- 1Department of Dermatology, Yueyang Hospital of Integrated Traditional Chinese and Western Medicine, Shanghai University of Traditional Chinese Medicine, Shanghai, China
- 2Shanghai Skin Disease Hospital of Tongji University, Shanghai, China
- 3Institute of Dermatology, Shanghai Academy of Traditional Chinese Medicine, Shanghai, China
Atopic dermatitis (AD) is a chronic and relapsing cutaneous disorder characterized by compromised immune system, excessive inflammation, and skin barrier disruption. Post-translational modifications (PTMs) are covalent and enzymatic modifications of proteins after their translation, which have been reported to play roles in inflammatory and allergic diseases. However, less attention has been paid to the effect of PTMs on AD. This review summarized the knowledge of six major classes (including phosphorylation, acetylation, ubiquitination, SUMOylation, glycosylation, o-glycosylation, and glycation) of PTMs in AD pathogenesis and discussed the opportunities for disease management.
Introduction
Atopic dermatitis (AD) is one of the most common heterogeneous diseases, affecting 2.7%–20.1% of children and 2.1%–4.9% of adults worldwide (Barbarot et al., 2018; Silverberg et al., 2021). It manifests as systemic inflammation and epidermal barrier disruption, with multifactorial genetics, age, ethnicity, and geography (Yew et al., 2019). Ameliorating clinical signs and allergy burdens, preventing of recurrence and comorbidities, and improving quality of life are the key points on the disease management. Meanwhile, the allergic symptoms of AD are varied and complex, which range from mild to severe and even life-threatening anaphylaxis.
Extrinsic environmental factors are responsible for tremendous impacts on allergic disease; besides, the epigenetic modification is thought to determine, at least partly, in etiology and pathophysiology of allergies (Alashkar Alhamwe et al., 2020). Studies based on quantitative proteomic analysis have identified alterations in the protein post-translational modifications (PTMs) profile of patients with AD lesions compared with non-lesional sites (Winget et al., 2016). Indicating that PTMs emerge key roles in AD development (Kim et al., 2016; Potaczek et al., 2017). In this review, we overview the roles of PTMs in the pathophysiology of AD, focusing on the known mechanisms and functions of PTMs with particular emphasis on their therapeutic potential.
Post-Translational Modifications Relevance
PTMs are the chemical modifications of proteins following translation that confer functional diversity and maintain proteomes stability (Banerjee Mustafi et al., 2017). It can rapidly regulate a variety of biological functions (including cellular signaling, growth, survival, and proliferation) and modify inflammatory signaling pathways (Wu et al., 2017; Liu et al., 2019). Notably, aberrant PTMs may drive the development of inflammatory dermatitis with genetic predisposition, such as psoriasis and AD (Yi and McGee, 2021). Identifying the relationship between AD and PTMs could shed light on the disease pathogenesis and provide targets for the development of novel therapeutic and diagnostic tools. This review summarizes the seminal discoveries of six major PTMs (phosphorylation, acetylation, ubiquitination, SUMOylation, glycosylation, and glycation during the progression of AD and arouse in-depth research into the pathogenesis of disease.
Clinical Relevance
Notably, AD ranks 15th among the largest nonfatal disease burdens worldwide, while its pathogenesis is multifactorial and unclear in most affected individuals (Laughter et al., 2021; Ständer, 2021). Moreover, PTMs show therapeutic potential for curbing inflammation and restoring barriers, which are closely linked to numerous skin and autoimmune disorders (Yang and Yan, 2022). Exploring the indexes of PTMs therefore yield novel therapeutic targets, predictive events, monitor trends, or prognostic indicators for the clinical outcomes of AD patients. Current PTMs-related agents are insufficient for implying clinical utility, and further mechanistic studies ought to be conducted.
