- 1The First School of Clinical Medicine, Gannan Medical University, Ganzhou, China
- 2Department of Otolaryngology, First Affiliated Hospital of Gannan Medical University, Ganzhou, China
- 3Laboratory Medicine, First Affiliated Hospital of Gannan Medical University, Ganzhou, China
- 4Precision Medicine Center, First Affiliated Hospital of Gannan Medical University, Ganzhou, China
Nasopharyngeal carcinoma (NPC) is a malignant tumor originating from the epithelium of the nasopharynx. The disease is insidious, and most patients are diagnosed at the advanced stage, resulting in poor prognosis. Early diagnosis is important to reduce NPC mortality. Small extracellular vesicles (sEVs) are rich in a variety of bioactive molecules, such as proteins, nucleic acids, and lipids, which can participate in the physiological and pathological regulation of the body by affecting the function of target cells. Numerous studies have shown that some RNAs and proteins in sEVs of tumor origin have a key role in the development of NPC and are potential candidates for malignancy detection. Studying the relationship between the cargoes of these sEVs and NPC may help in the diagnosis of the disease. Here in this review, we summarize the application of sEVs as biomarkers in the diagnosis of NPC and their role in NPC metastasis and prognosis. In addition, we discuss possible future applications and limitations of sEVs as biomarkers.
Introduction
Nasopharyngeal carcinoma (NPC) is a malignancy with strong ethnic and regional characteristics. It is very common in Southeast Asia, East Asia, and North Africa. It occurs at a peak age of > 45 years and is two to three times more common in men than in women (Xu et al., 2015; Chen Y. P. et al., 2019; Zhu et al., 2020). The World Health Organization has classified NPC into the following three subtypes based on histology: keratinizing squamous cell carcinoma, non-keratinizing carcinoma (either differentiated or undifferentiated), and basaloid carcinoma (Chua et al., 2016; Tang et al., 2021). Keratinizing squamous cell carcinoma is associated with Epstein–Barr virus (EBV) infection in approximately 70%–80% of the cases. Almost all cases of non-keratinizing carcinoma (either differentiated or undifferentiated) are related to EBV and occur in areas where EBV is endemic (Sinha and Gajra, 2022). Research suggests that differences in diet, lifestyle, and exposure to harmful environmental factors may be the root cause of geographic differences in the incidence of NPC (Chang et al., 2017; Lee et al., 2019; Xu et al., 2019). In 2020, approximately 133,000 people were diagnosed with NPC, and there were approximately 80,000 NPC-related deaths (a mortality rate of approximately 60%) (Sung et al., 2021). Due to the lack of effective early diagnostic indicators, most patients are diagnosed at an advanced stage and are prone to distant metastasis (Guinot et al., 2020; Brody-Camp et al., 2021). Therefore, early and accurate diagnosis is of great importance for the prevention and treatment of NPC.
Currently, screening for NPC is based on serum EBV antibody assays, plasma EBV DNA tests, imaging, and tissue biopsies (Lam et al., 2018; Mao et al., 2021; Tang et al., 2021). However, these methods have some limitations in diagnosing patients with early NPC. Serum EBV antibody tests are only effective in EBV-positive NPC patients, and there is no internationally accepted standardized procedure for plasma EBV DNA testing. Moreover, the origin of EBV DNA in the blood is not known. Imaging is only available for patients with no contraindications to imaging. Tissue biopsies are invasive, and their accuracy depends on the skill and experience of the operator (Chua et al., 2016; Chan et al., 2017; Lam and Chan, 2018; Sun et al., 2019). Therefore, non-invasive and more effective early diagnostic biomarkers for NPC are urgently needed.
Extracellular vesicle (EV) as the generic term for particles naturally released from the cell. EVs are classified into small EVs (sEVs, diameter: <200 nm), medium/large EVs (m/lEVs, diameter: >200 nm) according to their size. sEVs formed by the fusion of multivesicular bodies (MVBs) with the plasma membrane can be actively secreted by healthy, malignant, and virus-infected cells (Théry et al., 2018; van Niel et al., 2018; Xiao et al., 2019). All cells, including blood cells, immune cells, cancer cells, and stem cells, can release sEVs into various body fluids, including blood, urine, breast milk, ascitic fluid, amniotic fluid, saliva, and cerebrospinal fluid (Raposo and Stoorvogel, 2013; Teow et al., 2017; Xunian and Kalluri, 2020). sEVs are rich in proteins, nucleic acids, lipids, and other bioactive substances, and these active molecules reshape the tumor microenvironment and participate in the development of NPC (Juan and Fürthauer, 2018; Liu et al., 2021; Luo and Yi, 2021). Tumor-derived sEVs contain a variety of RNAs, such as microRNA (miRNA), long non-coding RNA (lncRNA), and circular RNA (circRNA) (Valadi et al., 2007). These RNAs hold great promise for diagnostic monitoring, prognostic assessment, and immunotherapy of NPC (Duan et al., 2019; Jiang et al., 2021; Liu et al., 2021).
sEVs are rich in proteins, including a wide range of transmembrane proteins, lipid-anchored membrane proteins, peripherally associated membrane proteins, and soluble proteins. Some proteins may have important roles in the early diagnosis, prognosis, and treatment of NPC (Pegtel and Gould, 2019; Hu et al., 2022). Studies have shown that the EBV-encoded latent membrane protein 1 (LMP1) is expressed in NPC cells and enhances the radioresistance of NPC cells possibly by affecting the infected host and modulating the tumor microenvironment (Zhang et al., 2019). Moreover, LMP1 increase the expression of syndecan-2 (SDC2) and synaptotagmin-4-like (SYTL4) through nuclear factor-κB (NF-κB) signaling and improve sEV formation and secretion (Liao et al., 2020). It has also been reported that CD63, a conserved tetraspanin protein, increases the LMP1-mediated release of sEVs (Hurwitz et al., 2017; Han et al., 2021). Increased number of sEVs result in P38 MAPK signaling activation in recipient cells, promoting the proliferation of recipient NPC cells and tumor growth (Zhang et al., 2019; Wu et al., 2020; Lo et al., 2021). Here, we summarize the relationship between sEVs and NPC and the application of sEV miRNAs and proteins in the diagnosis and prognosis of NPC.
sEVs and EBV-associated NPC
sEVs are generated within a subpopulation of MVBs. When sEVs are mature, MVBs are transported to the plasma membrane through microtubules, docking, and membrane fusion, resulting in sEV release into the extracellular space. sEVs influence cells in the microenvironment by interacting with the extracellular matrix. sEVs can also enter the circulation via lymph or blood (Bebelman et al., 2018; Théry et al., 2018; Verweij et al., 2018). In the tumor microenvironment, sEVs are key mediators of intercellular communication, regulating the pathophysiological processes of tumors (Zhang and Yu, 2019; Shehzad et al., 2021). NPC-derived sEVs significantly induce macrophages to produce the inflammatory cytokine interleukin-6 (IL-6), which activates signal transducer and activator of transcription 3 (STAT3) to promote malignant behavior of NPC cells (Han et al., 2021; Wang et al., 2021; Chen et al., 2022), such as immune escape, angiogenesis, and metastasis (Vader et al., 2014). Similarly, viruses can be transmitted through sEVs. In EBV-infected patients, EBV particles are transmitted between B cells and epithelial cells through sEVs, and specific RNAs and proteins are incorporated in sEVs to regulate intercellular signaling (Meckes et al., 2010; Raab-Traub and Dittmer, 2017; Zhao et al., 2019) (Figure 1). EBV-encoded LMP1 promotes NPC cell metastasis through store-operated calcium entry (SOCE) conduction of cytoplasmic calcium ions (Wei et al., 2015; Wei et al., 2020).
