- Molecular Cell Biology, Groningen Biomolecular Sciences and Biotechnology Institute, University of Groningen, Groningen, Netherlands
In the yeast Hansenula polymorpha, the ER protein Pex32 is required for associating peroxisomes to the ER. Here, we report on a structure–function analysis of Pex32. Localization studies of various Pex32 truncations showed that the N-terminal transmembrane domain of Pex32 is responsible for sorting. Moreover, this part of the protein is sufficient for the function of Pex32 in peroxisome biogenesis. The C-terminal DysF domain is required for concentrating Pex32 at ER-peroxisome contact sites and has the ability to bind to peroxisomes. In order to better understand the role of Pex32 in peroxisome biogenesis, we analyzed various peroxisomal proteins in pex32 cells. This revealed that Pex11 levels are strongly reduced in pex32 cells. This may explain the strong reduction in peroxisome numbers in pex32 cells, which also occurs in cells lacking Pex11.
Introduction
Proteins of the Pex23 family exclusively occur in yeast and filamentous fungi (Jansen et al., 2021). Members of this family contain an N-terminal domain with several predicted transmembrane (TM) helices and a DysF motif at the extreme C-terminus. So far, little is known about the function of both domains. For Saccharomyces cerevisiae Pex30 and Pex31, two members of the Pex23 family, a reticulon-like region in the membrane-bound domain was shown to display membrane tubulation activity (Joshi et al., 2016).
All yeast species contain several members of the Pex23 family. S. cerevisiae has five (Pex28, Pex29, Pex30, Pex31, and Pex32), while Hansenula polymorpha has four (Pex23, Pex24, Pex29, and Pex32) (Jansen et al., 2021). The absence of a member of the Pex23 family generally results in abnormal peroxisome numbers and/or size, explaining why these proteins were designated Pex. Some members also play a role in the formation of lipid bodies (Joshi et al., 2018; Wang et al., 2018) or accumulate at nuclear vacuole junctions (NVJs) (Wu et al., 2020; Ferreira and Carvalho, 2021). The function of Pex23 proteins at NVJs is still unknown.
Although Pex23 family proteins were initially reported to be peroxisomal membrane proteins, recent studies showed that they localize to ER (Mast et al., 2016; Wu et al., 2020). Often, Pex23 proteins accumulate at specialized ER regions, where contact sites are formed with other organelles, such as peroxisomes or the nucleus. These specialized ER regions are also implicated in the formation of pre-peroxisomal vesicles and lipid bodies (Joshi et al., 2018).
We recently showed that H. polymorpha Pex23, Pex24, and Pex32, but not Pex29, play important roles in the formation of ER-peroxisome contact sites (Wu et al., 2020). In the absence of HpPex23, HpPex24, or HpPex32, fewer peroxisome-ER contact sites occur, paralleled by a reduction in the average peroxisomal membrane surface and decreased peroxisome numbers (Wu et al., 2020; Yuan et al., 2022). The reduction in peroxisomal membrane surface suggests that contact sites may play a role in the transfer of lipids from ER to peroxisomes to allow organellar expansion. Recent data indicated that the bulk lipid transporter protein Vps13 may contribute to lipid transfer at these contacts (Yuan et al., 2022).
Why peroxisome numbers are decreased in cells lacking Pex23 family proteins is still unknown. Intriguingly, H. polymorpha pex23 and pex24 cells have very similar peroxisome abnormalities as H. polymorpha pex11 cells, namely reduced peroxisome abundance together with an increase in organellar size (Krikken et al., 2009; Yuan et al., 2022). Like in pex23, pex24, and pex32 cells, also in pex11 cells, peroxisome-ER contact sites are disrupted (Wu et al., 2020), suggesting that Pex23 family proteins at ER together with Pex11 at the peroxisomal membrane are involved in peroxisome-ER contact site formation. Pex11 is an abundant peroxisomal membrane protein (PMP), well known for its role in peroxisome multiplication (Erdmann and Blobel, 1995; Krikken et al., 2009; Carmichael and Schrader, 2022). Intriguingly, S. cerevisiae Pex11 was shown to be important for the formation of peroxisome-mitochondria contacts, suggesting that it may be a general contact site resident protein (Mattiazzi Ušaj et al., 2015; Wu et al., 2020).
H. polymorpha Pex32 is a crucial Pex23 family protein for peroxisome biogenesis because of absence of Pex32 results in most severe peroxisomal defects (Wu et al., 2020). Here, we investigated the function of different domains of HpPex32. We show that the second transmembrane (TM) helix harbors ER targeting information. The DysF domain has the capacity to associate with peroxisomes but is not essential for the Pex32 function. Unexpectedly, Pex11 levels are very low in pex32 cells. This may explain why peroxisome numbers are low in pex32 cells, like in pex11 cells.
Results
The overproduced Pex32 N-terminal domain localizes to the ER, while the C-terminal DysF domain can associate to peroxisomes
Sequence analysis of the H. polymorpha Pex32 protein revealed four predicted TM helices in the N-terminus and a DysF motif at the extreme C-terminus (Wu et al., 2020) (Figure 1A). To analyze which part of the protein is important for sorting to ER, we constructed several truncated variants containing GFP at the C-terminus. Considering the very low endogenous Pex32 levels (Wu et al., 2020), all truncations were produced under the control of the relatively strong ADH1 promoter (PADH1). We previously showed that overproduced full-length Pex32-GFP localizes to ER similar to the endogenously produced protein (Wu et al., 2020). The constructs were introduced in a pex32 strain, also producing BiP-mCherry-HDEL as the ER marker, and analyzed by confocal laser scanning microscopy (CLSM, Airy Scan). The western blot analysis using anti-GFP antibodies confirmed that all Pex32 variants were present at the expected molecular weight (Supplementary Figure S1).
