- 1Department of Obstetrics, Gynecology and Reproductive Sciences, University of Maryland School of Medicine, Baltimore, MD, United States
- 2Center for Metabolic Disease Research, Department of Cardiovascular Sciences, Temple University School of Medicine, Philadelphia, PA, United States
- 3Departmentof Physiology and Biophysics, Howard University College of Medicine, Washington, DC, United States
Maternal obesity is associated with a variety of obstetrical outcomes including stillbirth, preeclampsia, and gestational diabetes, and increases the risk of fetuses for congenital heart defects. Obesity during pregnancy represents a major contribution to metabolic dysregulation, which not only plays a key role in the pathogenesis of adverse outcome but also can potently induce endoplasmic reticulum (ER) stress. However, the mechanism associating such an obesogenic metabolic environment and adverse pregnancy outcomes has remained poorly understood. In this study, we aimed to determine whether the ER stress pathways (also named unfolded protein response (UPR)) were activated in the placenta by obesity. We collected placenta from the obese pregnancy (n = 12) and non-obese pregnancy (n = 12) following delivery by Caesarean-section at term. The specimens were assessed with immunocytochemistry staining and RT-QPCR. Our results revealed that in the obese placenta, p-IRE1α and XBP1s were significantly increased, CHOP and nine UPR chaperone genes were upregulated, including GRP95, PDIA6, Calnexin, p58IPK, SIL-1, EDEM, Herp, GRP58 and Calreticulin. However, Perk and BiP are not activated in the obese placenta. Our data suggest that upregulated p-IRE1α and XBP1s signaling, and UPR chaperone genes may play an important role in maternal obesity-induced placental pathology. In conclusion, this is the first report on ER stress and UPR activation in the placenta of maternal obesity. Our findings represent the first step in the understanding of one of the key ER signaling pathways, also referred to IRE1α-XBP1, in placental pathophysiology affected by obesity, which may be an important mechanism accounting for the observed higher maternal and perinatal risks.
Introduction
Modern society is continuing to face the increasing challenge of the elevated incidence rate of obesity, which presents a severe detriment to human health as well as a major cause of morbidity and mortality across generations (Brombach et al., 2022). Maternal obesity is significantly associated with a variety of obstetrical outcomes including stillbirth, preeclampsia, and gestational diabetes (Rubens et al., 2022). Furthermore, fetuses are at increased risk for congenital birth defects and offspring are more likely to develop cardiometabolic diseases in later life (Whitaker et al., 1997; Blomberg and Kallen, 2010; Reynolds et al., 2013; Gaillard et al., 2014a; Gaillard et al., 2014b).
Maternal obesity is typically characterized with alterations in circulating levels of various substances including hormones, nutrients, growth factors, cytokines, and lipids. However, the mechanisms associated with such an obesogenic metabolic environment and short- and long-term adverse pregnancy outcomes have remained poorly understood (Kelly et al., 2020). The placenta, constituting the interface between the mother and fetus, plays a key role in mediating nutrient and gas exchange to sustain and ensure a successful pregnancy (Jansson and Powell, 2013). Mounting evidence supports that maternal obesity is a leading cause of extensive changes in placental function, which predominantly accounts for maternal obesity-derived adverse effects on fetal development (Kelly et al., 2020). While the research on fetal and maternal outcomes has been well conducted, however, investigation on the effects of maternal obesity effects on the placenta and their subsequent link with obstetrical outcomes has remained relatively limited.
Obesity during pregnancy represents a major contributor to metabolic dysregulation, which not only plays a key role in the pathogenesis of adverse outcomes but also can potently induce endoplasmic reticulum (ER) stress (Park et al., 2020). ER is the major site allowing the folding and maturation of proteins. ER stress can be detected in nearly all tissues with elevated metabolic and endocrine demands in obese individuals. Nevertheless, under an obesogenic condition, ER stress is largely dependent on insulin resistance in adipose tissues. ER stress-response pathways, also known as the unfolded protein response (UPR), are comprised of three highly conserved signaling pathways: ATF6 and IRE1-XBP1 pathways that enhance folding capacity by upregulating the activity of ER chaperones such as GRP78 and heat shock protein 90 kDa beta ember 1 (GRP94) and promote phospholipid synthesis, and PERK-eIF2α pathway that attenuates non-essential protein synthesis (Yung et al., 2014; Adams et al., 2019). Furthermore, the ER-associated protein degradation (ERAD) pathway functions by promoting protein degradation (Schroder and Kaufman, 2005).
A number of studies have explicitly demonstrated that cell signaling downstream inflammatory, metabolic, and oxidative stress mediates the effects of maternal diabetes on placental function (Brombach et al., 2022). In this study, we focused on ER stress and UPR activation in the placenta from a pregnancy complicated with maternal obesity. We used a variety of biochemical approaches including immunohistochemistry and quantitative PCR to accomplish our goal.
