- 1Centre for Regenerative Medicine and Health, Hong Kong Institute of Science & Innovation, Chinese Academy of Sciences, Hong Kong, Hong Kong SAR, China
- 2The Fifth Affiliated Hospital of Guangzhou Medical University, Guangzhou, China
- 3Westlake Laboratory of Life Sciences and Biomedicine, Hangzhou, China
- 4The First Affiliated Hospital of Guangzhou University of Chinese Medicine, Guangzhou University of Chinese Medicine, Guangzhou, China
- 5Drug Discovery Pipeline at the Guangzhou Institutes for Biomedicine and Health, Chinese Academy of Sciences, Guangzhou, China
- 6Musculoskeletal Research Laboratory, Department of Orthopaedics & Traumatology, Faculty of Medicine, The Chinese University of Hong Kong, Prince of Wales Hospital, Hong Kong SAR, China
- 7Stem Cells and Regenerative Medicine Laboratory, Li Ka Shing Institute of Health Sciences, The Chinese University of Hong Kong, Prince of Wales Hospital, Hong Kong SAR, China
- 8Shenzhen Research Institute, The Chinese University of Hong Kong, Shenzhen, China
Cartilage organoids have emerged as powerful modelling technology for recapitulation of joint embryonic events, and cartilage regeneration, as well as pathophysiology of cartilage-associated diseases. Recent breakthroughs have uncovered “mini-joint” models comprising of multicellular components and extracellular matrices of joint cartilage for development of novel disease-modifying strategies for personalized therapeutics of cartilage-associated diseases. Here, we hypothesized that LGR5-expressing embryonic joint chondroprogenitor cells are ideal stem cells for the generation of cartilage organoids as “mini-joints” ex vivo “in a dish” for embryonic joint development, cartilage repair, and cartilage-associated disease modelling as essential research models of drug screening for further personalized regenerative therapy. The pilot research data suggested that LGR5-GFP-expressing embryonic joint progenitor cells are promising for generation of cartilage organoids through gel embedding method, which may exert various preclinical and clinical applications for realization of personalized regenerative therapy in the future.
1 Introduction
Cartilage remains among the most difficult tissues to regenerate, and integration of an implant with the surrounding tissue is also a major challenge in cartilage regeneration (Huey et al., 2012; Trengove et al., 2022). Regeneration of calcified cartilage regions is also a critical issue for stable and functional integration to subchondral bone besides cartilage–cartilage integration in the field of regenerative medicine and tissue engineering.
Organoids are self-assembling three-dimensional tissues containing multiple types of cell clusters that generated from pluripotent stem cells or adult stem cells, providing a powerful tool for developmental biology and disease modeling of various tissue and organ systems in vitro (Dutta et al, 2017; Hu et al, 2018). Originally, organoid technology mainly comprises of gel encapsulation method that developed by Hans Clevers lab, and air-liquid interface method developed by Calvin J. Kuo lab. To date, organoids have been successfully established from adult stem cells of multiple healthy and diseased tissues and organs, including stomach (Engevik et al., 2019; Murakami et al., 2021), colon (d'Aldebert et al., 2020), intestine (Gjorevski et al., 2016; Hirota et al., 2021), lung (Miller et al., 2019; Lim et al., 2023), liver (Vyas et al., 2018), kidney (Takasato et al., 2016; Yuan et al. 2022), pancreas (Broutier et al., 2016), ovary (Kopper et al., 2019), brain (Luo and Li, 2021; Luo et al., 2022), and prostate (Huang et al., 2021) ex vivo.
2 Advancements of cartilage organoids
The development of cartilage organoid technology as useful modelling tools and robust research platforms enables the definition and disease modelling of cartilage-tissue structures ex vivo to facilitate drug screening through identification of key signaling pathways, and recapitulation of developmental events during joint embryogenesis and cartilage regeneration, dynamics of stem cell chondrogenic differentiation, and aging-induced degenerative joint diseases “in a dish” (Clevers, 2016; Lacko and Chen, 2019; O'Connor et al., 2021; Rothbauer et al., 2021; Sun et al., 2021). In the early 1990s, C. Schröter-Kermani and his colleagues successfully established an ex vivo model of a prolonged, but almost identical of chondrogenesis events in vivo prior to endochondral mineralization, providing a useful tool for investigations on cartilage differentiation, maturation, and degeneration (Schroter-Kermani et al., 1991). Further research by Irie, Yutaka, et al. developed sheet-shaped organoids (organoid-sheet) of cartilage-like tissues, in which cells formed multicellular aggregates (organoids), through an effective cartilage-formation method (Irie et al., 2008). Cell clusters called spheroids exert promising therapeutic potential for cartilage tissue engineering research as building blocks (Baptista et al., 2018; Kronemberger et al., 2020). Intriguingly, recent breakthroughs have uncovered “mini-joint” models comprising of multicellular components and extracellular matrices of joint cartilage for potential realization of novel disease-modifying strategies for personalized therapeutics of cartilage-associated diseases (Delplace et al., 2021; Abraham et al., 2022). A recent striking study has developed a novel differentiation protocol that generated self-organizing craniofacial cartilage organoids from human embryonic stem cells via a neural crest cell intermediate (Foltz et al., 2021).
