- 1Department of Orthopaedic Surgery and Biomedical Engineering, University of Tennessee Health Science Center, Memphis, TN, United States
- 2College of Medicine, University of Tennessee Health Science Center, Memphis, TN, United States
- 3Department of Biological Sciences, University of Memphis, Memphis, TN, United States
- 4Center for Cancer Research, University of Tennessee Health Science Center, Memphis, TN, United States
- 5Department of Medicine, Division of Hematology and Oncology, College of Medicine, University of Tennessee Health Science Center, Memphis, TN, United States
WNT10B, a member of the WNT family of secreted glycoproteins, activates the WNT/β-catenin signaling cascade to control proliferation, stemness, pluripotency, and cell fate decisions. WNT10B plays roles in many tissues, including bone, adipocytes, skin, hair, muscle, placenta, and the immune system. Aberrant WNT10B signaling leads to several diseases, such as osteoporosis, obesity, split-hand/foot malformation (SHFM), fibrosis, dental anomalies, and cancer. We reviewed WNT10B a decade ago, and here we provide a comprehensive update to the field. Novel research on WNT10B has expanded to many more tissues and diseases. WNT10B polymorphisms and mutations correlate with many phenotypes, including bone mineral density, obesity, pig litter size, dog elbow dysplasia, and cow body size. In addition, the field has focused on the regulation of WNT10B using upstream mediators, such as microRNAs (miRNAs) and long non-coding RNAs (lncRNAs). We also discussed the therapeutic implications of WNT10B regulation. In summary, research conducted during 2012–2022 revealed several new, diverse functions in the role of WNT10B in physiology and disease.
1 Introduction
WNT10B was first discovered by the Leder group in the mammary gland of mice in 1995 (Lee et al., 1995). Since then, WNT10B has been shown to play important roles in many tissue types in normal development (including bone, adipocytes, teeth, skin, hair, immune system, muscle, placenta, and heart) and diseases [including cancer, obesity, osteoporosis, and split-hand/foot malformation (SHFM)]. WNT10B was reviewed a decade ago (Wend et al., 2012). Here, we provide an update on the current understanding of WNT10B in normal development and disease from 2012 to 2022.
2 WNT10B signaling
WNT ligands (e.g., WNT1, WNT3A, and WNT10B) can activate signal transduction in a β-catenin-dependent manner, referred to as “canonical” WNT/β-catenin signaling, and/or in a β-catenin-independent manner (e.g., WNT5A, WNT5B, and WNT7A), referred to as “non-canonical” WNT/β-catenin signaling. WNT10B is considered a “canonical”/β-catenin-dependent WNT ligand. Many WNT signaling reviews have summarized the signaling pathway with data combined from a variety of species and WNT ligands (MacDonald et al., 2009; Rim et al., 2022). Here, we will specifically summarize what is known for WNT10B (Figure 1).
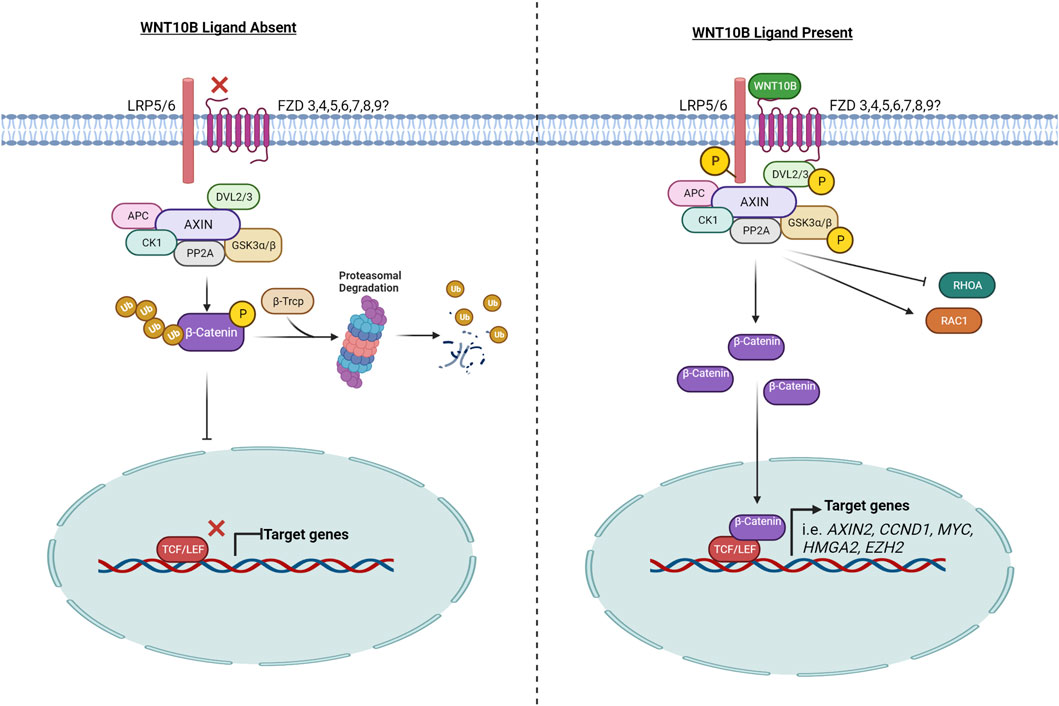
FIGURE 1. WNT10B signaling pathway. The left side of the figure represents the signaling pathway when WNT10B is absent and the signaling pathway is off. The right side of the figure models the signaling pathway when WNT10B is present, following from receptor binding to target gene activation. P, phosphorylation; Ub, ubiquitination. Created with BioRender.com.
Canonical WNTs bind to LRP5 and/or LRP6 and a frizzled (FZD) co-receptor to initiate signaling. WNT10B has been shown to interact with both LRP5 and LRP6 in different tissue-specific contexts. In the prostate cancer cell line PC3, WNT10B was shown by proximal ligation assay (PLA) to co-localize with FZD6 and LRP6 and then induce nuclear β-catenin in 5 min (Neuhaus et al., 2021). In osteoblasts, WNT10B upregulates the transcription of fatty acid metabolism genes via LRP5 (but not LRP6) (Frey et al., 2015). In addition, WNT10B expression is often correlated with LRP5 expression (Belaya et al., 2018; Li Z. et al., 2021). LRP5 and LRP6 are both required for WNT signaling reporter assay activity in response to WNT10B in mouse embryonic fibroblasts (Goel et al., 2012).
A molecular docking simulation study was performed to predict the binding affinity of WNTs with various human FZDs, and it predicted that human WNT10B has the highest binding affinity with human FZD3, 4, 5, 6, 7, 8, and 9 (Agostino et al., 2017). Tissue-specific receptor and co-receptor expression may determine ligand binding. Specifically in acute myeloid leukemia (AML), WNT10B signals through FZD4 and FZD5, while in T-cell acute lymphoblastic leukemia, WNT10B signals through FZD6 (Lazzaroni et al., 2016; Cassaro et al., 2021). WNT10B also interacted with FZD6 in a T-cell acute lymphoblastic leukemia cell line and HEK293T cells, as demonstrated by immunoprecipitation and PLA (Lu et al., 2019; Cassaro et al., 2021). In BeWo choriocarcinoma cells, WNT10B signaling requires FZD7 for migration but not the other expressed FZD family members (FZD1, FZD3, FZD5, and FZD10) (Wang et al., 2019).
The signaling output of the canonical WNT pathway is determined by the level of cytosolic β-catenin, which is under the strict control of the “destruction complex.” The core destruction complex is composed of AXIN, APC, and two constitutively active kinases [casein kinase 1 (CK1) and glycogen synthase kinase 3 (GSK3α/β)], which associate with β-catenin and promote its polyubiquitination by phosphorylating the degron motif of β-catenin (Stamos and Weis, 2013). Phosphorylated β-CATENIN is presented to the proteasome through its interaction with the F-box/WD-repeat containing E3-ligase protein β-TrCP, an adaptor protein that forms a complex with the SKP1/Cullin machinery, to facilitate assembly of K48-linked polyubiquitin chains on β-catenin, leading to it proteasome-dependent degradation (Kitagawa et al., 1999).
WNT ligands, including WNT10B (Lee et al., 2013), lead to the phosphorylation of GSK3β, which inhibits the destruction complex. Therefore, WNT10B stabilizes and activates β-catenin, as demonstrated by analysis of β-catenin (Abiola et al., 2009; Lei et al., 2015). Increased cytoplasmic β-catenin then translocates into the nucleus where it binds to members of the T cell factor/lymphoid enhancer factor (TCF/LEF) transcription factor family to drive transcription of WNT/β-catenin target genes, such as MYC, CCND1, and AXIN2. WNT10B activates the TOPFLASH reporter, a canonical WNT signaling reporter with TCF/LEF binding sites, which has been widely used as a readout of activated WNT10B signaling (Christodoulides et al., 2006; Suthon et al., 2022a).
WNT binding to FZD helps recruit DVL to the plasma membrane. Recruitment of DVL to the membrane provides a platform for AXIN and GSK3β to bind and phosphorylate LRP5/6, thereby preventing constitutive degradation of β-catenin (Sharma et al., 2018). In several studies, WNT10B was shown to induce DVL2 and/or DVL3 phosphorylation (Chen et al., 2008; Golestaneh et al., 2009; Lee and Heur, 2015).
WNT10B signals through RAC1, which is part of the canonical and non-canonical pathways. WNT10B can signal via FZD7 to activate RAC1 in placental extravillous trophoblasts to mediate migration (Wang et al., 2019). In corneal endothelial cells, WNT10B treatment caused an activation of RAC1 and an association of RAC1 and β-catenin in the nucleus. In contrast, WNT10B inhibited RHOA (a member of the same GTPase family as RAC1) activity (Lee and Heur, 2015).
3 WNT10B and the mammary gland
WNT10B, which was first identified in the mammary gland, plays a very fundamental role in mammary gland development. It is the earliest (E11.5) expressed WNT ligand in the mammary anlagen and is a characteristic of the definitive mammary line (Veltmaat et al., 2004). However, research in the past decade has not focused on WNT10B in the normal mammary gland but on its role in breast cancer and other tissues (discussed in following sections).
4 WNT10B and bone
WNT signaling is an important regulator of bone. Human mutations in LRP5 and SOST (sclerostin) alter WNT signaling and cause osteoporosis-pseudoglioma syndrome (OPPG) and van Buchem disease. Romosozumab (EVENITY), an antibody to sclerostin which activates WNT signaling, is an FDA-approved therapy for osteoporosis. In mice, knockouts of LRP5, LRP6, β-catenin, and many WNT ligands each have skeletal phenotypes (Maupin et al., 2013). In the previous decade, WNT10B-knockout mice and transgenic mice demonstrated the importance of WNT10B in bone and bone marrow-derived mesenchymal stem cells (Bennett et al., 2005; Bennett et al., 2007; Stevens et al., 2010). More recently, studies have focused on the regulation of WNT10B in bone and the therapeutic applications of WNT10B in bone.
Analysis of WNT10B single nucleotide polymorphisms (SNPs) has also supported a role for WNT10B in bone. A 2009 study demonstrated an association of two SNPs (rs1051886 and rs3741627) in the WNT10B gene with high hip bone-mass density and increased bone size in Afro-Caribbean men (Zmuda et al., 2009). A study on Danish men also showed a correlation of SNP rs10875902 (which is in high linkage disequilibrium with rs1051886) with bone mineral density (Van Camp et al., 2013). However, WNT10B-targeted studies in Spanish (Perez-Castrillon et al., 2009) and Chinese (Zheng et al., 2016) populations, as well as large GWAS (Estrada et al., 2012; Liu et al., 2020c), did not identify any correlations between WNT10B SNPs and bone properties.
WNT10B affects mesenchymal stem cells (MSCs), osteoblasts, osteoclasts, and T cells, which contribute to bone homeostasis. The effects of WNT10B from T cells affecting the bone are discussed later in the review (see Section 5: WNT10B and the immune system).
MSCs differentiate into osteoblasts, adipocytes, chondrocytes, myocytes, and other mesenchymal tissues. Osteoblasts and adipocytes have an inverse relationship; factors that increase osteoblastogenesis tend to decrease adipogenesis and vice versa. WNT10B is considered a master negative regulator of adipocyte differentiation (see Section 8). Thus, WNT10B is often used as a marker of increased osteoblastogenesis, decreased adipogenesis, or both (Liu et al., 2016; Matsushita et al., 2016; Yi et al., 2019; Palmieri et al., 2021), for example in bone marrow adipocytes (Georgiou et al., 2015; Lee et al., 2019).
An interesting model to study bone biology is the scales of goldfish. Teleost scales are formed by intramembranous ossification and comprise osteoblasts and osteoclasts. Tazaki et al. (2018) detected WNT10B by qPCR and in situ hybridization in multinucleated osteoclasts in fish scales. Furthermore, the inhibition of WNT10B with an anti-WNT10B antibody increased RANKL expression, increasing osteoclast differentiation (Tazaki et al., 2018), thereby supporting the role of WNT10B in increasing bone mineral density.
The majority of studies on WNT10B in the bone have focused on osteoblasts, and WNT10B is also expressed in osteoclasts. It is hypothesized that the WNT10B produced from osteoclasts links the coupling of osteoclasts and osteoblasts in the resorption cycle, inducing paracrine signaling to osteoblasts from the osteoclasts (Ota et al., 2013; Zheng et al., 2019). A loss-of-function mutation of c-kit in mice led to an increase in osteoclast expression of WNT10B and an increase in osteoblast differentiation and mineralization that could be blocked with a WNT10B neutralizing antibody or addition of recombinant DKK1 (Lotinun and Krishnamra, 2016). In the studies on WNT10B expression in osteoclasts, no direct effect of WNT10B on osteoclasts is shown, only the effect on osteoblasts. Therefore, it is unknown if WNT10B affects the osteoclast directly. Wnt10b-knockout mice or Wnt10b overexpressing mice (osteocalcin-Cre) have no differences in osteoclast numbers compared to wild-type mice (Bennett et al., 2007; Stevens et al., 2010), although the Wnt10b overexpressing mice have an increase in the bone resorption marker CTX (a collagen metabolite produced by type I collagen degradation due to osteoclast activity).
4.1 Regulation of WNT10B in bone
RUNX2 is considered the master transcription factor of osteoblasts, as it is necessary for osteoblast differentiation and induces many other osteoblast differentiation genes. WNT10B upregulates RUNX2 expression (Bennett et al., 2005), and transcription factors downstream of WNT signaling (β-catenin and TCF1) bind directly to the RUNX2 promoter (Gaur et al., 2005). In addition, WNT10B is a direct target of RUNX2, as RUNX2 binds to the WNT10B promoter, overexpression of RUNX2 increases WNT10B expression, and knockout of RUNX2 in osteoblasts decreases WNT10B expression (Qin et al., 2019; Qin et al., 2021). CBFβ, a non-DNA-binding partner of Runt-related transcription factors (RUNX1, RUNX2, and RUNX3), is also recruited to the promoter of WNT10B (Wu M. et al., 2017) to upregulate the transcription of WNT10B. Cbfβ-knockout mice have a low bone-mass phenotype similar to WNT10B knockout mice.
GATA4 binds at the promoter of WNT10B and at a downstream enhancer, as discovered by ChIP-sequencing. Furthermore, knockout of Gata4 decreases Wnt10b expression, corresponding with a decrease in trabecular bone properties. GATA4 also transcriptionally regulates other components of the WNT signaling pathway, including Wnt3a, Fzd6, and Dkk1 (Khalid et al., 2021; Suthon et al., 2022b).
BMP9, an osteogenic bone morphogenic protein (BMP) family member, induces phosphorylation of SMAD1/5/8, which binds to the promoter of Wnt10b, along with pCREB, leading to the upregulation of Wnt10b. Furthermore, the combination of BMP9 and WNT10B increases the expression of Runx2 and alkaline phosphatase (ALP) and induces mineralization, compared to either factor alone (Liao et al., 2019; Liang et al., 2020). Conversely, PTEN, which is anti-osteogenic, can decrease BMP9-mediated increases in Wnt10b expression (Li et al., 2020a).
Post-transcriptionally, WNT10B is regulated by microRNAs (miRNAs) and long non-coding RNAs (lncRNAs, Table 1). The expression of maternally expressed gene 3 (MEG3), a lncRNA, is upregulated in bones with a non-union fracture and binds to the promoter of Wnt10b to suppress its expression. Silencing of MEG3 leads to an increase in WNT10B protein levels and faster fracture healing (Liu Y. B. et al., 2019). miR-33b-5p binds to the 3’ end of WNT10B mRNA to regulate its protein expression. The expression of long intergenic non-protein coding RNA 2349 (linc02349) increases during osteogenesis and acts as a molecular “sponge” for miR-33b-5p, thus increasing the levels of WNT10B (Cao et al., 2020). In comparison, the knockout of Dicer1, an endoribonuclease in the miRNA maturation process, led to a decrease in Wnt10b expression and less osteoblast differentiation (Wu H. Y. et al., 2019).
In osteoclasts, calcitonin, a peptide hormone secreted from the parafollicular cells of the thyroid gland that inhibits osteoclastic resorption, has been shown to increase WNT10B expression (Hsiao et al., 2020). Similarly, calcitriol (vitamin D3) decreases osteoclast differentiation in vitro and simultaneously increases the expression of WNT10B (Lu C. L. et al., 2018). Several other factors, including TGFβ and the chronic kidney disease drug cinacalcet, a calcimimetic, also increase the expression of WNT10B in osteoclasts (Ota et al., 2013; Zheng et al., 2019).
4.2 WNT10B as a therapeutic agent in bone
Non-union fractures occur in 5%–10% of fractures (Panteli et al., 2022). Surgical treatments include bone graft or bone graft substitute, internal fixation, and/or external fixation. Each has advantages and disadvantages, and improved solutions are being investigated. WNT10B has been suggested as a growth factor that can improve bone healing in the following studies. A WNT10B-expressing lentivirus was shown to be sufficient to heal an atrophic non-union fracture in rat femurs without the addition of a scaffold or cells (Gao et al., 2015). In comparison, human umbilical cord MSCs that overexpress WNT10B resulted in better mineralization and accelerated bone defect healing in a calvarial defect model (Liu et al., 2020g). Similarly, human umbilical cord MSCs transfected with WNT10B in hydrogel placed into a femoral fracture accelerated healing. While there was a similar amount of a cartilaginous callus at 2 weeks, WNT10B induced more bone formation 4 weeks after the fracture. WNT10B also induced more angiogenesis at the site of the fracture, which is an important step in fracture healing (Hu et al., 2022). Liu et al. (2018) used adenoviral-delivered WNT10B in bone marrow-derived mesenchymal stem cells in an ovariectomized rat model, and the expression of WNT10B accelerated osseointegration of a femoral implant (Liu et al., 2018). Hsu et al. (2020) used CRISPR activation of WNT10B (along with FOXC2) to overexpress WNT10B in bone marrow-derived mesenchymal stem cells to efficiently heal critical-sized calvarial defects in rats (Hsu et al., 2020). Based on these studies, WNT10B optimized for delivery, cell type, and scaffold could potentially be used in humans with non-union fractures to increase bone healing.
In addition, WNT10B could potentially be used to treat osteoporosis. Overexpression of Wnt10b in osteoblasts via the osteocalcin promoter prevented diabetes-induced bone loss and marrow adiposity but did not affect blood glucose levels. The probiotic Lactobacillus reuteri (L. reuteri) also prevented diabetes-induced bone loss while increasing Wnt10b expression in a total bone analysis (Zhang et al., 2015).
Furthermore, overexpression of Wnt10b in osteoblasts protected against glucocorticoid-induced osteoporosis (GIO), the most common cause of secondary osteoporosis. Glucocorticoids decrease trabecular bone, decrease expression of Wnt10b, and alter the gut microbiome. Mice exposed to prednisolone had a decrease in BV/TV (bone volume/total volume) and trabecular properties, but the Wnt10b transgenic mice did not have these decreases. The suppression of Wnt10b in bone after glucocorticoid treatment was restored by L. reuteri (Schepper et al., 2020), as in the diabetes model described previously. In another model of secondary osteoporosis, in which osteoporosis is induced by vitamin A, Lactobacillus plantarum HFY15 or Lactobacillus fermentum ZS40 isolated from yak yogurt restored BV/TV and other trabecular properties in rats and increased Wnt10b levels in the bone (Liu et al., 2020d; Liu et al., 2020f).
Tyagi et al. (2018) showed that the probiotic Lactobacillus rhamnosus GG also increased bone mass. The microbial metabolite butyrate, which is a short-chain fatty acid, is produced from probiotics, including Lactobacillus rhamnosus GG. Butyrate stimulated bone formation via T-regulatory cell (Treg) activation that mediates the upregulation of Wnt10b from bone marrow CD8+ T cells (Tyagi et al., 2018). Mechanistically, the increase in butyrate-activated Tregs promoted the assembly of a NFAT1–SMAD3 transcriptional complex to the Wnt10b promoter in CD8+ T cells, which drove the activation of the T cells to secrete WNT10B. The authors reduced the number of Tregs using anti-CD25 antibodies or in reconstitution experiments using either the TCRβ−/− or CD8+ T cells from Wnt10bKO mice, which prevented the butyrate-mediated bone formation effects. Mechanistically, this work demonstrated that the probiotic increased butyrate production in the gut and that the butyrate increased the expression of Wnt10b specifically in CD8+ T cells compared to the Zhang et al. (2015) and Schepper et al. (2020) studies that analyzed whole bones, which included many cell types. Butyrate was also required for intermittent parathyroid hormone (iPTH) to increase the number of Tregs and Treg production of WNT10B (Li et al., 2020b). Therefore, the actions of iPTH and WNT10B are paracrine effects of T cells on osteoblasts (Tyagi et al., 2018).
5 WNT10B and the immune system
WNT signaling has been shown to regulate a vast array of biological functions in the immune system, including hematopoietic stem cells (HSCs), normal and malignant hematopoiesis, self-renewal, proliferation, and terminal differentiation of immune cells (Roozen et al., 2012; Bigas et al., 2020; Roo and Staal, 2020). The transcription factors downstream of WNT signaling, T cell factor 1 (TCF1) (Falk et al., 1983), and lymphoid enhancing factor-1 (LEF1) (Castrop et al., 1995) were first identified in immune cells. Of the 19 WNT family members, 12 play a role in immune cells, notably WNT2B, WNT3A, WNT5A, WNT5B, and WNT10B (Wend et al., 2012).