Pathological Manifestations in Atopic Dermatitis
The Intricate Immune Responses in Atopic Dermatitis
The lesional skin has two major cell compartments: resident skin cells, which include keratinocytes (KCs), and infiltrating cells, which include inflammatory cells. Those cells function in synergy and produce numerous inflammatory mediators to recruit immune cells, activate intracellular signaling pathways, and stimulate adaptive immune responses, resulting in exacerbating pathogenicity in AD (Ständer, 2021). The elevated immunoglobulin E (IgE) level is a hallmark of AD, which causes a systemic inflammatory response; moreover, the activated cytokines and chemokines, in turn, are responsible for inducing IgE production (Gandhi et al., 2016). Once the epidermal barriers have been destroyed, alarm-type cytokines [such as IL-25, IL-31, IL-33, and thymus stromal lymphopoietin (TSLP)] could initiate innate immune components group 2 innate lymphoid cells (ILC2s), followed by the dysregulation of the T helper (TH) 2 response (Ständer, 2021). Skin DCs, which include epidermis-resident DCs, Langerhans cells, and dermal DCs, could simultaneously recognize allergens and microbes, enter the dermis, and migrate to the draining lymph node in response to specific antigens by promoting the polarization of TH 2 cells (Callard and Harper, 2007; Novak, 2012).
Concerning adaptive immunity responses in AD, the presence of TH2/TH22 bias is shown in acute phases, while the TH1/TH17 bias is shown chronic phases (Patrick et al., 2021). In the acute phase, TH2 cells secreted cytokines (primarily IL-4, IL-5, and IL-13) that stimulate B cells to produce IgE antibodies and activate eosinophils, basophils, and mast cells in allergic responses; IL-5 is responsible for the trafficking and production of eosinophils in vivo (Gandhi et al., 2016). Activated ILC2s (expressing high levels of IL-5 and IL-13) could simultaneously facilitate TH2 differentiation and repress TH1 differentiation (Maggi et al., 2017), and IL-4 performs similar functions in AD pathogenesis (Murphy and Reiner, 2002; Chen et al., 2004; Lazarski et al., 2013). With the further identification of adaptive immunity in AD, acute lesions are always driven by the TH2/TH22 dominant allergic responses, while chronic lesions are driven by a TH1 response (Nograles et al., 2009; Lou et al., 2017). Local TH1 responses tend to induce KCs apoptosis through skin-infiltrating T cells (Grewe et al., 1998; Trautmann et al., 2000), and a correlation between TH17 cells and TH1 cells was found in the chronic phase of AD (Sugaya, 2020). The level of T regulatory cells (Tregs) is elevated during AD pathogenesis, which could suppress responses from allergen-specific T cells and participate in multi-directional immune reactions following TH2 and TH17 cells (Figure 1) (O’Shaughnessy et al., 2007; Agrawal et al., 2011; Jonak et al., 2011; Ma et al., 2014; Roesner et al., 2015; Niiyama et al., 2016).
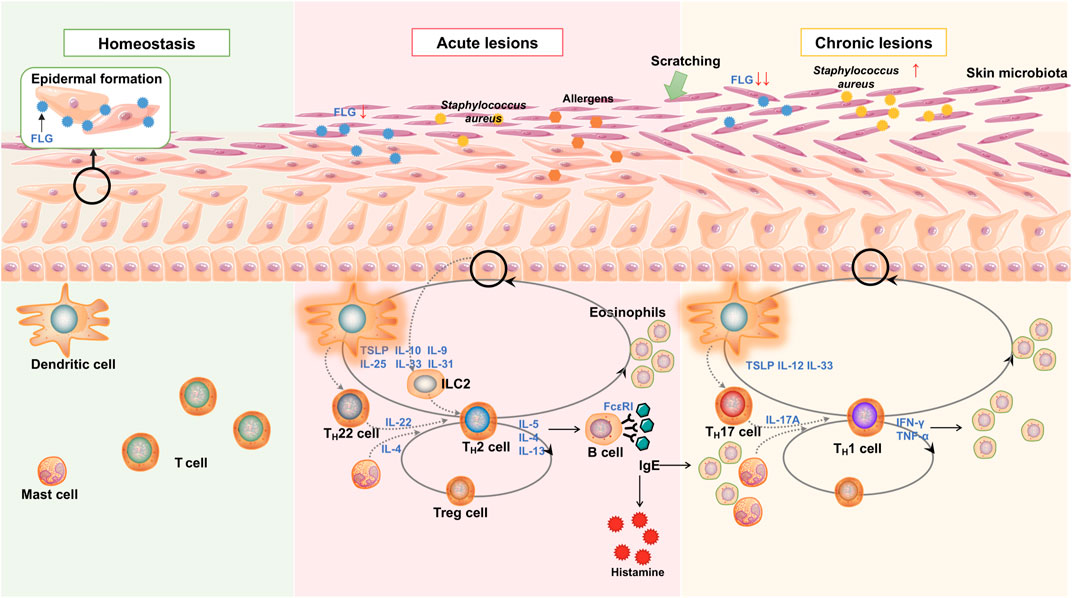
FIGURE 1. Main pathogenesis and mechanisms of AD. Epidermal barrier disruption mainly caused by mechanical scratch and aberrant inflammatory reactions. Reduced FLG contributes to inflammatory changes, while the releasing proinflammatory cytokines and chemokines result in further FLG deficiency. It is a positive feedback loop. LC and DC recognize the allergens and microbial components and stimulate adaptive immune responses—predominantly TH2/TH22 bias in the acute phase and TH1/TH17 bias in the chronic phase. The release of alarmins (including IL-10, IL-31, and TSLP) from epithelial cells promotes ILC2 