- Department of Life Science and Technology, Tokyo Institute of Technology, Yokohama, Kanagawa, Japan
Anterior and posterior paired appendages of vertebrates are notable examples of heterochrony in the relative timing of their development. In teleosts, posterior paired appendages (pelvic fin buds) emerge much later than their anterior paired appendages (pectoral fin buds). Pelvic fin buds of zebrafish (Danio rerio) appear at 3 weeks post-fertilization (wpf) during the larva-to-juvenile transition (metamorphosis), whereas pectoral fin buds arise from the lateral plate mesoderm on the yolk surface at the embryonic stage. Here we explored the mechanism by which presumptive pelvic fin cells maintain their fate, which is determined at the embryonic stage, until the onset of metamorphosis. Expression analysis revealed that transcripts of pitx1, one of the key factors for the development of posterior paired appendages, became briefly detectable in the posterior lateral plate mesoderm at early embryonic stages. Further analysis indicated that the pelvic fin–specific pitx1 enhancer was in the poised state at the larval stage and is activated at the juvenile stage. We discuss the implications of these findings for the heterochronic development of pelvic fin buds.
Introduction
Heterochrony is defined as changes in the timing or rate of developmental processes (Alberch et al., 1979). Anterior and posterior paired appendages (forelimbs/pectoral fins and hindlimbs/pelvic fins) of vertebrates have been well studied as examples of heterochrony with respect to the relative timing of their development (Richardson et al., 2009). Forelimb buds develop earlier than hindlimb buds in most mammals, and this difference is amplified in marsupials, such as the brushtail possum (Trichosurus vulpecula), tammar wallaby (Macropus eugenii) and marsupial cat (Dasyurus viverrinus) (Bininda-Emonds et al., 2007; Richardson et al., 2009; Chew et al., 2014). In contrast, hindlimb buds develop earlier than forelimb buds in African clawed frogs (Xenopus laevis) and coqui frogs (Eleutherodactylus coqui) (Richardson et al., 2009). In teleost fishes, pelvic fin buds appear much later than pectoral fin buds. For instance, in zebrafish (D. rerio), pelvic fin buds emerge at 3 wpf during the larva-to-juvenile transition (metamorphosis), whereas pectoral fin buds arise from the lateral plate mesoderm on the yolk surface by 23 hpf (Kimmel et al., 1995; Grandel and Schulte-Merker, 1998).
Although pelvic fin buds of zebrafish appear at 3 wpf, fate mapping studies have shown that future pelvic fin bud cells are already determined by 16 hpf during early embryogenesis (Murata et al., 2010). The positional relationship between prospective pelvic fin cells on the yolk surface and the body trunk is shifted after trunk protrusion from the yolk sphere, at least in zebrafish, medaka and Nile tilapia (Murata et al., 2010; Kaneko et al., 2014), and such an allometric growth of the trunk may underlie the variation in pelvic fin position seen among teleost fishes (Tanaka, 2011). However, it remains uncertain how prospective pelvic fin cells maintain their fate for several weeks until the larva-to-juvenile transition.
In the hindlimb-forming region of chick and mouse embryos, Pitx1, which encodes a paired-like homeodomain protein, is expressed and plays critical roles in hindlimb skeletal morphogenesis (Lanctot et al., 1999; Szeto et al., 1999; Marcil et al., 2003; Duboc and Logan, 2011). Pitx1 acts in concert with the T-box transcription factor Tbx4 to control the outgrowth of hindlimb buds (Logan and Tabin, 1999; Duboc and Logan, 2011). In zebrafish and stickleback (Gasterosteus aculeatus), transcripts of pitx1 and tbx4 were detected specifically in pelvic fin buds during the larva-to-juvenile transition (Tamura et al., 1999; Cole et al., 2003; Don et al., 2016). Furthermore, stickleback that lack the pelvic fin–specific pitx1 enhancer fail to form pelvic fins (Shapiro et al., 2004; Chan et al., 2010), and zebrafish deficient for tbx4 function lack pelvic fins (Don et al., 2016; Lin et al., 2016), indicating the critical roles of both genes in the development of pelvic fins. Although the fate of the presumptive pelvic fin cells are determined during early embryogenesis (Murata et al., 2010), expression of neither pitx1 nor tbx4 has been reported in the presumptive pelvic fin field prior to the larva-to-juvenile transition period in any teleost fishes.
Pelvic fin buds emerge during the larva-to-juvenile transition (metamorphosis) in teleost fishes (Kimmel et al., 1995; Grandel and Schulte-Merker, 1998; Murata et al., 2010; Kaneko et al., 2014; Okamoto et al., 2018), and thyroid hormones (THs) are suggested to be the major factors promoting this transition among teleosts (Campinho, 2019). THs, such as thyroxine (T4) and triiodothyronine (T3), are secreted from the thyroid gland, and T4 can be converted to the more biologically active T3 (Yen et al., 2006; Koibuchi, 2009; Cheng et al., 2010). Treatment of larvae with exogenous T3 and/or T4 alters the juvenile pigmentation patterns in several teleosts (Parichy and Turner, 2003; McMenamin et al., 2014). In contrast, ablation of the thyroid partially blocks the juvenile transition in zebrafish (McMenamin et al., 2014). Based on these and other results, THs are proposed to play pivotal roles in triggering the larva-to-juvenile transition (Miwa et al., 1992; Power et al., 2001; Campinho, 2019); however, treatment of zebrafish larvae with THs alone has not been shown to accelerate the timing of initiation of pelvic fin development (Brown, 1997).
In this study, we explored the mechanisms by which presumptive pelvic fin cells maintain their cell fate, which is determined at early embryonic stages, until the larva-to-juvenile transition. We show that expression of pitx1, which plays pivotal roles in the initiation of the posterior paired appendages, briefly appears in the posterior lateral plate mesoderm including the presumptive pelvic fin cells on the yolk during the embryonic stage, but it soon became undetectable until the onset of metamorphosis. Further analysis indicated that the pelvic fin–specific pitx1 enhancer is in a poised state at the larval stage and is activated at the juvenile stage. We discuss the implications of these findings for the heterochronic development of pelvic fin buds.
Materials and methods
Zebrafish embryos and larvae
Wild-type zebrafish (Danio rerio; strain TL) were reared and staged as described (Kimmel et al., 1995).
Whole-mount in situ hybridization
Whole-mount in situ hybridization was performed as described (Westerfield, 2000). D. rerio pitx1 (877 bp) and tbx4 (653 bp) were amplified by PCR using the following primers, which hybridized to the indicated published sequence: pitx1 (ENSDARG00000042785), 5′-GACGTCTATCCCACCTACAC-3′ and 5′-TGGAAACAACATTAGCACTG-3'; tbx4 (NM_130914), 5′-TATTTGCCTCTGAGACGAAC-3′ and 5′-ACATAGAGTCTTCCAGGCAT-3'.
Hybridization chain reaction (HCR)
HCR probes were targeted D. rerio pitx1 (NM_001040346) and tal1 (NM_213237) and purchased commercially from Molecular Instruments, Inc. HCR assays were conducted according to (Ibarra-Garcia-Padilla et al., 2021). Images were obtained using an LSM 780 confocal microscope (Zeiss).