WNT10B’s main functions in the immune system have been shown to arise from T cells. The thymus is the primary organ for T-cell development. As the cells develop, they undergo a negative and positive selection to ensure appropriate T-cell function and the prevention of autoimmune or autoreactive T cells. This is an intricate and complex procedure by which both thymic stromal cells, most notably dendritic cells, and the thymus epithelia play critical roles in normal T-cell lymphopoiesis. Wnt10b mRNA has been detected in embryonic thymic development at day 13 (E13) (Balciunaite et al., 2002). The mouse hematopoietic progenitors have migrated to the bone marrow at this stage, but thymic development has not been completed. Since our last review, important new findings on the role of WNT10B in immune cells are discussed in the following paragraphs.
Parathyroid hormone (PTH) regulates calcium and phosphate homeostasis, which has an impact on bone turnover. Primary hyperparathyroidism (PHP) manifests into an increased risk for bone fractures due to its catabolic effects on bone. In contrast, iPTH therapies using amino acids 1–34 of PTH are used clinically to treat post-menopausal osteoporosis. In 2009, the Pacifici Lab demonstrated that iPTH increases the production of WNT10B in bone marrow CD8+ T cells, increasing trabecular bone density (Terauchi et al., 2009), previously reviewed in Wend et al. (2012). A follow-up paper from the Pacifici Lab demonstrated that the ability of iPTH to induce WNT10B expression in T cells is dependent on the parathyroid hormone receptor (PPR). A T-cell-specific PPR disruption (T-cell PPRT-cells−/−mice) was generated by crossing PPRflx/flx mice with mice expressing Cre under the control of a T-cell-specific promoter (Lck). Comparisons of PPRflx/flx versus PPRT-cells−/− with or without iPTH therapies revealed that the PPRT-cells−/− mice had no increase in bone marrow density in response to iPTH and iPTH failed to induce the production of Wnt10b. The results argued that the iPTH influence on T cells to secrete WNT10B protein depends on T cells expressing PPR (Bedi et al., 2012).
Furthermore, iPTH treatment expands hematopoietic stem and progenitor cells (HSPCs), but the underlining mechanism has been unknown. Li et al. (2012) demonstrated that T cells were required for the expansion of short-term HPSC (ST-HPSCs) mediated by iPTH, as this effect was abrogated by the disruption of PPR signaling. Mechanistically, secretion of WNT10B was required from the T cells to expand ST-HPSCs. Concurrently, WNT10B also activated WNT signaling in bone marrow stromal cells (BM-SCs). In the absence of WNT10B expression in T cells, several bone marrow stromal cell genes (Ahr, Axin2, Cyr61, Nkd2, Tagin, Tgfβ3, Thhbs1, Twist1, and Wisp1) were not induced by iPTH therapies. These data prove that WNT10B secretion from the T cell is necessary for ST-HSPC expansion after iPTH therapies (Li et al., 2012).
In a follow-up manuscript, Li et al. (2013) showed that ovariectomized (OVX, mice lacking ovaries and consequently, estrogen production) female mice expanded ST-HPSCs, which was dependent on the T-cell costimulatory molecule CD40 ligand (CD40L). T-cell production of WNT10B required the expression of CD40L for OVX mice to expand ST-HPSCs and activate bone marrow stromal cells. This model would suggest that the use of antiestrogens would expand ST-HPSC function. Moreover, this activity requires CD40L expression on the T cells for the secretion of WNT10B (Li J. Y. et al., 2013). Robinson et al. (2015) demonstrated that T-cell expression of CD40L potentiated the activity of iPTH bone anabolic activity. CD40L−/− mice had decreased secretion of WNT10B from T cells, thus causing iPTH to be unable to increase trabecular bone density. Moreover, bone marrow stromal cells devoid of CD40L failed to upregulate the WNT10B-dependent WNT targets after iPTH therapy. This paper argues that CD40L expression is required for iPTH to induce the secretion of WNT10B from T cells (Robinson et al., 2015).
D’Amelio et al. (2015) evaluated the role of WNT10B production in T cells after iPTH therapy in a randomized trial of 82 women diagnosed with either osteoporosis or PHP. The randomized group included women treated with vitamin D and calcium alone (n = 22), 1–84 PTH (n = 42), or bisphosphonate ibandronate (n = 18). The results from the randomized trial indicated that patients with PHP did not increase WNT10B compared to healthy controls. In contrast, iPTH therapy increased WNT10B expression in T cells, compared to ibandronate treatment (D'Amelio et al., 2015). This was the first time it was shown that in humans, iPTH treatment increases WNT10B production in T cells but not in B cells or monocytes.
Cyclic adenosine monophosphate (cAMP) is generated following the engagement of the T-cell receptor (TCR) with antigen presented by antigen-presenting cells. cAMP-dependent phosphodiesterase (PDE) inhibitors such as pentoxifylline (PTX) can activate cAMP signaling. PTX, which activates cAMP signaling to downstream effectors PKA, had been recognized to increase bone formation, the mechanism of which was unknown. In a preclinical model, Roser-Page et al. (2022) used PTX to investigate bone turnover to determine if the mechanism of action was through T cells secreting WNT10B. PTX induced WNT10B production in CD3- and CD28-activated T cells. Suppression of cAMP mediator protein kinase A (PKA) decreased Wnt10b expression in the T cells. The increase in bone mass mediated by PTX occurs when T cells are activated.
WNT10B has differential expression in male and female immune cells. In wild-type CB57L/6 mice, the percentage of WNT10B-positive myeloid cells was higher in females than males. In contrast, males had higher levels of WNT10B-positive cells in lymphoid cells. Specifically in males, the absence of TNFα increased bone marrow WNT10B expression but significantly reduced the number of WNT10B-positive dendritic cells, CD4+ T cells, CD8+ T cells, macrophages, and granulocytes. These results suggest a possible role for TNFα activity in regulating the expression of WNT10B-positive immune cells. In contrast, ovariectomy reduced the expression of WNT10B in bone marrow cells, further demonstrating a role for estrogen and sex-specific regulation of WNT10B (Collins et al., 2017).
Periodontal disease (PDD) is a gum disease of the surrounding bone that supports the teeth and is mostly seen in adults. Wolf et al. (2016) used human periodontal ligament (hPDL) cells, which can exhibit osteoblastic properties, to model inflammatory PDD (Wolf et al., 2016). hPDL cells from the third molar, isolated from healthy subjects aged 12–14 years with no signs of periodontitis, and enriched CD8+ T cells, isolated from matched individuals, were either cultured alone or in combination and exposed to PTH (1–34). WNT10B expression was observed only in the T cells when PTH was added. hPDL cells were treated with recombinant WNT10B (rWNT10B) to measure the direct effects of WNT10B on the hPDL cells. rWNT10B marginally increased the proliferation of the primary hPDL cells, and over 3 days, the hPDL cells had increased osteoblast differentiation markers (alkaline phosphatase and osteocalcin protein levels). A co-culturing system with both the T cells and hPDL plus PTH (1–34) increased alkaline phosphatase and osteocalcin relative to control. A WNT10B neutralizing antibody reversed the effects mediated by PTH.
In addition to affecting bone mineral density, probiotics have been shown to prevent obesity and modulate the immune system. The probiotic bacterium Clostridium butyricum (CB) produces butyrate, which is known to inhibit pro-inflammatory cytokines. Li H. et al. (2022) evaluated the effects of CB on fat deposition (Li H. et al., 2022). CB administration increased blood butyrate suppressing adipogenic markers and size distribution of inguinal white adipocyte tissue (iWAT) and epididymal WAT (eWAT). Butyrate effectively increased anti-inflammatory peripheral regulatory FoxP3+ T cells (Arpaia et al., 2013). Li et al. (2022) showed an increased number of FoxP3+CD4+ Tregs after CB administration in iWAT tissue. FACS-sorted Tregs had an increase in Wnt10b mRNA levels. CB administration had no effects on fat accumulation in Wnt10bKO mice. These results determined that CB’s ability to block fat accumulation requires the secretion of WNT10B protein from Tregs. The role of WNT10B in adipocytes is further described in Section 8.
Rheumatoid arthritis (RA) is an inflammatory autoimmune disease that causes crippling disabilities in over 1.3–1.5 million people affected by this disease in the United States. RA-associated bone loss, and cartilage destruction is mediated by autoantigen autoreactive T cells (Fournier, 2005). In RA, T cells infiltrate the synovial membranes and initiate macrophages and synovial fibroblasts to transform into a tissue-destructive force. CD3 and CD28 mediate T-cell activation. A CD28-costimulatory pharmacological inhibitor abatacept (CTLA-4Ig), which is FDA-approved for intractable RA, causes T-cell dormancy (T-cell anergy), a form of perpetual tolerance after antigen interaction. Roser-Page et al. (2014) determined that abatacept increases bone mass in young (3 months) and mature (6 months) mice. Abatacept-treated mice had 200-fold higher Wnt10b mRNA in total bone marrow cells compared to the Ig control, and in vitro isolated T cells had a sixfold increase in Wnt10b expression compared to control-treated cells. Isolated T cells, activated by CD3, increased Wnt10b mRNA. In contrast, when CD3 and CD28 were co-activated, the increase in the Wnt10b mRNA expression was reversed. An antigen-presenting cell (APC) assay was conducted with isolated CD11C dendritic cells cultured with ovalbumin-specific peptide to serve as the APC. The challenged DCs were then co-cultured with CD8+ T cells expressing ovalbumin-specific TCR cells. A combination of both cell types increased Wnt10b mRNA levels over those of the two cell types alone. When abatacept was added to the APC assay, a 20-fold increase in Wnt10b mRNA was observed. These results suggest a novel role of antigen-presenting dendritic cells in increasing the levels of Wnt10b expression (Roser-Page et al., 2014). Roser-Page et al. (2018) paradoxically determined that, in the absence of Wnt10b, there was a decrease in bone mass rather than no change in response to abatacept (Roser-Page et al., 2018). The authors attribute this to an increase in sclerostin (a WNT inhibitor) in the bone marrow stroma of wild-type mice, but this is not shown in the Wnt10bKO mice, so this is not convincing.
Asthma is a chronic inflammatory disease that is very common among children and is often associated with the activation of CD4+ T-helper 2 cells (CD4Th2). Trischler et al. (2016) used an in vivo house dust mite (HDM) asthma model. The HDM-treated mice demonstrate upregulation of Wnt10b mRNA in the lung and splenocyte-enriched T cells. The Wnt10bKO mice were challenged with HDM resulting in an increased number of eosinophils, EMBP protein expression (a marker of activated eosinophils), and Il4, Il13, Arg1, and Ccl2 mRNA expression over wild-type mice. This signature is consistent with either Th2 polarization or macrophage activation. Subsequently, splenic T cells were isolated from wild-type and Wnt10bKO mice, activated via CD3/CD28, and cocultured in the presence or absence of IL4 and IL12. Wnt10bKO T cells in the presence of IL4 increased GATA3 and IL4. GATA3 and IL4 expression levels are known to be produced from activated Th2. In contrast, Wnt10bKO T cells cultured in the presence of IL12 did not upregulate the Th1-promoting marker T-bet mRNA. Moreover, Wnt10bKO HDM-challenged mice increased effector T cells (CD4+CD44hiCD62Llo, CD4+CD69hiCD11ahi, and CD8+CD44hiCD62Llo). The results suggest that WNT10B expression regulates type 2 inflammation and the activation of a Th2 response in HDM-challenged mice.
Post-traumatic stress disorder (PTSD) frequently occurs in the aftermath of a psychologically traumatic event. PTSD is associated with a heightened inflammation that can be assessed by determining the levels of the inflammatory chemokines IL17A and interferon-gamma (IFNγ) in a patient’s peripheral blood mononuclear cells (PBMC). Bam et al. (2020) demonstrated increased levels of WNT10B in PTSD patients compared to healthy controls. miR-7113-5p was identified by microRNA expression analysis to be downregulated in PTSD patients and decreased the levels of WNT10B protein expression in a monocyte cell line (Table 1). rWNT10B treatment in human PBMCs in combination with PMA increased IFNγ expression significantly, relative to PMA treatment alone (Bam et al., 2020). In a follow-up study to determine how WNT10B is upregulated in PTSD, Bam et al. (2022) conducted RNA-sequencing on PBMCs of PTSD patients and determined that LINC008926 was upregulated compared to controls (Table 1). An increase in the open chromatin mark H3K4me3 at the promoter of WNT10B is due to LINC00896 recruiting a histone methyltransferase (MML1) onto the promoter of WNT10B. Knockdown experiments of MML1 showed a loss of WNT10B transcripts and a subsequent reduction in IFNγ. Conversely, the authors knocked down KDM5B (a histone demethylase) and increased the levels of the WNT10B transcript. rWNT10B added to preactivated PBMCs from healthy donors increased IL17A and IFNγ expression. ICG-001, which blocks CBP-mediated acetylation of β-catenin preventing active gene expression, significantly reduced the IL17A and IFNγ expression levels. Knockdown of LINC008926 in PBMCs and the lymphoblastic cell line TALL-107 decreased the WNT10B and IFNγ expression levels (Bam et al., 2022).
Severe infection elicits an inflammatory response, which causes an estimated 20,000 deaths worldwide, even when antibiotics are used. To better understand humans with sepsis, whole blood was analyzed for the correlation of inflammatory cytokines and WNT ligands. WNT10B correlated with the number of monocytes and expression of IL6 and TNFα, but not IL10, in patients with sepsis but not in healthy patients. In comparison, mice were administered LPS to induce endotoxemia, a model for human sepsis. In splenocytes, Wnt10b expression was significantly associated with Tnfα, Il12b, and Il10 after 1.5 h of LPS treatment. They further showed that canonical TLR4 signaling events via MYD88 mediated Wnt10b mRNA expression in response to LPS. Inhibition of WNT signaling by either ICG-001 or IWP-2 (a porcupine inhibitor, thereby inhibiting WNT secretion) reversed the cytokine signatures. Therefore, WNT ligands, including WNT10B, are part of the immune response during septic shock (Gatica-Andrades et al., 2017).
6 WNT10B and teeth
In 1998, WNT10B was shown to be expressed at the beginning of tooth development in epithelial cells, signaling to the mesenchyme (Dassule and McMahon, 1998). In the past decade, polymorphisms and mutations in WNT10B have been associated with tooth abnormalities.
Tooth agenesis is a developmental absence of certain teeth. Up to 20% of people are missing their third molar. An SNP (rs833843) in the 5’ promoter of WNT10B correlates with tooth agenesis (Magruder et al., 2018). The T allele at rs833843 was shown to have less transcriptional activation activity in a luciferase reporter assay compared to the C allele (Williams et al., 2021), suggesting that WNT10B levels are critical for tooth formation in humans.
Oligodontia is a severe form of tooth agenesis in which at least six teeth are missing. Four different coding mutations in WNT10B (p.Arg211Gln, p.Pro190Arg, p.Trp262∗, and p.Phe284Cys) have been detected in families with oligodontia (Yu et al., 2016). In vitro expression of the mutant ligands decreased the activation of the TCF-luciferase reporter gene and decreased endothelial differentiation of dental pulp stem cells. The p.Arg211Gln mutation is predicted to decrease the binding affinity of the WNT10B ligand to FZD8, p.Trp262∗ is a truncating mutation, and the other two mutations have unknown consequences (Yu et al., 2016). In a second study, Kantaputra et al. (2018) found that WNT10B mutations are associated with oligodontia, microdontia (small teeth), short tooth roots, dental pulp stones, and taurodontism (elongation of the pulp chamber of the tooth) (Kantaputra et al., 2018). Mutations in other components of WNT signaling (WNT10A, LRP6, and KREMEN1) have also been shown to lead to tooth agenesis (Chu et al., 2021).
7 WNT10B and split-hand/foot malformation
SHFM is a congenital limb malformation characterized by the absence of certain digits, leading to a claw-like hand or foot. Mutations in WNT10B and several other genes have been shown to cause SHFM, with WNT10B mutations associated with SHFM type 6. WNT signaling plays a role in many stages of limb development during embryogenesis, and specifically, WNT10B is expressed in the limb bud at all stages (Wang and Shackleford, 1996). Mutations in WNT10B in patients with SHFM were first identified in a Turkish family in 2008 (c.994C→T, p.R332W) (Ugur and Tolun, 2008). Since then, several other case studies have shown a variety of WNT10B mutations in SHFM individuals (Khan et al., 2012; Ullah et al., 2018; Khan et al., 2019; Al Ghamdi et al., 2020; Bilal et al., 2020; Elalaoui et al., 2021). Many of these result in frameshifts or premature stop codons.
Interestingly, the patients with SHFM do not have tooth abnormalities and have different mutations than those with tooth agenesis (Yu et al., 2016). Individuals with tooth agenesis have mutations in amino acids between 190 and 284, and those with SHFM have mutations between 329 and 388, in addition to non-sense and frame-shift mutations. Furthermore, the knockout of Wnt10b in mice does not lead to an SHFM phenotype. Wnt10b knockout mice are obese and have low bone mineral density, which has not been reported in SHFM patients. Therefore, a mutated WNT10B is functionally different from the absence of WNT10B, or there are species-specific differences.
8 WNT10B and adipocytes
8.1 Function of WNT10B in adipocytes
As previously mentioned in Section 4, WNT10B is often used as a marker of increased osteoblastogenesis or decreased adipogenesis, based on in vitro and in vivo experiments in the previous decade (Wend et al., 2012). Several gain-of-function mouse models showed the effects of WNT10B in adipocytes: overexpression of Wnt10b in adipocytes with the FABP4 (fatty acid-binding protein 4) promoter (expressed in white and brown adipose tissues) inhibited adipogenesis (Longo et al., 2004) and overexpression of Wnt10b with the UCP1 promoter (expressed highly in interscapular tissue) converted brown adipose tissue to white adipose (Kang et al., 2005). Furthermore, the FABP4-Wnt10b mice are resistant to diet-induced obesity and do not gain significant bodyweight on the ob/ob background (Wright et al., 2007). In vitro, WNT10B decreased the adipogenic differentiation of 3T3-L1 preadipocytes while simultaneously increasing osteoblast differentiation, similar to the overexpression of β-catenin. Mechanistically, WNT10B signals through β-catenin to suppress the expression of PPARγ (a master adipogenic transcription factor) and ID2 (which is known to induce PPARγ expression and adipogenesis) (Cawthorn et al., 2012). WNT10A and WNT6 (Cawthorn et al., 2012), along with WNT1 and WNT3A, have similar roles as WNT10B (Liang et al., 2020) in adipocyte and osteoblast differentiation.
Bone marrow adipose tissue (BMAT) is a distinct form of adipose that regulates skeletal homeostasis and energy metabolism (Pachon-Pena and Bredella, 2022). WNT10B is often used as a marker of adipogenesis in bone marrow adipocytes (Georgiou et al., 2015; Lee et al., 2019), as in other adipose depots. Overexpression of Wnt10b in osteoblasts with the OCN promoter (OCN-Wnt10b) led to a decrease in BMAT. Calorie restriction increases the percent of BMAT, which is blunted in the OCN-Wnt10b mice (Cawthorn et al., 2014). WNT10B’s regulation of BMAT requires further investigation, such as determining if WNT10B represses BMAT with the same mechanism as in white adipose.
8.2 Regulation of WNT10B in adipose tissue
Research in the past decade on WNT10B in adipocytes has focused on the transcriptional regulation of WNT10B. Adipocytes use several mechanisms to repress WNT10B during adipogenesis: DNA methylation, WNT signaling inhibitors, and microRNAs.
During adipogenesis, the promoter of WNT10B becomes methylated, and the chromatin is in a closed conformation, preventing gene transcription. The methionine adenosyltransferase MAT2A and the lysine N-methyltransferase EZH2 are recruited to the WNT10B promoter during adipogenesis, leading to histone 3, lysine 27 (H3K27) methylation, and gene suppression (Zhao et al., 2018). WNT10B promoter methylation can be reversed with the inhibitor 5-azacytidine (Fox et al., 2008). In the absence of methylation, CREB regulates WNT10B expression. However, in the presence of methylation, CREB is prevented from binding the promoter, and WNT10B expression is repressed in adipogenesis. In addition to two CREB response elements (CRE), there are three HIF-responsive elements (HRE) in the promoter of WNT10B bound by HIF-2α. Hypoxia increases WNT10B expression and subsequently decreases adipogenesis (Park et al., 2013).
Another factor that decreases WNT10B levels in adipogenesis is microRNA-148a (miR-148a, Table 1). The expression of miR-148a increases in response to XBP1, a pro-adipocyte transcription factor, during adipogenesis, corresponding with a decrease in Wnt10b expression. miR-148a can bind to the 3′-UTR of Wnt10b mRNA and decrease the expression of Wnt10b (Cho et al., 2013; Cho et al., 2016).
Dickkopf (DKK) genes DKK1-4 encode secretory proteins that can antagonize WNT/β-catenin signaling by inhibiting WNT coreceptors LRP5 and LRP6 (Niehrs, 2006). The addition of recombinant DKK1 to human adipose-derived stem cells increased adipogenesis via upregulation of PPARγ and C/EBPα, with a corresponding decrease in WNT10B mRNA and protein. The addition of the GSK3β inhibitor SB 216763 downregulated DKK-1, activated canonical WNT signaling, and increased WNT10B expression (Lu et al., 2016). Thus, the activation of WNT signaling regulates WNT10B expression in a feed-forward loop, but this mechanism remains unclear.
WNT10B expression in adipocytes can also be regulated differentially by SNPs. In 2011, an SNP (rs833840) in the promoter of WNT10B was associated with body fat mass in Korean females (Kim et al., 2011). Subsequently, a genetic association between WNT10B polymorphisms and obesity in Belgian males was shown for three WNT10B SNPs (rs833841, rs4018511, and rs10875902) (Van Camp et al., 2012). The first (rs833841) is in linkage disequilibrium with the SNP found in the Korean study (rs833840). The other two SNPs are in the 3’ non-coding region of WNT10B. The same group investigated two additional populations and did not find the same association with BMI or adiposity. However, they did identify a correlation with bone mineral density (Van Camp et al., 2013). Thus, WNT10B polymorphisms may occur in different populations but appear at low frequencies in the published studies, limiting statistical significance, if any.
In summary, as evidenced by transgenic and knockout mice, in vitro experiments, and human polymorphisms, WNT10B, via canonical WNT signaling, is considered an inhibitor of adipogenesis, and WNT10B expression must be inhibited to allow adipogenesis.
