- 1Department of Pharmacy, Zhuhai People’s Hospital, Zhuhai Hospital Affiliated with Jinan University, Jinan University, Zhuhai, Guangdong, China
- 2School of Traditional Chinese Medicine, Southern Medical University, Guangzhou, China
- 3College of Pharmacy, Jinan University, Guangzhou, China
- 4Guangdong Provincial Key Laboratory of Tumor Interventional Diagnosis and Treatment, Zhuhai Institute of Translational Medicine, Zhuhai People’s Hospital Affiliated with Jinan University, Jinan University, Zhuhai, Guangdong, China
- 5School of Stomatology, Zunyi Medical University, Zunyi, Guizhou, China
Hepatocellular carcinoma (HCC) accounts for approximately 90% of all primary liver cancers and is one of the main malignant tumor types globally. It is essential to develop rapid, ultrasensitive, and accurate strategies for the diagnosis and surveillance of HCC. In recent years, aptasensors have attracted particular attention owing to their high sensitivity, excellent selectivity, and low production costs. Optical analysis, as a potential analytical tool, offers the advantages of a wide range of targets, rapid response, and simple instrumentation. In this review, recent progress in several types of optical aptasensors for biomarkers in early diagnosis and prognosis monitoring of HCC is summarized. Furthermore, we evaluate the strengths and limitations of these sensors and discuss the challenges and future perspectives for their use in HCC diagnosis and surveillance.
1 Introduction
Hepatocellular carcinoma (HCC) is the sixth most commonly diagnosed cancer and the fourth leading cause of cancer-related deaths globally (Singal et al., 2020). Owing to the lack of specific symptoms in the early stage of HCC, the diagnosis is often made at a late disease development stage, leading to a poor prognosis with an incidence-to-mortality ratio approaching one (Tsuchiya, 2015). Therefore, monitoring important HCC-related indicators has important clinical significance for their early prevention and prognostic monitoring. International guidelines broadly recommend surveillance of HCC to provide better treatment access and improvement of overall survival (Chen et al., 2020; Piñero et al., 2020).
Biomarkers refer to disease-related substances that can be detected in vivo or in vitro, such as proteins, genes, and metabolites (Liu X.-N. et al., 2019). Biomarkers can reflect the presence, development, and prognosis of the disease, serving as a basis for diagnosis, treatment, and prevention. There are several biomarkers for HCC, such as alpha-fetoprotein (AFP) (Colli et al., 2021), alpha-fetoprotein lens culinaris agglutin-3 (AFP-L3) (Choi et al., 2019), glypican-3 (GPC3) (Chen et al., 2022), sonic hedgehog ligand (SHh) (Li H.-Y. et al., 2021), and des-γ-carboxy prothrombin (DCP) (Volk et al., 2007), as well as some novel biomarkers, including osteopontin (OPN) (Cabiati et al., 2022), vascular endothelial growth factor (VEGF) (Xu et al., 2021), Golgi protein 73 (Gp-73) (Liu et al., 2022), insulin growth factor-1 (IGF-1) (Mazziotti et al., 2002), lipocalin-2 (LCN2) (Lee et al., 2015), and microRNAs (Liu et al., 2017; Chen et al., 2018). All these biomarkers are found in blood or tissue biopsies.
The sensitivity of the biomarkers varies based on the cut-off values. The cut-off values of biomarkers for HCC obtained from different clinical studies or retrospective analyses with different determination criteria are summarized in Table 1. However, the diagnostic accuracy of the absolute cut-off of these biomarkers has not been validated, and the values vary across institutions and patient populations. With a fixed tipping point of AFP (e.g., 200 ng/mL), a gradual increase rate of 7 ng/mL per month may be a useful diagnostic tool for HCC (Benson et al., 2021). The sensitivity and specificity of a single biomarker for early diagnosis of HCC vary greatly, indicating that using a single biomarker to detect early HCC is insufficient and has unsatisfactory diagnostic performance. In contrast, the combination of several biomarkers may improve early diagnostic rates.
AFP and liver ultrasound (US) are the most widely used methods for HCC screening. A review of serum protein biomarkers used for early detection of HCC showed that an AFP cut-off of 100 ng/mL was associated with high specificity (99%) but low sensitivity (31%). Combining AFP and US increased the sensitivity of HCC detection (97% and 78%, respectively) (Tzartzeva et al., 2018).
Abdominal multiphasic computed tomography (CT) or magnetic resonance imaging (MRI) is recommended in the setting of a rising serum AFP or following identification of a liver mass nodule ≥ 1 cm in the US, based on the guidelines of the American Association for the Study of Liver Diseases and Liver Imaging Reporting and Data System (Marrero et al., 2018), in which sensitivity for HCC is 87.5% and 83.1%, respectively (Tzartzeva et al., 2018). However, early HCC often presents as small nodules with a diameter usually less than 1 cm. CT and MRI are less sensitive in detecting small liver cancer, at 62.5% and 83.7%, respectively (Tzartzeva et al., 2018). Conversely, the detection effect of CT and MRI on HCC is susceptible to the interference of many factors such as technical level, lesions, and surrounding tissues, and false positive or false negative results may occur, leading to misdiagnosis or missed diagnosis. In contrast, biomarkers can show abnormal indications when the tumor is small or asymptomatic, detecting abnormal changes in the tumor earlier, with obvious advantages with regard to early HCC diagnosis. Consequently, clinical strategies for effectively detecting and preventing HCC metastasis recurrence are urgently required. In recent years, biosensors have provided new opportunities and have attracted great interest among researchers.
A typical biosensor consists of a biorecognition element and a transducer that can provide qualitative or quantitative results of the targets (Figure 1) (Lim et al., 2010). Common bioreceptors comprise antibodies, aptamers, and enzymes (Bhalla et al., 2016). Among them, aptamers are oligonucleotides, short DNA, or RNA obtained by SELEX screening (Hosseinzadeh and Mazloum-Ardakani, 2020). Aptamers offer numerous advantages over the already widely used antibodies, such as a wide range of targets, high specificity and affinity to targets, low synthetic cost, and easy chemical modification (Li W. et al., 2015; Dehghani et al., 2018; Ibau et al., 2019). Aptamer-based sensors are also referred to as aptasensors, which use aptamers as the biorecognition element. Among them, optical aptasensors are biosensors that incorporate aptamers as the biorecognition element and various optical analytical techniques as the signal transductions, integrating the advantages of the aptamer and optical analysis (Feng et al., 2014; Sadeghi et al., 2018; Zahra et al., 2021). Hence, optical aptasensors can provide potential opportunities for the detection of targets in clinical analysis.
Currently, biomarkers of HCC can be detected by various analytical methods, including enzyme-linked immunosorbent assay (ELISA) (Hanif et al., 2022), mass spectrometry (MS) (Lyman et al., 2022), fluorescence quantitative PCR (qPCR) (Yang J. et al., 2019), immunohistochemistry (IHC) (Yang J. et al., 2019), chemiluminescence (CLIA) (Sun et al., 2018), and biochip technology (Zhang et al., 2023). In contrast, the high specificity of the aptasensor is prominent such that targets as low as fg/mL levels can be detected. Furthermore, the aptasensor can complete the detection in a few minutes, which is more rapid than other detection methods. They can also monitor changes in biomarkers in real time and facilitate timely diagnosis and treatment decisions. Certainly, aptasensors have some limitations that need to be addressed. At present, most of the aptasensors can only detect a specific type of target, and it is necessary to develop aptasensors that can simultaneously detect multiple targets. In addition, although the synthesis of aptamers is relatively low, the cost of aptasensors is limited by the enzymes and nanomaterials.
In the present review, we outline the recent advancements in aptasensors based on several optical principles in HCC surveillance and diagnosis, including fluorescence, colorimetry, chemiluminescence, surface plasmon resonance (SPR), and surface-enhanced Raman scattering (SERS). We focused on the detection methods of biomarkers associated with the early diagnosis and poor prognosis of HCC. With examples of various sensors, the common optical and color rendering materials and the working principle of these aptasensors are introduced. Besides, Table 2 summarizes the developments and applications focusing on the biomarker, material, detection limit, and linear range. Furthermore, the strengths and limitations of the sensors are evaluated and summarized in Table 3. Ultimately, we discuss the challenges and opportunities encountered in the application of the sensors in the surveillance and diagnosis of HCC.
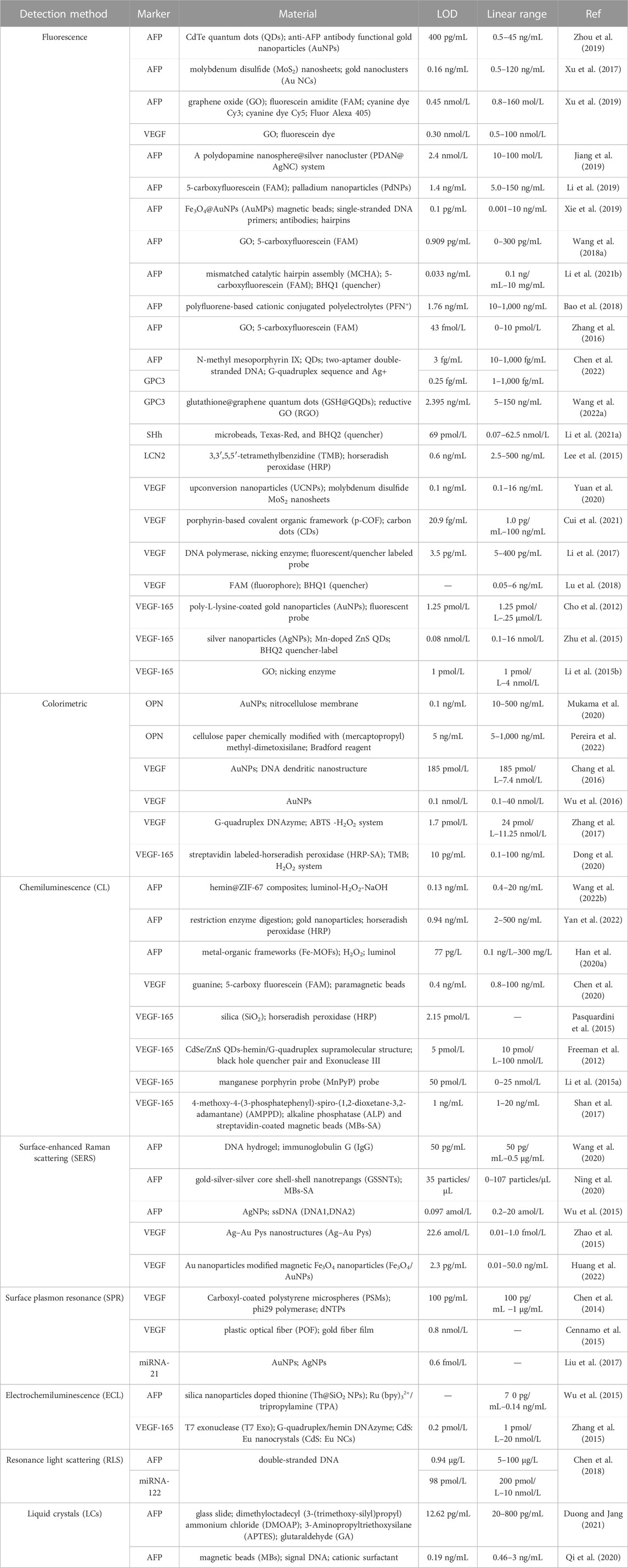
TABLE 2. | Summary of biomarkers related to hepatocellular carcinoma (HCC) surveillance and diagnosis using optical aptasensors.