Alignment of sequences
The sequence from the pitx1 region of stickleback (Gasterosteus aculeatus) (BROAD S1 assembly), which includes a previously reported pelvic fin–specific enhancer sequence (Chan et al., 2010), was aligned against that of D. rerio (GRCz10 assembly), the medaka Oryzias latipes (MEDAKA1 assembly), the tetraodon Tetraodon pustulatus (TETRAODON 8.0 assembly), the cod Gadus morhua (gadMor1 assembly) and the fugu Takifugu rubripes (FUGU 4.0 assembly). These aligned sequences were visualized using mVISTA Genome Browser (http://genome.lbl.gov/vista/mvista/submit.shtml). Binding sites of transcription factors were predicted by MATCH (http://gene-regulation.com).
Plasmid constructs
The zebrafish counterpart of the pelvic fin–specific pitx1 enhancer (the Pel enhancer in Chan et al., 2010, later termed the PelA enhancer in Thompson et al., 2018) was amplified by PCR with the specific primers 5′-CTTGATATCGAATTCCTATGCCTGCTTGTGTCTTTG-3′ and 5′- CGGGCTGCAGGAATTCCTCTGATTATCAACAGCAGG-3′. The amplified fragment was then cloned into the EcoRI sites of the pBSSK–vector (pBSSK-DrPel) via the In-Fusion reaction (Clontech). pT2AL200R150G (Urasaki et al., 2006) was digested with SalI, blunt-ended using T4 DNA polymerase and ligated (pT2AL200R150G-without SalI). Then, the basal promoter of EF1α was amplified with specific primes 5′-CCCAAGCTTCTAGAACTCGCCGCAGACCC-3′ and 5′-CCGCTCGAGACGCGTCGACCATGCAAGCTAGCTTATGACGC-3′ using pT2AL200R150G as the template. This amplified fragment was then cloned into the XhoI and HindIII sites of pT2AL200R150G-without SalI (pT2AL-EF1αmin-EGFP). pBSSK-DrPel was digested with NotI and blunt-ended using T4 DNA polymerase and then was digested with XhoI and cloned into the SmaI site of pT2AL-EF1αmin-EGFP (pT2AL-DrPel-EF1αmin-EGFP). Then, pBSSK-DrPel was digested with EcoRI and SmaI and cloned into the SmaI site of pT2AL-DrPel-EF1αmin-EGF (pT2AL-DrPel × 2-EF1αmin-EGFP).
Microinjection
For enhancer analysis, the plasmids of interest were co-injected with mRNA that encodes Tol2 transposase (Kawakami, 2004) into one-cell-stage embryos. Injected embryos were raised to adulthood and screened for germline transmission. Founders that expressed enhanced green fluorescent protein (EGFP) were crossed with TL fish, and the resulting embryos were screened for EGFP expression in the pelvic fin.
Chromatin immunoprecipitation and quantitative real-time PCR (ChIP-qPCR)
ChIP was performed as described (Visel et al., 2009; Nakato et al., 2013; Suda et al., 2014) with the following modifications. The presumptive pelvic fin field from ∼200 larvae at 2 wpf [total length (TL), 5.5–7.0 mm; Supplementary Figure S1A] and the early pelvic fin from ∼100 juveniles at 3–4 wpf (TL, 9.0–12.0 mm; Supplementary Figure S1B) were dissected (green areas shown in Figure 2C). These tissues were fixed in 1% formaldehyde (for H3K27ac and H3K27me3) or in 1% formaldehyde with 2 mM N,N′-disuccinimidyl glutarate (for CBP) for 10 min at room temperature, washed with phosphate-buffered saline (PBS) and stored at −80°C. ChIP was performed using these tissue samples with antibodies against H3K27ac (#ab4729, Abcam), H3K27me3 (#ab39536, Abcam) or CBP (GTX101249, GeneTex). Samples were homogenized in lysis buffer 1 [20 mM Tris-HCl (pH 7.5), 10 mM NaCl, 2.5 mM MgCl2, 0.2% nonyl phenoxypolyethoxylethanol 40, 1% protease inhibitor, 1% phenylmethanesulfonyl fluoride (PMSF)]. After centrifugation, pellets were suspended in the zebrafish lysis buffer [50 mM Tris-HCl (pH 8.0), 10 mM EDTA, 1% SDS, 1% protease inhibitor, 1% PMSF]. Samples from the whole-cell extract and ChIP fractions were sheared with an ultra sonicator (10 × 10 s and 30% amplitude; BRANSON SONIFIRE 250D). Sequences were amplified with the following primers: pitx1 enhancer (Chr21: 47,921,810–47,921,997), 5′-TTGTCACACCTTTACTTCGGA-3′ and 5′-AACGGAGAGCGTCTGTTGG-3′; positive control, β-actin (actb) promoter (Chr3: 40,386,829–40,386,986) (Lindeman et al., 2010), 5′-CAGTCGGTTCAGTTCATAGT-3′ and 5′-GGTTGTGTAATTGATCGCAG-3′; negative control, open reading frame (ORF)-free pitx1 enhancer intergenic region (IGR) (Chr21: 45,736,667–45,736,860), 5′-GTGGGTGGTGATTTCGTGTC-3′ and 5′- GCCTTTGTTGCTCTCTGTCA-3′. The mean ± standard deviation (s.d.) was calculated, and a statistical analysis was performed using Student’s t-test.
Treatment with hormones
For making stock solution, 10 mg of l-thyroxine (T4; SIGMA), or 20 mg of 3,3′,5-triiodo-l-thyronine (T3; SIGMA) was dissolved in 200 μL of 2N NaOH and added 800 μL of water, 10 mg of hydrocortisone (HC; Wako) was dissolved in 1 mL of ethanol and added 9 mL of water, and 1 mg of 9-cis-retinoic acid (RA; Funakoshi) was dissolved in 2 mL of ethanol. Each solution was diluted in water to the final administrated concentrations. The same amount of diluted NaOH solution was added to the control. Final administrated concentrations are as follows: T4 100 μM; T3 118 μM; RA 0.5 μM; HC 250 μM. For each condition, 10 larvae at 2 wpf (TL, 5.5–7.0 mm) were kept in 40 mL of fish water.
Results
Expression of pitx1 in developing zebrafish embryos, larvae and juveniles
We first examined the expression pattern of pitx1, known to be involved in the initiation of pelvic fin development in stickleback (Shapiro et al., 2004; Chan et al., 2010), in developing zebrafish embryos, larvae and juveniles (Figures 1A–E). At 18 hpf, pitx1 expression appeared in the posterior lateral plate mesoderm (arrowheads in Figure 1A). By 24 hpf, expression of pitx1 was intensified in the posterior lateral plate mesoderm (arrowheads in Figure 1B). By 48 hpf, pitx1 expression was hardly detectable in the lateral plate mesoderm, whereas it was detected in the pharyngeal region (arrowheads in Figure 1C), as in other teleosts (Shapiro et al., 2004; Tanaka et al., 2005; Chan et al., 2010). At 2 wpf (TL, 5.5–7.0 mm; Supplementary Figure S1A), pitx1 transcripts were still detectable in the pharyngeal region (arrowheads in Figure 1D) but not in the presumptive pelvic fin field (dashed oval in Figures 1D, 1D′). By 3 wpf (TL, 9.0–12.0 mm; Supplementary Figure S1B), expression of pitx1 was detected in the pelvic fin bud, as well as in the pharyngeal region, dorsal fin and anal fin (Figures 1E, 1E′).
