- 1Shi’s Center of Orthopedics and Traumatology, Shuguang Hospital Affiliated to Shanghai University of Traditional Chinese Medicine, Shanghai, China
- 2Institute of Traumatology and Orthopedics, Shanghai Academy of Traditional Chinese Medicine, Shanghai, China
- 3Shanghai University of Traditional Chinese Medicine, Shanghai, China
- 4Department of Dermatology, Shanghai Songjiang District Central Hospital, Shanghai, China
Primary ovarian insufficiency (POI) is an essential cause of young female fertility loss. At present, there are many treatments for primary ovarian insufficiency, but due to the complexity of the pathogenesis of primary ovarian insufficiency, the efficacy still could not be satisfactory. Stem cell transplantation is a feasible intervention protocol for primary ovarian insufficiency. However, its wide application in the clinic is limited by some defects such as tumorigenic and controversial ethical issues. Stem cell-derived extracellular vesicles (EVs) represent an important mode of intercellular communication attracting increasing interest. It is well documented that stem cell-derived extracellular vesicles for primary ovarian insufficiency with exciting therapeutic effects. Studies have found that stem cell-derived extracellular vesicles could improve ovarian reserve, increase the growth of follicles, reduce follicle atresia, and restore hormone levels of FSH and E2. Its mechanisms include inhibiting ovarian granulosa cells (GCs) apoptosis, reactive oxygen species, and inflammatory response and promoting granulosa cells proliferation and angiogenesis. Thus, stem cell-derived extracellular vesicles are a promising and potential method for primary ovarian insufficiency patients. However, stem cell-derived extracellular vesicles are still a long way from clinical translation. This review will provide an overview of the role and the mechanisms of stem cell-derived extracellular vesicles in primary ovarian insufficiency, and further elaborate on the current challenges. It may suggest new directions for future research.
Introduction
Primary ovarian insufficiency (POI), also known as premature ovarian failure (POF) or premature menopause, is defined as cessation of menstruation before the expected age of menopause (Qin et al., 2015). In recent years, according to epidemiological surveys, POI is becoming a common disease in women’s reproductive systems, with an incidence rate of 1% (Szeliga et al., 2021). Studies have shown that 1 in 1,000 women between the ages of 15 and 29 and 1 in 100 women between the ages of 30 and 39 experience POI (Nippita and Baber, 2007; Panay and Fenton, 2008). There is a close relationship between ovarian granulosa cells (GCs) quality and the occurrence of POI (Zhu et al., 2021; Geng et al., 2022a) because senescence and cell cycle disorders in GCs result in a significant reduction of ovarian reserve (Fu et al., 2017; Liu et al., 2021). In clinics, hormone replacement therapy is the most commonly used management for POI patients but has a higher risk of various complications such as breast and ovarian cancer (Shelling, 2010; Kovanci and Schutt, 2015; Szeliga et al., 2021). Therefore, it is essential to find a safer and more effective way to treat POI.
Not surprisingly, stem cell-based therapies hold tremendous potential for treating POI in both preclinical and clinical trials (Ding et al., 2018; Yin et al., 2020; Fu et al., 2021; Mashayekhi et al., 2021; Wang et al., 2022a). Recent research suggests stem cells may provide therapeutic effects by paracrine means, specifically using extracellular vesicles (EVs) that include exosomes (Zhang et al., 2019a; Liu et al., 2021; Qu et al., 2022). The diameters of EVs range from approximately 30 nm–3,000 nm, and their biomolecular composition determines their functions, as well as their source and conditions (Riazifar et al., 2017). EVs are initially considered to be cellular debris or a way to remove toxic or unneeded by-products from the cell. Although EVs have ancient evolutionary origins and conserved mechanisms of generation, they play crucial physiological roles in cell-to-cell communication (Zhang et al., 2015; Xu et al., 2018). It is common for cells to secrete EVs, and these EVs can also be found in body fluids (Villarroya-Beltri et al., 2014; Xu et al., 2021). Substantial evidence has implicated that stem cell-derived EVs play an obvious role in the treatment of various diseases (Gnecchi et al., 2005; Clevers et al., 2014; Doeppner et al., 2015). It is worth noting that stem cell-derived EVs have shown promising results in treating POI. In this review, we summarize the applications of stem cells-derived EVs in POI and expound on the underlying cellular and molecular mechanisms. We also discuss the expectations for the future of stem cells-derived EVs.
The difference between stem cells and stem cell-derived EVs
Stem cells have the potential for self-renewal and multidirectional differentiation, such as adipose stem cells (ADSCs), bone marrow mesenchymal stem cells (BMSCs), umbilical cord mesenchymal stem cells (UCMSCs) (Williams et al., 2008; Liao and Chen, 2014; Huang et al., 2021). Stem cells play vital roles in maintaining cellular homeostasis and restoring it upon tissue injury (Riazifar et al., 2017). Extensive research has shown that stem cells hold significant therapeutic potential in a variety of human diseases (Yamanaka, 2020). However, stem cells treatment carries an increased risk of conditions including organ failure and neurodegenerative disease (Poulos, 2018). Another potential safety risk for stem cell transplantation is increased immunogenicity (Nguyen et al., 2016). By far, the biggest concern is the tumorigenicity of stem cells due to their long-term culture, which may result in the accumulation of karyotypic abnormalities, copy number variation, and loss of heterozygosity (Lund et al., 2012). Hence, an increasing interest has been shifted toward stem cells-derived EVs. It is now clear from stem cell research that EVs are essential for cells to protect or regenerate injured cells, possibly through a paracrine effect mediated by the EVs (Han et al., 2021). In general, EVs, including exosomes and microvesicles (MVs), are membrane-enclosed vesicles containing proteins and nucleic acids (van Niel et al., 2018; Andaluz Aguilar et al., 2020). Exosomes are EVs with a size range of 40–160 nm (average 100 nm) in diameter with an endosomal origin (Kalluri and LeBleu, 2020). MVs, which vary from 50 to 1,000 nm in diameter, appear to have multiple points of origin, ranging from the selective outward pinching of the plasma membrane to membrane shedding and/or vesicles resulting from cell death (Stahl and Raposo, 2019). EVs comprise complex contents, such as nucleic acids including DNA, mRNAs, non-coding RNAs (ncRNAs), lipids, and various proteins (Mathivanan et al., 2010; Mashouri et al., 2019). Compared to stem cells, stem cell-derived EVs possess multiple advantages including ethical access, abundant source, and low immunogenicity (Fang et al., 2020; Watanabe et al., 2021; Hade et al., 2022; Xia et al., 2022). Hence, stem cell-derived EVs are considered to be a safer regenerative medicine approach for treating many otherwise untreatable diseases such as POI (Ding et al., 2020). But it should not be ignored that stem cells have the capacity for self-renewal, unlimited proliferation, and differentiation but not EVs.