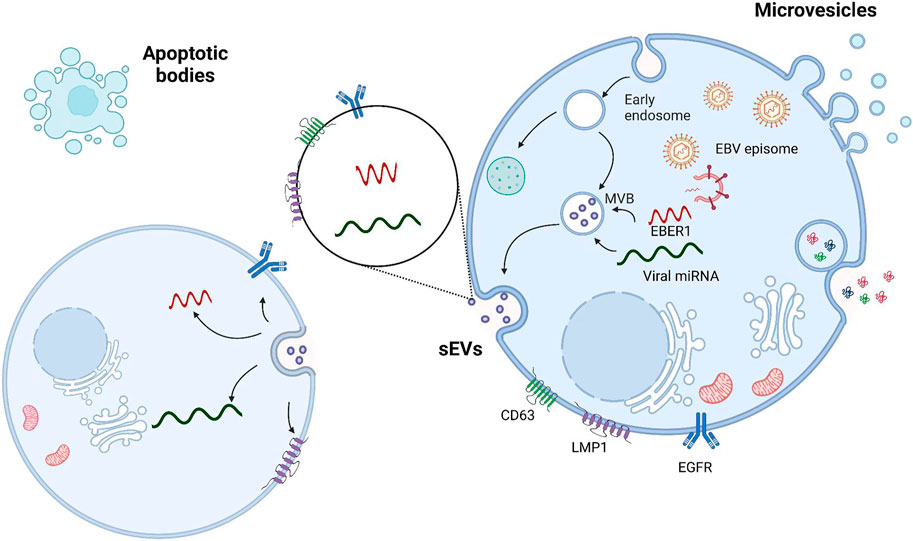
FIGURE 1. Viral particles and viral RNA are transported by sEVs from infected cells to uninfected cells. sEVs are formed by the fusion of MVBs with cell membranes; microvesicles are formed by the direct outgrowth of cell membranes; and apoptotic vesicles are formed by the shrinkage and fragmentation of apoptotic cells. The interaction between LMP1 and the tetraspanin CD63 may contribute to the selective incorporation of LMP1 into sEVs.
Many miRNAs in tumor-derived sEVs can promote angiogenesis in NPC through different pathways. For example, Studies have shown that miR-9 in sEVs is strongly associated with NPC patients’ prognosis and survival. miR-9 can directly inhibit the expression of its target gene midkine (MDK) in endothelial cells, and it can inhibit endothelial angiogenesis by regulating the PDK/AKT signaling pathway (Lu et al., 2018; Ramayanti et al., 2019; Wang et al., 2020a). miR-17-5p promotes neoangiogenesis in NPC by downregulating bone morphogenetic protein and activin membrane-bound inhibitor (BAMBI) and regulating AKT/vascular endothelial growth factor A (VEGF-A) signaling (Duan et al., 2019). miR-23a plays an important role in mediating angiogenesis by targeting testis-specific gene antigen 10 (TSGA10) (Bao et al., 2018). In addition, limb-bud and heart (LBH) of sEVs inhibits epithelial–mesenchymal transition (EMT) progression and angiogenesis in the NPC microenvironment. This is mainly achieved by regulating VEGF-A expression and secretion and associated signaling (Bebelman et al., 2018; Luo and Yi, 2021; Wu et al., 2022). sEV-derived EBV-encoded small RNAs (EBERs) regulate vascular cell adhesion molecule 1 (VCAM-1) expression via TLR3/RIG-I to induce angiogenesis (Ahmed et al., 2014; Cheng et al., 2019; Li et al., 2019). Hematopoietic cell-specific substrate protein 1-associated protein X-1 (HAX1) and phosphofructo-2-kinase/fructose-2,6-bisphosphatase 3 (PFKFB3), which are abundant in sEVs secreted by NPC cells, promote angiogenesis and accelerate the growth of NPC in vitro and in vivo (You et al., 2016; Gu et al., 2017). Thus, sEVs are closely associated with EBV transmission in humans, promoting the development of EBV-associated NPC and influencing the metastasis and prognosis of NPC.
sEVs as diagnostic biomarkers for NPC
During the latent phase of NPC infection, EBV releases various products, including Epstein–Barr nuclear antigen 1 (EBNA1), LMP1, lncRNAs (BARTs), small RNAs (EBERs), and miRNAs (EBV-miR-BART) (Tsao et al., 2017; Lo et al., 2021). Many studies have shown that miRNA, RNA, and proteins from sEVs of different origins have important roles in patients with NPC and that these molecules may serve as diagnostic indicators (Rahbarghazi et al., 2019; Liao et al., 2021; Lo et al., 2021) (Figure 2).
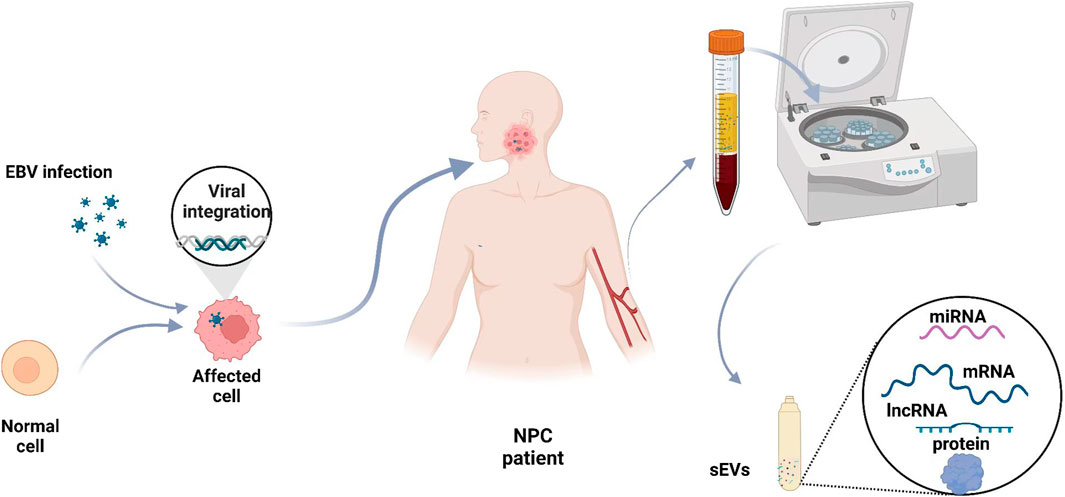
FIGURE 2. Blood small extracellular vesicles as diagnostic biomarkers for NPC. The levels of some sEV cargoes, such as miRNAs, mRNAs, lncRNAs, and proteins, are abnormal in NPC patients. Blood samples are taken from NPC patients, and sEVs are isolated to analyze the levels of various molecules.
sEV miRNAs
miRNAs are a small, highly conserved class of non-coding RNAs that can be used to diagnose NPC with high accuracy and specificity (Zhang and Lu, 2019; Kang et al., 2021; Liao et al., 2021). Studies have shown that sEVs of EBV BART-microRNAs secreted by NPC cells can spread from tumor sites into the peripheral blood. sEVs are sufficiently stable in blood and can transmit information through miRNAs, which may serve as novel tumor biomarkers (Wang et al., 2017; Di Santo et al., 2022; Lin et al., 2022).
Zou et al. (2020) identified five miRNAs that were significantly upregulated in EVs secreted by patients with NPC (let-7b-5p, miR-140-3p, miR-192-5p, miR-223-3p, and miR-24-3p), constituting a signature for NPC diagnosis with high sensitivity and specificity. Li et al. performed a comprehensive study of sEV-derived miRNAs and identified three miRNAs (miR-134-5p, miR-205-5p, and miR-409-3p) constituting a signature for NPC diagnosis (Jiang et al., 2021). Previous studies have shown that miR-205-5p is upregulated in NPC tissue or plasma; miR-205-5p is considered a potential diagnostic biomarker (Luan et al., 2016; Zhang et al., 2020). It was found that circulating miR-409-3p could serve as a biomarker for lung adenocarcinoma and prostate cancer (Wang et al., 2020b; Karadag et al., 2021). Jiang et al. (2021) conducted gene sequencing, selected seven candidate miRNAs, performed Gene Ontology and Kyoto Encyclopedia of Genes and Genomes pathway enrichment analyses, and constructed UpSet plots and assessment scales. Finally, they developed a model with an area under the receiver operating characteristic (ROC) curve value of 0.91, a sensitivity of 90%, and a specificity of 80%. In addition, their model can distinguish between patients with NPC at different clinical stages or with different EBV infection status and healthy people (Jiang et al., 2021). Ramayanti et al. (2019) found that BRAT13-3p, which binds to sEVs, is a promising biomarker in hematologic minimally invasive diagnostics, with an area under curve (AUC) value of 0.9 for BART13-3p miRNA in NPC patients. They identified patients with endemic and non-endemic nasopharyngeal cancer by measuring serum EV-bound BART13-3p levels, which could even be used as part of a screening strategy to diagnose NPC in endemic areas. In conclusion, these studies suggest that some miRNAs in sEVs can be used for the early diagnosis of NPC.