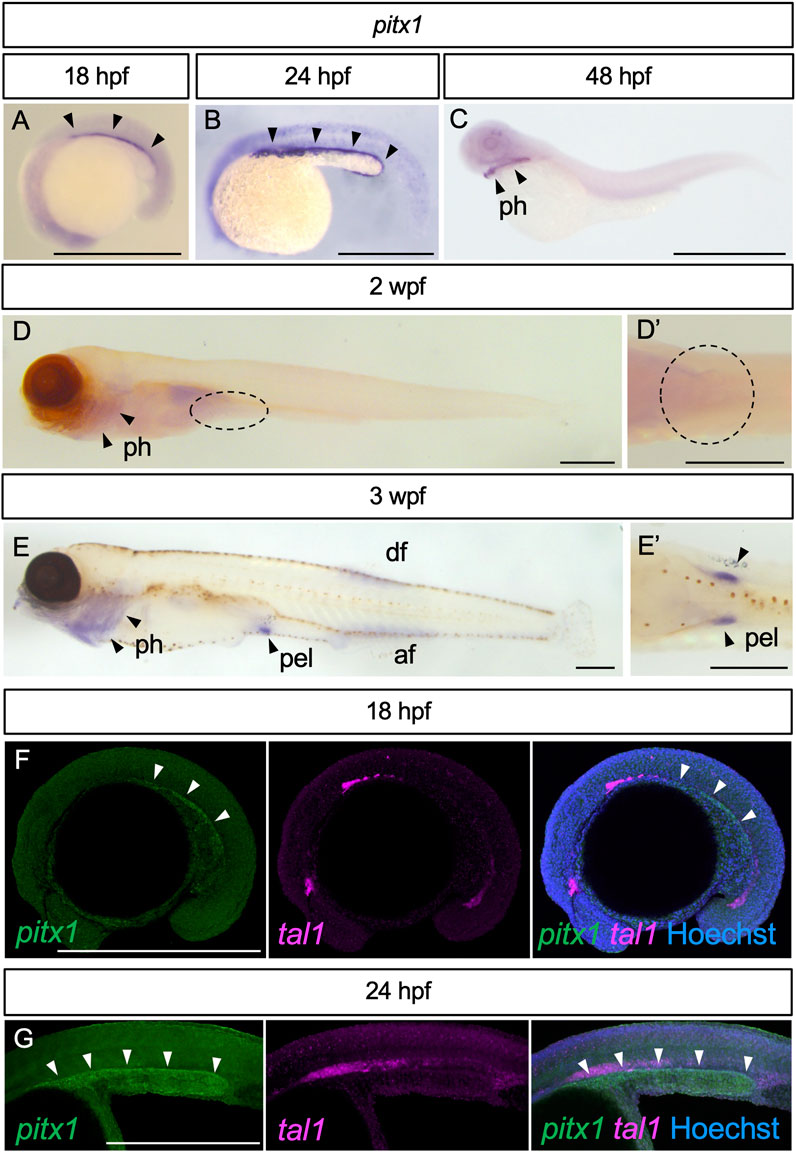
FIGURE 1. Expression of pitx1 in zebrafish. (A–E) Representative images of pitx1 expression in zebrafish at 18 hpf (A) (n = 3), 24 hpf (B) (n = 4), 48 hpf (C) (n = 3), 2 wpf (D) (n = 4) and 3 wpf (E) (n = 3). (D′,E′) Ventral views of the pelvic fin region in (D,E), respectively. (F,G) HCR in situ images of 18 hpf (F) and 24 hpf (G) embryos with pitx1 and tal1 signals. af, anal fin; df, dorsal fin; pel, pelvic fin; ph, pharynx. Scale bars, 0.5 mm.
Detection of pitx1 expression in the posterior lateral plate mesoderm during early embryogenesis is interesting. However, we cannot exclude the possibility that pitx1-positive cells are hemanigioblastic cells (precursors of blood and endothelial cells) derived from the posterior lateral plate mesoderm (Xiong, 2008). To address this possibility, we compared the expression of pitx1 with that of hemangioblast marker tal1 (also known as scl) (Gering et al., 1998) in early embryos by using Hybridization Chain Reaction (HCR) probe sets (Figures 1F, G). In early embryos, pitx1 signals were detected in the posterior lateral plate mesoderm, and not overlapped with tal1 signals (Figures 1F, G), suggesting that these pitx1-positive cells are at least not hemangioblastic cells.
Our results suggest that, in zebrafish, expression of pitx1 is activated in the posterior lateral plate mesoderm during early embryonic stages, is repressed during larval stages and re-appears in the pelvic fin bud during juvenile stages.
Pelvic fin–specific pitx1 enhancer is “poised” until the larva-to-juvenile transition
To investigate how the expression of pitx1 was repressed during larval stages, we assessed the function of the enhancer of pitx1 in driving its expression in the pelvic fin bud. For this, we compared the sequence from the pitx1 region in stickleback, including the pelvic fin–specific enhancer sequence (Pel enhancer in Chan et al., 2010), with that from zebrafish, medaka, tetraodon, cod and fugu (Figure 2A). This allowed us to identify the homolog of the pelvic fin–specific pitx1 enhancer in zebrafish ∼35 kilobase pairs (kbp) upstream of pitx1 (Figure 2A), which contains the predicted multiple transcription factor binding sites (Supplementary Figure S2). To assess the function of this putative enhancer, we cloned the 837-bp fragment in front of a basal promoter followed by an EGFP reporter gene (Figure 2B). This fragment did not drive EGFP expression in 2-wpf larvae (0/17 in F2 fishes; Figure 2B), whereas it did drive EGFP expression specifically in the pelvic fin in 4- to 5-wpf juveniles (5/17 in F2 fishes; Figure 2B). LPM-specific EGFP expression was not detected in 24 hpf-F0 founders or F1 fish (data not shown). These results suggest that the identified 837-bp non-coding sequence 35 kbp upstream of pitx1 contains a specific enhancer for pelvic fin expression in zebrafish, which we term the DrPel enhancer.