Cartilage organoids are specific three-dimensional and functional cartilage-like tissues through self-assembled reconstruction of chondrocytes or chondroprogenitor cells (Irie et al., 2008; Schon et al., 2017; Gryadunova et al., 2021), which is of essential clinical significance for tremendous translational applications to repair various cartilaginous structures throughout the body, as well as organoid biobanking, disease modeling, drug toxicity testing, personalized regenerative therapy, host–microbe interaction studies, and omics analysis (including transcriptomics, proteomics, epigenomics, and metabolomics) (Dutta et al., 2017). Cartilage organoids have been successfully generated both from induced pluripotent stem cells or mesenchymal stem cells (Li Z. et al, 2022). Cartilage organoid formation and their assembly into neo-hyaline-cartilage have paved a new way for large scale cartilage regeneration such as for entire joint surfaces (Crispim and Ito, 2021). And the development of cartilaginous organoids has been applied to diverse implications in preclinical research during recent years (Table 1).
Hyaline cartilages, fibrocartilages and elastic cartilages play multiple roles throughout human body including bearing loads in articular joints and intervertebral discs, providing joint lubrication, forming the external ears and nose, supporting the trachea, and forming the long bones during development and growth. Challenges associated with cartilage diseases include poor understanding of the etiology and pathogenesis and diagnostics due to the aneural and avascular nature of adult cartilages, and very limited chondroprogenitor cells within adult joint cartilage.(Krishnan and Grodzinsky, 2018; Bielajew et al., 2020; Liao et al., 2021; Lin et al., 2022). Age is a main risk factor for the development of rheumatoid arthritis, which is associated with accelerated immune aging and dysfunction of aging stem cells (Weyand and Goronzy, 2004; Goronzy et al., 2013; Weyand et al., 2014). Generally, joint cartilage usually degenerates spontaneously in elderly mammalians (Figure 1). As mitochondrial dysfunctions and age-associated systemic chronic inflammation (also termed as “inflamm-aging”) have been demonstrated linked to the development of diverse aging-associated degenerative diseases (Franceschi and Campisi, 2014; Laforge et al., 2016; Sanada et al., 2018; Josephson et al., 2019; Shin et al., 2022). To further decipher dynamic alterations of cellular and mitochondrial behaviors and structures (Tran-Khanh et al., 2005; Labbe et al., 2014; Guilak et al., 2018; Akatsu et al., 2019), and key signaling pathways involved in the interplay between mitochondrial remodeling and “inflamm-aging” may further advance the understanding of the pathophysiology of aging-associated cartilage degeneration.
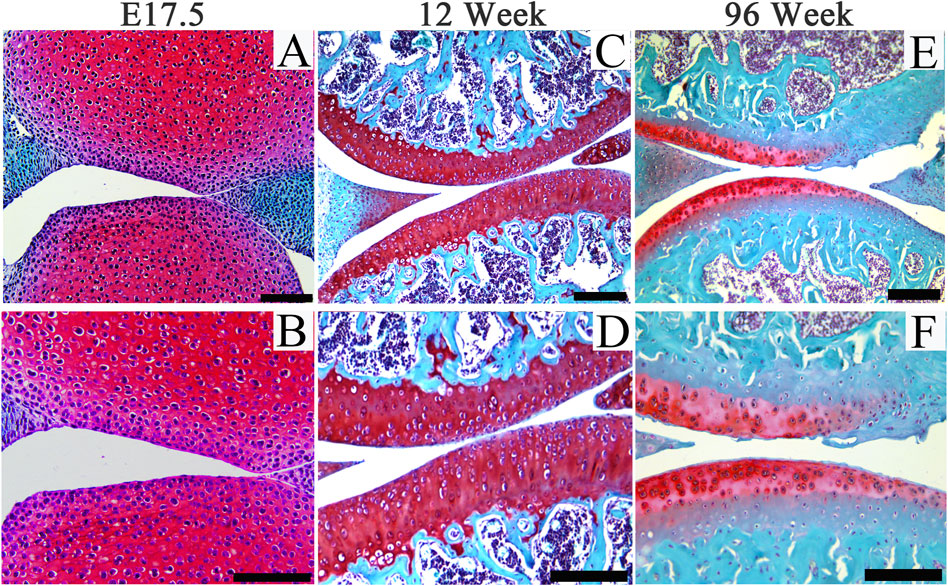
FIGURE 1. Representative images of Safranin O staining of articular cartilage of joints from embryos (E17.5), adult (12-week-old) and aging (96-week-old) mice. Scale bars = 200 μm.
3 Involvements of GPCRS in joint embryogenesis and cartilage pathophysiology
Leucine-rich repeat-containing G protein-coupled receptors 4–6 (LGR4–LGR6) are receptors for R-spondins, potent Wnt agonists that exert profound trophic effects on Wnt-driven stem cells compartments. The crystal structure of LGR5 has been discovered (Peng et al., 2013).
Notably, increasing evidence has demonstrated critical involvements of GPCRs during development and tissue homeostasis and regeneration in various tissue and organ systems (Luo et al., 2009; Cui et al., 2014; Feng et al., 2019; Montgomery et al., 2019; d'Aldebert et al., 2020; Lee et al., 2021; Li et al., 2022; Khedgikar et al., 2022). Crucial involvements of GPCRs, such as LGR5, in both embryonic joint development (Feng et al., 2019), and postnatal joint development in juvenile mammals (Zhou et al., 2018), as well as progression of arthritis development (Li R. et al, 2022), have been identified, suggesting targeted modulation of GPCRs on cartilage as potential novel therapeutics for arthritis management.