2 Optical aptasensors
2.1 Fluorescence aptasensors
The light emitted by the normal temperature material following the absorption of electromagnetic energy is called fluorescence. Fluorescence-based strategies are often employed in constructing aptasensors for the target molecules due to advantages such as high sensitivity, strong specificity, and simple operation (Jia et al., 2020). Förster resonance energy transfer (FRET) occurs where dipole-mediated energy transfer occurs from the excited donor fluorophore to a proximal ground-state receptor (Kaur et al., 2021). In the design of fluorescent aptasensors, FRET has been used extensively as the significant sensing form based on fluorescent quenching or fluorophore signal recovery strategies (Cao et al., 2012; Shan et al., 2014; Yang B. et al., 2018).
Over the past few decades, a series of FRET biosensors have been designed to detect diverse tumor markers with organic dyes or semiconductor quantum dots (QDs) as energy donors (Wang et al., 2014). Wang H. et al. (2022) labeled the GPC3 aptamer with glutathione@graphene QDs to construct the GSH@GQDs-GPC3Apt complex. Based on the complex, they designed a novel FRET biosensing platform for GPC3 detection, with a linear range from 5 ng/mL to 150 ng/mL and a limit of detection of 2.395 ng/mL at an S/N of three. Chen et al. reported a strategy for the simultaneous detection of AFP and glypican-3 GPC3 by developing a catalytic hairpin assembly amplification method with Nmethyl mesoporphyrin IX and QDs as signal reporters. The limits of detection of the assay were as low as 3 fg/mL for AFP and 0.25 fg/mL for GPC3 (Scheme 1) (Chen et al., 2022).
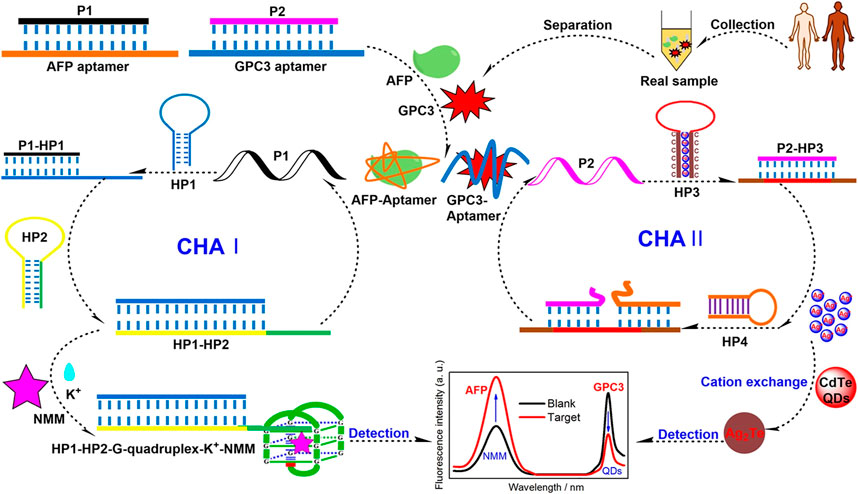
SCHEME 1. Schematic illustration of a Förster Resonance Energy Transfer (FRET) aptasensor for simultaneous detection of alpha-fetoprotein (AFP) and Glypican-3 (GPC3) (Chen et al., 2022). Reprinted (adapted) with permission from Chen et al. (2022). Copyright © 2022 American Chemical Society.
Li H.-Y. et al. (2021) devised a simple aptasensor for detecting SHh content by conjugating Texas-Red-labeled aptamer for SHh to microbeads. The broad detection range was from 0.07 to 62.5 nM, and the LOD was down to 69 pM. In addition, the test results of 28 HCC specimens demonstrated that the method compensated for detecting HCC in AFP-negative cases (Li H.-Y. et al., 2021).
The effective application of organic dyes and QDs is limited due to the easy photo-bleaching, poor photo-stability of organic dyes, and high toxicity for QDs. In comparison, gold nanoclusters (AuNCs) have become the new candidates owing to their great biocompatibility, stable fluorescence emission, and good photo-stability (Yang et al., 2014; Ding and Tian, 2015). Based on this, Xu et al. (2017) described a novel aptamer-induced “switch on” FRET aptasensor for the simultaneous determination of multiple tumor target markers (e.g., AFP) by virtue of combining MoS2 nanosheets and multicolored AuNCs through single wavelength excitation (Scheme 2). The FRET biosensor used AFP aptamer functionalized dual-colored gold nanoclusters (Au NCs) as energy donors and MoS2 as energy receptors. This method demonstrated that the detection limit decreased to 0.16 ng/mL at 3 s, with a linear range of 0.5–120 ng/mL for AFP. Moreover, the biosensing platform can visually and qualitatively discriminate serums from normal and hepatoma patients, demonstrating the assay potential for clinical diagnosis (Xu et al., 2017).
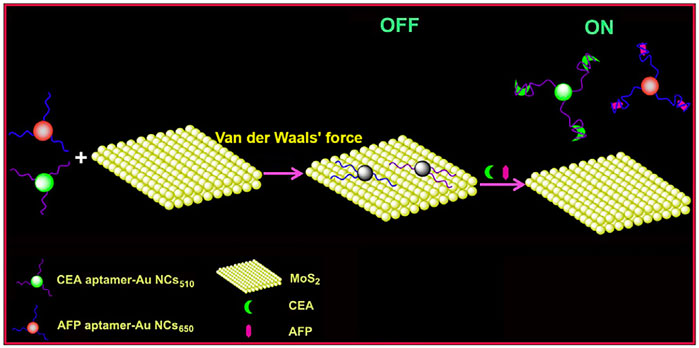
SCHEME 2. A Förster Resonance Energy Transfer (FRET) biosensor for simultaneous detection of multiple tumor markers (Xu et al., 2017).
2.2 Colorimetric aptasensors
Colorimetric aptasensors have been used to detect tumor biomarkers due to their ease of use, accessibility, and point-of-care detection (Wang D. et al., 2018). Colorimetric biosensing platforms are a promising technique as they translate invisible signals into visual color changes. The test results are directly assessed by the naked eye without the support of any instrument; hence, this type of sensor has the greatest commercial testing potential (Xiao et al., 2017; Akshaya et al., 2020). The most common catalysts in the colorimetric methods are horseradish peroxidase (HRP) and an HRP-mimicking DNAzyme named G-quadruplex-hemin DNAzyme for reactions between the chromogenic substrate and hydrogen peroxide (H2O2). These catalysts can effectively catalyze H2O2-mediated oxidation of 2,2-azino-bis (3-ethylbenzothiazoline-6-sulfonicacid), diammonium salt, and 3,3′,5,5′-tetramethylbenzidine (TMB), with a visible colorimetric output signal (Yang B. et al., 2019).
The lateral flow biosensor (LFB) is an ideal platform for the rapid detection of various cancer biomarkers, integrating four major parts: samples, conjugates, absorption pads, and the nitrocellulose membrane (Sajid et al., 2015; Baryeh et al., 2017; Huang et al., 2017; Mukama et al., 2017). Mukama et al. established a simple, rapid, and highly specific LFB for OPN detection (Scheme 3). This is an example of collaboration between the aptamer and antibody. The aptamer for OPN was used for pre-capture from samples, and the antibody for OPN was immobilized on the test line for a second identification. Finally, gold nanoparticles (AuNPs) sprayed on the conjugation pad were utilized for color detection. Notably, the LFB achieved a qualitative and semi-quantitative detection of OPN in clinical serum and could provide a rapid visual detection response within 5 min (Mukama et al., 2020).
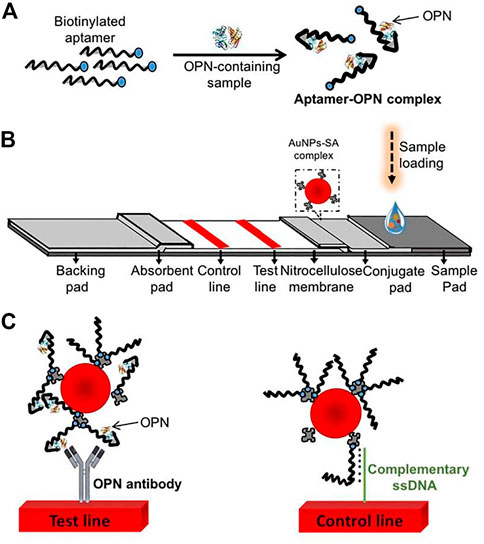
SCHEME 3. Scheme of the osteopontin (OPN) lateral flow biosensor (LFB). (A) Capture of the targets by the aptamers; (B) The LFB assembly with streptavidin-coated gold nanoparticles (AuNPs-SA) on the conjugate pad, immobilized OPN antibody, and a probe complementary to the aptamer on the nitrocellulose membrane; (C) the anti-OPN antibody at the test line captured the OPN-aptamer, and the spare aptamers are captured by the complementary ssDNA probes at the control line (Mukama et al., 2020).
To improve the sensitivity of the colorimetric biosensor based on G-quadruplex-hemin DNAzyme, Zhang et al., 2017 introduced strand displacement amplification (SDA) as an amplification technique for developing a label-free colorimetric aptasensor for sensitive detection of VEGF-165 (Scheme 4). The specific binding of VEGF-165 with the aptamer-based hairpin probe initiated the SDA to produce more triggers, which can further anneal with the hairpin probe and result in the opening of the hairpin structure, subsequently initiating the next round of SDA reaction and yielding more triggers. Finally, the abundant triggers hybridize with the linear template and subsequently initiate a new round of SDA reactions to generate additional G-quadruplex-hemin DNAzymes for further colorimetric reactions. The linear relation ranges from 24 pM to 11.25 nM, with a detection limit of 1.7 pM (Zhang et al., 2017). In addition, Dong et al. (2020) designed a high-sensitivity colorimetric aptasensor using HRP-catalyzed TMB and hydrogen peroxide systems as outcome signals for VEGF-165 detection in human serum. The strategy achieved a low detection limit of 10 pg/mL and had a broad linear range of 0.1–100 ng/mL (Dong et al., 2020).
