- 1Research Center for Prevention of Oral and Dental Diseases, Baqiyatallah University of Medical Sciences, Tehran, Iran
- 2School of Dentistry, Tehran Branch, Islamic Azad University, Tehran, Iran
- 3Department of Oral and Maxillofacial Surgery, School of Dentistry, Shahid Beheshti University of Medical Sciences, Tehran, Iran
- 4Department of Prosthodontics, School of Dentistry, Shahid Beheshti University of Medical Sciences, Tehran, Iran
- 5Student Research Committee, Dentistry Research Center, Research Institute of Dental Sciences, Dental School, Shahid Behesti University of Medical Sciences, Tehran, Iran
- 6Student Research Committee, Qazvin University of Medical Sciences, Qazvin, Iran
- 7Department of Clinical Sciences, Faculty of Veterinary Medicine, Lorestan University, Khorramabad, Iran
- 8Department of Science and Research, Islimic Azade University, Tehran, Iran
Craniofacial deformities (CFDs) develop following oncological resection, trauma, or congenital disorders. Trauma is one of the top five causes of death globally, with rates varying from country to country. They result in a non-healing composite tissue wound as they degenerate in soft or hard tissues. Approximately one-third of oral diseases are caused by gum disease. Due to the complexity of anatomical structures in the region and the variety of tissue-specific requirements, CFD treatments present many challenges. Many treatment methods for CFDs are available today, such as drugs, regenerative medicine (RM), surgery, and tissue engineering. Functional restoration of a tissue or an organ after trauma or other chronic diseases is the focus of this emerging field of science. The materials and methodologies used in craniofacial reconstruction have significantly improved in the last few years. A facial fracture requires bone preservation as much as possible, so tiny fragments are removed initially. It is possible to replace bone marrow stem cells with oral stem cells for CFDs due to their excellent potential for bone formation. This review article discusses regenerative approaches for different types of craniofacial diseases.
1 Introduction
Traumatic or oncological resection or congenital abnormalities can cause craniofacial defects (CFDs). A non-healing composite tissue wound is caused by soft tissue deficits, bone loss, or both. Treatment of craniofacial defects is difficult because there are many different types of tissues and structures (Nyberg et al., 2017). Maxillofacial (MF) fractures result from many factors, such as crashes in motor fights, firearms, vehicles, wars, and sports (Herford, 2017; Castro-Espicalsky et al., 2020). Those with musculoskeletal dysfunctions usually suffer significant negative effects from CFDs (Martín-Del-Campo et al., 2019). Iran had to provide prolonged medical care to over 400 thousand patients due to the Iraq–Iran war. Official organizations authorized to assist war victims invited international reconstruction surgeons to Iran. The leader of this team was Paul Tessier, the founder of craniofacial surgery. During this project, patients with severe trauma injuries in the lower part of the face were provided with MF and oral rehabilitation using the most current techniques (Simon et al., 2015). There are differences in the incidence rates of trauma according to countries worldwide. Trauma is one of the top five factors contributing to death worldwide (Herford, 2017). United States children suffer from it more often than any other disease. Based on the National Trauma Databank collected, pediatric trauma cases most commonly involve the head, with the face being the fourth most common body part to be injured. Case fatality rates of 3.74% and 3.07% were reported for pediatric head and face injuries in 2016 (Braun et al., 2017). The burden of oral diseases is heavily influenced by periodontal disease (Latimer et al., 2021). According to the World Health Organization (WHO), the number of people affected by this high-impact disease grew by 6% from 2017 to 2018 (Global and regional, 2018). A child is likely to die from pediatric trauma and present to an ED every year; fractures occur in 8%–15% of cases of pediatric facial trauma after sustained force; over 11,000 children die each year from pediatric trauma, and over eight million children visit the ED due to pediatric trauma (Andrew et al., 2019; Rogan and Fang, 2021). There were 1,146 patients with facial fractures treated at the SDM craniofacial unit for 10 years, from January 2003 to December 2013; among them, 68 were young children (Ghosh et al., 2018). A total of 599 men and 735 women were involved in one study; 66% of patients had more than one comorbidity, and the mean age was 79.3 years. Most injuries were caused by falls, and the most commonly observed injuries were zygomatic fractures. The number of patients with concurrent injuries was 27.3% (Brucoli et al., 2020). There were 4,783 craniomaxillofacial (CMF) injuries among the 2,014 injured service members of the United States service members injured in conflicts with Iraq and Afghanistan, of which 26% had injuries to CMF regions (Lew et al., 2010). Between 2003 and 2011, all patients with facial defects and problems referred to a United States- or United Kingdom-based MF trauma that were alive were examined in the Joint Theatre Trauma Registries. In total, 16,944 casualties suffered facial injuries, and the most common problem was bone fractures and muscle/skin injuries. Inner/middle ear injuries and injuries to the teeth also occurred in large numbers (Breeze et al., 2019). During World Wars I and II, tantalum and acrylic cranioplasty were developed as a result of battlefield medicine during the Crimean War and the American Civil War. With more durable protective armor, improved medical evacuation, and early “far-forward” neurosurgical treatments available in modern conflicts such as Afghanistan and Iraq, cranial injuries are more likely to be survived (Bonfield et al., 2014; Saadi et al., 2018). Because tissues are tissue-specific and anatomically complex in that region, CFDs can present many challenges during treatment. Clinicians, scientists, and engineers can create personalized CFDs using 3D printing. These technologies can be used to restore the appearance and function of patients using three main strategies: reconstruction, rehabilitation, and regeneration (Nyberg et al., 2017). Hyaluronic acid (HA) is one of the human body components. In recent decades, HA in bone regeneration has gained widespread acceptance and is an increasingly popular topic in craniofacial surgery and dentistry. The demand for regenerative bone therapy has improved considerably during the past years, from maxilla augmentation to craniofacial bone fractures (Tahmasebi et al., 2020; Yazdanian et al., 2020; Zhai et al., 2020; Yazdanian et al., 2021a; Yazdanian et al., 2021b; Hakim et al., 2021; Soudi et al., 2021; Yazdanian et al., 2022). In addition, additive manufacturing (AM) refers to the process by which layers of materials are deposited in layers according to a design generated by computers. There has been a paradigm shift in manufacturing at the individual patient level with the advent of AM, which is a concept presented as a paradigm shift in the manufacturing of previously mass-produced biomaterials for both soft and hard tissue defects caused by congenital or acquired pathologies, periodontal disease, and MF trauma (Latimer et al., 2021). Besides this, MSCs can turn into multiple types of cells. Several dental tissues contain MSCs, including the periodontal ligament, the dental follicle, the dental pulp, the apical papilla, and the deciduous teeth. The future of regenerative medicine (RM) lies in tissue regeneration or developing new structures such as those found in craniofacial structures. This will enable the treatment of diseases such as congenital malformations and traumas (Maxim et al., 2015). This review article discusses the current approaches of regenerative therapies in craniofacial diseases.
2 Craniofacial and dental diseases
Craniofacial dystrophy (CFD) may be one of the underlying factors causing malocclusion. Craniofacial syndromes arise from two factors: cleft palates and craniosynostoses. Surgical techniques vary according to the particular issues to be addressed. Cranial synostosis is a congenital disease that occurs when one or more cranial sutures merge prematurely (Buchanan et al., 2014; Mew, 2014). A step-by-step morphological and cellular process governs the reciprocal epithelial–mesenchymal interactions that drive tooth growth (Balic, 2018). In addition to many other oral illnesses, pulpitis, periodontitis, dental caries, and CF trauma are the most frequent. Some people are born with CFDs or oral cancer. Dental problems are not a trivial matter, especially when there is a connection between oral and systemic illness. Patients will inevitably lose tissue due to most oral conditions, and the goal of dental therapy is to restore craniofacial function and tissue healing (Xu et al., 2021). Malocclusion and acquired craniofacial dysmorphology are caused by chronic oral dysfunction and orofacial myofunctional disorder. Getting long-term facial stability requires understanding the underlying causes of malocclusion, open bites, and hard palate collapses (D’Onofrio, 2019). The Bardet−Biedl syndrome (Visscher et al., 2016) is a congenital disease of the craniofacial area. This autosomal recessive non-motile ciliopathy is a monogenic disorder. Dental malformations are common in people with BBS because of aberrant jaw and teeth development during embryonic life. Dental care is complicated by genetically encoded intrinsic oral anatomical defects, resulting in orofacial comorbidities and diverse BBS clinical presentations. A broad range of collateral oral diseases may result from the comorbidities of the BBS phenotype, including diabetes, renal dysfunction, obesity, sleep apnea, cardiovascular dysfunction, and cognitive impairments (Panny et al., 2017).
2.1 Mandible and maxilla diseases
Despite its rarity, osteomyelitis of the maxilla may occur even in immunocompetent individuals due to the solid vascular supply of the maxilla. The fungus Rhizopus, Rhizomucor, and Cunninghamella of the Mucoraceae family are responsible for mycosis of the tongue, nose, and paranasal sinuses. In 1885, Paultauf was the first to identify mucormycosis as an illness in humans. Mucormycosis is an opportunistic fungal disease caused by saprophytic fungi (Phycomycetes) (Srivastava et al., 2019). Paresthesia of the naso-facial area, periorbital cellulitis, rhinorrhea, rhinorrhea with and without fever, nasal crusting, stuffiness and epistaxis, arthralgia, and weight loss are some of the early symptoms, followed by eschar formation and necrosis (Ferguson, 2000). Ablative surgery is necessary in most oral and paranasal sinus cancer cases, combined with adjuvant therapy. Reconstruction of significant post-ablative anomalies in the mandible and maxilla poses numerous challenges to the reconstructive surgeon. Functional and aesthetical restoration can only be achieved when doctors are intimately familiar with the underlying disease process, have a clear understanding of head and neck anatomy, and can select suitable tissues for each patient (Likhterov et al., 2019). Osteosarcomas of the jawbone are uncommon. Unlike long bone sarcomas, the patients with this condition are typically older, and metastasis rarely occurs, indicating a distinct pattern of activity compared to long bone sarcomas. According to ElKordy et al. (2018), 21 osteosarcoma cases were examined between 2006 and 2013. A total of 6 cases affected the maxilla, and 15 affected the mandible. The second instance of a periosteal response to solar radiation was observed. Four patients had improper biopsy results when the final pathology reports were reviewed for surgical resection. More commonly found among people in their late twenties, soft tissue sarcomas such as myxofibrosarcoma are called myxofibrosarcomas. It typically occurs in the subcutaneous soft tissue in the extremities, with a high likelihood of recurrence at the original site. MFS in the head and neck are rare and uncommon to have MFS of the maxilla and mandible (Li et al., 2020).
2.2 Cancer
In the United States, oral cancer ranks sixth in terms of incidence, and it is also the sixth most fatal. Most oral cancers are squamous cell carcinomas. An invasive biopsy, biochemical studies, and a comprehensive clinical examination can detect oral malignancies. Biomarkers, such as those found in blood, urine, and saliva, may help diagnose diseases early. Saliva offers a promising tool for early cancer diagnosis that is easy and non-invasive because it can be collected from a patient without invasive procedures. Besides proteins, peptides, electrolytes, organic and inorganic salts, and complementing contributions from gingival crevicular fluids and mucosal transudates, whole-mouth saliva contains many other biological elements (Khurshid et al., 2018). The risk of lip and oral cavity cancer has been linked to potentially malignant oral diseases (OPMDs) (Warnakulasuriya et al., 2021). Brain metastases that have spread to the breast induce receptor-mediated signaling cascades. In turn, the inward currents would be stimulated in the malignant cells. Subsequently, the tumor would grow. The brain-metastasizing cells also express neurotransmitter receptors and extend perisynaptic processes to receive neurotransmitter signals (Monje et al., 2020). Neurotransmitters and growth factors derived from peripheral nerve fibers modulate the course of gastric, pancreatic, oral, prostate, colon, and breast cancer similarly (Magnon et al., 2013; Hayakawa et al., 2017; Renz et al., 2018). Gliomas are deadly brain tumors caused by abnormal neuronal activity. Neuronal activity plays a crucial role in glioma progression. Growth factors released by the brain during glioma formation have an essential role. Venkatesh et al. (2019) found that synapses between neurons and gliomas depend on AMPA receptors for electrochemical communication. According to the findings, synaptic integration and electrical activity with neuronal circuits allow tumor growth. As demonstrated in diverse disease models and human tumors, Venkataramani et al. (2019) determined that neurons and gliomas can communicate directly in functioning chemical synapses between postsynaptic neurons and presynaptic gliomas. The findings suggest that neurons and glioma cells are directly synaptically connected, which may have therapeutic implications. Ameloblastoma is a potentially recurrent tumor that damages local tissue unless completely removed. Ameloblastomas are notoriously challenging to treat in the neck and head surgery field owing to the need for correct resection and restoration of the remaining defect, which can be functional and aesthetically pleasing. According to Adeel et al. (2018), between 1991 and 2009, patients with histologically proven ameloblastoma were found. The ameloblastoma was histologically proven in 15 individuals. Nine men and six women were in the group, with patients ages 20–60 years. One of our patient groups reported painless facial swelling as the main symptom. Thirteen of the patients had tumors in the mandible, and two had tumors in the maxilla.
2.3 Fibrous dysplasia
Fibrous dysplasia (FD) is a rare sporadic benign condition of bones in which the normal cancellous bone is replaced by fibro-osseous tissue and immature osteogenesis (Couturier et al., 2017). FTD affects both bone resorption and bone production. Adenylyl cyclase and the cyclic AMP signal pathway are activated by genetic mutations in the GNAS gene, which cause fibrous tissue and aberrant (woven) bone to form. There are several ways bone disease may be caused, including the presence of one or more bones (monostotic) or the presence of numerous bones (polyostotic). A common symptom of FD is extensive dysmorphic characteristics, bone deformity, and dental deformities in the craniofacial skeleton (Burke et al., 2017). In addition to aneurysmal bone cysts, cement-ossifying fibromas, cement-osseous dysplastic lesions, giant cell tumors, cement-ossifying fibromas, and simple bone cysts, benign fibro-osseous lesions (BFOL) of the jaws commonly occur. Other illnesses may exhibit the same symptoms; they must be distinguished. As a result of this condition, asymmetry in facial features and significant bone growth can occur. There have been reports associated with FD, especially in the orbits, of dystopia and involvement of the orbital canal. Hearing loss can result from injuries to the ossicles or external or internal auditory canals (Frisch et al., 2015). Couturier et al. (2017) followed up ten patients with craniofacial FD between 2010 and 2015. Headaches (n = 3), vestibular disorders (n = 1), and recurrent anterior uveitis (n = 1) were the most common complaints. Monostotic instances were all observed. Sphenoid was the most frequent bone implicated, followed by ethmoids, frontals, fronto-ethmoids, temporals, and fronto-ethmoids-sphenoids. A bisphosphonate, pamidronate, was administered intravenously to five patients: three improved within 6 months with no headaches or vestibular symptoms and two remained stable. Maxillary and mandibular alveolar bones can be damaged by craniofacial FD, resulting in occlusion problems. On a panoramic radiograph, the radiopacity may be lost due to FD of the maxillary bones (Couturier et al., 2017).
2.4 Human congenital disorders
Acrofacial dysostoses (Cho et al., 2016) and mandibulofacial dysostoses (MFD) relate to splicing factor gene mutations. Congenital malformations characterized by faulty pharyngeal development are MFDs, whereas limb deformities are known as AFDs (Merkuri and Fish, 2019). Cranial synostosis, a pathologic craniofacial condition, occurs when one or more cranial sutures (calvary) reattach. A healthy craniofacial structure relies on healthy sutures and unossified mesenchymal cells that form fibrous joint structures: convulsions, brain damage, intellectual disability, malformations, strabismus, and breathing and vision problems. Genetic and epigenetic changes, teratogens, and environmental factors contribute to craniosynostosis’s diverse etiology, making the syndrome extremely complex (Yilmaz et al., 2019). Osteogenesis heterotopic is a phenomenon of ectopic bone formation in soft tissues. An underlying genetic condition is less likely to trigger this condition than traumatic brain injury or surgery. Heterotopic ossification (Cohn Yakubovich et al., 2017) is a form of progressive osseous heteroplasia (POH). This form of abnormal bone growth is the opposite of FD (Ibarra and Atit, 2020). A mutation in the GNAS gene leads to progressive osseous heteroplasia, which is an autosomal dominant condition. The symptom is an ectopic intramembranous bone formation in subcutaneous and dermal tissues (Favus, 1996). In FD and POH, a somatic mutation in GNAS causes the condition in the early stages of development. Activating missense mutations develop in the somatic cells of a post-zygotic embryo (Feller et al., 2009).
2.5 Periodontal disease
Periodontal disease is an inflammatory condition surrounding the bone that causes irreversible damage to the tooth attachment. Smoking and hereditary vulnerability are variables that alter the host’s immunological response to microbial colonization of the periodontium, causing periodontal disease. The presence of periodontal diseases has been linked to the development of various underlying systemic illnesses (Han et al., 2014a). According to the traditional classification of periodontitis, this disorder has two kinds: aggressive and chronic forms. Moreover, periodontitis may be categorized as a chronic inflammatory disorder with multiple causes, a multicausal, complicated, chronic inflammatory condition (Loos and Van Dyke, 2020). There is a connection between periodontitis and various health problems, including cardiovascular diseases, in the general population (Hsieh et al., 2018). In addition to chronic periodontitis, the sixth most common human disease, severe periodontitis is considered one of the most frequent chronic diseases (Sanz et al., 2020). Periodontitis and gingivitis have affected patients’ quality of life, with significant adverse effects affecting function and appearance. Poor pregnancy outcomes, cardiovascular illnesses, type II diabetes mellitus (Renz et al., 2018), respiratory problems, and pneumonia in patients with hemodialysis have been linked to epidural periodontitis (Fischer et al., 2020). The global distribution of periodontal disease is a mystery, despite its strong relationship with systemic illnesses. Nazir et al. (2020) analyzed data on periodontal diseases in low-, middle-, and high-income nations, as well as adolescents and adults. The percentage of adolescents without periodontal disease was 21.2%, compared to 9.3% for adults and 9.7% for the elderly. Teenagers were more likely than adults and the elderly to experience bleeding, with 18.8% doing so after probing. A similar proportion of teenagers, adults, and elderly adults developed calculus.
2.6 Gingivitis
The most common form of gingivitis is plaque-induced inflammation, but other forms can significantly impact patients, including non-plaque-induced gingival disorders. There are many underlying causes of non-plaque-induced gingival lesions, but they can be pathological changes localized in the gingiva. There is a discussion on genetic disorders and developmental problems, inflammatory diseases with lesions, immunological diseases, endocrine diseases, metabolic diseases, traumatic lesions, and neoplasms (Holmstrup et al., 2018). An uncommon form of gingivitis, necrotizing ulcerative gingivitis, appears abruptly, painfully, and destructively (Dufty et al., 2017). Inflammation of the gingiva is a well-defined site-specific disease that has been extensively measured and is consistent with epidemiological studies showing its prevalence worldwide. The distinction between grading a gingival inflammatory disease at the site level and grading a gingivitis case should be evident, and a “gingivitis site” does not always imply gingivitis (Trombelli et al., 2018).
