diabetic nephropathy. Kidney Int. 100 (1), 107–121. doi:10.1016/j.kint.2021.02.025
Wuthier, R. E., and Lipscomb, G. F. (2011). Matrix vesicles: structure, composition, formation and function in calcification. Front. Biosci. (Landmark Ed. 16, 2812–2902. doi:10.2741/3887
Xu, Y., Sui, L., Qiu, B., Yin, X., Liu, J., and Zhang, X. (2019). ANXA4 promotes trophoblast invasion via the PI3K/Akt/eNOS pathway in preeclampsia. Am. J. Physiol. Cell. Physiol. 316 (4), C481–C491. doi:10.1152/ajpcell.00404.2018
Xu, X., Gao, W., Li, L., Hao, J., Yang, B., Wang, T., et al. (2021). Annexin A1 protects against cerebral ischemia-reperfusion injury by modulating microglia/macrophage polarization via FPR2/ALX-dependent AMPK-mTOR pathway. J. Neuroinflammation 18 (1), 119. doi:10.1186/s12974-021-02174-3
Xue, G. L., Zhang, C., Zheng, G. L., Zhang, L. J., and Bi, J. W. (2020). Annexin A13 predicts poor prognosis for lung adenocarcinoma patients and accelerates the proliferation and migration of lung adenocarcinoma cells by modulating epithelial-mesenchymal transition. Fundam. Clin. Pharmacol. 34 (6), 687–696. doi:10.1111/fcp.12555
Yan, K. X., Meng, Q., He, H., Zhu, H. W., Wang, Z. C., Han, L., et al. (2022). iTRAQ-based quantitative proteomics reveals biomarkers/pathways in psoriasis that can predict the efficacy of methotrexate. J. Eur. Acad. Dermatology Venereol. JEADV 36 (10), 1784–1795. doi:10.1111/jdv.18292
Yang, J., Han, Q., Li, C., Yang, H., Chen, X., and Wang, X. (2020). Circular RNA circ_0001105 inhibits progression and metastasis of osteosarcoma by sponging miR-766 and activating YTHDF2 expression. Onco Targets Ther. 13, 1723–1736. doi:10.2147/ott.S234668
Yang, L., Lu, P., Yang, X., Li, K., and Qu, S. (2021). Annexin A3, a calcium-dependent phospholipid-binding protein: implication in cancer. Front. Mol. Biosci. 8, 716415. doi:10.3389/fmolb.2021.716415
Yang, F., Liu, S., Gu, Y., Yan, Y., Ding, X., Zou, L., et al. (2022). MicroRNA-22 promoted osteogenic differentiation of valvular interstitial cells by inhibiting CAB39 expression during aortic valve calcification. Cell. Mol. Life Sci. CMLS 79 (3), 146. doi:10.1007/s00018-022-04177-6
Zhang, Z., Deng, M., Huang, J., Wu, J., Li, Z., Xing, M., et al. (2020). Microglial annexin A3 downregulation alleviates bone cancer-induced pain through inhibiting the Hif-1α/vascular endothelial growth factor signaling pathway. Pain 161 (12), 2750–2762. doi:10.1097/j.pain.0000000000001962
Zhang, Y., Wang, F., and Yu, Y. (2023). LncRNA HOXD-AS1 promotes oral squamous cell carcinoma by sponging miR-203a-5p. Oral Dis. 29 (4), 1505–1512. doi:10.1111/odi.14152
Zhao, R.-R., Mao, X.-R., Wang, X.-F., Zheng, Y., Wang, Y.-P., and Zhou, Y.-N. (2022). Role of annexin A family in tumorigenesis and chemoresistance of gastric cancer. Neoplasma 69 (2), 251–263. doi:10.4149/neo_2021_210629N872
Zhong, G., Su, S., Li, J., Zhao, H., Hu, D., Chen, J., et al. (2023). Activation of Piezo1 promotes osteogenic differentiation of aortic valve interstitial cell through YAP-dependent glutaminolysis. Sci. Adv. 9 (22), eadg0478. doi:10.1126/sciadv.adg0478
Keywords: AnnexinA6, Ca2+ regulation, matrix vesicles, extracellular matrix mineralization, osteogenesis, bone regeneration
Citation: Yang J, Pei T, Su G, Duan P and Liu X (2023) AnnexinA6: a potential therapeutic target gene for extracellular matrix mineralization. Front. Cell Dev. Biol. 11:1201200. doi: 10.3389/fcell.2023.1201200
Received: 06 April 2023; Accepted: 10 August 2023;
Published: 04 September 2023.
Edited by:
Hinrich Peter Hansen, University of Cologne, GermanyReviewed by:
Ling Lin, The Pennsylvania State University, United StatesAlexander N. Kapustin, AstraZeneca, United Kingdom
Copyright © 2023 Yang, Pei, Su, Duan and Liu. This is an open-access article distributed under the terms of the Creative Commons Attribution License (CC BY). The use, distribution or reproduction in other forums is permitted, provided the original author(s) and the copyright owner(s) are credited and that the original publication in this journal is cited, in accordance with accepted academic practice. No use, distribution or reproduction is permitted which does not comply with these terms.
*Correspondence: Xiaoheng Liu, bGl1eGlhb2hnQHNjdS5lZHUuY24=
†These authors have contributed equally to this work