induction as an innate immune response and aggravates TH2 immune response. TH2 cytokines IL-4, IL-5, and IL-13 recruit eosinophils and increase B cell IgE production during the acute phase. IL-22, produced by TH22, promotes TH2-type inflammation. In the chronic stage, TH1 and cytokines predominate in skin lesions, leading to further inflammation and epidermal hyperplasia. Inflammatory cytokines simultaneously impair the skin barrier by inhibiting barrier proteins and disrupting skin microbiota, thereby increasing the risk of S. aureus colonization.
The Disruption of the Skin Barrier in Atopic Dermatitis
Cutaneous irritation and sensitization can give rise to barrier dysfunction and prolonged inflammation. Impaired epidermal barrier is indicated mainly by skin irritations, hypersensitivity, increased transepidermal water loss, decreased liposomes, and elevated pH (Ishikawa et al., 2010; Jungersted et al., 2010; Janssens et al., 2012). For instance, filaggrin (FLG, a major structural protein in the upper epidermis) assists in the formation of the epidermal barrier (O’Regan et al., 2008; Weidinger and Novak, 2016), which presents a loss-of-function in approximately 10%–40% of patients with AD (Rodríguez et al., 2009; Winge et al., 2011; Margolis et al., 2014; Feketea and Tsabouri, 2017). Besides, the breakdown of the skin barrier function is multi-factorial, involving disruption of the biological, immunological, and mechanical barriers (Weidinger and Novak, 2016), and its exacerbation is caused by infection, chronic irritation, inflammation, and immune dysregulation (Biniek et al., 2012; Yosipovitch et al., 2019). Notably, altered expressions of these epidermal proteins could favor TH2 dominance in T-cell differentiation (Figure 1) (Hönzke et al., 2016).
The Dysbiosis of the Skin Microbiome in Atopic Dermatitis
Dysbiosis of the skin microbiome was detected in the dermis of AD patients, which contributes as a prominent environmental factor in the pathogenesis (Nakatsuji et al., 2016; Nakatsuji and Gallo, 2019). Specifically, the colonization of Staphylococcus aureus in the dermal lesions is associated with increased disease severity (Nakatsuji et al., 2016), which could exacerbate skin inflammation by activating lymphocytes and macrophages in adaptive and innate immune responses (Nakatsuji and Gallo, 2019). In addition, TH2 cytokines inhibit the generation of antimicrobial peptides of S. aureus in the skin and contribute to strenuous dysbiosis and infection during AD progression (Figure 1) (Howell et al., 2006; Nakatsuji and Gallo, 2019).
Involvement of Post-Translational Modifications in ADS
PTMs are essential for regulating protein folding, stability, localization, and functional activities. With the deep exploration of proteomics, exploring the involvement of PTMs in AD pathogenesis could generate novel ideas for disease therapeutics, control, and prevention. Several types of PTMs including phosphorylation, acetylation, ubiquitination, SUMOylation, glycosylation and glycation were summarized under current research situation.
Phosphorylation
Protein phosphorylation is an important modification for modulating protein stability, which occurs in almost one-third of the human proteome. The reversible process of phosphorylation/dephosphorylation is catalyzed by protein kinases and phosphatases, and is involved in cellular proliferation, growth, differentiation, and signal transduction (Krebs and Beavo, 1979). Phosphorylation frequently occurs at serine, threonine and tyrosine, which modulates various cellular signaling pathways and biological processes.