Interestingly, a recent breakthrough by Rothbauer, M. et al. has successfully established microfluidic joint-on-a-chip organoid system to investigate reciprocal cross-talk between individual synovial and chondral organoids on tissue-level for modelling of arthritic diseases (Rothbauer et al., 2021). And our ongoing research suggest that LGR5-GFP+ embryonic joint progenitors embedded within hydrogels enable the generation of organoid-structures under appropriate culture conditions with expression of LGR5-GFP signal (Figure 2), suggesting that LGR5-expressing joint chondroprogenitor cells are potential ideal cells for cartilage-like organoids formation, disease modelling for cartilage-associated diseases, drug screening and cartilage regeneration for realization of personalized medicine.
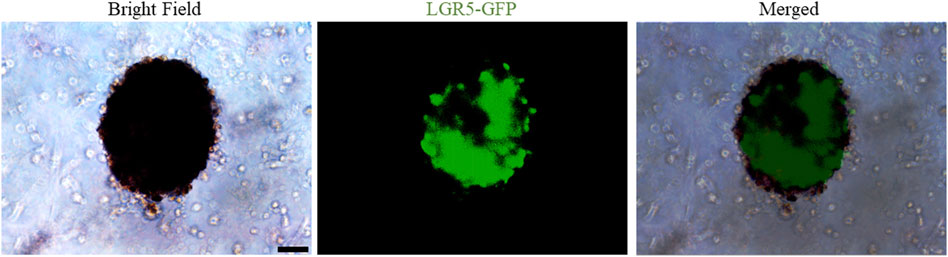
FIGURE 2. Representative images of bright-field and fluorescence of LGR5+-embryonic joint progenitors-based cartilage organoids formation. Scale bars = 100 µm.
4 Conclusions and future perspectives
Organoids have been firmly established as a robust platform to investigate organ development, normal and pathological processes, and drug screening in both basic preclinical science and translational research, to overcome the limitations associated with animal models (Singh et al., 2021). Optimization of superior cell source, and ex vivo culture conditions for phenotypic control of cartilage organoids after transplantation deserve further exploitation. Integrated with advanced technologies (such as 3D bioprinting, bio-assembly, and organ-on-chip-based models, and comprehensive in-depth organoid single-cell genomic atlas mapping through high-spatial-resolution multi-omics sequencing), cartilage organoid models may provide novel molecular, spatial, and temporal insights of embryonic joint development, and (patho)-physiology of cartilage-associated diseases for boosting the development of personalized regenerative therapy for treating cartilage-associated diseases (Liu et al., 2020; Singh et al., 2021). Cartilage organoids-based research on basic preclinical study and clinical transformation of personalized regenerative therapy will put forward a new era of regeneration medicine (Figure 3). Cartilage organoids provide an ideal platform for mechanistic biology at scale for establishment of cartilage organoid cell atlas through high-throughput drug screening or tissue-on-a-chip systems with molecular and phenotypic readout, and single cell multi-omics analysis (Figure 4; Figure 5). Collaborations among bioengineers, pharmacologists, clinicians, and developmental biologists, integrated with cutting-edge technologies and multi-disciplinary platforms, may accelerate the pace of discovery and precision of future clinical translation based on preclinical models of cartilage organoids (Li and Izpisua Belmonte, 2019; Xinaris, 2019; Berishvili et al., 2021; Bhamidipati and Wei, 2022).
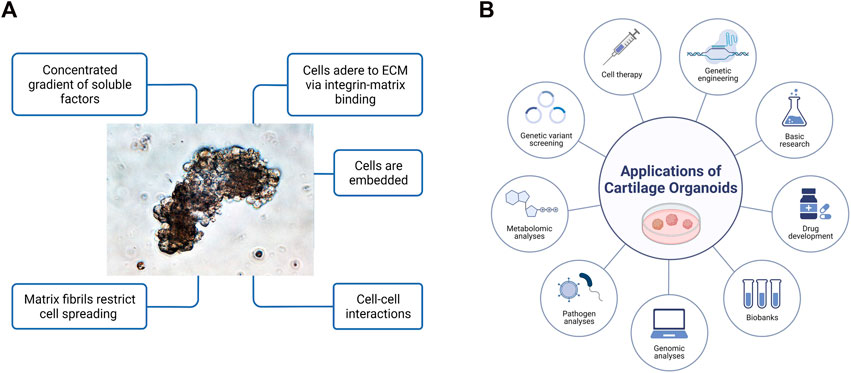
FIGURE 3. (A) General features of three-dimensional cartilage organoids ‘in a dish’. Cells embedded within gels concentrated with various gradients or soluble growth factors are able to adhere to extracellular matrix (ECM), spread and grow with cell-cell interactions in 3-dimensional space. (B) Diverse applications of cartilage organoids for preclinical research and clinical transformation of personalized medicine. Cartilage organoid-based implications mainly include cell therapy through multiple functional cell clusters, drug development, genetic engineering, biobanking, genomic analysis, pathogen analysis, metabolomic analysis, and basic preclinical research.