The pathogenesis of POI and the application of stem cell-derived EVs
The pathogenesis of POI has not been fully elucidated as it involves multiple factors including genetic, immunological, and environmental factors. A wide range of genetic defects is associated with POI, including X chromosome defects, which collectively account for 10%–25% of cases (Chen et al., 2018). More than 80 genes concern gonadal development, DNA replication/meiosis, DNA repair, and hormone synthesis (Franca and Mendonca, 2020). Furthermore, some POI patients suffer from autoimmune diseases, mainly thyroid autoimmune diseases (Dragojevic-Dikic et al., 2010). Common methods of anticancer treatment such as chemotherapy and radiotherapy could also cause female reproductive dysfunction (Meirow and Nugent, 2001). Cyclophosphamide and cisplatin are commonly used in clinical practice, and becoming the most common method of establishing an animal model of POI. In addition, other recognized causes of POI include metabolism disorders, infections, toxins, and environmental factors (Naleway et al., 2018; Moslehi et al., 2019; Rostami Dovom et al., 2019; El Bakly et al., 2020).
At present, there have been numerous researches on the treatment of stem cell-derived EVs for POI and has received considerable clinical attention. Among these studies, sources of EVs include umbilical cord mesenchymal stem cells, bone marrow mesenchymal stem cells, embryonic stem cells, amniotic fluid stem cells, adipose stem cells, and menstrual blood stem cells (Ling et al., 2019; Tracy et al., 2019; Liu et al., 2020; Yang et al., 2020; Li et al., 2021a; Zhang et al., 2021) (Table 1). Studies have found that stem cell-derived EVs could improve ovarian reserve, increase the growth of follicles, reduce follicle atresia, and restore hormone levels of FSH and E2 (Fu et al., 2021). EVs contain a variety of lipids, nucleic acids, and proteins, and play an important role in cell-cell communication by transporting several molecules from donors to recipients (Mittelbrunn and Sanchez-Madrid, 2012). In particular, miRNA. miRNA is a distinct class of small (approximately 22 nucleotides), single-stranded, and non-coding RNAs, and play critical functions in the regulation of cellular gene expression by binding to complementary sequences in the target mRNAs, leading to either translational repression or target degradation of the specific mRNAs (Ha and Kim, 2014). Several studies indicate that miRNA carried by EVs plays a key role in the treatment of POI (Xiao et al., 2016; Ding et al., 2020; Geng et al., 2022b; Cai et al., 2022). In the following section, we explore the potential mechanisms and application value of different EVs (Figure 1).
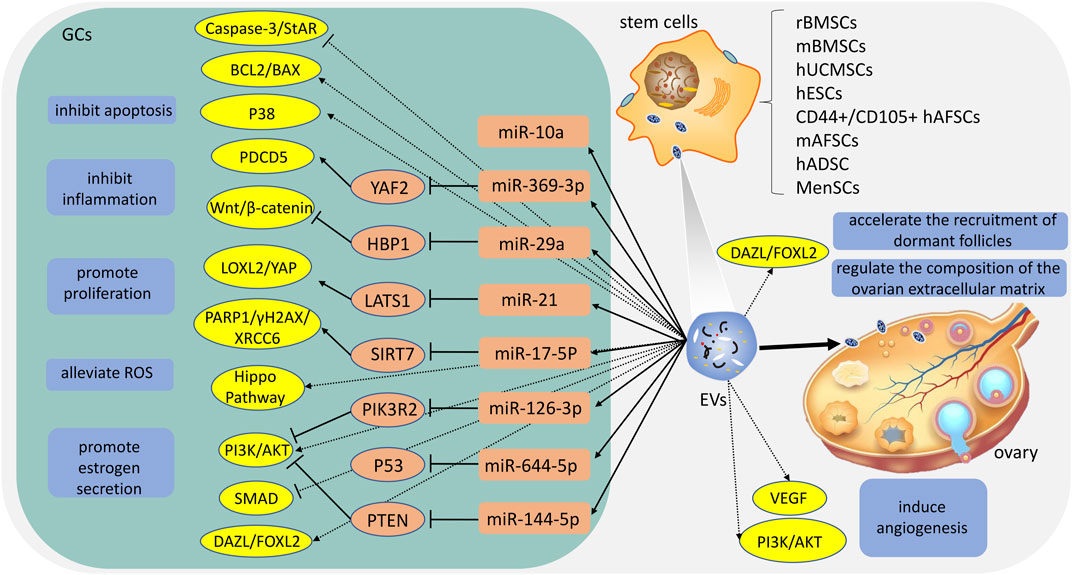
FIGURE 1. The potential mechanisms of different extracellular vesicles (EVs). rBMSCs: rat bone marrow mesenchymal stem cells; mBMSCs: mouse bone marrow mesenchymal stem cells; hUCMSCs: human umbilical cord mesenchymal stem cells; hESCs: human embryonic stem cells; hAFSCs: human amniotic fluid stem cells; mAFSCs: mouse amniotic fluid stem cells; hADSC: human adipose stem cells; MenSCs: menstrual blood-stem cells; ROS: reactive oxygen species; GCs: granulosa cells.
Human umbilical cord mesenchymal stem cells-derived EVs (hUCMSCs-EVs)
It is the placenta that supplies foetal nutrition and connects the mother and the foetus during pregnancy (Tang et al., 2021). hUCMSCs-EVs can be isolated from various hUCMSCs compartments or the complete hUCMSCs. There are round or oval membranous vesicles, which can be aggregated and distributed, and their membrane structure is clearly defined (Vohra et al., 2020). hUCMSCs-EVs express the EVs-specific four-transmembrane protein markers CD9, CD63, CD81 and tumor susceptibility gene 101 protein (TSG101), heat shock protein70 (HSP70), and the multivesicular biosynthesis-related protein ALIX (Sun et al., 2017; Yang et al., 2019; Zhang et al., 2020; Deng et al., 2021; Cai et al., 2022; Gao et al., 2022; Qu et al., 2022). In 2017, hUCMSCs- EVs were first described for application to POI by Sun et al. (2017). Studies show that hUCMSCs-EVs ameliorate GCs stress and apoptosis in vitro, and the underlying mechanism may be related to the upregulation of BCL2 and the downregulation of BAX, cleaved caspase-3, and PARP (Sun et al., 2017; Deng et al., 2021). In addition, Sun et al. (2017) suggest that microRNA-24, microRNA-106a, microRNA-19b, and microRNA-25 may be closely related to apoptosis. After then, multiple studies show that hUCMSCs-EVs could restore serum FSH and estrogen levels, preserve the ovarian reserve and avoid antral follicle atresia. The mechanism involves the Hippo pathway and PI3K-AKT pathway (Yang et al., 2019; Li et al., 2021a). Li et al. (2021a) propose that when the key Hippo molecule (YAP) is blocked, hUCMSCs-EVs suppress the proliferation and function of ovarian cells by regulating the Hippo pathway. Moreover, complex ovarian vascular systems are critical for ovarian function and follicle development, which make the follicle and/or corpus luteum receive nutrients, oxygen, and hormone support, as well as synthesize and release steroids (Kamat et al., 1995; Robinson et al., 2009; Ezoe et al., 2014). Yang et al. (2019) consider that angiogenesis also plays a critical role in the application of hUCMSCs-derived EVs. Furthermore, the EVs-mediated transfer of miRNA is an important way by which stem cell function. Some miRNA including miR-126-3p, miR-21, miR-29a, and miR-17-5P carried by hUCMSCs-EVs could play a role at the post-transcriptional level (Ding et al., 2020; Cai et al., 2022; Gao et al., 2022; Qu et al., 2022). By binding to the 3′UTR of target genes, these miRNAs inhibit the expression of particular molecules to inhibit reactive oxygen species (ROS) production and apoptosis and promote cell survival, proliferation, and angiogenesis in GCs. It is worth mentioning that the protective effect of EVs on cisplatin-damaged GCs showed a dose-dependent effect. GCs are significantly more viable when 15 μg/mL of hUCMSC-EVs are added to their culture for 24 h; 25 g/mL is even more effective when administered for 48 h (Zhang et al., 2020).