Mitogen-Activated Protein Kinases
MAPKs, a cluster of serine/threonine kinases protein, could be activated by phosphorylation, which participant in cell proliferation, differentiation, survival and regulate pivotal signal transcription (Song et al., 2015; Park et al., 2020). Three major MAPKs have been identified, including p38, extracellular signal-regulated protein kinase (ERK), and c-Jun N-terminal kinases (JNK).
MAPKs play facilitatory roles in the susceptibility to inflammation, which could activate the TNF-α signaling cascades and energize the NF-κB signaling pathways (Giridharan and Srinivasan, 2018); in turn, inflammatory cytokines could promote MAPK phosphorylation and aggravate the secretion of those mediators in AD development (Hong et al., 2015; Ryu et al., 2015; Noh et al., 2016; Pinto et al., 2018; Naruke et al., 2021; Ogura et al., 2021). Specifically, higher FcεRI (a receptor of IgE, can be upregulated by p38 phosphorylation) is detected in peripheral blood monocytes of AD patients compared with normal individuals, which increases the susceptibility of microbial antigens and allergens (Song et al., 2015). Besides, FcεRI-bound IgE migrates to the lymph nodes and stimulates naive T cells to expand and trigger the TH2 immune response in AD pathogenesis (Figure 1) (Song et al., 2015). Increased IL-9 levels in AD lesions suggest the activation of ERK phosphorylation, which contributes to the spontaneous induced inflammation (Hong et al., 2015). Intelectin-1 (ITLN1, can induce ERK phosphorylation and help to amplify the TH2 responses) is overexpressed in AD skin lesions, accompanied by significant expression of allergen-induced TSLP, IL-33, and IL-25 (Tsuji et al., 2001; Yi et al., 2017). In AD therapies for the pediatric population, vitamin K2 exerts an anti-inflammatory effect by means of inhibiting the JNK signaling and ERK1/2 phosphorylation (Zhang et al., 2021). Above studies present that MAPKs phosphorylation is a crucial biologic activator in triggering immune responses and amplifying allergic inflammation in the pathogenesis of AD.
Consistent with the results in AD patients, an increasing level of phosphorylated ERK1/2 was found in the 2,4-dinitrochlorobenzene (DNCB)-induced mouse model (Choi et al., 2021). Deletion of AnxA1 (an upstream regulator of ERK) leads to ERK hyperphosphorylation and aggravates local skin lesions with severe erythema, erosion, dryness, and epidermis thickening in the OVA-induced AD mice (Perretti and Gavins, 2003; Parisi et al., 2019). Alternatively, phosphorylated JNK and ERK inhibit the expression of FLG, which lead to the impaired epithelial integrity in AD mouse model (Cha et al., 2019a). Hence, we theorized that skin barrier homeostasis could be disrupted not only by phosphorylated MAPKs directly, but also by phosphorylation-activated inflammatory environment.
Based on the overexpressed ITLN1 in skin lesions of AD patients, further investigation confirmed that ITLN1 inhibitor could interfere with the phosphorylation of epidermal growth factor receptor (EGFR) and ERK and suppress the TH2 immune responses in vitro (Yi et al., 2017). High phosphorylated ERK1/2 was found in the TNF-α/IFN-γ induced HaCaT, which could activate the NF-κB and signal transducer and activator of transcription 1 (STAT1) signaling pathways and enhance inflammatory cytokines (Choi et al., 2021). Similar to other cytokine receptors, the activation of ERK phosphorylation requires calcium propagation; that is, the inhibition of calcium could, at least partially, disrupt ERK phosphorylation and ameliorate AD symptoms (Hong et al., 2015). IL-31, a relatively novel itch-relevant cytokine associated with TH2 cytokines, gives rise to calcium propagation and inflammation in primary human KCs in vitro (Lee et al., 2012). Moreover, phosphorylated JNK and ERK increases TSLP level in HaCaT (Jang et al., 2013), whereas the phosphorylation of p38 and ERK up-regulates IL-33 expression in IL-17A-induced normal human epidermal keratinocytes (NHEK) cells (Meephansan et al., 2013). Apart from proinflammatory properties, MAPKs phosphorylation is associated with fragile skin barrier as well. Hyperphosphorylated p38 can inhibit several junctional proteins (such as ZO-1 and RhoA) and destroy barrier function, and those barrier junctional proteins can be restored by inhibiting p38 kinase in NHEK cells (Jackson et al., 2011; Kanemaru et al., 2017). Furthermore, the inhibition of JNK could recover the reduction of FLG in TNF-α/IFN-γ induced HaCaT and block TNF-α-mediated inhibition of FLG and loricrin (LOR) in primary human KCs (Kim et al., 2011; Cha et al., 2019b).