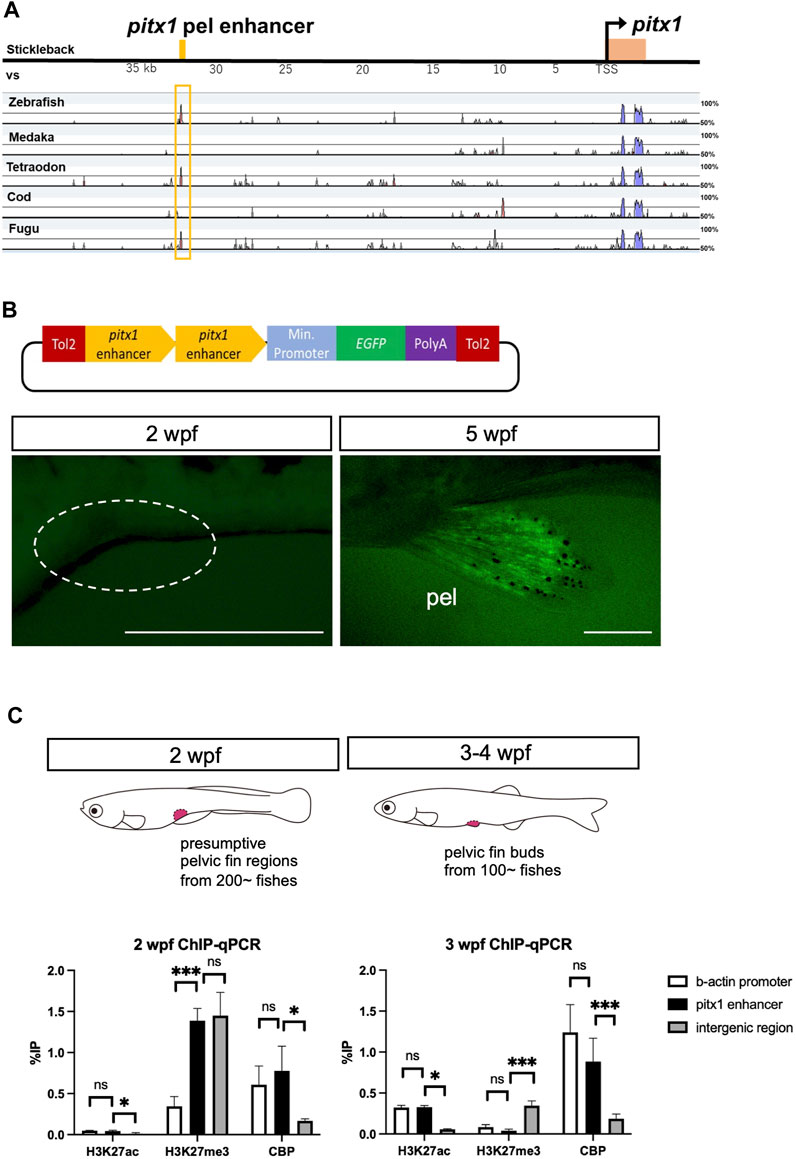
FIGURE 2. Pelvic fin–specific pitx1 enhancer is in a poised state until metamorphosis. (A) mVISTA alignment of sequences from the pitx1 region from stickleback, medaka, tetraodon, cod and fugu. Red peaks indicate >50% sequence identity in a 50-bp sliding window. The yellow bar at the top indicates the 501-bp pelvic fin–specific enhancer region identified 35 kb upstream of pitx1 in stickleback (Pel-501 bp in Chan et al., 2010). (B) DrPel enhancer does not drive EGFP expression in 2-wpf zebrafish larvae, whereas it does drive EGFP expression specifically in the pelvic fins of 5-wpf juveniles. Scale bars, 0.5 mm. (C) Epigenetic states of DrPel at different developmental time points were revealed by ChIP-qPCR analysis of the presumptive pelvic fin region from 2-wpf larvae and the pelvic fin bud from 3- to 4-wpf juveniles. The β-actin promoter sequence and the ORF-free IGR were used as positive and negative control regions, respectively. *p < 0.05, ***p < 0.001; ns, not significant. Mean ± s.d. (n = 3). See text for more details.
Poised enhancers have been suggested to be involved in the future activation of developmental genes in cells upon their differentiation into a specific cell fate (Rada-Iglesias et al., 2011; Crispatzu et al., 2021). In human embryonic stem cells, poised enhancers are enriched with universal co-activators, such as p300 (Kamei et al., 1996) and CBP, and display high chromatin accessibility; however, unlike active enhancers, they are not marked with acetylation of histone H3 at lysine 27 (H3K27ac) but are enriched for trimethylation of histone H3 at lysine 27 (H3K27me3), which is associated with Polycomb group protein complexes (Rada-Iglesias et al., 2011). Such poised enhancers have also been identified in chicken and mouse embryos (Crispatzu et al., 2021). Thus, we explored the possibility that the DrPel enhancer of presumptive pelvic fin cells of zebrafish larvae is already in a poised state prior to the emergence of the pelvic fin buds. We dissected the presumptive pelvic fin region from ∼200 larvae at 2 wpf (TL, 5.5–7.0 mm) and the pelvic fin bud from ∼100 juveniles at 3–4 wpf (TL, 9.0–12.0 mm) and performed ChIP with H3K27ac, H3K27me3 or CBP antibodies. Subsequently, qPCR was performed with a pair of primers that amplified a region within the DrPel enhancer, as well as two pairs of primers that amplified the β-actin promoter (Lindeman et al., 2010) or the ORF-free IGR (see details in Materials and Methods) as control regions. ChIP-qPCR results demonstrated the absence of H3K27ac and the enrichment of H3K27me3 in the DrPel sequence of the presumptive pelvic fin region of 2-wpf larvae (Figure 2C). Whereas we observed a large increase in H3K27ac levels in the DrPel sequence in the pelvic fin bud of juveniles at 3–4 wpf, H3K27me3 levels were hardly detectable (Figure 2C). We then examined the association of a universal transcriptional co-activator, CBP, with the DrPel enhancer to evaluate whether the enhancer was silenced, poised or activated (Rada-Iglesias et al., 2011). Our results showed that both in the presumptive pelvic fin region at 2 wpf and in the pelvic fin bud at 3–4 wpf, the DrPel enhancer was associated with high levels of CBP (Figure 2C), suggesting that the DrPel enhancer in the presumptive pelvic fin is in a poised state at 2 wpf and is activated in the pelvic fin bud at 3–4 wpf.
Exogenous thyroid hormones are not sufficient for the induction of pitx1 in the presumptive pelvic fin
Development of the pelvic fin bud initiates at 3 wpf during the larva-to-juvenile transition (metamorphosis) in zebrafish (Grandel and Schulte-Merker, 1998). Although an application of T3 or T4 alone does not accelerate the development of pelvic fin buds in zebrafish (Brown, 1997), thyroid hormones have been shown to play pivotal roles in metamorphosis in all teleost fishes studied (Campinho, 2019). We thus considered whether thyroid hormones are involved in the activation of pitx1 transcription in the pelvic fin bud during metamorphosis. To test this hypothesis, we incubated 2-wpf zebrafish larvae with 100 μM T4 (the biologically less-active thyroid hormone), with 100 μM T3 (active thyroid hormone) or with T3 with endocrine disruptors that mimic thyroid hormone activity, such as 0.5 μM 9-cis-RA and/or 250 μM hydrocortisone (HC) and examined the expression of pitx1 (Figures 3A–D; Supplementary Figure S3). After 24 h of incubation, decreased melanophore pigmentation was observed in the skins of 2-wpf larvae treated with T3 or with T3 and RA or HC, but not in those of control or T4-treated larvae (Figures 3A, C; Supplementary Figure S3). This suggests that these treatment conditions are sufficient to affect larval pigment development, as seen in several teleosts (Yoo et al., 2000; Parichy and Turner, 2003; McMenamin et al., 2014; Salis et al., 2021). Thus, we incubated 2-wpf larvae with the same concentrations of hormones and endocrine disruptors for 24 h and examined the expression pattern of pitx1 in the larvae. Although pitx1 expression was observed in the pharyngeal regions of 2-wpf larvae incubated with T3 or with T3 and RA or HC, as was observed in control larvae, no pitx1 transcripts were detected in the presumptive pelvic fin region in any of these larvae (Figures 3B, D; Supplementary Figures S3A–J). These results suggest that none of these hormones or endocrine disruptors are sufficient for the induction of pitx1 expression in the pelvic fin region of 2-wpf larvae, at least under these conditions.