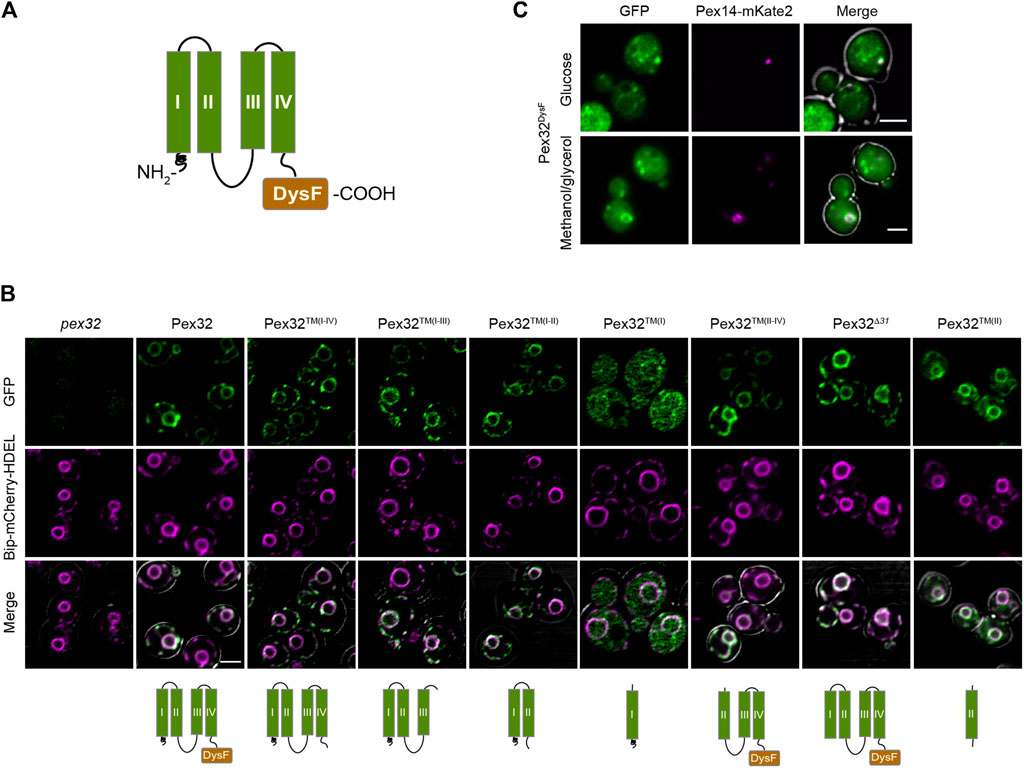
FIGURE 1. Pex32 TM helices contain ER sorting information, while the DysF domain has the capacity to associate with peroxisomes. (A) Predicted Pex32 structure. Transmembrane helixes (TMs) are numbered I, II, III and IV. (B) Confocal Laser Scanning Microscopy (CLSM) Airy-scan analysis of glucose-grown pex32 cells producing the ER marker Bip-mCherry-HDEL and the indicated Pex32 truncations fused to GFP and produced under control of the PADH1. Images were processed differently, in order to visualize the GFP signal optimally. Scale bars: 2 μm. (C) CLSM Airy-scan images of glucose-grown cells (top) and fluorescence microscopy (FM) images of methanol/glycerol-grown pex32 cells producing Pex14-mKate2 and PADH1Pex32DysF-mGFP (bottom). Scale bars: 2 μm.
In line with our earlier report overproduced full-length Pex32-GFP localizes to the peripheral ER and nuclear envelope [Figure 1B; (Wu et al., 2020)]. Removal of the extreme N-terminal 31 residues, which precede the first predicted TM helix (Pex32Δ31) or removal of the C-terminal DysF domain (Pex32™(I−IV)) did not affect sorting to ER, indicating that ER sorting information is present in the region containing four predicted TM helices, as expected.
To study which region in the membrane-bound domain of Pex32 is required for sorting to ER, the location of several truncated variants was determined by fluorescence microscopy. Of these constructs, a truncation consisting of only the first TM helix [PADH1Pex32™(I)] was mainly cytosolic, with only very little fluorescence detectable at the nuclear envelope. All other constructs are fully co-localized with the ER marker. These include a construct consisting of only the second TM helix [Pex32™(II)], the first second TM helices [Pex32™(I−II)] or the first third TM helices [Pex32™(I−III)]. Similarly, a construct lacking the first TM helix [Pex32™(II−IV)] is localized to the ER. The protein level of a construct consisting of the third and fourth TM helix [without the DysF domain; Pex32™(III−IV)] was below the limit of detection by fluorescence microscopy and therefore could not be localized.
To summarize, our study revealed that all constructs containing TM(II) localized to ER, indicating that TM(II) contains ER sorting information. Whether ER sorting information is also present in TM(III) or TM(IV) could not be established.
Overexpression of the soluble DysF domain containing a C-terminal GFP in pex32 cells (Pex32DysF-GFP) resulted in some spots of higher intensity in addition to cytosolic fluorescence (Figure 1C). Co-localization experiments showed that these spots frequently overlapped with the Pex14-mKate2 peroxisomal membrane marker (Figure 1C), indicating that the soluble DysF domain is capable to associate with peroxisomes. Note that not all pex32 cells contain peroxisomes, therefore many cells lack a Pex14-mKate2 spot (Wu et al., 2020).