Materials and methods
Study design
As described in our previous study (Yao et al., 2022), patients admitted for scheduled cesarean delivery at the University of Maryland Medical Center were enrolled for this study. The enrollment criteria: patients at least 18 years old, at least 37 weeks gestational age based on clinical dating criteria; unlabored and scheduled for cesarean delivery; and patients without a diagnosis of diabetes or hypertension, chorioamnionitis, abnormal liver function or fetal growth restriction. Patients were classified as non-obese if pre-pregnancy maternal BMI was between 18.5 and 24.9 kg/m2 or obese if pre-pregnancy BMI was greater than or equal to 30.0 kg/m2. A total of 24 subjects (12 normal-weight and 12 obese patients) were enrolled from October 2016 through December 2017. This study was approved by the University of Maryland Internal Review Board (IRB# HP-00066908).
Reverse transcription-quantitative PCR (RT-qPCR)
Total RNA was isolated from the placenta using a RNeasy minikit (Qiagene, Valencia, CA), reverse transcribed using the QuantiTect Reverse Transcription kit (Qiagen, Valencia, CA), and followed by QPCR. QPCR were performed using PowerUP Sybr Green Master Mix (Thermofisher Scientific, Waltham, MA; Cat #A25779). QPCR primer sequences for XBP1s, CHOP, and UPR chaperone genes were listed in Table 1.
Immunocytochemistry staining
Formalin-fixed, paraffin-embedded (FFPE) placenta tissues were sectioned. The immunocytochemistry staining was performed as described (Xu et al., 2021; Shen et al., 2022). The sections were deparaffined and rehydrated followed by antigen retrieval (Citrate buffer, pH6) and H2O2 treatment (2%, 15 min). Sections were incubated in blocking solution (phosphate-buffered saline (PBS) containing 4% normal goat serum, 0.2% Triton X-100) for 1 h, then with the primary antibodies diluted in block solution at 4°C overnight. Sections were incubated with biotinylated secondary antibody for 1 h and followed by 1 h with ABC solution (VectorLab, Elite Kit, Cat# PK6100, 1:500). Antibody labeling was visualized in diaminobenzidine (DAB, 10 mg/50 mL) chromogen in 0.175 M sodium acetate +0.003% H2O2 (brown products). Sections were counterstained with hematoxylin, dehydrated and coverslipped. Images were captured using a Nikon Eclipse Ni microscope (Nikon Instrument Inc., NY, United States).
Following antibodies were used in this study: rabbit anti-p-IRE1α antibody (Thermofisher Scientific, Cat #PA1-16927, 1:2,000); rabbit anti- XBP1s antibody (ProteinTech, Cat #24868-1-AP, 1:2,000); Rabbit anti-p-Perk antibody (Thermofisher Scientific, Cat #PA5-37773, 1:2,000); and rabbit anti-Bip antibody (Cell Signaling Technology, Cat #CST-31776, 1:2,000).
The immunocytochemistry staining was quantified with the use of ImageJ program, an open-source software for processing and analyzing images (Schneider et al., 2012). The percentage of staining area by DAB immunocytochemistry was derived with normalization to the total area with nuclear hematoxylin staining according to the instructions as specified by this program, and the mean was obtained from three or four random fields in the same cross-section of each sample. A total of ten placenta samples were from non-obese pregnancies (controls) and 12 samples were from obese pregnancies.
Statistical analysis
Maternal obesity was recorded as described (Yao et al., 2022). Briefly, maternal pre-pregnancy weight was obtained from medical records of the last recorded weight within 1 year prior to pregnancy. If this was not available, the earliest weight recorded within the first trimester was used. Maternal height was obtained from prenatal records. Pre-pregnancy BMI was calculated as the weight (kg) divided by the square of height m). Based on BMI information, the patients were categorized as non-obese (normal-weight, BMI: 18.5–24.9 kg/m2) or obese (BMI ≥30.0 kg/m2).
Images from the immunostaining and XBP1s/XBP1 agarose gel were quantified with ImageJ. The data from non-obese were compared with the obese group using non-parametric Mann-Whitney U-test. All statistical analyses were performed using Graphpad prism, Ver 5.0 (GraphPad Software, San Diego, CA).
Results
Maternal obesity activates p-IRE1a-XBP1s but not p-Perk signaling in the placenta
Excessive ER stress disrupts homeostasis in the placenta and contributes to the pathology of human pregnancy complications (Mizuuchi et al., 2016; Nakashima et al., 2019). Maternal obesity impacts both the placenta and the fetus (Howell and Powell, 2017). To Investigate the role of ER stress in maternal obesity-induced placental dysfunction, we assessed two ER stress markers—p-IRE1α and p-Perk on the placental FFPE sections by Immunocytochemistry. As shown in Figure 1, p-IRE1α was significantly increased in both syncytiotrophoblast and cytotrophoblasts in the obese placenta compared to non-obese controls. Upon activation, IRE1α is phosphorylated and cleaves its downstream target XBP1 to form spliced XBP1s mRNA. The translated XBP1s proteins translocated into the nucleus in which XBP1s activates transcription of ER chaperone genes (Yoshida et al., 2006). Indeed, placenta from the maternal obesity subjects showed increased XBP1s levels in both the cytosol and nucleus (Figures 2A–D). Electrophoresis of XBP1/XBP1s PCR products demonstrated increased XBP1s in the obese placenta (Figures 2C–E). Furthermore, RT-QPCR with XBP1s specific primer pair demonstrated 2.8-fold increase of XBP1s (Figure 2F). p-Perk protein was visualized with immunostaining in the cytosol of the syncytiotrophoblast. However, there is no difference of p-Perk intensity between the non-obese and the obese placenta (Figures 3A–C), suggesting Perk signaling may not impacted in the obese pregnancy.