9 WNT10B and muscle
WNT10B is expressed in muscle cells. Myoblasts can differentiate into myocytes or adipocytes, and WNT10B signaling regulates this process. Wnt10b knockout mice have increased adipogenesis in actively regenerating myofibers (Vertino et al., 2005). This balance between myocytes and adipocytes was also demonstrated in the rotator cuff. There is increased adipogenesis in muscle tissue near a torn rotator cuff, and this is a prognostic factor for poor recovery after rotator cuff surgery. The expression of WNT10B is decreased in the muscle, corresponding with increased adipogenesis, after rotator cuff injury in a rabbit model and human patients (Shah et al., 2017; Kuwahara et al., 2019).
Additional evidence that showed the inverse biology between adipose and muscle was presented in a study comparing patients with high insulin sensitivity (IS) to patients with low IS. Adipose tissue from the high-IS group had a higher expression of WNT10B than the low-IS group. In contrast, the skeletal muscle from the high-IS had lower WNT10B expression than the low-IS group (Karczewska-Kupczewska et al., 2016).
Intramuscular fat (IMF) is an important characteristic of beef quality and taste, and castration increases the fat content. The muscle of castrated cows had a decrease in WNT10B expression compared to intact bulls. In castrated cows, there was an inverse correlation between WNT10B (and β-catenin) expression and fat content (Jeong et al., 2013). Therefore, Jeong et al. (2013) suggested that the WNT10B/β-catenin signaling pathway could be used to predict beef quality. Another study showed that WNT10B is higher in the longissimus thoracis muscle (used for ribeye steaks) compared to subcutaneous fat in an age-dependent manner (Soret et al., 2016). WNT10B expression did not vary between four different muscles with different IMF levels (Martinez Del Pino et al., 2017). Therefore, further work on the level of WNT10B and other genes in muscle and the relationship to IMF should be performed to optimize beef quality.
IMF is also a consideration in donkey meat. Guangling donkeys are raised in China for consumption. RNA-sequencing was performed on high- and low-fat Guangling donkey muscles. One of the most important regulated genes, based on co-expression networks, was WNT10B. WNT10B had increased expression in the high-fat group, in contrast to the expected inverse correlation in studies on humans and cows (Li W. et al., 2022) (see Section 8).
Wnt10b in muscle has also been studied in the zebrafish model. Wnt10b signaling inhibits the synthesis of fatty acids in zebrafish myocytes (Liu D. et al., 2019). Knockdown of Wnt10b mRNA led to an increase in triglyceride, total cholesterol, and non-esterified fatty acids in the muscle and corresponding increases in the expression of fatty acid synthetase, acetyl-CoA carboxylase, and ATP-citrate lyase. Wnt10b signaling is activated by vitamin E, an antioxidant, and Wnt10b regulates the activities of antioxidant enzymes (superoxide dismutase, peroxidase, and glutathione peroxidase) in the muscle of zebrafish (Liu et al., 2020b).
10 WNT10B and fibrosis
Fibrosis is the development of fibrous connective tissue as a reparative response to injury or damage. It is characterized by chronic inflammation, excessive accumulation of mesenchymal proteins [e.g., α-smooth muscle actin (α-SMA), vimentin, fibronectin, and fibroblast-specific protein 1 (FSP-1)], and an increase in the extracellular matrix that disrupts the normal tissue function. WNT signaling plays a role in fibrosis (Hu et al., 2020). In 2011, it was reported that the ectopic expression of Wnt10b in skin fibroblasts caused enhanced dermal fibrosis. In addition, in the past decade, further work in systemic sclerosis (SSc) and fibrosis in additional tissues [heart (see Section 12), lungs, liver, and penis] demonstrate a function for WNT10B, specifically in fibrosis.
WNT10B expression correlates with lung fibrosis. The addition of WNT10B increased the expression of mesenchymal markers and epithelial-to-mesenchymal transition. Conversely, the reduction of WNT10B by siRNA decreased mesenchymal markers and pulmonary fibrosis (Yang Z. et al., 2021). Furthermore, a reduction in WNT10B levels by miR-16 and miR-148a (Table 1), found in extracellular vesicles from the bronchial epithelium, decreased myofibroblast differentiation (Kadota et al., 2021).
WNT pathway genes (including Wnt10b) are upregulated in activated hepatic stellate cells leading to liver fibrosis. The mechanism includes repression of PPARγ and liver adipogenesis (Cheng et al., 2008). MSCs reduce liver fibrosis, and the treatment of liver fibrosis in rats with exosomes from MSCs decreased Wnt10b expression (Rong et al., 2019). A WNT antagonist also reduced liver fibrosis. In addition to WNT10B, other WNTs, including WNT1, WNT3/3A, WNT4A, and WNT5A, are implicated in liver fibrosis (Cheng et al., 2008).
Fibrosis is also present in penile cavernous tissue from diabetic mice and correlates with erectile dysfunction. This tissue has an increase in Wnt10b expression, along with an increase in the expression of four other WNT ligands. TGFβ also increased the expression of Wnt10b (Shin et al., 2014). Because the downregulation of Wnt10b with small interfering RNA did not decrease the production of extracellular matrix proteins in the fibroblasts, additional research on the role of WNT10B in this system should be performed.
11 WNT10B in skin and hair
The skin is the largest organ in the body and consists of appendages that include hair follicles, sebaceous glands, sweat glands, and nails. Many WNT ligands are expressed in the skin and the hair follicle (Wend et al., 2012). Importantly, the earliest and highest expressed WNT ligand in hair follicle development and hair cycle induction is Wnt10b (Reddy et al., 2001). Hair follicles have periodic stages: the anagen (growth stage), catagen (regression), and telogen (resting) stages. Wnt10b is only expressed in the anagen stage (Li Y. H. et al., 2013; Bai et al., 2021). Specifically, Wnt10b is restricted to follicular epithelial cells overlying the dermal condensate (Reddy et al., 2001; Hawkshaw et al., 2020). Overexpression of Wnt10b induces the telogen hair follicle to proceed into anagen earlier (Li Y. H. et al., 2013). Adenoviral Wnt10b increased the number of proliferating cells and, subsequently, the size of the anagen hair follicle (Lei et al., 2014). Increased proliferating CD34+ stem cells were also observed in the bulge region and the outer root sheath of the hair follicle.
Dermal papilla cells are specialized fibroblasts located in hair follicles that work as a signaling center for hair growth. WNT10B promotes the proliferation of cultured dermal papilla cells from mice, Angora rabbits, and Rex rabbits in vitro (Ouji et al., 2012; Bai et al., 2019; Wu et al., 2020). Cultured dermal papilla cells with WNT10B had the greatest hair follicle induction in skin reconstitution assays (Ouji et al., 2013). Together these papers demonstrate that WNT10B is produced by the epithelial cells, acting upon the fibroblasts in a paracrine manner. AXIN2, the canonical WNT target gene, while expressed in the epithelial cells, is highest in the mesenchymal cells in the early anagen (Hawkshaw et al., 2020).
WNT10B also plays a role in the differentiation of melanocytes (melanin-producing cells) of the hair bulbs during the anagen stage. Intradermal injections of adenoviral Wnt10b in a mouse led to more melanocytes with more pigmentation. Similar results were obtained when whisker follicles were treated with adenoviral Wnt10b. Adenoviral Wnt10b also increased the differentiation of a melanocyte cell line in culture (Ye et al., 2013).
WNT10B is potentially involved in the development of apocrine sweat glands. Apocrine sweat glands are found in hairy regions such as the axilla and secrete nutrients to the opening of hair follicles. While mice and chickens do not have these, sheepskin sweat glands show some similarities to human glands. Examining the complex network of development and maturation of apocrine sweat glands has shown that WNT10B has decreased expression in the gland budding stage, compared to the pre-gland stage, as determined by RNA-sequencing. In contrast, WNT16, WNT5A, and β-catenin are upregulated in the gland budding stage (Li S. et al., 2018). Functional studies were not performed.
The role of WNT10B in the skin is further elucidated by examining skin pathologies, such as SSc and psoriasis. SSc is a chronic connective tissue disorder causing fibrosis of the skin and other organs due to overactive fibroblasts. Fibroblasts of those with SSc contain higher levels of nuclear β-catenin, which correlates with increased expression of Wnt1 and Wnt10b (Beyer et al., 2012). Transgenic mice in which Wnt10b is expressed from the fatty acid binding protein 4 (FABP4) promoter have increased skin thickness and are used to study SSc and anti-fibrotic therapies (Wei et al., 2011). For example, inhibition of the X-linked inhibitor of apoptosis protein (XIAP) reduced the skin thickness of Wnt10b transgenic mice (Bergmann and Distler, 2016).
Psoriasis is a chronic inflammatory skin condition causing patches of itchy, flaky skin with scales (lesions). Samples from the lesional skin of psoriasis patients show significantly decreased WNT10B gene expression compared to the non-lesional skin of patients and skin from healthy individuals. WNT7B showed a similar gene expression pattern, and TCF7L2, a transcription factor downstream of WNT signaling, decreased in the lesional skin compared to non-lesional skin. The gene expression of WNT10B and WNT7B increases after treatment with narrowband UV, which is a therapy for psoriasis. IL-17 acts on keratinocytes and releases inflammatory mediators that lead to psoriasis symptoms. IL-17A inhibits WNT signaling in bone (osteopenia and osteoporosis have been associated with psoriasis). Although this has to be shown in the skin yet, psoriasis patients have increased IL-17, which could explain the low WNT expression in lesional skin. Current psoriasis therapy incudes IL-17 inhibitors, which not only reduce IL-17 signaling but also may help increase downstream WNT signaling to reduce inflammation (Assarsson et al., 2019).
Agents to increase hair growth could be used to treat various conditions, such as alopecia. Increasing the expression of WNT10B either directly or indirectly could induce hair growth. Several hormones and growth factors have been shown to regulate WNT10B, including androgens, melatonin, hepatocyte growth factor (HGF), vitamin D, and bone morphogenic protein 6 (BMP6).
Androgenetic alopecia is the most common type of hair loss in men. The androgen DHT decreases WNT10B expression in dermal papilla cells while increasing DKK1 expression. DHT decreases hair follicle stem cell differentiation in vitro, and the addition of rWNT10B reverses this effect (Leiros et al., 2017).
HGF from dermal white adipose tissue induces WNT10B expression in dermal papilla cells (Nicu et al., 2021). The topical application of small-molecule antagonists to c-Met (the receptor for HGF) for excessive hair growth has been suggested. This is clinically possible as c-Met antagonists have been approved for cancer.
Aoi et al. (2012) showed that vitamin D3 increased WNT10B expression in dermal papilla cells; however, vitamin D decreased their proliferation, and WNT10B has been previously shown to increase their proliferation. These conflicting results need to be clarified. All-trans retinoic acid upregulated WNT10B expression in a screen of 20 growth factors (Aoi et al., 2012).
Furthermore, BMP6 regulates the hair cycle and is expressed at the highest level in early anagen. BMP6 was shown to repress WNT10B expression and decrease the proliferation of hair follicle stem cells, and conversely, WNT10B repressed BMP6 expression and increased the number of proliferating hair follicle stem cells (Wu P. et al., 2019).
The promoter of WNT10B is DNA methylated in the catagen and telogen stages, whereas there is a decrease in methylation at the anagen stage, correlating with WNT10B expression. Furthermore, there is an increase in histone H3 acetylation, a marker of open chromatin, only during the anagen stage (Bai et al., 2021). The transcription factors that regulate the expression of WNT10B at the WNT10B promoter in hair follicles remain unknown.
In dermal papilla stem cells, WNT10B mRNA expression is downregulated by miR-329 (Table 1). The long non-coding RNA PCAT1 sponges miR-329 to allow the expression of WNT10B. The upregulation of PCAT1 or a decrease in miR-329 corresponded with enhanced WNT10B expression and increased the proliferation of the dermal papilla stem cells (Nicu et al., 2021).
WNT10B is also regulated by miR-15b-5p in dermal papilla cells, which in turn is sponged by lncRNA-599547 (Table 1). This long non-coding RNA was shown to be expressed in the secondary hair follicles of cashmere goats at anagen, thus allowing for the expression of WNT10B at anagen (Leiros et al., 2017).
WNT10B expression can be increased by melatonin, a hormone induced in the brain in response to light and dark. Seasonally, there are changes in the length of the day and, subsequently, the amount of melatonin released. Interestingly, the administration of melatonin can increase WNT10B mRNA and protein levels and improve the cashmere yields from cashmere goats, which produce it seasonally (Liu et al., 2021). While melatonin increased the expression of WNT10B, it remains unclear if this is a direct or indirect effect.
12 WNT10B and the heart
WNT10B expression in the heart was detected along with the cloning of the human gene in 1997. In fact, the tissues with the highest expression levels of WNT10B included the heart (Hardiman et al., 1997). Only in the past decade has there been functional data about the role of WNT10B in the heart, along with an association with fibrosis and disease.
WNT10B is expressed in the intercalated discs of normal cardiomyocytes, and in ischemic cardiomyopathy patients, WNT10B accumulates along the lateral borders of cardiomyocytes. Furthermore, WNT10B protein is increased in cardiomyocytes after experimental myocardial infarction in mice. Overexpression of Wnt10b in cardiomyocytes led to improved cardiac function. After injury, Wnt10b overexpression led to increased neovascularization and endothelial cell recruitment. WNT10B in the cardiomyocytes induced canonical WNT signaling (β-catenin accumulation in the nucleus and AXIN2 expression) in the endothelial cells but not in the cardiomyocytes, demonstrating paracrine signaling. The authors show that Wnt10b overexpression led to decreased fibrosis (decreased scar formation and decreased collagen deposition after cardiac repair) (Paik et al., 2015). This contrasts with other studies (described here) on fibrosis in the heart and the other tissues presented in Section 10.
Kamimura et al. (2016) demonstrated that WNT10B expression levels in the left ventricle increased in heart failure, which was correlated with fibrosis. Pioglitazone, a PPARγ agonist used for type 2 diabetes mellitus, has cardiac antifibrotic effects in animals and human patients. Pioglitazone inhibited WNT signaling and prevented the development of left ventricle fibrosis (Kamimura et al., 2016). WNT10B has been shown to suppress PPARγ in adipose tissue (see Section 8).
Fibrosis is also observed in autoimmune myocarditis. In a mouse model of autoimmune myocarditis, the angiotensin II/angiotensin receptor (Agtr1a) pathway is activated. Wnt10b and Wnt1 are lower in Agtr1a knockout cells with lower fibrosis. The authors state that reduced WNT signaling explains the reduced profibrotic response of Agtr1a−/− cells (Czepiel et al., 2022).
Adipose tissue is also found in the heart, and its accumulation contributes to atrial fibrillation. Multipotent mesenchymal epicardium-derived cells, which can differentiate into adipocytes, express WNT10B. WNT10B expression is associated with repressed adipogenesis in these cells, as is seen in other MSCs (Suffee et al., 2017).
13 WNT10B and the nervous system
Only one study has shown a role for WNT10B in the nervous system (Tassew et al., 2017). In that work, WNT10B was shown to promote axonal regeneration. WNTs have previously been shown to be in exosomes. Tassew et al. (2017) showed that while fibroblast-derived exosomes are needed for axonal regeneration, WNT10B is not in the exosomes. Instead, WNT10B requires the exosomes for activity. What was present in the exosomes and affected WNT10B activity was not described. However, after the addition of exosomes, WNT10B re-localized to lipid rafts for endocytosis. Exosome-WNT10B induction of neurite outgrowth did not affect β-catenin. Instead, it led to mTOR activation and phosphorylation of S6 ribosomal protein (pS6K), indicating WNT signaling through the non-canonical pathway in neurons (Tassew et al., 2017).
14 WNT10B and endothelial cells/angiogenesis
WNT signaling is involved in angiogenesis (Olsen et al., 2017). In particular, a role for WNT10B in angiogenesis and endothelial cells has become apparent. Vascular endothelial growth factor (VEGF) is a key angiogenic growth factor. WNT10B regulates VEGFA in umbilical cord mesenchymal stem cells (Liu et al., 2020g) and vascular endothelial growth factor receptor 2 (VEGFR2) in endothelial cells ((Paik et al., 2015). Furthermore, the expression of WNT10B and VEGFA genes correlate in the subcutaneous white adipose tissue of 80 individuals, and hypoxia increases WNT10B and VEGFA in adipocytes (Pourdashti et al., 2022).
The vasculature plays a critical role in bone physiology and fracture healing (Owen-Woods and Kusumbe, 2022). Human umbilical cord mesenchymal stem cells transfected with WNT10B accelerated bone healing and induced endothelial cells to migrate and form capillary-like structures in a tube formation assay (Hu et al., 2022).
Treatment of corneal endothelial cells with IL-1β resulted in the activation of WNT10B expression and cellular proliferation. NFκB and c-Jun bound to the proximal promoter of WNT10B after IL-1β induction. Then, WNT10B stimulated proliferation by upregulating cyclin D1 expression. Co-treatment with a secreted frizzled-related peptide (sFRP), the β-catenin antagonist XAV939, or a disheveled-PDZ domain inhibitor blocked WNT10B signaling and proliferation. The modulation of WNT10B could treat vision loss secondary to corneal endothelial dysfunction (Lee and Heur, 2015).
15 WNT10B and the ovary
The biology of WNT10B in the ovary beyond gene expression has not been determined. Single-cell RNA-sequencing was performed on normal mouse ovaries and ovaries from mice exposed to cigarette smoke, as cigarette smoke affects fertility. Nine different cell types were identified, including oocytes. WNT10B was specifically expressed only in the granulosa cells, and WNT signaling pathway genes were enriched. Cigarette smoke increased the expression of WNT10B mRNA (Li F. et al., 2022). Others have identified WNT10B expression in the full-grown oocyte, ovulated oocyte, zygote, and two-cell stage embryo (Harwood et al., 2008). WNT10B expression was downregulated in a mouse model of premature ovulatory failure (Ruohonen et al., 2022). Further studies should be carried out to assess the functional implications of WNT10B expression in the ovaries.
16 WNT10B and placental development
Dysfunctional placental development is associated with several pregnancy complications, including fetal growth restriction, which impacts 3%–7% of pregnancies in the United States. Several key steps are required for the formation of a functional placenta, including implantation in the maternal endometrium, formation of a syncytium for the exchange of food and waste, generation of villi, and remodeling of the maternal spiral arteries. These steps are accomplished by different subtypes of mature, differentiated trophoblasts, such as syncytiotrophoblasts (STBs), proliferating villous cytotrophoblasts (vCTBs), and invasive extravillous cytotrophoblasts (EVTs). Early work from Sonderegger et al. (2007), using differential gene expression analyses of WNT expression, showed that WNT10B expression is the highest in first-trimester trophoblasts but not detected in third-trimester samples. Interestingly, examination of human trophoblast cell lines showed the highest WNT10B expression in the EVT-like SGHPL-5 cells and in JEG-3 cells derived from choriocarcinoma (Sonderegger et al., 2007). These findings are supported by more recent studies from Takahashi et al. (2021), who found high WNT10B expression in EVT-like cell lines and primary EVT (Takahashi et al., 2021).
Recent work has identified functional roles for WNT10B in the CTB, STB, and EVT. Treatment of EVT cell lines with WNT10B induced CD44 and MMP9 expression and invasiveness, whereas knockdown of WNT10B decreased CD44 expression and invasiveness. In primary EVT, WNT10B treatment also increased invasiveness (Takahashi et al., 2021). This work is consistent with Wang et al. (2019), showing that the transcription factor glial cells missing 1 (GCM1) binds the WNT10B promoter and induces WNT10B expression. Secreted WNT10B binds FZD7, inducing EVT migration (Wang et al., 2019). These data suggest an important role for WNT10B in promoting the invasiveness of EVT. Takahashi et al. (2021) claimed activation of the canonical pathway by WNT10B. In contrast, Wang et al. (2019) claimed non-canonical signaling induced by WNT10B, showing no effect of WNT10B on the expression of AXIN2 and the failure of WNT10B to affect the expression from a β-catenin/TCF luciferase reporter (Wang et al., 2019). Furthermore, they showed the activation of RAC1 by WNT10B stimulation of trophoblast cell lines. In support of these studies, β-catenin transcript expression is reduced in first-trimester EVT compared to CTB (DaSilva-Arnold et al., 2015). Canonical versus non-canonical pathway induction by WNT10B in the regulation of EVT remains an open question.
In addition to playing an important role in the invasiveness of EVT, WNT10B regulates the cell fusion of STBs to form a syncytium. STBs are formed through the process of cell fusion of cytotrophoblasts. Forskolin treatment of BeWo trophoblast cells increased cAMP production, PKA phosphorylation, and CREB phosphorylation, which induced cell fusion to form the syncytium. Forskolin also selectively induced WNT10B expression, as other WNTs were unaffected (Malhotra et al., 2015). Forskolin induced WNT10B expression in JEG3 cells, a different trophoblast cell line (Wang et al., 2019). Interestingly, forskolin induced total β-catenin expression, suggesting a canonical role for WNT10B in cell fusion (Malhotra et al., 2017).
The hormone human chorionic gonadotrophin (hCG) is critical for the maintenance of pregnancy. hCG binds to the G protein-coupled receptor LHCGR to induce cAMP production. Treatment of BeWo trophoblasts with hCG induced the expression of WNT10B and cell fusion. Knockdown of WNT10B in BeWo trophoblasts reduced cell fusion, formation of active β-catenin, and the expression of syncytin-1, a key cell fusion protein (Malhotra et al., 2017). These data suggest that hCG induction of WNT10B promotes the fusion of the epithelial cytotrophoblast cells to form the syncytium for the exchange of food and oxygen. Therefore, WNT10B plays key roles in human differentiated trophoblasts that form the functional placenta. Interestingly, the role for WNT10B in murine placental development and function has not been examined. Based on studies in the human placenta, we predict that Wnt10bKO mice may be an important tool for understanding the role of WNT10B in the placenta.
17 WNT10B and cancer
17.1 WNT10B and breast cancer
WNT10B was originally discovered as an oncogene in the mammary gland (Lee et al., 1995). Our prior review summarized the biology of WNT10B in mammary stem cells and mouse models of breast cancer (Wend et al., 2012). The last decade of research in breast cancer has produced solid evidence for the oncogenic effects of WNT10B in human triple-negative breast cancer (TNBC). WNT10B protein expression was more highly expressed in TNBC (80%) compared to other subtypes of breast cancer (10% in ER+/Her2+/PR+) and significantly predicted worse survival outcomes, tumor size, and grade, suggesting, for the first time, that WNT10B expression is clinically relevant in breast cancer. In contrast, WNT1 expression did not predict survival (Wend et al., 2013). WNT10B overexpression in MDA-MB-231 breast cancer stem cells increased tumor size in vivo, and shWNT10B decreased the number of mammospheres and colony-forming units compared to controls, further confirming a role for WNT10B in cancer stem cells (Li X. et al., 2022).