The DysF domain is not essential for Pex32 function in peroxisome biogenesis
To analyze the role of the different domains of Pex32 in peroxisome biology, we quantified peroxisome numbers in pex32 cells producing Pex32 truncations containing GFP at the C-terminus. To rule out overproduction artifacts, all truncations were produced under the control of the endogenous promoter (PPEX32). A WT strain producing full-length Pex32 containing GFP at the C-terminus (PPEX32Pex32FL-GFP) was used as a positive control, while pex32 was included as a negative control. Peroxisomes were marked with PMP47-mKate2 and images were obtained by wide-field microscopy. Cells were grown on a mixture of glycerol and methanol to induce peroxisome proliferation. In line with our previous observations, the absence of Pex32 resulted in a strong decrease in peroxisome numbers (Figures 2A,B). Peroxisome numbers are restored to WT levels upon introduction of a construct that contains the N-terminal domain [pex32:Pex32™(I−IV)], also including first 31 N-terminal residues (Figures 2A,B). Similarly, the growth defect of pex32 cells on glycerol/methanol was fully rescued upon introduction of the entire Pex32 N-terminal domain [pex32:Pex32™(I−IV); Figure 2C]. None of other smaller constructs fully complemented pex32 in terms of peroxisome numbers or growth on glycerol/methanol (Figures 2B,C). These findings indicate that the complete N-terminus (extreme N-terminal 31 residues together with the four predicted TM helices) is required and sufficient for Pex32 function. Consequently, the DysF domain is not required for the function of H. polymorpha Pex32 in peroxisome biology.
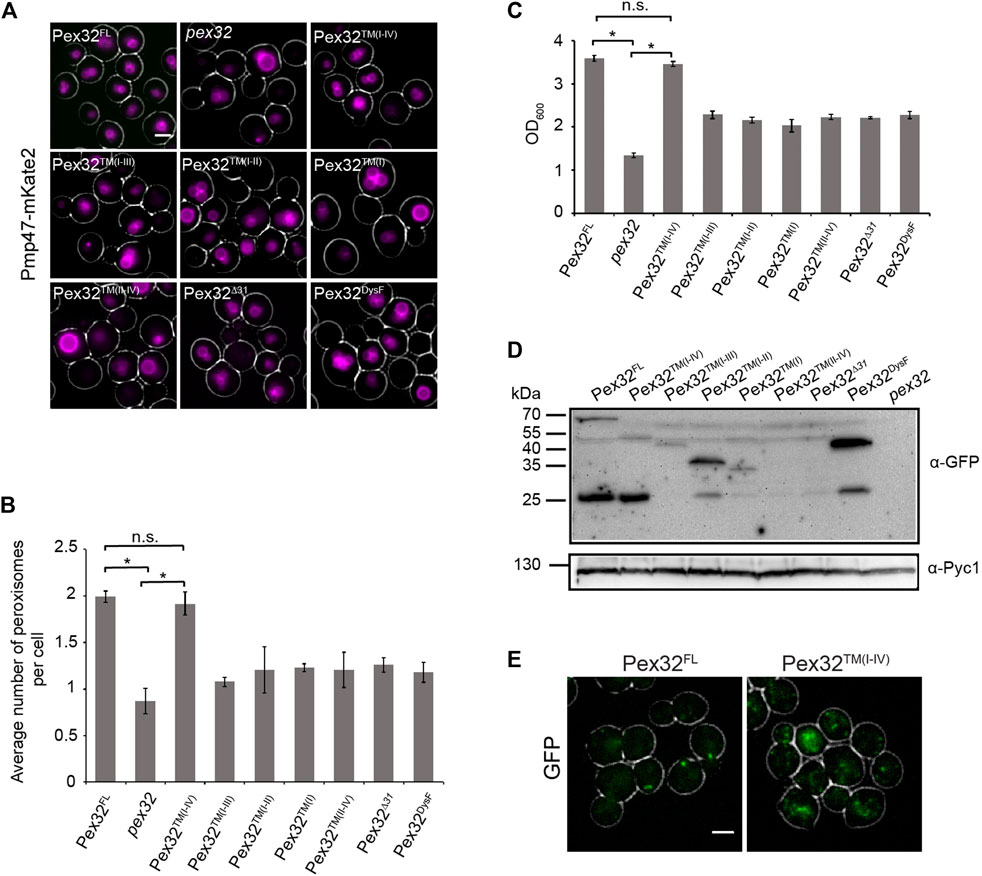
FIGURE 2. N-terminal domain of Pex32 is important for its function, while the DysF domain is required to accumulate Pex32 at peroxisome-ER contact sites. (A) FM analysis of methanol/glycerol-grown pex32 cells producing Pmp47-mKate2 and indicated Pex32 truncations containing GFP at the C-terminus and produced under the control of PPEX32. Scale bar: 2 μm. (B) Quantification of the average number of peroxisomes per cell in the indicated strains. Error bars represent s.d. from three independent experiments (n = 3 using 200 cells from each experiment). Significance indications: n. s. = p > 0.05, * = p < 0.01. (C) Optical densities of the indicated strains upon growth for 16 h on methanol/glycerol medium. Significance indications: n. s. = p > 0.05, * = p < 0.01. Error bars represent s.d. from three independent experiments. (D) Western blot analysis of the indicated strains. Cells were grown in a methanol/glycerol medium for 16 h. Blots were decorated with anti-GFP or anti-Pyc1 antibodies. Pyc1 was used as a loading control. (E) FM analysis of glucose-grown WT cells producing Pex32-GFP (left) or pex32 cells producing Pex32TM(I-IV) (right). Scale bar: 2 μm.
The western blot analysis using antibodies against GFP revealed that all Pex32 constructs were present at the expected size, except for Pex32™(II−IV) and Pex32∆31, which were below the limit of detection (Figure 2D). Therefore, the inability of the latter two constructs to complement pex32 cells maybe due to insufficient protein levels. For full-length Pex32 as well as for some of the truncations also a band of approx. 27 kDa was observed. This band most likely represents free GFP.
In glucose-grown WT cells Pex32-GFP, produced under the control of the endogenous promoter, accumulates in a single spot per cell, which represents the peroxisome-ER contact site (Wu et al., 2020) (Figure 2E, Pex32FL). However, upon removal of the DysF domain, GFP fluorescence was no longer concentrated in a single spot [pex32:Pex32™(I−IV)] (Figure 2E). This suggests that the DysF domain of Pex32 contributes to concentrating Pex32 at peroxisome-ER contacts.