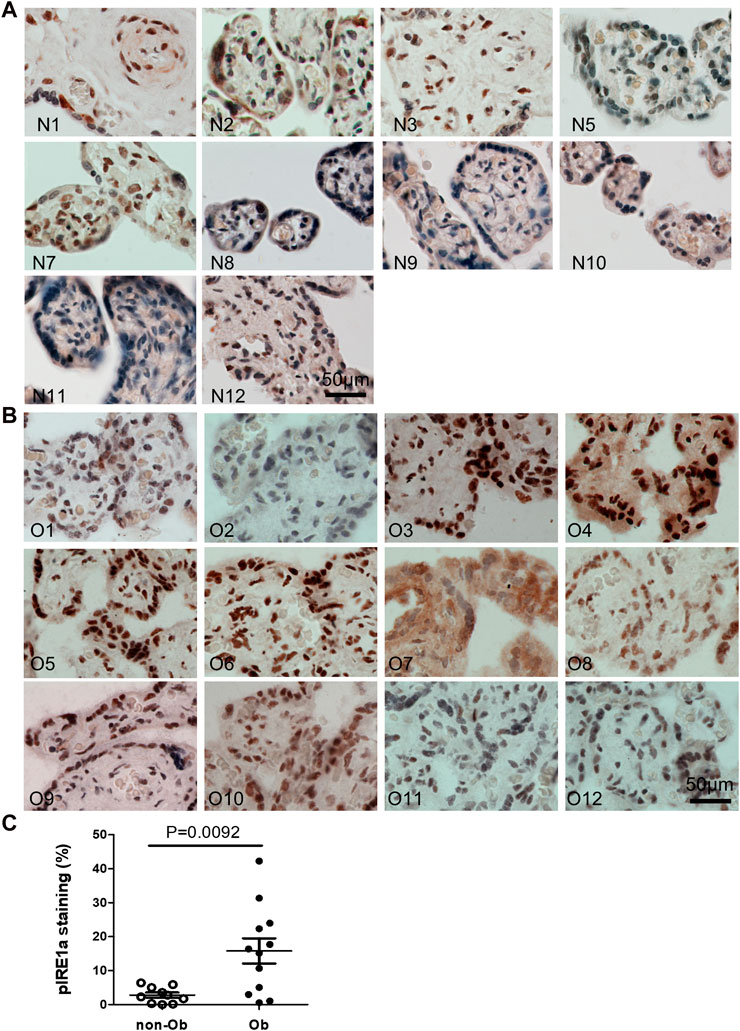
FIGURE 1. Maternal obesity induces IRE1α phosphorylation in the placenta. Placenta FFPE sections were labeled with anti-p-IRE1α specific antibody in the non-obese group (A), n = 10) and the obese group (B), n = 12). The labeling intensity was semi-quantified with ImageJ (C), showing the increased p-IRE1α in the obese placenta. Non-parametric Mann-Whitney U-test with p < 0.05 indicates a significant difference in p-IRE1α between the non-obese group to the obese group. Values are mean ± SEM. N1-N12: non-obese samples; O1-O12: obese samples.