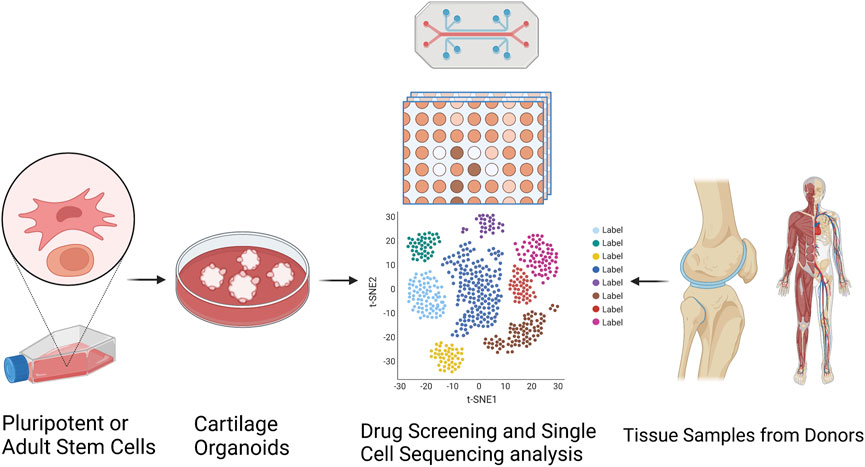
FIGURE 4. Establishment of cartilage organoid cell atlas through RNA sequencing-based drug discovery and single cell multi-omics analysis. Targeted organoid sequencing through a high-throughput, high-content drug discovery platform targeting RNA-seq to monitor the expression of large gene signatures for the detailed evaluation of cellular phenotypes in cartilage organoids generated from pluripotent or adult stem cells.
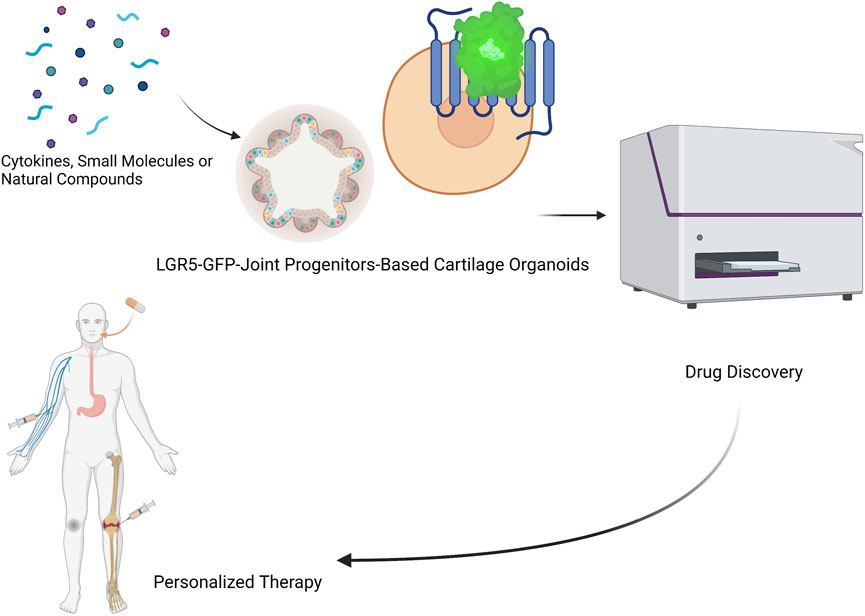
FIGURE 5. LGR5-joint progenitors-based cartilage organoids for realization of novel drug discovery (identification of novel cytokines, small molecules, and natural compounds), and personalized regenerative therapy of cartilage repair.
Data availability statement
The original contributions presented in the study are included in the article/supplementary files, further inquiries can be directed to the corresponding authors.
Ethics statement
The animal study was reviewed and approved by LX; Guangzhou University of Chinese Medicine.
Author contributions
WL and MW contributed to original draft writing and figure preparation; LX, MT, and GL conceived the study, edited the manuscript, and approved the final version of the manuscript.
Acknowledgments
The authors thank the grants support from National Natural Science Foundation of China (NSFC No. 82172430), Hong Kong Government Research Grants Council, Collaborative Research Fund (C7030-18G), General Research Fund (19-093-GRF, 14120118, 9054014, N_CityU102/15, and 14119115), and Health@InnoHK Program launched by Innovation Technology Commission of the Hong Kong SAR, P. R. China.
Conflict of interest
The authors declare that the research was conducted in the absence of any commercial or financial relationships that could be construed as a potential conflict of interest.
Publisher’s note
All claims expressed in this article are solely those of the authors and do not necessarily represent those of their affiliated organizations, or those of the publisher, the editors and the reviewers. Any product that may be evaluated in this article, or claim that may be made by its manufacturer, is not guaranteed or endorsed by the publisher.