3 The current surgical therapy in craniofacial diseases
It is vital to clean and debride the wound entirely and remove all foreign materials before the wound can be closed. If any tissue cannot determine its viability, it should be left alone. In the following days, serial debridement should be conducted every 48 h. Corrections should then be attempted on the facial skeleton. External fixation is typically used when there is substantial bone loss with minor soft tissue injury. Internal fixation is usually used for severe bone loss with limited soft tissue injury. The issue of transplanting vascularized bone from the iliac crest or fibula to fix defects in the midface and jaw longer than 5 cm remains under discussion (Braun and Maricevich, 2017). The treatment of condylar fractures can be accomplished with preauricular, retromandibular, postauricular, or submandibular techniques. It is more often to use the preauricular and retromandibular techniques (Shakya et al., 2020). Yang et al. (2013) used a 3D simulation system to reduce the fractured portion of the mandible to its remaining segment and obtain preoperative reference data for screw size and placement. This preoperative reference was used during surgery. An assessment of the condyle using virtual reality before surgery reduces the operating time and helps achieve a better reduction and minimize operational errors. A computer-assisted preoperative simulation method called virtual surgical planning (VSP) was recently employed to treat mandibular condylar fractures (Shakya et al., 2020). Using VSP to determine the optimal screw length, position, angle, and hole size for fixing a fractured condylar section improved intraoperative efficiency (Boffano et al., 2017). A surgeon can assess and optimize various surgical alternatives by virtual assessment. Surgical reduction and stabilization are required to mend the shattered section. The correct number and placement of titanium plates and screws are required to hamper the functional stresses experienced during bone healing. The condylar neck and base are identified by two holes on either side of the fracture line on L-shaped, straight, or four-hole mini plates. In case of condylar head fracture, two screws are inserted through the lateral pole of the condyle, above the condylar neck, or below the lateral attachment of the capsule. Although titanium plates are durable and biocompatible, there remains the possibility of future failure, necessitating their removal; in this case, a re-entry procedure is needed (Kanno et al., 2018; Shakya et al., 2020). In addition to nerve and vascular damage, hearing loss, and CSF fistulas, TFB can cause serious complications. The treatments for TBF without CSF leak should be tailored individually; however, antibiotics should not be used as a preventive measure. Antibiotics should be prophylactically prescribed when there is a CSF leak, but this use is controversial. Carotid intertemporal artery damage is uncommon and involves packing the ear canal followed by carotid artery ligation or balloon occlusion of the carotid (Diaz et al., 2016). Approximately 17% of patients with TBF experience CSF leaks because meningitis is a possible consequence. A CSF fistula should be evaluated whenever fluid discharges from the ear canal or nose. Fistulas that persist for more than 7–10 days must be surgically repaired. The fistula’s location and whether brain herniation occurs through the tegmen determine the approach (Diaz et al., 2016). In treating fractures of the face, it is crucial to preserve bone, and only very little detached tissue needs to be removed first. Surgically constructed subcutaneous pouches can be used to preserve large fragments of avulsed bone for later recovery and reconstruction. A nylon suture should be placed around damaged nerves and salivary ducts (Rajguru, 2013). 3D printing can simplify reproducing a patient-specific prosthesis’s color, texture, stiffness, and form. Craniofacial prostheses can improve the appearance of the oral, nasal, and auricular areas. In addition to its use as a stopgap measure, prosthetic rehabilitation can also be used before surgical reconstruction. In most cases, CFDs are treated with prosthetics that replace the lost tissue and cover the underlying tissues. These devices are typically polydimethylsiloxane (PDMS), a flexible polymer (Nyberg et al., 2017). 3D modeling and manufacturing technologies can help in the individualized surgical reconstruction of complicated CFDs with improved tissue cutting according to preoperative plans, less time during surgery, and more cost-effective use of alloplastic and metal components (Nyberg et al., 2017). The safety and efficacy of stem cell populations was evaluated in patients with histories of craniofacial trauma or cleft palates. Eighteen individuals with cleft palates and missing teeth participated in a randomized clinical experiment in which they also had horizontal bone abnormalities. Patients could receive either autogenous block grafts or stem cell treatment. Before implant insertion, a 4-month healing interval was followed to assess the bone width for the treated locations. In summary, stem cells can be safely used for treating significant alveolar anomalies, but they cannot wholly replace large alveolar defects. Using this strategy requires more refinement to achieve the outcomes achieved with existing treatments for significant anomalies such as cleft palates (Bajestan et al., 2017). According to Kinsella et al. (2012), rat and human bone morphogenic protein-2 (rhBMP-2) is more effective than autografts in treating scarred calvarial wounds in rabbits. CT scans were performed on each animal after secondary reconstructive surgery at 0, 2, 4, and 6 weeks postoperatively. Six weeks was then allowed for all animals to be killed and histologically examined. We estimated the percentage of defects that corrected themselves over time and with therapy using a four-three mixed-model analysis of variance. A 3D-printed polymer scaffold and a signaling growth factor were used to treat a significant periodontal osseous defect in Rasperini et al. (2015). The treated area covered a 12-m distance following treatment. It has been suggested that 3D-printed image-based scaffolds may be used for periodontal reconstruction. Both materials and techniques have advanced considerably in craniofacial reconstruction during the past few years. The ideal material for these restorations is still autogenous tissue; however, the harvesting process is time-consuming and frequently results in donor site morbidity (Visscher et al., 2016). 3D-printed regeneration constructs are intended to replace deficient tissues with organic ones completely. Therefore, a construct consistently packed with cells capable of forming tissue that has also been activated for regeneration should be created. Two methods are possible: acellular printing scaffolds before implantation or printing live, cellular constructions, a technique known as “bioprinting” (Nyberg et al., 2017). Reconstructive surgery faces a whole new set of challenges in the case of abnormal CMF development due to trauma, cancer, or congenital deformities. Composite tissue allotransplantation (CTA) was developed due to recent advances in craniofacial surgery and immunotherapy (Susarla et al., 2011). Surgery, including the regeneration of alveolar bone, cement, and periodontal apparatus, is often used in surgical periodontal treatment to maximize bone volume and attachment (Wu et al., 2021). Some surgical therapies for craniofacial diseases are included in Table 1.
4 Regenerative medicine in craniofacial diseases
One of RM’s goals is to treat damaged tissues or organs. The complexity of the human body poses considerable challenges, even though considerable progress has been made lately (Atala and Forgacs, 2019). Our body’s tissues, organs, and organ systems are built on stem cells, which are at the center of the RM strategy. Four types of stem cells exist: omnipotent, multipotent, pluripotent, and totipotent. There is only one totipotent stem cell in humans, the Zygote, which forms the basis of an entire organism. According to the regenerative characteristics of stem cells, they are classified as embryonic stem cells (ESCs), tissue-specific progenitor stem cells (TSPSCs), mesenchymal stem cells (MSCs), umbilical cord stem cells (UCSCs), bone marrow stem cells (BMSCs), and induced pluripotent stem cells (iPSCs) (Bijukumar et al., 2018). The RM combines molecular and cellular bases, bioengineering, and material sciences to restore the function of the organ/tissue (Borrelli et al., 2020). Restoring the natural facial appearance with custom face prostheses is called prosthetic rehabilitation. Various plastic surgery procedures can be used to reconstruct the craniofacial region, including fixation devices, cutting guides, implanted medical equipment, and practice models. Regeneration creates a new craniofacial tissue through stem cells and biologically active scaffolds (Nyberg et al., 2017). One of the most challenging surgeries is the secondary reconstruction of facial abnormalities following posttraumatic injury. Primary reconstruction may not always be possible unless the comminution or surgical treatment is severe (Aman et al., 2019). Several surgeons are choosing internal fixation and open reduction for repairing condylar fractures due to new technologies, improved fracture management, and evidence of superior results in the literature. Due to the difficulty of surgically treating such fractures, many factors must be considered to ensure a satisfactory outcome (Shakya et al., 2020).
4.1 Stem cell therapy in craniofacial diseases
Oral stem cells can effectively replace BMSCs with high bone production potential. Stem cells from apical papilla (SCAP) and stem cells from human exfoliated deciduous teeth (Jahanbin et al., 2016) require human testing in clinical trials before their use (Sybil et al., 2020). The risk of facial paralysis following a TBF is approximately 6%–7%; 25% experience immediate total paralysis, whereas 75% experience partial or incomplete paralysis. When the facial nerve has been injured, patients with an acute-onset, complete facial paralysis should be assessed between 3 and 7 days later, allowing time for Wallerian degeneration. Depending on the severity of the damage, clinical assessment could be performed using facial nerve stimulation using the Hilger stimulator, electromyography (EMG), or electroneurophysiology (ENOG). Patients with refractory traumatic facial nerve paralysis can use neurons and MSCs. Cell-based Schwann cell synthesis in vitro seems possible in vitro, especially when paired with material bridges, as animal studies have investigated neural and ADSCs (Diaz et al., 2016). Research is currently being conducted to use autologous stem cells to treat CFDs. Patel et al. (2013) reported that CSPCs can create elastic and long-lasting tissue regeneration. It is a connective tissue that makes up the pulp of the tooth. This tissue is made up of different kinds of cells, and it contains walls that protect the integrity of the tissue. In 2000, Griffos et al. could isolate some stem cells from impacted third molars as a new source of stem cells (Maxim et al., 2015; Estrela et al., 2011). Cells known as DPSCs continually proliferate and differentiate in their culture medium, depending on what medium they have been placed in. In some animal models, the ability of these cells to proliferate and differentiate into odontoblast-like cells, bone-like tissue, and pulp-like tissue shows their potential for use in cell-based therapies (Han et al., 2014b). Another resource is the apical papilla. SCAPs produce odontoblast-like cells in vivo and are ideal for regenerating odontogenic tissue in vitro, producing dentin in vivo, and developing roots (Hemmat et al., 2010).
Using periodontal stem cells in patients with periodontal deficiencies has been proven safe without immunological or inflammatory adverse effects. Consequently, successful periodontal tissue regeneration requires the recruitment of locally produced progenitor cells, resulting in differentiation into bone-forming cells, cementum, and PDL (Liu et al., 2019). Wang et al. (2016a) created a technique for injecting stem cells into the body using encapsulated MSC derived from iPSC. The microbeads remained viable even after injection. The microbeads released cells with a 10-fold increase in live cell density from 1 to 14-day. In the cells, osteogenic markers were upregulated along with mineral deposition. Therefore, they concluded that CPC-microbead-iPSMSC might benefit bone regeneration in orthopedics, dentistry, and craniofacial applications. When polymers break down, they create porosity, which can improve the degradation of weakly degradable injectable CPCs. The long-term biological performance of CPC-PLGA has not been determined, although it is biodegradable. Injectability might be improved by adding carboxymethyl cellulose (CMC). The in vivo results of a long-term study on CPC-PLGA without/with the lubricant CMC were compared with those of a devitalized bovine bone mineral predicate device (DBBM), Bio-Oss®. A 26-week study found that CPC-PLGA was helpful in bone regeneration, with >40% of new bone growing (Grosfeld et al., 2016). As part of their study on the feasibility of regenerating periodontal abnormalities with allogeneic BMMSCs in rats with periodontitis, Du et al. (2014) used rat models of periodontitis. Invasions of BMMSCs were performed on rats extracted and mixed with a 0.9% sodium chloride solution. Those in the control groups received NaCl solution at 0.9% or no treatment. According to the study, local injections of BMMSCs may act as an anti-inflammatory and immune modulator in periodontitis-related abnormalities treatment. For peri-implantitis defect regeneration, Park et al. (2015) used canine periodontal ligament stem cells (PDLSCs) in vivo in a dog. The PDLSCs of dogs were transduced using adenoviral vectors expressing BMP2. Six beagle dogs with peri-implantitis caused by ligature implantation were treated with HA particles and collagen gel containing autologous PDLSCs or BMP2/PDLSCs. The drug was delivered through PDLSCs to promote re-osseointegration and new bone formation. There is a schematic of palatal regeneration using stem cells in Figure 1.
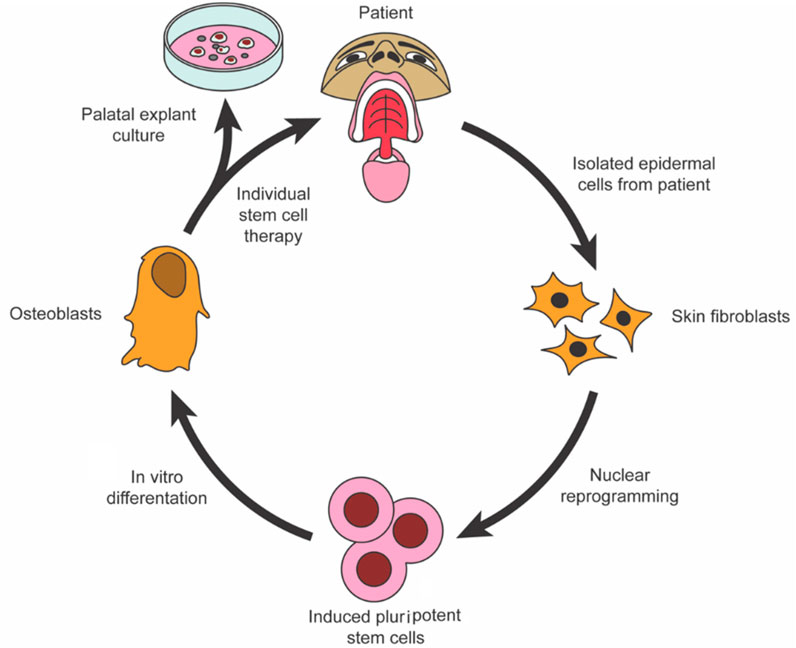
FIGURE 1. To restore palatal bone, iPSCs were isolated and programmed (Oliver et al., 2020).
4.2 Growth factor therapy in craniofacial diseases
In addition to vascularizing the bone and providing osteoprogenitor cells, the periosteum also contributes to bone growth and repair. Using PRP/BMSC gel membranes for regenerative bone repair, El Backly et al. (2013) presented a periosteal replacement wrapped around an osteoconductive scaffold. By adjusting the composition of PRP/BMSC gel membranes, it controlled the release of platelet-derived growth factor-β (PDGF-β) and VEGF. Lastly, in a mouse model, the PRP/BMSC gel membrane periosteal substitute was evaluated in vivo for its ability to biomimic a periosteal response enhancing bone regeneration. Yamada et al. (2013) also examined whether injectable regenerative bone pills containing PRP and stem cells could help alveolar deficits repair the functional bone. With no negative side effects, all patients experienced a significant increase in bone volume. At 3 months, newly generated bone regions reached levels comparable to natural bone, a significant improvement over the preoperative level. A significant amount of bone resorption did not occur during a long-term follow-up. Patients with masticatory impairments were helped by injectable tissue-engineered bone. Using bone tissue engineering for treating patients is a unique but effective treatment method. How each signaling pathway affects the overall shape of the anterior zebrafish neurocranium was determined, as well as how it affects the formation of the ethmoid plate in Cusack et al. (2017). Researchers determined that different signaling routes affect the ethmoid plates’ shape, size, and shape in dose- and time-dependent ways. Shen et al. (2021) hypothesized that VEGF or nerve growth factor (NGF) therapy during SCAP development might alter the expression of the osteogenic and endogenic markers. Using VEGF/NGF in regenerative endodontic procedures can reveal dentinogenic/neuronal/healing markers in SCAPs. Recent studies on growth factors and stem cell therapy in craniofacial and dental trauma and disease are summarized in Table 2.
4.3 Current biomaterial therapy in craniofacial diseases
Clinically effective bone repair has been enabled by developing biomaterials that are viable alternatives to autologous and allogeneic grafting methods. A biocompatible scaffold is often incorporated into surgery to induce new bone formation by allowing cells to migrate, multiply, and differentiate. Numerous biomaterials have been used in craniofacial bone augmentation. Inorganic and organic materials are often separated. CaP bioceramics are a common type of inorganic scaffold, whereas organic scaffolds are made from natural or synthetic polymers (Thrivikraman et al., 2017). The purpose of biomaterials is to replace parts of living systems or provide similar functions. Optimal mechanical strength, osteoconductivity, and cell adhesion and proliferation are essential characteristics in biomaterials that serve as tissues (Tevlin et al., 2014). Congenital disorders,trauma, and bone recessions are craniofacial bone abnormalities. Tissue engineering is entered to help bone regeneration, allowing patients to benefit from the supporting effects of 3D materials combined with the synergistic effects of osteoinductive chemicals and recruited stem cells (Martín-Del-Campo et al., 2019). Figure 2 presents an overview of the polymeric scaffold characteristics and categorization.
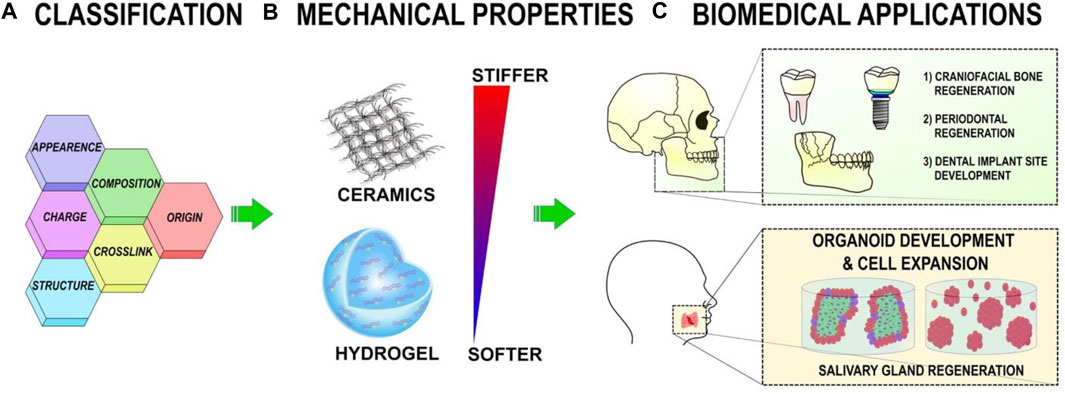
FIGURE 2. (A) Polymeric scaffolds can be categorized based on their content, structure, appearance, origin, cross-linking, and charge. (B) The mechanical properties of polymeric scaffolds can be altered to affect cellular activity. (C) Polymeric scaffolds help regenerate the oral, dental, and craniofacial regions in tissue engineering (Wu et al., 2021).
4.3.1 Bioactive materials therapy in craniofacial diseases
Providing bioactive polypeptide growth factors directly to the root surface can promote periodontal regeneration. This promotes wound healing, which results in new cementum and connective tissues. PDGF and IGF-I, for example, are effective in regenerating periodontal tissues in animals such as beagle dogs and monkeys (Han et al., 2014a). A study on n-HA/PA composite scaffolds with BMP-7 transduced MSCs in mandibular defect healing has been reported by Li et al. (2010). Radiographic, biomechanical, histomorphometric, and histological analyses of their mandibles were conducted. Group A animals developed more bone and mineralized than group B animals 4 and 8 weeks after implantation. Group B animals had more than group C. However, no differences were found at 16 weeks after implantation. Researchers found that MSCs-n-HA/PA composites transduced with BMP-7 dramatically accelerated bone formation. Using n-HA/PA scaffolds in combination with BMP-7-mediated ex vivo gene transfer may be another way to treat mandibular abnormalities (Li et al., 2010). Biomimetic synthesis can be used to restore and prevent damaged enamel. In recent years, it has been proven that chitosan–amelogenin (CS-AMEL) and calcium phosphate (CP) can be used to form an enamel-like layer that adheres to natural teeth surfaces seamlessly (Mukherjee et al., 2016). Prajapati et al. (2016) identified a unique function of the matrix metalloproteinase-20 (MMP-20) to prevent the entrapment of undesired organic materials in developing enamel crystals by cleaving precise amelogenin. Hydroxyapatite crystal crystallizes when MMP-20 regulates their growth morphology. In another study, MMP-20 was used to produce artificial enamel through a biomimetic process. Compared to the original enamel, both modulus and hardness were significantly enhanced by MMP-20CS-AMEL hydrogel. The findings of this study are significantly closer to achieving biomimetic enamel renewal, even though they require further study to include other enamel matrix proteins (Prajapati et al., 2018). Using hydroxyapatite, calcium sulfate hemihydrate, and Haladen collagenase (HAP/CS/HA-Col), Subramaniam et al. (2016) developed an alveolar bone replacement using hydroxyapatite, calcium sulfate, and Haladen collagenase. A WST-1 assay and mechanical testing evaluated the composite material’s biocompatibility. By using micro-CT images and histological testing, HAP/CS/HA-Col composites were validated by in vivo bone production in rats with alveolar bone deficiencies. Results showed that collagenase-infused HAP/CS/HA composites can effectively regenerate alveolar bone and that the same method may be applied to other tissues damaged by injury or disease. Extensive bone grafting remains a sensitive procedure due to the long and challenging integration of the grafted material into the physiological architecture. According to Simonpieri et al. (2009), platelet concentrates may speed up the integration process by speeding up the healing of mucosa and bone. Additionally, PRF membranes are functional during challenging implant rehabilitations, promoting periosteum healing and maturation. An extensive healing process on a PRF membrane layer is connected to the thick peri-implant gingiva, which may be responsible for the reduced marginal bone loss. Microthreaded collars and platform switching improved this result even more. A novel approach for improving the ultimate aesthetic outcome involves multiple healing on PRF membranes.