Bone marrow mesenchymal stem cells-derived EVs (BMSCs-EVs)
BMSCs is the first stem cell used to evaluate the efficacy in the treatment of POI (Fu et al., 2017). Studies have revealed that BMSCs or EVs infusion could decrease the expression of pro-inflammatory cytokines and oxidised biomolecules (He et al., 2018). BMSCs-EVs are cup-shaped or spherical in shape, with a clear model structure around them, and the diameters of EVs distribution ranged between 30 and 2,000 nm (Li et al., 2021b; Yu et al., 2021). BMSCs-EVs express the EVs-specific four-transmembrane protein markers CD9, CD63, CD81 and TSG101, HSP70, and the multivesicular biosynthesis-related protein ALIX (Tang et al., 2021). Moreover, BMSCs-EVs are negative for CD14, CD34, and CD45 (Li et al., 2019; Tang et al., 2021). BMSCs-EVs appear to have a significant anti-apoptotic effect in vitro and in vivo (Su et al., 2021; Wen et al., 2021; Xiong et al., 2021; Wang et al., 2022b). Sun et al. (2019) and Yang et al. (2020) also suggest that BMSCs-EVs inhibit GCs apoptosis by carrying miR-144-5p and miR-644-5p to inhibit PTEN and p53.
Embryonic stem cells-derived EVs (ESCs-EVs)
Embryonic stem cells, derived from the blastocyst stage embryos, are distinguished by their ability to self-renew and differentiate into all cell types (Ohtsuka and Dalton, 2008; Martello and Smith, 2014). Thus, ESCs-EVs are extensively studied. Many studies have shown that ESCs-EVs can suppress senescence, facilitate cell proliferation, and inhibit cell apoptosis and oxidation (Khan et al., 2015; Bae et al., 2019; Zhang et al., 2019b; Tavakoli Dargani and Singla, 2019; Abbaszadeh et al., 2020). But ESCs-EVs are poorly studied in POI. Only one study suggests that ESCs-EVs could improve ovarian function by regulating the PI3K/AKT signaling pathway (Liu et al., 2020). Due to ESCs exhibiting strong self-renewal capability and pluripotency, ESCs-EVs are worth further exploration in POI.
Amniotic fluid stem cell-derived EVs (AFSCs-EVs)
Amniotic fluid is a rich source of stem cells that can be easily obtained through amniocentesis during standard prenatal care procedures (Di Trapani et al., 2015). The procedure to obtain AFSCs is non-invasive, safe, and without social controversy (Soncini et al., 2007; Parolini et al., 2008; Diaz-Prado et al., 2010). Hence, amniotic fluid stem cells seem to be the optimal source of EVs. Xiao et al. (2016) reveal that mouse AFSC-EVs contained two microRNAs (miRNAs), miR-146a and miR-10a, which inhibited apoptosis in damaged GCs and prevented ovarian follicles from atresia in mice following cyclophosphamide (CTX). Liu et al. (2012) find that CD44+/CD105+ human AFCs possess the characteristics of mesenchymal stem cells (Zou et al., 2011) and it can survive and proliferate over the long term in the ovarian tissues of a mouse model of chemotherapy-induced POI . Subsequently, AFSCs-EVs are isolated and used in POI. Geng et al. (2022b) indicate that CD44+/CD105+ human AFSC-EVs carrying miR-369-3p could specifically downregulate the expression of YAF2, inhibit the stability of PDCD5/p53, and reduce the apoptosis of OGCs, thereby exerting therapeutic effects on POI. In addition, compared with BMSCs, AFSCs secreted higher levels of EVs (Tracy et al., 2019). Therefore, AFSCs-EVs may be more valuable than other EVs.
Human adipose stem cells-derived EVs (hADSC-EVs)
It is widely accepted that adult stem cells can be found in abundance, readily accessible, and replenishable in adipose tissue. ADSCs are obtained from the subcutaneous adipose tissue removed during liposuction surgeries or abdominoplasties (Fu et al., 2021). Nowadays, ADSCs are widely used to treat various ailments of the skin. However, few studies have been reported on POI. Huang et al. (2018) reveal that the hADSC-EVs recover the ovarian function of POI by downregulating SMAD2, SMAD3, and SMAD5 expression. HADSC-conditioned media containing various cytokines and microvesicles secreted by HADSCs is concentrated and injected into the bilateral ovaries of POI rats in one study. The results show that hADSC-conditioned media injection partially reduces ovarian injury and improved ovarian function in rats with POI (Ling et al., 2019). We have reasons to believe that hADSC-EVs play an indispensable role. Certainly, more evidence is needed to demonstrate these linkages.
Menstrual blood stem cells-derived EVs (MenSCs-EVs)
MenSC-derived small EVs were first reported in 2016 (Lopez-Verrilli et al., 2016), and the authors revealed that MenSC-derived small EVs promote axonal regeneration after nerve injury in the central and peripheral nervous systems. MenSCs-EVs are still in the early stages of study, unlike some common MSC sources such as bone marrow, umbilical cord, and adipose tissue (Chen et al., 2021). Until now the only evidence suggests that GCs were proliferated in primordial and primary follicles by MenSCs-EVs, and apoptosis was inhibited. MenSCs-EVs also increased the expression of early follicle markers, for example, DAZL and FOXL2 (Zhang et al., 2021). In vivo, transplantation of MenSCs-EVs in the rat model of POI promoted follicle development and restored estrous cyclicity and serum sex hormone levels. In addition, by transplanting MenSCs-EVs, the extracellular matrix of the ovary was regulated and dormant follicles were recruited sooner. As a result, MenSCs-EVs significantly promoted follicle development in vitro and in vivo and restored fertility in POI rats.
Future challenges of stem cell-derived EVs
In the last few years, stem cell-derived EVs have emerged as a new therapeutic strategy for many diseases. Although multiple preclinical studies have shown that stem cell-derived EVs have positive effects in treating POI, it is far from being sufficient. Therefore, in order to benefit POI patients as quickly as possible, further pre-clinical and clinical studies are warranted. But the optimal source of EVs should be found before this time. Regrettably, there are no studies to compare the therapeutic effects of different EVs for POI. Moreover, due to the ultra-short duration of the development of EVs, their safety and consistent regulatory issue are not conclusive (Hu et al., 2022).