MMTV-Wnt10b mice generate adenocarcinomas and are a good model for human TNBC (Lane and Leder, 1997; Miranda-Carboni et al., 2008). The most highly upregulated mRNA (by gene expression microarrays) in the MMTV-Wnt10b mice, compared to wild-type mice, is high mobility group A family member 2 (Hmga2). In contrast, MMTV-ErbB2 (HER2+) tumors do not express HMGA2. During mouse development, the mammary placode is identifiable at embryonic day E11.5, and by E14.5 (early mammogenesis), HMGA2 protein expression was detected in the mammary anlagen bud, epidermis, and mesenchymal tissue. In contrast, Wnt10bKO mice did not express HMGA2. WNT10B signaling increases the occupancy of β-catenin at the HMGA2 promoter, as detected by ChIP analysis, and treating the cells with the WNT inhibitor ICG-001, which blocks CBP-mediated acetylation on β-catenin at Lysine 49 (K49), coincides with the loss of HMGA2 expression in both mouse and human cells. Therefore, HMGA2 is a direct target of WNT10B/β-catenin signaling. HMGA2 expression also predicts recurrence-free survival, proliferation, tumor size, nuclear grade, and metastasis in TNBC patients (Wend et al., 2013).
In a follow-up manuscript, El Ayachi et al. (2019) further defined the WNT10B network, composed of β-catenin, HMGA2, and EZH2 signaling. Together, the network predicted a reduction in relapse-free survival and metastasis in chemoresistant TNBC. The expression of HMGA2 and EZH2 was concurrent in MMTV-Wnt10b-driven tumors during metastasis. To link this genetically, HMGA2 KO mice were backcrossed to the MMTV-Wnt10b tumor line and showed loss of EZH2 expression. Mechanistically, HMGA2-EZH2 protein–protein interactions were necessary to maintain lysine 49 (K49) acetylation (K49Ac, activation) of β-catenin and for the displacement of Groucho/TLE1 with TCF-4. TLE1 represses WNT-direct gene target expression by recruiting HDAC to WNT-directed gene expression. CRISPR knockout of both HMGA2 and EZH2 in the TNBC cell line MDA-MB-231 blocked primary tumor growth and lung metastasis and restored E-Cadherin in vivo. The loss of HMGA2 and EZH2 was necessary to restore a normal mammary epithelial phenotype. Moreover, the protein–protein interactions between HMGA2-EZH2 and EZH2 interaction with β-catenin, TCF4, and LEF1 were preserved in a patient-derived xenograft (PDX) TNBC sample, similar to what was observed in MMTV-Wnt10b tumors and the MDA-MB-231 model. HMGA2 was only shown to physically interact with EZH2 but not with WNT-nucleosome proteins (β-catenin, TCF4, and LEF1) (El Ayachi et al., 2019).
The WNT/β-catenin inhibitor ICG-001, or a derivative thereof such as PRI-724, could be used to treat WNT10B-driven tumors. ICG-001 prevented tumor growth and lung metastasis in a WNT10B-expressing TNBC PDX and MDA-MB-231 orthotopic models (El Ayachi et al., 2019; Fatima et al., 2019). Results from in vitro work in both mesenchymal-like (ML) TNBC cell lines (MDA-MB-231 and MDA-MB-157) and basal-subtypes (HCC-38 and MDA-MD-468) had similar IC50s (half maximal inhibitory concentration) when exposed to the WNT inhibitor ICG-001. The WNT10B direct target genes HMGA2, C-MYC, AXIN2, and CCND1 were downregulated after ICG-001 therapy. The authors also compared responses to ICG-001 in TNBC PDX cell line models from a naïve chemoresistant PDX (no neoadjuvant therapies) to that of the highly doxorubicin/taxol-chemoresistant PDX. The naïve cell lines were the most sensitive to ICG-001 exposure. In contrast, highly chemoresistant cHCI-10 was the most resistant of all the TNBC cell lines tested. ICG-001 was effective in combination with the conventional cytotoxic chemotherapeutics, cisplatin, and doxorubicin in decreasing the proliferation of MDA-MB-231 cells. WNT10B inhibition combined with FDA-approved therapies could have broad clinical importance.
WNT signaling is a hallmark of TNBC and is associated with specific metastatic pathways (Dey et al., 2013). TNBC can have simultaneous visceral metastases and be associated with poor prognosis if accompanied by bone metastasis (Luo et al., 2017). Fatima et al. (2019) interrogated the WNT10B, β-catenin, HMGA2, and EZH2 signaling axis in highly chemoresistant tumors that metastasize simultaneously to multiple organs, such as the liver, bone, and brain. ICG-001 blocked simultaneous visceral metastasis to bone, liver, ovary, kidneys, and brain in the MDA-MB-231 and HCI-10 TNBC PDX models. Mechanistically, the loss of metastasis was linked to the loss of SNAIL and VIMENTIN protein expression. Furthermore, the combination of ICG-001 and doxorubicin blocked simultaneous multi-organ and bone metastases in the chemoresistant PDX model. These results demonstrate that the addition of a WNT inhibitor to anthracycline therapy can inhibit simultaneous multi-organ metastases (Fatima et al., 2019).
Natural products have provided a direct source of therapeutic agents and have served as the basis for drug development for the past 60 years (Newman and Cragg, 2007). A natural products library of 2,300 compounds was screened for their anti-proliferation and anti-WNT signaling effects on MDA-MB-231 cells (amongst others), and several interesting candidates were identified (Ling et al., 2015; Fatima et al., 2017). The diterpene Jatrophone, derived from the plant Jatropha isabelli, interfered with the oncogenic WNT10B/β-catenin/HMGA2 signaling axis and inhibited proliferation in TNBC MSL-subtypes (MDA-MB-231 and MDA-MB-157), BSL-1 subtypes (HCC-38 and MDA-MB-468), and TNBC PDX-derived cells. Mechanistically, JATROPHONE blocked the expression of a WNT reporter (8XTOPFLASH) at the level between receptor complex (LRP6) and β-catenin activation. Jatrophone repressed WNT-direct targets AXIN2, HMGA2, C-MYC, PCNA, and CCND1. Interestingly, elevated WNT10B expression also coincided with increased resistance to jatrophone exposure in several metastatic cell lines (Fatima et al., 2017). The findings suggested that Jatrophone is poised to further develop as a lead molecule to combat highly chemoresistant metastatic TNBC.
Chen et al. (2022) showed that high expression of the histone lysine methyltransferase NSD1 (nuclear receptor binding SET domain-containing protein 1) could predict the overall survival of breast cancer patients, although the subtype of breast cancer that was analyzed was not discussed. Then, they investigated the role of NSD1 in paclitaxel-mediated drug resistance using parental MCF-7 (ER + breast cancer) versus MCF-7 PR (paclitaxel-resistant) cell lines. The MCF-7PR subline had significantly increased NSD1 protein levels. Overexpression of NSD1 in the parental MCF-7 cell line moderately increased proliferation and inhibited apoptosis. The converse was observed when an siNSD1 was used in the MCF-7PR subline. The authors identified a positive correlation between WNT10B and NSD1 in breast cancer tissue. siNSD1 decreased the expression of WNT10B protein with concurrent upregulation of H3K27me3 (a mark of transcriptionally inactive chromatin) at the WNT10B promoter. The MCF-7 parental line transduced with siNSD1 had decreased tumor growth in vivo relative to the control. Protein analysis of the tumors demonstrated loss of NSD1, WNT10B, β-catenin, CCND1, and C-MYC. Further studies will be required in the future to validate these findings in models of TNBC (Chen et al., 2022). WNT10B is also regulated by NSD1 in hepatocellular carcinoma (see Section 17.14).
Li et al. (2022) interrogated the effects of sinomenine hydrochloride (SH), which is extracted from a Chinese medicinal plant Sinomenium acutum, on breast cancer stem cells (BCSC) (Li X. et al., 2022). SH is known to have anti-inflammatory properties. Exposure of both MCF-7 (ER + breast cancer) and MDA-MB-231 (TNBC mesenchymal stem-like) cell lines to SH led to decreased ratios of CD44+/CD24- BCSC markers. SH exposure also decreased the self-renewal capacity of mammospheres, along with migration and invasion in both cell lines. SH decreased the expression of stemness-associated genes (HMGA2, MYC, and MET, amongst others). Other groups have shown that WNT signaling regulates both MET and MYC expression, and we have shown that both MYC and HMGA2 expression are direct downstream gene targets of WNT10B/β-catenin signaling (Miranda-Carboni et al., 2008; Wend et al., 2013). There is a loss of β-catenin after SH exposure, with a concurrent loss of WNT10B mRNA and protein in both MDA-MB-231 and MCF-7 cells. WNT10B overexpression reversed the inhibitory effects of SH in mammospheres and colony-forming assays in MCF-7 and MDA-MB-231. SH therapies in vivo decreased the tumor growth of BCSCs from MDA-MB-231 cells. The loss of tumor growth coincided with the loss of WNT10B, β-catenin, and canonical WNT target genes (MYC, JUN, CD44, and MET) in BCSCs. Therefore, SH inhibits TNBC in vivo by disrupting WNT10B signaling in BCSCs (Li X. et al., 2022).
Cancer-associated fibroblasts (CAFs) within the stroma of the tumor microenvironment provide epithelial cells with critical regulation of proliferation, invasion, and metastasis and can elicit an anti-immune tumor response. p85α acts as a tumor suppressor, but its functional role in the tumor microenvironment in breast cancer was limited until Chen et al. (2017) described low protein expression of p85α (PIK3R1) in the stroma of stage II and stage III breast cancer. The conditioned media from mouse embryonic fibroblasts with a deletion of p85α increased the proliferation of both 4T1 and MDA-MB-231 breast cancer cells. The fibroblasts also increased the size of tumors and liver metastasis of the breast cancer cell lines. Subsequent analysis showed an increase in EMT markers and a decrease in phosphorylated β-catenin at Ser33/Ser37/Thr41 in 4T1 and MDA-MB-231 cells cultured with the conditioned media from the knockout fibroblasts. The absence of the phosphorylation at Ser33/Ser37/Thr41 indicates engagement of a WNT-canonical ligand (e.g., WNT1 and/or WNT10B)-mediated event. The downstream loss of phosphorylation on β-catenin activates nuclear translocation to activate WNT direct gene targets. WNT10B ligand expression was the highest WNT expressed, >90-fold relative to others, in the absence of p85α in fibroblasts. Supporting the notion that WNT10B was responsible for the stabilization and activation of β-catenin and the increased aggressiveness of both 4T1 and MDA-MB-231 cells, silencing of WNT10B by short hairpin RNA in p85α−/− CAFs reversed invasion and tumor growth. Furthermore, an inverse correlation of WNT10B mRNA expression and p85α/PIK3R1 was shown in the breast stroma. WNT10B was shown in the exosomes from the p85α knockout fibroblasts. In conclusion, WNT10B expression from CAFs in a paracrine manner elicits breast cancer progression (Chen et al., 2017).
17.2 WNT10B and pancreatic cancer
At the time of our previous review (Wend et al., 2012), the only mention of WNT10B in pancreatic cancer was at the expression level. Since then, Peng et al. (2017) showed a mechanism of miR-148a in pancreatic cancer progression, signaling through WNT10B. They found that miR-148a (Table 1) is downregulated in pancreatic cancer and that this downregulation correlates with worse survival outcomes and greater rates of lymphatic metastasis (Peng et al., 2017). Furthermore, they reveal that WNT10B negatively correlates with miR-148a in these patients. They also show that miR-148a is predicted to bind to the WNT10B 3’-UTR, and a miR-148a mimic inhibits WNT10B expression. Furthermore, transfecting cells with either a miR-148a mimic or si-WNT10B is sufficient to reduce the invasion and migration of a pancreatic adenocarcinoma cell line (BxPC3) (Peng et al., 2017).
17.3 WNT10B and thyroid cancer
miR-148a inhibits WNT10B not only in pancreatic cancer (Peng et al., 2017), but also in thyroid cancer (Table 1) (Li C. et al., 2021). HOTAIRM1 (HOXA Transcript Antisense RNA Myeloid-Specific 1) is upregulated in patients with thyroid cancer and positively correlates with patient TNM stage and lymph node metastasis. shRNA (small hairpin RNAs) knockdown of HOTAIRM1 markedly increased expression of miR-148a and decreased expression of WNT10B in thyroid cancer cell lines (TPC-1 and BCPAP). HOTAIRM1 knockdown significantly reduced cell proliferation and WNT10B protein expression in TPC-1 and BCPAP cell lines, and treatment with an inhibitor of miR-148a rescued these phenotypes. Using the TargetScan database and luciferase assays, they demonstrated that miR-148a directly binds to and suppresses WNT10B at the 3′-UTR in thyroid cancer cells. Overall, HOTAIRM1 inhibits miR-148a, which allows for aberrant expression of WNT10B in thyroid cancer (Li C. et al., 2021).
17.4 WNT10B and oral squamous cell carcinoma
miR-148a also inhibits WNT10B in oral squamous cell carcinoma (OSCC) (Table 1). Cancer-associated fibroblasts (CAFs) isolated from OSCC tumors have significantly decreased expression of miR-148a and significant overexpression of WNT10B by qPCR compared to normal fibroblasts. Furthermore, WNT10B protein expression is decreased in CAFs transfected with stable miR-148a. Overexpression of miR-148a in CAFs inhibited migration and invasion in an OSCC cell line (SCC-25) by inhibiting WNT10B signaling (Min et al., 2016).
Dai et al. (2019) found that the Homeobox protein Hox-C10 (HOXC10) regulates the expression of WNT10B in OSCC. HOXC10 is overexpressed in OSCC patients by immunohistochemistry and RT-qPCR and correlates with worse overall survival in patients. Furthermore, HOXC10 knockdown reduced cell migration and invasion in OSCC cell lines (FaDu and SCC4). By using RNA-sequencing, WNT signaling pathway components were identified as targets of HOXC10 signaling. Then, by qPCR, shRNA knockdown of HOXC10 significantly reduced the expression of WNT10B and its downstream signaling components DVL2 and LRP5/6 in both FaDu and SCC4 cell lines. By immunoblot, they revealed that upon HOXC10 knockdown, the expression of WNT10B, N-cadherin, and vimentin was downregulated, whereas the E-cadherin expression increased. In vivo, loss of HOXC10 decreased tumor size in cell line xenograft models. Furthermore, by immunohistochemistry, HOXC10-knockdown tumors had less WNT10B, DVL2, N-cadherin, and Vimentin expression and greater expression of E-cadherin. This study proposes a mechanism by which HOXC10 promotes WNT10B signaling to enhance proliferation, migration, invasion, and epithelial-to-mesenchymal transition in OSCC (Dai et al., 2019).
17.5 WNT10B and lung cancer
Furthermore, Yang et al. (2021) reported that WNT10B was regulated by non-coding RNAs. Their study identified an overexpression of the circular RNA circTUBGPC3 in lung adenocarcinoma (LAC) patients (Table 1), and high expression of circTUBGPC3 correlated with worse overall survival. As demonstrated by RNA immunoprecipitation, RT-qPCR, and FISH, circTUBGPC3 binds to and inhibits miR-885-3p in SPC-A1 and A549 LAC cell lines. Using the TargetScan database, WNT10B was identified as a binding target of miR-885-3p at the 3’-UTR region. The direct binding of miR-885-3p to WNT10B was confirmed using luciferase assays with inhibitors and mimics of miR-885-3p. WNT10B was also overexpressed in paired LAC and normal tissue and correlated with worse overall survival, lymph node metastasis, and gender. Furthermore, the knockdown of WNT10B reduced colony formation, whereas overexpression restored colony formation. Knocking down circTUBGPC3 inhibited WNT10B expression while inhibiting miR-885-3p upregulated WNT10B in NCI-H460 cells. Overall, this study concluded that circTUBGPC3 promotes lung cancer progression by inhibiting miR-885-3p, thus allowing for the overexpression of WNT10B (Yang Y. et al., 2021). Only one paper has investigated WNT10B in the progression of lung cancer, and further work should be carried out to elucidate the actions of WNT10B in normal lung cells, lung fibrosis, and lung cancer.
17.6 WNT10B and osteosarcoma
Not only does WNT10B regulate normal bone, but WNT10B expression correlates with worse overall survival in osteosarcoma patients (Chen et al., 2008). Research to determine the mechanism by which WNT10B could be affecting osteosarcoma is limited. However, WNT10B has been further implicated in osteosarcoma tumorigenesis (Brun et al., 2013) and metastasis (Muff et al., 2015). In osteosarcoma, WNT10B expression is regulated by the transcriptional co-factor FHL2 (four and a half LIM domains protein 2). By immunohistochemistry, FHL2 is expressed in osteosarcoma samples with increased expression in metastatic and recurrent tumors compared to normal bone. Furthermore, short hairpin knockdown of Fhl2 in murine K7M2 osteosarcoma cells reduced β-catenin nuclear translocation and decreased the expression of Axin2 and Wisp-1. shFhl2 also decreased the expression of Wnt10b and Wnt5a. In vivo, shFHL2 reduced tumorigenesis and metastasis in mice, and shFhl2 tumors had significantly less mRNA expression of Wnt10b and Wnt5a than control tumors (Brun et al., 2013).
Using microarray and array genomic hybridization, Muff et al. (2015) examined changes in gene expression between three metastatic osteosarcoma cell lines (LM5, 143B, and LM8) compared to their respective parent lines (SAOS, HOS, and Dunn). In all three metastatic lines, there was a significant upregulation of WNT10B, suggesting the role of WNT10B in osteosarcoma metastasis (Muff et al., 2015).
17.7 WNT10B and leukemia
Several studies have been conducted on the role of WNT10B in AML. The first paper showed that AC133+ (a glycosylation-dependent epitope of CD133 that marks stem and progenitor cells) AML cells overexpressed and released WNT10B into the microenvironment of AML patients. However, the WNT10B overexpression was not detected in normal AC133+ cells (Beghini et al., 2012). The same lab then used a zebrafish embryo model of AML. They found that transiently overexpressed wnt10b resulted in an expansion of the erythromyeloid, myeloid and erythroid progenitor cell populations, while decreasing the population of circulating neutrophils. This expansion of hematopoietic precursors at the expense of differentiated cell types, such as neutrophils, resembles pre-leukemic HSC expansion suggesting a function for wnt10b in leukemia generation. Subsequently, using a cohort of healthy donors, favorable AML responders, and intermediate/unfavorable responders, WNT10B was shown to be expressed in only AML patients and not in healthy donors (Lazzaroni et al., 2016). They evaluated receptors of WNT10B signaling and found that in AML, WNT10B signals through FZD4 and FZD5, whereas in T-ALL, WNT10B signals through FZD6 (Lazzaroni et al., 2016; Cassaro et al., 2021).
17.8 WNT10B and skin cancer
WNT10B has been implicated as a driver of normal skin development [see Section 11 and Wend et al. (2012)]. WNT10B expression is upregulated in skin squamous cell carcinomas (Bhatia and Spiegelman, 2005) and older recurrent melanoma patients with tumor-positive sentinel lymph nodes (Menefee et al., 2020). In addition, Wnt10b is upregulated in mouse tumor models of melanoma (Lu H. J. et al., 2018).
Adenoviral expression of Wnt10b in normal skin keratinocytes led to cellular transformation, increased proliferation, and increased motility. Furthermore, WNT10B increased the expression of genes in the WNT, EGF, and MAPK pathways. These events could all be blocked by DKK1 (Lei et al., 2015).
In contrast to an oncogenic role for WNT10B in melanoma described previously, in a mouse cell line model of melanoma (B16F10), WNT10B reduced melanoma cell proliferation, induced cellular senescence, and enhanced tyrosinase (a marker of differentiation) activity in vitro. Furthermore, the injection of WNT10B protein into the tumor (subcutaneous injection of B16F10 melanoma cells) in mice inhibited tumor growth (Misu et al., 2015). These conflicting results present more questions that should be addressed on whether WNT10B in melanoma patients is beneficial, harmful, or both.
17.9 WNT10B and colorectal cancers
Almost all colorectal cancers (92%) have mutations and/or activation of the WNT pathway, notably mutations in APC (40%–80%) or β-catenin (5%–6%) (Schatoff et al., 2017). However, the role of WNT10B in colon adenocarcinoma is not well documented. A bioinformatics study in patients with colon adenocarcinoma demonstrated that high WNT10B expression was an independent predictor of worse overall survival in patients (Ruan et al., 2020). Low expression of miR-148a (Table 1), which targets WNT10B, was also correlated with worse overall survival, metastasis, and TNM stage, suggesting a role for WNT10B in prognosis as well (Shi et al., 2019). Cisplatin-resistant, stem-like SW480 cells had a decrease in miR-148a and an increase in WNT10B expression. Although a miR-148a mimic decreased sphere formation, invasion, and migration, WNT10B overexpression increased sphere formation, invasion, and migration (Shi et al., 2019).
Interestingly, in the CaCo-2 colon cancer cell line, filtrates from the blue–green microalga Spirulina platensis significantly decreased the autocrine secretion of WNT10B and had anti-proliferative and pro-apoptotic effects (Smieszek et al., 2017). However, the mechanism of WNT10B suppression was not elucidated.
Inositol hexaphosphate (IP6) and its molecular skeleton, inositol (INS), are natural compounds found in grains and legumes and have antitumor effects independently and in combination with each other. Liu et al. (2020e) evaluated gene expression changes in CRC liver metastasis in vivo in response to treatment with IP6, INS, or a combination of both (IP6 + INS) using the mouse CT26 colorectal cell line, orthotopically. They revealed through RNA-sequencing, RT-qPCR, and western blotting that the combinatorial treatment of IP6 + INS caused a decrease in the expression of WNT10B, TCF7, and c-MYC. They also found a decrease in rates of metastasis in the IP6 + INS group and proposed that this natural drug treatment inhibited metastasis by inhibiting WNT10B signaling. However, more studies should be conducted to make this conclusion (Liu et al., 2020e).
17.10 WNT10B and gastric cancer
WNT10B is overexpressed in gastric cancer compared to paired normal tissue and correlates with a higher rate of lymph node metastasis. Using shRNA-mediated WNT10B knockdown in SGC-7901 gastric cancer cells, Wu X. D. et al. (2017) showed that WNT10B knockdown inhibited gastric cancer proliferation and migration in vitro. Additionally, shWNT10B cells showed a switch from mesenchymal to epithelial with downregulation of N-cadherin and upregulation of E-cadherin in SGC-7901 cells. They also showed a positive correlation between WNT10B and cancer stem cell markers OCT4 and NANOG in gastric cancer tissues (Wu X. D. et al., 2017). This study provides a solid basis for further study on the mechanisms and potential therapeutic targets of WNT10B in gastric cancer.