In contrast to the DysF domain of Pex32, the DysF domain of H. polymorpha Pex23 is essential for its function (Supplementary Figure S2). Cells lacking Pex23 (pex23) or only producing the N-terminal membrane-bound domain of Pex23 without the DysF domain showed a similar phenotype as pex23 cells (Supplementary Figure S2). Replacing the DysF domain of Pex23 with the same domain of Pex32 did not restore peroxisome formation (Supplementary Figure S2), indicating that there is no functional redundancy among the DysF domains of Pex23 and Pex32.
The reduction of peroxisome abundance in pex32 cells is not caused by enhanced autophagy
Deletion of PEX32 results in a strong reduction of peroxisome numbers (Wu et al., 2020). To block autophagy, we deleted ATG1 in pex32. The quantitative analysis of FM images of cells producing the peroxisomal matrix marker DsRed-SKL revealed that peroxisome numbers were similar in pex32 and pex32 atg1 cells (Figures 3A,B). This indicates that the reduced peroxisome numbers in pex32 are not due to autophagy.
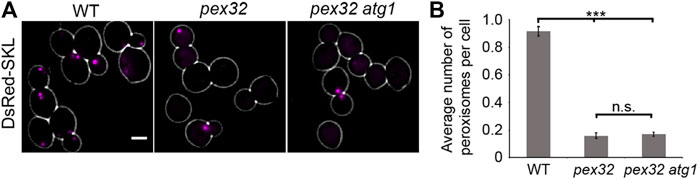
FIGURE 3. Reduced peroxisome abundance in pex32 cells is not caused by autophagy. (A) FM images of DsRed-SKL produced in WT, pex32, and pex32 atg1 cells grown on glucose for 4 h. Scale bar: 2 μm. (B) Quantification of the average number of peroxisome per cell in indicated strains. Error bars represent s.d. from three independent experiments (n = 3 using 200 cells from each experiment). Significance indications: n. s. = p > 0.05, *** = p < 0.001.
Deletion of PEX32 results in reduced Pex11 levels
To understand why peroxisome numbers are reduced in pex32 cells, we analyzed the levels of several peroxisomal proteins by western blotting. As shown in Figures 4A,B the levels of the peroxisomal matrix protein alcohol oxidase (AOX) were similar in pex32 and WT cells. Also, PMPs Pex3 and Pex14 were similar in both strains. However, Pex11 levels were strongly reduced in pex32 cells. As reported previously, Pex11 levels are also reduced in H. polymorpha pex3 cells, which were included as a negative control for the Pex3 blot (Knoops et al., 2014).
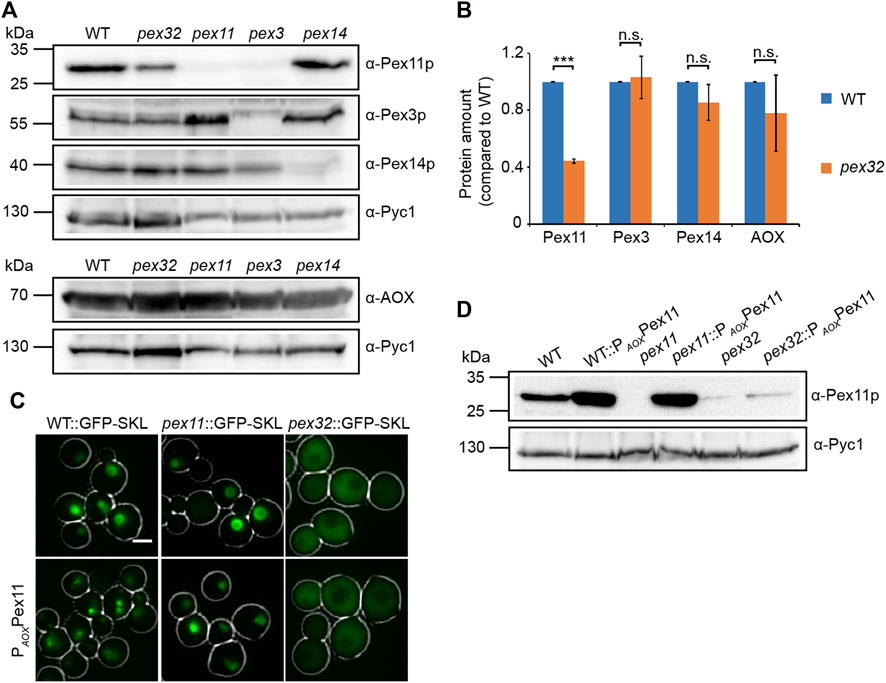
FIGURE 4. Pex11 levels are low in pex32 cells. (A) Western blot analysis and (B) quantification of indicated proteins in WT, pex32, and indicated negative control cells grown for 16 h on methanol/glycerol. Blots were decorated with anti-Pex11p, anti-Pex3p, anti-Pex14p, anti-AOX, or anti-Pyc1 antibodies. Pyc1 was used as a loading control. In B, the protein levels of WT cells were set to 1. Significance indications: n. s. = p > 0.05, ***p = < 0.001. The error bars represent s.d. from three independent experiments. (C) FM images of WT, pex11, and pex32 cells producing GFP-SKL grown on methanol/glycerol for 6 h. Scal e bar: 2 μm. (D) Western blot analysis of the indicated strains grown on methanol/glycerol for 6 h. Blots were decorated with anti-Pex11p or anti-Pyc1 antibodies. Pyc1 was used as a loading control.
To investigate whether the peroxisome phenotype was restored by artificially increasing Pex11 levels, PEX11 was overexpressed using the strong AOX promoter (PAOX) in pex32 cells producing the peroxisomal matrix protein GFP-SKL. As expected, PEX11 overexpression resulted in enhanced peroxisome proliferation in WT and pex11 controls (Figure 4C). The western blot analysis confirmed the increase in Pex11 protein levels in these two strains. In contrast, pex32:PAOXPEX11 cells still showed mislocalization of GFP-SKL to the cytosol, like in the pex32 control (Figure 4C). Also, Pex11 levels were not increased in pex32 cells upon overexpression of PEX11 (Figure 4D).