sEV proteins
Many studies have shown differences in the levels of proteins in blood sEVs of NPC patients. An earlier proteomic analysis of plasma sEVs in NPC patients showed upregulation of 51 proteins and downregulation of 89 proteins (Chan et al., 2015). sEVs from EBV-infected NPC cells contain hypoxia-induced factor-1α (HIF-1α) and LMP1, which can accelerate tumor development and metastasis. LMP1 has been shown to indirectly affect the composition of sEVs (Meckes et al., 2013; Teow et al., 2017). In addition, studies have identified both LMP1 and LMP2A, which are encoded by EBV, in EBV-infected cells. A study developed a model, called EVsum5, based on the combination of LMP1, LMP2A, and the tumor markers programmed death 1 (PD-L1), epidermal growth factor receptor (EGFR), and epithelial cell adhesion molecule (EpCAM) to identify five EV subpopulations. Furthermore, the study concluded that EVsum5 performed best in NPC diagnosis, with an AUC value of 1.0, a sensitivity of 100%, and a specificity of 100% (Hu et al., 2022).
In addition, studies have shown that the basic leucine zipper ATF-like transcription factor 2 (BATF2) gene is associated with a variety of malignant mechanisms. Immunohistochemistry (IHC) microarrays have been performed to determine the diagnostic value of BATF2 protein expression in NPC tissue. The results showed that BATF2 is downregulated in NPC, and the performance of serum and sEV BATF2 levels in the diagnosis of NPC was assessed using receiver operating characteristic (ROC) curve analysis. Interestingly, the sensitivity, specificity, and AUC value of plasma-derived sEV BATF2 in distinguishing between NPC patients and healthy controls were 81%, 82%, and 0.8983, respectively (Cui et al., 2021). In addition, several studies have shown that CD109 is highly expressed in both NPC cell lines and tumor tissues (Zhou et al., 2019). Applying the novel aptamer-CRISPR/Cas12a assay, Li et al. demonstrated that CD109+ EV and EGFR+ EV levels are much higher in NPC supernatant and plasma than in their normal counterparts. It is suggested that serum CD109+ and EGFR+ EVs could be used as biomarkers for nasopharyngeal cancer. A probability curve was constructed for the combination of CD109+ and EGFR+ tumor-derived EVs based on binary logistic regression (Logit (<b12>p = NPC) = −13.348 + 0.002 CD109 + 0.004 EGFR), with an AUC of 0.934, a sensitivity of 84.1%, and a specificity of 85% for NPC versus healthy subjects (Jia et al., 2016; Sakakura et al., 2016; Sunagawa et al., 2016; Mii et al., 2019; Li H. et al., 2021). Circulating EV procyclin A (CYPA), with peptidyl prolyl cis-trans isomerase (PPIase) activity, is involved in protein folding and transport, binds to membrane receptors or intracellular membrane chaperones, and activates downstream signaling pathways (Saleh et al., 2016). sEVs have much higher CYPA levels than plasma and are novel and promising biomarkers for NPC, with an AUC value of 0.844; combining CYPA protein analysis with EBV-VCA-IgA antibody assays can significantly improve the diagnosis of nasopharyngeal cancer (Yang et al., 2014; Liu et al., 2019).
Plasma sEVs in the prognosis of NPC
Previous studies have found that the occurrence and development of NPC are closely associated with differentially expressed RNAs and proteins in sEVs. These molecules can influence the occurrence, metastasis, chemoresistance, and recurrence of NPC and are expected to serve as potential diagnostic markers (Wang et al., 2017).
Confirmation of metastasis
Metastasis is not only a major obstacle to the clinical management of NPC but also a major cause of death in patients with NPC. Early diagnosis of NPC is important to improve patient survival.
It has been shown that overexpression of miR-34c in sEVs inhibits the development of NPC by targeting β-catenin, which is involved in proliferation, migration, invasion, and EMT (Okada et al., 2019; Wan et al., 2020). Lu et al. (2018) showed that sEVs overexpressing miR-9 inhibit angiogenesis and metastasis in NPC by targeting the pro-angiogenic protein MDK and regulating the PDK/AKT signaling pathway. In addition, another study showed that the glycolytic regulator PFKFB3 is overexpressed in NPC and that sEVs associated with NPC are highly enriched in PFKFB3, which could activate the ERK/AKT pathway and affect the proliferation, migration, and apoptosis of NPC cells (Gu et al., 2017). Hypoxic NPC cells secrete sEVs containing HIF-1α-activated matrix metalloproteinase-13 (MMP-13), which enhances migration and invasiveness, induces microenvironmental changes, and promotes NPC invasiveness (You et al., 2015). Yin et al. (2021) found that HIF-1α in adipocytes promotes CNE-2 cell metastasis by inhibiting miR-433-3p expression in hypoxic adipocyte-derived EVs (Shan et al., 2018). Furthermore, it has been demonstrated that EGFR is highly expressed in tumor tissues from distant metastatic NPC patients and is associated with a reduction in reactive oxygen species (ROS). Importantly, EGFR-enriched EVs promote the metastatic potential of NPC cells by downregulating intracellular ROS levels via the PI3K/AKT pathway (Li et al., 2020). sEVs secreted by NPC cells are rich in HAX1, which can be an important biomarker for NPC metastasis. HAX1 is associated with lymph node metastasis, metastasis classification, clinical staging, and poor prognosis. HAX1 regulates the focal adhesion kinase (FAK) pathway, affects microvascular formation, and promotes NPC metastasis by increasing the translation efficiency of integrin β6 (ITGB6) (You et al., 2016; You et al., 2022).
Identification of chemoradiotherapy resistance
Nasopharyngeal cancer treatment includes surgery and non-surgical treatment modalities. Non-surgical treatments include radiation therapy, radiation plus chemotherapy, and molecular targeted therapy. Since NPC is highly sensitive to radiotherapy and chemotherapy, the main treatment for patients with early and locally advanced NPC is single radiotherapy or combined chemotherapy and radiotherapy (Adelstein et al., 2017; Colevas et al., 2018; Guan et al., 2020). In most cases, early-stage NPC requires only radiation therapy (Chen L. et al., 2019). The main obstacle to radiation therapy is inherent and acquired radiation resistance of cancer cells. Chemotherapy resistance is also a major obstacle in curing patients with recurrent NPC (Guan et al., 2020; Shan et al., 2022). Cisplatin is one of the commonly used chemotherapeutic agents for NPC and many other cancers (Riddell, 2018). Expression levels of miR-106a-5p in sEVs are significantly increased in the last cycle of cisplatin-based chemotherapy, and miR-106a-5p is enriched in cisplatin-resistant cell-derived EVs and promotes cisplatin resistance in NPC cells in vivo by regulating the ARNT2/AKT axis (Li J. et al., 2021). Recently, it has been shown that sEV endoplasmic reticulum-resident protein 44 (ERp44), which is produced upon endoplasmic reticulum (ER) stress in NPC cells, is involved in resistance to chemotherapy with platinum drugs, suggesting that ERp44 might be a new therapeutic target (Xia et al., 2021).