4.3.2 Polymer, graft, and composite therapy in craniofacial diseases
These scaffolds are highly promising for tissue regeneration because of their many advantages. An array of biomaterials has been proposed for injectable scaffolds. A biomaterial may be classified according to its natural or synthetic source. In addition to being produced from natural resources, natural biomaterials exhibit biological recognition, which may aid in the adhesion and development of cells. They usually do not cause inflammatory or immunological reactions, and they are biocompatible and biodegradable. Conversely, natural materials present potential health risks, such as introducing diseases from batch to batch. Synthetic biomaterials are created as alternatives to natively generated ones to address these issues. Massive-scale production of biomaterials with well-controlled properties can be implemented. Synthetic biomaterials do not transmit pathogens (Chang et al., 2017). Among these biomaterials are polymers. A polymer is a chemical compound with long chains of atoms linked together by covalent bonds. Collagen, for example, can be synthesized or can be made naturally (Koons et al., 2020). The temporomandibular joint (TMJ) tissues can be repaired using tissue engineering techniques that use biodegradable polymeric scaffolds. These sponges may provide a platform for cell infiltration and remodeling. These sponges include synthetic polymer poly(glycerol sebacate) scaffolds and natural gelatin scaffolds. The authors examined the regeneration capability of these scaffolds combined with magnesium (Mg) (MCC) using a new fibrocartilage defect model in goat mandibular condylar cartilage. An osteochondral lesion was produced bilaterally in a mandibular condyle in adult Spanish Boer goats. A 1 mm diameter drill hole was made on the articular surface to create a trough defect. Tests included an empty control without an implant, a PGS matrix with magnesium ions, a gelatin matrix with magnesium ions, and a gelatin matrix with magnesium ions and trimagnesium phosphate (TMP) powder. A 3-month recuperation period was given to the goats before tissue samples were taken (Chin et al., 2018). In craniofacial microsomia, the skull and face are asymmetrically developed before birth. Developing resorbable scaffolds is an alternative to help restore muscle function and improve symmetry in the face while reducing risks to the donor site. To create two resorbable weft-knitted scaffolds (NPRs), Deshpande et al. (2020) used Poly (ε-caprolactone) multifilament yarns with auxetic architecture that had a negative Poisson’s ratio. Two knitted textile scaffolds (called PCL A and PCL B) utilize multifilament yarns made from poly(-caprolactone). Both samples reached porosities of more than 90% using an open-weft knit construction. Although PCL A and PCL B did not unravel, PCL A had better dimensional stability than PCL B. Following 7 days in cell culture, PCL fabric A promoted cell development more effectively than PCL B (Deshpande et al., 2020). Schleund et al. (2019) demonstrated a novel method to repair extensive mandibular discontinuities with autologous iliac bone marrow allograft seeded with radial forearm flap vascularized with autologous iliac bone marrow aspirate. In addition to the iliac crest’s low morbidity rate, hematopoietic stem cells and MSCs may develop into osteoblasts when seeded on an allograft scaffold. BTE is now often achieved using bioceramics, but bone remains the ideal substrate for engineering bone, and only allografts prevent donor-site morbidity. In BTE, one of the most challenging aspects is vascularization, which can be achieved, for example, by using pedicles or free flaps. The radial forearm flap was chosen considering the length of the pedicle and the authors’ need for a little soft tissue (Schlund et al., 2019). Zaky et al. (2017) examined the mechanical properties of poly(glycerol sebacate) (PGS), compatibility with osteoprogenitor cells, and application to a rabbit ulna considerable defect regeneration in vitro and in vivo. Polylactic acid/glycolic acid polymeric scaffolds were compared in vitro to a stiffer PGS with similar porosity and interconnectivity. According to Zaky’s research, because PGS is more closely related to osteoid tissue than mineralized bone, it promotes skeletal maturation by allowing osteoprogenitor/stem cells to differentiate on a substrate that is mechanically closer to how mature bone forms (Zaky et al., 2017). Oncologic head and neck reconstruction using autologous fat grafting was evaluated by Vitagliano et al. (2018) for surgical, oncological, and aesthetic outcomes. Based on their analysis of the data from this study, they discovered that autologous fat grafting might complement or replace reconstructive flaps in oncological head and neck reconstruction, with fewer complications and no evidence of cancer recurrence (Vitagliano et al., 2016).
4.3.3 Collagen therapy in craniofacial diseases
Natural biomaterials, such as collagen, are widely used in injectable form. Dentin, bone, and PDL are the leading organic components of many mammals (Chang et al., 2017). Due to the complex and dynamic features of native/natural tissues, RM strategies aimed at retreating articular cartilage have a considerable challenge ahead. Parmar et al. (2015) modified streptococcal collagen-like two proteins with chondroitin sulfate (CS)-binding peptides, then cross-linked with an MMP7-responsive peptide to form biodegradable hydrogels. It was discovered that this biomaterial has the potential to influence cellular processes and build viable tissue-engineered structures for RM. Injuries to the skull and face may be treated with these materials. Primary cilia are needed for bone formation via intraflagellar transfer (IFT). According to Yamaguchi et al. (2020), IFT20, the minor IFT protein in the IFT-B complex, plays a crucial role in collagen synthesis in mice.
The deletion of Ift20 in craniofacial osteoblasts caused facial bone defects. By deleting Ift20, collagen protein levels remain unchanged, but collagen cross-linking is severely altered. Research indicated that IFT20 regulates telopeptidyl lysine hydroxylation and cross-linking in bone, a critical step in collagen synthesis. The success of regenerating tissues and bones in the oral and craniofacial regions can be attributed to biodegradable synthetic scaffolds. Qian et al. (2019) created an electrospun poly-lactic-co-glycolic, acid/polycaprolactone, and silver-coated collagen scaffold (PP-pDA-Ag-COL) to improve osteogenic and antibacterial features. A PP-pDA-Ag-COL scaffold inhibited the pathogenesis of mouse periodontal disease (31.8%) and promoted alveolar bone regeneration. They have demonstrated improved biocompatibility and osteogenic and antibacterial properties of our unique PP-pDA-Ag-COL scaffold for alveolar/craniofacial complex tissue reconstruction. Using collagen scaffolds and human ASDCs for oral mucosal and calvarial bone regeneration, Wang et al. (2018) utilized resveratrol (RSV), affecting MSC differentiation. A collagen scaffold containing RSV (/RSV) scaffold was assessed in vivo and in vitro for wound healing and bone reconstruction. On a collagen scaffold, RSV induced a more significant differentiation of hASC, indicating that scaffolds containing collagen and RSV can stimulate the growth of craniofacial tissue (Wang et al., 2018a). A group of mineralized collagen scaffolds was developed for bones, and complex tissue reconstruction using CMF was altered with zinc ions in Tiffany et al.’s (2019) study. Zinc sulfate is added to mineralized collagen-glycosaminoglycan precursor suspensions and freeze-dried to form porous biomaterials that are zinc functionalized. In addition to promoting zinc transporter expression, zinc functionalized scaffolds promote mineral biosynthesis, osteogenic differentiation, and stem cell viability.
4.3.4 Gelatin therapy in craniofacial diseases
Restoring function to periodontal tissues includes root bio-modification, guided tissue regeneration, bone replacement grafting, soft tissue grafting, and a combination of all procedures (Nyamsuren et al., 2018). A variety of bone transplantations have been tested for their ability to induce new bone growth. A list includes alloplastic materials (generally synthetic fillers), autografts (same individual tissue transferred from one site to another), allografts (same species tissue transferred to another), and xenografts (same species tissue transferred to other species) (Han et al., 2014a). It would be ideal to regenerate periodontal attachments, cementum, and alveolar bone as part of the optimal repair strategy for periodontal tissue defects. Researchers developed a tissue-engineered “sandwich” complex (a tissue-engineered membrane sandwiched between two mineralized tissues) to heal periodontal defects. Beagles’ premolar areas were treated using periodontal membranes. The sandwich tissue-engineered complex effectively healed periodontal abnormalities 10 days after surgery. Optimal periodontal repair may be achieved with tissue-engineered sandwich complexes, showing a mandibular defect treatment in a dog model (Wu et al., 2018). Octa CaP (OCaP) and collagen composites (OCaP-collagen) have boosted bone healing after cystectomy in humans. A canine or mouse model with a critical-size lesion benefited from OCaP-collagen-mediated bone regeneration (Kawai et al., 2017). Kawai et al. (2017) reported that OCaP-collagen was successfully used to restore a human mandibular bone defect, suggesting that it might be a viable bone replacement material in patients with significant bone defects that do not heal spontaneously. Schweikle et al. (2018) demonstrated that pH could efficiently regulate gelation time without affecting the flexibility of hydrogels. Hydrogels made of hybrid poly(ethylene glycol)-co-peptides are flexible platforms for bone regeneration. In situ gelation can be enhanced by hybrid PEG-peptide hydrogels. The increased structural characteristics of maleimide functionalized hydrogels and their enhanced tolerance to low pH makes them a preferred solution for injectable applications (Schweikle et al., 2018). Du and his colleagues (2015) coated nano-hydroxyapatite/coralline (nHA/coral) blocks with recombinant human VEGF by physical adsorption. Following the random implanting of VEGF/nHA/coral and nHA/coral blocks into a mandibular defect model, histomorphometric, immunohistochemical, and histological studies were performed to evaluate the healing process. nHA/coral blocks are suitable scaffolds for block grafting in critical-size mandibular defects, and angiogenesis can be enhanced by additional VEGF coating that can act through physical adsorption in the early stages of bone healing that present them as valuable biomaterials for bone healing (Du et al., 2015). It was the first time that gold nanoparticles were incorporated into a CPC (GNP-CPC), and the potential for osteogenesis was measured with human DPSCs. Furthermore, GNP-CPC significantly improved the osteogenic activities of hDPSCs. Through nanotopography and a bioactive addition, GNPs can enhance bone regeneration (Xia et al., 2018). Chitosan and glycerol phosphate composite (CS/-GP) was used by Cui et al. (2014) during bone regeneration with a thermosensitive transition in the sol-gel phase. Initially, ST2 stromal cells adhered to the CS/GP composite membrane better than CS alone. A significant difference between CS and GP composite membranes was observed in cell proliferation and osteoblast differentiation (p 0.05). CS/GP composite membrane implanted during surgery resulted in a mild inflammatory reaction, with no foreign body reaction lasting 4 weeks after surgery. Finally, the CS/-GP composite membrane was bioactive properties for bone tissue regeneration in vivo and in vitro.
4.3.5 Chitosan therapy in craniofacial diseases
Chitosan (CH) is a deacetylated derivative of chitin, consisting of N-acetylglucosamine and glucosamine. The polysaccharidechitosan has free amino and hydroxyl groups, and can incorporated with other bioactive compounds. Because of its biocompatibility, biodegradability, low toxicity, and controlled dissolution by enzymes, chitosan is commonly used in tissue engineering as a biomaterial (Chang et al., 2017). According to Raisi et al. (2010), chitosan conduits improve peripheral nerve regeneration in rats suffering sciatic nerve transections. According to the researchers, the chitosan conduit might be used in clinical settings for peripheral nerve regeneration because it is easy to access, inexpensive, simple to produce, and biodegradable. Furthermore, it does not contain any foreign ingredients that may cause an allergic reaction in the human body. Therefore, this scaffold shows promising results for the sciatic nerve and craniofacial injuries, which might cause facial nerve damage. Using experimental injectable chitosan hydrogel, Moreira et al. (2021) expected that PBMT would not interfere with the distribution of endogenous stem cells in the dental pulp to result in a suitable reconstruction. Researchers used chitosan hydrogel in combination with a PBMT and blood clot and discovered that dental pulp regeneration results could be improved. Tsao et al. (2011) developed a new polyelectrolyte hydrogel composed of chitosan-PGA, which can be used as a wound dressing. Chang et al. (2014) used this scaffold to stimulate the healing of the alveolar socket after tooth extraction. Each rat’s extraction socket was examined at 1, 2, 4, and 6 weeks following extraction. Radiography and histology indicate that C-PGA-treated extraction sockets grew lamellar bone (6.5%) 2 weeks following extraction. Furthermore, they conclude that C-PGA holds great promise in promoting new alveolar bone growth. Posnick and Sami (2015) examined the results of 50 patients treated with interposition grafting and Le Fort I in a 3-year timeline. It was necessary to use an interpositional graft for maxillary repositioning because horizontal advances and vertical and transverse changes had to be made. All the implants were made of corticocancellous (iliac) bone. The analysis of each patient’s maxillary vector change points was done using an analytical model. According to the current study, iliac corticocancellous allografts have few systemic problems and are used to replace complex 3D bone defects resulting from Le Fort I osteotomies or relocations. A person’s overall health needs to include identifying and treating extra-skeletal conditions. Medical treatment is usually available for endocrinopathies. Females suffering from precocious puberty may benefit from the usage of Letrozole, an inhibitor of the aromatase enzyme. An aromatase inhibitor and an antagonist of testosterone receptors are usually needed by men instead. Analogs of somatostatin, such as pegvisomant, an antagonist of growth hormone receptors, benefit patients with excess growth hormone (Feuillan et al., 2007; Boyce et al., 2012; Salenave et al., 2014). At present, no medicines have been proven to be effective for FD. Craniofacial fractures are still treated most effectively with surgery. Sixty-eight percent of procedures result in regrowth, especially when growth hormone excess is not treated, so an endocrinology evaluation and treatment can be recommended before surgery (Boyce et al., 2016). Bisphosphonates are often prescribed to patients with craniofacial FD who experience discomfort (Burke et al., 2017).
4.3.6 Drug therapy in craniofacial diseases
Zebrafish have proven to be an excellent model organism for in vivo drug testing. Zebrafish are well-known for their genetic tractability, significant fertility, external embryonic development, large clutch size, and visual transparency as vertebrate models (although newly discovered compensatory mechanisms and gene redundancy suggest zebrafish are redundant as genetic models). The ability of zebrafish embryos to diffuse oxygen is a critical feature for studying the development of drugs in vivo since drug treatments that alter cardiovascular system formation are likely to influence embryo survival (Wiley et al., 2017; Seda et al., 2019). Orofacial clefts (OFC) have been linked to valproic acid (VPA), an anti-epileptic medicine. Gebuijs et al. (2020) investigated how VPA affects bone and cartilage development in zebrafish larval heads in the early development stage (1–13 h after fertilization) and the late development stage (25–37 h after fertilization) when cranial neural crest cells (CNCCs) start arising, increasing, and differentiating. Despite the bone and cartilage absence, the body compensates by forming other tissues. A central mechanism of VPA causing craniofacial deformities (CDFs) seems to be the disruption of CNCC activity, leading to abnormal cartilage and bone growth. Furthermore, the army has learned to treat acute hearing loss as early as possible on the battlefield to avoid further complications. Anti-oxidants and steroids have proven to reduce the risk of additional damage after an explosion by combating free radicals created during noise-induced hearing loss (Rajguru, 2013). Thrombosis of the vascular system prevents medication concentrations from being adequate at the site of infection, which results in ineffective medical therapy. A systemic abnormality needs to be corrected, such as diabetes management, immunosuppressant withdrawal or modification, or corticosteroid administration. Surgical intervention (sequestrectomy and debridement) and adjuvant therapy are used in conjunction with antifungal treatment. The best antifungal agent is AmB treatment (Ferguson, 2000). P. Spicer et al. (2013) used gelatin hydrogel substrates combined with poly(dl-lactic-co-glycolic acid) (PLGA) particle carriers to develop antibiotic-releasing, porous polymethylmethacrylate space maintainers. Three formulations with two different releasing speeds were tested: 1) burst and 2) prolonged. They were evaluated in different dosages in vivo on rabbits whose mandibular bone was infected with Acinetobacter baumannii (2 × 107 CFU/ml-1). The results revealed that implants with a high-dose prolonged release had better effects on soft tissue healing than implants with a fast release. Implants with an extended release of colistin through PLGA microparticle carrier demonstrated enhanced soft tissue treatment compared to implants with the fast release of colistin in a gelatin-based carrier (Spicer et al., 2013). Many treatments are available to treat craniofacial diseases, including drugs, surgery, stem cells, and bioactive materials. The development of fundamental and predictable periodontal regeneration techniques has been documented since the 1980s. For periodontal disease treatment, graft materials are used to replace the bone loss caused by the disease, barrier membranes are used to prevent tissue regeneration, and bioactive chemicals stimulate tissue regeneration (Han et al., 2014a). In tissue loss, trauma, periodontitis, developmental, dental caries, pulpitis, or oral cancer illnesses, allograft or autograft bone is used to fill the hole created by lesion excision. Clinical researchers and clinicians are interested in regenerative dental tissue or tissue engineering for potential functional restoration following tissue volume repair (Xu et al., 2021). Silver diamine fluoride (SDF) is an effective substance for non-invasive therapy. SDF is one of five breakthrough treatments recognized by the Food and Medicine Administration (FDA), which could be the first FDA-approved caries treatment (Horst, 2018). The current biomaterial and synthetic materials therapy in craniofacial and dental trauma and disease are summarized in Table 3.
4.4 Soft tissue regenerative medicine in craniofacial diseases
4.4.1 Regenerative medicine in facial organ injuries
The pinna hematoma should be incised and drained immediately to prevent cartilaginous hematoma. It is common for the tympanic membrane to rupture, and it typically happens in conjunction with other injuries, more severe. Blast injury should be considered in every service member exposed to blast injuries, even if there are no prominent head, face, or neck injuries. An audiogram should be performed after other injuries have been ruled out. The war conditions necessitate aid, even when most perforations heal independently. One study found that five of 14 membranes left untreated did not heal after the bombing of the US embassy in Kenya, whereas all those treated did (Rajguru, 2013). Research has shown that porous polyethylene repair will provide better aesthetic results, shorter surgical times, fewer treatments required, and a faster postoperative recovery than autologous reconstruction (Ali et al., 2017). Medpor’s ability to contour and mold the polyethylene implant intraoperatively allows for a good fit with the contralateral ear in most instances following auricular reconstruction (Wilkes et al., 2014). Fractures and hematomas in the septum can result from nasal trauma. Septal hematomas require immediate incision and drainage to prevent infection and cartilaginous necrosis. The treatment plan for epistaxis should include anterior and, if needed, posterior nasal packing. The anterior packing of the nose can be done with Merocel or Net cell nasal packs and ribbon gauze soaked in antibiotic solution. Foley catheters of 12G or 14G should be inflated to three-quarter capacity in the nasopharynx, then dragged anteriorly until they impact posteriorly. It is possible to remove the packs and reevaluate the situation in 24–48 h (Rajguru, 2013). Gentile et al. (2016) used autologous chondrocyte micrografts and platelet-rich plasma (PRP). The utilization of chondrocyte micrografts produced from the nasal septum poured PRP in solid form was proven to regenerate the cartilage of external nasal valve collapse in this study.
Reconstructing the lip requires realigning anatomical markers and restoring functional muscle and sensory function. Unless the affected portion of the lip covers more than 30%, a primary closure may be attempted. Skin grafting may be an option and local advancement flaps, but it is best to repair the defect with accessible lip tissue (Braun and Maricevich, 2017). According to Grewal et al. (2021), two-stage cleft lip surgery improves facial symmetry and final lip, nose, and palate restoration. This research supports their theory that a two-stage repair can provide functional and aesthetically pleasing results instead of presurgical nasoalveolar shaping. Vitagliano et al. (2016) described a new lip-repair procedure involving nasolabial flaps and adipose tissue transplants in a single study. It has been concluded that surgical removal of a tumor alone does not restore facial eurythmic. Transplanting adipose tissue can accomplish this. Lip repair can be significantly improved functionally and cosmetically with these two methods, ensuring excellent long-term results. According to Pushpoth et al. (2008), AlloDerm is a helpful lamellar graft used in treating full-thickness lid deformities. The potential for AlloDerm to be an excellent posterior lamellar replacement is relatively high when there is enough skin cover to drape over it. Conjunctiva grows smoothly over its structure because it replaces the tarsus and acts as a scaffold.
4.4.2 Regenerative medicine in skin injuries
The first skin replacements were made from porous matrices mimicking the dermis and serving as dermal regeneration templates. In addition to enhancing wound healing, these matrices can be used to deliver medications and aid in regenerative cell adhesion. Dermal scaffolds or autografts of epidermal keratinocytes were previously implanted (Borrelli et al., 2020). Certain GF families may enhance wound healing if correctly used because they are involved in endogenous wound healing. In a double-blind clinical study, epidermal growth factor (EGF) was applied topically to skin transplant recipient sites and significantly enhanced epidermal regeneration (Brown et al., 1989). For wound dressings, Oh et al. (2016) employed an electrospinning process to create composite fibrous mats made up of chitosan (CH), poly(caprolactone) (PCL), or chitosan-caffeic acid conjugate (CCA). These findings suggest that PCL/CCA fiber mats can be used in skin tissue engineering. The nanoparticle-in-nanofiber system developed by Xie et al. (2016) for wound healing applications releases two growth factors simultaneously. The nanofibrous meshes mimic the natural healing process, but they support it by electrospinning chitosan and poly(ethylene oxide). Angiogenesis was enhanced by nanofibers containing vascular endothelial growth factor (VEGF).