Discussion
Here, we show that the second predicted TM in the N-terminal domain of H. polymorpha Pex32 is important for sorting the protein to ER, while the C-terminal DysF domain is capable to associate with peroxisomes. DysF is important to concentrate Pex32 at peroxisome-ER contact sites. Unexpectedly, the DysF domain is redundant for the function of Pex32 in peroxisome biogenesis. Finally, we show that Pex11 levels are strongly reduced in pex32, which explains low peroxisome abundance, as observed in pex11 cells.
Localization studies of various truncated Pex32 variants revealed that removal of the first N-terminal 31 residues or the DysF domain had no effect on ER sorting, indicating that the region with the four predicted TM helices contains ER sorting information. The western blot analysis revealed that deletion of the first 31 N-terminal residues caused a strong decrease in protein levels (Supplementary Figure S1; Figure 2D). Possibly, the absence of this part of the protein makes Pex32 more susceptible to proteolytic degradation.
A construct consisting of only TM(I) was mainly cytosolic, while the levels of the construct consisting of TM(III-IV) were too low to allow its localization. All constructs containing TM(II) localized to ER, indicating that this domain contains ER sorting information.
We show that the DysF domain is redundant for the role of H. polymorpha Pex32 in peroxisome biology because peroxisome defects are restored upon introduction of a Pex32 construct lacking the DysF domain in pex32 cells. This observation differs from earlier findings in P. pastoris and S. cerevisiae. In P. pastoris, DysF domains of Pex30 and Pex31 are essential for the regulation of peroxisome number and size (Yan et al., 2008). Similarly, the DysF domain of S. cerevisiae Pex30 was shown to be essential for normal peroxisome biology (Ferreira and Carvalho, 2021). Moreover, removal of the ScPex30 DysF domain results in defects in the NVJ organization and lipid body clustering (Ferreira and Carvalho, 2021).
Our data show that accumulation of HpPex32-GFP at peroxisome-ER contact sites requires the DysF domain because GFP fluorescence no longer is present in a single spot per cell when the DysF motif was removed. Moreover, we showed that the Pex32 DysF domain has the ability to associate with peroxisomes. Most likely the Pex32 DysF domain has a binding partner at the peroxisomal membrane, which keeps the protein concentrated at peroxisome-ER contact sites. This accumulation apparently is not essential, because a construct lacking the DysF domain, which does not accumulate at contact sites, still can functionally complement a pex32 deletion strain. Possibly in these cells, sufficient Pex32 is present at the contact sites for their function.
We show that cells lacking Pex32 have strongly reduced Pex11 levels, while the levels of other peroxisomal proteins tested were normal. Moreover, upon placing PEX11 under the control of a strong promoter in pex32 cells, Pex11 protein levels were not enhanced, suggesting that Pex32 is required to maintain normal Pex11 levels. This observation may explain why in pex32 cells peroxisome numbers are strongly reduced as observed for pex11 cells. Why Pex11 levels are low in pex32 is not yet understood and requires further analysis.
Materials and methods
Strains and growth conditions
H. polymorpha cells were grown in batch cultures at 37°C on mineral media (Van Dijken et al., 1976) supplemented with 0.5% glucose or 0.5% methanol, or a mixture of 0.5% methanol and 0.05% glycerol as carbon source. When required leucine was added to a final concentration of 60 μg/ml. For growth on plates, YPD media (1% yeast extract, 1% peptone, and 1% glucose) or YND media (0.67% yeast nitrogen base without amino acids (YNB; Difco; BD) and 0.5% glucose) were supplemented with 2% agar. Resistant transformants were selected using 100 μg/ml zeocin (Invitrogen), 100 μg/ml nourseothricin (WERNER BioAgents), or 300 μg/ml hygromycin (Invitrogen).
Escherichia coli strain DH5α was used for cloning. E. coli cells were grown at 37°C in Luria broth (LB) media (1% Bactotryptone, 0.5% Yeast Extract, and 0.5% NaCl) supplemented with 100 μg/ml ampicillin or 50 μg/ml kanamycin. 2% agar was added in LB medium for growth on plates.
Construction of H. polymorpha strains
All strains, plasmids, and primers used in this study are listed in Supplementary Tables S1, S2, and S3, respectively. Plasmid integration was performed as described previously (Faber et al., 1994). All integrations were confirmed by PCR. Gene deletions were confirmed by PCR and Southern blotting.
Construction of strains expressing GFP-tagged Pex32 truncations
Plasmids encoding PADH1Pex32™(I−IV)-mGFP and PADH1Pex32DysF-mGFP were constructed as follows: a PCR fragment encoding the N-terminus (all 4 TMs) of PEX32 was obtained using primers Fw Pex321-696 and Rv Pex321-696 with H. polymorpha yku80 genomic DNA as a template. Similarly, a PCR fragment encoding the DysF domain of PEX32 was obtained with primers Fw Pex32697-1062 and Rv Pex32697-1062. The obtained PCR fragments were digested with HindIII and BglII and separately inserted between the HindIII and BglII sites of plasmid pHIPZ18-INP1-GFP, resulting in pHIPZ18-PEX32™(I−IV)-mGFP and pHIPZ18-PEX32DysF-mGFP. Both plasmids and pAMK106 were digested by HindIII and SalI separately, and ligated, resulting in pHIPN18-PEX32™(I−IV)-mGFP and pHIPN18-PEX32DysF-mGFP.
