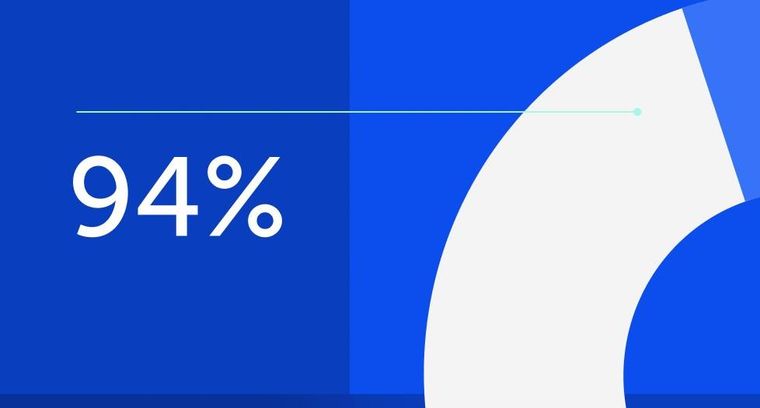
94% of researchers rate our articles as excellent or good
Learn more about the work of our research integrity team to safeguard the quality of each article we publish.
Find out more
OPINION article
Front. Cell Dev. Biol., 13 April 2023
Sec. Signaling
Volume 11 - 2023 | https://doi.org/10.3389/fcell.2023.1149009
Retinoic acid (RA) is a potent epigenetic regulator that directly controls transcription of key genes during development. This article addresses concerns about recently published data suggesting that RA influences the timing and scaling of limb development. RA gain-of-function (GOF) studies in chick embryos where RA is added at pharmacological levels were used to conclude that RA signaling controls the timing and scaling of limb development. However, previous RA loss-of-function (LOF) studies in mouse contradict the conclusions derived from chick GOF studies, instead demonstrating that endogenous RA is not required for timing, scaling, and patterning of limbs during development. As genetic LOF studies are generally considered superior to GOF studies for determining the normal functions of genes, proteins, and signaling molecules (such as RA), readers need to be aware that chick studies do not provide conclusive evidence on the normal function of endogenous RA during limb development.
Studies on the mechanisms underlying development of limbs have led to great insight into how epigenetic regulators such as RA control transcription (Cunningham and Duester, 2015). However, studies on how retinoic acid (RA) regulates limb development have been much more controversial. Chick GOF studies have shown that limbs treated with RA have altered proximodistal patterning that forces expression of Meis1/Meis2 to extend from its normal proximal position to a distal position; this observation led to the conclusion that RA normally controls limb proximodistal patterning by activating Meis1/Meis2 in the proximal limb (Mercader et al., 2000; Rosello-Diez et al., 2011). In contrast, mouse RA genetic LOF studies show that RA is not required for limb proximodistal patterning; reviewed in (Cunningham and Duester, 2015). The main problem concerning chick RA GOF studies revolves around the fact that RA is a small molecule that can be added at very high micromolar (μM) levels in GOF studies (Mercader et al., 2000; Rosello-Diez et al., 2011) compared to its normal endogenous levels which are nanomolar (nM) in various tissues of both mouse and chick embryos including limb buds that have ∼25 nM RA (Dong and Zile, 1995; Horton and Maden, 1995). In essence, RA added at pharmacological μM levels to embryos or cell lines has side-effects on transcription that allow RA to now control genes that it would not normally control when RA is present at endogenous nM levels (Cunningham and Duester, 2015).
Recently, Stainton and Towers (2022) reported studies using chick embryo limb buds treated with pharmacological levels of RA (GOF) or an RA receptor antagonist (to simulate LOF) along with tissue grafting to make the conclusion that RA normally controls the timing and scaling of limb development. In these studies, beads soaked with high levels of RA [0.05 mg/mL; ∼0.1 millimolar (mM)] or an RAR antagonist (1 mg/mL; ∼2 mM) were implanted in the limb, with the reagent then diffusing into the limb (Stainton and Towers, 2022). Although the limb tissue near the bead may not achieve the extremely high mM concentration of reagent present in the bead, it is clear that the nearby limb tissue is exposed to a much higher concentration than the 25 nM of endogenous RA (probably μM levels), thus resulting in potential off-target effects. Also, since RA receptor antagonists silence genes in the vicinity of RA receptor-bound RA response elements (Germain et al., 2002), a high concentration may dominantly switch off numerous genes that happen to have a RA response element nearby but that normally use other control elements under physiological RA conditions. RA receptor chromatin immunoprecipitation studies have discovered 13,000–15,000 potential RA response elements (Lalevee et al., 2011; Moutier et al., 2012), most of which have not been attributed to endogenous RA signaling, but many of which may become off-targets during treatment with high amounts of RA or RA receptor antagonists (Cunningham and Duester, 2015). Thus, RA receptor antagonists may not provide the intended LOF result.