Additionally, poly(lactic-co-glycolic acid) (PLAGA) nanoparticles encapsulated with PDGF were injected into nanofibers to promote organ regeneration and tissue remodeling. Our findings indicate that nanoparticles embedded in nanofibers might be a therapeutic tool for chronic and routine wound healing (Xie et al., 2013). Similarly, Guo et al. (2015) found that the attractive chemokine SDF-1/CXCR4 is involved in epidermal stem cell migration during wound healing. A significant increase in SDF-1 levels was seen at wound edges after damage, and CXCR4 was examined in epidermal stem cells and proliferative epithelial cells. An experiment conducted by Kim et al. (2011) examined whether adipose-derived stem cells (ADSCs) have the potential to reverse the signs of aging, in particular by increasing dermal collagen production and angiogenesis. By growth factor production and fibroblast activation, it has been shown that ADSCs have wound-healing, whitening, and antioxidant properties on the skin. According to their findings, using ADSCs for treating age-related skin deterioration was useful. Clinically, the aim is to develop injectable nanocomposite conductive hydrogel dressings that can function as adhesive dressings, antibacterial, and radical scavengers and have superior mechanical properties that improve the healing of full-thickness skin wounds. Liang et al. (2019) made wound dressings from HA-grafted-dopamine and reduced graphene oxide (Dominiak et al., 2020) using an H2 O2/HPR system. Therefore, they are ideal wound dressings for promoting full-thickness skin restoration thanks to antioxidative, conductive, and adhesive hydrogels with sustained drug-release properties.
4.4.3 Regenerative medicine in nerve and muscle injuries
Surgical removal of a parotid tumor, trauma, or petrous bone surgery can injure the facial nerve or be absent at birth. An abnormality of the facial nerve can cause functional movement difficulties and facial asymmetry, significantly lowering the quality of life (Borrelli et al., 2020). A minimally invasive liposuction process can be used to obtain ADSCs that are useful in nerve regeneration Watanabe et al. (2017). According to the results, both undifferentiated and differentiated ADSCs have therapeutic potential for facial nerve regeneration when used in a cell-based treatment. A mouse model with severe axon damage showed dramatic functional recovery from sciatic nerve crush after ADSCs was administered intravenously 1 week after the injury. Marconi et al. (2012) reported that the regeneration of nerves might also be assisted by other cells besides ADSCs. bFGF stimulates the growth of proximal axons from the stump of the proximal nerve and impacts nerve cells, Schwann cells, and fibroblasts, which play an essential role in the regeneration of the peripheral nerve. Matsumine et al. (2016) developed acidic gelatin hydrogels to deliver bFGF. They aimed to determine whether it would release bFGF and trigger peripheral nerve regeneration in vivo for many weeks. Seven weeks after implantation, histology revealed a significantly higher rate of regeneration, the induction of a large number of newly developed nerve axons, and a higher rate of mature nerve axons in the BGF microsphere group than in the BGF-free group.
4.5 Hard tissue regenerative medicine in craniofacial diseases
4.5.1 Regenerative medicine in the frontal process of the maxilla (FFPM)
Traditional treatment ways for FFPM include open reduction and internal fixation with bone plates or wire. Although these procedures are faster than surgery, some drawbacks exist, such as more significant trauma, scars on the face, and a more extended recuperation period. Meng et al. (2020) performed fracture reduction using an endoscopic endonasal procedure, a novel surgical technique. It provided good exposure to the FFPM. FFPM reduction provides an anatomical reduction with an outstanding aesthetic result, a straightforward process, and a visible operation. FFPM can be treated more effectively with this less invasive method.
4.5.2 Regenerative medicine in maxilla and mandible
Open reduction of craniofacial bone fractures generally requires metal plates and screws. Alternatively, metal implants may result in protrusion, palpation, or a foreign body response, requiring another procedure after removal. Metal implants have recently demonstrated promising results when combined with absorbable implants. The absorbable mesh and screws employed by Ju et al. (2019) were utilized to decrease the open fractures and fragments of bone. Maxillary fractures can be treated with absorbable implants and screws without requiring additional surgery to remove the metal plate. Furthermore, fixing small bone pieces with cyanoacrylate is an exact and secure process, meaning that screws cannot be used. Chen et al. (2021) studied a midline palatal suture’s fracture mechanics and stress distribution during surgically assisted fast palatal expansion in maxillary transverse deficit under dynamic stresses. CBCT data from a 21-year-old female volunteer were used to create a model of the CMF complex (including the palatal suture). A finite element analysis model was developed based on meshwork. In order to determine the time-load curve, palatal suture yield strength was obtained within 140 m after a force was applied that increased from 0 to 500 N, simulating non-surgical bone expansion (model A). This approach examined the fracture process, time, and stress distribution of the palatal suture in both maxillary lateral osteotomies (model B) and LeFort osteotomy I-assisted (model C) extension of the maxillary arch. In the model B arch expansion with lateral osteotomy, the palatal suture rupture and lateral maxillary extension were comparable to the arch expansion with LFIO (model C). Maxillary lateral wall-osteotomy may be an alternative to LFIO due to its stress and significant consequences. Physiological and structural changes following extraction threaten the integrity of the anterior maxilla’s bone walls. A regenerating strategy is essential to enable hard and soft tissue dimensions to be preserved throughout time. Chappuis et al. (2018) observed 20 patients over 10 years to examine the impact of early implant placement with simultaneous contour augmentation by guided bone regeneration. This study revealed an effective technique for early implant placement and contour augmentation using a two-layer composite graft in post-extraction single tooth sites, resulting in stable bone conditions and low mucosal recession risks over a 10-year observation period. Orthodontic treatment is not automatically recommended when the maxilla and the mandible have severe sagittal differences. Such cases require the participation of a surgeon, an orthodontist, and a periodontist, coordinating an interdisciplinary approach. Each patient’s treatment is tailored to their specific needs and developed individually, regardless of the use of conventional treatment approaches. A variety of complications may arise from the surgical correction of bone abnormalities of the jawbones. Orthognathic abnormalities can now be treated using RM, already commonly used in reconstructive surgery. Using allogenic 3D graft blocks as an alternative for issues during regular orthodontic treatment, Dominiak et al. (2020) uncovered a technique for reconstructing sagittal alveolar bone with a 3D reconstruction style. High-frequency acceleration (HFA) promotes alveolar bone growth in physiological settings and during the healing process following tooth extractions. Alikhani et al. (2019) investigated HFA’s impact on the osteoporotic alveolar bone, finding that it acted as a regenerative agent. Vibration enhanced anabolic metabolism and reduced catabolic metabolism in the alveolar bone of osteoporotic rats. Their study suggests that HFA could be a potential therapy in patients with low alveolar bone density due to osteoporosis. An investigation was conducted by Pourebrahim et al. (2013) to assess bone regeneration following autogenous bone transplantation and stem cells obtained from adipose tissue in canine maxillary alveolar clefts. From under the skin of a dog, MSCs were extracted. In addition to autograft, tissue-generated bone seems a viable alternative to bone regeneration. Common congenital abnormalities include cleft lip and palate (CLP). It can occur unilaterally or bilaterally and has acquired and hereditary causes. Alveolar cleft grafting remains contentious regarding time, materials, and surgical procedures. Alveolar cleft defects can be repaired by several grafting strategies, according to Mossaad et al. (2019). Artificial iliac crest bone grafting has proven more reliable than using nano calcium hydroxyapatite or BMSCs as substitutes. Using human dental pulp stem cells (DPSCs) obtained from deciduous teeth, Jahanbin et al. (2016) investigated the ability of DPSCs to regenerate maxillary alveolar lesions in Wistar rats. In rats, human DPSCs provided a viable model for restoring maxillary alveolar defects in CLP patients, providing an extra resource of cells for healing. In another investigation, Feng et al. (2016) used MSCs to treat the bone regeneration of mandibular distraction osteogenesis in rabbits plus Runt-related transcription factor 2 (Runx2). They found that rabbit mandibular distraction osteogenesis was efficiently enhanced, and the fixed period was reduced using Runx2 ex vivo gene therapy. Therefore, there would benefit from repairing craniofacial fractures. The osteogenic drug dipyridamole incorporated into 3D-printed bioceramic scaffolds is effective for critical-sized calvarial lesions healing in skeletally mature translational animals. Yet, no studies have developed craniofacial models with this design. Maliha et al. (2020) implanted bioceramic/dipyridamole scaffolds into a developing calvaria limb and measured bone formation according to geometry and dipyridamole concentration. The suture’s growth may be adversely affected and aimed to improve bone formation in children using a pediatric scaffold and a dipyridamole concentration to maintain the cranial suture. The condition of osteoporosis results in an increased risk of fractures as the structural integrity of bone tissue deteriorates. Bone tissue engineering procedures typically utilize calcium phosphates (CaPs), which are similar to bone apatite, except that they do not contain any trace elements (TE). Bioactive glass is used in dentistry and CMF surgery due to its capacity for bonding with bone and stimulating osteoblastic development. Using rat bone marrow mesenchymal stem cells (BMMSCs) from osteoporotic and normal animals, Chen et al. (2017) studied the osteogenic effects of quaternary Si-Sr-Zn-Mg-cooped CaP or 45S5 BG ionic dissolution products. The results indicate that TE-CaP and 45S5 BG work together to promote osteoporotic and normal growth and differentiation of rMSCs.
4.5.3 Regenerative medicine in orbital floor
Orbital fractures possess a unique trauma mechanism because of the complex architecture of the bones and soft tissue elements involved. An orbital blowout fracture can increase the orbital volume, causing conjunctival hypertrophy and enophthalmos. Other cases may require surgical intervention, even if conservative methods are used (Koenen and Waseem, 2021).
4.5.4 Regenerative medicine in periodontal diseases
Several treatments and medicines can be used to eliminate or alleviate periodontal disease. Plague-induced gingivitis is prevented by mechanical cleaning, removing plaque, and cleaning teeth. Periodontal disease and its damaging effects cannot be treated with this approach. Alternative treatments are needed to achieve better results. Mouthwashes and dentifrice, for instance, act as plaque-removal agents. In addition to mechanical methods, these products can also be used. Statistically significant reductions in plaque and gingivitis were shown in one study (Teughels et al., 2013). According to a second study, the best dentifrices reduce plaque most effectively when they contain chlorhexidine and triclosan (Faggion et al., 2014). The tooth brushing technique can be used in addition to other mechanical practices, such as subgingival debridement. Nevertheless, it has several limitations; for example, it cannot be used in all situations. When people who smoke regularly recover from surgery or non-surgical treatment, their pocket depth decreases by 0.23–1 mm (Heasman et al., 2006). PDL fibers anchor the tooth in the socket by binding the cementum on the tooth root surface to the alveolar bone and reducing occlusal pressures. Regeneration of PDL is crucial to successful periodontitis treatment. A great result would be for the newly restored bone and cementum to be perpendicularly connected to the newly implanted collagen fibers (Liu et al., 2019). Bone loss resulting from periodontal disease can be replaced by guided bone regeneration (GBR). Several drawbacks to the GBR procedure include the requirement for a stable barrier membrane during surgery and a new creative cavity. Tsai et al. (2016) used an innovative microinjector to overcome these drawbacks and this innovation could provide bone graft replacements for the healing of periodontal disease bone deficiency through the use of a microinjector.
Dental caries is considered one of the most frequent chronic diseases in the United States, affecting 92% of people aged 20–64 years. An alternative technique for replacing damaged tooth tissues is scaffold-based tissue engineering. NF-gelatin/MAGP hybrid scaffolds were created based on nanofiber gelatin and magnesium phosphate using biomimetic techniques by Qu et al. (2014). DPSC proliferation, differentiation, and biomineralization are facilitated by these scaffolds that mimic the nanostructure and chemical composition of the natural dentin matrix. Developing biomimetic nanostructured hybrid scaffolds that release metallic ions regulated in a controlled manner may enhance the scaffolds’ ability to regenerate tooth tissues. Researchers showed that a new lipoxin analog (benzo-lipoxin A4, bLXA4) could induce the hard and soft tissue regeneration permanently lost to periodontitis. They concluded that NPRM-bLXA4 mimics endogenous resolving mechanisms and can be helpful in therapeutic tissue engineering for chronic osteolytic inflammatory diseases (Van Dyke et al., 2015). Multifunctional growth factors, including transforming growth factors-βσ ((TGFs), are important for many cell types. Hassan and Alagl (2015) used a bovine bone mineral matrix with TGF-1 for intrabony defect treatment compared to using only inorganic bovine bone, and they found it effective. According to their results, the sole usage of inorganic bovine bone was not helpful. A modern injection method using microporous CaP cement (CPC) and either fibroblast growth factor-2 (FGF-2) or BMP-2 was used by Oortgiesen et al. (2014) to determine the regeneration capabilities of the implant. Comparing CaP + FGF-2 with CaP + BMP-2, the combination therapy differed considerably in PDL repair score. Compared to CaP alone, FGF-2 + CaP led to significant improvements in bone healing, whereas CaP + BMP-2 did not demonstrate any significant changes. A possible periodontal regeneration therapy could combine a topical FGF-2 application with an injectable CaP. Tang et al. (2015) studied how bone resorption in experimental periodontitis rats was affected by osteoprotegerin (OPG) gene therapy. We examined the effects of OPG on alveolar bone protection using an experimental periodontitis model in which a bacterial plaque-retentive silk ligature was placed around the maxillary second molar tooth, Porphyromonas gingivalis (a bacterial plaque-retentive bacteria) was injected, and a high-carbohydrate diet was consumed. Experimental periodontitis decreases alveolar bone height in rats, and local recombinant OPG gene therapy slows it down. The prevention of periodontal bone loss might be possible through OPG gene therapy. This study found a novel regeneration therapy for teeth and periodontal defects and posttraumatic fractures of the alveolar bone in the place of the maxillary front teeth had been found by Kiyokawa et al. (2009). Dislocated teeth are removed first, followed by the removal of crushed alveolar bone. Reimplanting the displaced teeth is followed by grafting the surrounding bone (marrow) with a cancellous iliac bone to promote periodontal bone growth. This procedure offers a cosmetic and functional recovery superior to the standard treatment in situations like these because no good teeth nearby are sacrificed, and no-good teeth are lost. This can be applied in the future as a new treatment for alveolar fractures. Using mineral trioxide aggregates (MTA), apexification is now preferred for permanent anterior immature necrotic teeth. Apexification decreases the canal wall’s thickness and makes them short so that they are likely to cervical fracture because it prevents teeth from maturing and developing further. Yoshpe et al. (2021) introduced a new method called the regenerative endodontic procedure (REP) used in immature necrotic molars treatment patients. In addition to root extension and expansion, REP allows periapical healing in the dentinal root canals. They discovered that platelet-rich fibrin (PRF) with REP has more advantages than MTA apexification due to extending roots, thickening woody root canal walls, and narrowing the apical foramen. An osteoinductive protein/peptide may be employed with a nanofibrous extraction or bone graft to improve alveolar bone regeneration. Researchers at Boda et al. (2019) demonstrated that calcium-binding peptides mimicking BMP-2 could help regenerate periodontal bony structures. Mineralized nanofiber pieces, such as MF bone defect fillers, can offer a viable alternative to replace conventional dental bone graft formulations. Biological applications, such as soft tissue engineering, wound healing, and hemostasis, may benefit from these nanofiber pieces. Du et al. (2014) used allogeneic BMMSCs to create a tissue regeneration-inducted in a rat model. They suggested that injections of BMMSCs may work as anti-inflammatories and immunomodulators to reduce periodontitis-related abnormalities. Recent studies on RM in craniofacial and dental trauma and disease are summarized in Table 4.
5 Conclusion
The current tissue engineering and RM approaches in face aesthetics surgery and craniofacial restoring and reconstruction offer ways to repair congenital or acquired abnormalities of tissue types, such as nerves, soft tissue, blood vessels, bone, and cartilage. However, significant progress has been achieved, and preclinical and clinical trial results seem promising; discrepancies in some study designs may draw incorrect results, so they cannot be used in clinical experiments. Some research has shown success with TE of cartilage and bone. The early findings suggest that soft tissue can also be successfully regrown. There is a need for some research on scaffold properties, cell types, and delivery techniques in the most efficient way before RM/TE can be used clinically in craniofacial surgery. This will allow more advances and advancements as interdisciplinary cooperation between polymer chemistry, molecular genetics, robotics, molecular biology, mechanical engineering, and materials science gains strength. There are some limitations in damaged tissue or tissue defect healing. The limitation of surgery is that it neither guarantees nor promotes healing. As best as they can, surgeons seek to remove known barriers to healing, including infections, instability, and foreign bodies. However, most studies had enough sample size, and some lacked enough samples to be considered vital. On the other side, however, many studies have been performed on biomaterials and natural materials. All of their effects are not revealed yet, and it is not possible to use them with no caution in preclinical procedures. Cell therapy (especially stem cells) and growth factor usage have been focused on in recent decades, but their full impacts are not discovered either. Therefore, there is a need to conduct more in vitro and in vivo studies and develop a simulator bioreactor for performing ex vivo experiments. More in vitro and in vivo studies should be performed, and it is one of the most critical limitations regarding RM.
6 Future direction
A recent technological advance in craniofacial and dental reconstruction is the 3D printing of biomaterials. Nanotechnology has also been shown to be highly beneficial. TE is potentially replacing more sophisticated template manufacturing procedures with 3D printing, a rapidly evolving technology that uses computer-enabled printers to organize a template into a proper 3D form. In craniofacial restoration, 3D printing may be utilized to fabricate porous materials with more vital interconnections and produce suitable templates for replacing and treating bone defects with precise anatomical forms. A few case reports on humans have shown successful results with few problems. Studies have demonstrated that nanoparticles, nanotubes, and nanofibers can improve mechanical scaffold properties, cellular adhesion, and tissue regeneration. The biomechanical and biochemical capabilities of nanoparticles have been demonstrated in multiple studies, making TE a promising treatment for MF and oral tissues. Nanomaterials may build up in several organs because accurate dose-response and toxicity screening approaches must be developed before regular clinical trials.
Author contributions
MM, MY, HT, ET, KA, MA, and PEFB coordinated the draft and wrote the manuscript. SGB, RK, and MH helped draft and review the manuscript. MM, MY, HT, ET, KA, MA, and PEFB contributed to the conceptualization, editing, and funding acquisition. All authors read and approved the final manuscript.
Acknowledgments
The authors would like to acknowledge colleagues who gave valuable comments.
Conflict of interest
The authors declare that the research was conducted in the absence of any commercial or financial relationships that could be construed as a potential conflict of interest.
Publisher’s note
All claims expressed in this article are solely those of the authors and do not necessarily represent those of their affiliated organizations or those of the publisher, the editors, and the reviewers. Any product that may be evaluated in this article, or claim that may be made by its manufacturer, is not guaranteed or endorsed by the publisher.
References
Abd-Elrahman, A., Shaheen, M., Askar, N., and Atef, M. (2020). Socket shield technique vs conventional immediate implant placement with immediate temporization. Randomized clinical trial. Clin. implant Dent. Relat. Res. 22, 602–611. doi:10.1111/cid.12938
Adeel, M., Rajput, M. S. A., Arain, A. A., Baloch, M., and Khan, M. (2018). Ameloblastoma: Management and outcome. Cureus 10, e3437. doi:10.7759/cureus.3437
Al-Ahmady, H. H., Abd Elazeem, A. F., Bellah Ahmed, N. E., Shawkat, W. M., Elmasry, M., Abdelrahman, M. A., et al. (2018). Combining autologous bone marrow mononuclear cells seeded on collagen sponge with Nano Hydroxyapatite, and platelet-rich fibrin: Reporting a novel strategy for alveolar cleft bone regeneration. J. cranio-maxillo-facial Surg. 46, 1593–1600.