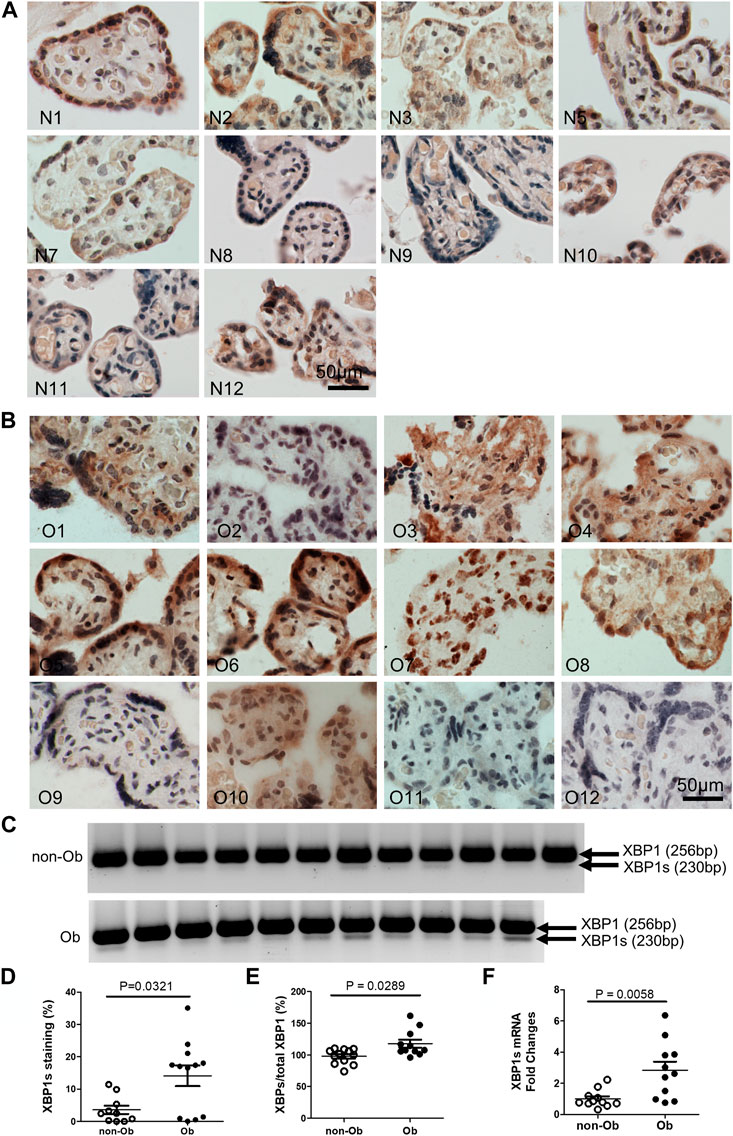
FIGURE 2. Obesity activates XBP1 splicing in the placenta. XBP1s specific antibody was used to label activated XBP1s. Compared to the non-obese placenta (A), n = 10), the obese placenta exhibited increased XBP1s (B), n = 12). Elevated XBP1s was visualized by PCR with a primer pair flanking splicing regions followed by electrophoresis (C) (unspliced, 256 bp; spliced XBP1, 230 bp) (Yoon et al., 2019). Quantified with ImageJ on the immunocytochemically labeled sections (D) or XBP1s PCR gel images (E) showed increased XBP1s in the obese placenta. Furthermore, Reverse Transcription (RT)-QPCR demonstrated increase of XBP1s in the obese placenta (F). Non-parametric Mann-Whitney U-test was used to compare the XBP1s between the non-obese and obese placenta. Values are mean ± SEM. N1-N12: non-obese samples; O1-O12: obese samples.
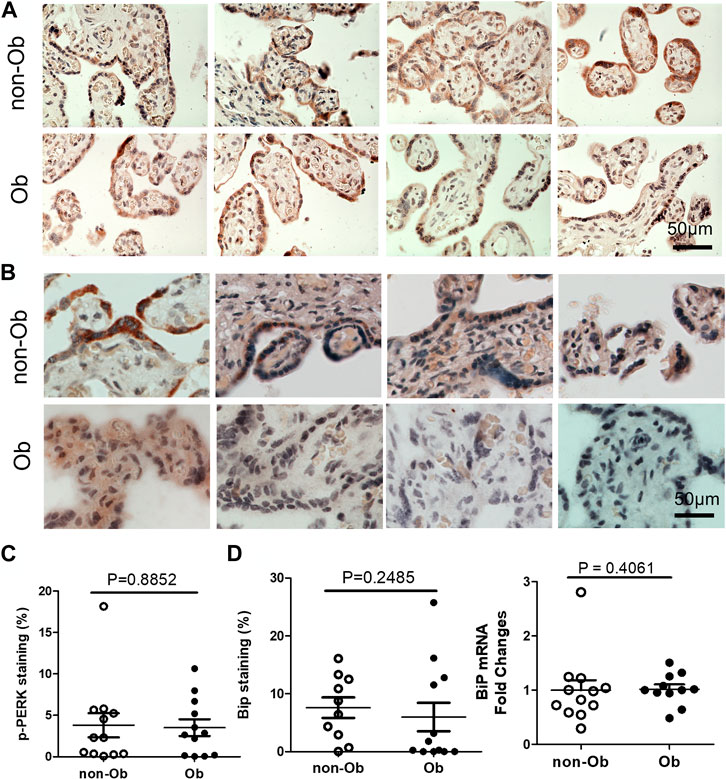
FIGURE 3. Obesity does not activate Perk signaling and BiP in the placenta. p-Perk was at similar levels in the non-obese (n = 12) and obese placenta (n = 12). Four representative p-Perk labeled images from either non-obese or obese were showed (A). One of the chaperone gene, BiP, were at similar intensity in the non-obese and obese placenta (non-obese, n = 10; obese, n = 12). (B), Four representative BiP labeled images were shown from each group. Quantification of p-Perk (C) or BiP (D) labeling was performed with ImageJ. Non-parametric Mann-Whitney U-test was used to compare the controls to others. Values are mean ± SEM.