CircMYC, a newly identified circRNA in circulating sEVs of NPC patients, is significantly expressed in radiotherapy-resistant cells, and knockdown of circMYC increases the radiosensitivity of cells, suggesting that circMYC overexpression contributes to radiotherapy resistance. Luo et al. evaluated its diagnostic performance and reported an AUC value of 0.945, a sensitivity of 90.24%, and a specificity of 94.51%, suggesting that sEV circMYC can be used as a biomarker to discriminate between radioresistant and radiosensitive NPC patients (Luo et al., 2020). It was found that EVs of LMP1-positive NPC cells could influence the infected host and modulate the tumor microenvironment to enhance the radioresistance of NPC cells. Transmitted LMP1 subsequently exerts its oncogenic effects by activating P38 MAPK signaling in the recipient cells, and inhibition of P38 activity effectively restores the sensitivity of NPC cells to ionizing radiation (Zhang et al., 2019). In addition, it has also been shown that tumor-derived EVs may enhance the radiosensitivity of NPC by delivering miR-142-5p to radiotherapy-resistant NPC cells to inhibit the HGF/c-Met and EGF/EGFR pathways (Zhu et al., 2022). Interestingly, it has also been found that miR-34c-5p when overexpressed in sEVs can directly target the 3′-UTR region of β-linked protein mRNA and reduce the expression level of β-linked protein, thereby improving the resistance of NPC cells to radiation therapy (Wan et al., 2020). Overall, sEVs and their contents are potential indicators to differentiate NPC patients with or without radiotherapy resistance and are expected to be new therapeutic targets.
Prediction of recurrence
Despite the availability of effective treatment strategies for NPC, relapse is a common challenge. Therefore, accurate and timely monitoring of recurrence is crucial to prolong the survival of NPC patients. There is a growing body of evidence that RNA and protein molecules in sEVs are strongly associated with the prognosis of NPC. Studies have shown that high levels of circMYC in tumor-derived sEVs may be associated with NPC recurrence. CircMYC can interact with the tumor suppressor genes miR-20b-5p and let-7e-3p to target the CRY2 gene, which is involved in cell proliferation and apoptosis processes such as EMT, AKT signaling, and p53 signaling in tumors (Huber et al., 2016; Rahman et al., 2019; Luo et al., 2020). CYPA levels are much higher in sEVs of NPC patients than in serum, and CYPA can be used for prognosis and monitoring of EBV-associated NPC (Liu et al., 2019). Moreover, sEV miR-24-3p mediates T cell suppression through inhibition of FGF11, is involved in tumorigenesis, and may serve as a potential prognostic biomarker for NPC (Ye et al., 2016).
Discussion
In recent years, sEVs have become an active research topic as non-invasive diagnostic and prognostic markers for NPC. sEV-derived miRNAs and proteins have been reported to play various roles in inflammation, cellular communication, tumor proliferation, angiogenesis, and metastasis. These functions highlight their potential as biomarkers (Mao et al., 2018). Nasopharyngeal cancer, which is associated with a high mortality rate, is often at an advanced stage when diagnosed. The main goal of early screening is to reduce the rate of missed diagnosis and keep the rate of misdiagnosis within an acceptable range (Ramsey et al., 2019; Feng et al., 2020). The false-positive rate of EBV-associated antibody testing limits the effectiveness of nasopharyngeal cancer screening and may have only limited value in screening patients with early-stage disease or in predicting cancer progression in those with elevated IgA antibodies (Ji et al., 2014).
sEV RNAs and proteins hold great promise as diagnostic markers for NPC (Table 1).
sEV markers have an important role in metastasis, prognosis, and radiotherapy resistance. Models based on combinations of multiple miRNAs provide a direction for using sEVs as efficient and accurate tumor markers in the future. Screening for the differential expression of proteins in tumor tissues and circulating sEVs is also a promising tool. In the entire process of cancer development, the early up- or downregulation of multiple markers in sEVs in NPC has an indispensable role in the prognosis of the disease. For example, the metastasis and migration capabilities of tumor cells are used for therapeutic selection of radiotherapy modalities, identification of therapeutic targets, and prediction of the risk of tumor recurrence.
sEVs have great advantages as new tumor markers. They contain a variety of bioactive molecules, have little serum interference, are highly stable, do not degrade in the extracellular environment, can be detected in a variety of humoral environments, are non-invasive to extract, and can be developed as carriers for drug and nucleic acid delivery using their biocompatibility and permeability (Arrighetti et al., 2019; Pullan et al., 2019; Tang et al., 2020). Therefore, sEVs are extremely attractive as new biomarkers. Of course, they also have some limitations. First of all, the accurate acquisition of sEVs from samples is a necessity. Current isolation and extraction techniques still have some limitations, such as cumbersome steps, and they are time-consuming and costly. Targeting receptor cells with sEVs remains very challenging (Luo and Yi, 2021). Moreover, it is necessary to further analyze the origin of sEVs in the blood of EBV-positive and -negative NPC patients. Therefore, in the future, we hope we will be able to more accurately identify specific sEVs in patients with different NPC types.
The identification of generic biomarkers based on sEVs in patients with NPC has not yet been achieved. We think that a mixture of models based on several miRNAs or proteins is more advantageous than using only one marker since it increases accuracy. To further address this issue, comparative studies with larger sample sizes are necessary. sEVs are expected to be a source of biomarkers for NPC, and it is hoped that soon, non-invasive diagnosis and extensive screening of patients based on sEVs can be achieved. It is expected that future studies will provide evidence for the large-scale clinical application of sEVs.
Author contributions
JZ searched for literature and wrote the first draft of this article. DH and XL edited the manuscript. DD and JL edited tables and figures. DZ and YL revised the manuscript. TZ and SP reviewed the manuscript and polished the grammar. All authors approved the final version and agreed on its submission to this journal.
Funding
This work was supported by the National Natural Science Foundation of China (Grant No. 82260422), Key R&D Planning Project of Jiangxi Science and Technology Commission, China (No. 20203BBGL73126).
Conflict of interest
The authors declare that the research was conducted in the absence of any commercial or financial relationships that could be construed as a potential conflict of interest.
Publisher’s note
All claims expressed in this article are solely those of the authors and do not necessarily represent those of their affiliated organizations, or those of the publisher, the editors and the reviewers. Any product that may be evaluated in this article, or claim that may be made by its manufacturer, is not guaranteed or endorsed by the publisher.
References
Adelstein, D., Gillison, M. L., Pfister, D. G., Spencer, S., Adkins, D., Brizel, D. M., et al. (2017). NCCN guidelines insights: Head and neck cancers, version 2.2017. J. Natl. Compr. Canc Netw. 15 (6), 761–770. doi:10.6004/jnccn.2017.0101
Ahmed, W., Philip, P. S., Tariq, S., and Khan, G. (2014). Epstein-Barr virus-encoded small RNAs (EBERs) are present in fractions related to exosomes released by EBV-transformed cells. PLoS One 9 (6), e99163. doi:10.1371/journal.pone.0099163
Arrighetti, N., Corbo, C., Evangelopoulos, M., Pasto, A., Zuco, V., and Tasciotti, E. (2019). Exosome-like nanovectors for drug delivery in cancer. Curr. Med. Chem. 26 (33), 6132–6148. doi:10.2174/0929867325666180831150259
Bao, L., You, B., Shi, S., Shan, Y., Zhang, Q., Yue, H., et al. (2018). Metastasis-associated miR-23a from nasopharyngeal carcinoma-derived exosomes mediates angiogenesis by repressing a novel target gene TSGA10. Oncogene 37 (21), 2873–2889. doi:10.1038/s41388-018-0183-6
Bebelman, M. P., Smit, M. J., Pegtel, D. M., and Baglio, S. R. (2018). Biogenesis and function of extracellular vesicles in cancer. Pharmacol. Ther. 188, 1–11. doi:10.1016/j.pharmthera.2018.02.013