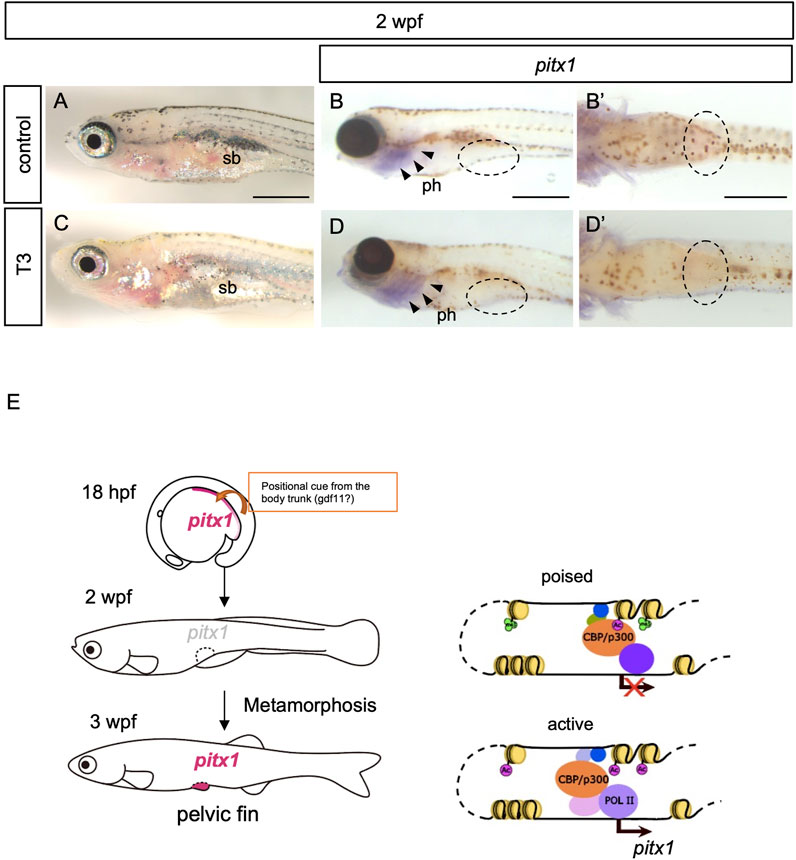
FIGURE 3. Exogenous thyroid hormones are not sufficient for the induction of pitx1 in the presumptive pelvic fin. (A–D) Zebrafish larvae at 2 wpf were treated with active T3 (C,D) or with vehicle (control; (A,B) for 24 h. Both the resulting pigment pattern [(A), n = 4; (C), n = 3] and the expression of pitx1 [(B), n = 4; (D), n = 3] were examined. (B′,D′) Ventral views indicated by the dashed ovals in (B,D), respectively. Expression of pitx1 was observed in the pharyngeal region [arrowheads in (B,D)] but not in the presumptive pelvic fin region of either T3-treated or control larvae [dashed ovals in (B,D)]. ph, pharynx; sb, swim bladder. Scale bars, 0.5 mm. (E) A proposed model of how pitx1 expression is controlled in the presumptive pelvic fin regions. Presumptive pelvic fin cells are likely to receive a positional cue, probably gdf11 or an unknown factor controlled by gdf11, from the body trunk by at least 16 hpf (Murata et al., 2010; Tanaka, 2011). pitx1 expression appears in the lateral plate mesoderm, including the presumptive pelvic fin cells, at least by 18 hpf. However, at 2 wpf, the DrPel enhancer becomes in a poised state, and thus pitx1 expression is not activated within the presumptive pelvic fin region. The DrPel enhancer is activated in the pelvic fin buds during the larva-to-juvenile transition (metamorphosis).
Discussion
Our data indicate that, in zebrafish, expression of pitx1 appears in the posterior lateral plate mesoderm during the embryonic stage, but becomes downregulated during the larval stage. We further showed that the pelvic fin–specific pitx1 (DrPel) enhancer is in a poised state in presumptive pelvic fin cells of larvae, and becomes activated in pelvic fin buds of juveniles during the metamorphosis (Figure 3E).
In zebrafish, pectoral fin buds appear as bulges of mesenchymal cells on the yolk surface at around 23 hpf, whereas pelvic fin buds emerge in the ventral body wall at 3 wpf, during the larva-to-juvenile transition (Kimmel et al., 1995; Grandel and Schulte-Merker, 1998). However, the fate of the presumptive pelvic fin cells has already been determined at least by 16 hpf and is maintained for 3 weeks by an unknown mechanism (Murata et al., 2010). In tetrapod embryos, the caudal expression of Growth differentiation factor 11 (Gdf11) is critical for the positioning of hindlimb-forming fields along the body axis (McPherron et al., 1999; Liu, 2006; Jurberg et al., 2013; Matsubara et al., 2017). In zebrafish, expression of gdf11 appears in the caudal end of the body trunk during early embryogenesis (10 hpf), and a reduction in gdf11 transcripts results in a caudal displacement of the pelvic fin position (Murata et al., 2010), suggesting that the function of gdf11 for defining the position of posterior paired appendages is conserved in zebrafish, even though the expression of gdf11 persists only during early embryogenesis. In this study, we showed that the pitx1 expression in the posterior region of the lateral plate mesoderm also persisted during early embryogenesis. The pitx1-positive region observed at 18 hpf (Figure 1A) is consistent with the presumptive pelvic fin cells as determined by fate-mapping experiments (Murata et al., 2010). Therefore, it is likely that the presumptive pelvic fin cells are already determined by pitx1 at the early embryonic stage, but its expression is not sufficient for the induction of pelvic fin buds. Indeed, tbx4, another factor required for the development of hindlimbs (Logan and Tabin, 1999; Duboc and Logan, 2011), was not detected in the lateral plate mesoderm of early zebrafish embryos (Supplementary Figure S4), as reported (Tamura et al., 1999; Ruvinsky et al., 2000). The inability of tbx4 induction during early embryogenesis may explain the heterochronic development of pelvic fins. In zebrafish, the pitx1-positive-posterior region of the lateral plate mesoderm apparently does not split into the somatic and splanchnic layers at the embryonic stage. Subdivision of the lateral plate mesoderm into the somatic and splanchnic layers is suggested to be the critical step for limb bud formation (Onimaru et al., 2011; Tanaka and Onimaru, 2012; Tanaka, 2013). Therefore, the unseparated lateral plate mesoderm at the posterior region of the embryo might also be one of the reasons why the pitx1-positive cells do not initiate the development of pelvic fin buds during embryogenesis in zebrafish.