To isolate EVs from various cell types, tremendous effort has been devoted in the past. Currently, there is numerous separative technique of EVs including precipitation, membrane affinity, size-exclusion chromatography, iodixanol gradient, and phosphatidylserine affinity (Lee et al., 2021). But no method is perfect. Furthermore, many studies have reported the Effect of storage temperature and frozen/thawed cycles on EVs size and biological activity, and 80°C was chosen as the optimum temperature to ensure both treatment outcomes and transport capacity (Kusuma et al., 2018; Le Saux et al., 2020). It is also one of the main challenges for EVs to mass culture. The strategies for mass-production of EVs include modulating the components or secretary machinery proteins of EVs, increasing the intracellular Ca ions, adjusting biochemical cues such as extracellular DNA, liposomes, and proton concentration, and applying physicomechanical cues such as forces and other stimuli (e.g., electricity, thermal, photodynamic, and radiative stress) (Lee et al., 2021). It is worth mentioning that the development of nanotechnology and biomaterials provide a viable means with which to tackle the previously mentioned problems (Lee et al., 2021). However, related studies were not available in POI.
In conclusion, although stem cell-derived EVs hold great prospects in treating POI, the following questions also need to be addressed: 1) the optimal source of EVs; 2) the safety of stem cell-derived EVs; 3) the mass cultivation and preservation of stem cell-derived EVs; 4) the clinical evaluation of stem cell-derived EVs; 5) the mechanism of stem cell-derived EVs.
Conclusion
It is evident that stem cell-derived EVs have the potential in treating POI. However, more research was needed to investigate the mechanism of stem cell-derived EVs in POI and more efficient means for obtaining and preserving EVs to make benefit patients safer and faster.
Author contributions
ZXG: Draft the manuscript. YL: Data collection. HLG: Revise the manuscript. YFL: Revise the manuscript. YFZ: Quality control.
Funding
This work was supported by the Shanghai Chronic Osteopathy Clinical Medical Research Center (20MC1920600), Three-year Clinical Action Plan of Shanghai Shenkang Hospital Development Center (SHDC2020CR3090B), and Major Project of “Medical Innovation Research Project” of Shanghai Municipal Commission of Science and Technology (21Y3192020).
Conflict of interest
The authors declare that the research was conducted in the absence of any commercial or financial relationships that could be construed as a potential conflict of interest.
Publisher’s note
All claims expressed in this article are solely those of the authors and do not necessarily represent those of their affiliated organizations, or those of the publisher, the editors and the reviewers. Any product that may be evaluated in this article, or claim that may be made by its manufacturer, is not guaranteed or endorsed by the publisher.
References
Abbaszadeh, H., Ghorbani, F., Derakhshani, M., Movassaghpour, A., and Yousefi, M. (2020). Human umbilical cord mesenchymal stem cell-derived extracellular vesicles: A novel therapeutic paradigm. J. Cell Physiol. 235 (2), 706–717. doi:10.1002/jcp.29004
Andaluz Aguilar, H., Iliuk, A. B., Chen, I. H., and Tao, W. A. (2020). Sequential phosphoproteomics and N-glycoproteomics of plasma-derived extracellular vesicles. Nat. Protoc. 15 (1), 161–180. doi:10.1038/s41596-019-0260-5
Bae, Y. U., Son, Y., Kim, C. H., Kim, K. S., Hyun, S. H., Woo, H. G., et al. (2019). Embryonic stem cell-derived mmu-miR-291a-3p inhibits cellular senescence in human dermal fibroblasts through the TGF-beta receptor 2 pathway. J. Gerontol. A Biol. Sci. Med. Sci. 74 (9), 1359–1367. doi:10.1093/gerona/gly208
Cai, J. H., Sun, Y. T., and Bao, S. (2022). HucMSCs-exosomes containing miR-21 promoted estrogen production in ovarian granulosa cells via LATS1-mediated phosphorylation of LOXL2 and YAP. Gen. Comp. Endocrinol. 321-322, 114015. doi:10.1016/j.ygcen.2022.114015
Chen, A., Tiosano, D., Guran, T., Baris, H. N., Bayram, Y., Mory, A., et al. (2018). Mutations in the mitochondrial ribosomal protein MRPS22 lead to primary ovarian insufficiency. Hum. Mol. Genet. 27 (11), 1913–1926. doi:10.1093/hmg/ddy098
Chen, L., Qu, J., Mei, Q., Chen, X., Fang, Y., Chen, L., et al. (2021). Small extracellular vesicles from menstrual blood-derived mesenchymal stem cells (MenSCs) as a novel therapeutic impetus in regenerative medicine. Stem Cell Res. Ther. 12 (1), 433. doi:10.1186/s13287-021-02511-6
Clevers, H., Loh, K. M., and Nusse, R. (2014). Stem cell signaling. An integral program for tissue renewal and regeneration: Wnt signaling and stem cell control. Science 346 (6205), 1248012. doi:10.1126/science.1248012
Deng, T., He, J., Yao, Q., Wu, L., Xue, L., Wu, M., et al. (2021). Human umbilical cord mesenchymal stem cells improve ovarian function in chemotherapy-induced premature ovarian failure mice through inhibiting apoptosis and inflammation via a paracrine mechanism. Reprod. Sci. 28 (6), 1718–1732. doi:10.1007/s43032-021-00499-1
Di Trapani, M., Bassi, G., Fontana, E., Giacomello, L., Pozzobon, M., Guillot, P. V., et al. (2015). Immune regulatory properties of CD117(pos) amniotic fluid stem cells vary according to gestational age. Stem Cells Dev. 24 (1), 132–143. doi:10.1089/scd.2014.0234
Diaz-Prado, S., Muinos-Lopez, E., Hermida-Gomez, T., Rendal-Vazquez, M. E., Fuentes-Boquete, I., de Toro, F. J., et al. (2010). Multilineage differentiation potential of cells isolated from the human amniotic membrane. J. Cell. Biochem. 111 (4), 846–857. doi:10.1002/jcb.22769