Maternal obesity upregulates CHOP and ER chaperones in the placenta
UPR upregulates the expression of C/EBP Homologous Protein (CHOP) and chaperone genes. We found that CHOP was significantly elevated (Figure 4) (Szegezdi et al., 2006; Dong et al., 2018). CHOP has been known to involve ER-stress-induced pathology and cell apoptosis (Hu et al., 2018). The chaperone genes function as mediator for the post-transcriptional modifications and post-translation modifications of newly translated proteins (Ni and Lee, 2007). In this study, we evaluated CHOP and 10 chaperone genes by RT-QPCR and found that CHOP and nine chaperones were upregulated in the obese placenta than the non-obesity (Figure 4). These upregulated chaperone genes include Calnexin, GRP94/gp96, protein disulfide isomerase family A member 6 (PDIA6), calreticulin, glucose regulated protein-58 kDa (GRP58) or ERp57, suppressor of Ire1 and Lhs1 deletion one factor (SIL-1), P58IPK, homocysteine-induced endoplasmic reticulum protein, isoform A (Herp), ERAD-enhancing α-mannosidase-like proteins (EDEM), and ERp72 (Ni and Lee, 2007). Among the chaperone genes we analyzed, only Bip (GRP78) had similar expression levels in both non-obese and obese samples (Figures 3B–D).
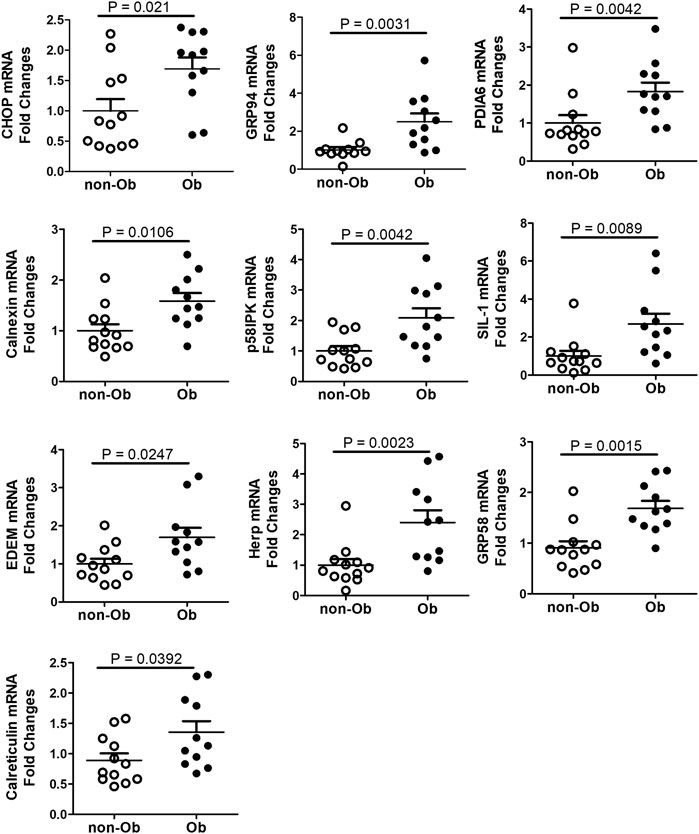
FIGURE 4. CHOP and UPR chaperones were upregulated in the placenta of obese pregnancy. CHOP was upregulated in obese placenta. Nine out of ten UPR chaperone genes we assessed with RT-QPCR were upregulated in the obese placenta. Non-parametric Mann-Whitney U-test was used to compare the chaperone gene expression in the non-obese placenta with the obese placenta, and p < 0.05 is considered significance. For non-obese group, n = 12; for obese group, n = 11. Values are mean ± SEM.
Discussion
We showed that trophoblast is sensitive to ER stress and UPR activation in the obese placenta, which was indeed limited to the IRE1α-XBP1 signaling pathway. Our results reveal that IRE1α was significantly increased at the protein levels compared to those non-obese controls. Furthermore, we found that maternal obesity induces a significant increase of the splice form of XBP1 at both mRNA and protein levels, and a set of UPR chaperon proteins including CHOP, calnexin, EDEM, calreticulin, GPR94, GPR58, p58IPK, Herp, PDIA6, and SIL-1 at mRNA levels. In contrast, no significant difference was observed in p-PERK signaling between the obese and non-obese control. Collectively, this study establishes that ER stress and UPR activation in the placenta is molecular characteristics of maternal obesity.
Our study supports that maternal obesity-induced specific activation of IRE1α-XBP1 plays a critical role in placental pathophysiology associated with obesity. All three UPR conserved signaling pathways share three domains: ER luminal domain, cytosolic domain, and pass membrane spanning domain, which are organized to enable proteins crossing ER membrane into the cytosol (Adams et al., 2019). The cytosolic part of IRE1α harbors kinase domains and can be autophosphorylated, which in turn stimulates the endoribonuclease activity and splices XBP1 to form a potent transcription activator, XBP1s (Cox and Walter, 1996; Calfon et al., 2002). XBP1s-induced upregulation of UPR target chaperone genes enhances a cell’s capacity to assist in protein maturation as well as promotes protein degradation and transportation, thereby releasing the burden of protein misfolding within the ER (Lee et al., 2003). PERK signaling is involved in regulation of the translation response of the UPR. PERK activation can phosphorylate eukaryotic translation initiation factor-2α (EIF2α), a key component of EIF2 complex, and causes ribosome inhibition and a transient attenuation of global protein translation (Harding et al., 2000), which subsequently alleviates the consequences because of protein misfolding to the cellular homeostasis. In this study, we were not able to identify the effects posed by maternal obesity on activity of PERK signaling in the placenta. While the exact mechanism responsible for such differential stimulatory effects remains elusive, obviously, it deserves further investigations.