In summary, MAPKs work as inflammatory amplification regulators and skin homeostasis destroyers in the pathogenesis of AD. The initial inflammatory responses activate MAPKs phosphorylation and the downstream factors, and then phosphorylated MAPKs urge the release of multiple pro-inflammatory factors, exacerbate the TH2 immune skew (Park et al., 2015; Choi et al., 2021), and involve in the regulation of skin barrier functions. Although there are no direct functional evidences of MAPKs necessity in AD pathogenesis, it demonstrated that MAPKs is associated with the disease attack and their inhibitors may provide new insights into the therapeutic drugs of AD treatment.
AKT (Protein Kinase B)
AKT, also called protein kinase B, is a serine/threonine kinase phosphorylated by phosphoinositide-dependent kinase-1 and mammalian target of rapamycin (the mTORC1 inhibitor) complex2 (Laplante and Sabatini, 2012; Nitulescu et al., 2018). Phosphoinositide 3-kinase (PI3K) could activate AKT and its downstream mTOR pathways, and AKT phosphorylates downstream factors in the promotion of diverse cellular functions, including growth, proliferation, and metabolism (Liu et al., 2014; Huang et al., 2018). Recent research have demonstrated that the PI3K/AKT/mTOR pathway contributes to the development of hyperproliferative and inflammatory skin diseases via regulating KCs proliferation and immune responses (Mercurio et al., 2021).
The PI3K/AKT/mTOR pathway is activated abnormally in AD patients’ skin lesions and peripheral T cells, as well as in the skin of DNCB-induced and oxazolone-induced AD mouse models (Xiao et al., 2017; Hu et al., 2021; Zheng et al., 2022). LY294002 (an inhibitor of PI3K signaling) rescues claudin1 expression in AD mice via inhibiting AKT phosphorylation (Hu et al., 2021). Rapamycin proved to balance TH1 and TH2 immune responses via influencing cytokines production and suppressing serum IgE in an AD mouse model (Yang F. et al., 2014a); besides, it could increase the levels of FLG, LOR, and involucrin in vitro (Jia and Zeng, 2020).
AKT phosphorylation has been implicated in skin inflammation, which determines the activation of NF-κB cascade and participants in the hyperproliferation and expanded inflammation in human primary KCs (Lee et al., 2011; Madonna et al., 2012; Mercurio et al., 2021; Xiao et al., 2017). In parallel, normal AKT activity is required for epidermal barrier function, while the AKT/mTOR pathway controls the formation and maintenance of skin barrier (Naeem et al., 2017; Ding et al., 2020; Hu et al., 2021; Mercurio et al., 2021). Knock-down or deletion of AKT could decrease the expression of FLG and instigate hyperkeratosis both in vivo and ex vivo (Thrash et al., 2006; O’Shaughnessy et al., 2007; Naeem et al., 2017); the absence of AKT could impede KCs proliferation in HaCaT (Buerger et al., 2017). Further, mTORC2 and its related proteins control the expression of FLG through AKT in a phosphoinositide-dependent kinase 1-dependent manner (Naeem et al., 2017; Ding et al., 2020). Alternatively, LY294002 could attenuate the hyperproliferation of T cells and the secretion of pro-inflammatory cytokines in AD patient-derived T cells (Xiao et al., 2017).
In summary, these studies suggest that the inhibitor of AKT phosphorylation could act as putative candidates in inhibiting skin immunological reactions and restoring skin barrier dysfunction in AD.