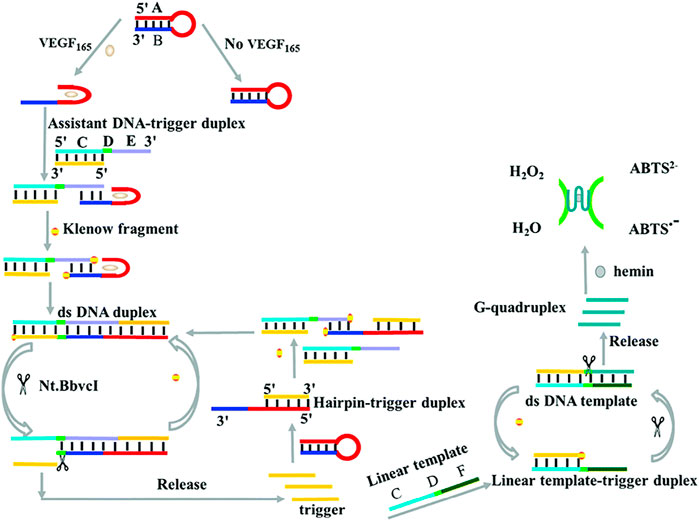
SCHEME 4. Illustration of VEGF-165 detection based on a colorimetric biosensor (Zhang et al., 2017).
2.3 Chemiluminescence aptasensors
A phenomenon where the light emitted by a substance does not result from heat is called luminescence, including chemiluminescence (CL) and electrochemiluminescence (ECL). CL is a photoradiation phenomenon that occurs during a chemical reaction. C-based strategies are widely applied in developing aptasensors employed in cancer biomarker detection owing to their relatively high sensitivity, wide calibration ranges, and repeatability (Eivazzadeh-Keihan et al., 2017; Kou et al., 2020). The monitoring of VEGF based on CL development has been studied extensively.
The luminol reaction with H2O2 is one of the most commonly used and reliable CL reactions (Khan et al., 2014). Li W. et al. (2015) designed a chemiluminescence aptasensor based on the aptamer-controlled non-covalent porphyrin probe self-assembly for VEGF detection (Scheme 5). A positively charged porphyrin probe (Mn-PyP) as a catalyst could catalyze the luminol CL reaction. Without VEGF, the Mn-PyP catalyzed CL reaction is efficiently suppressed because of the VEGF aptamer-induced aggregation of Mn-PyP. Therefore, the target turns on the CL reaction because of the high affinity between VEGF and the VEGF aptamer. The detection limit of the method for VEGF is 50 pM, and the concentration ranges from 0 to 25 nM (Li W. et al., 2015). Wang J. et al. (2022) detected AFP based on the CL system of hemin@ZIF-67–H2O2-NaOH, achieving a detection limit of 0.13 ng/mL. In a study conducted by Han R. et al. (2020), a sensing platform based on the luminol–H2O2–metal organic framework (MOF) system was constructed for AFP detection. The aptasensor had an LOD of 77 pg/L for AFP (Han R. et al., 2020).
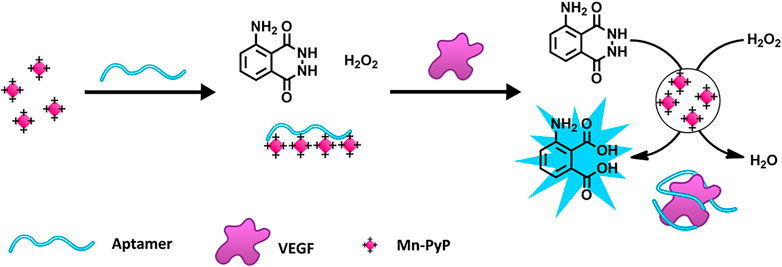
SCHEME 5. Schematic Illustration of the porphyrin probe (Mn-PyP) based on a vascular endothelial growth factor (VEGF) sensing strategy (Li W. et al., 2015). Reprinted (adapted) with permission from Li W. et al. (2015). Copyright 2015 © American Chemical Society.
Another common reaction used for CL is the hydrolysis of 4-methoxy-4-(3-phosphatephenyl)-spiro-(1,2-dioxetane-3,2-adamantane; AMPPD) under the catalysis of alkaline phosphatase (ALP) (Li et al., 2010; Yue and Liu, 2013; Fan et al., 2021). Shan et al. (2017) developed a sensitive and selective CL method for quantitative VEGF-165 detection (Scheme 6). Considering the two binding domains in VEGF-165, the researchers designed a dual-aptamer-based sandwich structure, where one capture aptamer was immobilized on magnetic beads (MBs), and another aptamer was labeled by biotin for further ALP conjunction. After the target-induced Apt-VEGF-Apt sandwich formed on the surface of the MBs, ALP would bind to the second aptamer by primary magnetic separation to remove excess Apt-biotin. Subsequently, after secondary magnetic separation, ALP catalyzed AMPPD, and a strong chemiluminescence signal was produced. Notably, the method has been successfully applied to a hypoxic co-culture model with excellent accuracy, equal to that of an ELISA Kit (Shan et al., 2017).
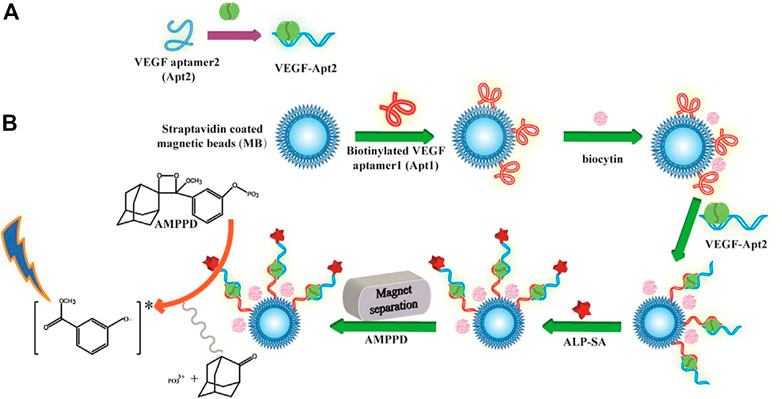
SCHEME 6. Schematic representation of the chemiluminescence aptasensor for the quantitative determination of VEGF-165 (Shan et al., 2017). (A) VEGF165 sample was incubated with Apt2 solution. (B) Apt1 immobilization on MBs and coupling with VEGF-Apt2 for following ALP-AMPPD CL detection.
2.4 Surface-enhanced Raman scattering-based aptasensors
The underlying principle of surface-enhanced Raman scattering (SERS) is that the Raman scattering intensity after molecular adsorption on the metal surface is improved, including electromagnetic enhancement (EM) and chemical enhancement (CM) (Ding et al., 2017; Mohammadpour et al., 2017; Joseph et al., 2018). Precious metal nanoparticles (i.e., Au or Ag NPs) are often used as probes to label SERS due to their plasmonic properties (Mosier-Boss, 2017). SERS has been applied extensively in biomedical research, clinical diagnosis, and food safety control (Jiang et al., 2015; Bryche et al., 2017).
Ning et al. (2020) developed a SERS-based aptasensor for the simultaneous sensitive detection of multiple cancer-related exosomes, including AFP (Scheme 7). They synthesized multiplexed capture probes composed of bumpy surface nanorod (gold nanotrepang, GNT) cores and bilayer silver shells. The three types of gold-silver-silver core shell-shell nanotrepangs (GSSNTs) were decorated on the MBs via the functionalization of linker DNAs, which were complementary to the aptamers for the targets. In the absence of the target, SERS detection probes were coupled with MBs via specific DNA hybridization as an aptamer-based SERS sensor. In the presence of the target, the aptamer specifically captured the target, and GSSNTs were subsequently released into the supernatant resulting in attenuated SERS signals. The quantitative detection of every single target and multiplex assays renders this sensor highly promising in clinical diagnosis (Ning et al., 2020).
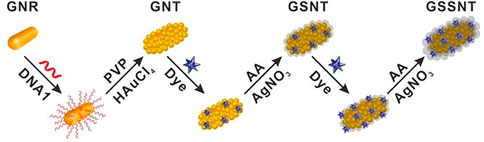
SCHEME 7. Schematic illustration of the fabrication of Surface-enhanced Raman scattering (SERS)-active nanotags (Ning et al., 2020).
Zhao et al. (2015) demonstrated the fabrication of a novel 6-nm Ag ornamented–10 nm Au nanoparticle pyramid-like superstructure (Ag–Au Pys), in which the number of hotspots between silver nanoparticles (AgNPs) and AuNPs had a good relationship with the SERS intensity (Scheme 8). The investigators used the aptamer for VEGF and its partially complementary sequence to assemble Ag–Au Pys nanostructures under optimized conditions. The detection limits of VEGF decreased to 22.6 aM within a wide linear range of the VEGF concentration of approximately 0.01–1.0 fM (Zhao et al., 2015).
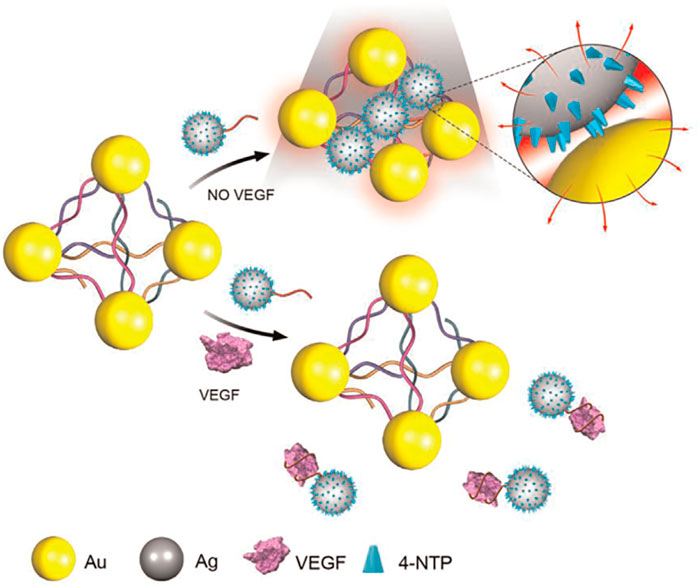
SCHEME 8. Schematic illustration for Surface-enhanced Raman scattering (SERS) assay detection of Vascular Endothelial Growth Factor (VEGF) based on self-assembled Ag ornamented–Au pyramid superstructure (Zhao et al., 2015).
2.5 Surface plasmon resonance-based aptasensors
Surface plasmon resonance (SPR) is a sensitive surface analysis technology that detects changes in the refractive index when molecular adsorption occurs on heavy metal membranes (Nguyen et al., 2015). SPR has been used widely for studies of biomolecular interactions since the 1990s. However, routine SPR testing cannot detect small changes in refractive coefficient, limiting its application in hypersensitivity detection. Therefore, SPR techniques have been developed with amplification methods.