References
Abraham, D. M., Herman, C., Witek, L., Cronstein, B. N., Flores, R. L., and Coelho, P. G. (2022). Self-assembling human skeletal organoids for disease modeling and drug testing. J. Biomed. Mater Res. B Appl. Biomater. 110, 871–884. doi:10.1002/jbm.b.34968
Akatsu, Y., Enomoto, T., Yamaguchi, S., Tahara, M., Fukawa, T., Endo, J., et al. (2019). Age-dependent differences in response to partial-thickness cartilage defects in a rat model as a measure to evaluate the efficacy of interventions for cartilage repair. Cell Tissue Res. 375, 425–435. doi:10.1007/s00441-018-2914-y
Baptista, L. S., Kronemberger, G. S., Cortes, I., Charelli, L. E., Matsui, R. A. M., Palhares, T. N., et al. (2018). Adult stem cells spheroids to optimize cell colonization in scaffolds for cartilage and bone tissue engineering. Int. J. Mol. Sci. 19, 1285. doi:10.3390/ijms19051285
Berishvili, E., Casiraghi, F., Amarelli, C., Scholz, H., Piemonti, L., Berney, T., et al. (2021). Mini-organs forum: How to advance organoid technology to organ transplant community. Transpl. Int. 34, 1588–1593.
Bhamidipati, K., and Wei, K. (2022). Precision medicine in rheumatoid arthritis. Best Pract. Res. Clin. Rheumatol., 101742.
Bielajew, B. J., Hu, J. C., and Athanasiou, K. A. (2020). Collagen: quantification, biomechanics and role of minor subtypes in cartilage. Nature Reviews Materials. 5(10), 730–747.
Broutier, L., Andersson-Rolf, A., Hindley, C. J., Boj, S. F., Clevers, H., Koo, B-K., et al. (2016). Culture and establishment of self-renewing human and mouse adult liver and pancreas 3D organoids and their genetic manipulation. Nat. Protoc. 11, 1724–1743. doi:10.1038/nprot.2016.097
Clevers, H. (2016). Modeling development and disease with organoids. Cell 165, 1586–1597. doi:10.1016/j.cell.2016.05.082
Crispim, J. F., and Ito, K. (2021). De novo neo-hyaline-cartilage from bovine organoids in viscoelastic hydrogels. Acta Biomater. 128, 236–249. doi:10.1016/j.actbio.2021.04.008
Cui, H., Wang, Y., Huang, H., Yu, W., Bai, M., Zhang, L., et al. (2014). GPR126 protein regulates developmental and pathological angiogenesis through modulation of VEGFR2 receptor signaling. J. Biol. Chem. 289, 34871–34885.
d'Aldebert, E., Quaranta, M., Sebert, M., Bonnet, D., Kirzin, S., Portier, G., et al. (2020). Characterization of human colon organoids from inflammatory bowel disease patients, Front. Cell Dev. Biol. 8, 363. doi:10.3389/fcell.2020.00363
Delplace, V., Boutet, M. A., Le Visage, C., Maugars, Y., Guicheux, J., and Vinatier, C. (2021). Osteoarthritis: From upcoming treatments to treatments yet to come. Jt. Bone Spine 88, 105206. doi:10.1016/j.jbspin.2021.105206
Doran, M. F., Pond, G. R., Crowson, C. S., O'Fallon, W. M., and Gabriel, S. E. (2002). Trends in incidence and mortality in rheumatoid arthritis in Rochester, Minnesota, over a forty-year period. Arthritis Rheum. 46, 625–631. doi:10.1002/art.509
Driehuis, E., Kretzschmar, K., and Clevers, H. (2020). Establishment of patient-derived cancer organoids for drug-screening applications. Nat. Protoc. 15, 3380–3409. doi:10.1038/s41596-020-0379-4
Dutta, D., Heo, I., and Clevers, H. (2017). Disease modeling in stem cell-derived 3D organoid systems. Trends Mol. Med. 23, 393–410. doi:10.1016/j.molmed.2017.02.007
Engevik, K. A., Hanyu, H., Matthis, A. L., Zhang, T., Frey, M. R., Oshima, Y., et al. (2019). Trefoil factor 2 activation of CXCR4 requires calcium mobilization to drive epithelial repair in gastric organoids. J. Physiol. 597, 2673–2690. doi:10.1113/JP277259
Feng, C., Chan, W. C. W., Lam, Y., Wang, X., Chen, P., Niu, B., et al. (2019). Lgr5 and Col22a1 mark progenitor cells in the lineage toward juvenile articular chondrocytes. Stem Cell Rep. 13, 713–729. doi:10.1016/j.stemcr.2019.08.006
Foltz, L., Levy, T., Possemato, A., and Grimes, M. (2021). Craniofacial cartilage organoids from human embryonic stem cells via a neural crest cell intermediate. bioRxiv.