By using pHIPN18-PEX32™(I−IV)-mGFP as the template, primer pairs: 1) Fw Pex321-696/Rev Pex321-501, 2) Fw Pex321-696/Rev Pex321-312, and 3) Fw Pex321-696/Rev Pex321-177 were used to amplify constructs containing: 1) PEX32™(I−III), 2) PEX32™(I−II), and 3) PEX32™(I), respectively. PCR products were digested with HindIII and BglII, and inserted between the HindIII and BglII sites of pHIPN18-PEX32™(I−IV)-mGFP separately to obtain pHIPN18-PEX32™(I−III)-mGFP, pHIPN18-PEX32™(I−II)-mGFP, and pHIPN18-PEX32™(I)-mGFP.
Plasmids pHIPN18-PEX32™(II−IV)-mGFP and pHIPN18-PEX32∆31-mGFP were constructed by using the same method. H. polymorpha Pex32-mGFP genomic DNA was used as the template, using primer pairs Fw Pex32(169–1062)/Rv DysFPEX32-mGFP and Fw Pex32(94–1062)/Rv DysFPEX32-mGFP to amplify fragments containing PEX32™(II−IV)-mGFP and PEX32∆31-mGFP, respectively. Both PCR products and pHIPN18-PEX32™(I−IV)-GFP were restricted by HindIII and XhoI separately, resulting in pHIPN18-PEX32™(II−IV)-mGFP and pHIPN18-PEX32∆31-mGFP.
The plasmid for PEX32 overexpression was constructed as follows: a PCR fragment containing full-length PEX32 was obtained using primers Fw Pex321-696 and Rv DysFPEX32-mGFP with Pex32-mGFP strain as a template. The PCR product and pHIPN18-Pex32™(I−IV)-mGFP were digested by HindIII and XhoI and ligated resulting in pHIPN18-PEX32-mGFP.
All aforementioned plasmids were linearized with AatII and integrated into pex32 strains separately to produce PADH1Pex32-mGFP, PADH1Pex32™(I−IV)-mGFP, PADH1Pex32™(I−III)-mGFP, PADH1Pex32™(I−II)-mGFP, PADH1Pex32™(I)-mGFP, PADH1Pex32™(II−IV)-mGFP, PADH1Pex32∆31-mGFP, or PADH1Pex32DysF-mGFP. DraI-linearized pHIPX7-BiPN30-mCherry-HDEL was integrated into various truncations independently to express BiP-mCherry-HDEL.
To obtain pHIPN18-PEX32™(II)-mGFP and pHIPN18-PEX32™(III−IV)-mGFP, plasmid pHIPN18-PEX32™(I−IV)-mGFP was used as a template, and primer pairs Fw Pex32o2TM/Rev Pex321-312, Fw Pex32TM3+4/Rv Pex321-696 were used to amplify fragments containing PEX32™(II) and PEX32™(II−IV), respectively. These PCR products and pHIPN18-PEX32™(I−IV)-mGFP were digested with HindIII and BglII and ligated to obtain pHIPN18-PEX32™(II)-mGFP and pHIPN18-PEX32™(III−IV)-mGFP. AatII-linearized plasmids were integrated into pex32:BiP-mCherry-HDEL separately to produce PADH1Pex32™(II)-mGFP and PADH1Pex32™(III−IV)-mGFP.
Plasmids for producing various Pex32 truncations under the control of the PEX32 promoter were constructed as follows: PCR was performed on yku80 genomic DNA to amplify the PEX32 promoter using primers PPEX32 fw and PPEX32 rev. The obtained PCR fragment was digested with NotI and HindIII, and then replaced the ADH1 promoter (PADH1) in NotI/HindIII digested variants of Pex32 truncations. All constructions under the control of PPEX32 were linearized with EcoRV and integrated into pex32:Pmp47-mKate2 cells separately to produce Pex32™(I−IV)-mGFP, Pex32™(I−III)-mGFP, Pex32™(I−II)-mGFP, Pex32™(I)-mGFP, Pex32™(II−IV)-mGFP, Pex32∆31-mGFP, and Pex32DysF-mGFP.
Construction of the pex32 atg1 double deletion strain
To construct pex32 atg1, a PCR fragment containing the ATG1 deletion cassette was amplified with primers pDEL-ATG1-fwd and pDEL-ATG1-rev using plasmid pARM011 as a template. The resulting ATG1 deletion cassette was transformed into pex32 cells to get a double mutant of pex32 atg1. DraI-linearized pAMK15 plasmid was transformed into pex32 atg1 cells to produce DsRed-SKL.
Construction of strains expressing PEX11 under control of the alcohol oxidase promoter (PAOX)
Plasmid pHIPH4-PEX11 was produced by ligation of NotI and SmaI digested pHIPX4-PEX11 and pHIPH7-PEX11. The plasmid pHIPX4-PEX11 was constructed as follows: a PCR fragment containing PEX11 was obtained using primers Pex11-3 and Pex11-4 with WT genomic DNA as a template. The PCR product and pHIPX4 were restricted by HindIII and SalI, ligated which result in pHIPX4-PEX11. pHIPH7-PEX11 was constructed from the ligation of BamHI and XmaI digested pHIPH5-PEX11 and pHIPH7-DsRed-SKL. To get pHIPH5-PEX11, the PEX11 gene was amplified with primers PEX11-01 and PEX11-02 by using the WT genomic DNA as templates, BamHI and XmaI digested PCR fragment was inserted between BamHI and XmaI sites of pSEM04. NsiI-linearized pHIPH4-PEX11 was integrated into WT:GFP-SKL, pex11:GFP-SKL, and pex32:GFP-SKL strains, respectively, to produce PAOXPex11.