Chen and his team described a rolling circle amplification (RCA)-assisted SPR sensor for detecting VEGF (Scheme 9). In the strategy, two aptamers and the primer that possessed the complementary sequence of the padlock probes were used to initiate the RCA reactions. The 30-NH2 Aptamer2 and 30-NH2 primer were loaded on carboxyl-coated polystyrene microspheres through amidation. Aptamer1 was immobilized on the gold surface to bind VEGF. To obtain a well-aligned Aptamer1, monolayers of mercaptohexanol were modified to fill the gold chip to prevent non-specific binding. When the target activated the sensor, it indirectly triggered the RCA reactions. With the help of ligase, the RCA is performed under the phi29 polymerase and dNTPs. Therefore, VEGF can be monitored very sensitively and selectively using this RCA-assisted SPR sensor. With the sensitive and selective platform, VEGF can be monitored in a linear range of 100 pg/mL–1 μg/mL, with a detection limit of 100 pg/mL (Chen et al., 2014).
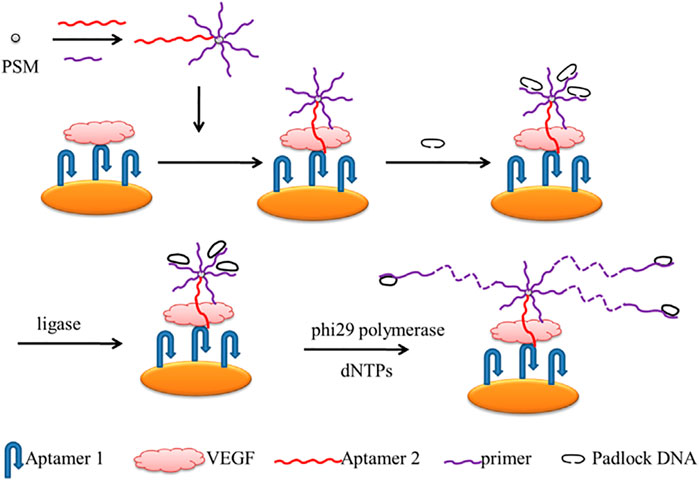
SCHEME 9. Scheme of the Rolling Circle Amplification (RCA)-assisted Surface Plasmon Resonance (SPR) sensing platform (Chen et al., 2014).
Based on the SPR mechanism, Cennamo et al. developed a rapid, portable, and low-cost diagnostic platform taking advantage of a plastic optical fiber (POF) to detect VEGF (Scheme 10). To achieve good sensitivity and signal-to-noise ratio, the preparation process is complex. In brief, the POF was first designed as a PMMA core of 980 μm with a fluorinated polymer cladding of 20 μm. In addition, the coating of the POF was removed along half of the circumference, and the Micropotential S1813 photoresist buffer was rotated on the exposed core and finally sputtered with a thin gold fiber film using a sputtering machine. Researchers also compared two different types of modification on the surface of the gold fiber film; one immobilized with aptamer only (Apt) and one with aptamer and passivating agent (mercaptoethanol) (Apt-MPET). The results indicated that a better interface was fabricated when the passivation molecule was fixed on the sensor surface after the aptamer (Apt-MPET). The sensitivity was about 8.3 times superior to that of the non-passivated sensor, and the detection limits were 0.8 nM and 3 nM, respectively. However, the passivation process needs to be optimized further to enhance the affinity and kinetic properties of the aptamer. For example, using the method mentioned by Cennamo et al., adding a polyethylene glycol (PEG) layer between the gold fiber layer and the aptamer layer can reduce non-specific adsorption and result in a partial loss of molecule affinity for the target. The underlying mechanism may be the spatial repulsion generated by the high affinity and compression of the PEG chain (Cennamo et al., 2015).
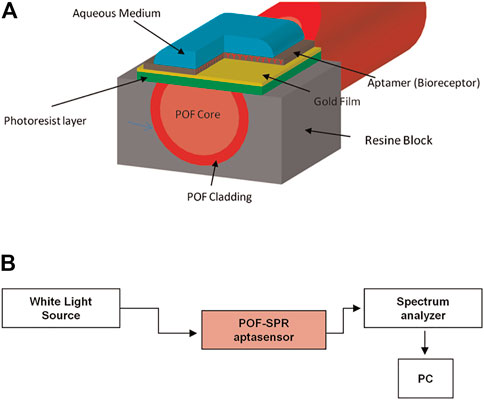
SCHEME 10. (A) Schematic illustration of the Plastic Optical Fiber-Surface Plasmon Resonance (POF-SPR) aptasensor, with a cross-section of the sensor system. (B) Experimental setup (Cennamo et al., 2015).
In addition, Liu and his colleagues devised a novel, enzyme-free, and versatile SPR sensor for detecting microRNA-21 and the human HCC cell line SMMC-7721. Their SPR aptasensor achieved a significantly enhanced SPR response using multiple signal amplification strategies where the target triggered the formation of a DNA super sandwich structure, and numerous positively charged AgNPs were absorbed (Liu et al., 2017).
Although AFP, as a star marker, has currently been utilized in clinical settings for the detection of various cancers, including HCC, gastric cancer, and breast cancer (Sun et al., 2017; Zhan et al., 2022), to date, there is no SPR aptasensor for AFP.
2.6 Other optical aptasensors
2.6.1 Electrochemiluminescence aptasensors
The ECL technique combines the advantages and merits of the luminescence assay with the merits of electrochemical analysis, which has attracted considerable attention in developing ultrasensitive biosensing platforms for biomarker detection (Han et al., 2020; Hiramoto et al., 2020). Wu et al. (2014) developed an ECL imaging strategy for the simultaneous detection of multiple cancer biomarkers, including AFP, based on a closed bipolar electrode array (Wu et al., 2015). In addition, Zhang et al. (2015) described an ECL method based on DNA aptamer–target recognition and T7 exonuclease-assisted cycling signal amplification for VEGF-165 detection. Consequently, the ECL-based aptasensor had a detection limit of 0.2 pM within a wide range of VEGF-165 concentrations of 1 pM–20 nM (Zhang et al., 2015).
2.6.2 Resonance light scattering aptasensor
The resonance scattering spectral (RLS) method has also been applied in biomarker aptasensors due to its simplification and rapidness (Gong et al., 2020). Based on the RLS technique, Chen et al. (2018) designed an intelligent and label-free sensor for the detection of AFP and miRNA-122 simultaneously. In the present study, cDNA1, which was part of the AFP aptamer strand region, hybridized with cDNA2, which was part of the region completely complementary to miRNA-122, to form double-stranded DNA. When the target (AFP or miRNA-122) was present, the cDNA (cDNA1 or cDNA2) could bind to the target. Therefore, RLS intensity changed proportionally with the concentration of AFP or miRNA-122. The proposed biosensor had detection limits as low as 0.94 μg/L and 98 pM for AFP and miRNA-122, respectively (Chen et al., 2018).
2.6.3 Liquid crystals aptasensors
Liquid crystal (LC) sensors achieve target detection by transducing and amplifying chemical and biological events into visible optical signals. In the LC sensor by Duong and Jang (2021), the aptamer was immobilized on the surface of substrates, and then the binding of the AFP to its aptamer disrupted the LC alignment to generate the detection signal. The quantification limit of AFP achieved was 12.62 pg/mL (Duong and Jang, 2021). Qi et al. (2020) designed a platform where the two aptamers for AFP captured targets synergistically to format sandwich complexes of apt1/protein/apt2, which induced the release of signal DNA into the aqueous solution (Qi et al., 2020a).
3 Conclusion and future perspectives
In recent years, the global incidence and mortality of HCC have been on the rise. This review summarizes the development of optical aptasensors for biomarkers in the surveillance and early diagnosis of HCC. Each aptasensor based on an optical analysis strategy has its unique characteristics. Fluorescent aptasensors, one of the most prevalent sensors, can detect a large number of targets, whether in solution or on the membrane. However, there is a stability challenge referred to as photobleaching, which can affect the long-term usability of the sensor. Colorimetric aptasensors represent the simplest sensing mode, as they translate invisible signals into megascopic color changes. Based on this advantage, the colorimetric method has the most potential for commercial application outside the laboratory. Furthermore, the main challenge of such a type of sensor is that they have high detection limits compared to other methods. Although, as the signal amplification strategies progress, the problem is being resolved. Another optical-based strategy, chemiluminescence, has been exploited widely in recent years due to high sensitivity, wide calibration ranges, and simple instrumentation. Other optical analysis methods have also been recommended, including SPR, SERS, electrochemiluminescence, and resonance light scattering.
Studies associated with HCC based on optical aptasensors are disproportionate. VEGF is a prognostic monitoring marker for HCC and also a biomarker of multiple cancers (Volk et al., 2007); therefore, the detection of VEGF has been well studied. In contrast, there is a lack of optical aptasensors for detecting several commonly used serum biomarkers for HCC surveillance, such as DCP, Gp-73, IGF-1, and even AFP-L3, which is slightly more sensitive than AFP for HCC diagnosis in a biomarker screening test (Shiraki et al., 1995). The possible reason is that no corresponding high specificity and high-affinity aptamers for such markers have been selected. Additionally, although SPR is a widely used and highly sensitive detection method, to date, there is still not an aptasensor-based SPR for the star marker AFP.
Despite the considerable advantages of aptamer sensors over traditional methods in clinical analysis, some major challenges still warrant further investigation. Notably, defining biomarkers for diagnosing early HCC with better specificity and sensitivity scores than existing ones remains a challenge. In addition, despite the considerable specificity of aptamers in experimental studies, the detection accuracy of aptasensors is associated with false negatives or false positives in practical applications under the influence of complex matrixes of the samples. Consequently, few optical aptasensors have been made commercially available compared to the expansive academic literature in the area. Furthermore, the high development cost limits commercialization. Although the cost of aptamers is much lower than that of antibodies, there are still some enzymatic reactions and nanomaterials involved in aptasensors that increase their costs. Therefore, researchers should commit to producing commercial biosensors, which could facilitate the establishment of a clear consensus on HCC diagnosis and enhance visible light application. Finally, in cases where clinical studies are temporarily unable to provide a specific diagnostic marker, identifying multiple biomarkers simultaneously can improve detection efficiency and minimize false diagnosis cases. This will require the development and integration of more highly specific aptamers into the sensor platforms, posing a challenge to the specificity of the aptamer.
In view of the above challenges, future opportunities, and research directions are as follows:
First, the time is right for robust biomarker validation. Specifically, comparisons between early HCC patients and high-risk individuals with cirrhosis or with non-alcoholic fatty liver disease, rather than any stage HCC and the healthy subjects, are required. Besides, standardized collection, processing, storage, and analysis methods need to be established to ensure the consistency of clinical application. Prediction models combining multiple biomarkers with other parameters (such as sex and age) will emerge as trends.