In contrast to these chick studies (Stainton and Towers, 2022), several previously published genetic LOF studies describing RA-deficient mouse embryos all contradict their main conclusion (Sandell et al., 2007; Zhao et al., 2009; Cunningham et al., 2011; Cunningham et al., 2013; Berenguer et al., 2020). Our questioning of the conclusions made by Stainton and Towers (2022) is reinforced by several review articles indicating that RA is not required for limb proximodistal patterning (Lewandoski and Mackem, 2009; Kawakami, 2013; Cunningham and Duester, 2015; Ghyselinck and Duester, 2019; Berenguer and Duester, 2021). Stainton and Towers (2022) reference only one old paper related to mouse RA LOF studies, (Mic et al., 2004), in order to support the view that RA controls limb proximodistal patterning, without referencing more recent mouse papers that reject this idea. Mic et al. (2004) partially supported the limb RA proximodistal hypothesis by showing that RA signaling activity is indeed present in proximal but not distal tissue of mouse limb buds; the proximal limb does not generate RA itself but instead receives RA by diffusion from the adjacent trunk that expresses RA-generating enzymes. Mic et al. (2004) also analyzed mouse knockout embryos for Aldh1a2 encoding retinaldehyde dehydrogenase 2 (ALDH1A2; RALDH2) that performs the final step of RA synthesis (retinaldehyde to RA); however, Aldh1a2−/− embryos were not able to verify the RA limb proximodistal hypothesis as these embryos completely lack trunk RA and undergo lethality prior to limb bud formation. Subsequently, an article was published in 2007 by Sandell et al. (2007) who generated a mouse mutant for Rdh10 encoding retinol dehydrogenase 10 (RDH10) that performs the first step of RA synthesis (retinol to retinaldehyde); Rdh10 mutant embryos survive long enough to develop limbs because they retain a small amount of trunk RA activity (probably due to another unknown retinol-metabolizing enzyme), but limb buds do not exhibit RA signaling activity and proximodistal patterning of hindlimbs is normal whereas forelimbs are stunted. Interestingly, a recent genetic LOF study shows that conditional incomplete loss of all three mouse RA receptors results in embryos with a stunted forelimb but a hindlimb of normal size similar to Rdh10 mutants (Teletin et al., 2023). These mouse LOF studies suggest that loss of RA signaling does not effect the timing and scaling of limb buds, especially hindlimb buds.
Further studies on the mouse Rdh10 knockout showed that RA is not required for limb proximodistal patterning, but that RA is required for initiation of forelimb budding through repression of the gene encoding fibroblast growth factor 8 (Fgf8) in the forelimb field (Zhao et al., 2009; Cunningham et al., 2013); Fgf8 is normally expressed anteriorly in the heart and posteriorly in the tail bud progenitors, but if FGF8 is present between these domains in the trunk region where the forelimb field lies, FGF8 will inhibit expression of Tbx5 that is required to initiate forelimb budding (Zhao et al., 2009; Cunningham et al., 2013). Although loss of RA inhibits initiation of forelimb budding, these more recent papers all show that hindlimbs grow normally in the complete absence of RA and the stunted forelimbs as well as the normally-sized hindlimbs both have normal proximodistal patterning, i.e., they still exhibit a proximal domain with Meis1/Meis2 expression and a distal domain with Hoxa11 expression (Zhao et al., 2009; Cunningham et al., 2011; Cunningham et al., 2013). Some concerns have been raised that maybe RA signaling has not been efficiently removed from limbs of Rdh10 mutants, however our analysis of RA levels demonstrated that the RA concentration in mouse limb buds, which is normally about 25 nM (Horton and Maden, 1995), was easily detected in wild-type but undetectable in Rdh10 mutant limbs with the results showing a reduction of at least 100-fold to less than 0.25 nM in both forelimbs and hindlimbs (Cunningham et al., 2013). Thus, contrary to the conclusions of Stainton and Towers (2022) based on chick GOF studies, mouse genetic LOF studies show that endogenous RA is not required for patterning of forelimbs and hindlimbs (Zhao et al., 2009; Cunningham et al., 2013). In addition, the mouse studies show that loss of RA has no effect on timing and scaling of hindlimbs, and forelimb timing is not changed by loss of RA in Rdh10 mutants while the stunted forelimb is best