Ding, C., Zhu, L., Shen, H., Lu, J., Zou, Q., Huang, C., et al. (2020). Exosomal miRNA-17-5p derived from human umbilical cord mesenchymal stem cells improves ovarian function in premature ovarian insufficiency by regulating SIRT7. Stem Cells 38 (9), 1137–1148. doi:10.1002/stem.3204
Ding, L., Yan, G., Wang, B., Xu, L., Gu, Y., Ru, T., et al. (2018). Transplantation of UC-MSCs on collagen scaffold activates follicles in dormant ovaries of POF patients with long history of infertility. Sci. China Life Sci. 61 (12), 1554–1565. doi:10.1007/s11427-017-9272-2
Doeppner, T. R., Herz, J., Gorgens, A., Schlechter, J., Ludwig, A. K., Radtke, S., et al. (2015). Extracellular vesicles improve post-stroke neuroregeneration and prevent postischemic immunosuppression. Stem Cells Transl. Med. 4 (10), 1131–1143. doi:10.5966/sctm.2015-0078
Dragojevic-Dikic, S., Marisavljevic, D., Mitrovic, A., Dikic, S., Jovanovic, T., and Jankovic-Raznatovic, S. (2010). An immunological insight into premature ovarian failure (POF). Autoimmun. Rev. 9 (11), 771–774. doi:10.1016/j.autrev.2010.06.008
El Bakly, W., Medhat, M., Shafei, M., Tash, R., Elrefai, M., Shoukry, Y., et al. (2020). Optimized platelet rich plasma releasate (O-rPRP) repairs galactosemia-induced ovarian follicular loss in rats by activating mTOR signaling and inhibiting apoptosis. Heliyon 6 (9), e05006. doi:10.1016/j.heliyon.2020.e05006
Ezoe, K., Murata, N., Yabuuchi, A., Okuno, T., Kobayashi, T., Kato, O., et al. (2014). Long-term adverse effects of cyclophosphamide on follicular growth and angiogenesis in mouse ovaries. Reprod. Biol. 14 (3), 238–242. doi:10.1016/j.repbio.2014.04.007
Fang, S. B., Zhang, H. Y., Wang, C., He, B. X., Liu, X. Q., Meng, X. C., et al. (2020). Small extracellular vesicles derived from human mesenchymal stromal cells prevent group 2 innate lymphoid cell-dominant allergic airway inflammation through delivery of miR-146a-5p. J. Extracell. Vesicles 9 (1), 1723260. doi:10.1080/20013078.2020.1723260
Franca, M. M., and Mendonca, B. B. (2020). Genetics of primary ovarian insufficiency in the next-generation sequencing era. J. Endocr. Soc. 4 (2), bvz037. doi:10.1210/jendso/bvz037
Fu, X., He, Y., Wang, X., Peng, D., Chen, X., Li, X., et al. (2017). Overexpression of miR-21 in stem cells improves ovarian structure and function in rats with chemotherapy-induced ovarian damage by targeting PDCD4 and PTEN to inhibit granulosa cell apoptosis. Stem Cell Res. Ther. 8 (1), 187. doi:10.1186/s13287-017-0641-z
Fu, Y. X., Ji, J., Shan, F., Li, J., and Hu, R. (2021). Human mesenchymal stem cell treatment of premature ovarian failure: New challenges and opportunities. Stem Cell Res. Ther. 12 (1), 161. doi:10.1186/s13287-021-02212-0
Gao, T., Cao, Y., Hu, M., and Du, Y. (2022). Human umbilical cord mesenchymal stem cell-derived extracellular vesicles carrying MicroRNA-29a improves ovarian function of mice with primary ovarian insufficiency by targeting HMG-box transcription factor/wnt/β-catenin signaling. Dis. Markers 2022, 5045873. doi:10.1155/2022/5045873
Geng, Z., Chen, H., Zou, G., Yuan, L., Liu, P., Li, B., et al. (2022). Human amniotic fluid mesenchymal stem cell-derived exosomes inhibit apoptosis in ovarian granulosa cell via miR-369-3p/YAF2/PDCD5/p53 pathway. Oxid. Med. Cell Longev. 2022, 3695848. doi:10.1155/2022/3695848
Geng, Z., Liu, P., Yuan, L., Zhang, K., Lin, J., Nie, X., et al. (2022). Electroacupuncture attenuates ac4C modification of P16 mRNA in the ovarian granulosa cells of a mouse model premature ovarian failure. Acupunct. Med. J. Br. Med. Acupunct. Soc. 41, 27–37. doi:10.1177/09645284221085284
Gnecchi, M., He, H., Liang, O. D., Melo, L. G., Morello, F., Mu, H., et al. (2005). Paracrine action accounts for marked protection of ischemic heart by Akt-modified mesenchymal stem cells. Nat. Med. 11 (4), 367–368. doi:10.1038/nm0405-367
Ha, M., and Kim, V. N. (2014). Regulation of microRNA biogenesis. Nat. Rev. Mol. Cell Biol. 15 (8), 509–524. doi:10.1038/nrm3838
Hade, M. D., Suire, C. N., Mossell, J., and Suo, Z. (2022). Extracellular vesicles: Emerging frontiers in wound healing. Med. Res. Rev. 42 (6), 2102–2125. doi:10.1002/med.21918
Han, Z., Liu, S., Pei, Y., Ding, Z., Li, Y., Wang, X., et al. (2021). Highly efficient magnetic labelling allows MRI tracking of the homing of stem cell-derived extracellular vesicles following systemic delivery. J. Extracell. Vesicles 10 (3), e12054. doi:10.1002/jev2.12054
He, Y., Chen, D., Yang, L., Hou, Q., Ma, H., and Xu, X. (2018). The therapeutic potential of bone marrow mesenchymal stem cells in premature ovarian failure. Stem Cell Res. Ther. 9 (1), 263. doi:10.1186/s13287-018-1008-9
Hu, J. C., Zheng, C. X., Sui, B. D., Liu, W. J., and Jin, Y. (2022). Mesenchymal stem cell-derived exosomes: A novel and potential remedy for cutaneous wound healing and regeneration. World J. Stem Cells 14 (5), 318–329. doi:10.4252/wjsc.v14.i5.318
Huang, B., Lu, J., Ding, C., Zou, Q., Wang, W., and Li, H. (2018). Exosomes derived from human adipose mesenchymal stem cells improve ovary function of premature ovarian insufficiency by targeting SMAD. Stem Cell Res. Ther. 9 (1), 216. doi:10.1186/s13287-018-0953-7
Huang, L. H., Rau, C. S., Wu, S. C., Wu, Y. C., Wu, C. J., Tsai, C. W., et al. (2021). Identification and characterization of hADSC-derived exosome proteins from different isolation methods. J. Cell Mol. Med. 25 (15), 7436–7450. doi:10.1111/jcmm.16775
Kalluri, R., and LeBleu, V. S. (2020). The biology, function, and biomedical applications of exosomes. Science 2020367 (6478). doi:10.1126/science.aau6977
Kamat, B. R., Brown, L. F., Manseau, E. J., Senger, D. R., and Dvorak, H. F. (1995). Expression of vascular permeability factor/vascular endothelial growth factor by human granulosa and theca lutein cells. Role in corpus luteum development. Am. J. Pathol. 146 (1), 157–165.