Alam, M., Hughart, R., Champlain, A., Geisler, A., Paghdal, K., Whiting, D., et al. (2018). Effect of platelet-rich plasma injection for rejuvenation of photoaged facial skin: A randomized clinical trial. JAMA dermatol. 154, 1447–1452. doi:10.1001/jamadermatol.2018.3977
Ali, K., Trost, J. G., Truong, T. A., and Harshbarger, R. J. (2017). Total ear reconstruction using porous polyethylene. Semin. Plast. Surg. 31, 161–172. doi:10.1055/s-0037-1604261
Alikhani, M., Alikhani, M., Alansari, S., Almansour, A., Hamidaddin, M. A., Khoo, E., et al. (2019). Therapeutic effect of localized vibration on alveolar bone of osteoporotic rats. PLoS One 14, e0211004. doi:10.1371/journal.pone.0211004
Alovisi, M., Pasqualini, D., Musso, E., Bobbio, E., Giuliano, C., Mancino, D., et al. (2018). Influence of contracted endodontic access on root canal geometry: An in vitro study. J. Endod. 44, 614–620. doi:10.1016/j.joen.2017.11.010
Aman, H., Shokri, T., Reddy, L. V., and Ducic, Y. (2019). Secondary management of midface fractures. Facial plast. Surg. FPS 35, 640–644. doi:10.1055/s-0039-3399494
Andrew, T. W., Morbia, R., and Lorenz, H. P. (2019). Pediatric facial trauma. Clin. plastic Surg. 46, 239–247. doi:10.1016/j.cps.2018.11.008
Atala, A., and Forgacs, G. (2019). Three-dimensional bioprinting in regenerative medicine: Reality, hype, and future. STEM CELLS Transl. Med. 8, 744–745. doi:10.1002/sctm.19-0089
Avila-Ortiz, G., Gubler, M., Romero-Bustillos, M., Nicholas, C. L., Zimmerman, M. B., and Barwacz, C. A. (2020). Efficacy of alveolar ridge preservation: A randomized controlled trial. J. Dent. Res. 99, 402–409. doi:10.1177/0022034520905660
Bahammam, M. A. (2016). Effectiveness of bovine-derived xenograft versus bioactive glass with periodontally accelerated osteogenic orthodontics in adults: A randomized, controlled clinical trial. BMC oral health 16, 126. doi:10.1186/s12903-016-0321-x
Bajestan, M. N., Rajan, A., Edwards, S. P., Aronovich, S., Cevidanes, L. H. S., Polymeri, A., et al. (2017). Stem cell therapy for reconstruction of alveolar cleft and trauma defects in adults: A randomized controlled, clinical trial. Clin. implant Dent. Relat. Res. 19, 793–801. doi:10.1111/cid.12506
Balic, A. (2018). Biology explaining tooth repair and regeneration: A mini-review. Gerontology 64, 382–388. doi:10.1159/000486592
Berger, M., Probst, F., Schwartz, C., Cornelsen, M., Seitz, H., Ehrenfeld, M., et al. (2015). A concept for scaffold-based tissue engineering in alveolar cleft osteoplasty. J. cranio-maxillo-facial Surg. 43, 830–836.
Bijukumar, D. R., McGeehan, C., and Mathew, M. T. (2018). Regenerative medicine strategies in biomedical implants. Curr. Osteoporos. Rep. 16, 236–245. doi:10.1007/s11914-018-0441-0
Blanco-Elices, C., España-Guerrero, E., Mateu-Sanz, M., Sánchez-Porras, D., García-García Ó, D., Sánchez-Quevedo, M. D. C., et al. (2020). Vitro generation of novel functionalized biomaterials for use in oral and dental regenerative medicine applications. Running Title: Fibrin-agarose functionalized scaffolds. Materials 13.
Boda, S. K., Almoshari, Y., Wang, H., Wang, X., Reinhardt, R. A., Duan, B., et al. (2019). Mineralized nanofiber segments coupled with calcium-binding BMP-2 peptides for alveolar bone regeneration. Acta biomater. 85, 282–293. doi:10.1016/j.actbio.2018.12.051
Boëck-Neto, R. J., Artese, L., Piattelli, A., Shibli, J. A., Perrotti, V., Piccirilli, M., et al. (2009). VEGF and MVD expression in sinus augmentation with autologous bone and several graft materials. Oral Dis. 15, 148–154. doi:10.1111/j.1601-0825.2008.01502.x
Boffano, P., Corre, P., and Righi, S. (2017). The role of intra-articular surgery in the management of mandibular condylar head fractures. Atlas oral Maxillofac. Surg. Clin. N. Am. 25, 25–34. doi:10.1016/j.cxom.2016.10.001
Bonfield, C. M., Kumar, A. R., and Gerszten, P. C. (2014). The history of military cranioplasty. Neurosurg. focus 36, E18. doi:10.3171/2014.1.FOCUS13504
Boos, A. M., Loew, J. S., Weigand, A., Deschler, G., Klumpp, D., Arkudas, A., et al. (2013). Engineering axially vascularized bone in the sheep arteriovenous-loop model. J. tissue Eng. Regen. Med. 7, 654–664. doi:10.1002/term.1457
Borrelli, M. R., Hu, M. S., Longaker, M. T., and Lorenz, H. P. (2020). Tissue engineering and regenerative medicine in craniofacial reconstruction and facial aesthetics. J. craniofacial Surg. 31, 15–27. doi:10.1097/SCS.0000000000005840
Bourne, D. A., Bliley, J., James, I., Donnenberg, A. D., Donnenberg, V. S., Bft, B., et al. (2021). Changing the paradigm of craniofacial reconstruction: A prospective clinical trial of autologous fat transfer for craniofacial deformities. Ann. Surg. 273, 1004–1011. doi:10.1097/SLA.0000000000003318
Bowes, D. E., Gamble, J. J., and Bajwa, J. S. (2020). Using automated pump-delivery devices to reduce the incidence of excessive fluid administration during pediatric dental surgery: A randomized-controlled trial. Can. J. Anaesth. = J. Can. d'anesthesie 67, 1535–1540. doi:10.1007/s12630-020-01776-4
Boyce, A. M., Burke, A., Cutler Peck, C., DuFresne, C. R., Lee, J. S., and Collins, M. T. (2016). Surgical management of polyostotic craniofacial fibrous dysplasia: Long-term outcomes and predictors for postoperative regrowth. Plastic Reconstr. Surg. 137, 1833–1839. doi:10.1097/PRS.0000000000002151
Boyce, A. M., Chong, W. H., Shawker, T. H., Pinto, P. A., Linehan, W. M., Bhattacharryya, N., et al. (2012). Characterization and management of testicular pathology in McCune-Albright syndrome. J. Clin. Endocrinol. metabolism 97, E1782–E1790. doi:10.1210/jc.2012-1791
Braun, T. L., and Maricevich, R. S. (2017). Soft tissue management in facial trauma. Semin. Plast. Surg. 31, 73–79. doi:10.1055/s-0037-1601381
Braun, T. L., Xue, A. S., and Maricevich, R. S. (2017). Differences in the management of pediatric facial trauma. Semin. Plast. Surg. 31, 118–122. doi:10.1055/s-0037-1601380
Breeze, J., Bowley, D. M., Combes, J. G., Baden, J., Rickard, R. F., DuBose, J., et al. (2019). Facial injury management undertaken at US and UK medical treatment facilities during the Iraq and Afghanistan conflicts: A retrospective cohort study. BMJ open 9, e033557. doi:10.1136/bmjopen-2019-033557
Brown, G. L., Nanney, L. B., Griffen, J., Cramer, A. B., Yancey, J. M., Curtsinger, L. J., et al. (1989). Enhancement of wound healing by topical treatment with epidermal growth factor. N. Engl. J. Med. 321, 76–79. doi:10.1056/NEJM198907133210203
Brucoli, M., Boffano, P., Romeo, I., Corio, C., Benech, A., Ruslin, M., et al. (2020). Epidemiology of maxillofacial trauma in the elderly: A European multicenter study. J. stomatology, oral Maxillofac. Surg. 121, 330–338. doi:10.1016/j.jormas.2019.09.002
Buchanan, E. P., Xue, A. S., and Hollier, L. H. (2014). Craniofacial syndromes. Plastic Reconstr. Surg. 134, 128e–53e. doi:10.1097/PRS.0000000000000308
Burke, A. B., Collins, M. T., and Boyce, A. M. (2017). Fibrous dysplasia of bone: Craniofacial and dental implications. Oral Dis. 23, 697–708. doi:10.1111/odi.12563
Castro-Espicalsky, T. L., Costa, S. T., Santiago, B. M., Freire, A. R., Daruge Júnior, E., Prado, F. B., et al. (2020). Craniofacial injuries by firearms projectiles: An analysis of 868 deaths in the five regions of Brazil. J. forensic Leg. Med. 69, 101888. doi:10.1016/j.jflm.2019.101888
Castro-Govea, Y., Cervantes-Kardasch, V. H., Borrego-Soto, G., Martínez-Rodríguez, H. G., Espinoza-Juarez, M., Romero-Díaz, V., et al. (2012). Human bone morphogenetic protein 2-transduced mesenchymal stem cells improve bone regeneration in a model of mandible distraction surgery. J. craniofacial Surg. 23, 392–396. doi:10.1097/SCS.0b013e318240fe9b
Cavalcanti, B. N., Zeitlin, B. D., and Nör, J. E. (2013). A hydrogel scaffold that maintains viability and supports differentiation of dental pulp stem cells. Dent. Mater. 29, 97–102.
Chang, B., Ahuja, N., Ma, C., and Liu, X. (2017). Injectable scaffolds: Preparation and application in dental and craniofacial regeneration. Mater Sci. Eng. R. Rep. 111, 1–26. doi:10.1016/j.mser.2016.11.001
Chang, H-H., Wang, Y-L., Chiang, Y-C., Chen, Y-L., Chuang, Y-H., Tsai, S-J., et al. (2014). A novel chitosan-γPGA polyelectrolyte complex hydrogel promotes early new bone formation in the alveolar socket following tooth extraction. PLoS One 9, e92362. doi:10.1371/journal.pone.0092362
Chappuis, V., Rahman, L., Buser, R., Janner, S. F. M., Belser, U. C., and Buser, D. (2018). Effectiveness of contour augmentation with guided bone regeneration: 10-Year results. J. Dent. Res. 97, 266–274. doi:10.1177/0022034517737755
Chen, J., Xu, Y., Li, C., Zhang, L., Yi, F., and Lu, Y. (2021). Displacement and stress distribution of the craniomaxillofacial complex under different surgical conditions: A three-dimensional finite element analysis of fracture mechanics. BMC oral health 21, 596. doi:10.1186/s12903-021-01941-1
Chen, W., Liu, J., Manuchehrabadi, N., Weir, M. D., Zhu, Z., and Xu, H. H. (2013). Umbilical cord and bone marrow mesenchymal stem cell seeding on macroporous calcium phosphate for bone regeneration in rat cranial defects. Biomaterials 34, 9917–9925. doi:10.1016/j.biomaterials.2013.09.002
Chen, X. Y., Xu, S. Z., Wang, X. W., Yang, X. Y., Ma, L., Zhang, L., et al. (2017). Systematic comparison of biologically active foreign ions-codoped calcium phosphate microparticles on osteogenic differentiation in rat osteoporotic and normal mesenchymal stem cells. Oncotarget 8, 36578–36590. doi:10.18632/oncotarget.16618
Chin, A. R., Gao, J., Wang, Y., Taboas, J. M., and Almarza, A. J. (2018). Regenerative potential of various soft polymeric scaffolds in the temporomandibular joint condyle. J. Oral Maxillofac. Surg. 76, 2019–2026. doi:10.1016/j.joms.2018.02.012
Cho, H., Tarafder, S., Fogge, M., Kao, K., and Lee, C. H. (2016). Periodontal ligament stem/progenitor cells with protein-releasing scaffolds for cementum formation and integration on dentin surface. Connect. tissue Res. 57, 488–495. doi:10.1080/03008207.2016.1191478
Clark, D., Rajendran, Y., Paydar, S., Ho, S., Cox, D., Ryder, M., et al. (2018). Advanced platelet-rich fibrin and freeze-dried bone allograft for ridge preservation: A randomized controlled clinical trial. J. periodontology 89, 379–387. doi:10.1002/JPER.17-0466
Cohn Yakubovich, D., Eliav, U., Yalon, E., Schary, Y., Sheyn, D., Cook-Wiens, G., et al. (2017). Teriparatide attenuates scarring around murine cranial bone allograft via modulation of angiogenesis. Bone 97, 192–200. doi:10.1016/j.bone.2017.01.020
Couturier, A., Aumaître, O., Gilain, L., Jean, B., Mom, T., and André, M. (2017). Craniofacial fibrous dysplasia: A 10-case series. Eur. Ann. otorhinolaryngology, head neck Dis. 134, 229–235. doi:10.1016/j.anorl.2017.02.004
Cui, J., Liang, J., Wen, Y., Sun, X., Li, T., Zhang, G., et al. (2014). In vitro and in vivo evaluation of chitosan/β-glycerol phosphate composite membrane for guided bone regeneration. J. Biomed. Mater. Res. Part A 102, 2911–2917. doi:10.1002/jbm.a.34874
Cusack, B. J., Parsons, T. E., Weinberg, S. M., Vieira, A. R., and Szabo-Rogers, H. L. (2017). Growth factor signaling alters the morphology of the zebrafish ethmoid plate. J. Anat. 230, 701–709. doi:10.1111/joa.12592
D'Onofrio, L. (2019). Oral dysfunction as a cause of malocclusion. Orthod. craniofacial Res. 22 (1), 43–48. doi:10.1111/ocr.12277
Dadsetan, M., Guda, T., Runge, M. B., Mijares, D., LeGeros, R. Z., LeGeros, J. P., et al. (2015). Effect of calcium phosphate coating and rhBMP-2 on bone regeneration in rabbit calvaria using poly(propylene fumarate) scaffolds. Acta biomater. 18, 9–20. doi:10.1016/j.actbio.2014.12.024
Das, A., Fishero, B. A., Christophel, J. J., Li, C. J., Kohli, N., Lin, Y., et al. (2016). Poly(lactic-co-glycolide) polymer constructs cross-linked with human BMP-6 and VEGF protein significantly enhance rat mandible defect repair. Cell tissue Res. 364, 125–135. doi:10.1007/s00441-015-2301-x
Denadai, R., Sabbag, A., Vieira, P. R., Raposo-Amaral, C. A., Buzzo, C. L., and Raposo-Amaral, C. E. (2020). Predictors of speech outcome in posterior pharyngeal fat graft surgery for velopharyngeal insufficiency management. J. craniofacial Surg. 31, 41–45. doi:10.1097/SCS.0000000000005802
Deshpande, M. V., West, A. J., Bernacki, S. H., Luan, K., and King, M. W. (2020). Poly(ε-Caprolactone) resorbable auxetic designed knitted scaffolds for craniofacial skeletal muscle regeneration. Bioeng. (Basel) 7, 134. doi:10.3390/bioengineering7040134
Diaz, R. C., Cervenka, B., and Brodie, H. A. (2016). Treatment of temporal bone fractures. J. neurological Surg. Part B, Skull base. 77, 419–429. doi:10.1055/s-0036-1584197
Dominiak, M., Dominiak, S., Targonska, S., and Gedrange, T. (2020). Three-dimensional bone block planning for mandibular sagittal bone defect reconstruction. J. Healthc. Eng. 2020, 8829288. doi:10.1155/2020/8829288
Du, B., Liu, W., Deng, Y., Li, S., Liu, X., Gao, Y., et al. (2015). Angiogenesis and bone regeneration of porous nano-hydroxyapatite/coralline blocks coated with rhVEGF165 in critical-size alveolar bone defects in vivo. Int. J. nanomedicine 10, 2555–2565. doi:10.2147/IJN.S78331
Du, J., Shan, Z., Ma, P., Wang, S., and Fan, Z. (2014). Allogeneic bone marrow mesenchymal stem cell transplantation for periodontal regeneration. J. Dent. Res. 93, 183–188. doi:10.1177/0022034513513026
Dufty, J., Gkranias, N., and Donos, N. (2017). Necrotising ulcerative gingivitis: A literature review. Oral health and Prev. Dent. 15, 321–327. doi:10.3290/j.ohpd.a38766
El Backly, R. M., Zaky, S. H., Muraglia, A., Tonachini, L., Brun, F., Canciani, B., et al. (2013). A platelet-rich plasma-based membrane as a periosteal substitute with enhanced osteogenic and angiogenic properties: A new concept for bone repair. Tissue Eng. Part A 19, 152–165. doi:10.1089/ten.TEA.2012.0357
El-Domyati, M., Abdel-Wahab, H., and Hossam, A. (2018). Combining microneedling with other minimally invasive procedures for facial rejuvenation: A split-face comparative study. Int. J. dermatology 57, 1324–1334. doi:10.1111/ijd.14172
ElKordy, M. A., ElBaradie, T. S., ElSebai, H. I., KhairAlla, S. M., and Amin, A. A. E. (2018). Osteosarcoma of the jaw: Challenges in the diagnosis and treatment. J. Egypt. Natl. Cancer Inst. 30, 7–11. doi:10.1016/j.jnci.2018.02.001
Elsaesser, A. F., Schwarz, S., Joos, H., Koerber, L., Brenner, R. E., and Rotter, N. (2016). Characterization of a migrative subpopulation of adult human nasoseptal chondrocytes with progenitor cell features and their potential for in vivo cartilage regeneration strategies. Cell and Biosci. 6, 11. doi:10.1186/s13578-016-0078-6
Epple, C., Haumer, A., Ismail, T., Lunger, A., Scherberich, A., Schaefer, D. J., et al. (2019). Prefabrication of a large pedicled bone graft by engineering the germ for de novo vascularization and osteoinduction. Biomaterials 192, 118–127. doi:10.1016/j.biomaterials.2018.11.008
Estrela, C., Alencar, A. H., Kitten, G. T., Vencio, E. F., and Gava, E. (2011). Mesenchymal stem cells in the dental tissues: Perspectives for tissue regeneration. Braz. Dent. J. 22, 91–98. doi:10.1590/s0103-64402011000200001
Faggion, C., Cullinan, M., Atieh, M., and Wasiak, J. (2014). An overview of systematic reviews of the use of systemic antimicrobials for the treatment of periodontitis. Br. Dent. J. 217, 443–451. doi:10.1038/sj.bdj.2014.909
Fang, D., Roskies, M., Abdallah, M. N., Bakkar, M., Jordan, J., Lin, L. C., et al. (2017). Three-dimensional printed scaffolds with multipotent mesenchymal stromal cells for rabbit mandibular reconstruction and engineering. Methods Mol. Biol. Clift. NJ) 1553, 273–291. doi:10.1007/978-1-4939-6756-8_22
Feller, L., Wood, N. H., Khammissa, R. A., Lemmer, J., and Raubenheimer, E. J. (2009). The nature of fibrous dysplasia. Head face Med. 5, 22. doi:10.1186/1746-160X-5-22
Feng, G., Zheng, K., Song, D., Wu, S., Zhu, S., and Hu, J. (2016). [Mesenchymal stem cells modified with Runt-related transcription factor 2 promote bone regeneration in rabbit mandibular distraction osteogenesis]. Hua xi kou qiang yi xue za zhi = Huaxi kouqiang yixue zazhi = West. China J. stomatology 34, 125–129. doi:10.7518/hxkq.2016.02.004
Ferguson, B. J. (2000). Mucormycosis of the nose and paranasal sinuses. Otolaryngologic Clin. N. Am. 33, 349–365. doi:10.1016/s0030-6665(00)80010-9
Feuillan, P., Calis, K., Hill, S., Shawker, T., Robey, P. G., and Collins, M. T. (2007). Letrozole treatment of precocious puberty in girls with the McCune-albright syndrome: A pilot study. J. Clin. Endocrinol. metabolism 92, 2100–2106. doi:10.1210/jc.2006-2350
Fischer, R. G., Lira Junior, R., Retamal-Valdes, B., Figueiredo, L. C., Malheiros, Z., Stewart, B., et al. (2020). Periodontal disease and its impact on general health in Latin America. Section V: Treatment of periodontitis. Braz. oral Res. 34, e026. doi:10.1590/1807-3107bor-2020.vol34.0026
Frisch, C. D., Carlson, M. L., Kahue, C. N., Pelosi, S., Haynes, D. S., Lane, J. I., et al. (2015). Fibrous dysplasia of the temporal bone: A review of 66 cases. Laryngoscope 125, 1438–1443. doi:10.1002/lary.25078
Fujioka-Kobayashi, M., Schaller, B., Kobayashi, E., Hernandez, M., Zhang, Y., and Miron, R. J. (2016). Hyaluronic acid gel-based scaffolds as potential carrier for growth factors: An in vitro bioassay on its osteogenic potential. J. Clin. Med. 5, 112. doi:10.3390/jcm5120112
Fulco, I., Miot, S., Haug, M. D., Barbero, A., Wixmerten, A., Feliciano, S., et al. (2014). Engineered autologous cartilage tissue for nasal reconstruction after tumour resection: An observational first-in-human trial. Lancet 384, 337–346. doi:10.1016/S0140-6736(14)60544-4
Gebuijs, I. G. E., Metz, J. R., Zethof, J., Carels, C. E. L., Wagener, F., and Von den Hoff, J. W. (2020). The anti-epileptic drug valproic acid causes malformations in the developing craniofacial skeleton of zebrafish larvae. Mech. Dev. 163, 103632. doi:10.1016/j.mod.2020.103632
Gentile, P., Scioli, M. G., Bielli, A., Orlandi, A., and Cervelli, V. (2016). Reconstruction of alar nasal cartilage defects using a tissue engineering technique based on a combined use of autologous chondrocyte micrografts and platelet-rich plasma: Preliminary clinical and instrumental evaluation. Plast. Reconstr. Surg. Glob. Open 4, e1027. doi:10.1097/GOX.0000000000001027
Ghosh, R., Gopalkrishnan, K., and Anand, J. (2018). Pediatric facial fractures: A 10-year study. J. Maxillofac. oral Surg. 17, 158–163. doi:10.1007/s12663-016-0965-8
Giuliani, A., Iezzi, G., Mazzoni, S., Piattelli, A., Perrotti, V., and Barone, A. (2018). Regenerative properties of collagenated porcine bone grafts in human maxilla: Demonstrative study of the kinetics by synchrotron radiation microtomography and light microscopy. Clin. oral Investig. 22, 505–513. doi:10.1007/s00784-017-2139-6
Gjerde, C., Mustafa, K., Hellem, S., Rojewski, M., Gjengedal, H., Yassin, M. A., et al. (2018). Cell therapy induced regeneration of severely atrophied mandibular bone in a clinical trial. Stem cell Res. Ther. 9, 213. doi:10.1186/s13287-018-0951-9
Global, regional (2018). Global, regional, and national incidence, prevalence, and years lived with disability for 354 diseases and injuries for 195 countries and territories, 1990-2017: A systematic analysis for the global burden of disease study 2017. Lancet 392, 1789–1858. doi:10.1016/S0140-6736(18)32279-7
Grewal, J. S., Yanik, S. C., Strohl-Bryan, A. M., and Tatum, S. A. (2021). The unilateral cleft lip repair. Am. J. otolaryngology 42, 102908. doi:10.1016/j.amjoto.2021.102908
Grosfeld, E. C., Hoekstra, J. W., Herber, R. P., Ulrich, D. J., Jansen, J. A., and van den Beucken, J. J. (2016). Long-term biological performance of injectable and degradable calcium phosphate cement. Biomed. Mater. (Bristol, Engl. 12, 015009.