described as a toxic effect of excess trunk/heart FGF8 signaling diffusing into the forelimb field prior to outgrowth rather than an effect on scaling (Zhao et al., 2009; Cunningham et al., 2013). As Stainton and Towers (2022) examined only forelimbs (wings), one might say that their results on timing and scaling do not apply to hindlimbs (legs). However, it is reasonable to assume that their conclusions would also apply to hindlimbs as the original hypothesis stated that both forelimbs and hindlimbs use RA to control limb proximodistal patterning (Mercader et al., 2000). As the mouse LOF data very clearly show that complete loss of RA signaling has no effect on hindlimb size, timing, or patterning, this raises concerns that the original chick conclusion (Mercader et al., 2000) and subsequent studies (Cooper et al., 2011; Rosello-Diez et al., 2011; Stainton and Towers, 2022) are based on side-effects of pharmacological RA treatments and grafting experiments rather than an essential role of endogenous RA. One can conclude that a role for endogenous RA in limb size, timing, and patterning does not exist in mice. Although the conclusion made from chick limb studies have been suggested to apply to vertebrates in general (Mercader et al., 2000; Cooper et al., 2011; Rosello-Diez et al., 2011), a role for endogenous RA might exist in the chick limb but not all vertebrate limbs. However, a chick genetic LOF study would be needed to confirm that RA is required in the chick limb.
A mouse knockout of Cyp26b1, encoding an RA-degrading enzyme expressed in the distal limb, exhibits stunted limbs and ectopic expression of Meis1/Meis2 in the distal limb; these observations were suggested to support the hypothesis that RA is required for limb proximodistal patterning, with CYP26B1 setting a border where RA can activate Meis1/Meis2 proximally but not distally (Yashiro et al., 2004). In the Cyp26b1 knockout, although RA activity and Meis1/Meis2 expression expand into the distal limb, similar to chick RA-treatment studies, limbs exhibit proximal as well as distal truncation likely due to higher than normal RA activity in the proximal limb as well as RA distally (Yashiro et al., 2004); these observations are inconsistent with RA functioning to induce proximal identity. In our opinion, the Cyp26b1 knockout results in a teratogenic phenotype similar to embryos treated with exogenous RA that exhibit increased apoptosis and a block in chondrogenesis along the entire axis of the limb (Pennimpede et al., 2010; Dranse et al., 2011). Thus, Cyp26b1 expression distally may not function to restrict RA signaling proximally to set the boundary of Meis1/Meis2 expression for proximodistal patterning, but instead Cyp26b1 may function to simply eliminate RA activity distally as a sink to reduce RA all along the limb axis to prevent teratogenesis.
Studies on a conditional mouse Meis1/Meis2 double knockout that reduces expression confirmed that Meis1/Meis2 functions as a proximal signal needed to control limb proximodistal patterning, and that normal expression of these genes is prevented distally by FGF8 signals from the apical ectodermal ridge (Delgado et al., 2020). Further studies demonstrated that a complete Meis1/Meis2 double knockout fails to initiate forelimb development, showing that Meis1/Meis2 are required not only for limb proximodistal patterning but also for initiation of forelimb buds (Berenguer et al., 2020). Combined with studies showing that the mouse Rdh10 knockout (which lacks RA signaling in limb buds) still expresses Meis1/Meis2 in forelimbs and hindlimbs, the original hypothesis for control of limb proximodistal patterning (Mercader et al., 2000) needs revision. A revised hypothesis proposes the following: (a) trunk RA signaling acts permissively to allow limb bud initiation by directly repressing Fgf8 in the limb field to separate it from Fgf8 expression domains anteriorly in the heart and posteriorly in the tail bud where the body axis is extending; (b) Meis1/Meis2 expressed in trunk mesoderm fated to become the limb field functions instructively along with Tbx5 to initiate limb budding; (c) after limb outgrowth begins, Meis1/Meis2 expression is activated in proximal limb by a signal other than RA; (d) Meis1/Meis2 expression is limited to the proximal limb due to expression of Fgf8 distally in the apical ectodermal ridge; (e) Meis1/Meis2 but not RA then functions to control limb proximodistal patterning (Berenguer and Duester, 2021).