The results presented here demonstrate that the DrPel enhancer is in a poised state in the presumptive pelvic fin cells of larvae, and activated during the metamorphosis. Although it is tempting to speculate that the poised state of the DrPel enhancer is related to the maintenance of the fate of pelvic fin cells, it is difficult to ascertain due to the limited knowledge of the regulatory mechanism of metamorphic development in teleosts. Metamorphosis is shown to be triggered by thyroid hormones in all teleost fishes studied (Campinho, 2019). In amphibians, the presence of thyroid hormones induces the removal of HDAC-containing corepressor complexes from thyroid hormone receptors (TRs) (Chen and Evans, 1995; Horlein et al., 1995), and the recruitment of histone acetyltransferase (HAT; renamed as lysine acetyltransferase, KAT) coactivators such as p300 and CBP for activation of the transcription of target genes (Glass and Rosenfeld, 2000). This cofactor exchange identified during the metamorphosis of Xenopus laevis has been suggested to control the timing of metamorphic development of various organs (Sachs et al., 2002; Tomita et al., 2004). Thus, it is interesting to speculate that the heterochronic development of pelvic fins during the metamorphosis of zebrafish is regulated by the similar mechanisms, however, the application of thyroid hormones is not sufficient for accelerating the induction of pelvic fin formation (Brown, 1997), or pitx1 expression (Figure 3) in zebrafish larvae. In our study, the DrPel enhancer in presumptive pelvic fin cells of 2-wpf larvae had already recruited CBP, and thyroid hormones are not sufficient for the activation of pitx1 expression in the pelvic fin cells. Although we cannot exclude the possibility that TRs are directly bound to the DrPel enhancer, thyroid hormone response element (TRE) sequences were not identified within DrPel, at least in our survey, while a predicted retinoid X receptor (RXR) binding site was found (Supplementary Figure S2). It is still possible that another enhancer or promoter topologically associated with the DrPel is silenced by unliganded TRs, or that TRs are involved in regulating the transcription of unknown factor(s) directly or indirectly controlling the chromatin state of the DrPel enhancer. Future studies are needed to identify unknown factor(s) required for pitx1 induction and to investigate the temporal association of chromatin structural modifications of the pelvic fin/posterior paired appendages–specific pitx1 enhancer. The delayed timing of pelvic fin development in teleosts was suggested to be correlated with variation in the pelvic fin position seen among teleost fishes, which ranges from the jugular to abdominal level (Murata et al., 2010; Tanaka, 2011; Kaneko et al., 2014). Such a variation in the pelvic fin position is a notable feature of teleost species from behavioral and taxonomic viewpoints (Gosline, 1971; Rosen, 1982; Standen, 2008). Elucidating the regulatory mechanism of the pelvic fin–specific pitx1 enhancer is expected to provide insights into the diversification of pelvic fin position among teleosts.
Data availability statement
The original contributions presented in the study are included in the article/Supplementary Material, further inquiries can be directed to the corresponding author.
Ethics statement
The experimental procedures were approved by the Gene Modification Experiments Safety Committee of the Tokyo Institute of Technology. All animal experiments were performed in accordance with guideline for animal experiments of the Tokyo Institute of Technology.
Author contributions
HP and MT designed the project and wrote the manuscript, HP performed most of the experiments, with the following exceptions. MH, KK, and TK performed some tissue culture experiments and expression analyses, RY constructed plasmids, KM performed some transgenic analyses, SU helped to perform ChIP analyses and YM performed some comparative genomic analyses. All authors contributed to the article and approved the submitted version.
Funding
This work was supported by MEXT KAKENHI Grant Numbers 24113508 and JSPS KAKENHI Grant Number 25291086 and 23H00385 and the Inamori Foundation to MT, and by a Sasakawa Scientific Research Grant, and the Sato Yo International Scholarship Foundation to HP.
Acknowledgments
We thank Koichi Kawakami and Yumie Murata for plasmids, Nobuhiro Nakamura for use of his facilities, and the Biomaterials Analysis Division in the Technical Department of Tokyo Institute of Technology for sequencing.
Conflict of interest
The authors declare that the research was conducted in the absence of any commercial or financial relationships that could be construed as a potential conflict of interest.
Publisher’s note
All claims expressed in this article are solely those of the authors and do not necessarily represent those of their affiliated organizations, or those of the publisher, the editors and the reviewers. Any product that may be evaluated in this article, or claim that may be made by its manufacturer, is not guaranteed or endorsed by the publisher.
Supplementary material
The Supplementary Material for this article can be found online at https://www.frontiersin.org/articles/10.3389/fcell.2023.1170691/full#supplementary-material
References
Alberch, P., Gould, S. J., Oster, G. F., and Wake, D. B. (1979). Size and shape in ontogeny and phylogeny. Paleobiology 5 (3), 296–317. doi:10.1017/S0094837300006588
Bininda-Emonds, O. R., Jeffery, J. E., Sanchez-Villagra, M. R., Hanken, J., Colbert, M., Pieau, C., et al. (2007). Forelimb-hindlimb developmental timing changes across tetrapod phylogeny. BMC Evol. Biol. 7, 182. doi:10.1186/1471-2148-7-182
Brown, D. D. (1997). The role of thyroid hormone in zebrafish and axolotl development. Proc. Natl. Acad. Sci. U. S. A. 94 (24), 13011–13016. doi:10.1073/pnas.94.24.13011
Campinho, M. A. (2019). Teleost metamorphosis: the role of thyroid hormone. Front. Endocrinol. (Lausanne) 10, 383. doi:10.3389/fendo.2019.00383
Chan, Y. F., Marks, M. E., Jones, F. C., Villarreal, G., Shapiro, M. D., Brady, S. D., et al. (2010). Adaptive evolution of pelvic reduction in sticklebacks by recurrent deletion of a Pitx1 enhancer. Science 327 (5963), 302–305. doi:10.1126/science.1182213
Chen, J. D., and Evans, R. M. (1995). A transcriptional co-repressor that interacts with nuclear hormone receptors. Nature 377 (6548), 454–457. doi:10.1038/377454a0
Cheng, S. Y., Leonard, J. L., and Davis, P. J. (2010). Molecular aspects of thyroid hormone actions. Endocr. Rev. 31 (2), 139–170. doi:10.1210/er.2009-0007
Chew, K. Y., Shaw, G., Yu, H., Pask, A. J., and Renfree, M. B. (2014). Heterochrony in the regulation of the developing marsupial limb. Dev. Dyn. official Publ. Am. Assoc. Anatomists 243 (2), 324–338. doi:10.1002/dvdy.24062
Cole, N. J., Tanaka, M., Prescott, A., and Tickle, C. (2003). Expression of limb initiation genes and clues to the morphological diversification of threespine stickleback. Curr. Biol. 13 (24), R951–R952. doi:10.1016/j.cub.2003.11.039
Crispatzu, G., Rehimi, R., Pachano, T., Bleckwehl, T., Cruz-Molina, S., Xiao, C., et al. (2021). The chromatin, topological and regulatory properties of pluripotency-associated poised enhancers are conserved in vivo. Nat. Commun. 12 (1), 4344. doi:10.1038/s41467-021-24641-4
Don, E. K., de Jong-Curtain, T. A., Doggett, K., Hall, T. E., Heng, B., Badrock, A. P., et al. (2016). Genetic basis of hindlimb loss in a naturally occurring vertebrate model. Biol. Open 5 (3), 359–366. doi:10.1242/bio.016295
Duboc, V., and Logan, M. P. (2011). Pitx1 is necessary for normal initiation of hindlimb outgrowth through regulation of Tbx4 expression and shapes hindlimb morphologies via targeted growth control. Development 138 (24), 5301–5309. doi:10.1242/dev.074153
Gering, M., Rodaway, A. R., Gottgens, B., Patient, R. K., and Green, A. R. (1998). The SCL gene specifies haemangioblast development from early mesoderm. EMBO J. 17 (14), 4029–4045. doi:10.1093/emboj/17.14.4029
Glass, C. K., and Rosenfeld, M. G. (2000). The coregulator exchange in transcriptional functions of nuclear receptors. Genes Dev. 14 (2), 121–141. doi:10.1101/gad.14.2.121
Gosline, W. A. (1971). Functional morphology and classification of teleostean fishes. Honolulu: The University Press of Hawaii.