Multiple studies have shown that ER stress is implicated in a variety of adverse pregnancy outcomes, suggesting that it may constitute a molecular mechanism underlying such associations. Indeed, UPR has been found to account for pathological changes in the placenta in patients complicated with preeclampsia (Yung et al., 2014; Yung et al., 2019; Thomson et al., 2021), miscarriage (Tang et al., 2021), stillbirth and preterm birth (Kawakami et al., 2014). Adverse pregnancy outcomes including preeclampsia are frequently associated with an enhanced apoptosis and stress. To this end, we speculate that UPR activation in the placenta may constitute an important mechanism accounting for maternal obesity-associated adverse pregnancy outcomes.
Our further efforts would include recapitulating pathophysiology of maternal obesity-induced effects on ER stress effects and UPR activation in vivo. There are multiple models of causing obesity in animals, which are classified as both genetic including monogenic, polygenic, and transgenic models and non-genetic including dietary, exotic, and large animals (Suleiman et al., 2020). Animal models have been widely used for revealing underlying causes for obesity, which are exemplified by environmental, hereditary, physiological, and epigenetic factors. These obesity animal models will provide an extremely useful model for dissecting molecular signaling pathways including ER stress and UPR responsible for maternal obesity-associated clinical manifestations of adverse obstetrical outcomes.
In conclusion, this is the first report for ER stress and UPR activation in the placenta of maternal obesity. Our findings represent the first step in understanding of one of key ER signaling pathway, also referred to IRE1α-XBP1, in placental pathophysiology affected by obesity, which may be an important mechanism accounting for the observed higher maternal and perinatal risks. Future studies are needed to confirm these findings in vivo animal studies and explore the value of p-IRE1α and XBP1s as important biomarkers to predict the adverse pregnancy outcomes (APOs) because of maternal obesity. Finally, we will assess any modifiable exposures that may reduce ER stress and UPR activation in the placenta, which can be largely facilitated by development of selective chemical compounds targeting genes constituting the IRE1α-XBP1 signaling pathway, thus improving clinical outcomes of maternal obesity and its associated APOs while providing individualized medicine.
Data availability statement
The original contributions presented in the study are included in the article/supplementary material, further inquiries can be directed to the corresponding author.
Ethics statement
The studies involving human participants were reviewed and approved by The Institutional Review Board of the University of Maryland, Baltimore, under the protocol IRB# HP-00066908. The patients/participants provided their written informed consent to participate in this study.
Author contributions
PY, WB-S conceived the study. WB-S and BW performed the experiment. RY and KG collected placental specimens and interpreted data. WB-S, BW, PY, and SW drafted and edited the manuscript. HG reviewed and edited the manuscript. All authors have reviewed and approved the manuscript.
Funding
This study was supported by NIH R01 grants to PY(R01HL139060, R01HL153141, and R01HL151108) and a R01 grant to SW(5R01HD095512).
Conflict of interest
The authors declare that the research was conducted in the absence of any commercial or financial relationships that could be construed as a potential conflict of interest.
Publisher’s note
All claims expressed in this article are solely those of the authors and do not necessarily represent those of their affiliated organizations, or those of the publisher, the editors and the reviewers. Any product that may be evaluated in this article, or claim that may be made by its manufacturer, is not guaranteed or endorsed by the publisher.