Next, valuable biomarkers will naturally attract researchers’ interest with regard to the screening of their specific adapters. The aptamer with good selectivity and specificity is one of the keys to the performance of the aptamer sensor.
With the continuous optimization of functionalized nanomaterials and the increasing synergy with aptamers in the sensing fields, aptasensors can solve the bottleneck issue in detecting biomolecules with low abundance and monitoring ultra-weak biological signals in samples with complex stroma. For instance, molecular-imprinted polymers (MIPs) can provide rebinding interactions and spatial structures complementary to the target (Zhang et al., 2019). Numerous recent studies have used the apt-MIP hybrid probe to improve aptamer stability and strengthen the specificity of the probe. Integrating MIP-aptamers may be ideal candidates for novel hybrid nanoprobes with high specificity and affinity compared to other multi-hybrid composites. In addition, single-atom nanozymes (Wu et al., 2020) that contain maximum atomic utilization features and exhibit a highly assembled enzyme-like structure and remarkable enzyme-like activity (Liu D. et al., 2019; Lyu et al., 2020) enable signal amplification strategy already holds great promise in biosensors and shows satisfactory sensitivity and selectivity in biosensing (Jiao et al., 2021). In summary, considering the versatility of sensing substrates, the expansion of functionalized nanomaterials, the development of lab-on-a-chip and 3D printed devices, as well as microfluid-based nano aptamer sensors will advance and offer novel perspectives on the challenges of aptasensors in point-of-care diagnostics.
With advances in clinical research, nanotechnology, and signal amplification methods, and the tireless efforts of researchers, great strides will be made with regard to optical aptasensors, enabling the accurate and early diagnosis of liver and other cancers, providing patients with earlier diagnosis and longer survival probability.
Author contributions
J-MD and R-QW wrote the manuscript and generated figures. J-MD, N-NY, J-HG, LX, X-YY, A-HP, and J-YC contributed to the concept and design and critically edited the manuscript. Z-LZ, Y-HS, and Y-YC performed critical revision and editing of the scientific content. All authors contributed to the article and approved the submitted version.
Funding
This work was supported by the Guangdong Basic and Applied Basic Research Foundation (2020A1515110057), The Xiangshan Talented Scientific Research Foundation of Zhuhai People’s Hospital (2020XSYC-07, 2021XSYC-02, 2020XSYC-10, 2020XSYC-03), and National Natural Science Foundation of China (32100561, 32100447).
Conflict of interest
The authors declare that the research was conducted in the absence of any commercial or financial relationships that could be construed as a potential conflict of interest.
Publisher’s note
All claims expressed in this article are solely those of the authors and do not necessarily represent those of their affiliated organizations, or those of the publisher, the editors and the reviewers. Any product that may be evaluated in this article, or claim that may be made by its manufacturer, is not guaranteed or endorsed by the publisher.
References
Akshaya, K., Arthi, C., Pavithra, A. J., Poovizhi, P., Antinate, S. S., Hikku, G. S., et al. (2020). Bioconjugated gold nanoparticles as an efficient colorimetric sensor for cancer diagnostics. Photodiagnosis Photodyn. Ther. 30, 101699. doi:10.1016/j.pdpdt.2020.101699
Bao, B., Su, P., Zhu, J., Chen, J., Xu, Y., Gu, B., et al. (2018). Rapid aptasensor capable of simply detect tumor markers based on conjugated polyelectrolytes. Talanta 190, 204–209. doi:10.1016/j.talanta.2018.07.072
Baryeh, K., Takalkar, S., Lund, M., and Liu, G. (2017). Development of quantitative immunochromatographic assay for rapid and sensitive detection of carbohydrate antigen 19-9 (CA 19-9) in human plasma. J. Pharm. Biomed. Analysis 146, 285–291. doi:10.1016/j.jpba.2017.09.004
Benson, A. B., D’Angelica, M. I., Abbott, D. E., Anaya, D. A., Anders, R., Are, C., et al. (2021). Hepatobiliary cancers, version 2.2021, NCCN clinical practice guidelines in oncology. J. Natl. Compr. Cancer Netw. 19, 541–565. doi:10.6004/jnccn.2021.0022
Best, J., Bechmann, L. P., Sowa, J.-P., Sydor, S., Dechêne, A., Pflanz, K., et al. (2020). GALAD score detects early hepatocellular carcinoma in an international cohort of patients with nonalcoholic steatohepatitis. Clin. Gastroenterology Hepatology 18, 728–735.e4. doi:10.1016/j.cgh.2019.11.012
Bhalla, N., Jolly, P., Formisano, N., and Estrela, P. (2016). Introduction to biosensors. Essays Biochem. 60, 1–8. doi:10.1042/ebc20150001
Bryche, J.-F., Bélier, B., Bartenlian, B., and Barbillon, G. (2017). Low-cost SERS substrates composed of hybrid nanoskittles for a highly sensitive sensing of chemical molecules. Sensors Actuators B Chem. 239, 795–799. doi:10.1016/j.snb.2016.08.049
Cabiati, M., Di Giorgi, N., Salvadori, C., Finamore, F., Del Turco, S., Cecchettini, A., et al. (2022). Transcriptional level evaluation of osteopontin/miRNA-181a axis in hepatocellular carcinoma cell line-secreted extracellular vesicles. Pathology - Res. Pract. 238, 154088. doi:10.1016/j.prp.2022.154088
Cao, L., Cheng, L., Zhang, Z., Wang, Y., Zhang, X., Chen, H., et al. (2012). Visual and high-throughput detection of cancer cells using a graphene oxide-based FRET aptasensing microfluidic chip. Lab a Chip 12, 4864–4869. doi:10.1039/c2lc40564d
Cennamo, N., Pesavento, M., Lunelli, L., Vanzetti, L., Pederzolli, C., Zeni, L., et al. (2015). An easy way to realize SPR aptasensor: A multimode plastic optical fiber platform for cancer biomarkers detection. Talanta 140, 88–95. doi:10.1016/j.talanta.2015.03.025
Chang, C.-C., Chen, C.-Y., Chuang, T.-L., Wu, T.-H., Wei, S.-C., Liao, H., et al. (2016). Aptamer-based colorimetric detection of proteins using a branched DNA cascade amplification strategy and unmodified gold nanoparticles. Biosens. Bioelectron. 78, 200–205. doi:10.1016/j.bios.2015.11.051
Chen, F., Zhang, F., Liu, Y., and Cai, C. (2018). Simply and sensitively simultaneous detection hepatocellular carcinoma markers AFP and miRNA-122 by a label-free resonance light scattering sensor. Talanta 186, 473–480. doi:10.1016/j.talanta.2018.04.060
Chen, H., Hou, Y., Qi, F., Zhang, J., Koh, K., Shen, Z., et al. (2014). Detection of vascular endothelial growth factor based on rolling circle amplification as a means of signal enhancement in surface plasmon resonance. Biosens. Bioelectron. 61, 83–87. doi:10.1016/j.bios.2014.05.005
Chen, P., Jiang, P., Lin, Q., Zeng, X., Liu, T., Li, M., et al. (2022). Simultaneous homogeneous fluorescence detection of AFP and GPC3 in hepatocellular carcinoma clinical samples assisted by enzyme-free catalytic hairpin assembly. ACS Appl. Mater. Interfaces 14, 28697–28705. doi:10.1021/acsami.2c09135
Chen, X., Gole, J., Gore, A., He, Q., Lu, M., Min, J., et al. (2020). Non-invasive early detection of cancer four years before conventional diagnosis using a blood test. Nat. Commun. 11, 3475. doi:10.1038/s41467-020-17316-z
Cho, H., Yeh, E.-C., Sinha, R., Laurence, T. A., Bearinger, J. P., and Lee, L. P. (2012). Single-step nanoplasmonic VEGF165Aptasensor for early cancer diagnosis. ACS Nano 6, 7607–7614. doi:10.1021/nn203833d
Choi, J., Kim, G., Han, S., Lee, W., Chun, S., and Lim, Y. (2019). Longitudinal assessment of three serum biomarkers to detect very early-stage hepatocellular carcinoma. Hepatology 69, 1983–1994. doi:10.1002/hep.30233
Colli, A., Nadarevic, T., Miletic, D., Giljaca, V., Fraquelli, M., Štimac, D., et al. (2021). Abdominal ultrasound and alpha-foetoprotein for the diagnosis of hepatocellular carcinoma in adults with chronic liver disease. Cochrane Database Syst. Rev. 4, CD013346. doi:10.1002/14651858.cd013346.pub2
Cui, J., Kan, L., Li, Z., Yang, L., Wang, M., He, L., et al. (2021). Porphyrin-based covalent organic framework as bioplatfrom for detection of vascular endothelial growth factor 165 through fluorescence resonance energy transfer. Talanta 228, 122060. doi:10.1016/j.talanta.2020.122060
Dehghani, S., Nosrati, R., Yousefi, M., Nezami, A., Soltani, F., Taghdisi, S. M., et al. (2018). Aptamer-based biosensors and nanosensors for the detection of vascular endothelial growth factor (VEGF): A review. Biosens. Bioelectron. 110, 23–37. doi:10.1016/j.bios.2018.03.037
Ding, C., and Tian, Y. (2015). Gold nanocluster-based fluorescence biosensor for targeted imaging in cancer cells and ratiometric determination of intracellular pH. Biosens. Bioelectron. 65, 183–190. doi:10.1016/j.bios.2014.10.034
Ding, S.-Y., You, E.-M., Tian, Z.-Q., and Moskovits, M. (2017). Electromagnetic theories of surface-enhanced Raman spectroscopy. Chem. Soc. Rev. 46, 4042–4076. doi:10.1039/C7CS00238F
Dong, J., He, L., Wang, Y., Yu, F., Yu, S., Liu, L., et al. (2020). A highly sensitive colorimetric aptasensor for the detection of the vascular endothelial growth factor in human serum. Spectrochimica Acta Part A Mol. Biomol. Spectrosc. 226, 117622. doi:10.1016/j.saa.2019.117622