Khan, M., Nickoloff, E., Abramova, T., Johnson, J., Verma, S. K., Krishnamurthy, P., et al. (2015). Embryonic stem cell-derived exosomes promote endogenous repair mechanisms and enhance cardiac function following myocardial infarction. Circ. Res. 117 (1), 52–64. doi:10.1161/CIRCRESAHA.117.305990
Kovanci, E., and Schutt, A. K. (2015). Premature ovarian failure: Clinical presentation and treatment. Obstet. Gynecol. Clin. North Am. 42 (1), 153–161. doi:10.1016/j.ogc.2014.10.004
Kusuma, G. D., Barabadi, M., Tan, J. L., Morton, D. A. V., Frith, J. E., and Lim, R. (2018). To protect and to preserve: Novel preservation strategies for extracellular vesicles. Front. Pharmacol. 9, 1199. doi:10.3389/fphar.2018.01199
Le Saux, S., Aarrass, H., Lai-Kee-Him, J., Bron, P., Armengaud, J., Miotello, G., et al. (2020). Post-production modifications of murine mesenchymal stem cell (mMSC) derived extracellular vesicles (EVs) and impact on their cellular interaction. Biomaterials 231, 119675. doi:10.1016/j.biomaterials.2019.119675
Lee, J. H., Yoon, J. Y., Lee, J. H., Lee, H. H., Knowles, J. C., and Kim, H. W. (2021). Emerging biogenesis technologies of extracellular vesicles for tissue regenerative therapeutics. J. Tissue Eng. 12, 20417314211019015. doi:10.1177/20417314211019015
Li, G. Q., Fang, Y. X., Liu, Y., Meng, F. R., Wu, X., Zhang, C. W., et al. (2021). MicroRNA-21 from bone marrow mesenchymal stem cell-derived extracellular vesicles targets TET1 to suppress KLF4 and alleviate rheumatoid arthritis. Ther. Adv. Chronic Dis. 12, 20406223211007369. doi:10.1177/20406223211007369
Li, Z., Liu, F., He, X., Yang, X., Shan, F., and Feng, J. (2019). Exosomes derived from mesenchymal stem cells attenuate inflammation and demyelination of the central nervous system in EAE rats by regulating the polarization of microglia. Int. Immunopharmacol. 67, 268–280. doi:10.1016/j.intimp.2018.12.001
Li, Z., Zhang, M., Zheng, J., Tian, Y., Zhang, H., Tan, Y., et al. (2021). Human umbilical cord mesenchymal stem cell-derived exosomes improve ovarian function and proliferation of premature ovarian insufficiency by regulating the Hippo signaling pathway. Front. Endocrinol. (Lausanne) 12, 711902. doi:10.3389/fendo.2021.711902
Liao, H. T., and Chen, C. T. (2014). Osteogenic potential: Comparison between bone marrow and adipose-derived mesenchymal stem cells. World J. Stem Cells 6 (3), 288–295. doi:10.4252/wjsc.v6.i3.288
Ling, L., Feng, X., Wei, T., Wang, Y., Wang, Y., Wang, Z., et al. (2019). Human amnion-derived mesenchymal stem cell (hAD-MSC) transplantation improves ovarian function in rats with premature ovarian insufficiency (POI) at least partly through a paracrine mechanism. Stem Cell Res. Ther. 10 (1), 46. doi:10.1186/s13287-019-1136-x
Liu, M., Qiu, Y., Xue, Z., Wu, R., Li, J., Niu, X., et al. (2020). Small extracellular vesicles derived from embryonic stem cells restore ovarian function of premature ovarian failure through PI3K/AKT signaling pathway. Stem Cell Res. Ther. 11 (1), 3. doi:10.1186/s13287-019-1508-2
Liu, T., Huang, Y., Guo, L., Cheng, W., and Zou, G. (2012). CD44+/CD105+ human amniotic fluid mesenchymal stem cells survive and proliferate in the ovary long-term in a mouse model of chemotherapy-induced premature ovarian failure. Int. J. Med. Sci. 9 (7), 592–602. doi:10.7150/ijms.4841
Liu, T., Jing, F., Huang, P., Geng, Z., Xu, J., Li, J., et al. (2021). Thymopentin alleviates premature ovarian failure in mice by activating YY2/Lin28A and inhibiting the expression of let-7 family microRNAs. Cell Prolif. 54 (8), e13089. doi:10.1111/cpr.13089
Lopez-Verrilli, M. A., Caviedes, A., Cabrera, A., Sandoval, S., Wyneken, U., and Khoury, M. (2016). Mesenchymal stem cell-derived exosomes from different sources selectively promote neuritic outgrowth. Neuroscience 320, 129–139. doi:10.1016/j.neuroscience.2016.01.061
Lund, R. J., Narva, E., and Lahesmaa, R. (2012). Genetic and epigenetic stability of human pluripotent stem cells. Nat. Rev. Genet. 13 (10), 732–744. doi:10.1038/nrg3271
Martello, G., and Smith, A. (2014). The nature of embryonic stem cells. Annu. Rev. Cell Dev. Biol. 30, 647–675. doi:10.1146/annurev-cellbio-100913-013116
Mashayekhi, M., Mirzadeh, E., Chekini, Z., Ahmadi, F., Eftekhari-Yazdi, P., Vesali, S., et al. (2021). Evaluation of safety, feasibility and efficacy of intra-ovarian transplantation of autologous adipose derived mesenchymal stromal cells in idiopathic premature ovarian failure patients: Non-randomized clinical trial, phase I, first in human. J. Ovarian Res. 14 (1), 5. doi:10.1186/s13048-020-00743-3
Mashouri, L., Yousefi, H., Aref, A. R., Ahadi, A. M., Molaei, F., and Alahari, S. K. (2019). Exosomes: Composition, biogenesis, and mechanisms in cancer metastasis and drug resistance. Mol. Cancer 18 (1), 75. doi:10.1186/s12943-019-0991-5
Mathivanan, S., Ji, H., and Simpson, R. J. (2010). Exosomes: Extracellular organelles important in intercellular communication. J. Proteomics 73 (10), 1907–1920. doi:10.1016/j.jprot.2010.06.006
Meirow, D., and Nugent, D. (2001). The effects of radiotherapy and chemotherapy on female reproduction. Hum. Reprod. Update 7 (6), 535–543. doi:10.1093/humupd/7.6.535
Mittelbrunn, M., and Sanchez-Madrid, F. (2012). Intercellular communication: Diverse structures for exchange of genetic information. Nat. Rev. Mol. Cell Biol. 13 (5), 328–335. doi:10.1038/nrm3335
Moslehi, N., Mirmiran, P., Azizi, F., and Tehrani, F. R. (2019). Do dietary intakes influence the rate of decline in anti-mullerian hormone among eumenorrheic women? A population-based prospective investigation. Nutr. J. 18 (1), 83. doi:10.1186/s12937-019-0508-5