Grandel, H., and Schulte-Merker, S. (1998). The development of the paired fins in the zebrafish (Danio rerio). Mech. Dev. 79 (1-2), 99–120. doi:10.1016/s0925-4773(98)00176-2
Horlein, A. J., Naar, A. M., Heinzel, T., Torchia, J., Gloss, B., Kurokawa, R., et al. (1995). Ligand-independent repression by the thyroid hormone receptor mediated by a nuclear receptor co-repressor. Nature 377 (6548), 397–404. doi:10.1038/377397a0
Ibarra-Garcia-Padilla, R., Howard, A. G. A. t., Singleton, E. W., and Uribe, R. A. (2021). A protocol for whole-mount immuno-coupled hybridization chain reaction (WICHCR) in zebrafish embryos and larvae. Star. Protoc. 2 (3), 100709. doi:10.1016/j.xpro.2021.100709
Jurberg, A. D., Aires, R., Varela-Lasheras, I., Novoa, A., and Mallo, M. (2013). Switching axial progenitors from producing trunk to tail tissues in vertebrate embryos. Dev. Cell 25 (5), 451–462. doi:10.1016/j.devcel.2013.05.009
Kamei, Y., Xu, L., Heinzel, T., Torchia, J., Kurokawa, R., Gloss, B., et al. (1996). A CBP integrator complex mediates transcriptional activation and AP-1 inhibition by nuclear receptors. Cell 85 (3), 403–414. doi:10.1016/s0092-8674(00)81118-6
Kaneko, H., Nakatani, Y., Fujimura, K., and Tanaka, M. (2014). Development of the lateral plate mesoderm in medaka Oryzias latipes and Nile tilapia Oreochromis niloticus: insight into the diversification of pelvic fin position. J. Anat. 225, 659–674. doi:10.1111/joa.12244
Kawakami, K. (2004). Transgenesis and gene trap methods in zebrafish by using the Tol2 transposable element. Methods Cell Biol. 77, 201–222. doi:10.1016/s0091-679x(04)77011-9
Kimmel, C. B., Ballard, W. W., Kimmel, S. R., Ullmann, B., and Schilling, T. F. (1995). Stages of embryonic development of the zebrafish. Dev. Dyn. official Publ. Am. Assoc. Anatomists 203 (3), 253–310. doi:10.1002/aja.1002030302
Koibuchi, N. (2009). Animal models to study thyroid hormone action in cerebellum. Cerebellum 8 (2), 89–97. doi:10.1007/s12311-008-0089-x
Lanctot, C., Moreau, A., Chamberland, M., Tremblay, M. L., and Drouin, J. (1999). Hindlimb patterning and mandible development require the Ptx1 gene. Development 126 (9), 1805–1810. doi:10.1242/dev.126.9.1805
Lin, Q., Fan, S., Zhang, Y., Xu, M., Zhang, H., Yang, Y., et al. (2016). The seahorse genome and the evolution of its specialized morphology. Nature 540 (7633), 395–399. doi:10.1038/nature20595
Lindeman, L. C., Reiner, A. H., Mathavan, S., Alestrom, P., and Collas, P. (2010). Tiling histone H3 lysine 4 and 27 methylation in zebrafish using high-density microarrays. PloS one 5 (12), e15651. doi:10.1371/journal.pone.0015651
Liu, J. P. (2006). The function of growth/differentiation factor 11 (Gdf11) in rostrocaudal patterning of the developing spinal cord. Development 133 (15), 2865–2874. doi:10.1242/dev.02478
Logan, M., and Tabin, C. J. (1999). Role of Pitx1 upstream of Tbx4 in specification of hindlimb identity. Science 283 (5408), 1736–1739. doi:10.1126/science.283.5408.1736
Marcil, A., Dumontier, E., Chamberland, M., Camper, S. A., and Drouin, J. (2003). Pitx1 and Pitx2 are required for development of hindlimb buds. Development 130 (1), 45–55. doi:10.1242/dev.00192
Matsubara, Y., Hirasawa, T., Egawa, S., Hattori, A., Suganuma, T., Kohara, Y., et al. (2017). Anatomical integration of the sacral-hindlimb unit coordinated by GDF11 underlies variation in hindlimb positioning in tetrapods. Nat. Ecol. Evol. 1 (9), 1392–1399. doi:10.1038/s41559-017-0247-y
McMenamin, S. K., Bain, E. J., McCann, A. E., Patterson, L. B., Eom, D. S., Waller, Z. P., et al. (2014). Thyroid hormone-dependent adult pigment cell lineage and pattern in zebrafish. Science 345 (6202), 1358–1361. doi:10.1126/science.1256251
McPherron, A. C., Lawler, A. M., and Lee, S. J. (1999). Regulation of anterior/posterior patterning of the axial skeleton by growth/differentiation factor 11. Nat. Genet. 22 (3), 260–264. doi:10.1038/10320
Miwa, S., Yamano, K., and Inui, Y. (1992). Thyroid-hormone stimulates gastric development in flounder larvae during metamorphosis. J. Exp. Zoology 261 (4), 424–430. doi:10.1002/jez.1402610409
Murata, Y., Tamura, M., Aita, Y., Fujimura, K., Murakami, Y., Okabe, M., et al. (2010). Allometric growth of the trunk leads to the rostral shift of the pelvic fin in teleost fishes. Dev. Biol. 347, 236–245. doi:10.1016/j.ydbio.2010.07.034
Nakato, R., Itoh, T., and Shirahige, K. (2013). Drompa: easy-to-handle peak calling and visualization software for the computational analysis and validation of ChIP-seq data. Genes cells devoted Mol. Cell. Mech. 18 (7), 589–601. doi:10.1111/gtc.12058
Okamoto, E., Van Mai, H., Ishimatsu, A., and Tanaka, M. (2018). Modification of pectoral fins occurs during the larva-to-juvenile transition in the mudskipper (Periophthalmus modestus). Zool. Lett. 4, 23. doi:10.1186/s40851-018-0105-z
Onimaru, K., Shoguchi, E., Kuratani, S., and Tanaka, M. (2011). Development and evolution of the lateral plate mesoderm: comparative analysis of amphioxus and lamprey with implications for the acquisition of paired fins. Dev. Biol. 359 (1), 124–136. doi:10.1016/j.ydbio.2011.08.003
Parichy, D. M., and Turner, J. M. (2003). Zebrafish puma mutant decouples pigment pattern and somatic metamorphosis. Dev. Biol. 256 (2), 242–257. doi:10.1016/s0012-1606(03)00015-0
Power, D. M., Llewellyn, L., Faustino, M., Nowell, M. A., Bjornsson, B. T., Einarsdottir, I. E., et al. (2001). Thyroid hormones in growth and development of fish. Comp. Biochem. Physiol. C Toxicol. Pharmacol. 130 (4), 447–459. doi:10.1016/s1532-0456(01)00271-x
Rada-Iglesias, A., Bajpai, R., Swigut, T., Brugmann, S. A., Flynn, R. A., and Wysocka, J. (2011). A unique chromatin signature uncovers early developmental enhancers in humans. Nature 470 (7333), 279–283. doi:10.1038/nature09692