References
Adams, C. J., Kopp, M. C., Larburu, N., Nowak, P. R., and Ali, M. M. U. (2019). Structure and molecular mechanism of ER stress signaling by the unfolded protein response signal activator IRE1. Front. Mol. Biosci. 6, 11. doi:10.3389/fmolb.2019.00011
Blomberg, M. I., and Kallen, B. (2010). Maternal obesity and morbid obesity: The risk for birth defects in the offspring. Birth Defects Res. A Clin. Mol. Teratol. 88, 35–40. doi:10.1002/bdra.20620
Brombach, C., Tong, W., and Giussani, D. A. (2022). Maternal obesity: New placental paradigms unfolded. Trends Mol. Med. 28, 823–835. doi:10.1016/j.molmed.2022.05.013
Calfon, M., Zeng, H., Urano, F., Till, J. H., Hubbard, S. R., Harding, H. P., et al. (2002). IRE1 couples endoplasmic reticulum load to secretory capacity by processing the XBP-1 mRNA. Nature 415, 92–96. doi:10.1038/415092a
Cox, J. S., and Walter, P. (1996). A novel mechanism for regulating activity of a transcription factor that controls the unfolded protein response. Cell 87, 391–404. doi:10.1016/s0092-8674(00)81360-4
Dong, D., Zielke, H. R., Yeh, D., and Yang, P. (2018). Cellular stress and apoptosis contribute to the pathogenesis of autism spectrum disorder. Autism Res. 11, 1076–1090. doi:10.1002/aur.1966
Gaillard, R., Felix, J. F., Duijts, L., and Jaddoe, V. W. (2014a). Childhood consequences of maternal obesity and excessive weight gain during pregnancy. Acta Obstet. Gynecol. Scand. 93, 1085–1089. doi:10.1111/aogs.12506
Gaillard, R., Steegers, E. A., Duijts, L., Felix, J. F., Hofman, A., Franco, O. H., et al. (2014b). Childhood cardiometabolic outcomes of maternal obesity during pregnancy: The generation R study. Hypertension 63, 683–691. doi:10.1161/HYPERTENSIONAHA.113.02671
Harding, H. P., Zhang, Y., Bertolotti, A., Zeng, H., and Ron, D. (2000). Perk is essential for translational regulation and cell survival during the unfolded protein response. Mol. Cell 5, 897–904. doi:10.1016/s1097-2765(00)80330-5
Howell, K. R., and Powell, T. L. (2017). Effects of maternal obesity on placental function and fetal development. Reproduction 153, R97–R108. doi:10.1530/REP-16-0495
Hu, H., Tian, M., Ding, C., and Yu, S. (2018). The C/EBP homologous protein (CHOP) transcription factor functions in endoplasmic reticulum stress-induced apoptosis and microbial infection. Front. Immunol. 9, 3083. doi:10.3389/fimmu.2018.03083
Jansson, T., and Powell, T. L. (2013). Role of placental nutrient sensing in developmental programming. Clin. Obstet. Gynecol. 56, 591–601. doi:10.1097/GRF.0b013e3182993a2e
Kawakami, T., Yoshimi, M., Kadota, Y., Inoue, M., Sato, M., and Suzuki, S. (2014). Prolonged endoplasmic reticulum stress alters placental morphology and causes low birth weight. Toxicol. Appl. Pharmacol. 275, 134–144. doi:10.1016/j.taap.2013.12.008
Kelly, A. C., Powell, T. L., and Jansson, T. (2020). Placental function in maternal obesity. Clin. Sci. (Lond) 134, 961–984. doi:10.1042/CS20190266
Lee, A. H., Iwakoshi, N. N., and Glimcher, L. H. (2003). XBP-1 regulates a subset of endoplasmic reticulum resident chaperone genes in the unfolded protein response. Mol. Cell Biol. 23, 7448–7459. doi:10.1128/mcb.23.21.7448-7459.2003
Mizuuchi, M., Cindrova-Davies, T., Olovsson, M., Charnock-Jones, D. S., Burton, G. J., and Yung, H. W. (2016). Placental endoplasmic reticulum stress negatively regulates transcription of placental growth factor via ATF4 and ATF6β: Implications for the pathophysiology of human pregnancy complications. J. Pathol. 238, 550–561. doi:10.1002/path.4678
Nakashima, A., Cheng, S. B., Kusabiraki, T., Motomura, K., Aoki, A., Ushijima, A., et al. (2019). Endoplasmic reticulum stress disrupts lysosomal homeostasis and induces blockade of autophagic flux in human trophoblasts. Sci. Rep. 9, 11466. doi:10.1038/s41598-019-47607-5
Ni, M., and Lee, A. S. (2007). ER chaperones in mammalian development and human diseases. FEBS Lett. 581, 3641–3651. doi:10.1016/j.febslet.2007.04.045
Park, S. G., Lin, C., Gwon, L. W., Lee, J. G., Baek, I. J., Lee, B. J., et al. (2020). Protection of lycopene against embryonic anomalies and yolk sac placental vasculogenic disorders induced by nicotine exposure. Biomed. Res. Int. 2020, 7957045. doi:10.1155/2020/7957045
Reynolds, R. M., Allan, K. M., Raja, E. A., Bhattacharya, S., Mcneill, G., Hannaford, P. C., et al. (2013). Maternal obesity during pregnancy and premature mortality from cardiovascular event in adult offspring: Follow-up of 1 323 275 person years. BMJ 347, f4539. doi:10.1136/bmj.f4539
Rubens, M., Ramamoorthy, V., Saxena, A., Mcgranaghan, P., Veledar, E., and Hernandez, A. (2022). Obstetric outcomes during delivery hospitalizations among obese pregnant women in the United States. Sci. Rep. 12, 6862. doi:10.1038/s41598-022-10786-9