In conclusion, genetic LOF studies are needed to make major conclusion on the function of endogenous RA. Even though LOF studies can be difficult, they are required to determine the normal function of any molecule, protein, or gene as GOF studies (such as treatments with small molecules or proteins as well as overexpression of genes) may lead to side-effects that prevent insight into how endogenous signaling systems function.
The manuscript was written by MB and GD.
This work was funded by the National Institutes of Health (National Institute of Arthritis and Musculoskeletal and Skin Diseases) grant R56 AR067731 (GD).
The authors declare that the research was conducted in the absence of any commercial or financial relationships that could be construed as a potential conflict of interest.
All claims expressed in this article are solely those of the authors and do not necessarily represent those of their affiliated organizations, or those of the publisher, the editors and the reviewers. Any product that may be evaluated in this article, or claim that may be made by its manufacturer, is not guaranteed or endorsed by the publisher.
Berenguer, M., and Duester, G. (2021). Role of retinoic acid signaling, FGF signaling and Meis genes in control of limb development. Biomolecules 11 (1), 80. doi:10.3390/biom11010080
Berenguer, M., Meyer, K. F., Yin, J., and Duester, G. (2020). Discovery of genes required for body axis and limb formation by global identification of retinoic acid-regulated epigenetic marks. PLOS Biol. 18, e3000719. doi:10.1371/journal.pbio.3000719
Cooper, K. L., Hu, J. K., ten Berge, D., Fernandez-Teran, M., Ros, M. A., and Tabin, C. J. (2011). Initiation of proximal-distal patterning in the vertebrate limb by signals and growth. Science 332 (6033), 1083–1086. doi:10.1126/science.1199499
Cunningham, T. J., Chatzi, C., Sandell, L. L., Trainor, P. A., and Duester, G. (2011). Rdh10 mutants deficient in limb field retinoic acid signaling exhibit normal limb patterning but display interdigital webbing. Dev. Dyn. 240, 1142–1150. doi:10.1002/dvdy.22583
Cunningham, T. J., and Duester, G. (2015). Mechanisms of retinoic acid signalling and its roles in organ and limb development. Nat. Rev. Mol. Cell Biol. 16, 110–123. doi:10.1038/nrm3932
Cunningham, T. J., Zhao, X., Sandell, L. L., Evans, S. M., Trainor, P. A., and Duester, G. (2013). Antagonism between retinoic acid and fibroblast growth factor signaling during limb development. Cell Rep. 3, 1503–1511. doi:10.1016/j.celrep.2013.03.036
Delgado, I., Lopez-Delgado, A. C., Rosello-Diez, A., Giovinazzo, G., Cadenas, V., Fernandez-de-Manuel, L., et al. (2020). Proximo-distal positional information encoded by an Fgf-regulated gradient of homeodomain transcription factors in the vertebrate limb. Sci. Adv. 6 (23), eaaz0742. doi:10.1126/sciadv.aaz0742
Dong, D., and Zile, M. H. (1995). Endogenous retinoids in the early avian embryo. Biochem. Biophys. Res. Comm. 217, 1026–1031. doi:10.1006/bbrc.1995.2872
Dranse, H. J., Sampaio, A. V., Petkovich, M., and Underhill, T. M. (2011). Genetic deletion of Cyp26b1 negatively impacts limb skeletogenesis by inhibiting chondrogenesis. J. Cell Sci. 124 (16), 2723–2734. doi:10.1242/jcs.084699
Germain, P., Iyer, J., Zechel, C., and Gronemeyer, H. (2002). Co-regulator recruitment and the mechanism of retinoic acid receptor synergy. Nature 415, 187–192. doi:10.1038/415187a
Ghyselinck, N. B., and Duester, G. (2019). Retinoic acid signaling pathways. Development 146 (13), dev167502. doi:10.1242/dev.167502
Horton, C., and Maden, M. (1995). Endogenous distribution of retinoids during normal development and teratogenesis in the mouse embryo. Dev. Dyn. 202, 312–323. doi:10.1002/aja.1002020310
Kawakami, Y. (2013). Redefining the role of retinoic Acid in limb development. Cell Rep. 3 (5), 1337–1338. doi:10.1016/j.celrep.2013.05.010
Lalevee, S., Anno, Y. N., Chatagnon, A., Samarut, E., Poch, O., Laudet, V., et al. (2011). Genome-wide in silico identification of new conserved and functional retinoic acid receptor response elements (direct repeats separated by 5 bp). J. Biol. Chem. 286 (38), 33322–33334. doi:10.1074/jbc.M111.263681