Preparation of yeast TCA lysates, SDS-PAGE, and western blotting
Cell extracts of TCA-treated cells were prepared for SDS-PAGE as described previously (Baerends et al., 2000). Equal amounts of protein were loaded per lane and blots were probed with anti-mGFP antibodies (sc-9996, Santa Cruz Biotech; 1:2000 dilution), anti-Pex11 antibodies (Knoops et al., 2014; 1:2,000 dilution), anti-Pex14 antibodies (Komori et al., 1997; 1:10,000 dilution), anti-Pex3 antibodies (Baerends et al., 1997; 1:5,000 dilution), anti-AOX antibodies (van der Klei et al., 1995; 1:10,000 dilution), or anti-pyruvate carboxylase 1 (Pyc1) antibodies (Ozimek et al., 2007; 1:10,000 dilution). Secondary goat anti-rabbit (31,460) or goat anti-mouse (31,430) antibodies conjugated to horseradish peroxidase (HRP) (Thermo Scientific; 1:5,000 dilution) were used for detection. Pyc1 was used as a loading control.
Quantification of western blots
Blots were scanned using a densitometer (Bio-Rad, GS-710) and protein levels were quantified using ImageJ software. The intensity of each band measured was normalized by dividing by the intensity of the corresponding Pyc1 band (loading control). Normalized values obtained for Pex11, Pex3, Pex14, and AOX levels in WT cells were set to one and levels in pex32 cells were displayed relative to WT control. Standard deviations were calculated using Excel. Significance was determined using two-tailed Student’s t-test. n. s. represents p-values > 0.05 and *** represents p-values < 0.001. The data presented are derived from three independent experiments.
Fluorescence microscopy
Wide-field FM images were captured at room temperature using a 100 × 1.30 NA objective (Carl Zeiss, Oberkochen, Germany). Images were acquired using a Zeiss Axioscope A1 fluorescence microscope (Carl Zeiss), Micro-Manager 1.4 software, and a CoolSNAP HQ2 digital camera. GFP fluorescence was visualized with a 470/40 nm band-pass excitation filter, a 495 nm dichromatic mirror, and a 525/50 nm band-pass emission filter. DsRed fluorescence was visualized with a 546/12 nm band-pass excitation filter, a 560 nm dichromatic mirror, and a 575–640 nm band-pass emission filter. mCherry and mKate2 fluorescence were visualized with a 587/25 nm band-pass excitation filter, a 605 nm dichromatic mirror, and a 670/70 nm band-pass emission filter.
Airy-scan images were captured with a confocal microscope (LSM800; Carl Zeiss) equipped with a 32-channel gallium arsenide phosphide photomultiplier tube (GaAsP-PMT), Zen 2009 software (Carl Zeiss) and a 63 × 1.40 NA objective (Carl Zeiss, Oberkochen, Germany). The GFP, mKate2, and mCherry fluorescence were visualized with a 488, 561, and 587 nm laser, respectively.
Image analysis was performed using ImageJ. Bright field images have been adjusted to only show cell outlines. Figures were prepared using Adobe Illustrator software.
Quantification of peroxisomes numbers
Peroxisome numbers were quantified using 200 randomly selected cells from three independent cultures. Numbers correspond to the average number of peroxisomes per cell. Standard deviations were calculated using Excel. Significance was determined using two-tailed Student’s t-test. n. s. represents p-values > 0.05 and *** represents p-values < 0.001.
Data availability statement
The raw data supporting the conclusion of this article will be made available by the authors, without undue reservation.
Author contributions
FW and IK conceived the project and reviewed and edited the manuscript. FW performed the experiments, analyzed the data, prepared the figures, and wrote the original draft.
Funding
This work was supported by a grant from the China Scholarship Council (CSC) to FW.
Acknowledgments
The authors thank Eline de Lange for strain construction and for performing the DysF domain swap experiment in Supplementary Figure S2. They thank Rinse de Boer for fruitful discussions and help with image analysis.
Conflict of interest
The authors declare that the research was conducted in the absence of any commercial or financial relationships that could be construed as a potential conflict of interest.
Publisher’s note
All claims expressed in this article are solely those of the authors and do not necessarily represent those of their affiliated organizations, or those of the publisher, the editors, and the reviewers. Any product that may be evaluated in this article, or claim that may be made by its manufacturer, is not guaranteed or endorsed by the publisher.
Supplementary material
The Supplementary Material for this article can be foundSupplementary Material online at: https://www.frontiersin.org/articles/10.3389/fcell.2022.957871/full#supplementary-material
References
Baerends, R. J. S., Salomons, F. A., Faber, K. N., Kiel, J. A. K. W., Van der Klei, I. J., Veenhuis, M., et al. (1997). Deviant Pex3p levels affect normal peroxisome formation in Hansenula polymorpha : High steady-state levels of the protein fully abolish matrix protein import. Yeast 13 (15), 1437–1448. doi:10.1002/(SICI)1097-0061
Baerends, R. J., Faber, K. N., Kram, A. M., Kiel, J. A., van der Klei, I. J., and VeenhuisM., (2000). A stretch of positively charged amino acids at the N terminus of Hansenula polymorpha Pex3p is involved in incorporation of the protein into the peroxisomal membrane. J. Biol. Chem. 275 (14), 9986–9995. doi:10.1074/jbc.275.14.9986
Carmichael, R. E., and Schrader, M. (2022). Determinants of peroxisome membrane dynamics. Front. Physiol. 13, 834411. doi:10.3389/fphys.2022.834411