Schneider, C. A., Rasband, W. S., and Eliceiri, K. W. (2012). NIH image to ImageJ: 25 years of image analysis. Nat. Methods 9, 671–675. doi:10.1038/nmeth.2089
Schroder, M., and Kaufman, R. J. (2005). ER stress and the unfolded protein response. Mutat. Res. 569, 29–63. doi:10.1016/j.mrfmmm.2004.06.056
Shen, W. B., Turan, S., Wang, B., Cojocaru, L., Harman, C., Logue, J., et al. (2022). A SARS-CoV-2 delta variant case manifesting as extensive placental infection and fetal transmission. Gynecol. Obstet. Invest 87, 165–172. doi:10.1159/000524905
Suleiman, J. B., Mohamed, M., and Bakar, A. B. A. (2020). A systematic review on different models of inducing obesity in animals: Advantages and limitations. J. Adv. Vet. Anim. Res. 7, 103–114. doi:10.5455/javar.2020.g399
Szegezdi, E., Logue, S. E., Gorman, A. M., and Samali, A. (2006). Mediators of endoplasmic reticulum stress-induced apoptosis. EMBO Rep. 7, 880–885. doi:10.1038/sj.embor.7400779
Tang, Y., Zhang, X., Zhang, Y., Feng, H., Gao, J., Liu, H., et al. (2021). Senescent changes and endoplasmic reticulum stress may Be involved in the pathogenesis of missed miscarriage. Front. Cell Dev. Biol. 9, 656549. doi:10.3389/fcell.2021.656549
Thomson, S., Waters, K. A., Hennessy, A., and Machaalani, R. (2021). The unfolded protein response and apoptotic regulation in the human placenta due to maternal cigarette smoking and pre-eclampsia. Reprod. Toxicol. 105, 120–127. doi:10.1016/j.reprotox.2021.09.001
Whitaker, R. C., Wright, J. A., Pepe, M. S., Seidel, K. D., and Dietz, W. H. (1997). Predicting obesity in young adulthood from childhood and parental obesity. N. Engl. J. Med. 337, 869–873. doi:10.1056/NEJM199709253371301
Xu, C., Shen, W. B., Reece, E. A., Hasuwa, H., Harman, C., Kaushal, S., et al. (2021). Maternal diabetes induces senescence and neural tube defects sensitive to the senomorphic rapamycin. Sci. Adv. 7. doi:10.1126/sciadv.abf5089
Yang, P., Shen, W. B., Reece, E. A., Chen, X., and Yang, P. (2016). High glucose suppresses embryonic stem cell differentiation into neural lineage cells. Biochem. Biophys. Res. Commun. 472, 306–312. doi:10.1016/j.bbrc.2016.02.117
Yao, R., Yang, P., Goetzinger, K. R., Atkins, K. L., Shen, W. B., Wang, B., et al. (2022). Maternal obesity-associated disruption of polarized lactate transporter MCT4 expression in human placenta. Reprod. Toxicol. 112, 1–6. doi:10.1016/j.reprotox.2022.06.009
Yoon, S. B., Park, Y. H., Choi, S. A., Yang, H. J., Jeong, P. S., Cha, J. J., et al. (2019). Real-time PCR quantification of spliced X-box binding protein 1 (XBP1) using a universal primer method. PLoS One 14, e0219978. doi:10.1371/journal.pone.0219978
Yoshida, H., Oku, M., Suzuki, M., and Mori, K. (2006). pXBP1(U) encoded in XBP1 pre-mRNA negatively regulates unfolded protein response activator pXBP1(S) in mammalian ER stress response. J. Cell Biol. 172, 565–575. doi:10.1083/jcb.200508145
Yung, H. W., Atkinson, D., Campion-Smith, T., Olovsson, M., Charnock-Jones, D. S., and Burton, G. J. (2014). Differential activation of placental unfolded protein response pathways implies heterogeneity in causation of early- and late-onset pre-eclampsia. J. Pathol. 234, 262–276. doi:10.1002/path.4394
Yung, H. W., Colleoni, F., Dommett, E., Cindrova-Davies, T., Kingdom, J., Murray, A. J., et al. (2019). Noncanonical mitochondrial unfolded protein response impairs placental oxidative phosphorylation in early-onset preeclampsia. Proc. Natl. Acad. Sci. U. S. A. 116, 18109–18118. doi:10.1073/pnas.1907548116
Keywords: placenta, maternal obese, endoplasmic reticulum stress, IRE1α, XBP1S, chaperones
Citation: Shen W-B, Wang B, Yao R, Goetzinger KR, Wu S, Gao H and Yang P (2023) Obesity impacts placental function through activation of p-IRE1a-XBP1s signaling. Front. Cell Dev. Biol. 11:1023327. doi: 10.3389/fcell.2023.1023327
Received: 19 August 2022; Accepted: 16 January 2023;
Published: 01 February 2023.
Edited by:
Athina Samara, Karolinska Institutet (KI), SwedenReviewed by:
Ugo Soffientini, Institute of Hepatology London, Foundation for Liver Research, United KingdomYi Tan, University of Louisville, United States
Copyright © 2023 Shen, Wang, Yao, Goetzinger, Wu, Gao and Yang. This is an open-access article distributed under the terms of the Creative Commons Attribution License (CC BY). The use, distribution or reproduction in other forums is permitted, provided the original author(s) and the copyright owner(s) are credited and that the original publication in this journal is cited, in accordance with accepted academic practice. No use, distribution or reproduction is permitted which does not comply with these terms.
*Correspondence: Peixin Yang, cHlhbmdAc29tLnVtYXJ5bGFuZC5lZHU=