17.11 WNT10B and brain cancer
A bioinformatics analysis of all 19 WNT ligands in human gliomas revealed an overexpression of WNT5A and a decreased expression of WNT10B in tumors compared to normal tissue. Furthermore, the overexpression of WNT5A and WNT16 correlated with worse overall survival in glioma patients, whereas high expression of WNT5B, WNT10B, and WNT3 correlated with better overall survival probability. In addition, WNT5A was positively correlated and WNT10B inversely correlated with glioma grade (Xu et al., 2020).
Conversely, Li et al. (2018) suggested that WNT10B is a driver of tumor progression in glioblastoma cells. They found that WNT10B and fatty acid-binding protein 4 (FABP4) were upregulated by RT-qPCR in malignant gliomas versus normal tissue. In glioma-derived U-87MG cells, shFABP4 significantly reduced the expression of WNT10B by immunofluorescence and RT-qPCR. Additionally, short hairpin knockdown of WNT10B reduced wound healing and invasion compared to control U-87MG cells. FABP4-silenced cells treated with constitutively active WNT10B rescued the reduced migration and invasion of the FABP4-silenced cells alone (Li H. Y. et al., 2018). Future studies will be needed to better understand the mechanistic role of WNT10B to determine if WNT10B is an oncogene or tumor suppressor in brain cancer.
17.12 WNT10B and endometrial cancer
WNT10B is significantly upregulated in endometrial cancerous tissues and cell lines compared to normal tissue (Chen et al., 2013; Zhou et al., 2016; Liu et al., 2020a). By immunohistochemistry of the proliferative phase, secretory phase, simple hyperplasia, complex hyperplasia, atypical hyperplasia, and endometrial carcinoma tissues, WNT10B had increased expression in the cancerous endometrial carcinoma samples. Furthermore, high expression of WNT10B was correlated with endometroid-type tumors, lower metastasis, and better overall survival (Chen et al., 2013). In comparison, in vitro cell studies show that the proliferation of Ishikawa 3-H-12 and AN3CA endometrial carcinoma cell lines was higher when WNT10B was overexpressed relative to control. This finding was confirmed using siWNT10B transfection. Additionally, WNT10B knockdown increased cell apoptosis rates compared to the control groups (Chen et al., 2013; Zhou et al., 2016).
In endometrial cancer stem cells, WNT10B activity is regulated by the matricellular glycoprotein SPARC-related modular calcium binding 2 (SMOC-2). SMOC-2 activates WNT/β-catenin signaling and cannot directly interact with WNT3A or WNT10B. Instead, SMOC-2 can enhance the binding between FZD6 and WNTs −3A and −10B or the binding between LRP6 and WNTs −3A or −10B. In the cancer stem cells, as assessed by proximal ligation assay, SMOC-2 can bind in a trimer with FZD6 and LRP6, and this trimer increases chemoresistance to paclitaxel and cisplatin (Lu et al., 2019).
Two studies have shown post-transcriptional regulation of WNT10B in endometrial cancer. One group found that miR-148a (Table 1) is significantly downregulated in cancer-associated fibroblasts in endometrial carcinoma patients and that WNT10B was upregulated. They showed through TargetScan predictions and luciferase assays that miR-148a binds directly to WNT10B mRNA to inhibit its expression. Furthermore, they demonstrated that WNT10B is directly responsible for the increase in tumor cell motility in endometrial cancer cells (Aprelikova et al., 2013).
Second, a positive association between WNT10B and the lncRNA HOXB-AS1 was found, along with a negative correlation between WNT10B and miR-149-3p (Table 1). miR-149-3p binds to the 3’-UTR of WNT10B to suppress expression, whereas HOXB-AS1 sponges miR-149-3p to allow for expression of WNT10B. Furthermore, HOXB-AS1 increased proliferation, migration, and invasion, whereas the addition of miR-149-3p mimics reduced this phenotype (Liu et al., 2020a).
17.13 WNT10B and prostate cancer
Madueke et al. (2019) compared the temporal and spatial expression of Wnt10b in normal and cancerous rat prostate development and metastasis. They found that when Wnt10b is expressed in early development, it slows growth. This reduced expression of Wnt10b is essential for branching morphogenesis. Then, they examined the Probasin/TAg prostate cancer rat model and found that prostate cancer had a significantly decreased expression of Wnt10b compared to normal prostate tissue. However, the metastatic prostate cancer cell lines HuSLC and PC3 had higher WNT10B expression than normal prostate tissue, and the very aggressive cell line PC3M31 had even higher WNT10B expression, significantly more so than PC3 cells, demonstrating different effects of WNT10B, depending on the timing of expression. Metastatic castrate-resistant patient samples also had higher WNT10B expression than localized prostate cancer. In PC3 cells, WNT10B knockdown produced significantly higher proliferation and migration rates. Furthermore, in vivo, WNT10B knockdown in PC3 cells led to significantly larger tumors than in the PC3 control cells. However, the WNT10B knockdown cells completely lost their metastatic capability. WNT10B knockdown cells resulted in a mesenchymal to epithelial switch. They also found a reduction in the stem cell population in WNT10B knockdown cells by FACS analysis and a reduction in SOX2 and NANOG expression (Madueke et al., 2019). To support this conclusion, Hu et al. (2017) found that WNT10B expression was localized in normal prostate spheroids that were quiescent and stem-like (Hu et al., 2017).
The endocrine-disrupting chemicals 17β-estradiol-3-benzoate (EB) and bisphenol A (BPA), which increase susceptibility to prostate cancer, decreased the DNA methylation of Wnt10b and subsequently increased the expression of Wnt10b in young rats. The combined expression of Wnt10b and six other methylated genes is associated with shorter recurrence-free survival in human patients (Cheong et al., 2016).
In contrast to the study by Madueke et al. (2019), in PC3 cells, WNT10B knockdown in the human prostate stromal cell line 19I in vivo decreased tumor formation and weight. Furthermore, they analyzed changes in stemness genes between control and WNT10B knockdown groups and found the mesenchymal stem cell markers OCT4, LIF, and CD90 downregulated in WNT10B-knockdown 19I cells (Dakhova et al., 2014). Overall, WNT10B’s mechanism in prostate cancer, particularly in cancer stem cell population, requires further study.
17.14 WNT10B and liver cancer
In hepatocellular carcinoma (HCC) specimens, WNT10B is significantly upregulated compared to normal liver tissue (Zhang J. et al., 2021). In HepG2 cells, WNT10B shRNA knockdown significantly reduced proliferation and migration. Furthermore, WNT10B knockdown resulted in an increased rate of apoptosis and a slowing of the cell cycle, with more cells present in the G0–G1 phase and fewer cells in the S phase compared to control HepG2 cells (Wu et al., 2015).
Overexpression of CORO6, an actin-binding protein and a possible driver of HCC progression, upregulated WNT10B by RT-qPCR. CORO6 overexpression also enhanced WNT signaling activation in TOPFLASH reporter assays compared to control cells. In vivo, Hep3B HCC cells with shRNA knockdown of CORO6 significantly decreased the growth rate of tumors. Protein levels of WNT10B, c-MYC, AXIN2, and cyclin D1 were downregulated in the CORO6-depleted tumors compared to the control Hep3B tumors (Zhang J. et al., 2021).
WNT10B has been identified as the target of several lncRNAs and miRNAs in HCC progression. Zhou et al. (2020) investigated lncRNAs and miRNAs in HCC and found that LINC003355:8 enhanced cell proliferation, migration, and invasion, whereas miR-6777-3p reduced proliferation, migration, and invasion. They identified WNT10B as a target of miR-6777-3p and that LINC00355:8 activates WNT10B by sponging miR-6777-3p to increase the expression of WNT signaling proteins β-catenin and c-MYC and inducing epithelial-to-mesenchymal transition markers (Table 1) (Zhou et al., 2020).
Two papers by Zhang et al. investigated the roles of WNT10B in HCC progression. First, while studying the effects of the transcriptional regulator NSD1 on HCC progression, they found a significantly positive correlation between NSD1 and WNT10B in HCC cells. CRISPR-mediated knockout of NSD1 since its gene KO enhanced the closed chromatin mark H3K27me3 in the promoter region of WNT10B, thereby reducing the expression of WNT10B. They also found that NSD1 knockout reduced WNT/β-catenin signaling in cells and observed a reduction in protein expression of β-catenin, c-MYC, and cyclin D1. They found that silencing WNT10B in conjunction with NSD1 further reduced WNT/β-catenin signaling as well as proliferation, migration, and invasion, whereas knocking down WNT10B in cells overexpressing NSD1 neutralized the effects. In vivo, WNT10B knockdown and NSD1 knockout were sufficient to reduce tumor volume and weight, and the combination knockdown/knockout further suppressed tumor volume and weight and reduced pulmonary metastasis significantly (Zhang et al., 2019). Using The Cancer Genome Atlas Liver Hepatocellular Carcinoma dataset, Zhang S. et al. (2021) identified the lncRNA KB-68A7.1, which inversely correlated with HCC prognosis. In their model system, they found that expression of KB-68A7.1 reduced the demethylation of histone 3 at lysine 36 (H3K36me2) and increased histone 3 lysine 27 trimethylation (H3K27me3) at the WNT10B promoter to decrease WNT10B expression. Additionally, overexpression of KB-68A7.1 sequestered NSD1 to the cytoplasm (Table 1). Consistent with this result, overexpression of KB-68A7.1 reduced the protein expression of WNT10B in HCC and hepatoma cell lines SNU-398 and HuH-7, and those with KB-68A7.1 knockdown increased expression of WNT10B in SNU-398, SK-HEP1, and THLE-3 cells. Furthermore, overexpression of KB-68A7.1 reduced proliferation, migration, invasion, and increased apoptosis rates, whereas WNT10B overexpression attenuated these effects. These results suggest a mechanism in which KB-68A7.1 functions as a tumor suppressor by sequestering NSD1 to the cytoplasm and inhibiting the transcription of WNT10B (Zhang S. et al., 2021).
Another lncRNA, CTB-193M12.5, is overexpressed in HCC and correlates with aggressiveness and poor overall survival. CTB-193M12.5 bound to NSD1 in RNA-protein pull-down assays and enhanced the binding of NSD1 to the WNT10B promoter for increased transcription of WNT10B (Table 1). Knockout of CTB-193M12.5 decreased proliferation, apoptosis, migration, and invasion, whereas overexpression of WNT10B reversed these effects (Zhang et al., 2022). In summary, NSD1 increased the transcription of WNT10B in HCC, and upstream lncRNAs modulated this interaction by inhibiting (KB-68A7.1) or activating (CTB-193M12.5) NSD1 and WNT10B, thereby contributing to HCC progression.
17.15 WNT10B and cholangiocarcinoma
In the first study of WNT10B in cholangiocarcinoma (biliary tract cancer), WNT10B was inhibited by miR-370 (Table 1), which in turn is inhibited by IL6. While the function of WNT10B in cholangiocarcinoma was not described, miR-370 overexpression reduced proliferation and was downregulated in cholangiocarcinoma versus normal tissue (An et al., 2012). Therefore, although the mechanism of WNT10B in cholangiocarcinoma is unknown, WNT10B reduction appears to correlate with less tumor proliferation.
18 Additional WNT10B polymorphisms
Many WNT10B polymorphisms have been identified in various conditions across species. Thus far, we have discussed polymorphisms in the WNT10B locus associated with obesity, dental anomalies, SFHM, and bone mineral density in humans. Additionally, the G607C polymorphism in the WNT10B promoter is associated with the Yin Deficiency pattern (a type of stroke in traditional Korean medicine that results from a deficiency of yin fluid and essence, incapable of restraining yang) and a phenomenon called bi-sup (a kind of body shape in Korean medicine) in Korean cerebral infarction patients (Ko et al., 2012; Ko et al., 2015). In cows, WNT10B polymorphisms in different breeds associate with body height, body length, body weight, and chest circumference (Zhao et al., 2012). In pigs, a polymorphism in the promoter of WNT10B is associated with litter size, as do two other WNT pathway genes [TCF12 and catenin alpha-like protein 1 (CTNNAL1)] (Tao et al., 2013). In Bernese mountain dogs, an SNP near WNT10B is associated with elbow dysplasia (Pfahler and Distl, 2012).
19 Conclusion
The knowledge and understanding of WNT10B have been greatly enhanced in the last decade. Interestingly, WNT10B has been studied not only in human, rodent, and zebrafish model systems, but also in goats, rabbits, sheep, cows, pigs, donkeys, and dogs. WNT10B is an oncogene in over 15 cancer types. WNT10B plays a role in cancer and has been implicated in the pathogenesis of osteoporosis, obesity, oligodontia, tooth agenesis, SHFM, fibrosis, PTSD, asthma, and rheumatoid arthritis (Figure 2).
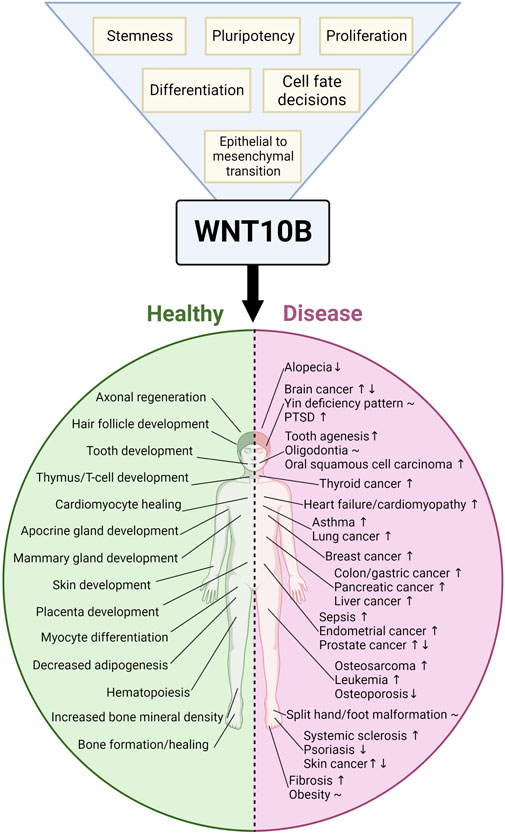
FIGURE 2. Summarizing the role of WNT10B in physiology and disease. The blue triangle with yellow squares represents across-tissue phenotypic observations of WNT10B’s function in physiology and disease, whereas the pink/green circle represents the tissue-specific roles of WNT10B. The green side represents tissues that are affected by WNT10B in a healthy developmental model, whereas the pink side represents the role of WNT10B in diseases. To the right of the disease is a symbol representing overexpression (↑), decreased expression (↓), or both (↑↓) of WNT10B. Additionally, diseases characterized by polymorphisms or mutations in WNT10B are given a (∼) symbol. Created with BioRender.com.
WNT ligands regulate stem cell biology, and WNT10B has been ascribed roles in mesenchymal, mammary, prostate, skin, hematopoietic, dental pulp, and cancer stem cells. WNT10B expression correlates with stem cell proliferation or differentiation, but the mechanism of action of WNT10B in stem cells is not well defined. This is an important area for future studies in regenerative medicine and anti-cancer therapies.
Although several WNT-signaling inhibitors are in early clinical trials for various cancer types, no WNT-specific drugs have been FDA-approved for cancer to date (Harb et al., 2019). In contrast, the WNT signaling activator (the anti-sclerostin antibody EVENITY) is approved for osteoporosis. However, this may not be through the activation of WNT10B signaling, as there remains an increase in bone mineral density in the WNT10B knockout mice treated with the anti-sclerostin antibody. Nevertheless, targeting WNT10B signaling could lead to therapies for many conditions.
The past decade has focused on WNT10B regulation. Numerous miRNAs, lncRNAs, and a circRNA regulate WNT10B (Table 1). miR-148a, the most studied of the RNAs, regulates WNT10B in adipocytes, lung fibrosis, pancreatic cancer, oral squamous cell carcinoma, colon adenocarcinoma, endometrial carcinoma, and thyroid cancer. In addition, many transcription factors and chemicals (such as butyrate from probiotics) regulate WNT10B.
In addition to therapeutics and understanding more about WNT10B regulation in different cell types, emerging areas for WNT10B studies may include fetal growth restriction and placenta biology, immune-oncology, and the tumor microenvironment in various cancer types. The work in the last decade on understanding the role of WNT10B in a variety of normal and disease processes is fascinating and holds exciting promise for enhancing our future understanding of normal development, pathogenesis, and treatment.
Author contributions
Conception and design: SK and GM-C. Writing, review, and/or revisions of the manuscript: RP, RS, AA, SK, and GM-C. Table and figures: RP, SK, and GM-C.
Conflict of interest
The authors declare that the research was conducted in the absence of any commercial or financial relationships that could be construed as a potential conflict of interest.
Publisher’s note
All claims expressed in this article are solely those of the authors and do not necessarily represent those of their affiliated organizations or those of the publisher, the editors, and the reviewers. Any product that may be evaluated in this article, or claim that may be made by its manufacturer, is not guaranteed or endorsed by the publisher.