AMP-Activated Protein Kinase
AMPK is a serine/threonine kinase as well, whose early activation was evidenced by phosphorylation (Giansanti et al., 2020). It is always phosphorylated at threonine172 by liver kinase B1 and calcium/calmodulin-dependent protein kinase β (Shaw, 2009). AMPK is a principal cellular regulatory system, which maintains immune homeostasis and controls metabolic processes (Garcia and Shaw, 2017; Herzig and Shaw, 2018). Aberrant AMPK activation may contribute to diseases with abnormal proliferation of histiocytes, such as psoriasis and cancers (Garcin et al., 2015; Shen et al., 2021). Besides, AMPK is reported as a negative regulator of ERK signaling, as its deletion increased the ERK phosphorylation and the upstream pathway of ERK activation (Wu et al., 2013).
IL-37, an anti-inflammatory and immunosuppressive cytokine, counterbalances excess inflammation via activating AMPK signaling (Pan et al., 2020). Clinically, increased IL-37 expression was detectable in the skin lesions and serum of AD patients (Fujita et al., 2013), whereas IL-37 could attenuate inflammation symptoms, eliminate eosinophil infiltration, and increase Treg cells through the AMPK/mTOR signaling (Hou et al., 2020). Furthermore, AMPK could regulate the mTOR signaling to influence a range of cellular functions, including cell proliferation and metabolism (Shaw, 2009). The loss of AMPK performs direct effects on KC hyperproliferation and hyperactive mTOR signaling in a transgenic mice (Crane et al., 2021). Moreover, nicotine could decrease the risk of AD by inhibiting the expression of TSLP through a AMPK-mediated suppression of NF-κB signaling both in vivo and in vitro (Dong et al., 2016).
Above results reflect the mechanism of maintaining immunologic homeostasis and suppressing inflammation via AMPK phosphorylation in AD disorders; whereas additional molecular mechanisms are required to further clarify the relationship between AMPK and AD.
Other Proteins
In addition to the aforementioned signaling molecules, we identified the other known proteins and their status associated with AD. Phosphorylation of ribosomal protein S6 is required for translation initiation and occurs on the serine residues of carboxyl-terminus, which is associated with the inflamed extent (Ruvinsky and Meyuhas, 2006; Ruf et al., 2014). Increased phosphorylated ribosomal protein S6 has been observed in epidermal lesions of AD patients, which is activated by the mTOR and Ras/ERK signaling (Ruf et al., 2014). Phosphorylated l-plastin (a leukocyte-specific actin-binding protein) increases in eosinophils from AD patients compared to healthy individuals (Noh et al., 2016), which could enhance inflammatory cell migration and aggravate phosphorylation in turn (Wabnitz et al., 2007; Pazdrak et al., 2011; Noh et al., 2016). Consistently, l-plastin phosphorylation could increase the migration of eosinophils (Pazdrak et al., 2011). Reflected that proteins phosphorylation play important roles in the migration of inflammatory cells and the amplification of immune responses. Heat shock proteins 27 (HSP27) can directly bind to AKT and result in increased phosphorylation of AKT and MAPK, which are required for KCs differentiation and epidermis formation (O’Shaughnessy et al., 2007; Jonak et al., 2011); further, elevated HSP27 levels were correlated with increased severity of the AD disease severity (Niiyama et al., 2016).
In general, phosphorylation is involved in the major pathogenesis of AD, including inflammation infiltration, immunization amplification, increased allergen sensitivity, and barrier maintenance. Multiple targets regulate distinct signaling pathways and provide diagnostic potential in AD management.
Acetylation
Protein acetylation is one of the most common PTMs in which the acetyl group is introduced to a specific site on a polypeptide chain. Acetylation is a reversible process regulated by acetylase and deacetylase enzymes, involved in modulating chromatin structure, gene expression, and protein function (Verdin and Ott, 2015). The caveat here is that acetylation of histones (catalyzed by histone acetyltransferases) is associated with transcriptional activation; while histone deacetylation (catalyzed by histone deacetylases) is associated with transcriptional repression. Evidence suggests that histone acetylation and deacetylation are essential regulators of pro-inflammatory genes in allergic diseases, including histone deacetylase 3 (HDAC3), HDAC6, and sirtuin1 (SIRT1) (Alaskhar Alhamwe et al., 2018).