Du, L., Wang, M., Li, H., Li, N., and Wang, F. (2022). Identification of CCL20 and LCN2 as efficient serological tools for detection of hepatocellular carcinoma. Dis. Markers 2022, 7758735–7758737. doi:10.1155/2022/7758735
Duong, D. S. T., and Jang, C.-H. (2021). Highly sensitive label-free liquid crystal-based aptasensor to detect alpha-fetoprotein. Liq. Cryst. 49, 709–718. doi:10.1080/02678292.2021.2005165
Eivazzadeh-Keihan, R., Pashazadeh, P., Hejazi, M., de la Guardia, M., and Mokhtarzadeh, A. (2017). Recent advances in Nanomaterial-mediated Bio and immune sensors for detection of aflatoxin in food products. TrAC Trends Anal. Chem. 87, 112–128. doi:10.1016/j.trac.2016.12.003
El-Houseini, M. E., Mohammed, M. S., Elshemey, W. M., Hussein, T. D., Desouky, O. S., and Elsayed, A. A. (2005). Enhanced detection of hepatocellular carcinoma. Cancer 12, 248–253. doi:10.1177/107327480501200407
Elmashad, N., Ibrahim, W. S., Mayah, W. W., Farouk, M., AboAli, L., Taha, A., et al. (2015). Predictive value of serum insulin-like growth factor-1 in hepatocellular carcinoma. Asian Pac. J. Cancer Prev. 16, 613–619. doi:10.7314/apjcp.2015.16.2.613
Fan, N., Li, P., Wu, C., Wang, X., Zhou, Y., and Tang, B. (2021). ALP-activated chemiluminescence PDT nano-platform for liver cancer-specific theranostics. ACS Appl. Bio Mater. 4, 1740–1748. doi:10.1021/acsabm.0c01504
Farag, R. M. A., Al Ayobi, D., Alsaleh, K. A., Kwon, H.-J., El-Ansary, A., and Dawoud, E. A. (2019). Studying the impact of Golgi protein 73 serving as a candidate biomarker in early diagnosis for hepatocellular carcinoma among Saudi patients. Asian Pac. J. Cancer Prev. 20, 215–220. doi:10.31557/apjcp.2019.20.1.215
Feng, C., Dai, S., and Wang, L. (2014). Optical aptasensors for quantitative detection of small biomolecules: A review. Biosens. Bioelectron. 59, 64–74. doi:10.1016/j.bios.2014.03.014
Freeman, R., Girsh, J., Fang-ju Jou, A., Ho, J. A., Hug, T., Dernedde, J., et al. (2012). Optical aptasensors for the analysis of the vascular endothelial growth factor (VEGF). Anal. Chem. 84, 6192–6198. doi:10.1021/ac3011473
Gong, L. J., Li, Y. F., Zou, H. Y., and Huang, C. Z. (2020). Resonance light scattering technique for sensitive detection of heparin using plasmonic Cu2-xSe nanoparticles. Talanta 216, 120967. doi:10.1016/j.talanta.2020.120967
Han, R., Sun, Y., Lin, Y., Liu, H., Dai, Y., Zhu, X., et al. (2020a). A simple chemiluminescent aptasensor for the detection of α-fetoprotein based on iron-based metal organic frameworks. New J. Chem. 44, 4099–4107. doi:10.1039/c9nj05870b
Han, S., Zhao, Y., Zhang, Z., and Xu, G. (2020b). Recent advances in electrochemiluminescence and chemiluminescence of metal nanoclusters. Molecules 25, 5208. doi:10.3390/molecules25215208
Hanif, H., Ali, M. J., Susheela, A. T., Khan, I. W., Luna-Cuadros, M. A., Khan, M. M., et al. (2022). Update on the applications and limitations of alpha-fetoprotein for hepatocellular carcinoma. World J. Gastroenterology 28, 216–229. doi:10.3748/wjg.v28.i2.216
Hiramoto, K., Villani, E., Iwama, T., Komatsu, K., Inagi, S., Inoue, K., et al. (2020). Recent advances in electrochemiluminescence-based systems for mammalian cell analysis. Micromachines 11, 530. doi:10.3390/mi11050530
Hosseinzadeh, L., and Mazloum-Ardakani, M. (2020). Advances in aptasensor technology. Adv. Clin. Chem. 99, 237–279. doi:10.1016/bs.acc.2020.02.010
Huang, L., Zhang, Z., and Li, G. (2022). DNA strand displacement based surface-enhanced Raman scattering-fluorescence dual-mode nanoprobes for quantification and imaging of vascular endothelial growth factor in living cells. Biosens. Bioelectron. 204, 114069. doi:10.1016/j.bios.2022.114069
Huang, Y., Wen, Y., Baryeh, K., Takalkar, S., Lund, M., Zhang, X., et al. (2017). Lateral flow assay for carbohydrate antigen 19–9 in whole blood by using magnetized carbon nanotubes. Microchim. Acta 184, 4287–4294. doi:10.1007/s00604-017-2464-0
Ibau, C., Md Arshad, M. K., Gopinath, S. C. B., Nuzaihan, M. N, M., Fathil, M., and Estrela, P. (2019). Gold interdigitated triple-microelectrodes for label-free prognosticative aptasensing of prostate cancer biomarker in serum. Biosens. Bioelectron. 136, 118–127. doi:10.1016/j.bios.2019.04.048
Ji, J., Wang, H., Li, Y., Zheng, L., Yin, Y., Zou, Z., et al. (2016). Diagnostic evaluation of des-gamma-carboxy prothrombin versus α-fetoprotein for hepatitis B virus-related hepatocellular carcinoma in China: A large-scale, multicentre study. PLOS ONE 11, e0153227. doi:10.1371/journal.pone.0153227
Jia, Y., Zhou, G., Wang, X., Zhang, Y., Li, Z., Liu, P., et al. (2020). A metal-organic framework/aptamer system as a fluorescent biosensor for determination of aflatoxin B1 in food samples. Talanta 219, 121342. doi:10.1016/j.talanta.2020.121342
Jiang, T., Zhang, L., Jin, H., Wang, X., and Zhou, J. (2015). In situ controlled sputtering deposition of gold nanoparticles on MnO2 nanorods as surface-enhanced Raman scattering substrates for molecular detection. Dalton Trans. 44, 7606–7612. doi:10.1039/c4dt03774j
Jiang, Y., Tang, Y., and Miao, P. (2019). Polydopamine nanosphere@silver nanoclusters for fluorescence detection of multiplex tumor markers. Nanoscale 11, 8119–8123. doi:10.1039/c9nr01307e
Jiao, L., Xu, W., Wu, Y., Yan, H., Gu, W., Du, D., et al. (2021). Single-atom catalysts boost signal amplification for biosensing. Chem. Soc. Rev. 50, 750–765. doi:10.1039/d0cs00367k
Joseph, M. M., Narayanan, N., Nair, J. B., Karunakaran, V., Ramya, A. N., Sujai, P. T., et al. (2018). Exploring the margins of SERS in practical domain: An emerging diagnostic modality for modern biomedical applications. Biomaterials 181, 140–181. doi:10.1016/j.biomaterials.2018.07.045
Kaur, A., Kaur, P., and Ahuja, S. (2021). Correction: Förster resonance energy transfer (FRET) and applications thereof. Anal. Methods 13, 730. doi:10.1039/d1ay90011k
Khan, P., Idrees, D., Moxley, M. A., Corbett, J. A., Ahmad, F., von Figura, G., et al. (2014). Luminol-based chemiluminescent signals: Clinical and non-clinical application and future uses. Appl. Biochem. Biotechnol. 173, 333–355. doi:10.1007/s12010-014-0850-1
Kou, X., Zhang, X., Shao, X., Jiang, C., and Ning, L. (2020). Recent advances in optical aptasensor technology for amplification strategies in cancer diagnostics. Anal. Bioanal. Chem. 412, 6691–6705. doi:10.1007/s00216-020-02774-7
Lee, K.-A., Ahn, J.-Y., Lee, S.-H., Singh Sekhon, S., Kim, D.-G., Min, J., et al. (2015). Aptamer-based sandwich assay and its clinical outlooks for detecting lipocalin-2 in hepatocellular carcinoma (HCC). Sci. Rep. 5, 10897. doi:10.1038/srep10897
Li, G., Zeng, J., Liu, H., Ding, P., Liang, J., Nie, X., et al. (2019). A fluorometric aptamer nanoprobe for alpha-fetoprotein by exploiting the FRET between 5-carboxyfluorescein and palladium nanoparticles. Microchim. Acta 186, 314. doi:10.1007/s00604-019-3403-z
Li, H.-Y., Yin, F.-F., Li, X.-Y., Jia, W.-N., Ding, J., Zhang, L., et al. (2021a). Novel aptasensor-based assay of sonic hedgehog ligand for detection of portal vein invasion of hepatocellular carcinoma. Biosens. Bioelectron. 174, 112738. doi:10.1016/j.bios.2020.112738
Li, J., Sun, K., Chen, Z., Shi, J., Zhou, D., and Xie, G. (2017). A fluorescence biosensor for VEGF detection based on DNA assembly structure switching and isothermal amplification. Biosens. Bioelectron. 89, 964–969. doi:10.1016/j.bios.2016.09.078
Li, S., Liu, X., Liu, S., Guo, M., Liu, C., and Pei, M. (2021b). Fluorescence sensing strategy based on aptamer recognition and mismatched catalytic hairpin assembly for highly sensitive detection of alpha-fetoprotein. Anal. Chim. Acta 1141, 21–27. doi:10.1016/j.aca.2020.10.030
Li, W., Zhang, Q., Zhou, H., Chen, J., Li, Y., Zhang, C., et al. (2015a). Chemiluminescence detection of a protein through the aptamer-controlled catalysis of a porphyrin probe. Anal. Chem. 87, 8336–8341. doi:10.1021/acs.analchem.5b01511
Li, X., Ding, X., and Fan, J. (2015b). Nicking endonuclease-assisted signal amplification of a split molecular aptamer beacon for biomolecule detection using graphene oxide as a sensing platform. Analyst 140, 7918–7925. doi:10.1039/c5an01759a
Li, Z., He, L., He, N., Shi, Z., Wang, H., Li, S., et al. (2010). Chemiluminescent detect of E. coli O157:H7 using immunological method based on magnetic nanoparticles. J. Nanosci. Nanotechnol. 10, 696–701. doi:10.1166/jnn.2010.1811
Lim, T. S., Kim, D. Y., Han, K.-H., Kim, H.-S., Shin, S. H., Jung, K. S., et al. (2015). Combined use of AFP, PIVKA-II, and AFP-L3 as tumor markers enhances diagnostic accuracy for hepatocellular carcinoma in cirrhotic patients. Scand. J. Gastroenterology 51, 344–353. doi:10.3109/00365521.2015.1082190
Lim, Y. C., Kouzani, A. Z., and Duan, W. (2010). Aptasensors: A review. J. Biomed. Nanotechnol. 6, 93–105. doi:10.1166/jbn.2010.1103