Franceschi, C., and Campisi, J. (2014). Chronic inflammation (inflammaging) and its potential contribution to age-associated diseases. J. Gerontol. A Biol. Sci. Med. Sci. 69 Suppl 1, S4–S9. doi:10.1093/gerona/glu057
Gjorevski, N., Sachs, N., Manfrin, A., Giger, S., Bragina, M. E., Ordonez-Moran, P., et al. (2016). Designer matrices for intestinal stem cell and organoid culture. Nature 539, 560–564. doi:10.1038/nature20168
Goronzy, J. J., Li, G., Yang, Z., and Weyand, C. M. (2013). The janus head of T cell aging - autoimmunity and immunodeficiency. Front. Immunol. 4, 131. doi:10.3389/fimmu.2013.00131
Gryadunova, A., Kasamkattil, J., Gay, M. H. P., Dasen, B., Pelttari, K., Mironov, V., et al. (2021). Nose to spine: Spheroids generated by human nasal chondrocytes for scaffold-free nucleus pulposus augmentation. Acta Biomater. 134, 240–251. doi:10.1016/j.actbio.2021.07.064
Guilak, F., Nims, R. J., Dicks, A., Wu, C. L., and Meulenbelt, I. (2018). Osteoarthritis as a disease of the cartilage pericellular matrix. Matrix Biol. 71-72, 40–50. doi:10.1016/j.matbio.2018.05.008
Hall, G. N., Tam, W. L., Andrikopoulos, K. S., Casas-Fraile, L., Voyiatzis, G. A., Geris, L., et al. (2021). Patterned, organoid-based cartilaginous implants exhibit zone specific functionality forming osteochondral-like tissues in vivo. Biomaterials 273, 120820. doi:10.1016/j.biomaterials.2021.120820
Hirota, A., AlMusawi, S., Nateri, A. S., Ordonez-Moran, P., and Imajo, M. (2021). Biomaterials for intestinal organoid technology and personalized disease modeling. Acta Biomater. 132, 272–287. doi:10.1016/j.actbio.2021.05.010
Hu, J. L., Todhunter, M. E., LaBarge, M. A., and Gartner, Z. J. (2018). Opportunities for organoids as new models of aging. J. Cell Biol. 217, 39–50. doi:10.1083/jcb.201709054
Huang, W. K., Wong, S. Z. H., Pather, S. R., Nguyen, P. T. T., Zhang, F., Zhang, D. Y., et al. (2021). Generation of hypothalamic arcuate organoids from human induced pluripotent stem cells. Cell Stem Cell 28, 1657–1670.e10. e1610. doi:10.1016/j.stem.2021.04.006
Huey, D. J., Hu, J. C., and Athanasiou, K. A. (2012). Unlike bone, cartilage regeneration remains elusive. Science 338, 917–921. doi:10.1126/science.1222454
Irie, Y., Mizumoto, H., Fujino, S., and Kajiwara, T. (2008). Development of articular cartilage grafts using organoid formation techniques. Transpl. Proc. 40, 631–633. doi:10.1016/j.transproceed.2008.01.024
Josephson, A. M., Bradaschia-Correa, V., Lee, S., Leclerc, K., Patel, K. S., Muinos Lopez, E., et al. (2019). Age-related inflammation triggers skeletal stem/progenitor cell dysfunction. Proc. Natl. Acad. Sci. U. S. A. 116, 6995–7004. doi:10.1073/pnas.1810692116
Khedgikar, V., Charles, J. F., and Lehoczky, J. A. (2022). Mouse LGR6 regulates osteogenesis in vitro and in vivo through differential ligand use. Bone 155, 116267. doi:10.1016/j.bone.2021.116267
Kopper, O., de Witte, C. J., Lohmussaar, K., Valle-Inclan, J. E., Hami, N., Kester, L., et al. (2019). An organoid platform for ovarian cancer captures intra- and interpatient heterogeneity. Nat. Med. 25, 838–849. doi:10.1038/s41591-019-0422-6
Kronemberger, G. S., Matsui, R. A. M., Miranda, G., Granjeiro, J. M., and Baptista, L. S. (2020). Cartilage and bone tissue engineering using adipose stromal/stem cells spheroids as building blocks. World J. Stem Cells 12, 110–122. doi:10.4252/wjsc.v12.i2.110
Labbe, K., Murley, A., and Nunnari, J. (2014). Determinants and functions of mitochondrial behavior. Annu. Rev. Cell Dev. Biol. 30, 357–391. doi:10.1146/annurev-cellbio-101011-155756
Lacko, L. A., and Chen, S. (2019). Organoid-based chemical approach to dissect the mechanism controlling cellular dynamics. J. Mol. Cell Biol. 12, 666–667. doi:10.1093/jmcb/mjz100
Laforge, M., Rodrigues, V., Silvestre, R., Gautier, C., Weil, R., Corti, O., et al. (2016). NF-κB pathway controls mitochondrial dynamics. Cell Death Differ. 23, 89–98. doi:10.1038/cdd.2015.42
Lee, Y. H., Sharma, A. R., Jagga, S., Lee, S. S., and Nam, J. S. (2021). Differential expression patterns of rspondin family and leucine-rich repeat-containing G-protein coupled receptors in chondrocytes and osteoblasts. Cell J. 22, 437–449. doi:10.22074/cellj.2021.6927
Leijten, J., Teixeira, L. S., Bolander, J., Ji, W., Vanspauwen, B., Lammertyn, J., et al. (2016). Bioinspired seeding of biomaterials using three dimensional microtissues induces chondrogenic stem cell differentiation and cartilage formation under growth factor free conditions. Sci. Rep. 6, 36011. doi:10.1038/srep36011
Li, M., Abrahante, J. E., Vegoe, A., Chai, Y. W., Lindborg, B., Toth, F., et al. (2021). Self-organized emergence of hyaline cartilage in hiPSC-derived multi-tissue organoids. bioRxiv.
Li, R., Guan, Z., Bi, S., Wang, F., He, L., Niu, X., et al. (2022). The proton-activated G protein-coupled receptor GPR4 regulates the development of osteoarthritis via modulating CXCL12/CXCR7 signaling. Cell Death Dis. 13(2), 1–14. doi:10.1038/s41419-021-04455-4
Li, M., and Izpisua Belmonte, J. C. (2019). Organoids—preclinical models of human disease. N. Engl. J. Med. 380, 569–579.