Guo, R., Chai, L., Chen, L., Chen, W., Ge, L., Li, X., et al. (2015). Stromal cell-derived factor 1 (SDF-1) accelerated skin wound healing by promoting the migration and proliferation of epidermal stem cells. vitro Cell. Dev. Biol. Animal 51, 578–585. doi:10.1007/s11626-014-9862-y
Herford, A. S. (2017). The use of recombinant human bone morphogenetic protein-2 (rhBMP-2) in maxillofacial trauma. Chin. J. Traumatol. 20, 1–3. doi:10.1016/j.cjtee.2016.05.004
Hakim, L. K., Yazdanian, M., Alam, M., Abbasi, K., Tebyaniyan, H., Tahmasebi, E., et al. (2021). Biocompatible and biomaterials application in drug delivery system in oral cavity. Evid. Based Complement. Altern. Med. 2021, 9011226. doi:10.1155/2021/9011226
Han, J., Menicanin, D., Gronthos, S., and Bartold, P. (2014). Stem cells, tissue engineering and periodontal regeneration. Aust. Dent. J. 59, 117–130. doi:10.1111/adj.12100
Han, J., Menicanin, D., Gronthos, S., and Bartold, P. M. (2014). Stem cells, tissue engineering and periodontal regeneration. Aust. Dent. J. 59 (1), 117–130. doi:10.1111/adj.12100
Hassan, K., and Alagl, A. (2015). A comparative study of bovine bone used alone and in combination with transforming growth factor-beta for the treatment of periodontal osseous defects in humans. Saudi J. Med. Med. Sci. 3, 33–39. doi:10.4103/1658-631x.149671
Hayakawa, Y., Sakitani, K., Konishi, M., Asfaha, S., Niikura, R., Tomita, H., et al. (2017). Nerve growth factor promotes gastric tumorigenesis through aberrant cholinergic signaling. Cancer cell 31, 21–34. doi:10.1016/j.ccell.2016.11.005
Hayashi, K., Ochiai-Shino, H., Shiga, T., Onodera, S., Saito, A., Shibahara, T., et al. (2016). Transplantation of human-induced pluripotent stem cells carried by self-assembling peptide nanofiber hydrogel improves bone regeneration in rat calvarial bone defects. BDJ open 2, 15007. doi:10.1038/bdjopen.2015.7
Heasman, L., Stacey, F., Preshaw, P., McCracken, G., Hepburn, S., and Heasman, P. (2006). The effect of smoking on periodontal treatment response: A review of clinical evidence. J. Clin. periodontology 33, 241–253. doi:10.1111/j.1600-051X.2006.00902.x
Hemmat, S., Lieberman, D. M., and Most, S. P. (2010). An introduction to stem cell biology. Facial plast. Surg. FPS 26, 343–349. doi:10.1055/s-0030-1265015
Hixon, K. R., Melvin, A. M., Lin, A. Y., Hall, A. F., and Sell, S. A. (2017). Cryogel scaffolds from patient-specific 3D-printed molds for personalized tissue-engineered bone regeneration in pediatric cleft-craniofacial defects. J. biomaterials Appl. 32, 598–611. doi:10.1177/0885328217734824
Holmstrup, P., Plemons, J., and Meyle, J. (2018). Non-plaque-induced gingival diseases. J. Clin. periodontology 45 (20), S28–S45. doi:10.1002/JPER.17-0163
Horst, J. A. (2018). Silver fluoride as a treatment for dental caries. Adv. Dent. Res. 29, 135–140. doi:10.1177/0022034517743750
Hsieh, K., Murthy, S., Heller, T., Rimmer, J. H., and Yen, G. (2018). Reported gum disease as a cardiovascular risk factor in adults with intellectual disabilities. J. Intellect. Disabil. Res. JIDR 62, 187–198. doi:10.1111/jir.12438
Ibarra, B. A., and Atit, R. (2020). What do animal models teach us about congenital craniofacial defects? Adv. Exp. Med. Biol. 1236, 137–155. doi:10.1007/978-981-15-2389-2_6
Imber, J-C., Roccuzzo, A., Stähli, A., Saulacic, N., Deschner, J., Sculean, A., et al. (2021). Immunohistochemical evaluation of periodontal regeneration using a porous collagen scaffold. Int. J. Mol. Sci. 22, 10915. doi:10.3390/ijms222010915
Jahanbin, A., Rashed, R., Alamdari, D. H., Koohestanian, N., Ezzati, A., Kazemian, M., et al. (2016). Success of maxillary alveolar defect repair in rats using osteoblast-differentiated human deciduous dental pulp stem cells. J. oral Maxillofac. Surg. official J. Am. Assoc. Oral Maxillofac. Surg. 74, 829.e1–9. doi:10.1016/j.joms.2015.11.033
Janssen, N. G., de Ruiter, A. P., van Hout, W., van Miegem, V., Gawlitta, D., Groot, F. B., et al. (2017). “Microstructured β-tricalcium phosphate putty versus autologous bone for repair of alveolar clefts in a goat model,” in The Cleft palate-craniofacial journal (official publication of the American Cleft Palate-Craniofacial Association), 54, 699–706.
Ju, G. S., Son, K. M., Choi, W. Y., and Cheon, J. S. (2019). Clinical usefulness of fixation of absorbable implants with cyanoacrylate in comminuted fractures of the maxilla. Archives craniofacial Surg. 20, 233–238. doi:10.7181/acfs.2019.00325
Kaigler, D., Avila-Ortiz, G., Travan, S., Taut, A. D., Padial-Molina, M., Rudek, I., et al. (2015). Bone engineering of maxillary sinus bone deficiencies using enriched CD90+ stem cell therapy: A randomized clinical trial. J. bone mineral Res. official J. Am. Soc. Bone Mineral Res. 30, 1206–1216. doi:10.1002/jbmr.2464
Kaigler, D., Pagni, G., Park, C. H., Braun, T. M., Holman, L. A., Yi, E., et al. (2013). Stem cell therapy for craniofacial bone regeneration: A randomized, controlled feasibility trial. Cell Transplant. 22, 767–777. doi:10.3727/096368912X652968
Kämmerer, P. W., Scholz, M., Baudisch, M., Liese, J., Wegner, K., Frerich, B., et al. (2017). Guided bone regeneration using collagen scaffolds, growth factors, and periodontal ligament stem cells for treatment of peri-implant bone defects in vivo. Stem cells Int. 2017, 3548435. doi:10.1155/2017/3548435
Kanno, T., Sukegawa, S., Furuki, Y., Nariai, Y., and Sekine, J. (2018). Overview of innovative advances in bioresorbable plate systems for oral and maxillofacial surgery. Jpn. Dent. Sci. Rev. 54, 127–138. doi:10.1016/j.jdsr.2018.03.003
Kato, Y., Iwata, T., Washio, K., Yoshida, T., Kuroda, H., Morikawa, S., et al. (2017). Creation and transplantation of an adipose-derived stem cell (ASC) sheet in a diabetic wound-healing model. J. Vis. Exp. JoVE., 54539. doi:10.3791/54539
Kawai, T., Suzuki, O., Matsui, K., Tanuma, Y., Takahashi, T., and Kamakura, S. (2017). Octacalcium phosphate collagen composite facilitates bone regeneration of large mandibular bone defect in humans. J. tissue Eng. Regen. Med. 11, 1641–1647. doi:10.1002/term.2110
Khojasteh, A., Behnia, H., Hosseini, F. S., Dehghan, M. M., Abbasnia, P., and Abbas, F. M. (2013). The effect of PCL-TCP scaffold loaded with mesenchymal stem cells on vertical bone augmentation in dog mandible: A preliminary report. J. Biomed. Mater. Res. Part B, Appl. biomaterials 101, 848–854. doi:10.1002/jbm.b.32889
Khurshid, Z., Zafar, M. S., Khan, R. S., Najeeb, S., Slowey, P. D., and Rehman, I. U. (2018). Role of salivary biomarkers in oral cancer detection. Adv. Clin. Chem. 86, 23–70. doi:10.1016/bs.acc.2018.05.002
Kim, B. C., Yoon, J. H., Choi, B., and Lee, J. (2013). Mandibular reconstruction with autologous human bone marrow stem cells and autogenous bone graft in a patient with plexiform ameloblastoma. J. craniofacial Surg. 24, e409–e411. doi:10.1097/SCS.0b013e318292c91d
Kim, J. H., Jung, M., Kim, H. S., Kim, Y. M., and Choi, E. H. (2011). Adipose-derived stem cells as a new therapeutic modality for ageing skin. Exp. Dermatol. 20, 383–387. doi:10.1111/j.1600-0625.2010.01221.x
Kinsella, C. R., Cray, J. J., Smith, D. M., Rottgers, S. A., Mooney, M. P., Cooper, G. M., et al. (2012). Novel model of calvarial defect in an infected unfavorable wound: Reconstruction with rhBMP-2. Part II. J. craniofacial Surg. 23, 410–414. doi:10.1097/SCS.0b013e318240feb8
Kiyokawa, K., Kiyokawa, M., Takagi, M., Rikimaru, H., and Fukaya, T. (2009). New regenerative treatment for tooth and periodontal bone defect associated with posttraumatic alveolar bone crush fracture. J. craniofacial Surg. 20, 780–783. doi:10.1097/SCS.0b013e3181a14b7b
Koenen, L., and Waseem, M. (2021). Orbital floor fracture. StatPearls. Treasure Island (FL): StatPearls Publishing Copyright © 2021 StatPearls Publishing LLC.
Koons, G. L., Diba, M., and Mikos, A. G. (2020). Materials design for bone-tissue engineering. Nat. Rev. Mater. 5, 584–603. doi:10.1038/s41578-020-0204-2
Korn, P., Hauptstock, M., Range, U., Kunert-Keil, C., Pradel, W., Lauer, G., et al. (2017). Application of tissue-engineered bone grafts for alveolar cleft osteoplasty in a rodent model. Clin. oral Investig. 21, 2521–2534. doi:10.1007/s00784-017-2050-1
Küseler, A., Heliövaara, A., Mølsted, K., Marcusson, A., Karsten, A., Bellardie, H., et al. (2021). Scandcleft trial of primary surgery for unilateral cleft lip and palate: Craniofacial cephalometrics at 8 years. Eur. J. Orthod. 43, 374–380. doi:10.1093/ejo/cjaa060
Latimer, J. M., Maekawa, S., Yao, Y., Wu, D. T., Chen, M., and Giannobile, W. V. (2021). Regenerative medicine technologies to treat dental, oral, and craniofacial defects. Front. Bioeng. Biotechnol. 9, 704048. doi:10.3389/fbioe.2021.704048
Lew, T. A., Walker, J. A., Wenke, J. C., Blackbourne, L. H., and Hale, R. G. (2010). Characterization of craniomaxillofacial battle injuries sustained by United States service members in the current conflicts of Iraq and Afghanistan. J. oral Maxillofac. Surg. official J. Am. Assoc. Oral Maxillofac. Surg. 68, 3–7. doi:10.1016/j.joms.2009.06.006
Li, J., Li, Y., Ma, S., Gao, Y., Zuo, Y., and Hu, J. (2010). Enhancement of bone formation by BMP-7 transduced MSCs on biomimetic nano-hydroxyapatite/polyamide composite scaffolds in repair of mandibular defects. J. Biomed. Mater. Res. Part A 95, 973–981. doi:10.1002/jbm.a.32926
Li, X., Tang, L., Lin, Y. F., and Xie, G. F. (2018). Role of vitamin C in wound healing after dental implant surgery in patients treated with bone grafts and patients with chronic periodontitis. Clin. implant Dent. Relat. Res. 20, 793–798. doi:10.1111/cid.12647
Li, Z., Liu, X., Zhang, Q., Zhang, J., Huang, M., and Liu, S. (2020). Myxofibrosarcoma of the mandible: A case report and review of the literature. BMC oral health 20, 113. doi:10.1186/s12903-020-01094-7
Liang, Y., Zhao, X., Hu, T., Chen, B., Yin, Z., Ma, P. X., et al. (2019). Adhesive hemostatic conducting injectable composite hydrogels with sustained drug release and photothermal antibacterial activity to promote full-thickness skin regeneration during wound healing. Small (Weinheim der Bergstrasse, Ger. 15, e1900046. doi:10.1002/smll.201900046
Likhterov, I., Roche, A. M., and Urken, M. L. (2019). Contemporary osseous reconstruction of the mandible and the maxilla. Oral Maxillofac. Surg. Clin. N. Am. 31, 101–116. doi:10.1016/j.coms.2018.08.005
Liu, J., Ruan, J., Weir, M. D., Ren, K., Schneider, A., Wang, P., et al. (2019). Periodontal bone-ligament-cementum regeneration via scaffolds and stem cells. Cells 8, 537. doi:10.3390/cells8060537
Liu, K., Gao, Y., Abdelrehem, A., Zhang, L., Chen, X., Xie, L., et al. (2021). Augmented reality navigation method for recontouring surgery of craniofacial fibrous dysplasia. Sci. Rep. 11, 10043. doi:10.1038/s41598-021-88860-x
Liu, X., Li, Q., Wang, F., and Wang, Z. (2016). Maxillary sinus floor augmentation and dental implant placement using dentin matrix protein-1 gene-modified bone marrow stromal cells mixed with deproteinized boving bone: A comparative study in beagles. Archives oral Biol. 64, 102–108. doi:10.1016/j.archoralbio.2016.01.004
Liu, Y., Li, T., Ma, H., Zhai, D., Deng, C., Wang, J., et al. (2018). 3D-printed scaffolds with bioactive elements-induced photothermal effect for bone tumor therapy. Acta biomater. 73, 531–546. doi:10.1016/j.actbio.2018.04.014
Loos, B. G., and Van Dyke, T. E. (2020). The role of inflammation and genetics in periodontal disease. Periodontology 83, 26–39. doi:10.1111/prd.12297
Lopatina, T., Kalinina, N., Karagyaur, M., Stambolsky, D., Rubina, K., Revischin, A., et al. (2011). Adipose-derived stem cells stimulate regeneration of peripheral nerves: BDNF secreted by these cells promotes nerve healing and axon growth de novo. PLoS One 6, e17899. doi:10.1371/journal.pone.0017899
Lynn, J. V., Ranganathan, K., Urlaub, K. M., Luby, A. O., Stephan, C. J., Donneys, A., et al. (2020). Noncultured minimally processed adipose-derived stem cells improve radiated fracture healing. Ann. Plast. Surg. 85, 83–88. doi:10.1097/SAP.0000000000002354
Magnon, C., Hall, S. J., Lin, J., Xue, X., Gerber, L., Freedland, S. J., et al. (2013). Autonomic nerve development contributes to prostate cancer progression. Sci. (New York, NY) 341, 1236361. doi:10.1126/science.1236361
Magrin, G. L., Rafael, S. N. F., Passoni, B. B., Magini, R. S., Benfatti, C. A. M., Gruber, R., et al. (2020). Clinical and tomographic comparison of dental implants placed by guided virtual surgery versus conventional technique: A split-mouth randomized clinical trial. J. Clin. periodontology 47, 120–128. doi:10.1111/jcpe.13211
Mahajan, A., and Kedige, S. (2015). Periodontal bone regeneration in intrabony defects using osteoconductive bone graft versus combination of osteoconductive and osteostimulative bone graft: A comparative study. Dent. Res. J. 12, 25–30. doi:10.4103/1735-3327.150307
Maliha, S. G., Lopez, C. D., Coelho, P. G., Witek, L., Cox, M., Meskin, A., et al. (2020). Bone tissue engineering in the growing calvaria using dipyridamole-coated, three-dimensionally-printed bioceramic scaffolds: Construct optimization and effects on cranial suture patency. Plastic Reconstr. Surg. 145, 337e–47e. doi:10.1097/PRS.0000000000006483
Marconi, S., Castiglione, G., Turano, E., Bissolotti, G., Angiari, S., Farinazzo, A., et al. (2012). Human adipose-derived mesenchymal stem cells systemically injected promote peripheral nerve regeneration in the mouse model of sciatic crush. Tissue Eng. Part A 18, 1264–1272. doi:10.1089/ten.TEA.2011.0491
Martin-Del-Campo, M., Rosales-Ibañez, R., Alvarado, K., Sampedro, J. G., Garcia-Sepulveda, C. A., Deb, S., et al. (2016). Strontium folate loaded biohybrid scaffolds seeded with dental pulp stem cells induce in vivo bone regeneration in critical sized defects. Biomaterials Sci. 4, 1596–1604. doi:10.1039/c6bm00459h
Martín-Del-Campo, M., Rosales-Ibañez, R., and Rojo, L. (2019). Biomaterials for cleft lip and palate regeneration. Int. J. Mol. Sci. 20, 2176. doi:10.3390/ijms20092176
Martin-Piedra, M-A., Gironés-Camarasa, B., España-López, A., Fernández-Valadés Gámez, R., Blanco-Elices, C., Garzón, I., et al. (2021). Usefulness of a nanostructured fibrin-agarose bone substitute in a model of severely critical mandible bone defect. Polym. (Basel) 13, 3939. doi:10.3390/polym13223939
Martín-Piedra, M. A., Alaminos, M., Fernández-Valadés-Gámez, R., España-López, A., Liceras-Liceras, E., Sánchez-Montesinos, I., et al. (2017). Development of a multilayered palate substitute in rabbits: A histochemical ex vivo and in vivo analysis. Histochem. cell Biol. 147, 377–388. doi:10.1007/s00418-016-1489-5
Martínez, M. L., Escario, E., Poblet, E., Sánchez, D., Buchón, F. F., Izeta, A., et al. (2016). Hair follicle-containing punch grafts accelerate chronic ulcer healing: A randomized controlled trial. J. Am. Acad. Dermatology 75, 1007–1014. doi:10.1016/j.jaad.2016.02.1161
Maruyama, T., Jeong, J., Sheu, T-J., and Hsu, W. (2016). Stem cells of the suture mesenchyme in craniofacial bone development, repair and regeneration. Nat. Commun. 7, 10526. doi:10.1038/ncomms10526
Matsumine, H., Numakura, K., Climov, M., Watanabe, Y., Giatsidis, G., and Orgill, D. P. (2017). Facial-nerve regeneration ability of a hybrid artificial nerve conduit containing uncultured adipose-derived stromal vascular fraction: An experimental study. Microsurgery 37, 808–818. doi:10.1002/micr.30060
Matsumine, H., Sasaki, R., Tabata, Y., Matsui, M., Yamato, M., Okano, T., et al. (2016). Facial nerve regeneration using basic fibroblast growth factor-impregnated gelatin microspheres in a rat model. J. tissue Eng. Regen. Med. 10, E559–E567. doi:10.1002/term.1884
Maxim, M. A., Soritau, O., Baciut, M., Bran, S., and Baciut, G. (2015). The role of dental stem cells in regeneration. Clujul Med. 88, 479–482. doi:10.15386/cjmed-475
Mehrabani, D., Khodakaram-Tafti, A., Shaterzadeh-Yazdi, H., Zamiri, B., and Omidi, M. (2018). Comparison of the regenerative effect of adipose-derived stem cells, fibrin glue scaffold, and autologous bone graft in experimental mandibular defect in rabbit. Dent. Traumatol. 34, 413–420.