Liu, D., Li, J.-C., Shi, Q., Feng, S., Lyu, Z., Ding, S., et al. (2019a). Atomically isolated iron atom anchored on carbon nanotubes for oxygen reduction reaction. ACS Appl. Mater. Interfaces 11, 39820–39826. doi:10.1021/acsami.9b12054
Liu, H., Li, P., Zhai, Y., Qu, C. F., Zhang, L. J., Tan, Y. F., et al. (2010). Diagnostic value of glypican-3 in serum and liver for primary hepatocellular carcinoma. World J. Gastroenterology 16, 4410–4415. doi:10.3748/wjg.v16.i35.4410
Liu, M.-Y., Huang, L., Wu, J.-F., Zhang, H.-B., Ai, W.-B., and Zhang, R.-T. (2022). Possible roles of Golgi protein-73 in liver diseases. Ann. Hepatology 27, 100720. doi:10.1016/j.aohep.2022.100720
Liu, M., Wu, R., Liu, X., Xu, H., Chi, X., Wang, X., et al. (2020). Validation of the GALAD model and establishment of GAAP model for diagnosis of hepatocellular carcinoma in Chinese patients. J. Hepatocell. Carcinoma 7, 219–232. doi:10.2147/jhc.s271790
Liu, R., Wang, Q., Li, Q., Yang, X., Wang, K., and Nie, W. (2017). Surface plasmon resonance biosensor for sensitive detection of microRNA and cancer cell using multiple signal amplification strategy. Biosens. Bioelectron. 87, 433–438. doi:10.1016/j.bios.2016.08.090
Liu, X.-N., Cui, D.-N., Li, Y.-F., Liu, Y.-H., Liu, G., and Liu, L. (2019b). Multiple “Omics” data-based biomarker screening for hepatocellular carcinoma diagnosis. World J. Gastroenterology 25, 4199–4212. doi:10.3748/wjg.v25.i30.4199
Lu, S., Wang, S., Zhao, J., Sun, J., and Yang, X. (2018). Classical triplex molecular beacons for MicroRNA-21 and vascular endothelial growth factor detection. ACS Sensors 3, 2438–2445. doi:10.1021/acssensors.8b00996
Lyman, D. F., Bell, A., Black, A., Dingerdissen, H., Cauley, E., Gogate, N., et al. (2022). Modeling and integration of N-glycan biomarkers in a comprehensive biomarker data model. Glycobiology 32 (10), 855–870. doi:10.1093/glycob/cwac046
Lyu, Z., Ding, S., Zhang, N., Zhou, Y., Cheng, N., Wang, M., et al. (2020). Single-atom nanozymes linked immunosorbent assay for sensitive detection of aβ 1-40: A biomarker of alzheimer’s disease. Research 2020, 4724505–4724511. doi:10.34133/2020/4724505
Marrero, J. A., Kulik, L. M., Sirlin, C. B., Zhu, A. X., Finn, R. S., Abecassis, M. M., et al. (2018). Diagnosis, staging, and management of hepatocellular carcinoma: 2018 practice guidance by the American association for the study of liver diseases. Hepatology 68, 723–750. doi:10.1002/hep.29913
Mazziotti, G., Sorvillo, F., Morisco, F., Carbone, A., Rotondi, M., Stornaiuolo, G., et al. (2002). Serum insulin-like growth factor I evaluation as a useful tool for predicting the risk of developing hepatocellular carcinoma in patients with hepatitis C virus-related cirrhosis: A prospective study. Cancer 95, 2539–2545. doi:10.1002/cncr.11002
Mohammadpour, M., Khodabandeh, M. H., Visscher, L., and Jamshidi, Z. (2017). Elucidation of charge-transfer SERS selection rules by considering the excited state properties and the role of electrode potential. Phys. Chem. Chem. Phys. 19, 7833–7843. doi:10.1039/c6cp07585a
Mosier-Boss, P. (2017). Review of SERS substrates for chemical sensing. Nanomaterials 7, 142. doi:10.3390/nano7060142
Mukama, O., Sinumvayo, J. P., Shamoon, M., Shoaib, M., Mushimiyimana, H., Safdar, W., et al. (2017). An update on aptamer-based multiplex system approaches for the detection of common foodborne pathogens. Food Anal. Methods 10, 2549–2565. doi:10.1007/s12161-017-0814-5
Mukama, O., Wu, W., Wu, J., Lu, X., Liu, Y., Liu, Y., et al. (2020). A highly sensitive and specific lateral flow aptasensor for the detection of human osteopontin. Talanta 210, 120624. doi:10.1016/j.talanta.2019.120624
Nguyen, H., Park, J., Kang, S., and Kim, M. (2015). Surface plasmon resonance: A versatile technique for biosensor applications. Sensors 15, 10481–10510. doi:10.3390/s150510481
Ning, C.-F., Wang, L., Tian, Y.-F., Yin, B.-C., and Ye, B.-C. (2020). Multiple and sensitive SERS detection of cancer-related exosomes based on gold–silver bimetallic nanotrepangs. Analyst 145, 2795–2804. doi:10.1039/C9AN02180A
Pasquardini, L., Pancheri, L., Potrich, C., Ferri, A., Piemonte, C., Lunelli, L., et al. (2015). SPAD aptasensor for the detection of circulating protein biomarkers. Biosens. Bioelectron. 68, 500–507. doi:10.1016/j.bios.2015.01.042
Pereira, A. C., Moreira, F. T. C., Rodrigues, L. R., and Sales, M. G. F. (2022). Paper-based aptasensor for colorimetric detection of osteopontin. Anal. Chim. Acta 1198, 339557. doi:10.1016/j.aca.2022.339557
Piñero, F., Dirchwolf, M., and Pessôa, M. G. (2020). Biomarkers in hepatocellular carcinoma: Diagnosis, prognosis and treatment response assessment. Cells 9, 1370. doi:10.3390/cells9061370
Qi, L., Liu, S., Jiang, Y., Lin, J.-M., Yu, L., and Hu, Q. (2020). Simultaneous detection of multiple tumor markers in blood by functional liquid crystal sensors assisted with target-induced dissociation of aptamer. Anal. Chem. 92, 3867–3873. doi:10.1021/acs.analchem.9b05317
Qi, P., Cheng, S., Wang, H., Li, N., Chen, Y., and Gao, C. (2011). Serum MicroRNAs as biomarkers for hepatocellular carcinoma in Chinese patients with chronic hepatitis B virus infection. PLoS ONE 6, e28486. doi:10.1371/journal.pone.0028486
Sadeghi, A. S., Ansari, N., Ramezani, M., Abnous, K., Mohsenzadeh, M., Taghdisi, S. M., et al. (2018). Optical and electrochemical aptasensors for the detection of amphenicols. Biosens. Bioelectron. 118, 137–152. doi:10.1016/j.bios.2018.07.045
Sajid, M., Kawde, A.-N., and Daud, M. (2015). Designs, formats and applications of lateral flow assay: A literature review. J. Saudi Chem. Soc. 19, 689–705. doi:10.1016/j.jscs.2014.09.001
Shan, S., He, Z., Mao, S., Jie, M., Yi, L., and Lin, J.-M. (2017). Quantitative determination of VEGF165 in cell culture medium by aptamer sandwich based chemiluminescence assay. Talanta 171, 197–203. doi:10.1016/j.talanta.2017.04.057
Shan, W., Pan, Y., Fang, H., Guo, M., Nie, Z., Huang, Y., et al. (2014). An aptamer-based quartz crystal microbalance biosensor for sensitive and selective detection of leukemia cells using silver-enhanced gold nanoparticle label. Talanta 126, 130–135. doi:10.1016/j.talanta.2014.03.056
Shiraki, K., Takase, K., Tameda, Y., Hamada, M., Kosaka, Y., and Nakano, T. (1995). A clinical study of lectin-reactive alpha-fetoprotein as an early indicator of hepatocellular carcinoma in the follow-up of cirrhotic patients. Hepatology 22, 802–807. doi:10.1002/hep.1840220317
Singal, A. G., Lampertico, P., and Nahon, P. (2020). Epidemiology and surveillance for hepatocellular carcinoma: New trends. J. Hepatology 72, 250–261. doi:10.1016/j.jhep.2019.08.025
Sun, T., Tang, Y., Sun, D., Bu, Q., and Li, P. (2018). Osteopontin versus alpha-fetoprotein as a diagnostic marker for hepatocellular carcinoma: A meta-analysis. OncoTargets Ther. 11, 8925–8935. doi:10.2147/ott.s186230
Sun, W., Liu, B., Chen, J., Gong, P., Wu, X., Liu, C., et al. (2017). Novel characteristics of alpha-fetoprotein (AFP)-producing gastric cancer. Oncotarget 8, 101944–101951. doi:10.18632/oncotarget.22109
Tayob, N., Christie, I., Richardson, P., Feng, Z., White, D. L., Davila, J., et al. (2019). Validation of the hepatocellular carcinoma early detection screening (HES) algorithm in a cohort of veterans with cirrhosis. Clin. Gastroenterology Hepatology 17, 1886–1893.e5. doi:10.1016/j.cgh.2018.12.005
Tsuchiya, N., Sawada, Y., Endo, I., Saito, K., Uemura, Y., and Nakatsura, T. (2015). Biomarkers for the early diagnosis of hepatocellular carcinoma. World J. Gastroenterology 21, 10573–10583. doi:10.3748/wjg.v21.i37.10573
Tzartzeva, K., Obi, J., Rich, N. E., Parikh, N. D., Marrero, J. A., Yopp, A., et al. (2018). Surveillance imaging and alpha fetoprotein for early detection of hepatocellular carcinoma in patients with cirrhosis: A meta-analysis. Gastroenterology 154, 1706–1718.e1. doi:10.1053/j.gastro.2018.01.064
Volk, M. L., Hernandez, J. C., Su, G. L., Lok, A. S., and Marrero, J. A. (2007). Risk factors for hepatocellular carcinoma may impair the performance of biomarkers: A comparison of AFP, DCP, and AFP-L3. Cancer Biomarkers 3, 79–87. doi:10.3233/cbm-2007-3202
Wang, C.-F., Wang, Z.-G., Sun, X.-Y., Chen, M.-J., and Lv, Y.-K. (2018a). An ultrasensitive fluorescent aptasensor for detection of cancer marker proteins based on graphene oxide–ssDNA. RSC Adv. 8, 41143–41149. doi:10.1039/c8ra08078j
Wang, D., Guo, R., Wei, Y., Zhang, Y., Zhao, X., and Xu, Z. (2018b). Sensitive multicolor visual detection of telomerase activity based on catalytic hairpin assembly and etching of Au nanorods. Biosens. Bioelectron. 122, 247–253. doi:10.1016/j.bios.2018.09.064
Wang, H., Liu, J., Chen, W., Na, J., Huang, Y., and Li, G. (2022a). A fluorescence aptasensor based on GSH@GQDs and RGO for the detection of Glypican-3. Spectrochimica Acta Part A Mol. Biomol. Spectrosc. 270, 120798. doi:10.1016/j.saa.2021.120798
Wang, J., Hou, Y., Sun, Y., Fang, F., Luo, C., and Wang, X. (2022b). A chemiluminescence aptasensor for sensitive detection of alpha-fetoprotein based on hemin@ZIF-67. Anal. Bioanal. Chem. 414, 4757–4765. doi:10.1007/s00216-022-04099-z