Lewandoski, M., and Mackem, S. (2009). Limb development: The rise and fall of retinoic acid. Curr. Biol. 19 (14), R558–R561. doi:10.1016/j.cub.2009.06.017
Mercader, N., Leonardo, E., Piedra, M. E., Martínez, -A. C., Ros, M. A., and Torres, M. (2000). Opposing RA and FGF signals control proximodistal vertebrate limb development through regulation of Meis genes. Development 127, 3961–3970. doi:10.1242/dev.127.18.3961
Mic, F. A., Sirbu, I. O., and Duester, G. (2004). Retinoic acid synthesis controlled by Raldh2 is required early for limb bud initiation and then later as a proximodistal signal during apical ectodermal ridge formation. J. Biol. Chem. 279, 26698–26706. doi:10.1074/jbc.M401920200
Moutier, E., Ye, T., Choukrallah, M. A., Urban, S., Osz, J., Chatagnon, A., et al. (2012). Retinoic acid receptors recognize the mouse genome through binding elements with diverse spacing and topology. J. Biol. Chem. 287 (31), 26328–26341. doi:10.1074/jbc.M112.361790
Pennimpede, T., Cameron, D. A., MacLean, G. A., and Petkovich, M. (2010). Analysis of Cyp26b1/Rarg compound-null mice reveals two genetically separable effects of retinoic acid on limb outgrowth. Dev. Biol. 339 (1), 179–186. doi:10.1016/j.ydbio.2009.12.024
Rosello-Diez, A., Ros, M. A., and Torres, M. (2011). Diffusible signals, not autonomous mechanisms, determine the main proximodistal limb subdivision. Science 332 (6033), 1086–1088. doi:10.1126/science.1199489
Sandell, L. L., Sanderson, B. W., Moiseyev, G., Johnson, T., Mushegian, A., Young, K., et al. (2007). RDH10 is essential for synthesis of embryonic retinoic acid and is required for limb, craniofacial, and organ development. Genes Dev. 21 (9), 1113–1124. doi:10.1101/gad.1533407
Stainton, H., and Towers, M. (2022). Retinoic acid influences the timing and scaling of avian wing development. Cell Rep. 38 (4), 110288. doi:10.1016/j.celrep.2021.110288
Teletin, M., Mark, M., Wendling, O., Vernet, N., Feret, B., Klopfenstein, M., et al. (2023). Timeline of developmental defects generated upon genetic inhibition of the retinoic acid receptor signaling pathway. Biomedicines 11 (1), 198. doi:10.3390/biomedicines11010198
Yashiro, K., Zhao, X., Uehara, M., Yamashita, K., Nishijima, M., Nishino, J., et al. (2004). Regulation of retinoic acid distribution is required for proximodistal patterning and outgrowth of the developing mouse limb. Dev. Cell 6, 411–422. doi:10.1016/s1534-5807(04)00062-0
Keywords: retinoic acid signaling, limb development, genetic loss-of-function, gain-of-function, limb patterning
Citation: Berenguer M and Duester G (2023) Genetic loss-of-function does not support gain-of-function studies suggesting retinoic acid controls limb bud timing and scaling. Front. Cell Dev. Biol. 11:1149009. doi: 10.3389/fcell.2023.1149009
Received: 20 January 2023; Accepted: 03 April 2023;
Published: 13 April 2023.
Edited by:
Jesus Chimal-Monroy, National Autonomous University of Mexico, MexicoReviewed by:
Joshua Waxman, Cincinnati Children’s Hospital Medical Center, United StatesCopyright © 2023 Berenguer and Duester. This is an open-access article distributed under the terms of the Creative Commons Attribution License (CC BY). The use, distribution or reproduction in other forums is permitted, provided the original author(s) and the copyright owner(s) are credited and that the original publication in this journal is cited, in accordance with accepted academic practice. No use, distribution or reproduction is permitted which does not comply with these terms.
*Correspondence: Gregg Duester, ZHVlc3RlckBzYnBkaXNjb3Zlcnkub3Jn
Disclaimer: All claims expressed in this article are solely those of the authors and do not necessarily represent those of their affiliated organizations, or those of the publisher, the editors and the reviewers. Any product that may be evaluated in this article or claim that may be made by its manufacturer is not guaranteed or endorsed by the publisher.
Research integrity at Frontiers
Learn more about the work of our research integrity team to safeguard the quality of each article we publish.