Li, Z. A., Shang, J., Xiang, S., Li, E. N., Yagi, H., Riewruja, K., et al. (2022). Articular tissue-mimicking organoids derived from mesenchymal stem cells and induced pluripotent stem cells. Organoids 1, 135–148. doi:10.3390/organoids1020011
Lim, K., Donovan, A. P., Tang, W., Sun, D., He, P., Pett, J. P., et al. (2023). Organoid modeling of human fetal lung alveolar development reveals mechanisms of cell fate patterning and neonatal respiratory disease. Cell Stem Cell. 30(1), 20–37.
Liu, Y., Yang, M., Deng, Y., Su, G., Enninful, A., Guo, C. C., et al. (2020). High-spatial-resolution multi-omics sequencing via deterministic barcoding in tissue. Cell 183, 1665–1681. doi:10.1016/j.cell.2020.10.026
Liao, S., Meng, H., Li, J., Zhao, J., Xu, Y., Wang, A., et al. (2021). Potential and recent advances of microcarriers in repairing cartilage defects. J. Orthop. Translat. 27, 101–109.
Luo, J., and Li, P. (2021). Human pluripotent stem cell-derived brain organoids as in vitro models for studying neural disorders and cancer. Cell Biosci. 11, 99. doi:10.1186/s13578-021-00617-1
Lin, W., Yang, Z., Shi, L., Wang, H., Pan, Q., Zhang, X., et al. (2022). Alleviation of osteoarthritis by intra-articular transplantation of circulating mesenchymal stem cells. Biochem. Biophys. Res. Commun. 636, 25–32.
Luo, J., Zhou, W., Zhou, X., Li, D., Weng, J., Yi, Z., et al. (2009). Regulation of bone formation and remodeling by G-protein-coupled receptor 48. Development 136, 2747–2756. doi:10.1242/dev.033571
Luo, J., Zou, H., Guo, Y., Huang, K., Ngan, E. S., and Li, P. (2022). BACE2 variant identified from HSCR patient causes AD-like phenotypes in hPSC-derived brain organoids. Cell Death Discov. 8, 47. doi:10.1038/s41420-022-00845-5
Miller, A. J., Dye, B. R., Ferrer-Torres, D., Hill, D. R., Overeem, A. W., Shea, L. D., et al. (2019). Generation of lung organoids from human pluripotent stem cells in vitro. Nat. Protoc. 14, 518–540. doi:10.1038/s41596-018-0104-8
Montgomery, M. K., Osborne, B., Brandon, A. E., O'Reilly, L., Fiveash, C. E., Brown, S. H. J., et al. (2019). Regulation of mitochondrial metabolism in murine skeletal muscle by the medium-chain fatty acid receptor Gpr84. FASEB J. 33, 12264–12276. doi:10.1096/fj.201900234R
Murakami, K., Terakado, Y., Saito, K., Jomen, Y., Takeda, H., Oshima, M., et al. (2021). A genome-scale CRISPR screen reveals factors regulating Wnt-dependent renewal of mouse gastric epithelial cells. Proc. Natl. Acad. Sci. U. S. A. 118, e2016806118. doi:10.1073/pnas.2016806118
Neal, J. T., Li, X., Zhu, J., Giangarra, V., Grzeskowiak, C. L., Ju, J., et al. (2018). Organoid modeling of the tumor immune microenvironment. Cell 175, 1972–1988. doi:10.1016/j.cell.2018.11.021
Nilsson Hall, G., Mendes, L. F., Gklava, C., Geris, L., Luyten, F. P., and Papantoniou, I. (2020). Developmentally engineered callus organoid bioassemblies exhibit predictive in vivo long bone healing. Adv. Sci. (Weinh) 7, 1902295. doi:10.1002/advs.201902295
O'Connor, S. K., Katz, D. B., Oswald, S. J., Groneck, L., and Guilak, F. (2021). Formation of osteochondral organoids from murine induced pluripotent stem cells. Tissue Eng. Part A 27, 1099–1109. doi:10.1089/ten.TEA.2020.0273
Peng, W. C., de Lau, W., Forneris, F., Granneman, J. C., Huch, M., Clevers, H., et al. (2013). Structure of stem cell growth factor R-spondin 1 in complex with the ectodomain of its receptor LGR5. Cell Rep. 3, 1885–1892. doi:10.1016/j.celrep.2013.06.009
Rothbauer, M., Byrne, R. A., Schobesberger, S., Olmos Calvo, I., Fischer, A., Reihs, E. I., et al. (2021). Establishment of a human three-dimensional chip-based chondro-synovial coculture joint model for reciprocal cross talk studies in arthritis research. Lab. Chip 21, 4128–4143. doi:10.1039/d1lc00130b
Sanada, F., Taniyama, Y., Muratsu, J., Otsu, R., Shimizu, H., Rakugi, H., et al. (2018). Source of chronic inflammation in aging. Front. Cardiovasc Med. 5, 12. doi:10.3389/fcvm.2018.00012
Schon, B. S., Hooper, G. J., and Woodfield, T. B. (2017). Modular tissue assembly strategies for biofabrication of engineered cartilage. Ann. Biomed. Eng. 45, 100–114. doi:10.1007/s10439-016-1609-3
Schroter-Kermani, C., Hinz, N., Risse, P., Zimmermann, B., and Merker, H. J. (1991). The extracellular matrix in cartilage organoid culture: Biochemical, immunomorphological and electron microscopic studies. Matrix 11, 428–441. doi:10.1016/s0934-8832(11)80197-6
Shin, J., Hong, S. G., Choi, S. Y., Rath, M. E., Saredy, J., Jovin, D. G., et al. (2022). Flow-induced endothelial mitochondrial remodeling mitigates mitochondrial reactive oxygen species production and promotes mitochondrial DNA integrity in a p53-dependent manner. Redox Biol. 50, 102252. doi:10.1016/j.redox.2022.102252
Singh, Y. P., Moses, J. C., Bhardwaj, N., and Mandal, B. B. (2021). Overcoming the dependence on animal models for osteoarthritis therapeutics–the promises and prospects of in vitro models. Adv. Healthc. Mater. 10, 2100961.