Meng, X., Wen, Q., Gu, J., and Wang, Y. (2020). Endoscopic endonasal open reduction for fractures of the frontal process of the maxilla. J. Int. Med. Res. 48, 300060520920043. doi:10.1177/0300060520920043
Merkuri, F., and Fish, J. L. (2019). Developmental processes regulate craniofacial variation in disease and evolution, 57. New York, NY: Genesis, e23249.
Mew, M. (2014). Craniofacial dystrophy. A possible syndrome? Br. Dent. J. 216, 555–558. doi:10.1038/sj.bdj.2014.401
Miller, M. Q., McColl, L. F., Arul, M. R., Nip, J., Madhu, V., Beck, G., et al. (2019). Assessment of hedgehog signaling pathway activation for craniofacial bone regeneration in a critical-sized rat mandibular defect. JAMA facial plast. Surg. 21, 110–117. doi:10.1001/jamafacial.2018.1508
Mishra, R., Yadav, D., Tripathi, S., Kandel, L., Baral, P. P., Shubham, S., et al. (2020). Submental intubations in panfacial fractures. Clin. Cosmet. investigational Dent. 12, 41–48. doi:10.2147/CCIDE.S228326
Mohammed, E., Khalil, E., and Sabry, D. (2018). Effect of adipose-derived stem cells and their exo as adjunctive therapy to nonsurgical periodontal treatment: A histologic and histomorphometric study in rats. Biomolecules 8, 167. doi:10.3390/biom8040167
Monje, M., Borniger, J. C., D'Silva, N. J., Deneen, B., Dirks, P. B., Fattahi, F., et al. (2020). Roadmap for the emerging field of cancer neuroscience. Cell 181, 219–222. doi:10.1016/j.cell.2020.03.034
Moreira, M. S., Sarra, G., Carvalho, G. L., Gonçalves, F., Caballero-Flores, H. V., Pedroni, A. C. F., et al. (2021). Physical and biological properties of a chitosan hydrogel scaffold associated to photobiomodulation therapy for dental pulp regeneration: An in vitro and in vivo study. Biomed. Res. Int. 2021, 6684667. doi:10.1155/2021/6684667
Mossaad, A., Badry, T. E., Abdelrahaman, M., Abdelazim, A., Ghanem, W., Hassan, S., et al. (2019). Alveolar cleft reconstruction using different grafting techniques. Open access Macedonian J. Med. Sci. 7, 1369–1373. doi:10.3889/oamjms.2019.236
Mukherjee, K., Ruan, Q., Liberman, D., White, S. N., and Moradian-Oldak, J. (2016). Repairing human tooth enamel with leucine-rich amelogenin peptide–chitosan hydrogel. J. Mater. Res. 31, 556–563. doi:10.1557/jmr.2016.64
Munoz, F., Jiménez, C., Espinoza, D., Vervelle, A., Beugnet, J., and Haidar, Z. (2016). Use of leukocyte and platelet-rich fibrin (L-PRF) in periodontally accelerated osteogenic orthodontics (PAOO): Clinical effects on edema and pain. J. Clin. Exp. Dent. 8, e119–e124. doi:10.4317/jced.52760
Mussano, F., Genova, T., Petrillo, S., Roato, I., Ferracini, R., and Munaron, L. (2018). Osteogenic differentiation modulates the cytokine, chemokine, and growth factor profile of ASCs and SHED. Int. J. Mol. Sci. 19, 1454. doi:10.3390/ijms19051454
Nazir, M., Al-Ansari, A., Al-Khalifa, K., Alhareky, M., Gaffar, B., and Almas, K. (2020). Global prevalence of periodontal disease and lack of its surveillance. TheScientificWorldJournal 2020, 2146160. doi:10.1155/2020/2146160
Novais, A., Lesieur, J., Sadoine, J., Slimani, L., Baroukh, B., Saubaméa, B., et al. (2019). Priming dental pulp stem cells from human exfoliated deciduous teeth with fibroblast growth factor-2 enhances mineralization within tissue-engineered constructs implanted in craniofacial bone defects. Stem Cells Transl. Med. 8, 844–857. doi:10.1002/sctm.18-0182
Nyamsuren, E., Bayarchimeg, B., Urjinlkham, J., Oyun-enkh, P., Kh, O., Batsuuri, M., et al. (2018). Efficacy of natural biopolymer chitosan membrane for guided tissue regeneration. Innovation 12, 16–20.
Nyberg, E. L., Farris, A. L., Hung, B. P., Dias, M., Garcia, J. R., Dorafshar, A. H., et al. (2017). 3D-Printing technologies for craniofacial rehabilitation, reconstruction, and regeneration. Ann. Biomed. Eng. 45, 45–57. doi:10.1007/s10439-016-1668-5
Oh, G. W., Ko, S. C., Je, J. Y., Kim, Y. M., Oh, J., and Jung, W. K. (2016). Fabrication, characterization and determination of biological activities of poly(ε-caprolactone)/chitosan-caffeic acid composite fibrous mat for wound dressing application. Int. J. Biol. Macromol. 93, 1549–1558. doi:10.1016/j.ijbiomac.2016.06.065
Oliver, J. D., Madhoun, W., Graham, E. M., Hendrycks, R., Renouard, M., and Hu, M. S. (2020). Stem cells regenerating the craniofacial skeleton: Current state-of-the-art and future directions. J. Clin. Med. 9, 3307. doi:10.3390/jcm9103307
Oortgiesen, D. A., Walboomers, X. F., Bronckers, A. L., Meijer, G. J., and Jansen, J. A. (2014). Periodontal regeneration using an injectable bone cement combined with BMP-2 or FGF-2. J. tissue Eng. Regen. Med. 8, 202–209. doi:10.1002/term.1514
Osugi, M., Katagiri, W., Yoshimi, R., Inukai, T., Hibi, H., and Ueda, M. (2012). Conditioned media from mesenchymal stem cells enhanced bone regeneration in rat calvarial bone defects. Tissue Eng. Part A 18, 1479–1489. doi:10.1089/ten.TEA.2011.0325
Panny, A., Glurich, I., Haws, R. M., and Acharya, A. (2017). Oral and craniofacial anomalies of bardet-biedl syndrome: Dental management in the context of a rare disease. J. Dent. Res. 96, 1361–1369. doi:10.1177/0022034517716913
Park, B., Jung, B. T., Kim, W. H., Lee, J. H., Kim, B., and Lee, J. H. (2020). The stability of hydroxyapatite/poly-L-lactide fixation for unilateral angle fracture of the mandible assessed using a finite element analysis model. Materials 13.
Park, J. Y., Park, C. H., Yi, T., Kim, S. N., Iwata, T., and Yun, J. H. (2020). rhBMP-2 pre-treated human periodontal ligament stem cell sheets regenerate a mineralized layer mimicking dental cementum. Int. J. Mol. Sci. 21, 3767. doi:10.3390/ijms21113767
Park, S. Y., Kim, K. H., Gwak, E. H., Rhee, S. H., Lee, J. C., Shin, S. Y., et al. (2015). Ex vivo bone morphogenetic protein 2 gene delivery using periodontal ligament stem cells for enhanced re-osseointegration in the regenerative treatment of peri-implantitis. J. Biomed. Mater. Res. Part A 103, 38–47. doi:10.1002/jbm.a.35145
Parmar, P. A., Chow, L. W., St-Pierre, J. P., Horejs, C. M., Peng, Y. Y., Werkmeister, J. A., et al. (2015). Collagen-mimetic peptide-modifiable hydrogels for articular cartilage regeneration. Biomaterials 54, 213–225. doi:10.1016/j.biomaterials.2015.02.079
Patel, K. H., Nayyer, L., and Seifalian, A. M. (2013). Chondrogenic potential of bone marrow-derived mesenchymal stem cells on a novel, auricular-shaped, nanocomposite scaffold. J. tissue Eng. 4, 2041731413516782. doi:10.1177/2041731413516782
Pedroni, A. C. F., Sarra, G., de Oliveira, N. K., Moreira, M. S., Deboni, M. C. Z., and Marques, M. M. (2019). Cell sheets of human dental pulp stem cells for future application in bone replacement. Clin. oral Investig. 23, 2713–2721. doi:10.1007/s00784-018-2630-8
Pine, C. M., Adair, P. M., Burnside, G., Brennan, L., Sutton, L., Edwards, R. T., et al. (2020). Dental RECUR randomized trial to prevent caries recurrence in children. J. Dent. Res. 99, 168–174. doi:10.1177/0022034519886808
Posnick, J. C., and Sami, A. (2015). Use of allogenic (iliac) corticocancellous graft for Le Fort I interpositional defects: Technique and results. J. oral Maxillofac. Surg. official J. Am. Assoc. Oral Maxillofac. Surg. 73, 168.e1–12. doi:10.1016/j.joms.2014.09.002
Pourebrahim, N., Hashemibeni, B., Shahnaseri, S., Torabinia, N., Mousavi, B., Adibi, S., et al. (2013). A comparison of tissue-engineered bone from adipose-derived stem cell with autogenous bone repair in maxillary alveolar cleft model in dogs. Int. J. oral Maxillofac. Surg. 42, 562–568. doi:10.1016/j.ijom.2012.10.012
Prabha, R. D., Kraft, D. C. E., Harkness, L., Melsen, B., Varma, H., Nair, P. D., et al. (2018). Bioactive nano-fibrous scaffold for vascularized craniofacial bone regeneration. J. tissue Eng. Regen. Med. 12, e1537–e1548. doi:10.1002/term.2579
Prajapati, S., Ruan, Q., Mukherjee, K., Nutt, S., and Moradian-Oldak, J. (2018). The presence of MMP-20 reinforces biomimetic enamel regrowth. J. Dent. Res. 97, 84–90. doi:10.1177/0022034517728504
Prajapati, S., Tao, J., Ruan, Q., De Yoreo, J. J., and Moradian-Oldak, J. (2016). Matrix metalloproteinase-20 mediates dental enamel biomineralization by preventing protein occlusion inside apatite crystals. Biomaterials 75, 260–270. doi:10.1016/j.biomaterials.2015.10.031
Pushpoth, S., Tambe, K., and Sandramouli, S. (2008). The use of AlloDerm in the reconstruction of full-thickness eyelid defects. Orbit (Amsterdam, Neth. 27, 337–340. doi:10.1080/01676830802319054
Qian, Y., Zhou, X., Zhang, F., Diekwisch, T. G. H., Luan, X., and Yang, J. (2019). Triple PLGA/PCL scaffold modification including silver impregnation, collagen coating, and electrospinning significantly improve biocompatibility, antimicrobial, and osteogenic properties for orofacial tissue regeneration. ACS Appl. Mater. interfaces 11, 37381–37396. doi:10.1021/acsami.9b07053
Qu, T., Jing, J., Jiang, Y., Taylor, R. J., Feng, J. Q., Geiger, B., et al. (2014). Magnesium-containing nanostructured hybrid scaffolds for enhanced dentin regeneration. Tissue Eng. Part A 20, 2422–2433. doi:10.1089/ten.TEA.2013.0741
Raisi, A., Azizi, S., Delirezh, N., Heshmatian, B., and Amini, K. (2010). Use of chitosan conduit for bridging small-gap peripheral nerve defect in sciatic nerve transection model of rat. Iran. J. Veterinary Surg. 05, 89–100.
Rajan, A., Eubanks, E., Edwards, S., Aronovich, S., Travan, S., Rudek, I., et al. (2014). Optimized cell survival and seeding efficiency for craniofacial tissue engineering using clinical stem cell therapy. Stem Cells Transl. Med. 3, 1495–1503. doi:10.5966/sctm.2014-0039
Rajguru, R. (2013). Role of ENT surgeon in managing battle trauma during deployment. Indian J. Otolaryngol. Head. Neck Surg. 65, 89–94. doi:10.1007/s12070-012-0598-2
Rasperini, G., Pilipchuk, S. P., Flanagan, C. L., Park, C. H., Pagni, G., Hollister, S. J., et al. (2015). 3D-printed bioresorbable scaffold for periodontal repair. J. Dent. Res. 94, 153S–7S. doi:10.1177/0022034515588303
Renz, B. W., Tanaka, T., Sunagawa, M., Takahashi, R., Jiang, Z., Macchini, M., et al. (2018). Cholinergic signaling via muscarinic receptors directly and indirectly suppresses pancreatic tumorigenesis and cancer stemness. Cancer Discov. 8, 1458–1473. doi:10.1158/2159-8290.CD-18-0046
Riccio, M., Maraldi, T., Pisciotta, A., La Sala, G. B., Ferrari, A., Bruzzesi, G., et al. (2012). Fibroin scaffold repairs critical-size bone defects in vivo supported by human amniotic fluid and dental pulp stem cells. Tissue Eng. Part A 18, 1006–1013. doi:10.1089/ten.TEA.2011.0542
Rivera, K. O., Russo, F., Boileau, R. M., Tomlinson, R. E., Miclau, T., Marcucio, R. S., et al. (2020). Local injections of β-NGF accelerates endochondral fracture repair by promoting cartilage to bone conversion. Sci. Rep. 10, 22241. doi:10.1038/s41598-020-78983-y
Rocchietta, I., Simion, M., Hoffmann, M., Trisciuoglio, D., Benigni, M., and Dahlin, C. (2016). Vertical bone augmentation with an autogenous block or particles in combination with guided bone regeneration: A clinical and histological preliminary study in humans. Clin. implant Dent. Relat. Res. 18, 19–29. doi:10.1111/cid.12267
Rogan, D. T., and Fang, A. (2021). Pediatric facial trauma. StatPearls. Treasure Island (FL): StatPearls Publishing Copyright © 2021 StatPearls Publishing LLC.
Rotundo, R., Genzano, L., Nieri, M., Covani, U., Peñarrocha-Oltra, D., and Peñarrocha-Diago, M. (2021). Smile esthetic evaluation of mucogingival reconstructive surgery. Odontology 109, 295–302. doi:10.1007/s10266-020-00544-6
Roy, T., and Reid, R. (2021). A novel approach to coronoidectomy: The modified keen technique. J. craniofacial Surg. 32, 1150–1151. doi:10.1097/SCS.0000000000007275
Ruan, Q., and Moradian-Oldak, J. (2014). Development of amelogenin-chitosan hydrogel for <em>in vitro</em> enamel regrowth with a dense interface. J. Vis. Exp. JoVE., 51606. doi:10.3791/51606
Saadi, R., Oberman, B. S., and Lighthall, J. G. (2018). Dog-bite-related craniofacial fractures among pediatric patients: A case series and review of literature. Craniomaxillofac Trauma Reconstr. 11, 249–255. doi:10.1055/s-0037-1604073
Salenave, S., Boyce, A. M., Collins, M. T., and Chanson, P. (2014). Acromegaly and McCune-Albright syndrome. J. Clin. Endocrinol. metabolism 99, 1955–1969. doi:10.1210/jc.2013-3826
Santos, P. S., Cestari, T. M., Paulin, J. B., Martins, R., Rocha, C. A., Arantes, R. V. N., et al. (2018). Osteoinductive porous biphasic calcium phosphate ceramic as an alternative to autogenous bone grafting in the treatment of mandibular bone critical-size defects. J. Biomed. Mater. Res. Part B, Appl. biomaterials 106, 1546–1557. doi:10.1002/jbm.b.33963
Sanz, M., Marco Del Castillo, A., Jepsen, S., Gonzalez-Juanatey, J. R., D'Aiuto, F., Bouchard, P., et al. (2020). Periodontitis and cardiovascular diseases: Consensus report. J. Clin. periodontology 47, 268–288. doi:10.1111/jcpe.13189
Schlund, M., Nicot, R., Depeyre, A., Alkasbi, J., and Ferri, J. (2019). Reconstruction of a large posttraumatic mandibular defect using bone tissue engineering with fresh-frozen humeral allograft seeded with autologous bone marrow aspirate and vascularized with a radial forearm flap. J. craniofacial Surg. 30, 2085–2087. doi:10.1097/SCS.0000000000005980
Schmidt, V. J., Wietbrock, J. O., Leibig, N., Gloe, T., Henn, D., Hernekamp, J. F., et al. (2017). Collagen-elastin and collagen-glycosaminoglycan scaffolds promote distinct patterns of matrix maturation and axial vascularization in arteriovenous loop-based soft tissue flaps. Ann. Plast. Surg. 79, 92–100. doi:10.1097/SAP.0000000000001096
Schweikle, M., Zinn, T., Lund, R., and Tiainen, H. (2018). Injectable synthetic hydrogel for bone regeneration: Physicochemical characterisation of a high and a low pH gelling system. Mater. Sci. Eng. C, Mater. Biol. Appl. 90, 67–76. doi:10.1016/j.msec.2018.04.049
Seda, M., Geerlings, M., Lim, P., Jeyabalan-Srikaran, J., Cichon, A-C., Scambler, P. J., et al. (2019). An FDA-approved drug screen for compounds influencing craniofacial skeletal development and Craniosynostosis. Mol. Syndromol. 10, 98–114. doi:10.1159/000491567
Shakya, S., Zhang, X., and Liu, L. (2020). Key points in surgical management of mandibular condylar fractures. Chin. J. Traumatol. 23, 63–70. doi:10.1016/j.cjtee.2019.08.006
Shen, Z., Tsao, H., LaRue, S., Liu, R., Kirkpatrick, T. C., Souza, L. C., et al. (2021). Vascular endothelial growth factor and/or nerve growth factor treatment induces expression of dentinogenic, neuronal, and healing markers in stem cells of the apical papilla. J. Endod. 47, 924–931. doi:10.1016/j.joen.2021.02.011
Shin, H. S., Kim, M. S., Kim, B. H., Lim, H. J., Kim, B. C., and Lee, J. (2020). Reconstruction of mandibular defects with bone marrow-derived stem cells in odontogenic myxoma. J. craniofacial Surg. 31, e236–e239. doi:10.1097/SCS.0000000000006147
Shiu, S-T., Lee, W-F., Chen, S-M., Hao, L-T., Hung, Y-T., Lai, P-C., et al. (2021). Effect of different bone grafting materials and mesenchymal stem cells on bone regeneration: A micro-computed tomography and histomorphometric study in a rabbit calvarial defect model. Int. J. Mol. Sci. 22, 8101. doi:10.3390/ijms22158101
Sikora, M., Baranowska-Bosiacka, I., Goschorska, M., and Chlubek, D. (2020). In vitro effect of three-dimensional (3D) titanium mini-plate systems used for surgical treatment of condylar fractures on interleukin 1 (IL-1) and interleukin 6 (IL-6) concentration in THP-1 macrophages. Tissue and cell 67, 101404. doi:10.1016/j.tice.2020.101404
Simon, F., Ketoff, S., Guichard, B., Wolfe, S. A., Tulasne, J. F., Bertolus, C., et al. (2015). Lower jaw reconstruction and dental rehabilitation after war injuries: The experience of Paul Tessier in Iran in the late 1980s. J. cranio-maxillo-facial Surg. 43, 606–610.