Richardson, M. K., Gobes, S. M., van Leeuwen, A. C., Polman, J. A., Pieau, C., and Sanchez-Villagra, M. R. (2009). Heterochrony in limb evolution: developmental mechanisms and natural selection. J. Exp. zoology. Part B, Mol. Dev. Evol. 312 (6), 639–664. doi:10.1002/jez.b.21250
Rosen, D. E. (1982). Teleostean interrelationships, morphological function and evolutionary inference. Amer. Zool. 22, 261–273. doi:10.1093/icb/22.2.261
Ruvinsky, I., Oates, A. C., Silver, L. M., and Ho, R. K. (2000). The evolution of paired appendages in vertebrates: T-Box genes in the zebrafish. Dev. Genes Evol. 210 (2), 82–91. doi:10.1007/s004270050014
Sachs, L. M., Jones, P. L., Havis, E., Rouse, N., Demeneix, B. A., and Shi, Y. B. (2002). Nuclear receptor corepressor recruitment by unliganded thyroid hormone receptor in gene repression during Xenopus laevis development. Mol. Cell. Biol. 22 (24), 8527–8538. doi:10.1128/MCB.22.24.8527-8538.2002
Salis, P., Roux, N., Huang, D., Marcionetti, A., Mouginot, P., Reynaud, M., et al. (2021). Thyroid hormones regulate the formation and environmental plasticity of white bars in clownfishes. Proc. Natl. Acad. Sci. U. S. A. 118 (23), e2101634118. doi:10.1073/pnas.2101634118
Shapiro, M. D., Marks, M. E., Peichel, C. L., Blackman, B. K., Nereng, K. S., Jonsson, B., et al. (2004). Genetic and developmental basis of evolutionary pelvic reduction in threespine sticklebacks. Nature 428 (6984), 717–723. doi:10.1038/nature02415
Standen, E. M. (2008). Pelvic fin locomotor function in fishes: three-dimensional kinematics in rainbow trout (Oncorhynchus mykiss). J. Exp. Biol. 211, 2931–2942. doi:10.1242/jeb.018572
Suda, N., Itoh, T., Nakato, R., Shirakawa, D., Bando, M., Katou, Y., et al. (2014). Dimeric combinations of MafB, cFos and cJun control the apoptosis-survival balance in limb morphogenesis. Development 141, 2885–2894. doi:10.1242/dev.099150
Szeto, D. P., Rodriguez-Esteban, C., Ryan, A. K., O'Connell, S. M., Liu, F., Kioussi, C., et al. (1999). Role of the Bicoid-related homeodomain factor Pitx1 in specifying hindlimb morphogenesis and pituitary development. Genes Dev. 13 (4), 484–494. doi:10.1101/gad.13.4.484
Tamura, K., Yonei-Tamura, S., and Belmonte, J. C. (1999). Differential expression of Tbx4 and Tbx5 in zebrafish fin buds. Mech. Dev. 87 (1-2), 181–184. doi:10.1016/s0925-4773(99)00126-4
Tanaka, M., Hale, L. A., Amores, A., Yan, Y. L., Cresko, W. A., Suzuki, T., et al. (2005). Developmental genetic basis for the evolution of pelvic fin loss in the pufferfish Takifugu rubripes. Dev. Biol. 281 (2), 227–239. doi:10.1016/j.ydbio.2005.02.016
Tanaka, M. (2013). Molecular and evolutionary basis of limb field specification and limb initiation. Dev. Growth Differ. 55 (1), 149–163. doi:10.1111/dgd.12017
Tanaka, M., and Onimaru, K. (2012). Acquisition of the paired fins: A view from the sequential evolution of the lateral plate mesoderm. Evol. Dev. 14 (5), 412–420. doi:10.1111/j.1525-142X.2012.00561.x
Tanaka, M. (2011). Revealing the mechanisms of the rostral shift of pelvic fins among teleost fishes. Evol. Dev. 13 (4), 382–390. doi:10.1111/j.1525-142X.2011.00493.x
Tomita, A., Buchholz, D. R., and Shi, Y. B. (2004). Recruitment of N-CoR/SMRT-TBLR1 corepressor complex by unliganded thyroid hormone receptor for gene repression during frog development. Mol. Cell. Biol. 24 (8), 3337–3346. doi:10.1128/MCB.24.8.3337-3346.2004
Urasaki, A., Morvan, G., and Kawakami, K. (2006). Functional dissection of the Tol2 transposable element identified the minimal cis-sequence and a highly repetitive sequence in the subterminal region essential for transposition. Genetics 174 (2), 639–649. doi:10.1534/genetics.106.060244
Visel, A., Blow, M. J., Li, Z., Zhang, T., Akiyama, J. A., Holt, A., et al. (2009). ChIP-seq accurately predicts tissue-specific activity of enhancers. Nature 457 (7231), 854–858. doi:10.1038/nature07730
Westerfield, M. (2000). “A guide for the laboratory use of zebrafish (Danio rerio),” in The zebrafish book (Eugene: University of Oregon Press).
Xiong, J. W. (2008). Molecular and developmental biology of the hemangioblast. Dev. Dyn. official Publ. Am. Assoc. Anatomists 237 (5), 1218–1231. doi:10.1002/dvdy.21542
Yen, P. M., Ando, S., Feng, X., Liu, Y., Maruvada, P., and Xia, X. (2006). Thyroid hormone action at the cellular, genomic and target gene levels. Mol. Cell Endocrinol. 246 (1-2), 121–127. doi:10.1016/j.mce.2005.11.030
Keywords: fin development, zebrafish, heterochrony, poised enhancer, thyroid hormone
Citation: Pratiwi HM, Hirasawa M, Kato K, Munakata K, Ueda S, Moriyama Y, Yu R, Kawanishi T and Tanaka M (2023) Heterochronic development of pelvic fins in zebrafish: possible involvement of temporal regulation of pitx1 expression. Front. Cell Dev. Biol. 11:1170691. doi: 10.3389/fcell.2023.1170691
Received: 21 February 2023; Accepted: 04 August 2023;
Published: 24 August 2023.
Edited by:
Yuji Atsuta, Kyushu University, JapanReviewed by:
Tetsuya Nakamura, Rutgers, The State University of New Jersey, United StatesMichael Hawkins, Boston Children’s Hospital and Harvard Medical School, United States
Copyright © 2023 Pratiwi, Hirasawa, Kato, Munakata, Ueda, Moriyama, Yu, Kawanishi and Tanaka. This is an open-access article distributed under the terms of the Creative Commons Attribution License (CC BY). The use, distribution or reproduction in other forums is permitted, provided the original author(s) and the copyright owner(s) are credited and that the original publication in this journal is cited, in accordance with accepted academic practice. No use, distribution or reproduction is permitted which does not comply with these terms.
*Correspondence: Mikiko Tanaka, mitanaka@bio.titech.ac.jp
†Present addresses: Hilda Mardiana Pratiwi, Atmosphere and Ocean Research Institute, The University of Tokyo, Kashiwa, Japan; Yuuta Moriyama, Department of Physical Sciences, College of Science and Engineering, Aoyama Gakuin University, Sagamihara, Japan