Wang, Q., Hu, Y., Jiang, N., Wang, J., Yu, M., and Zhuang, X. (2020). Preparation of aptamer responsive DNA functionalized hydrogels for the sensitive detection of α-fetoprotein using SERS method. Bioconjugate Chem. 31, 813–820. doi:10.1021/acs.bioconjchem.9b00874
Wang, Y., Gao, D., Zhang, P., Gong, P., Chen, C., Gao, G., et al. (2014). A near infrared fluorescence resonance energy transfer based aptamer biosensor for insulin detection in human plasma. Chem. Commun. 50, 811–813. doi:10.1039/c3cc47649a
Wu, D., Gao, T., Lei, L., Yang, D., Mao, X., and Li, G. (2016). Colorimetric detection of proteins based on target-induced activation of aptazyme. Anal. Chim. Acta 942, 68–73. doi:10.1016/j.aca.2016.09.010
Wu, M.-S., Liu, Z., Shi, H.-W., Chen, H.-Y., and Xu, J.-J. (2014). Visual electrochemiluminescence detection of cancer biomarkers on a closed bipolar electrode array chip. Anal. Chem. 87, 530–537. doi:10.1021/ac502989f
Wu, W., Huang, L., Wang, E., and Dong, S. (2020). Atomic engineering of single-atom nanozymes for enzyme-like catalysis. Chem. Sci. 11, 9741–9756. doi:10.1039/d0sc03522j
Wu, X., Fu, P., Ma, W., Xu, L., Kuang, H., and Xu, C. (2015). SERS-active silver nanoparticle trimers for sub-attomolar detection of alpha fetoprotein. RSC Adv. 5, 73395–73398. doi:10.1039/C5RA12629K
Xiao, L., Zhu, A., Xu, Q., Chen, Y., Xu, J., and Weng, J. (2017). Colorimetric biosensor for detection of cancer biomarker by Au nanoparticle-decorated Bi2Se3 nanosheets. ACS Appl. Mater. Interfaces 9, 6931–6940. doi:10.1021/acsami.6b15750
Xie, L., Cao, Y., Hu, F., Li, T., Wang, Q., and Gan, N. (2019). Microfluidic chip electrophoresis for simultaneous fluorometric aptasensing of alpha-fetoprotein, carbohydrate antigen 125 and carcinoembryonic antigen by applying a catalytic hairpin assembly. Microchim. Acta 186, 547. doi:10.1007/s00604-019-3594-3
Xu, J., Chen, W., Shi, M., Huang, Y., Fang, L., Zhao, S., et al. (2019). An aptamer-based four-color fluorometic method for simultaneous determination and imaging of alpha-fetoprotein, vascular endothelial growth factor-165, carcinoembryonic antigen and human epidermal growth factor receptor 2 in living cells. Microchim. Acta 186, 204. doi:10.1007/s00604-019-3312-1
Xu, K., Wu, C., Wang, Z., Wang, H., Yin, F., Li, W., et al. (2021). VEGF family gene expression as prognostic biomarkers for alzheimer’s disease and primary liver cancer. Comput. Math. Methods Med. 2021, 1–15. doi:10.1155/2021/3422393
Xu, S., Feng, X., Gao, T., Liu, G., Mao, Y., Lin, J., et al. (2017). Aptamer induced multicoloured Au NCs-MoS2 “switch on” fluorescence resonance energy transfer biosensor for dual color simultaneous detection of multiple tumor markers by single wavelength excitation. Anal. Chim. Acta 983, 173–180. doi:10.1016/j.aca.2017.06.023
Yan, X., Zhao, K., Yang, Y., Qiu, A., Zhang, X., Liu, J., et al. (2022). Utilizing dual carriers assisted by enzyme digestion chemiluminescence signal enhancement strategy simultaneously detect tumor markers CEA and AFP. Anal. Sci. 38, 889–897. doi:10.1007/s44211-022-00109-3
Yang, B., Chen, B., He, M., Yin, X., Xu, C., and Hu, B. (2018a). Aptamer-based dual-functional probe for rapid and specific counting and imaging of MCF-7 cells. Anal. Chem. 90, 2355–2361. doi:10.1021/acs.analchem.7b04927
Yang, B., Shi, L., Lei, J., Li, B., and Jin, Y. (2019a). Advances in optical assays for detecting telomerase activity. Luminescence 34, 136–152. doi:10.1002/bio.3595
Yang, H., Zhao, C., Li, R., Shen, C., Cai, X., Sun, L., et al. (2018b). Noninvasive and prospective diagnosis of coronary heart disease with urine using surface-enhanced Raman spectroscopy. Analyst 143, 2235–2242. doi:10.1039/c7an02022h
Yang, J., Zhang, L., Jiang, Z., Ge, C., Zhao, F., Jiang, J., et al. (2019b). TCF12 promotes the tumorigenesis and metastasis of hepatocellular carcinoma via upregulation of CXCR4 expression. Theranostics 9, 5810–5827. doi:10.7150/thno.34973
Yang, T., Xing, H., Wang, G., Wang, N., Liu, M., Yan, C., et al. (2019c). A novel online calculator based on serum biomarkers to detect hepatocellular carcinoma among patients with hepatitis B. Clin. Chem. 65, 1543–1553. doi:10.1373/clinchem.2019.308965
Yang, X., Luo, Y., Zhuo, Y., Feng, Y., and Zhu, S. (2014). Novel synthesis of gold nanoclusters templated with l-tyrosine for selective analyzing tyrosinase. Anal. Chim. Acta 840, 87–92. doi:10.1016/j.aca.2014.05.050
Yuan, Y., Yu, H., and Yin, Y. (2020). A highly sensitive aptasensor for vascular endothelial growth factor based on fluorescence resonance energy transfer from upconversion nanoparticles to MoS2 nanosheets. Anal. Methods 12, 4466–4472. doi:10.1039/d0ay01067g
Yue, L., and Liu, Y.-J. (2013). Mechanism of AMPPD chemiluminescence in a different voice. J. Chem. Theory Comput. 9, 2300–2312. doi:10.1021/ct400206k
Zahra, Q. ul ain, Khan, Q. A., and Luo, Z. (2021). Advances in optical aptasensors for early detection and diagnosis of various cancer types. Front. Oncol. 11, 632165. doi:10.3389/fonc.2021.632165
Zhan, Z., Chen, B., Yu, J., Zheng, J., Zeng, Y., Sun, M., et al. (2022). Elevated serum alpha-fetoprotein is a significant prognostic factor for patients with gastric cancer: Results based on a large-scale retrospective study. Front. Oncol. 12, 901061. doi:10.3389/fonc.2022.901061
Zhang, H., Li, M., Li, C., Guo, Z., Dong, H., Wu, P., et al. (2015). G-quadruplex DNAzyme-based electrochemiluminescence biosensing strategy for VEGF165 detection: Combination of aptamer–target recognition and T7 exonuclease-assisted cycling signal amplification. Biosens. Bioelectron. 74, 98–103. doi:10.1016/j.bios.2015.05.069
Zhang, H., Peng, L., Li, M., Ma, J., Qi, S., Chen, H., et al. (2017). A label-free colorimetric biosensor for sensitive detection of vascular endothelial growth factor-165. Analyst 142, 2419–2425. doi:10.1039/c7an00541e
Zhang, N., Zhang, N., Xu, Y., Li, Z., Yan, C., Mei, K., et al. (2019). Molecularly imprinted materials for selective biological recognition. Macromol. Rapid Commun. 40, 1900096. doi:10.1002/marc.201900096
Zhang, S., Gao, C., Zhou, Q., Chen, L., Huang, G. F., Tang, H., et al. (2023). Identification and validation of circulating biomarkers for detection of liver cancer with antibody array. Neoplasma 70 (1), 36–45. doi:10.4149/neo_2022_220606n600
Zhang, Y., Bai, Y., Feng, F., and Shuang, S. (2016). A graphene oxide-based fluorescent aptasensor for alpha-fetoprotein detection. Anal. Methods 8, 6131–6134. doi:10.1039/c6ay01949h
Zhang, Z., Meng, H., Wang, N., Liang, L., Liu, L., Lu, S., et al. (2014). Serum microRNA 143 and microRNA 215 as potential biomarkers for the diagnosis of chronic hepatitis and hepatocellular carcinoma. Diagn. Pathol. 9, 135. doi:10.1186/1746-1596-9-135
Zhao, S., Ma, W., Xu, L., Wu, X., Kuang, H., Wang, L., et al. (2015). Ultrasensitive SERS detection of VEGF based on a self-assembled Ag ornamented–AU pyramid superstructure. Biosens. Bioelectron. 68, 593–597. doi:10.1016/j.bios.2015.01.056
Zhou, J.-M., Wang, T., and Zhang, K.-H. (2021). AFP-L3 for the diagnosis of early hepatocellular carcinoma: A meta-analysis. Medicine 100, e27673. doi:10.1097/md.0000000000027673
Zhou, L., Ji, F., Zhang, T., Wang, F., Li, Y., Yu, Z., et al. (2019). An fluorescent aptasensor for sensitive detection of tumor marker based on the FRET of a sandwich structured QDs-AFP-AuNPs. Talanta 197, 444–450. doi:10.1016/j.talanta.2019.01.012
Zhu, D., Li, W., Wen, H.-M., Yu, S., Miao, Z.-Y., Kang, A., et al. (2015). Silver nanoparticles-enhanced time-resolved fluorescence sensor for VEGF165 based on Mn-doped ZnS quantum dots. Biosens. Bioelectron. 74, 1053–1060. doi:10.1016/j.bios.2015.08.005
Keywords: optical aptasensor, hepatocellular carcinoma, biomarker, diagnosis, prognosis, monitor
Citation: Dong J-M, Wang R-Q, Yuan N-N, Guo J-H, Yu X-Y, Peng A-H, Cai J-Y, Xue L, Zhou Z-L, Sun Y-H and Chen Y-Y (2023) Recent advances in optical aptasensors for biomarkers in early diagnosis and prognosis monitoring of hepatocellular carcinoma. Front. Cell Dev. Biol. 11:1160544. doi: 10.3389/fcell.2023.1160544
Received: 07 February 2023; Accepted: 06 April 2023;
Published: 18 April 2023.
Edited by:
Daniela Capello, University of Eastern Piedmont, ItalyCopyright © 2023 Dong, Wang, Yuan, Guo, Yu, Peng, Cai, Xue, Zhou, Sun and Chen. This is an open-access article distributed under the terms of the Creative Commons Attribution License (CC BY). The use, distribution or reproduction in other forums is permitted, provided the original author(s) and the copyright owner(s) are credited and that the original publication in this journal is cited, in accordance with accepted academic practice. No use, distribution or reproduction is permitted which does not comply with these terms.
*Correspondence: Zhi-Ling Zhou, emhvdXpoaWxpbmdAZXh0LmpudS5lZHUuY24=; Yi-Hao Sun, c3loQGFsdW1uaS50b25namkuZWR1LmNu; Ying-Yin Chen, Y2hlbnlpbmd5aW5AeWVhaC5uZXQ=
†These authors have contributed equally to this work and share first authorship