Simonpieri, A., Del Corso, M., Sammartino, G., and Dohan Ehrenfest, D. M. (2009). The relevance of choukroun's platelet-rich fibrin and metronidazole during complex maxillary rehabilitations using bone allograft. Part II: Implant surgery, prosthodontics, and survival. Implant Dent. 18, 220–229. doi:10.1097/ID.0b013e31819b5e3f
Singh, V., Garg, A., Bhagol, A., Savarna, S., and Agarwal, S. K. (2019). Photobiomodulation alleviates postoperative discomfort after mandibular third molar surgery. J. oral Maxillofac. Surg. official J. Am. Assoc. Oral Maxillofac. Surg. 77, 2412–2421. doi:10.1016/j.joms.2019.06.009
Smitkarn, P., Subbalekha, K., Mattheos, N., and Pimkhaokham, A. (2019). The accuracy of single-tooth implants placed using fully digital-guided surgery and freehand implant surgery. J. Clin. periodontology 46, 949–957. doi:10.1111/jcpe.13160
Song, J. S., Takimoto, K., Jeon, M., Vadakekalam, J., Ruparel, N. B., and Diogenes, A. (2017). Decellularized human dental pulp as a scaffold for regenerative endodontics. J. Dent. Res. 96, 640–646. doi:10.1177/0022034517693606
Soriano-Lerma, A., Magán-Fernández, A., Gijón, J., Sánchez-Fernández, E., Soriano, M., García-Salcedo, J. A., et al. (2020). Short-term effects of hyaluronic acid on the subgingival microbiome in peri-implantitis: A randomized controlled clinical trial. J. periodontology 91, 734–745. doi:10.1002/JPER.19-0184
Soudi, A., Yazdanian, M., Ranjbar, R., Tebyanian, H., Yazdanian, A., Tahmasebi, E., et al. (2021). Role and application of stem cells in dental regeneration: A comprehensive overview. EXCLI J. 20, 454–489. doi:10.17179/excli2021-3335
Sowmya, S., Mony, U., Jayachandran, P., Reshma, S., Kumar, R. A., Arzate, H., et al. (2017). Tri-layered nanocomposite hydrogel scaffold for the concurrent regeneration of cementum, periodontal ligament, and alveolar bone. Adv. Healthc. Mater. 6, 1601251. doi:10.1002/adhm.201601251
Spicer, P. P., Shah, S. R., Henslee, A. M., Watson, B. M., Kinard, L. A., Kretlow, J. D., et al. (2013). Evaluation of antibiotic releasing porous polymethylmethacrylate space maintainers in an infected composite tissue defect model. Acta biomater. 9, 8832–8839. doi:10.1016/j.actbio.2013.07.018
Srivastava, D., Mishra, S., Chandra, L., and Passi, D. (2019). Mucormycotic osteomyelitis of maxilla following maxillofacial trauma: The disease of the diseased. J. Fam. Med. Prim. Care 8, 748–750. doi:10.4103/jfmpc.jfmpc_410_18
Subramaniam, S., Fang, Y. H., Sivasubramanian, S., Lin, F. H., and Lin, C. P. (2016). Hydroxyapatite-calcium sulfate-hyaluronic acid composite encapsulated with collagenase as bone substitute for alveolar bone regeneration. Biomaterials 74, 99–108. doi:10.1016/j.biomaterials.2015.09.044
Susarla, S. M., Swanson, E., and Gordon, C. R. (2011). Craniomaxillofacial reconstruction using allotransplantation and tissue engineering: Challenges, opportunities, and potential synergy. Ann. Plastic Surg. 67, 655–661. doi:10.1097/SAP.0b013e31822c00e6
Sybil, D., Jain, V., Mohanty, S., and Husain, S. A. (2020). Oral stem cells in intraoral bone formation. J. oral Biosci. 62, 36–43. doi:10.1016/j.job.2019.12.001
Tahmasebi, E., Alam, M., Yazdanian, M., Tebyanian, H., Yazdanian, A., Seifalian, A., et al. (2020). Current biocompatible materials in oral regeneration: A comprehensive overview of composite materials. J. Mater Res. Technol. 9, 11731–11755. doi:10.1016/j.jmrt.2020.08.042
Tang, H., Mattheos, N., Yao, Y., Jia, Y., Ma, L., and Gong, P. (2015). In vivo osteoprotegerin gene therapy preventing bone loss induced by periodontitis. J. periodontal Res. 50, 434–443. doi:10.1111/jre.12224
Teughels, W., Teughels, W., Durukan, A., Ozcelik, O., Pauwels, M., Quirynen, M., et al. (2013). Clinical and microbiological effects of lactobacillus reuteri probiotics in the treatment of chronic periodontitis: A randomized placebo-controlled study. J. Clin. Periodontol. 40, 1025–1035. doi:10.1111/jcpe.12155
Tevlin, R., McArdle, A., Atashroo, D., Walmsley, G. G., Senarath-Yapa, K., Zielins, E. R., et al. (2014). Biomaterials for craniofacial bone engineering. J. Dent. Res. 93, 1187–1195. doi:10.1177/0022034514547271
Thoma, D. S., Bienz, S. P., Payer, M., Hüsler, J., Schmidlin, P. R., Hämmerle, C. H. F., et al. (2019). Randomized clinical study using xenograft blocks loaded with bone morphogenetic protein-2 or autogenous bone blocks for ridge augmentation - a three-dimensional analysis. Clin. oral implants Res. 30, 872–881. doi:10.1111/clr.13492
Thrivikraman, G., Athirasala, A., Twohig, C., Boda, S. K., and Bertassoni, L. E. (2017). Biomaterials for craniofacial bone regeneration. Dent. Clin. N. Am. 61, 835–856. doi:10.1016/j.cden.2017.06.003
Tiffany, A. S., Gray, D. L., Woods, T. J., Subedi, K., and Harley, B. A. C. (2019). The inclusion of zinc into mineralized collagen scaffolds for craniofacial bone repair applications. Acta biomater. 93, 86–96. doi:10.1016/j.actbio.2019.05.031
Trombelli, L., Farina, R., Silva, C. O., and Tatakis, D. N. (2018). Plaque-induced gingivitis: Case definition and diagnostic considerations. J. periodontology 89 (1), S46–s73. doi:10.1002/JPER.17-0576
Tsai, H. C., Li, Y. C., Young, T. H., and Chen, M. H. (2016). Novel microinjector for carrying bone substitutes for bone regeneration in periodontal diseases. J. Formos. Med. Assoc. = Taiwan yi zhi. 115, 45–50. doi:10.1016/j.jfma.2014.10.009
Tsao, C. T., Chang, C. H., Lin, Y. Y., Wu, M. F., Wang, J. L., Young, T. H., et al. (2011). Evaluation of chitosan/γ-poly (glutamic acid) polyelectrolyte complex for wound dressing materials. Carbohydr. Polym. 84, 812–819. doi:10.1016/j.carbpol.2010.04.034
Uswatta, S. P., Okeke, I. U., and Jayasuriya, A. C. (2016). Injectable porous nano-hydroxyapatite/chitosan/tripolyphosphate scaffolds with improved compressive strength for bone regeneration. Mater. Sci. Eng. C, Mater. Biol. Appl. 69, 505–512. doi:10.1016/j.msec.2016.06.089
Van Dyke, T. E., Hasturk, H., Kantarci, A., Freire, M. O., Nguyen, D., Dalli, J., et al. (2015). Proresolving nanomedicines activate bone regeneration in periodontitis. J. Dent. Res. 94, 148–156. doi:10.1177/0022034514557331
Venkatesh, H. S., Morishita, W., Geraghty, A. C., Silverbush, D., Gillespie, S. M., Arzt, M., et al. (2019). Electrical and synaptic integration of glioma into neural circuits. Nature 573, 539–545. doi:10.1038/s41586-019-1563-y
Venkataramani, V., Tanev, D. I., Strahle, C., Studier-Fischer, A., Fankhauser, L., Kessler, T., et al. (2019). Glutamatergic synaptic input to glioma cells drives brain tumour progression. Nature 573, 532–538. doi:10.1038/s41586-019-1564-x
Vhora, I., Lalani, R., Bhatt, P., Patil, S., Patel, H., Patel, V., et al. (2018). Colloidally stable small unilamellar stearyl amine lipoplexes for effective BMP-9 gene delivery to stem cells for osteogenic differentiation. AAPS PharmSciTech 19, 3550–3560. doi:10.1208/s12249-018-1161-6
Visscher, D. O., Farré-Guasch, E., Helder, M. N., Gibbs, S., Forouzanfar, T., van Zuijlen, P. P., et al. (2016). Advances in bioprinting technologies for craniofacial reconstruction. Trends Biotechnol. 34, 700–710. doi:10.1016/j.tibtech.2016.04.001
Vitagliano, T., Curto, L. S., Greto Ciriaco, A., Gareri, P., Ribuffo, D., and Greco, M. (2016). Two-thirds lip defects: A new combined reconstructive technique for patients with epithelial cancer. J. craniofacial Surg. 27, 1995–2000. doi:10.1097/SCS.0000000000003087
Wang, C. C., Wang, C. H., Chen, H. C., Cherng, J. H., Chang, S. J., Wang, Y. W., et al. (2018). Combination of resveratrol-containing collagen with adipose stem cells for craniofacial tissue-engineering applications. Int. wound J. 15, 660–672. doi:10.1111/iwj.12910
Wang, L., Zhang, C., Li, C., Weir, M. D., Wang, P., Reynolds, M. A., et al. (2016). Injectable calcium phosphate with hydrogel fibers encapsulating induced pluripotent, dental pulp and bone marrow stem cells for bone repair. Mater. Sci. Eng. C, Mater. Biol. Appl. 69, 1125–1136. doi:10.1016/j.msec.2016.08.019
Wang, P., Liu, X., Zhao, L., Weir, M. D., Sun, J., Chen, W., et al. (2015). Bone tissue engineering via human induced pluripotent, umbilical cord and bone marrow mesenchymal stem cells in rat cranium. Acta biomater. 18, 236–248. doi:10.1016/j.actbio.2015.02.011
Wang, P., Song, Y., Weir, M. D., Sun, J., Zhao, L., Simon, C. G., et al. (2016). A self-setting iPSMSC-alginate-calcium phosphate paste for bone tissue engineering. Dent. Mater. 32, 252–263.
Wang, Y. T., Huang, S. F., Fang, Y. T., Huang, S. C., Cheng, H. F., Chen, C. H., et al. (2018). Anatomical thin titanium mesh plate structural optimization for zygomatic-maxillary complex fracture under fatigue testing. Biomed. Res. Int. 2018, 9398647. doi:10.1155/2018/9398647
Warnakulasuriya, S., Kujan, O., Aguirre-Urizar, J. M., Bagan, J. V., González-Moles, M., Kerr, A. R., et al. (2021). Oral potentially malignant disorders: A consensus report from an international seminar on nomenclature and classification, convened by the WHO collaborating centre for oral cancer. Oral Dis. 27, 1862–1880. doi:10.1111/odi.13704
Watanabe, Y., Sasaki, R., Matsumine, H., Yamato, M., and Okano, T. (2017). Undifferentiated and differentiated adipose-derived stem cells improve nerve regeneration in a rat model of facial nerve defect. J. tissue Eng. Regen. Med. 11, 362–374. doi:10.1002/term.1919
Wiley, D. S., Redfield, S. E., and Zon, L. I. (2017). Chemical screening in zebrafish for novel biological and therapeutic discovery. Methods cell Biol. 138, 651–679. doi:10.1016/bs.mcb.2016.10.004
Wilkes, G. H., Wong, J., and Guilfoyle, R. (2014). Microtia reconstruction. Plastic Reconstr. Surg. 134, 464e–79e. doi:10.1097/PRS.0000000000000526
Wu, D. T., Munguia-Lopez, J. G., Cho, Y. W., Ma, X., Song, V., Zhu, Z., et al. (2021). Polymeric scaffolds for dental, oral, and craniofacial regenerative medicine. Molecules 26, 7043. doi:10.3390/molecules26227043
Wu, M., Wang, J., Zhang, Y., Liu, H., and Dong, F. (2018). Mineralization induction of gingival fibroblasts and construction of a sandwich tissue-engineered complex for repairing periodontal defects. Med. Sci. Monit. 24, 1112–1123. doi:10.12659/msm.908791
Xia, Y., Chen, H., Zhang, F., Bao, C., Weir, M. D., Reynolds, M. A., et al. (2018). Gold nanoparticles in injectable calcium phosphate cement enhance osteogenic differentiation of human dental pulp stem cells. Nanomedicine Nanotechnol. Biol. Med. 14, 35–45. doi:10.1016/j.nano.2017.08.014
Xie, Z., Paras, C. B., Weng, H., Punnakitikashem, P., Su, L. C., Vu, K., et al. (2013). Dual growth factor releasing multi-functional nanofibers for wound healing. Acta biomater. 9, 9351–9359. doi:10.1016/j.actbio.2013.07.030
Xu, R., Zhou, C., Zhang, Y., Zhang, S., Xie, J., and Yuan, Q. (2021). Challenges of stem-cell-based craniofacial regeneration. Curr. stem cell Res. Ther. 16, 670–682. doi:10.2174/1574888X16999210128193910
Yamada, Y., Nakamura, S., Ito, K., Umemura, E., Hara, K., Nagasaka, T., et al. (2013). Injectable bone tissue engineering using expanded mesenchymal stem cells. Stem Cells 31, 572–580. doi:10.1002/stem.1300
Yamaguchi, H., Terajima, M., Kitami, M., Wang, J., He, L., Saeki, M., et al. (2020). IFT20 is critical for collagen biosynthesis in craniofacial bone formation. Biochem. biophysical Res. Commun. 533, 739–744. doi:10.1016/j.bbrc.2020.09.033
Yang, M. L., Zhang, B., Zhou, Q., Gao, X. B., Liu, Q., and Lu, L. (2013). Minimally-invasive open reduction of intracapsular condylar fractures with preoperative simulation using computer-aided design. Br. J. oral Maxillofac. Surg. 51, e29–e33. doi:10.1016/j.bjoms.2012.03.005
Yazdanian, M., Arefi, A. H., Alam, M., Abbasi, K., Tebyaniyan, H., Tahmasebi, E., et al. (2021). Decellularized and biological scaffolds in dental and craniofacial tissue engineering: A comprehensive overview. J. Mater Res. Technol. 15, 1217–1251. doi:10.1016/j.jmrt.2021.08.083
Yazdanian, M., Rahmani, A., Tahmasebi, E., Tebyanian, H., Yazdanian, A., and Mosaddad, S. A. (2021). Current and advanced nanomaterials in dentistry as regeneration agents: An update. Mini-Rev Med. Chem. 21, 899–918. doi:10.2174/1389557520666201124143449
Yazdanian, M., Rostamzadeh, P., Rahbar, M., Alam, M., Abbasi, K., Tahmasebi, E., et al. (2022). The potential application of green-synthesized metal nanoparticles in dentistry: A comprehensive review. Bioinorg. Chem. Appl. 2022, 2311910. doi:10.1155/2022/2311910
Yazdanian, M., Tabesh, H., Houshmand, B., Tebyanian, H., Soufdoost, R. S., Tahmasebi, E., et al. (2020). Fabrication and properties of βtcp/zeolite/gelatin scaffold as developed scaffold in bone regeneration: In vitro and in vivo studies. Biocybern. Biomed. Eng. 40, 1626–1637. doi:10.1016/j.bbe.2020.10.006
Yilmaz, E., Mihci, E., Nur, B., Alper Ö, M., and Taçoy, Ş. (2019). Recent advances in Craniosynostosis. Pediatr. Neurol. 99, 7–15. doi:10.1016/j.pediatrneurol.2019.01.018
Yoshida, K., Sumita, Y., Marukawa, E., Harashima, M., and Asahina, I. (2013). Effect of platelet-rich plasma on bone engineering with an alloplastic substitute containing BMP2. Bio-medical Mater. Eng. 23, 163–172. doi:10.3233/BME-130741
Yoshpe, M., Kaufman, A. Y., Lin, S., and Ashkenazi, M. (2021). Regenerative endodontics: A promising tool to promote periapical healing and root maturation of necrotic immature permanent molars with apical periodontitis using platelet-rich fibrin (PRF). Eur. archives Paediatr. Dent. official J. Eur. Acad. Paediatr. Dent. 22, 527–534. doi:10.1007/s40368-020-00572-4
Zaky, S. H., Lee, K. W., Gao, J., Jensen, A., Verdelis, K., Wang, Y., et al. (2017). Poly (glycerol sebacate) elastomer supports bone regeneration by its mechanical properties being closer to osteoid tissue rather than to mature bone. Acta biomater. 54, 95–106. doi:10.1016/j.actbio.2017.01.053
Zhai, P., Peng, X., Li, B., Liu, Y., Sun, H., and Li, X. (2020). The application of hyaluronic acid in bone regeneration. Int. J. Biol. Macromol. 151, 1224–1239. doi:10.1016/j.ijbiomac.2019.10.169
Zhang, C., Yan, B., Cui, Z., Cui, S., Zhang, T., Wang, X., et al. (2017). Bone regeneration in minipigs by intrafibrillarly-mineralized collagen loaded with autologous periodontal ligament stem cells. Sci. Rep. 7, 10519. doi:10.1038/s41598-017-11155-7
Zhang, W., Zhu, C., Ye, D., Xu, L., Zhang, X., Wu, Q., et al. (2014). Porous silk scaffolds for delivery of growth factors and stem cells to enhance bone regeneration. PLoS One 9, e102371. doi:10.1371/journal.pone.0102371
Glossary
3D Three-dimensional
ADSC Adipose-derived stem cells
AFD Acrofacial dysostoses
AFT Autologous fat transfer
AM Additive manufacturing
A-PRF Advanced platelet-rich fibrin
AR Augmented reality
ARP Alveolar ridge preservation
AV Arteriovenous
bFGF Basic fibroblast growth factor
BGC Bioactive glass-ceramic
BMMSC Bone marrow mononuclear cell
BMP Bone morphogenetic protein
BMSC Bone marrow stromal cell
CAF Coronally advanced flap
CaP Calcium phosphate
CBCT Cone-beam computed tomography
CFD Craniofacial defect
CH Chitosan
CLP Cleft lip and palate
CMF Craniomaxillofacial
CMX Collagen matrix
CPC Calcium phosphate cement
CSF Cerebrospinal fluid
CTA Composite tissue allotransplantation
DM Diabetes mellitus
DPSC Dental pulp stem cells
ECM Extracellular matrix
EGF Epidermal growth factor
ESC Embryonic stem cells
FD Fibrous dysplasia
FFPM Fractures of the frontal process of the maxilla
FGF Fibroblast growth factor
FPM Frontal process of the maxilla
GA General anesthesia
HA Hyaluronic acid
HFA High-frequency acceleration
IGF-I Insulin-like growth factor-I
IL Interleukin
iPSC Induced pluripotent stem cell
MBCaP Macro biphasic calcium phosphate
MF Maxillofacial
MMP Matrix metalloproteinase
MSC Mesenchymal stem cell
MTA Mineral trioxide aggregates
NGF Nerve growth factor
PDLSC Periodontal ligament stem cell
OPG Osteoprotegerin
OPMD Oral potentially malignant disorders
PCL Poly(ε-caprolactone)
PDMS Polydimethylsiloxane
PLAGA Poly(lactic-co-glycolic acid)
POH Progressive osseus heteroplasia
PPF Poly(propylene fumarate)
PRF Platelet-rich fibrin
PRP Platelet-rich plasma
PTE Potentially traumatic event
RSV Resveratrol
SCAP Stem cells from apical papilla
SHED Stem cells from human-exfoliated deciduous teeth
TBF Temporal bone fracture
TCA Trichloroacetic acid
TE Trace element
TRC Tissue repair cell
TSPSC Tissue-specific progenitor stem cell
VEGF Vascular endothelial growth factor
VSP Virtual surgical planning
WHO World Health Organization.
Keywords: regenerative medicine, mandible, maxilla, disease, craniofacial
Citation: Tahmasebi E, Mohammadi M, Alam M, Abbasi K, Gharibian Bajestani S, Khanmohammad R, Haseli M, Yazdanian M, Esmaeili Fard Barzegar P and Tebyaniyan H (2023) The current regenerative medicine approaches of craniofacial diseases: A narrative review. Front. Cell Dev. Biol. 11:1112378. doi: 10.3389/fcell.2023.1112378
Received: 30 November 2022; Accepted: 08 February 2023;
Published: 28 February 2023.
Edited by:
Mariappan Rajan, Madurai Kamaraj University, IndiaReviewed by:
Lei Hu, Beijing Stomatological Hospital, Capital Medical University, ChinaHuang Li, Nanjing University, China
Copyright © 2023 Tahmasebi, Mohammadi, Alam, Abbasi, Gharibian Bajestani, Khanmohammad, Haseli, Yazdanian, Esmaeili Fard Barzegar and Tebyaniyan. This is an open-access article distributed under the terms of the Creative Commons Attribution License (CC BY). The use, distribution or reproduction in other forums is permitted, provided the original author(s) and the copyright owner(s) are credited and that the original publication in this journal is cited, in accordance with accepted academic practice. No use, distribution or reproduction is permitted which does not comply with these terms.
*Correspondence: Mohsen Yazdanian, myazdaniandr@gmail.com; Hamid Tebyaniyan, tebyan.hamid@yahoo.com