References
Abiola, M., Favier, M., Christodoulou-Vafeiadou, E., Pichard, A. L., Martelly, I., and Guillet-Deniau, I. (2009). Activation of Wnt/beta-catenin signaling increases insulin sensitivity through a reciprocal regulation of Wnt10b and SREBP-1c in skeletal muscle cells. PloS one 4, e8509. doi:10.1371/journal.pone.0008509
Agostino, M., Pohl, S. O., and Dharmarajan, A. (2017). Structure-based prediction of Wnt binding affinities for Frizzled-type cysteine-rich domains. J. Biol. Chem. 292, 11218–11229. doi:10.1074/jbc.M117.786269
Al Ghamdi, M. A., Al-Qattan, M. M., Hadadi, A., Alabdulrahman, A., Almuzzaini, B., Alatwi, N., et al. (2020). A classification system for split-hand/foot malformation (SHFM): A proposal based on 3 pedigrees with WNT10B mutations. Eur. J. Med. Genet. 63, 103738. doi:10.1016/j.ejmg.2019.103738
An, F., Yamanaka, S., Allen, S., Roberts, L. R., Gores, G. J., Pawlik, T. M., et al. (2012). Silencing of miR-370 in human cholangiocarcinoma by allelic loss and interleukin-6 induced maternal to paternal epigenotype switch. PLoS One 7, e45606. doi:10.1371/journal.pone.0045606
Aoi, N., Inoue, K., Chikanishi, T., Fujiki, R., Yamamoto, H., Kato, H., et al. (2012). 1α,25-dihydroxyvitamin D3 modulates the hair-inductive capacity of dermal papilla cells: Therapeutic potential for hair regeneration. Stem Cells Transl. Med. 1, 615–626. doi:10.5966/sctm.2012-0032
Aprelikova, O., Palla, J., Hibler, B., Yu, X., Greer, Y. E., Yi, M., et al. (2013). Silencing of miR-148a in cancer-associated fibroblasts results in WNT10B-mediated stimulation of tumor cell motility. Oncogene 32, 3246–3253. doi:10.1038/onc.2012.351
Arpaia, N., Campbell, C., Fan, X., Dikiy, S., van der Veeken, J., deRoos, P., et al. (2013). Metabolites produced by commensal bacteria promote peripheral regulatory T-cell generation. Nature 504, 451–455. doi:10.1038/nature12726
Assarsson, M., Soderman, J., Duvetorp, A., Mrowietz, U., Skarstedt, M., and Seifert, O. (2019). Narrowband UVB treatment induces expression of WNT7B, WNT10B and TCF7L2 in psoriasis skin. Arch. Dermatol. Res. 311, 535–544. doi:10.1007/s00403-019-01931-y
Bai, L., Gao, S., Sun, H., Zhao, X., Yang, L., Hu, H., et al. (2019). Effects of Wnt10b on dermal papilla cells via the canonical Wnt/β-catenin signalling pathway in the Angora rabbit. J. Anim. Physiol. Anim. Nutr. Berl. 103, 1602–1609. doi:10.1111/jpn.13141
Bai, L., Sun, H., Jiang, W., Yang, L., Liu, G., Zhao, X., et al. (2021). DNA methylation and histone acetylation are involved in Wnt10b expression during the secondary hair follicle cycle in Angora rabbits. J. Anim. Physiol. Anim. Nutr. Berl. 105, 599–609. doi:10.1111/jpn.13481
Balciunaite, G., Keller, M. P., Balciunaite, E., Piali, L., Zuklys, S., Mathieu, Y. D., et al. (2002). Wnt glycoproteins regulate the expression of FoxN1, the gene defective in nude mice. Nat. Immunol. 3, 1102–1108. doi:10.1038/ni850
Bam, M., Yang, X., Busbee, B. P., Aiello, A. E., Uddin, M., Ginsberg, J. P., et al. (2020). Increased H3K4me3 methylation and decreased miR-7113-5p expression lead to enhanced Wnt/β-catenin signaling in immune cells from PTSD patients leading to inflammatory phenotype. Mol. Med. 26, 110. doi:10.1186/s10020-020-00238-3
Bam, M., Yang, X., Ginsberg, J. P., Aiello, A. E., Uddin, M., Galea, S., et al. (2022). Long non-coding RNA LINC00926 regulates WNT10B signaling pathway thereby altering inflammatory gene expression in PTSD. Transl. Psychiatry 12, 200. doi:10.1038/s41398-022-01971-5
Bedi, B., Li, J. Y., Tawfeek, H., Baek, K. H., Adams, J., Vangara, S. S., et al. (2012). Silencing of parathyroid hormone (PTH) receptor 1 in T cells blunts the bone anabolic activity of PTH. Proc. Natl. Acad. Sci. U. S. A. 109, E725–E733. doi:10.1073/pnas.1120735109
Beghini, A., Corlazzoli, F., Del Giacco, L., Re, M., Lazzaroni, F., Brioschi, M., et al. (2012). Regeneration-associated WNT signaling is activated in long-term reconstituting AC133bright acute myeloid leukemia cells. Neoplasia 14, 1236–1248. doi:10.1593/neo.121480
Belaya, Z. E., Grebennikova, T. A., Melnichenko, G. A., Nikitin, A. G., Solodovnikov, A. G., Brovkina, O. I., et al. (2018). Effects of endogenous hypercortisolism on bone mRNA and microRNA expression in humans. Osteoporos. Int. 29, 211–221. doi:10.1007/s00198-017-4241-7
Bennett, C. N., Longo, K. A., Wright, W. S., Suva, L. J., Lane, T. F., Hankenson, K. D., et al. (2005). Regulation of osteoblastogenesis and bone mass by Wnt10b. Proc. Natl. Acad. Sci. U. S. A. 102, 3324–3329. doi:10.1073/pnas.0408742102
Bennett, C. N., Ouyang, H., Ma, Y. L., Zeng, Q., Gerin, I., Sousa, K. M., et al. (2007). Wnt10b increases postnatal bone formation by enhancing osteoblast differentiation. J. Bone Mineral Res. Off. J. Am. Soc. Bone Mineral Res. 22, 1924–1932. doi:10.1359/jbmr.070810
Bergmann, C., and Distler, J. H. (2016). Canonical Wnt signaling in systemic sclerosis. Lab. Invest. a J. Tech. Methods Pathol. 96, 151–155. doi:10.1038/labinvest.2015.154
Beyer, C., Schramm, A., Akhmetshina, A., Dees, C., Kireva, T., Gelse, K., et al. (2012). β-catenin is a central mediator of pro-fibrotic Wnt signaling in systemic sclerosis. Ann. Rheum. Dis. 71, 761–767. doi:10.1136/annrheumdis-2011-200568
Bhatia, N., and Spiegelman, V. S. (2005). Activation of Wnt/beta-catenin/Tcf signaling in mouse skin carcinogenesis. Mol. Carcinog. 42, 213–221. doi:10.1002/mc.20077
Bigas, A., Guillen, Y., Schoch, L., and Arambilet, D. (2020). Revisiting beta-catenin signaling in T-cell development and T-cell acute lymphoblastic leukemia. BioEssays News Rev. Mol. Cell. Dev. Biol. 42, e1900099. doi:10.1002/bies.201900099
Bilal, M., Hayat, A., Umair, M., Ullah, A., Khawaja, S., Malik, E., et al. (2020). Sequence variants in the WNT10B and TP63 genes underlying isolated split-hand/split-foot malformation. Genet. Test. Mol. Biomarkers 24, 600–607. doi:10.1089/gtmb.2020.0024
Brun, J., Dieudonne, F. X., Marty, C., Muller, J., Schule, R., Patino-Garcia, A., et al. (2013). FHL2 silencing reduces Wnt signaling and osteosarcoma tumorigenesis in vitro and in vivo. PLoS One 8, e55034. doi:10.1371/journal.pone.0055034
Cao, L., Liu, W., Zhong, Y., Zhang, Y., Gao, D., He, T., et al. (2020). Linc02349 promotes osteogenesis of human umbilical cord-derived stem cells by acting as a competing endogenous RNA for miR-25-3p and miR-33b-5p. Cell Prolif. 53, e12814. doi:10.1111/cpr.12814
Cassaro, A., Grillo, G., Notaro, M., Gliozzo, J., Esposito, I., Reda, G., et al. (2021). FZD6 triggers Wnt-signalling driven by WNT10B(IVS1) expression and highlights new targets in T-cell acute lymphoblastic leukemia. Hematol. Oncol. 39, 364–379. doi:10.1002/hon.2840
Castrop, J., van Wichen, D., Koomans-Bitter, M., van de Wetering, M., de Weger, R., van Dongen, J., et al. (1995). The human TCF-1 gene encodes a nuclear DNA-binding protein uniquely expressed in normal and neoplastic T-lineage lymphocytes. Blood 86, 3050–3059. doi:10.1182/blood.v86.8.3050.bloodjournal8683050
Cawthorn, W. P., Bree, A. J., Yao, Y., Du, B., Hemati, N., Martinez-Santibanez, G., et al. (2012). Wnt6, Wnt10a and Wnt10b inhibit adipogenesis and stimulate osteoblastogenesis through a beta-catenin-dependent mechanism. Bone 50, 477–489. doi:10.1016/j.bone.2011.08.010
Cawthorn, W. P., Scheller, E. L., Learman, B. S., Parlee, S. D., Simon, B. R., Mori, H., et al. (2014). Bone marrow adipose tissue is an endocrine organ that contributes to increased circulating adiponectin during caloric restriction. Cell Metab. 20, 368–375. doi:10.1016/j.cmet.2014.06.003
Chen, K., Fallen, S., Abaan, H. O., Hayran, M., Gonzalez, C., Wodajo, F., et al. (2008). Wnt10b induces chemotaxis of osteosarcoma and correlates with reduced survival. Pediatr. blood cancer 51, 349–355. doi:10.1002/pbc.21595
Chen, H., Wang, Y., and Xue, F. (2013). Expression and the clinical significance of Wnt10a and Wnt10b in endometrial cancer are associated with the Wnt/β-catenin pathway. Oncol. Rep. 29, 507–514. doi:10.3892/or.2012.2126
Chen, Y., Zeng, C., Zhan, Y., Wang, H., Jiang, X., and Li, W. (2017). Aberrant low expression of p85α in stromal fibroblasts promotes breast cancer cell metastasis through exosome-mediated paracrine Wnt10b. Oncogene 36, 4692–4705. doi:10.1038/onc.2017.100
Chen, Y., Li, X., Xu, J., Xiao, H., Tang, C., Liang, W., et al. (2022). Knockdown of nuclear receptor binding SET domain-containing protein 1 (NSD1) inhibits proliferation and facilitates apoptosis in paclitaxel-resistant breast cancer cells via inactivating the Wnt/β-catenin signaling pathway. Bioengineered 13, 3526–3536. doi:10.1080/21655979.2021.2018973
Cheng, J. H., She, H., Han, Y. P., Wang, J., Xiong, S., Asahina, K., et al. (2008). Wnt antagonism inhibits hepatic stellate cell activation and liver fibrosis. Am. J. Physiol. Gastrointest. Liver Physiol. 294, G39–G49. doi:10.1152/ajpgi.00263.2007
Cheong, A., Zhang, X., Cheung, Y. Y., Tang, W. Y., Chen, J., Ye, S. H., et al. (2016). DNA methylome changes by estradiol benzoate and bisphenol A links early-life environmental exposures to prostate cancer risk. Epigenetics 11, 674–689. doi:10.1080/15592294.2016.1208891
Cho, Y. M., Kim, D. H., Kwak, S. N., Jeong, S. W., and Kwon, O. J. (2013). X-box binding protein 1 enhances adipogenic differentiation of 3T3-L1 cells through the downregulation of Wnt10b expression. FEBS Lett. 587, 1644–1649. doi:10.1016/j.febslet.2013.04.005
Cho, Y. M., Kim, T. M., Hun Kim, D., Hee Kim, D., Jeong, S. W., and Kwon, O. J. (2016). miR-148a is a downstream effector of X-box-binding protein 1 that silences Wnt10b during adipogenesis of 3T3-L1 cells. Exp. Mol. Med. 48, e226. doi:10.1038/emm.2016.3
Christodoulides, C., Scarda, A., Granzotto, M., Milan, G., Dalla Nora, E., Keogh, J., et al. (2006). WNT10B mutations in human obesity. Diabetologia 49, 678–684. doi:10.1007/s00125-006-0144-4
Chu, K. Y., Wang, Y. L., Chou, Y. R., Chen, J. T., Wang, Y. P., Simmer, J. P., et al. (2021). Synergistic mutations of LRP6 and WNT10A in familial tooth agenesis. J. Pers. Med. 11, 1217. doi:10.3390/jpm11111217
Collins, F. L., Rios-Arce, N. D., McCabe, L. R., and Parameswaran, N. (2017). Cytokine and hormonal regulation of bone marrow immune cell Wnt10b expression. PloS one 12, e0181979. doi:10.1371/journal.pone.0181979
Czepiel, M., Diviani, D., Jazwa-Kusior, A., Tkacz, K., Rolski, F., Smolenski, R. T., et al. (2022). Angiotensin II receptor 1 controls profibrotic Wnt/β-catenin signalling in experimental autoimmune myocarditis. Cardiovasc Res. 118, 573–584. doi:10.1093/cvr/cvab039
Dai, B. W., Yang, Z. M., Deng, P., Chen, Y. R., He, Z. J., Yang, X., et al. (2019). HOXC10 promotes migration and invasion via the WNT-EMT signaling pathway in oral squamous cell carcinoma. J. Cancer 10, 4540–4551. doi:10.7150/jca.30645
Dakhova, O., Rowley, D., and Ittmann, M. (2014). Genes upregulated in prostate cancer reactive stroma promote prostate cancer progression in vivo. Clin. Cancer Res. 20, 100–109. doi:10.1158/1078-0432.CCR-13-1184
D'Amelio, P., Sassi, F., Buondonno, I., Fornelli, G., Spertino, E., D'Amico, L., et al. (2015). Treatment with intermittent PTH increases Wnt10b production by T cells in osteoporotic patients. Osteoporos. Int. 26, 2785–2791. doi:10.1007/s00198-015-3189-8
DaSilva-Arnold, S., James, J. L., Al-Khan, A., Zamudio, S., and Illsley, N. P. (2015). Differentiation of first trimester cytotrophoblast to extravillous trophoblast involves an epithelial-mesenchymal transition. Placenta 36, 1412–1418. doi:10.1016/j.placenta.2015.10.013
Dassule, H. R., and McMahon, A. P. (1998). Analysis of epithelial-mesenchymal interactions in the initial morphogenesis of the mammalian tooth. Dev. Biol. 202, 215–227. doi:10.1006/dbio.1998.8992
Dey, N., Barwick, B. G., Moreno, C. S., Ordanic-Kodani, M., Chen, Z., Oprea-Ilies, G., et al. (2013). Wnt signaling in triple negative breast cancer is associated with metastasis. BMC Cancer 13, 537. doi:10.1186/1471-2407-13-537
El Ayachi, I., Fatima, I., Wend, P., Alva-Ornelas, J. A., Runke, S., Kuenzinger, W. L., et al. (2019). The WNT10B network is associated with survival and metastases in chemoresistant triple-negative breast cancer. Cancer Res. 79, 982–993. doi:10.1158/0008-5472.CAN-18-1069
Elalaoui, S. C., Fejjal, N., Li, Y., Thiele, H., Altmuller, J., Guaoua, S., et al. (2021). Homozygous nonsense mutation of WNT10B gene in a Moroccan family with split-hand foot malformation identified by exome sequencing: A case report. Pan Afr. Med. J. 39, 21. doi:10.11604/pamj.2021.39.21.26176
Estrada, K., Styrkarsdottir, U., Evangelou, E., Hsu, Y. H., Duncan, E. L., Ntzani, E. E., et al. (2012). Genome-wide meta-analysis identifies 56 bone mineral density loci and reveals 14 loci associated with risk of fracture. Nat. Genet. 44, 491–501. doi:10.1038/ng.2249
Falk, W., Mannel, D. N., and Droge, W. (1983). Activation of cytotoxic T lymphocytes requires at least two spleen cell-derived helper factors besides interleukin 2. J. Immunol. 130, 2214–2218. doi:10.4049/jimmunol.130.5.2214
Fatima, I., El-Ayachi, I., Taotao, L., Lillo, M. A., Krutilina, R. I., Seagroves, T. N., et al. (2017). The natural compound Jatrophone interferes with Wnt/β-catenin signaling and inhibits proliferation and EMT in human triple-negative breast cancer. PloS one 12, e0189864. doi:10.1371/journal.pone.0189864
Fatima, I., El-Ayachi, I., Playa, H. C., Alva-Ornelas, J. A., Khalid, A. B., Kuenzinger, W. L., et al. (2019). Simultaneous multi-organ metastases from chemo-resistant triple-negative breast cancer are prevented by interfering with WNT-signaling. Cancers 11, 2039. doi:10.3390/cancers11122039
Fournier, C. (2005). Where do T cells stand in rheumatoid arthritis? Jt. Bone Spine 72, 527–532. doi:10.1016/j.jbspin.2004.12.012
Fox, K. E., Colton, L. A., Erickson, P. F., Friedman, J. E., Cha, H. C., Keller, P., et al. (2008). Regulation of cyclin D1 and Wnt10b gene expression by cAMP-responsive element-binding protein during early adipogenesis involves differential promoter methylation. J. Biol. Chem. 283, 35096–35105. doi:10.1074/jbc.M806423200
Frey, J. L., Li, Z., Ellis, J. M., Zhang, Q., Farber, C. R., Aja, S., et al. (2015). Wnt-Lrp5 signaling regulates fatty acid metabolism in the osteoblast. Mol. Cell Biol. 35, 1979–1991. doi:10.1128/MCB.01343-14
Gao, F., Zhang, C. Q., Chai, Y. M., and Li, X. L. (2015). Lentivirus-mediated Wnt10b overexpression enhances fracture healing in a rat atrophic non-union model. Biotechnol. Lett. 37, 733–739. doi:10.1007/s10529-014-1703-2
Gatica-Andrades, M., Vagenas, D., Kling, J., Nguyen, T. T. K., Benham, H., Thomas, R., et al. (2017). WNT ligands contribute to the immune response during septic shock and amplify endotoxemia-driven inflammation in mice. Blood Adv. 1, 1274–1286. doi:10.1182/bloodadvances.2017006163
Gaur, T., Lengner, C. J., Hovhannisyan, H., Bhat, R. A., Bodine, P. V., Komm, B. S., et al. (2005). Canonical WNT signaling promotes osteogenesis by directly stimulating Runx2 gene expression. J. Biol. Chem. 280, 33132–33140. doi:10.1074/jbc.M500608200
Georgiou, K. R., Nadhanan, R. R., Fan, C. M., and Xian, C. J. (2015). Methotrexate-induced bone marrow adiposity is mitigated by folinic acid supplementation through the regulation of Wnt/β-catenin signalling. J. Cell Physiol. 230, 648–656. doi:10.1002/jcp.24788
Goel, S., Chin, E. N., Fakhraldeen, S. A., Berry, S. M., Beebe, D. J., and Alexander, C. M. (2012). Both LRP5 and LRP6 receptors are required to respond to physiological Wnt ligands in mammary epithelial cells and fibroblasts. J. Biol. Chem. 287, 16454–16466. doi:10.1074/jbc.M112.362137
Golestaneh, N., Beauchamp, E., Fallen, S., Kokkinaki, M., Uren, A., and Dym, M. (2009). Wnt signaling promotes proliferation and stemness regulation of spermatogonial stem/progenitor cells. Reproduction 138, 151–162. doi:10.1530/REP-08-0510
Harb, J., Lin, P. J., and Hao, J. (2019). Recent development of wnt signaling pathway inhibitors for cancer therapeutics. Curr. Oncol. Rep. 21, 12. doi:10.1007/s11912-019-0763-9
Hardiman, G., Kastelein, R. A., and Bazan, J. F. (1997). Isolation, characterization and chromosomal localization of human WNT10B. Cytogenet Cell Genet. 77, 278–282. doi:10.1159/000134597
Harwood, B. N., Cross, S. K., Radford, E. E., Haac, B. E., and De Vries, W. N. (2008). Members of the WNT signaling pathways are widely expressed in mouse ovaries, oocytes, and cleavage stage embryos. Dev. Dyn. 237, 1099–1111. doi:10.1002/dvdy.21491
Hawkshaw, N. J., Hardman, J. A., Alam, M., Jimenez, F., and Paus, R. (2020). Deciphering the molecular morphology of the human hair cycle: Wnt signalling during the telogen-anagen transformation. Br. J. Dermatol. 182, 1184–1193. doi:10.1111/bjd.18356
Hsiao, C. Y., Chen, T. H., Chu, T. H., Ting, Y. N., Tsai, P. J., and Shyu, J. F. (2020). Calcitonin induces bone formation by increasing expression of Wnt10b in osteoclasts in ovariectomy-induced osteoporotic rats. Front. Endocrinol. 11, 613. doi:10.3389/fendo.2020.00613
Hsu, M. N., Huang, K. L., Yu, F. J., Lai, P. L., Truong, A. V., Lin, M. W., et al. (2020). Coactivation of endogenous Wnt10b and foxc2 by CRISPR activation enhances BMSC osteogenesis and promotes calvarial bone regeneration. Mol. Ther. 28, 441–451. doi:10.1016/j.ymthe.2019.11.029
Hu, W. Y., Hu, D. P., Xie, L., Li, Y., Majumdar, S., Nonn, L., et al. (2017). Isolation and functional interrogation of adult human prostate epithelial stem cells at single cell resolution. Stem Cell Res. 23, 1–12. doi:10.1016/j.scr.2017.06.009
Hu, H. H., Cao, G., Wu, X. Q., Vaziri, N. D., and Zhao, Y. Y. (2020). Wnt signaling pathway in aging-related tissue fibrosis and therapies. Ageing Res. Rev. 60, 101063. doi:10.1016/j.arr.2020.101063
Hu, Y., He, Y., Fang, J., Liu, Y., Cao, Y., Tong, W., et al. (2022). Wnt10b-overexpressing umbilical cord mesenchymal stem cells promote fracture healing via accelerated cartilage callus to bone remodeling. Bioengineered 13, 10313–10323. doi:10.1080/21655979.2022.2062954
Jeong, J. Y., Kim, J. S., Nguyen, T. H., Lee, H. J., and Baik, M. (2013). Wnt/β-catenin signaling and adipogenic genes are associated with intramuscular fat content in the longissimus dorsi muscle of Korean cattle. Anim. Genet. 44, 627–635. doi:10.1111/age.12061
Kadota, T., Fujita, Y., Araya, J., Watanabe, N., Fujimoto, S., Kawamoto, H., et al. (2021). Human bronchial epithelial cell-derived extracellular vesicle therapy for pulmonary fibrosis via inhibition of TGF-beta-WNT crosstalk. J. Extracell. Vesicles 10, e12124. doi:10.1002/jev2.12124
Kamimura, D., Uchino, K., Ishigami, T., Hall, M. E., and Umemura, S. (2016). Activation of peroxisome proliferator-activated receptor gamma prevents development of heart failure with preserved ejection fraction; inhibition of wnt-beta-catenin signaling as a possible mechanism. J. Cardiovasc Pharmacol. 68, 155–161. doi:10.1097/FJC.0000000000000397
Kang, S., Bajnok, L., Longo, K. A., Petersen, R. K., Hansen, J. B., Kristiansen, K., et al. (2005). Effects of Wnt signaling on Brown adipocyte differentiation and metabolism mediated by PGC-1alpha. Mol. Cell Biol. 25, 1272–1282. doi:10.1128/MCB.25.4.1272-1282.2005
Kantaputra, P. N., Hutsadaloi, A., Kaewgahya, M., Intachai, W., German, R., Koparal, M., et al. (2018). WNT10B mutations associated with isolated dental anomalies. Clin. Genet. 93, 992–999. doi:10.1111/cge.13218
Karczewska-Kupczewska, M., Stefanowicz, M., Matulewicz, N., Nikolajuk, A., and Straczkowski, M. (2016). Wnt signaling genes in adipose tissue and skeletal muscle of humans with different degrees of insulin sensitivity. J. Clin. Endocrinol. Metab. 101, 3079–3087. doi:10.1210/jc.2016-1594
Khalid, A. B., Pence, J., Suthon, S., Lin, J., Miranda-Carboni, G. A., and Krum, S. A. (2021). GATA4 regulates mesenchymal stem cells via direct transcriptional regulation of the WNT signalosome. Bone 144, 115819. doi:10.1016/j.bone.2020.115819
Khan, S., Basit, S., Zimri, F. K., Ali, N., Ali, G., Ansar, M., et al. (2012). A novel homozygous missense mutation in WNT10B in familial split-hand/foot malformation. Clin. Genet. 82, 48–55. doi:10.1111/j.1399-0004.2011.01698.x
Khan, A., Wang, R., Han, S., Umair, M., Alshabeeb, M. A., Ansar, M., et al. (2019). A novel homozygous nonsense mutation p.Cys366* in the WNT10B gene underlying split-hand/split foot malformation in a consanguineous Pakistani family. Front. Pediatr. 7, 526. doi:10.3389/fped.2019.00526
Kim, I. C., Cha, M. H., Kim, D. M., Lee, H., Moon, J. S., Choi, S. M., et al. (2011). A functional promoter polymorphism -607G>C of WNT10B is associated with abdominal fat in Korean female subjects. J. Nutr. Biochem. 22, 252–258. doi:10.1016/j.jnutbio.2010.02.002
Kitagawa, M., Hatakeyama, S., Shirane, M., Matsumoto, M., Ishida, N., Hattori, K., et al. (1999). An F-box protein, FWD1, mediates ubiquitin-dependent proteolysis of beta-catenin. EMBO J. 18, 2401–2410. doi:10.1093/emboj/18.9.2401
Ko, M. M., Park, T. Y., Lim, J. H., Cha, M. H., and Lee, M. S. (2012). WNT10B polymorphism in Korean stroke patients with yin deficiency pattern. Evid. Based Complement. Altern. Med. 2012, 798131. doi:10.1155/2012/798131
Ko, M. M., Lee, M. S., and Cha, M. H. (2015). Genetic association of G-607C Located at wnt10b promoter with bi-sup type among Korean cerebral infarction patients. Int. J. Clin. Exp. Med. 8, 21407–21413.