Sun, Y., Wu, Q., Dai, K., You, Y., and Jiang, W. (2021). Generating 3D-cultured organoids for pre-clinical modeling and treatment of degenerative joint disease. Signal Transduct. Target Ther. 6, 380. doi:10.1038/s41392-021-00675-4
Takasato, M., Er, P. X., Chiu, H. S., and Little, M. H. (2016). Generation of kidney organoids from human pluripotent stem cells. Nature protocols, 11(9), 1681–1692.
Tam, W. L., Freitas Mendes, L., Chen, X., Lesage, R., Van Hoven, I., Leysen, E., et al. (2021). Human pluripotent stem cell-derived cartilaginous organoids promote scaffold-free healing of critical size long bone defects. Stem Cell Res. Ther. 12, 513. doi:10.1186/s13287-021-02580-7
Tran-Khanh, N., Hoemann, C. D., McKee, M. D., Henderson, J. E., and Buschmann, M. D. (2005). Aged bovine chondrocytes display a diminished capacity to produce a collagen-rich, mechanically functional cartilage extracellular matrix. J. Orthop. Res. 23, 1354–1362. doi:10.1016/j.orthres.2005.05.009.1100230617
Trengove, A., Di Bella, C., and O'Connor, A. J. (2022). The challenge of cartilage integration: Understanding a major barrier to chondral repair. Tissue Eng. Part B Rev. 28, 114–128. doi:10.1089/ten.TEB.2020.0244
Vyas, D., Baptista, P. M., Brovold, M., Moran, E., Gaston, B., Booth, C., et al. (2018). Self-assembled liver organoids recapitulate hepatobiliary organogenesis in vitro. Hepatology 67, 750–761. doi:10.1002/hep.29483
Weyand, C. M., and Goronzy, J. J. (2004). Stem cell aging and autoimmunity in rheumatoid arthritis. Trends Mol. Med. 10, 426–433. doi:10.1016/j.molmed.2004.07.006
Weyand, C. M., Yang, Z., and Goronzy, J. J. (2014). T-cell aging in rheumatoid arthritis. Curr. Opin. Rheumatol. 26, 93–100. doi:10.1097/BOR.0000000000000011
Xinaris, C. (2019). Organoids for replacement therapy: Expectations, limitations and reality. Curr. Opin. Organ. Transplant. 24, 555–561.
Yuan, Y., Chen, H., Ou, S., Cai, B., Zhang, R., Qin, Y., et al. (2022). Generation of mitochondria-rich kidney organoids from expandable intermediate mesoderm progenitors reprogrammed from human urine cells under defined medium. Cell & Bioscience, 12(1), 174.
Zhou, Y., Chen, M., Ricupero, C. L., He, L., Wu, J., Chen, K., et al. (2018). Profiling of stem/progenitor cell regulatory genes of the synovial joint by genome-wide RNA-seq analysis. Biomed. Res. Int. 2018, 9327487. doi:10.1155/2018/9327487
Keywords: cartilage organoids, LGR5, mini-joint, regenerative therapy, stem cells
Citation: Lin W, Wang M, Xu L, Tortorella M and Li G (2023) Cartilage organoids for cartilage development and cartilage-associated disease modeling. Front. Cell Dev. Biol. 11:1125405. doi: 10.3389/fcell.2023.1125405
Received: 16 December 2022; Accepted: 09 January 2023;
Published: 30 January 2023.
Edited by:
Quanbo Ji, Chinese PLA General Hospital, ChinaReviewed by:
Pengzhen Cheng, Fourth Military Medical University, ChinaCopyright © 2023 Lin, Wang, Xu, Tortorella and Li. This is an open-access article distributed under the terms of the Creative Commons Attribution License (CC BY). The use, distribution or reproduction in other forums is permitted, provided the original author(s) and the copyright owner(s) are credited and that the original publication in this journal is cited, in accordance with accepted academic practice. No use, distribution or reproduction is permitted which does not comply with these terms.
*Correspondence: Weiping Lin, d2VpcGluZy5saW5AY3JtaC1jYXMub3JnLmhr; Liangliang Xu, eHVsbC0yMDE2QGd6dWNtLmVkdS5jbg==; Micky Tortorella, bS50b3J0b3JlbGxhQGdpYmguYWMuY24=; Gang Li, Z2FuZ2xpQGN1aGsuZWR1Lmhr