Brody-Camp, S., McCoul, E. D., Lefante, J. J., and Aslam, R. (2021). Socioeconomic status and survival in nasopharyngeal carcinoma: A population-based study. Laryngoscope 131 (12), 2719–2723. doi:10.1002/lary.29702
Chan, Y. K., Zhang, H., Liu, P., Tsao, S. W., Lung, M. L., Mak, N. K., et al. (2015). Proteomic analysis of exosomes from nasopharyngeal carcinoma cell identifies intercellular transfer of angiogenic proteins. Int. J. Cancer 137 (8), 1830–1841. doi:10.1002/ijc.29562
Chan, K. C. A., Woo, J. K. S., King, A., Zee, B. C. Y., Lam, W. K. J., Chan, S. L., et al. (2017). Analysis of plasma epstein-barr virus DNA to screen for nasopharyngeal cancer. N. Engl. J. Med. 377 (6), 513–522. doi:10.1056/NEJMoa1701717
Chang, E. T., Liu, Z., Hildesheim, A., Liu, Q., Cai, Y., Zhang, Z., et al. (2017). Active and passive smoking and risk of nasopharyngeal carcinoma: A population-based case-control study in southern China. Am. J. Epidemiol. 185 (12), 1272–1280. doi:10.1093/aje/kwx018
Chen, P., Liu, R., Yu, Z., Cui, G., Zong, W., Wang, M., et al. (2022). MiR196a-5p in extracellular vesicles released from human nasopharyngeal carcinoma enhance the phagocytosis and secretion of microglia by targeting ROCK1. Exp. Cell Res. 411 (2), 112988. doi:10.1016/j.yexcr.2021.112988
Chen, L., Zhang, Y., Lai, S. Z., Li, W. F., Hu, W. H., Sun, R., et al. (2019). 10-Year results of therapeutic ratio by intensity-modulated radiotherapy versus two-dimensional radiotherapy in patients with nasopharyngeal carcinoma. Oncologist 24 (1), e38–e45. doi:10.1634/theoncologist.2017-0577
Chen, Y. P., Chan, A. T. C., Le, Q. T., Blanchard, P., Sun, Y., and Ma, J. (2019). Nasopharyngeal carcinoma. Lancet 394 (10192), 64–80. doi:10.1016/S0140-6736(19)30956-0
Cheng, S., Li, Z., He, J., Fu, S., Duan, Y., Zhou, Q., et al. (2019). Epstein-Barr virus noncoding RNAs from the extracellular vesicles of nasopharyngeal carcinoma (NPC) cells promote angiogenesis via TLR3/RIG-I-mediated VCAM-1 expression. Biochim. Biophys. Acta Mol. Basis Dis. 1865 (6), 1201–1213. doi:10.1016/j.bbadis.2019.01.015
Chua, M. L. K., Wee, J. T. S., Hui, E. P., and Chan, A. T. C. (2016). Nasopharyngeal carcinoma. Lancet 387 (10022), 1012–1024. doi:10.1016/S0140-6736(15)00055-0
Colevas, A. D., Yom, S. S., Pfister, D. G., Spencer, S., Adelstein, D., Adkins, D., et al. (2018). NCCN guidelines insights: Head and neck cancers, version 1.2018. J. Natl. Compr. Canc Netw. 16 (5), 479–490. doi:10.6004/jnccn.2018.0026
Cui, Z., Lin, Y., Hu, D., Wu, J., Peng, W., and Chen, Y. (2021). Diagnostic and prognostic potential of circulating and tissue BATF2 in nasopharyngeal carcinoma. Front. Mol. Biosci. 8, 724373. doi:10.3389/fmolb.2021.724373
Di Santo, R., Vaccaro, M., Romano, S., Di Giacinto, F., Papi, M., Rapaccini, G. L., et al. (2022). Machine learning-assisted FTIR analysis of circulating extracellular vesicles for cancer liquid biopsy. J. Pers. Med. 12 (6), 949. doi:10.3390/jpm12060949
Duan, B., Shi, S., Yue, H., You, B., Shan, Y., Zhu, Z., et al. (2019). Exosomal miR-17-5p promotes angiogenesis in nasopharyngeal carcinoma via targeting BAMBI. J. Cancer 10 (26), 6681–6692. doi:10.7150/jca.30757
Feng, Y., Xia, W., He, G., Liu, L., Xie, M., Tang, A., et al. (2020). Accuracy evaluation and comparison of 14 diagnostic markers for nasopharyngeal carcinoma: A meta-analysis. Front. Oncol. 10, 1779. doi:10.3389/fonc.2020.01779
Gu, M., Li, L., Zhang, Z., Chen, J., Zhang, W., Zhang, J., et al. (2017). PFKFB3 promotes proliferation, migration and angiogenesis in nasopharyngeal carcinoma. J. Cancer 8 (18), 3887–3896. doi:10.7150/jca.19112
Guan, S., Wei, J., Huang, L., and Wu, L. (2020). Chemotherapy and chemo-resistance in nasopharyngeal carcinoma. Eur. J. Med. Chem. 207, 112758. doi:10.1016/j.ejmech.2020.112758
Guinot, J. L., Moya, A., Santos, M. A., Pena, M., Quiles, B., Sanchez-Relucio, J. C., et al. (2020). Should high-dose-rate brachytherapy boost be used in early nasopharyngeal carcinomas? Rep. Pract. Oncol. Radiother. 25 (4), 479–483. doi:10.1016/j.rpor.2020.04.001
Han, C., Zhang, C., Wang, H., and Zhao, L. (2021). Exosome-mediated communication between tumor cells and tumor-associated macrophages: Implications for tumor microenvironment. Oncoimmunology 10 (1), 1887552. doi:10.1080/2162402X.2021.1887552
Hu, Y., Tian, Y., Di, H., Xue, C., Zheng, Y., Hu, B., et al. (2022). Noninvasive diagnosis of nasopharyngeal carcinoma based on phenotypic profiling of viral and tumor markers on plasma extracellular vesicles. Anal. Chem. 94 (27), 9740–9749. doi:10.1021/acs.analchem.2c01311
Huber, A. L., Papp, S. J., Chan, A. B., Henriksson, E., Jordan, S. D., Kriebs, A., et al. (2016). CRY2 and FBXL3 cooperatively degrade c-MYC. Mol. Cell 64 (4), 774–789. doi:10.1016/j.molcel.2016.10.012
Hurwitz, S. N., Nkosi, D., Conlon, M. M., York, S. B., Liu, X., Tremblay, D. C., et al. (2017). CD63 regulates epstein-barr virus LMP1 exosomal packaging, enhancement of vesicle production, and noncanonical NF-κB signaling. J. Virol. 91 (5), e02251. doi:10.1128/JVI.02251-16
Ji, M. F., Huang, Q. H., Yu, X., Liu, Z., Li, X., Zhang, L. F., et al. (2014). Evaluation of plasma Epstein-Barr virus DNA load to distinguish nasopharyngeal carcinoma patients from healthy high-risk populations in Southern China. Cancer 120 (9), 1353–1360. doi:10.1002/cncr.28564
Jia, W., Ren, C., Wang, L., Zhu, B., Jia, W., Gao, M., et al. (2016). CD109 is identified as a potential nasopharyngeal carcinoma biomarker using aptamer selected by cell-SELEX. Oncotarget 7 (34), 55328–55342. doi:10.18632/oncotarget.10530
Jiang, L., Zhang, Y., Li, B., Kang, M., Yang, Z., Lin, C., et al. (2021). miRNAs derived from circulating small extracellular vesicles as diagnostic biomarkers for nasopharyngeal carcinoma. Cancer Sci. 112 (6), 2393–2404. doi:10.1111/cas.14883
Juan, T., and Fürthauer, M. (2018). Biogenesis and function of ESCRT-dependent extracellular vesicles. Semin. Cell Dev. Biol. 74, 66–77. doi:10.1016/j.semcdb.2017.08.022
Kang, J. W., Eun, Y. G., and Lee, Y. C. (2021). Diagnostic value of salivary miRNA in head and neck squamous cell cancer: Systematic review and meta-analysis. Int. J. Mol. Sci. 22 (13), 7026. doi:10.3390/ijms22137026
Karadag, A., Ozen, A., Ozkurt, M., Can, C., Bozgeyik, I., Kabadere, S., et al. (2021). Identification of miRNA signatures and their therapeutic potentials in prostate cancer. Mol. Biol. Rep. 48 (7), 5531–5539. doi:10.1007/s11033-021-06568-7
Lam, W. K. J., and Chan, J. Y. K. (2018). Recent advances in the management of nasopharyngeal carcinoma. F1000Res 7, f1000. doi:10.12688/f1000research.15066.1
Lam, W. K. J., Jiang, P., Chan, K. C. A., Cheng, S. H., Zhang, H., Peng, W., et al. (2018). Sequencing-based counting and size profiling of plasma Epstein-Barr virus DNA enhance population screening of nasopharyngeal carcinoma. Proc. Natl. Acad. Sci. U S A 115 (22), E5115-E5124–e5124. doi:10.1073/pnas.1804184115
Lee, H. M., Okuda, K. S., Gonzalez, F. E., and Patel, V. (2019). Current perspectives on nasopharyngeal carcinoma. Adv. Exp. Med. Biol. 1164, 11–34. doi:10.1007/978-3-030-22254-3_2
Li, Z., Tsai, M. H., Shumilov, A., Baccianti, F., Tsao, S. W., Poirey, R., et al. (2019). Epstein-Barr virus ncRNA from a nasopharyngeal carcinoma induces an inflammatory response that promotes virus production. Nat. Microbiol. 4 (12), 2475–2486. doi:10.1038/s41564-019-0546-y
Li, F., Zhao, X., Sun, R., Ou, J., Huang, J., Yang, N., et al. (2020). EGFR-rich extracellular vesicles derived from highly metastatic nasopharyngeal carcinoma cells accelerate tumour metastasis through PI3K/AKT pathway-suppressed ROS. J. Extracell. Vesicles 10 (1), e12003. doi:10.1002/jev2.12003
Li, H., Xing, S., Xu, J., He, Y., Lai, Y., Wang, Y., et al. (2021). Aptamer-based CRISPR/Cas12a assay for the ultrasensitive detection of extracellular vesicle proteins. Talanta 221, 121670. doi:10.1016/j.talanta.2020.121670
Li, J., Hu, C., Chao, H., Zhang, Y., Li, Y., Hou, J., et al. (2021). Exosomal transfer of miR-106a-5p contributes to cisplatin resistance and tumorigenesis in nasopharyngeal carcinoma. J. Cell Mol. Med. 25 (19), 9183–9198. doi:10.1111/jcmm.16801
Liao, C., Zhou, Q., Zhang, Z., Wu, X., Zhou, Z., Li, B., et al. (2020). Epstein-Barr virus-encoded latent membrane protein 1 promotes extracellular vesicle secretion through syndecan-2 and synaptotagmin-like-4 in nasopharyngeal carcinoma cells. Cancer Sci. 111 (3), 857–868. doi:10.1111/cas.14305
Liao, C., Liu, H., and Luo, X. (2021). The emerging roles of exosomal miRNAs in nasopharyngeal carcinoma. Am. J. Cancer Res. 11 (6), 2508–2520.