Kuwahara, Y., Kishimoto, K. N., Itoigawa, Y., Okuno, H., Hatta, T., Matsuzawa, G., et al. (2019). Fatty degeneration and wnt10b expression in the supraspinatus muscle after surgical repair of torn rotator cuff tendon. J. Orthop. Surg. 27, 2309499019864817. doi:10.1177/2309499019864817
Lane, T. F., and Leder, P. (1997). Wnt-10b directs hypermorphic development and transformation in mammary glands of male and female mice. Oncogene 15, 2133–2144. doi:10.1038/sj.onc.1201593
Lazzaroni, F., Del Giacco, L., Biasci, D., Turrini, M., Prosperi, L., Brusamolino, R., et al. (2016). Intronless WNT10B-short variant underlies new recurrent allele-specific rearrangement in acute myeloid leukaemia. Sci. Rep. 6, 37201. doi:10.1038/srep37201
Lee, J. G., and Heur, M. (2015). WNT10B enhances proliferation through beta-catenin and RAC1 GTPase in human corneal endothelial cells. J. Biol. Chem. 290, 26752–26764. doi:10.1074/jbc.M115.677245
Lee, F. S., Lane, T. F., Kuo, A., Shackleford, G. M., and Leder, P. (1995). Insertional mutagenesis identifies a member of the Wnt gene family as a candidate oncogene in the mammary epithelium of int-2/Fgf-3 transgenic mice. Proc. Natl. Acad. Sci. U. S. A. 92, 2268–2272. doi:10.1073/pnas.92.6.2268
Lee, Y. H., Kim, S. H., Lee, Y. J., Kang, E. S., Lee, B. W., Cha, B. S., et al. (2013). Transcription factor Snail is a novel regulator of adipocyte differentiation via inhibiting the expression of peroxisome proliferator-activated receptor γ. Cell Mol. Life Sci. 70, 3959–3971. doi:10.1007/s00018-013-1363-8
Lee, A. M. C., Bowen, J. M., Su, Y. W., Plews, E., Chung, R., Keefe, D. M. K., et al. (2019). Individual or combination treatments with lapatinib and paclitaxel cause potential bone loss and bone marrow adiposity in rats. J. Cell. Biochem. 120, 4180–4191. doi:10.1002/jcb.27705
Lei, M., Guo, H., Qiu, W., Lai, X., Yang, T., Widelitz, R. B., et al. (2014). Modulating hair follicle size with Wnt10b/DKK1 during hair regeneration. Exp. Dermatol. 23, 407–413. doi:10.1111/exd.12416
Lei, M., Lai, X., Bai, X., Qiu, W., Yang, T., Liao, X., et al. (2015). Prolonged overexpression of Wnt10b induces epidermal keratinocyte transformation through activating EGF pathway. Histochem Cell Biol. 144, 209–221. doi:10.1007/s00418-015-1330-6
Leiros, G. J., Ceruti, J. M., Castellanos, M. L., Kusinsky, A. G., and Balana, M. E. (2017). Androgens modify Wnt agonists/antagonists expression balance in dermal papilla cells preventing hair follicle stem cell differentiation in androgenetic alopecia. Mol. Cell Endocrinol. 439, 26–34. doi:10.1016/j.mce.2016.10.018
Li, J. Y., Adams, J., Calvi, L. M., Lane, T. F., DiPaolo, R., Weitzmann, M. N., et al. (2012). PTH expands short-term murine hemopoietic stem cells through T cells. Blood 120, 4352–4362. doi:10.1182/blood-2012-06-438531
Li, J. Y., Adams, J., Calvi, L. M., Lane, T. F., Weitzmann, M. N., and Pacifici, R. (2013). Ovariectomy expands murine short-term hemopoietic stem cell function through T cell expressed CD40L and Wnt10B. Blood 122, 2346–2357. doi:10.1182/blood-2013-03-487801
Li, Y. H., Zhang, K., Yang, K., Ye, J. X., Xing, Y. Z., Guo, H. Y., et al. (2013). Adenovirus-mediated Wnt10b overexpression induces hair follicle regeneration. J. Invest. Dermatol. 133, 42–48. doi:10.1038/jid.2012.235
Li, H. Y., Lv, B. B., and Bi, Y. H. (2018). FABP4 accelerates glioblastoma cell growth and metastasis through Wnt10b signalling. Eur. Rev. Med. Pharmacol. Sci. 22, 7807–7818. doi:10.26355/eurrev_201811_16405
Li, S., Zheng, X., Nie, Y., Chen, W., Liu, Z., Tao, Y., et al. (2018). Defining key genes regulating morphogenesis of apocrine sweat gland in sheepskin. Front. Genet. 9, 739. doi:10.3389/fgene.2018.00739
Li, F. S., Li, P. P., Li, L., Deng, Y., Hu, Y., and He, B. C. (2020a). PTEN reduces BMP9-induced osteogenic differentiation through inhibiting Wnt10b in mesenchymal stem cells. Front. Cell Dev. Biol. 8, 608544. doi:10.3389/fcell.2020.608544
Li, J. Y., Yu, M., Pal, S., Tyagi, A. M., Dar, H., Adams, J., et al. (2020b). Parathyroid hormone-dependent bone formation requires butyrate production by intestinal microbiota. J. Clin. Invest. 130, 1767–1781. doi:10.1172/JCI133473
Li, C., Chen, X., Liu, T., and Chen, G. (2021a). lncRNA HOTAIRM1 regulates cell proliferation and the metastasis of thyroid cancer by targeting Wnt10b. Oncol. Rep. 45, 1083–1093. doi:10.3892/or.2020.7919
Li, Z., Tian, Y., Zhang, L., Zhang, T., Wang, P., and Wang, J. (2021b). Type II collagen from squid cartilage mediated myogenic IGF-I and irisin to activate the Ihh/PThrp and Wnt/β-catenin pathways to promote fracture healing in mice. Food Funct. 12, 6502–6512. doi:10.1039/d0fo03069d
Li, F., Wang, Y., Xu, M., Hu, N., Miao, J., Zhao, Y., et al. (2022). Single-nucleus RNA Sequencing reveals the mechanism of cigarette smoke exposure on diminished ovarian reserve in mice. Ecotoxicol. Environ. Saf. 245, 114093. doi:10.1016/j.ecoenv.2022.114093
Li, H., Jia, Y., Weng, D., Ju, Z., Zhao, Y., Liu, S., et al. (2022). Clostridium butyricum inhibits fat deposition via increasing the frequency of adipose tissue-resident regulatory T cells. Mol. Nutr. Food Res. 66, e2100884. doi:10.1002/mnfr.202100884
Li, W., Qiu, L., Guan, J., Sun, Y., Zhao, J., and Du, M. (2022). Comparative transcriptome analysis of longissimus dorsi tissues with different intramuscular fat contents from Guangling donkeys. BMC Genomics 23, 644. doi:10.1186/s12864-022-08857-2
Li, X., Chen, W., Huang, L., Zhu, M., Zhang, H., Si, Y., et al. (2022). Sinomenine hydrochloride suppresses the stemness of breast cancer stem cells by inhibiting Wnt signaling pathway through down-regulation of WNT10B. Pharmacol. Res. 179, 106222. doi:10.1016/j.phrs.2022.106222
Liang, K., Du, Y., Chen, L., Wang, L., Li, R., Yan, Z., et al. (2020). Contrary roles of wnt/β-catenin signaling in BMP9-induced osteogenic and adipogenic differentiation of 3T3-L1 preadipocytes. Cell Biochem. Biophys. 78, 347–356. doi:10.1007/s12013-020-00935-0
Liao, Y. P., Du, W. M., Hu, Y., Li, F. S., Ma, Y., Wang, H., et al. (2019). CREB/Wnt10b mediates the effect of COX-2 on promoting BMP9-induced osteogenic differentiation via reducing adipogenic differentiation in mesenchymal stem cells. J. Cell. Biochem. 120, 9572–9587. doi:10.1002/jcb.28234
Lin, B. J., Lin, G. Y., Zhu, J. Y., Yin, G. Q., Huang, D., and Yan, Y. Y. (2020). LncRNA-PCAT1 maintains characteristics of dermal papilla cells and promotes hair follicle regeneration by regulating miR-329/Wnt10b axis. Exp. Cell Res. 394, 112031. doi:10.1016/j.yexcr.2020.112031
Ling, T., Tran, M., Gonzalez, M. A., Gautam, L. N., Connelly, M., Wood, R. K., et al. (2015). (+)-Dehydroabietylamine derivatives target triple-negative breast cancer. Eur. J. Med. Chem. 102, 9–13. doi:10.1016/j.ejmech.2015.07.034
Liu, Y., Almeida, M., Weinstein, R. S., O'Brien, C. A., Manolagas, S. C., and Jilka, R. L. (2016). Skeletal inflammation and attenuation of Wnt signaling, Wnt ligand expression, and bone formation in atherosclerotic ApoE-null mice. Am. J. Physiol. Endocrinol. Metab. 310, E762–E773. doi:10.1152/ajpendo.00501.2015
Liu, H., Zhang, N., Liu, Y., Liu, L., Yin, G., and En, L. (2018). Effect of human Wnt10b transgene overexpression on peri-implant osteogenesis in ovariectomized rats. Hum. gene Ther. 29, 1416–1427. doi:10.1089/hum.2018.003
Liu, D., Pang, Q., Han, Q., Shi, Q., Zhang, Q., and Yu, H. (2019a). Wnt10b participates in regulating fatty acid synthesis in the muscle of zebrafish. Cells 8, 1011. doi:10.3390/cells8091011
Liu, Y. B., Lin, L. P., Zou, R., Zhao, Q. H., and Lin, F. Q. (2019b). Silencing long non-coding RNA MEG3 accelerates tibia fraction healing by regulating the Wnt/β-catenin signalling pathway. J. Cell Mol. Med. 23, 3855–3866. doi:10.1111/jcmm.14229
Liu, D., Qiu, M., Jiang, L., and Liu, K. (2020a). Long noncoding RNA HOXB-AS1 is upregulated in endometrial carcinoma and sponged miR-149-3p to upregulate Wnt10b. Technol. Cancer Res. Treat. 19, 1533033820967462. doi:10.1177/1533033820967462
Liu, D., Yu, H., and Zhang, Q. (2020b). Dietary vitamin E regulates the activity of antioxidant enzymes through Wnt10b signaling in the muscle of zebrafish. Food Funct. 11, 10665–10674. doi:10.1039/d0fo02083d
Liu, L., Zhao, M., Xie, Z. G., Liu, J., Peng, H. P., Pei, Y. F., et al. (2020c). Twelve new genomic loci associated with bone mineral density. Front. Endocrinol. 11, 243. doi:10.3389/fendo.2020.00243
Liu, X., Fan, J. B., Hu, J., Li, F., Yi, R., Tan, F., et al. (2020d). Lactobacillus fermentum ZS40 prevents secondary osteoporosis in wistar rat. Food Sci. Nutr. 8, 5182–5191. doi:10.1002/fsn3.1824
Liu, X., Liu, C., Chen, C., Sun, W., Ci, Y., Li, Q., et al. (2020e). Combination of inositol hexaphosphate and inositol inhibits liver metastasis of colorectal cancer in mice through the wnt/β-catenin pathway. Onco Targets Ther. 13, 3223–3235. doi:10.2147/OTT.S247646
Liu, X., Zheng, J., Li, F., Yi, R., Mu, J., Tan, F., et al. (2020f). Lactobacillus plantarum HFY15 helps prevent retinoic acid-induced secondary osteoporosis in wistar rats. Evid. Based Complement. Altern. Med. 2020, 2054389. doi:10.1155/2020/2054389
Liu, Y., Fang, J., Zhang, Q., Zhang, X., Cao, Y., Chen, W., et al. (2020g). Wnt10b-overexpressing umbilical cord mesenchymal stem cells promote critical size rat calvarial defect healing by enhanced osteogenesis and VEGF-mediated angiogenesis. J. Orthop. Transl. 23, 29–37. doi:10.1016/j.jot.2020.02.009
Liu, J., Mu, Q., Liu, Z., Wang, Y., Liu, J., Wu, Z., et al. (2021). Melatonin regulates the periodic growth of cashmere by upregulating the expression of Wnt10b and beta-catenin in inner Mongolia cashmere goats. Front. Genet. 12, 665834. doi:10.3389/fgene.2021.665834
Longo, K. A., Wright, W. S., Kang, S., Gerin, I., Chiang, S. H., Lucas, P. C., et al. (2004). Wnt10b inhibits development of white and Brown adipose tissues. J. Biol. Chem. 279, 35503–35509. doi:10.1074/jbc.M402937200
Lotinun, S., and Krishnamra, N. (2016). Disruption of c-kit signaling in kit(W-sh/W-sh) growing mice increases bone turnover. Sci. Rep. 6, 31515. doi:10.1038/srep31515
Lu, H., Li, X., Mu, P., Qian, B., Jiang, W., and Zeng, L. (2016). Dickkopf-1 promotes the differentiation and adipocytokines secretion via canonical Wnt signaling pathway in primary cultured human preadipocytes. Obes. Res. Clin. Pract. 10, 454–464. doi:10.1016/j.orcp.2015.08.016
Lu, C. L., Shyu, J. F., Wu, C. C., Hung, C. F., Liao, M. T., Liu, W. C., et al. (2018a). Association of anabolic effect of calcitriol with osteoclast-derived wnt 10b secretion. Nutrients 10, 1164. doi:10.3390/nu10091164
Lu, H. J., Yan, J., Jin, P. Y., Zheng, G. H., Zhang, H. L., Bai, M., et al. (2018b). Mechanism of MicroRNA-708 targeting BAMBI in cell proliferation, migration, and apoptosis in mice with melanoma via the wnt and TGF-beta signaling pathways. Technol. Cancer Res. Treat. 17, 1533034618756784. doi:10.1177/1533034618756784
Lu, H., Ju, D. D., Yang, G. D., Zhu, L. Y., Yang, X. M., Li, J., et al. (2019). Targeting cancer stem cell signature gene SMOC-2 Overcomes chemoresistance and inhibits cell proliferation of endometrial carcinoma. EBioMedicine 40, 276–289. doi:10.1016/j.ebiom.2018.12.044
Luo, A., Wu, F., Han, R., Huang, S., Zhang, Y., Jing, X., et al. (2017). Clinicopathological features and prognostic evaluation of bone metastasis in triple-negative breast cancer. J. Cancer Res. Ther. 13, 778–784. doi:10.4103/jcrt.JCRT_543_17
MacDonald, B. T., Tamai, K., and He, X. (2009). Wnt/beta-catenin signaling: Components, mechanisms, and diseases. Dev. Cell 17, 9–26. doi:10.1016/j.devcel.2009.06.016
Madueke, I., Hu, W. Y., Hu, D., Swanson, S. M., Vander Griend, D., Abern, M., et al. (2019). The role of WNT10B in normal prostate gland development and prostate cancer. Prostate 79, 1692–1704. doi:10.1002/pros.23894
Magruder, S., Carter, E., Williams, M. A., English, J., Akyalcin, S., and Letra, A. (2018). Further evidence for the role of WNT10A, WNT10B and GREM2 as candidate genes for isolated tooth agenesis. Orthod. Craniofac Res. 21, 258–263. doi:10.1111/ocr.12248
Malhotra, S. S., Suman, P., and Gupta, S. K. (2015). Alpha or beta human chorionic gonadotropin knockdown decrease BeWo cell fusion by down-regulating PKA and CREB activation. Sci. Rep. 5, 11210. doi:10.1038/srep11210
Malhotra, S. S., Banerjee, P., Chaudhary, P., Pal, R., and Gupta, S. K. (2017). Relevance of Wnt10b and activation of beta-catenin/GCMa/syncytin-1 pathway in BeWo cell fusion. Am. J. Reprod. Immunol. 78, e12676. doi:10.1111/aji.12676
Martinez Del Pino, L., Arana, A., Alfonso, L., Mendizabal, J. A., and Soret, B. (2017). Adiposity and adipogenic gene expression in four different muscles in beef cattle. PloS one 12, e0179604. doi:10.1371/journal.pone.0179604
Matsushita, K., Wu, Y., Pratt, R. E., and Dzau, V. J. (2016). Deletion of angiotensin II type 2 receptor accelerates adipogenesis in murine mesenchymal stem cells via Wnt10b/beta-catenin signaling. Lab. Invest. 96, 909–917. doi:10.1038/labinvest.2016.66
Maupin, K. A., Droscha, C. J., and Williams, B. O. (2013). A comprehensive overview of skeletal phenotypes associated with alterations in wnt/β-catenin signaling in humans and mice. Bone Res. 1, 27–71. doi:10.4248/BR201301004
Menefee, D. S., McMasters, A., Pan, J., Li, X., Xiao, D., Waigel, S., et al. (2020). Age-related transcriptome changes in melanoma patients with tumor-positive sentinel lymph nodes. Aging (Albany NY) 12, 24914–24939. doi:10.18632/aging.202435
Min, A., Zhu, C., Peng, S., Shuai, C., Sun, L., Han, Y., et al. (2016). Downregulation of microrna-148a in cancer-associated fibroblasts from oral cancer promotes cancer cell migration and invasion by targeting Wnt10b. J. Biochem. Mol. Toxicol. 30, 186–191. doi:10.1002/jbt.21777
Miranda-Carboni, G. A., Krum, S. A., Yee, K., Nava, M., Deng, Q. E., Pervin, S., et al. (2008). A functional link between Wnt signaling and SKP2-independent p27 turnover in mammary tumors. Genes Dev. 22, 3121–3134. doi:10.1101/gad.1692808
Misu, M., Ouji, Y., Kawai, N., Nishimura, F., Nakamura-Uchiyama, F., and Yoshikawa, M. (2015). Effects of Wnt-10b on proliferation and differentiation of murine melanoma cells. Biochem. Biophys. Res. Commun. 463, 618–623. doi:10.1016/j.bbrc.2015.05.110
Muff, R., Rath, P., Ram Kumar, R. M., Husmann, K., Born, W., Baudis, M., et al. (2015). Genomic instability of osteosarcoma cell lines in culture: Impact on the prediction of metastasis relevant genes. PLoS One 10, e0125611. doi:10.1371/journal.pone.0125611
Neuhaus, J., Weimann, A., and Berndt-Paetz, M. (2021). Immunocytochemical analysis of endogenous frizzled-(Co-)Receptor interactions and rapid wnt pathway activation in mammalian cells. Int. J. Mol. Sci. 22, 12057. doi:10.3390/ijms222112057
Newman, D. J., and Cragg, G. M. (2007). Natural products as sources of new drugs over the last 25 years. J. Nat. Prod. 70, 461–477. doi:10.1021/np068054v
Nicu, C., O'Sullivan, J. D. B., Ramos, R., Timperi, L., Lai, T., Farjo, N., et al. (2021). Dermal adipose tissue secretes HGF to promote human hair growth and pigmentation. J. Invest. Dermatol. 141, 1633–1645.e13. doi:10.1016/j.jid.2020.12.019
Niehrs, C. (2006). Function and biological roles of the Dickkopf family of Wnt modulators. Oncogene 25, 7469–7481. doi:10.1038/sj.onc.1210054
Olsen, J. J., Pohl, S. O., Deshmukh, A., Visweswaran, M., Ward, N. C., Arfuso, F., et al. (2017). The role of wnt signalling in angiogenesis. Clin. Biochem. Rev. 38, 131–142.