A clinical study confirmed that HDAC inhibitors could mitigate pruritus via decreasing IL-31 expression in peripheral blood from leukemic cutaneous T cell lymphoma patients (Cedeno-Laurent et al., 2015). As we know, to some extent, AD and T-cell mediated dermatitis share a similar underlying pathological mechanism. SIRT1 (an NAD-dependent protein deacetylase, which exists interdependent functions with HDAC6) plays a critical role in skin barrier maintenance, and is decreased in skin lesions of AD patients (Ming et al., 2015). Besides, belinostat (a histone deacetylase inhibitor) could restore skin barrier function and increase FLG expression in an ex vivo human skin culture model (Liew et al., 2020). Aryl hydrocarbon receptor nuclear translocator (ARNT; the loss-of-ARNT could increase HDAC levels) is up-regulated in skin lesions of AD patients compared to normal participants (Robertson et al., 2012; Kim et al., 2014; Hong et al., 2016). Results showed that some protein deacetylation could promote skin barrier renovation, while the relationship between barrier restoration and deacetylation degree remains obscure.
Furthermore, HDAC3 (a regulator of gene expression) is proven to mediate allergic inflammation, and its inhibitor could alleviate the skin inflammation of dinitrofluorbenzol (DNFB) -induced mice model (Kim et al., 2012). Similarly, HDAC6 (a regulator of immune responses) is a key point in regulating the activation and function of CD8+ T-cell inflammation responses in an AD mouse model (Tsuji et al., 2015) Phenylbutyrate (a kind of HDAC inhibitor) shows therapeutic effects on both acute and chronic skin inflammation via inhibiting local mast cells and activating Tregs in a DNFB-induced AD mouse model (Chung and Pui, 2011). Trichostatin A (TSA, another kind of HDAC inhibitor) can alleviate the DNFB-induced AD-like skin lesions in NC/Nga mice by exerting anti-inflammatory protective effects and increasing Treg cell population (Kim et al., 2010; Shi et al., 2012). In addition, SIRT1 is decreased in the cutaneous tissues of AD mouse model, and its modification provides relief from inflammation symptoms via suppressing the expressions of TSLP, cyclooxygenase-2, macrophage inflammatory protein 2, and C-X-C motif chemokine ligand 13 in vivo (Kwon et al., 2021). Previous studies have documented that SIRT1 plays an anti-inflammation role mainly by deacetylating NF-κB signaling (Yang et al., 2012; Kauppinen et al., 2013). On the other hand, mice lacking SIRT1 in KCs became susceptible to obtaining a fragile epithelial barrier with low expression of FLG (Ming et al., 2015). These findings suggest that HDAC inhibitors are found to be protective in various aspects of AD.
Notably, many studies focused on the use of HDAC inhibitors, and several active agents could work as HDAC inhibitors. Butyric acid and its derivatives, conducting as HDAC inhibitors, exert in vitro anti-inflammatory and antibacterial properties in HaCaT (Traisaeng et al., 2019). Similarly, sodium butyrate promotes cellular terminal differentiation and alleviates inflammatory responses induced by EGFR inhibition in NHEK cells (Leon Carrion et al., 2014). Propionate and valerate can also inhibit HDAC activity; they could increase pro-inflammatory factors secreted by KCs and decrease pro-inflammatory factors secreted by myeloid-derived immunocytes and ultimately exert a strong anti-inflammatory potential (Sanford et al., 2016). Given that those HDAC inhibitors should also be a group of anti-infective and anti-inflammatory proteins that play vital roles in inflammatory hyperproliferative diseases. Another, belinostat has been shown as a potential treatment for AD for its role in restoring FLG expression via inducing sustained miR-335 expression in N/TERT-1 cells (Liew et al., 2020). TSA decreases levels of FLG and LOR in N/TERT cells, resulting in worsen skin barrier (Robertson et al., 2012).
Overall, these findings suggest that acetylation modifications play an essential role in the skin barrier maintenance and inflammatory responses in AD progression. HDAC inhibitors emerging as promising therapeutic targets for AD treatment, while further in-depth studies are required.