Lin, C., Lin, K., Zhang, B., Su, Y., Guo, Q., Lu, T., et al. (2022). Plasma epstein-barr virus MicroRNA BART8-3p as a diagnostic and prognostic biomarker in nasopharyngeal carcinoma. Oncologist 27 (4), e340–e349. doi:10.1093/oncolo/oyac024
Liu, L., Zuo, L., Yang, J., Xin, S., Zhang, J., Zhou, J., et al. (2019). Exosomal cyclophilin A as a novel noninvasive biomarker for Epstein-Barr virus associated nasopharyngeal carcinoma. Cancer Med. 8 (6), 3142–3151. doi:10.1002/cam4.2185
Liu, Y., Wen, J., and Huang, W. (2021). Exosomes in nasopharyngeal carcinoma. Clin. Chim. Acta 523, 355–364. doi:10.1016/j.cca.2021.10.013
Lo, A. K., Dawson, C. W., Lung, H. L., Wong, K. L., and Young, L. S. (2021). The role of EBV-encoded LMP1 in the NPC tumor microenvironment: From function to therapy. Front. Oncol. 11, 640207. doi:10.3389/fonc.2021.640207
Lu, J., Liu, Q. H., Wang, F., Tan, J. J., Deng, Y. Q., Peng, X. H., et al. (2018). Exosomal miR-9 inhibits angiogenesis by targeting MDK and regulating PDK/AKT pathway in nasopharyngeal carcinoma. J. Exp. Clin. Cancer Res. 37 (1), 147. doi:10.1186/s13046-018-0814-3
Luan, J., Wang, J., Su, Q., Chen, X., Jiang, G., and Xu, X. (2016). Meta-analysis of the differentially expressed microRNA profiles in nasopharyngeal carcinoma. Oncotarget 7 (9), 10513–10521. doi:10.18632/oncotarget.7013
Luo, H., and Yi, B. (2021). The role of exosomes in the pathogenesis of nasopharyngeal carcinoma and the involved clinical application. Int. J. Biol. Sci. 17 (9), 2147–2156. doi:10.7150/ijbs.59688
Luo, Y., Ma, J., Liu, F., Guo, J., and Gui, R. (2020). Diagnostic value of exosomal circMYC in radioresistant nasopharyngeal carcinoma. Head. Neck 42 (12), 3702–3711. doi:10.1002/hed.26441
Mao, L., Li, X., Gong, S., Yuan, H., Jiang, Y., Huang, W., et al. (2018). Serum exosomes contain ECRG4 mRNA that suppresses tumor growth via inhibition of genes involved in inflammation, cell proliferation, and angiogenesis. Cancer Gene Ther. 25 (9-10), 248–259. doi:10.1038/s41417-018-0032-3
Mao, M., Wang, X., Sheng, H., Li, H., Liu, W., Han, R., et al. (2021). Heat shock protein 90α provides an effective and novel diagnosis strategy for nasopharyngeal carcinoma. Adv. Ther. 38 (1), 413–422. doi:10.1007/s12325-020-01518-4
Meckes, D. G., Shair, K. H. Y., Marquitz, A. R., Kung, C. P., Edwards, R. H., and Raab-Traub, N. (2010). Human tumor virus utilizes exosomes for intercellular communication. Proc. Natl. Acad. Sci. U S A 107 (47), 20370–20375. doi:10.1073/pnas.1014194107
Meckes, D. G., Menaker, N. F., and Raab-Traub, N. (2013). Epstein-Barr virus LMP1 modulates lipid raft microdomains and the vimentin cytoskeleton for signal transduction and transformation. J. Virol. 87 (3), 1301–1311. doi:10.1128/JVI.02519-12
Mii, S., Enomoto, A., Shiraki, Y., Taki, T., Murakumo, Y., and Takahashi, M. (2019). CD109: A multifunctional GPI-anchored protein with key roles in tumor progression and physiological homeostasis. Pathol. Int. 69 (5), 249–259. doi:10.1111/pin.12798
Okada, T., Sinha, S., Esposito, I., Schiavon, G., Lopez-Lago, M. A., Su, W., et al. (2019). Author Correction: The Rho GTPase Rnd1 suppresses mammary tumorigenesis and EMT by restraining Ras-MAPK signalling. Nat. Cell Biol. 21 (4), 534. doi:10.1038/s41556-019-0288-3
Pegtel, D. M., and Gould, S. J. (2019). Exosomes. Annu Rev. Biochem. 88, 487–514. doi:10.1146/annurev-biochem-013118-111902
Pullan, J. E., Confeld, M. I., Osborn, J. K., Kim, J., Sarkar, K., and Mallik, S. (2019). Exosomes as drug carriers for cancer therapy. Mol. Pharm. 16 (5), 1789–1798. doi:10.1021/acs.molpharmaceut.9b00104
Raab-Traub, N., and Dittmer, D. P. (2017). Viral effects on the content and function of extracellular vesicles. Nat. Rev. Microbiol. 15 (9), 559–572. doi:10.1038/nrmicro.2017.60
Rahbarghazi, R., Jabbari, N., Sani, N. A., Asghari, R., Salimi, L., Kalashani, S. A., et al. (2019). Tumor-derived extracellular vesicles: Reliable tools for cancer diagnosis and clinical applications. Cell Commun. Signal 17 (1), 73. doi:10.1186/s12964-019-0390-y
Rahman, S., Kraljević Pavelić, S., and Markova-Car, E. (2019). Circadian (De)regulation in head and neck squamous cell carcinoma. Int. J. Mol. Sci. 20 (11), 2662. doi:10.3390/ijms20112662
Ramayanti, O., Verkuijlen, S. A. W. M., Novianti, P., Scheepbouwer, C., Misovic, B., Koppers-Lalic, D., et al. (2019). Vesicle-bound EBV-BART13-3p miRNA in circulation distinguishes nasopharyngeal from other head and neck cancer and asymptomatic EBV-infections. Int. J. Cancer 144 (10), 2555–2566. doi:10.1002/ijc.31967
Ramsey, T., Hojjat, H., Yuhan, B., Svider, P. F., Eloy, J. A., Folbe, A. J., et al. (2019). Disparities in impact of nasopharyngeal cancer: An analysis of global health burden. Laryngoscope 129 (11), 2482–2486. doi:10.1002/lary.27532
Raposo, G., and Stoorvogel, W. (2013). Extracellular vesicles: Exosomes, microvesicles, and friends. J. Cell Biol. 200 (4), 373–383. doi:10.1083/jcb.201211138
Riddell, I. A. (2018). Cisplatin and oxaliplatin: Our current understanding of their actions. Met. Ions Life Sci. 18, 1. doi:10.1515/9783110470734-001
Sakakura, H., Mii, S., Hagiwara, S., Kato, T., Yamamoto, N., Hibi, H., et al. (2016). CD109 is a component of exosome secreted from cultured cells. Biochem. Biophys. Res. Commun. 469 (4), 816–822. doi:10.1016/j.bbrc.2015.12.063
Saleh, T., Jankowski, W., Sriram, G., Rossi, P., Shah, S., Lee, K. B., et al. (2016). Cyclophilin A promotes cell migration via the Abl-Crk signaling pathway. Nat. Chem. Biol. 12 (2), 117–123. doi:10.1038/nchembio.1981
Shan, Y., You, B., Shi, S., Shi, W., Zhang, Z., Zhang, Q., et al. (2018). Hypoxia-induced matrix metalloproteinase-13 expression in exosomes from nasopharyngeal carcinoma enhances metastases. Cell Death Dis. 9 (3), 382. doi:10.1038/s41419-018-0425-0
Shan, Y., Zhou, P., Zhou, Q., and Yang, L. (2022). Extracellular vesicles in the progression and therapeutic resistance of nasopharyngeal carcinoma. Cancers (Basel) 14 (9), 2289. doi:10.3390/cancers14092289
Shehzad, A., Islam, S. U., Shahzad, R., Khan, S., and Lee, Y. S. (2021). Extracellular vesicles in cancer diagnostics and therapeutics. Pharmacol. Ther. 223, 107806. doi:10.1016/j.pharmthera.2021.107806
Sinha, S., and Gajra, A. (2022). Nasopharyngeal cancer, in StatPearls. Treasure Island (FL): StatPearls Publishing. Copyright © 2022.