Naleway, A. L., Mittendorf, K. F., Irving, S. A., Henninger, M. L., Crane, B., Smith, N., et al. (2018). Primary ovarian insufficiency and adolescent vaccination. Pediatrics 142 (3), e20180943. doi:10.1542/peds.2018-0943
Nguyen, P. K., Neofytou, E., Rhee, J. W., and Wu, J. C. (2016). Potential strategies to address the major clinical barriers facing stem cell regenerative therapy for cardiovascular disease: A review. JAMA Cardiol. 1 (8), 953–962. doi:10.1001/jamacardio.2016.2750
Nippita, T. A., and Baber, R. J. (2007). Premature ovarian failure: A review. Climacteric 10 (1), 11–22. doi:10.1080/13697130601135672
Ohtsuka, S., and Dalton, S. (2008). Molecular and biological properties of pluripotent embryonic stem cells. Gene Ther. 15 (2), 74–81. doi:10.1038/sj.gt.3303065
Panay, N., and Fenton, A. (2008). Premature ovarian failure: A growing concern. Climacteric 11 (1), 1–3. doi:10.1080/13697130701878635
Parolini, O., Alviano, F., Bagnara, G. P., Bilic, G., Buhring, H. J., Evangelista, M., et al. (2008). Concise review: Isolation and characterization of cells from human term placenta: Outcome of the first international workshop on placenta derived stem cells. Stem Cells 26 (2), 300–311. doi:10.1634/stemcells.2007-0594
Poulos, J. (2018). The limited application of stem cells in medicine: A review. Stem Cell Res. Ther. 9 (1), 1. doi:10.1186/s13287-017-0735-7
Qin, Y., Jiao, X., Simpson, J. L., and Chen, Z. J. (2015). Genetics of primary ovarian insufficiency: New developments and opportunities. Hum. Reprod. Update 21 (6), 787–808. doi:10.1093/humupd/dmv036
Qu, Q., Liu, L., Cui, Y., Liu, H., Yi, J., Bing, W., et al. (2022). miR-126-3p containing exosomes derived from human umbilical cord mesenchymal stem cells promote angiogenesis and attenuate ovarian granulosa cell apoptosis in a preclinical rat model of premature ovarian failure. Stem Cell Res. Ther. 13 (1), 352. doi:10.1186/s13287-022-03056-y
Riazifar, M., Pone, E. J., Lotvall, J., and Zhao, W. (2017). Stem cell extracellular vesicles: Extended messages of regeneration. Annu. Rev. Pharmacol. Toxicol. 57, 125–154. doi:10.1146/annurev-pharmtox-061616-030146
Robinson, R. S., Woad, K. J., Hammond, A. J., Laird, M., Hunter, M. G., and Mann, G. E. (2009). Angiogenesis and vascular function in the ovary. Reproduction 138 (6), 869–881. doi:10.1530/REP-09-0283
Rostami Dovom, M., Noroozzadeh, M., Mosaffa, N., Zadeh-Vakili, A., Piryaei, A., and Ramezani Tehrani, F. (2019). Induced premature ovarian insufficiency by using D galactose and its effects on reproductive profiles in small laboratory animals: A systematic review. J. Ovarian Res. 12 (1), 96. doi:10.1186/s13048-019-0565-6
Shelling, A. N. (2010). Premature ovarian failure. Reproduction 140 (5), 633–641. doi:10.1530/REP-09-0567
Soncini, M., Vertua, E., Gibelli, L., Zorzi, F., Denegri, M., Albertini, A., et al. (2007). Isolation and characterization of mesenchymal cells from human fetal membranes. J. Tissue Eng. Regen. Med. 1 (4), 296–305. doi:10.1002/term.40
Stahl, P. D., and Raposo, G. (2019). Extracellular vesicles: Exosomes and microvesicles, integrators of homeostasis. Physiol. (Bethesda) 34 (3), 169–177. doi:10.1152/physiol.00045.2018
Su, Y., Song, X., Teng, J., Zhou, X., Dong, Z., Li, P., et al. (2021). Mesenchymal stem cells-derived extracellular vesicles carrying microRNA-17 inhibits macrophage apoptosis in lipopolysaccharide-induced sepsis. Int. Immunopharmacol. 95, 107408. doi:10.1016/j.intimp.2021.107408
Sun, B., Ma, Y., Wang, F., Hu, L., and Sun, Y. (2019). miR-644-5p carried by bone mesenchymal stem cell-derived exosomes targets regulation of p53 to inhibit ovarian granulosa cell apoptosis. Stem Cell Res. Ther. 10 (1), 360. doi:10.1186/s13287-019-1442-3
Sun, L., Li, D., Song, K., Wei, J., Yao, S., Li, Z., et al. (2017). Exosomes derived from human umbilical cord mesenchymal stem cells protect against cisplatin-induced ovarian granulosa cell stress and apoptosis in vitro. Sci. Rep. 7 (1), 2552. doi:10.1038/s41598-017-02786-x
Szeliga, A., Calik-Ksepka, A., Maciejewska-Jeske, M., Grymowicz, M., Smolarczyk, K., Kostrzak, A., et al. (2021). Autoimmune diseases in patients with premature ovarian insufficiency-our current state of knowledge. Int. J. Mol. Sci. 22 (5), 2594. doi:10.3390/ijms22052594
Tang, Y., Zhou, Y., and Li, H. J. (2021). Advances in mesenchymal stem cell exosomes: A review. Stem Cell Res. Ther. 12 (1), 71. doi:10.1186/s13287-021-02138-7
Tavakoli Dargani, Z., and Singla, D. K. (2019). Embryonic stem cell-derived exosomes inhibit doxorubicin-induced TLR4-NLRP3-mediated cell death-pyroptosis. Am. J. Physiol. Heart Circ. Physiol. 317 (2), H460–H471. doi:10.1152/ajpheart.00056.2019
Tracy, S. A., Ahmed, A., Tigges, J. C., Ericsson, M., Pal, A. K., Zurakowski, D., et al. (2019). A comparison of clinically relevant sources of mesenchymal stem cell-derived exosomes: Bone marrow and amniotic fluid. J. Pediatr. Surg. 54 (1), 86–90. doi:10.1016/j.jpedsurg.2018.10.020
van Niel, G., D'Angelo, G., and Raposo, G. (2018). Shedding light on the cell biology of extracellular vesicles. Nat. Rev. Mol. Cell Biol. 19 (4), 213–228. doi:10.1038/nrm.2017.125
Villarroya-Beltri, C., Baixauli, F., Gutierrez-Vazquez, C., Sanchez-Madrid, F., and Mittelbrunn, M. (2014). Sorting it out: Regulation of exosome loading. Semin. Cancer Biol. 28, 3–13. doi:10.1016/j.semcancer.2014.04.009
Vohra, M., Sharma, A., Bagga, R., and Arora, S. K. (2020). Human umbilical cord-derived mesenchymal stem cells induce tissue repair and regeneration in collagen-induced arthritis in rats. J. Clin. Transl. Res. 6 (6), 203–216.