Ota, K., Quint, P., Ruan, M., Pederson, L., Westendorf, J. J., Khosla, S., et al. (2013). TGF-beta induces Wnt10b in osteoclasts from female mice to enhance coupling to osteoblasts. Endocrinology 154, 3745–3752. doi:10.1210/en.2013-1272
Ouji, Y., Ishizaka, S., and Yoshikawa, M. (2012). Dermal papilla cells serially cultured with Wnt-10b sustain their hair follicle induction activity after transplantation into nude mice. Cell Transpl. 21, 2313–2324. doi:10.3727/096368912X636867
Ouji, Y., Nakamura-Uchiyama, F., and Yoshikawa, M. (2013). Canonical Wnts, specifically Wnt-10b, show ability to maintain dermal papilla cells. Biochem. Biophys. Res. Commun. 438, 493–499. doi:10.1016/j.bbrc.2013.07.108
Owen-Woods, C., and Kusumbe, A. (2022). Fundamentals of bone vasculature: Specialization, interactions and functions. Semin. Cell Dev. Biol. 123, 36–47. doi:10.1016/j.semcdb.2021.06.025
Pachon-Pena, G., and Bredella, M. A. (2022). Bone marrow adipose tissue in metabolic health. Trends Endocrinol. Metab. 33, 401–408. doi:10.1016/j.tem.2022.03.003
Paik, D. T., Rai, M., Ryzhov, S., Sanders, L. N., Aisagbonhi, O., Funke, M. J., et al. (2015). Wnt10b gain-of-function improves cardiac repair by arteriole formation and attenuation of fibrosis. Circ. Res. 117, 804–816. doi:10.1161/CIRCRESAHA.115.306886
Palmieri, M., Almeida, M., Nookaew, I., Gomez-Acevedo, H., Joseph, T. E., Que, X., et al. (2021). Neutralization of oxidized phospholipids attenuates age-associated bone loss in mice. Aging Cell 20, e13442. doi:10.1111/acel.13442
Panteli, M., Vun, J. S. H., Pountos, I., Howard, A. J., Jones, E., and Giannoudis, P. V. (2022). Biological and molecular profile of fracture non-union tissue: A systematic review and an update on current insights. J. Cell Mol. Med. 26, 601–623. doi:10.1111/jcmm.17096
Park, Y. K., Park, B., Lee, S., Choi, K., Moon, Y., and Park, H. (2013). Hypoxia-inducible factor-2α-dependent hypoxic induction of Wnt10b expression in adipogenic cells. J. Biol. Chem. 288, 26311–26322. doi:10.1074/jbc.M113.500835
Peng, L., Liu, Z., Xiao, J., Tu, Y., Wan, Z., Xiong, H., et al. (2017). MicroRNA-148a suppresses epithelial-mesenchymal transition and invasion of pancreatic cancer cells by targeting Wnt10b and inhibiting the Wnt/β-catenin signaling pathway. Oncol. Rep. 38, 301–308. doi:10.3892/or.2017.5705
Perez-Castrillon, J. L., Olmos, J. M., Nan, D. N., Castillo, J., Arozamena, J., Montero, A., et al. (2009). Polymorphisms of the WNT10B gene, bone mineral density, and fractures in postmenopausal women. Calcif. Tissue Int. 85, 113–118. doi:10.1007/s00223-009-9256-4
Pfahler, S., and Distl, O. (2012). Identification of quantitative trait loci (QTL) for canine hip dysplasia and canine elbow dysplasia in Bernese mountain dogs. PloS one 7, e49782. doi:10.1371/journal.pone.0049782
Pourdashti, S., Faridi, N., Yaghooti, H., Jalali, M. T., Soroush, A., and Bathaie, S. Z. (2022). Possible role of WNT10B in increased proliferation and tubule formation of human umbilical vein endothelial cell cultures treated with hypoxic conditioned medium from human adipocytes. Biotech. Histochem 97, 168–179. doi:10.1080/10520295.2021.1923801
Qin, X., Jiang, Q., Miyazaki, T., and Komori, T. (2019). Runx2 regulates cranial suture closure by inducing hedgehog, Fgf, Wnt and Pthlh signaling pathway gene expressions in suture mesenchymal cells. Hum. Mol. Genet. 28, 896–911. doi:10.1093/hmg/ddy386
Qin, X., Jiang, Q., Komori, H., Sakane, C., Fukuyama, R., Matsuo, Y., et al. (2021). Runt-related transcription factor-2 (Runx2) is required for bone matrix protein gene expression in committed osteoblasts in mice. J. Bone Min. Res. 36, 2081–2095. doi:10.1002/jbmr.4386
Reddy, S., Andl, T., Bagasra, A., Lu, M. M., Epstein, D. J., Morrisey, E. E., et al. (2001). Characterization of Wnt gene expression in developing and postnatal hair follicles and identification of Wnt5a as a target of Sonic hedgehog in hair follicle morphogenesis. Mech. Dev. 107, 69–82. doi:10.1016/s0925-4773(01)00452-x
Rim, E. Y., Clevers, H., and Nusse, R. (2022). The wnt pathway: From signaling mechanisms to synthetic modulators. Annu. Rev. Biochem. 91, 571–598. doi:10.1146/annurev-biochem-040320-103615
Robinson, J. W., Li, J. Y., Walker, L. D., Tyagi, A. M., Reott, M. A., Yu, M., et al. (2015). T cell-expressed CD40L potentiates the bone anabolic activity of intermittent PTH treatment. J. Bone Mineral Res. Off. J. Am. Soc. Bone Mineral Res. 30, 695–705. doi:10.1002/jbmr.2394
Rong, X., Liu, J., Yao, X., Jiang, T., Wang, Y., and Xie, F. (2019). Human bone marrow mesenchymal stem cells-derived exosomes alleviate liver fibrosis through the Wnt/β-catenin pathway. Stem Cell Res. Ther. 10, 98. doi:10.1186/s13287-019-1204-2
Roo, J. J. D., and Staal, F. J. T. (2020). Cell signaling pathway reporters in adult hematopoietic stem cells. Cells 9, 2264. doi:10.3390/cells9102264
Roozen, P. P., Brugman, M. H., and Staal, F. J. (2012). Differential requirements for Wnt and Notch signaling in hematopoietic versus thymic niches. Ann. N. Y. Acad. Sci. 1266, 78–93. doi:10.1111/j.1749-6632.2012.06626.x
Roser-Page, S., Vikulina, T., Zayzafoon, M., and Weitzmann, M. N. (2014). CTLA-4Ig-induced T cell anergy promotes Wnt-10b production and bone formation in a mouse model. Arthritis Rheumatol. 66, 990–999. doi:10.1002/art.38319
Roser-Page, S., Vikulina, T., Weiss, D., Habib, M. M., Beck, G. R., Pacifici, R., et al. (2018). CTLA-4Ig (abatacept) balances bone anabolic effects of T cells and Wnt-10b with antianabolic effects of osteoblastic sclerostin. Ann. N. Y. Acad. Sci. 1415, 21–33. doi:10.1111/nyas.13643
Roser-Page, S., Weiss, D., Vikulina, T., Yu, M., Pacifici, R., and Weitzmann, M. N. (2022). Cyclic adenosine monophosphate (cAMP)-dependent phosphodiesterase inhibition promotes bone anabolism through CD8(+) T cell Wnt-10b production in mice. JBMR Plus 6, e10636. doi:10.1002/jbm4.10636
Ruan, G. T., Zhu, L. C., Gong, Y. Z., Liao, X. W., Wang, X. K., Liao, C., et al. (2020). The diagnosis and prognosis values of WNT mRNA expression in colon adenocarcinoma. J. Cell Biochem. 121, 3145–3161. doi:10.1002/jcb.29582
Ruohonen, S. T., Gaytan, F., Usseglio Gaudi, A., Velasco, I., Kukoricza, K., Perdices-Lopez, C., et al. (2022). Selective loss of kisspeptin signaling in oocytes causes progressive premature ovulatory failure. Hum. Reprod. 37, 806–821. doi:10.1093/humrep/deab287
Schatoff, E. M., Leach, B. I., and Dow, L. E. (2017). Wnt signaling and colorectal cancer. Curr. Colorectal Cancer Rep. 13, 101–110. doi:10.1007/s11888-017-0354-9
Schepper, J. D., Collins, F., Rios-Arce, N. D., Kang, H. J., Schaefer, L., Gardinier, J. D., et al. (2020). Involvement of the gut microbiota and barrier function in glucocorticoid-induced osteoporosis. J. Bone Min. Res. 35, 801–820. doi:10.1002/jbmr.3947
Shah, S. A., Kormpakis, I., Cavinatto, L., Killian, M. L., Thomopoulos, S., and Galatz, L. M. (2017). Rotator cuff muscle degeneration and tear severity related to myogenic, adipogenic, and atrophy genes in human muscle. J. Orthop. Res. 35, 2808–2814. doi:10.1002/jor.23593
Sharma, M., Castro-Piedras, I., Simmons, G. E., and Pruitt, K. (2018). Dishevelled: A masterful conductor of complex wnt signals. Cell Signal 47, 52–64. doi:10.1016/j.cellsig.2018.03.004
Shi, L., Xi, J., Xu, X., Peng, B., and Zhang, B. (2019). MiR-148a suppressed cell invasion and migration via targeting WNT10b and modulating beta-catenin signaling in cisplatin-resistant colorectal cancer cells. Biomed. Pharmacother. 109, 902–909. doi:10.1016/j.biopha.2018.10.080
Shin, S. H., Kim, W. J., Choi, M. J., Park, J. M., Jin, H. R., Yin, G. N., et al. (2014). Aberrant expression of Wnt family contributes to the pathogenesis of diabetes-induced erectile dysfunction. Andrology 2, 107–116. doi:10.1111/j.2047-2927.2013.00162.x
Smieszek, A., Giezek, E., Chrapiec, M., Murat, M., Mucha, A., Michalak, I., et al. (2017). The influence of Spirulina platensis filtrates on caco-2 proliferative activity and expression of apoptosis-related microRNAs and mRNA. Mar. Drugs 15, 65. doi:10.3390/md15030065
Sonderegger, S., Husslein, H., Leisser, C., and Knofler, M. (2007). Complex expression pattern of Wnt ligands and frizzled receptors in human placenta and its trophoblast subtypes. Placenta 28, S97–S102. doi:10.1016/j.placenta.2006.11.003,
Soret, B., Mendizabal, J. A., Arana, A., and Alfonso, L. (2016). Expression of genes involved in adipogenesis and lipid metabolism in subcutaneous adipose tissue and longissimus muscle in low-marbled Pirenaica beef cattle. Animal 10, 2018–2026. doi:10.1017/S175173111600118X
Stamos, J. L., and Weis, W. I. (2013). The beta-catenin destruction complex. Cold Spring Harb. Perspect. Biol. 5, a007898. doi:10.1101/cshperspect.a007898
Stevens, J. R., Miranda-Carboni, G. A., Singer, M. A., Brugger, S. M., Lyons, K. M., and Lane, T. F. (2010). Wnt10b deficiency results in age-dependent loss of bone mass and progressive reduction of mesenchymal progenitor cells. J. Bone Mineral Res. Off. J. Am. Soc. Bone Mineral Res. 25, 2138–2147. doi:10.1002/jbmr.118
Suffee, N., Moore-Morris, T., Farahmand, P., Rucker-Martin, C., Dilanian, G., Fradet, M., et al. (2017). Atrial natriuretic peptide regulates adipose tissue accumulation in adult atria. Proc. Natl. Acad. Sci. U. S. A. 114, E771–E780. doi:10.1073/pnas.1610968114
Suthon, S., Lin, J., Perkins, R. S., Crockarell, J. R., Miranda-Carboni, G. A., and Krum, S. A. (2022a). Estrogen receptor alpha and NFATc1 bind to a bone mineral density-associated SNP to repress WNT5B in osteoblasts. Am. J. Hum. Genet. 109, 97–115. doi:10.1016/j.ajhg.2021.11.018
Suthon, S., Perkins, R. S., Lin, J., Crockarell, J. R., Miranda-Carboni, G. A., and Krum, S. A. (2022b). GATA4 and estrogen receptor alpha bind at SNPs rs9921222 and rs10794639 to regulate AXIN1 expression in osteoblasts. Hum. Genet. 141, 1849–1861. doi:10.1007/s00439-022-02463-8
Takahashi, H., Ogoyama, M., Nagayama, S., Suzuki, H., Ohkuchi, A., Matsubara, S., et al. (2021). Extravillous trophoblast invasion accelerated by WNT3A, 5A, and 10B via CD44. J. Matern. Fetal Neonatal Med. 34, 3377–3385. doi:10.1080/14767058.2019.1684891
Tao, H., Mei, S., Sun, X., Peng, X., Zhang, X., Ma, C., et al. (2013). Associations of TCF12, CTNNAL1 and WNT10B gene polymorphisms with litter size in pigs. Anim. Reprod. Sci. 140, 189–194. doi:10.1016/j.anireprosci.2013.05.013
Tassew, N. G., Charish, J., Shabanzadeh, A. P., Luga, V., Harada, H., Farhani, N., et al. (2017). Exosomes mediate mobilization of autocrine Wnt10b to promote axonal regeneration in the injured CNS. Cell Rep. 20, 99–111. doi:10.1016/j.celrep.2017.06.009
Tazaki, Y., Sugitani, K., Ogai, K., Kobayashi, I., Kawasaki, H., Aoyama, T., et al. (2018). RANKL, Ephrin-Eph and Wnt10b are key intercellular communication molecules regulating bone remodeling in autologous transplanted goldfish scales. Comp. Biochem. physiology. Part A, Mol. Integr. Physiol. 225, 46–58. doi:10.1016/j.cbpa.2018.06.011
Terauchi, M., Li, J. Y., Bedi, B., Baek, K. H., Tawfeek, H., Galley, S., et al. (2009). T lymphocytes amplify the anabolic activity of parathyroid hormone through Wnt10b signaling. Cell Metab. 10, 229–240. doi:10.1016/j.cmet.2009.07.010
Trischler, J., Shiomi, T., Turner, D. L., Sklepkiewicz , P. L., Goldklang, M. P., Tanaka, K. F., et al. (2016). Immune modulation of the T cell response in asthma through Wnt10b. Am. J. Respir. Cell Mol. Biol. 54, 584–593. doi:10.1165/rcmb.2014-0425OC
Tyagi, A. M., Yu, M., Darby, T. M., Vaccaro, C., Li, J. Y., Owens, J. A., et al. (2018). The microbial metabolite butyrate stimulates bone formation via T regulatory cell-mediated regulation of WNT10B expression. Immunity 49, 1116–1131. doi:10.1016/j.immuni.2018.10.013
Ugur, S. A., and Tolun, A. (2008). Homozygous WNT10b mutation and complex inheritance in Split-Hand/Foot Malformation. Hum. Mol. Genet. 17, 2644–2653. doi:10.1093/hmg/ddn164
Ullah, A., Gul, A., Umair, M., IrfanullahAhmad, F., Aziz, A., Wali, A., et al. (2018). Homozygous sequence variants in the WNT10B gene underlie split hand/foot malformation. Genet. Mol. Biol. 41, 1–8. doi:10.1590/1678-4685-GMB-2016-0162
Van Camp, J. K., Beckers, S., Zegers, D., Verrijken, A., Van Gaal, L. F., and Van Hul, W. (2012). Genetic association between WNT10B polymorphisms and obesity in a Belgian case-control population is restricted to males. Mol. Genet. Metab. 105, 489–493. doi:10.1016/j.ymgme.2011.11.197
Van Camp, J. K., Beckers, S., Zegers, D., Boudin, E., Nielsen, T. L., Andersen, M., et al. (2013). Genetic association study of WNT10B polymorphisms with BMD and adiposity parameters in Danish and Belgian males. Endocrine 44, 247–254. doi:10.1007/s12020-012-9869-7
Veltmaat, J. M., Van Veelen, W., Thiery, J. P., and Bellusci, S. (2004). Identification of the mammary line in mouse by Wnt10b expression. Dev. Dyn. 229, 349–356. doi:10.1002/dvdy.10441
Vertino, A. M., Taylor-Jones, J. M., Longo, K. A., Bearden, E. D., Lane, T. F., McGehee, R. E., et al. (2005). Wnt10b deficiency promotes coexpression of myogenic and adipogenic programs in myoblasts. Mol. Biol. Cell 16, 2039–2048. doi:10.1091/mbc.e04-08-0720
Wang, J., and Shackleford, G. M. (1996). Murine Wnt10a and Wnt10b: Cloning and expression in developing limbs, face and skin of embryos and in adults. Oncogene 13, 1537–1544.
Wang, L. J., Lo, H. F., Lin, C. F., Ng, P. S., Wu, Y. H., Lee, Y. S., et al. (2019). SFRP3 negatively regulates placental extravillous trophoblast cell migration mediated by the GCM1-WNT10B-FZD7 axis. FASEB J. 33, 314–326. doi:10.1096/fj.201800124R
Wei, J., Melichian, D., Komura, K., Hinchcliff, M., Lam, A. P., Lafyatis, R., et al. (2011). Canonical wnt signaling induces skin fibrosis and subcutaneous lipoatrophy: A novel mouse model for scleroderma? Arthritis Rheum. 63, 1707–1717. doi:10.1002/art.30312
Wend, P., Wend, K., Krum, S. A., and Miranda-Carboni, G. A. (2012). The role of WNT10B in physiology and disease. Acta physiol. 204, 34–51. doi:10.1111/j.1748-1716.2011.02296.x
Wend, P., Runke, S., Wend, K., Anchondo, B., Yesayan, M., Jardon, M., et al. (2013). WNT10B/β-catenin signalling induces HMGA2 and proliferation in metastatic triple-negative breast cancer. EMBO Mol. Med. 5, 264–279. doi:10.1002/emmm.201201320
Williams, M., Zeng, Y., Chiquet, B., Jacob, H., Kurtis Kasper, F., Harrington, D. A., et al. (2021). Functional characterization of ATF1, GREM2 AND WNT10B variants associated with tooth agenesis. Orthod. Craniofac Res. 24, 486–493. doi:10.1111/ocr.12462
Wolf, M., Lossdorfer, S., Marciniak, J., Romer, P., Kirschneck, C., Craveiro, R., et al. (2016). CD8+ T cells mediate the regenerative PTH effect in hPDL cells via Wnt10b signaling. Innate Immun. 22, 674–681. doi:10.1177/1753425916669417
Wright, W. S., Longo, K. A., Dolinsky, V. W., Gerin, I., Kang, S., Bennett, C. N., et al. (2007). Wnt10b inhibits obesity in ob/ob and agouti mice. Diabetes 56, 295–303. doi:10.2337/db06-1339
Wu, G., Fan, X., and Sun, L. (2015). Silencing of Wnt10B reduces viability of heptocellular carcinoma HepG2 cells. Am. J. Cancer Res. 5, 1911–1920.
Wu, M., Wang, Y., Shao, J. Z., Wang, J., Chen, W., and Li, Y. P. (2017). Cbfβ governs osteoblast-adipocyte lineage commitment through enhancing β-catenin signaling and suppressing adipogenesis gene expression. Proc. Natl. Acad. Sci. U. S. A. 114, 10119–10124. doi:10.1073/pnas.1619294114
Wu, X. D., Bie, Q. L., Zhang, B., Yan, Z. H., and Han, Z. J. (2017). Wnt10B is critical for the progression of gastric cancer. Oncol. Lett. 13, 4231–4237. doi:10.3892/ol.2017.5992
Wu, H. Y., Bi, R., Sun, T., and Xie, F. (2019a). Deletion of Dicer blocks osteogenic differentiation via the inhibition of Wnt signalling. Mol. Med. Rep. 19, 2897–2905. doi:10.3892/mmr.2019.9941
Wu, P., Zhang, Y., Xing, Y., Xu, W., Guo, H., Deng, F., et al. (2019b). The balance of Bmp6 and Wnt10b regulates the telogen-anagen transition of hair follicles. Cell Commun. Signal 17, 16. doi:10.1186/s12964-019-0330-x
Wu, Z., Zhu, Y., Liu, H., Liu, G., and Li, F. (2020). Wnt10b promotes hair follicles growth and dermal papilla cells proliferation via Wnt/β-Catenin signaling pathway in Rex rabbits. Biosci. Rep. 40. doi:10.1042/BSR20191248
Xu, A., Yang, H., Gao, K., Zhan, Z., Song, Z., Huang, T., et al. (2020). Expression profiles and prognostic significance of WNT family members in glioma via bioinformatic analysis. Biosci. Rep. 40, BSR20194255. doi:10.1042/BSR20194255
Yang, Y., Fan, X., Nie, Y., Liu, D., Zhu, D., Wu, K., et al. (2021). CircTUBGCP3 facilitates the tumorigenesis of lung adenocarcinoma by sponging miR-885-3p. Cancer Cell Int. 21, 651. doi:10.1186/s12935-021-02356-2
Yang, Z., Wang, M., Ren, Y., Li, L., Cao, L., Zhang, W., et al. (2021). Inhibition of Wnt10b/β-catenin signaling alleviates pulmonary fibrogenesis induced by paraquat in vivo and in vitro. Life Sci. 286, 120027. doi:10.1016/j.lfs.2021.120027
Ye, J., Yang, T., Guo, H., Tang, Y., Deng, F., Li, Y., et al. (2013). Wnt10b promotes differentiation of mouse hair follicle melanocytes. Int. J. Med. Sci. 10, 691–698. doi:10.7150/ijms.6170
Yi, S. A., Nam, K. H., Kim, S., So, H. M., Ryoo, R., Han, J. W., et al. (2019). Vulpinic acid controls stem cell fate toward osteogenesis and adipogenesis. Genes (Basel) 11, 18. doi:10.3390/genes11010018
Yin, R. H., Zhao, S. J., Wang, Z. Y., Zhu, Y. B., Yin, R. L., Bai, M., et al. (2022). LncRNA-599547 contributes the inductive property of dermal papilla cells in cashmere goat through miR-15b-5p/Wnt10b axis. Anim. Biotechnol. 33, 493–507. doi:10.1080/10495398.2020.1806860
Yu, P., Yang, W., Han, D., Wang, X., Guo, S., Li, J., et al. (2016). Mutations in WNT10B are identified in individuals with oligodontia. Am. J. Hum. Genet. 99, 195–201. doi:10.1016/j.ajhg.2016.05.012
Zhang, J., Motyl, K. J., Irwin, R., MacDougald, O. A., Britton, R. A., and McCabe, L. R. (2015). Loss of bone and Wnt10b expression in male type 1 diabetic mice is blocked by the probiotic Lactobacillus reuteri. Endocrinology 156, 3169–3182. doi:10.1210/EN.2015-1308
Zhang, S., Zhang, F., Chen, Q., Wan, C., Xiong, J., and Xu, J. (2019). CRISPR/Cas9-mediated knockout of NSD1 suppresses the hepatocellular carcinoma development via the NSD1/H3/Wnt10b signaling pathway. J. Exp. Clin. Cancer Res. 38, 467. doi:10.1186/s13046-019-1462-y
Zhang, J., Li, P., Li, T., Zhou, Z., Wu, H., and Zhang, L. (2021a). Coronin 6 promotes hepatocellular carcinoma progression by enhancing canonical Wnt/beta-catenin signaling pathway. J. Cancer 12, 7465–7476. doi:10.7150/jca.62873
Zhang, S., Xu, J., Cao, H., Jiang, M., and Xiong, J. (2021b). KB-68A7.1 inhibits hepatocellular carcinoma development through binding to NSD1 and suppressing wnt/β-catenin signalling. Front. Oncol. 11, 808291. doi:10.3389/fonc.2021.808291
Zhang, S., Jiang, M., Cao, H., Xiong, J., and Xu, J. (2022). CTB-193M12.5 promotes hepatocellular carcinoma progression via enhancing NSD1-mediated WNT10B/Wnt/β-Catenin signaling activation. J. Hepatocell. Carcinoma 9, 553–569. doi:10.2147/JHC.S365302
Zhao, J., Zhang, C., Fang, X., Zhang, H., Liu, X., Li, J., et al. (2012). Polymorphisms of the bovine WNT10B gene and their associations with growth traits. Res. Vet. Sci. 93, 1301–1306. doi:10.1016/j.rvsc.2012.07.008
Zhao, C., Wu, H., Qimuge, N., Pang, W., Li, X., Chu, G., et al. (2018). MAT2A promotes porcine adipogenesis by mediating H3K27me3 at Wnt10b locus and repressing Wnt/β-catenin signaling. Biochim. Biophys. Acta Mol. Cell Biol. Lipids 1863, 132–142. doi:10.1016/j.bbalip.2017.11.001
Zheng, Y., Wang, C., Zhang, H., Shao, C., Gao, L. H., Li, S. S., et al. (2016). Polymorphisms in Wnt signaling pathway genes are associated with peak bone mineral density, lean mass, and fat mass in Chinese male nuclear families. Osteoporos. Int. 27, 1805–1815. doi:10.1007/s00198-015-3457-7
Zheng, C. M., Hsu, Y. H., Wu, C. C., Lu, C. L., Liu, W. C., Zheng, J. Q., et al. (2019). Osteoclast-released wnt-10b underlies Cinacalcet related bone improvement in chronic kidney disease. Int. J. Mol. Sci. 20, 2800. doi:10.3390/ijms20112800
Zhou, Y., Zhao, X. L., Guo, R. X., Qiao, Y. H., Zhang, X. Y., and Chen, Z. H. (2016). Flow cytometer analysis of cell apoptosis of endometrial carcinoma with Wnt10b. J. Biol. Regul. Homeost. Agents 30, 547–552.
Zhou, F., Lei, Y., Xu, X., Zhou, H., Liu, H., Jiang, J., et al. (2020). LINC00355:8 promotes cell proliferation and migration with invasion via the MiR-6777-3p/Wnt10b axis in Hepatocellular Carcinoma. J. Cancer 11, 5641–5655. doi:10.7150/jca.43831
Keywords: WNT10B, Wnt, Wnt signaling, bone, disease, cancer, development
Citation: Perkins RS, Singh R, Abell AN, Krum SA and Miranda-Carboni GA (2023) The role of WNT10B in physiology and disease: A 10-year update. Front. Cell Dev. Biol. 11:1120365. doi: 10.3389/fcell.2023.1120365
Received: 09 December 2022; Accepted: 16 January 2023;
Published: 06 February 2023.
Edited by:
Gunes Ozhan, Izmir Institute of Technology, TürkiyeReviewed by:
Anjali P. Kusumbe, University of Oxford, United KingdomUwe Lendeckel, University of Greifswald, Germany
Copyright © 2023 Perkins, Singh, Abell, Krum and Miranda-Carboni. This is an open-access article distributed under the terms of the Creative Commons Attribution License (CC BY). The use, distribution or reproduction in other forums is permitted, provided the original author(s) and the copyright owner(s) are credited and that the original publication in this journal is cited, in accordance with accepted academic practice. No use, distribution or reproduction is permitted which does not comply with these terms.
*Correspondence: Gustavo A. Miranda-Carboni, gmirand1@uthsc.edu
†These authors share senior authorship