Sun, X. S., Liu, S. L., Luo, M. J., Li, X. Y., Chen, Q. Y., Guo, S. S., et al. (2019). The association between the development of radiation therapy, image Technology, and chemotherapy, and the survival of patients with nasopharyngeal carcinoma: A cohort study from 1990 to 2012. Int. J. Radiat. Oncol. Biol. Phys. 105 (3), 581–590. doi:10.1016/j.ijrobp.2019.06.2549
Sunagawa, M., Mii, S., Enomoto, A., Kato, T., Murakumo, Y., Shiraki, Y., et al. (2016). Suppression of skin tumorigenesis in CD109-deficient mice. Oncotarget 7 (50), 82836–82850. doi:10.18632/oncotarget.12653
Sung, H., Ferlay, J., Siegel, R. L., Laversanne, M., Soerjomataram, I., Jemal, A., et al. (2021). Global cancer statistics 2020: GLOBOCAN estimates of incidence and mortality worldwide for 36 cancers in 185 countries. CA Cancer J. Clin. 71 (3), 209–249. doi:10.3322/caac.21660
Tang, Z., Li, D., Hou, S., and Zhu, X. (2020). The cancer exosomes: Clinical implications, applications and challenges. Int. J. Cancer 146 (11), 2946–2959. doi:10.1002/ijc.32762
Tang, L. L., Chen, Y. P., Chen, C. B., Chen, M. Y., Chen, N. Y., Chen, X. Z., et al. (2021). The Chinese Society of Clinical Oncology (CSCO) clinical guidelines for the diagnosis and treatment of nasopharyngeal carcinoma. Cancer Commun. (Lond) 41 (11), 1195–1227. doi:10.1002/cac2.12218
Teow, S. Y., Liew, K., Khoo, A. S. B., and Peh, S. C. (2017). Pathogenic role of exosomes in epstein-barr virus (EBV)-Associated cancers. Int. J. Biol. Sci. 13 (10), 1276–1286. doi:10.7150/ijbs.19531
Théry, C., Witwer, K. W., Aikawa, E., Alcaraz, M. J., Anderson, J. D., Andriantsitohaina, R., et al. (2018). Minimal information for studies of extracellular vesicles 2018 (MISEV2018): A position statement of the international society for extracellular vesicles and update of the MISEV2014 guidelines. J. Extracell. Vesicles 7 (1), 1535750. doi:10.1080/20013078.2018.1535750
Tsao, S. W., Tsang, C. M., and Lo, K. W. (2017). Epstein-Barr virus infection and nasopharyngeal carcinoma. Philos. Trans. R. Soc. Lond B Biol. Sci. 372 (1732), 20160270. doi:10.1098/rstb.2016.0270
Vader, P., Breakefield, X. O., and Wood, M. J. (2014). Extracellular vesicles: Emerging targets for cancer therapy. Trends Mol. Med. 20 (7), 385–393. doi:10.1016/j.molmed.2014.03.002
Valadi, H., Ekstrom, K., Bossios, A., Sjostrand, M., Lee, J. J., and Lotvall, J. O. (2007). Exosome-mediated transfer of mRNAs and microRNAs is a novel mechanism of genetic exchange between cells. Nat. Cell Biol. 9 (6), 654–659. doi:10.1038/ncb1596
van Niel, G., D'Angelo, G., and Raposo, G. (2018). Shedding light on the cell biology of extracellular vesicles. Nat. Rev. Mol. Cell Biol. 19 (4), 213–228. doi:10.1038/nrm.2017.125
Verweij, F. J., Bebelman, M. P., Jimenez, C. R., Garcia-Vallejo, J. J., Janssen, H., Neefjes, J., et al. (2018). Quantifying exosome secretion from single cells reveals a modulatory role for GPCR signaling. J. Cell Biol. 217 (3), 1129–1142. doi:10.1083/jcb.201703206
Wan, F. Z., Chen, K. H., Sun, Y. C., Chen, X. C., Liang, R. B., Chen, L., et al. (2020). Exosomes overexpressing miR-34c inhibit malignant behavior and reverse the radioresistance of nasopharyngeal carcinoma. J. Transl. Med. 18 (1), 12. doi:10.1186/s12967-019-02203-z
Wang, Y., Guo, Z., Shu, Y., Zhou, H., Wang, H., and Zhang, W. (2017). BART miRNAs: An unimaginable force in the development of nasopharyngeal carcinoma. Eur. J. Cancer Prev. 26 (2), 144–150. doi:10.1097/CEJ.0000000000000221
Wang, J., Jiang, Q., Faleti, O. D., Tsang, C. M., Zhao, M., Wu, G., et al. (2020a). Exosomal delivery of AntagomiRs targeting viral and cellular MicroRNAs synergistically inhibits cancer angiogenesis. Mol. Ther. Nucleic Acids 22, 153–165. doi:10.1016/j.omtn.2020.08.017
Wang, J., Zhang, C., Peng, X., Liu, K., Zhao, L., Chen, X., et al. (2020b). A combination of four serum miRNAs for screening of lung adenocarcinoma. Hum. Cell 33 (3), 830–838. doi:10.1007/s13577-020-00346-6
Wang, X., Xiang, Z., Tsao, G. S. W., and Tu, W. (2021). Exosomes derived from nasopharyngeal carcinoma cells induce IL-6 production from macrophages to promote tumorigenesis. Cell Mol. Immunol. 18 (2), 501–503. doi:10.1038/s41423-020-0420-0
Wei, J., Zhang, J., Si, Y., Kanada, M., Zhang, Z., Terakawa, S., et al. (2015). Blockage of LMP1-modulated store-operated Ca(2+) entry reduces metastatic potential in nasopharyngeal carcinoma cell. Cancer Lett. 360 (2), 234–244. doi:10.1016/j.canlet.2015.02.032
Wei, J., Ye, J., Luo, Y., Weng, J., He, Q., Liu, F., et al. (2020). EB virus promotes metastatic potential by boosting STIM1-dependent Ca(2+) signaling in nasopharyngeal carcinoma cells. Cancer Lett. 478, 122–132. doi:10.1016/j.canlet.2020.03.005
Wu, X., Zhou, Z., Xu, S., Liao, C., Chen, X., Li, B., et al. (2020). Extracellular vesicle packaged LMP1-activated fibroblasts promote tumor progression via autophagy and stroma-tumor metabolism coupling. Cancer Lett. 478, 93–106. doi:10.1016/j.canlet.2020.03.004
Wu, A., Luo, N., Xu, Y., Du, N., Li, L., and Liu, Q. (2022). Exosomal LBH inhibits epithelial-mesenchymal transition and angiogenesis in nasopharyngeal carcinoma via downregulating VEGFA signaling. Int. J. Biol. Sci. 18 (1), 242–260. doi:10.7150/ijbs.66506
Xia, T., Tian, H., Zhang, K., Zhang, S., Chen, W., Shi, S., et al. (2021). Exosomal ERp44 derived from ER-stressed cells strengthens cisplatin resistance of nasopharyngeal carcinoma. BMC Cancer 21 (1), 1003. doi:10.1186/s12885-021-08712-9