Erdmann, R., and Blobel, G. (1995). Giant peroxisomes in oleic acid-induced Saccharomyces cerevisiae lacking the peroxisomal membrane protein Pmp27p. J. Cell Biol. 128 (4), 509–523. doi:10.1083/jcb.128.4.509
Faber, K. N., Haima, P., Harder, W., VeenhuisM., , and Ab, G. (1994). Highly-efficient electrotransformation of the yeast Hansenula polymorpha. Curr. Genet. 25 (4), 305–310. doi:10.1007/BF00351482
Ferreira, J. V., and Carvalho, P. (2021). Pex30-like proteins function as adaptors at distinct ER membrane contact sites. J. Cell Biol. 220 (10), e202103176. doi:10.1083/jcb.202103176
Jansen, R. L. M., Santana-Molina, C., van den Noort, M., Devos, D. P., and van der Klei, I. J. (2021). Comparative genomics of peroxisome biogenesis proteins: Making sense of the PEX proteins. Front. Cell Dev. Biol. 9, 654163. doi:10.3389/fcell.2021.654163
Joshi, A. S., Huang, X., Choudhary, V., Levine, T. P., Hu, J., and Prinz, W. A. (2016). A family of membrane-shaping proteins at ER subdomains regulates pre-peroxisomal vesicle biogenesis. J. Cell Biol. 215 (4), 515–529. doi:10.1083/jcb.201602064
Joshi, A. S., Nebenfuehr, B., Choudhary, V., Satpute-Krishnan, P., Levine, T. P., Golden, A., et al. (2018). Lipid droplet and peroxisome biogenesis occur at the same ER subdomains. Nat. Commun. 9 (1), 2940. doi:10.1038/s41467-018-05277-3
Knoops, K., Manivannan, S., Cepinska, M. N., Krikken, A. M., Kram, A. M., Veenhuis, M., et al. (2014). Preperoxisomal vesicles can form in the absence of Pex3. J. Cell Biol. 204 (5), 659–668. doi:10.1083/jcb.201310148
Komori, M., Rasmussen, S. W., Kiel, J. A., Baerends, R. J., Cregg, J. M., van der Klei, I. J., et al. (1997). The Hansenula polymorpha PEX14 gene encodes a novel peroxisomal membrane protein essential for peroxisome biogenesis. EMBO J. 16 (1), 44–53. doi:10.1093/emboj/16.1.44
Krikken, A. M., Veenhuis, M., and van der Klei, I. J. (2009). Hansenula polymorpha pex11 cells are affected in peroxisome retention. FEBS J. 276 (5), 1429–1439. doi:10.1111/j.1742-4658.2009.06883.x
Mast, F. D., Jamakhandi, A., Saleem, R. A., Dilworth, D. J., Rogers, R. S., Rachubinski, R. A., et al. (2016). Peroxins Pex30 and Pex29 dynamically associate with reticulons to regulate peroxisome biogenesis from the endoplasmic reticulum. J. Biol. Chem. 291 (30), 15408–15427. doi:10.1074/jbc.M116.728154
Ozimek, P. Z., Klompmaker, S. H., Visser, N., Veenhuis, M., and van der Klei, I. J. (2007). The transcarboxylase domain of pyruvate carboxylase is essential for assembly of the peroxisomal flavoenzyme alcohol oxidase. FEMS Yeast Res. 7 (7), 1082–1092. doi:10.1111/j.1567-1364.2007.00214.x
Ušaj, M., Brloznik, M., Kaferle, P., Zitnik, M., Wolinski, H., Leitner, F., et al. (2015). Genome-wide localization study of yeast pex11 identifies peroxisome-mitochondria interactions through the ERMES complex. J. Mol. Biol. 427 (11), 2072–2087. doi:10.1016/j.jmb.2015.03.004
van der Klei, I. J., Hilbrands, R. E., Swaving, G. J., Waterham, H. R., Vrieling, E. G., Titorenko, V. I., et al. (1995). The Hansenula polymorpha PER3 gene is essential for the import of PTS1 proteins into the peroxisomal matrix. J. Biol. Chem. 270 (29), 17229–17236. doi:10.1074/jbc.270.29.17229
Van Dijken, L. P., Otto, R., and Harder, W. (1976). Growth of Hansenula polymorpha in a methanol-limited chemostat. Physiological responses due to the involvement of methanol oxidase as a key enzyme in methanol metabolism. Arch. Microbiol. 111 (1–2), 137–144. doi:10.1007/BF00446560
Wang, S., Idrissi, F. Z., Hermansson, M., Grippa, A., Ejsing, C. S., and Carvalho, P. (2018). Seipin and the membrane-shaping protein Pex30 cooperate in organelle budding from the endoplasmic reticulum. Nat. Commun. 9 (1), 2939. doi:10.1038/s41467-018-05278-2
Wu, F., de Boer, R., Krikken, A. M., Aksit, A., Bordin, N., Devos, D. P., et al. (2020). Pex24 and Pex32 are required to tether peroxisomes to the ER for organelle biogenesis, positioning and segregation in yeast. J. Cell Sci. 133 (16), jcs246983. doi:10.1242/jcs.246983
Yan, M., Rachubinski, D. A., Joshi, S., Rachubinski, R. A., and Subramani, S. (2008). Dysferlin domain-containing proteins, Pex30p and Pex31p, localized to two compartments, control the number and size of oleate-induced peroxisomes in Pichia pastoris. Mol. Biol. Cell 19 (3), 885–898. doi:10.1091/mbc.e07-10-1042
Keywords: peroxisome, endoplasmic reticulum, pex32, PEX11, contact site
Citation: Wu F and van der Klei IJ (2022) Structure–function analysis of the ER-peroxisome contact site protein Pex32. Front. Cell Dev. Biol. 10:957871. doi: 10.3389/fcell.2022.957871
Received: 31 May 2022; Accepted: 12 July 2022;
Published: 09 August 2022.
Edited by:
Michael Schrader, University of Exeter, United KingdomReviewed by:
Johannes Freitag, University of Marburg, GermanyCelien Lismont, KU Leuven, Belgium
Harald W. Platta, Ruhr University Bochum, Germany
Copyright © 2022 Wu and van der Klei. This is an open-access article distributed under the terms of the Creative Commons Attribution License (CC BY). The use, distribution or reproduction in other forums is permitted, provided the original author(s) and the copyright owner(s) are credited and that the original publication in this journal is cited, in accordance with accepted academic practice. No use, distribution or reproduction is permitted which does not comply with these terms.
*Correspondence: Ida J. van der Klei, i.j.van.der.klei@rug.nl
†Present Address: Fei Wu, Division of Industrial Biotechnology, Department of Biology and Biological Engineering, Chalmers University of Technology, Gothenburg, Sweden