Wang, L., Mei, Q., Xie, Q., Li, H., Su, P., Zhang, L., et al. (2022). A comparative study of Mesenchymal Stem Cells transplantation approach to antagonize age-associated ovarian hypofunction with consideration of safety and efficiency. J. Adv. Res. 38, 245–259. doi:10.1016/j.jare.2021.09.001
Wang, W., Peng, X., Zhao, L., Zhao, H., and Gu, Q. (2022). Extracellular vesicles from bone marrow mesenchymal stem cells inhibit apoptosis and autophagy of ischemia-hypoxia cardiomyocyte line in vitro by carrying miR-144-3p to inhibit ROCK1. Curr. Stem Cell Res. Ther. 18, 247–259. doi:10.2174/1574888X17666220503192941
Watanabe, Y., Tsuchiya, A., and Terai, S. (2021). The development of mesenchymal stem cell therapy in the present, and the perspective of cell-free therapy in the future. Clin. Mol. Hepatol. 27 (1), 70–80. doi:10.3350/cmh.2020.0194
Wen, T., Wang, H., Li, Y., Lin, Y., Zhao, S., Liu, J., et al. (2021). Bone mesenchymal stem cell-derived extracellular vesicles promote the repair of intervertebral disc degeneration by transferring microRNA-199a. Cell Cycle 20 (3), 256–270. doi:10.1080/15384101.2020.1863682
Williams, K. J., Picou, A. A., Kish, S. L., Giraldo, A. M., Godke, R. A., and Bondioli, K. R. (2008). Isolation and characterization of porcine adipose tissue-derived adult stem cells. Cells Tissues Organs 188 (3), 251–258. doi:10.1159/000121431
Xia, L., Zhang, C., Lv, N., Liang, Z., Ma, T., Cheng, H., et al. (2022). AdMSC-derived exosomes alleviate acute lung injury via transferring mitochondrial component to improve homeostasis of alveolar macrophages. Theranostics 12 (6), 2928–2947. doi:10.7150/thno.69533
Xiao, G. Y., Cheng, C. C., Chiang, Y. S., Cheng, W. T., Liu, I. H., and Wu, S. C. (2016). Exosomal miR-10a derived from amniotic fluid stem cells preserves ovarian follicles after chemotherapy. Sci. Rep. 6, 23120. doi:10.1038/srep23120
Xiong, W. P., Yao, W. Q., Wang, B., and Liu, K. (2021). BMSCs-exosomes containing GDF-15 alleviated SH-SY5Y cell injury model of Alzheimer's disease via AKT/GSK-3β/β-catenin. Brain Res. Bull. 177, 92–102. doi:10.1016/j.brainresbull.2021.09.008
Xu, M., Feng, T., Liu, B., Qiu, F., Xu, Y., Zhao, Y., et al. (2021). Engineered exosomes: Desirable target-tracking characteristics for cerebrovascular and neurodegenerative disease therapies. Theranostics 11 (18), 8926–8944. doi:10.7150/thno.62330
Xu, R., Rai, A., Chen, M., Suwakulsiri, W., Greening, D. W., and Simpson, R. J. (2018). Extracellular vesicles in cancer - implications for future improvements in cancer care. Nat. Rev. Clin. Oncol. 15 (10), 617–638. doi:10.1038/s41571-018-0036-9
Yamanaka, S. (2020). Pluripotent stem cell-based cell therapy-promise and challenges. Cell Stem Cell 27 (4), 523–531. doi:10.1016/j.stem.2020.09.014
Yang, M., Lin, L., Sha, C., Li, T., Zhao, D., Wei, H., et al. (2020). Bone marrow mesenchymal stem cell-derived exosomal miR-144-5p improves rat ovarian function after chemotherapy-induced ovarian failure by targeting PTEN. Lab. Invest 100 (3), 342–352. doi:10.1038/s41374-019-0321-y
Yang, Z., Du, X., Wang, C., Zhang, J., Liu, C., Li, Y., et al. (2019). Therapeutic effects of human umbilical cord mesenchymal stem cell-derived microvesicles on premature ovarian insufficiency in mice. Stem Cell Res. Ther. 10 (1), 250. doi:10.1186/s13287-019-1327-5
Yin, N., Wu, C., Qiu, J., Zhang, Y., Bo, L., Xu, Y., et al. (2020). Protective properties of heme oxygenase-1 expressed in umbilical cord mesenchymal stem cells help restore the ovarian function of premature ovarian failure mice through activating the JNK/Bcl-2 signal pathway-regulated autophagy and upregulating the circulating of CD8(+)CD28(-) T cells. Stem Cell Res. Ther. 11 (1), 49. doi:10.1186/s13287-019-1537-x
Yu, T., Chu, S., Liu, X., Li, J., Chen, Q., Xu, M., et al. (2021). Extracellular vesicles derived from EphB2-overexpressing bone marrow mesenchymal stem cells ameliorate DSS-induced colitis by modulating immune balance. Stem Cell Res. Ther. 12 (1), 181. doi:10.1186/s13287-021-02232-w
Zhang, J., Yin, H., Jiang, H., Du, X., and Yang, Z. (2020). The protective effects of human umbilical cord mesenchymal stem cell-derived extracellular vesicles on cisplatin-damaged granulosa cells. Taiwan J. Obstet. Gynecol. 59 (4), 527–533. doi:10.1016/j.tjog.2020.05.010
Zhang, L., Zhang, S., Yao, J., Lowery, F. J., Zhang, Q., Huang, W. C., et al. (2015). Microenvironment-induced PTEN loss by exosomal microRNA primes brain metastasis outgrowth. Nature 527 (7576), 100–104. doi:10.1038/nature15376
Zhang, Q., Sun, J., Huang, Y., Bu, S., Guo, Y., Gu, T., et al. (2019). Human amniotic epithelial cell-derived exosomes restore ovarian function by transferring MicroRNAs against apoptosis. Mol. Ther. Nucleic Acids 16, 407–418. doi:10.1016/j.omtn.2019.03.008
Zhang, S., Huang, B., Su, P., Chang, Q., Li, P., Song, A., et al. (2021). Concentrated exosomes from menstrual blood-derived stromal cells improves ovarian activity in a rat model of premature ovarian insufficiency. Stem Cell Res. Ther. 12 (1), 178. doi:10.1186/s13287-021-02255-3
Zhang, Y., Xu, J., Liu, S., Lim, M., Zhao, S., Cui, K., et al. (2019). Embryonic stem cell-derived extracellular vesicles enhance the therapeutic effect of mesenchymal stem cells. Theranostics 9 (23), 6976–6990. doi:10.7150/thno.35305
Zhu, X., Liu, J., Pan, H., Geng, Z., Huang, W., Liu, T., et al. (2021). Thymopentin treatment of murine premature ovarian failure via attenuation of immune cell activity and promotion of the BMP4/Smad9 signalling pathway. Int. J. Med. Sci. 18 (15), 3544–3555. doi:10.7150/ijms.61975
Keywords: primary ovarian insufficiency, premature ovarian failure, stem cells, extracellular vesicles, exosomes
Citation: Geng Z, Guo H, Li Y, Liu Y and Zhao Y (2023) Stem cell-derived extracellular vesicles: A novel and potential remedy for primary ovarian insufficiency. Front. Cell Dev. Biol. 11:1090997. doi: 10.3389/fcell.2023.1090997
Received: 06 November 2022; Accepted: 06 February 2023;
Published: 15 February 2023.
Edited by:
Dolores Busso, Universidad de los Andes, ChileReviewed by:
Jinxiang Wu, The Second Affiliated Hospital of Fujian Medical University, ChinaSusana B. Rulli, Universidad Maimónides, Argentina
Hang-Soo Park, The University of Chicago, United States
Copyright © 2023 Geng, Guo, Li, Liu and Zhao. This is an open-access article distributed under the terms of the Creative Commons Attribution License (CC BY). The use, distribution or reproduction in other forums is permitted, provided the original author(s) and the copyright owner(s) are credited and that the original publication in this journal is cited, in accordance with accepted academic practice. No use, distribution or reproduction is permitted which does not comply with these terms.
*Correspondence: Zixiang Geng, gengzx@foxmail.com; Ying Liu, liuying19@mail.jlu.edu.cn; Yongfang Zhao, zhao_dingding@126.com