Xiao, Y., Zheng, L., Zou, X., Wang, J., Zhong, J., and Zhong, T. (2019). Extracellular vesicles in type 2 diabetes mellitus: Key roles in pathogenesis, complications, and therapy. J. Extracell. Vesicles 8 (1), 1625677. doi:10.1080/20013078.2019.1625677
Xu, Z. X., Lin, Z. X., Fang, J. Y., Wu, K. S., Du, P. L., Zeng, Y., et al. (2015). Mortality characteristic and prediction of nasopharyngeal carcinoma in China from 1991 to 2013. Asian Pac J. Cancer Prev. 16 (15), 6729–6734. doi:10.7314/apjcp.2015.16.15.6729
Xu, M., Yao, Y., Chen, H., Zhang, S., Cao, S. M., Zhang, Z., et al. (2019). Genome sequencing analysis identifies Epstein-Barr virus subtypes associated with high risk of nasopharyngeal carcinoma. Nat. Genet. 51 (7), 1131–1136. doi:10.1038/s41588-019-0436-5
Xunian, Z., and Kalluri, R. (2020). Biology and therapeutic potential of mesenchymal stem cell-derived exosomes. Cancer Sci. 111 (9), 3100–3110. doi:10.1111/cas.14563
Yang, J., Zhou, M., Zhao, R., Peng, S., Luo, Z., Li, X., et al. (2014). Identification of candidate biomarkers for the early detection of nasopharyngeal carcinoma by quantitative proteomic analysis. J. Proteomics 109, 162–175. doi:10.1016/j.jprot.2014.06.025
Ye, S. B., Zhang, H., Cai, T. T., Liu, Y. N., Ni, J. J., He, J., et al. (2016). Exosomal miR-24-3p impedes T-cell function by targeting FGF11 and serves as a potential prognostic biomarker for nasopharyngeal carcinoma. J. Pathol. 240 (3), 329–340. doi:10.1002/path.4781
Yin, H., Qiu, X., Shan, Y., You, B., Xie, L., Zhang, P., et al. (2021). HIF-1α downregulation of miR-433-3p in adipocyte-derived exosomes contributes to NPC progression via targeting SCD1. Cancer Sci. 112 (4), 1457–1470. doi:10.1111/cas.14829
You, Y., Shan, Y., Chen, J., Yue, H., You, B., Shi, S., et al. (2015). Matrix metalloproteinase 13-containing exosomes promote nasopharyngeal carcinoma metastasis. Cancer Sci. 106 (12), 1669–1677. doi:10.1111/cas.12818
You, B., Cao, X., Shao, X., Ni, H., Shi, S., Shan, Y., et al. (2016). Clinical and biological significance of HAX-1 overexpression in nasopharyngeal carcinoma. Oncotarget 7 (11), 12505–12524. doi:10.18632/oncotarget.7274
You, B., Pan, S., Gu, M., Zhang, K., Xia, T., Zhang, S., et al. (2022). Extracellular vesicles rich in HAX1 promote angiogenesis by modulating ITGB6 translation. J. Extracell. Vesicles 11 (5), e12221. doi:10.1002/jev2.12221
Zhang, J. M., and Lu, M. Z. (2019). Circulating MicroRNA in diagnosis of nasopharyngeal carcinoma:a Meta-analysis. Lin. Chung Er Bi Yan Hou Tou Jing Wai Ke Za Zhi 33 (6), 517–524. doi:10.13201/j.issn.1001-1781.2019.06.011
Zhang, L., and Yu, D. (2019). Exosomes in cancer development, metastasis, and immunity. Biochim. Biophys. Acta Rev. Cancer 1871 (2), 455–468. doi:10.1016/j.bbcan.2019.04.004
Zhang, Z., Yu, X., Zhou, Z., Li, B., Peng, J., Wu, X., et al. (2019). LMP1-positive extracellular vesicles promote radioresistance in nasopharyngeal carcinoma cells through P38 MAPK signaling. Cancer Med. 8 (13), 6082–6094. doi:10.1002/cam4.2506
Zhang, H., Zou, X., Wu, L., Zhang, S., Wang, T., Liu, P., et al. (2020). Identification of a 7-microRNA signature in plasma as promising biomarker for nasopharyngeal carcinoma detection. Cancer Med. 9 (3), 1230–1241. doi:10.1002/cam4.2676
Zhao, M., Nanbo, A., Sun, L., and Lin, Z. (2019). Extracellular vesicles in epstein-barr virus' life cycle and pathogenesis. Microorganisms 7 (2), 48. doi:10.3390/microorganisms7020048
Zhou, S., da Silva, S. D., Siegel, P. M., and Philip, A. (2019). CD109 acts as a gatekeeper of the epithelial trait by suppressing epithelial to mesenchymal transition in squamous cell carcinoma cells in vitro. Sci. Rep. 9 (1), 16317. doi:10.1038/s41598-019-50694-z
Zhu, Q. Y., Zhao, G., Li, Y., Talakatta, G., Mai, H., Le, Q., et al. (2020). Advances in pathogenesis and precision medicine for nasopharyngeal carcinoma. MedComm 2 (2), 175–206. doi:10.1002/mco2.32
Zhu, C., Jiang, X., Xiao, H., and Guan, J. (2022). Tumor-derived extracellular vesicles inhibit HGF/c-Met and EGF/EGFR pathways to accelerate the radiosensitivity of nasopharyngeal carcinoma cells via microRNA-142-5p delivery. Cell Death Discov. 8 (1), 17. doi:10.1038/s41420-021-00794-5
Keywords: nasopharyngeal carcinoma, small extracellular vesicles, prognosis, diagnosis, biomarker
Citation: Zhang J, Huang D, Lan X, Deng D, Li J, Zhang D, Li Y, Zhong T and Peng S (2023) Application of small extracellular vesicles in the diagnosis and prognosis of nasopharyngeal carcinoma. Front. Cell Dev. Biol. 11:1100941. doi: 10.3389/fcell.2023.1100941
Received: 17 November 2022; Accepted: 02 March 2023;
Published: 10 March 2023.
Edited by:
Shobha Vasudevan, Massachusetts General Hospital and Harvard Medical School, United StatesReviewed by:
Shu Feng Zhou, Massachusetts General Hospital and Harvard Medical School, United StatesChandreyee Datta, Massachusetts General Hospital Cancer Center, United States
Copyright © 2023 Zhang, Huang, Lan, Deng, Li, Zhang, Li, Zhong and Peng. This is an open-access article distributed under the terms of the Creative Commons Attribution License (CC BY). The use, distribution or reproduction in other forums is permitted, provided the original author(s) and the copyright owner(s) are credited and that the original publication in this journal is cited, in accordance with accepted academic practice. No use, distribution or reproduction is permitted which does not comply with these terms.
*Correspondence: Tianyu Zhong, emhvbmd0aWFueXVAZ21haWwuY29t; Shaoping Peng, cHNwOTk5QDEyNi5jb20=