Ubiquitination
Ubiquitination is a dynamic and reversible PTM conserved in eukaryotic cells, which can tag proteins by proteasomes degradation (Feng et al., 2017). Proteasome is a part of the ubiquitin-proteasome system. The ubiquitin-proteasome system mediates the cellular polyubiquitination of substrate proteins and proteolytic degradation, including three types of enzymes: E1 ubiquitin-activating enzymes, E2 ubiquitin-conjugating enzymes, and E3 ubiquitin ligases. Deubiquitinating enzymes (DUBs) are responsible for specifically removing ubiquitin from ubiquitinated proteins and contributing to their stability (Ciechanover, 2015). Thus, ubiquitin could be re-used rather than degraded. Ubiquitinating and deubiquitinating can change intracellular homeostasis and modulate the cellular cycle, proliferation, and survival (Kwon and Ciechanover, 2017). Notably, current studies confirmed that E3 ubiquitin ligases and DUBs play roles in the AD pathophysiology (Fang et al., 2002; Loeser and Penninger, 2007a; Mohapatra et al., 2013a; G’Sell et al., 2015; Theivanthiran et al., 2015; Liu et al., 2017; Salva et al., 2017; Tang et al., 2018; Devos et al., 2019; Harirchian et al., 2019; Sundberg et al., 2020; Wang et al., 2021).
Genome-wide analysis found that AD pathogenesis involves a variety of gene expression abnormalities and ubiquitinated proteins (Acevedo et al., 2020). The tripartite motif 32 (Trim32, a member of the Trim E3-ubiquitin ligase family) is decreased in the skin lesions of AD patients, which implicates in inflammatory and immune processes (Guttman-Yassky et al., 2009; Liu et al., 2017). Sharpin (an adaptor protein for the linear ubiquitin chain assembly complex) is decreased in the lesions of AD patients, which appears as a potential anti-inflammatory candidate (Tang et al., 2018). Besides, casitas B-lineage lymphoma (c-CBL, a ring-type E3 ubiquitin ligase) presents the high expression level in the skin lesions from AD patients, which associated with T cell activation dysregulation and excess inflammatory infiltration (Loeser and Penninger, 2007b; Mohapatra et al., 2013b; Salva et al., 2017). Zinc finger protein A20 (A20, a ubiquitin-editing enzyme) is an endogenous anti-inflammatory factor related to NF-κB signaling, which is down-regulation in the epidermis from AD patients by transcriptome analysis (Devos et al., 2019; Mooney and Sahingur, 2021). Evidences above present a strong correlation between ubiquitinated proteins and AD mechanisms, involving genetic factors, inflammation extents, and immune dysregulation.
According to the down-regulation of Trim32 and Sharpin in AD patients, similar findings were observed that Trim32-deficient mice present TH2-biased inflammation spontaneously developed in imiquimod-induced psoriatic dermatitis (Liu et al., 2017; Wang et al., 2021), while KCs-specific Sharpin knockout mice developed more severe inflammatory AD lesions compared to normal ones (Sundberg et al., 2020). Transmembrane protein 79 (TMEM79, a predisposition gene for AD) can specifically inhibit ubiquitin-specific peptidase 8 deubiquitination, and its deficiency shows more susceptible to developing skin inflammation, compromised barrier function, and spontaneous dermatitis (Lim et al., 2013; Sasaki et al., 2013; Saunders et al., 2013; Chen et al., 2020). ITCH (an E3 ubiquitin ligase of the HECT family) inhibit p38α signaling by ubiquitylation of TGF-activated kinase 1–binding protein 1; hence suppressing skin inflammation in vivo (Theivanthiran et al., 2015). Itch-deficient mice exhibit TH2 inflammation and a scratching phenotype, and their lymphocytes exhibit a preference for TH2 differentiation, which is associated with the inhibition of JunB ubiquitylation caused by ITCH (Fang et al., 2002). Based on the anti-inflammatory properties of A20, additional experiments showed that A20-deficient mice developed TH2-biased immune responses, systemic pro-inflammatory changes, epidermal hyperplasia, and a disrupted skin barrier (Devos et al., 2019). Further indicated that deregulated ubiquitination events were closely related to TH2 cell differentiation, barrier reparation, and worsened inflammatory responses in AD pathogenesis.
Recent scRNA-seq data demonstrated that overexpressed A20 could suppress inflammatory transcripts induced by IL-17A in NHEK cells (Harirchian et al., 2019), indicating that A20 provides a potential AD therapeutic modality against inflammation. Reportedly, MID-1, a kind of E3 ubiquitin ligase, promotes TH2 type inflammation in allergic asthma (Collison et al., 2013). Nedd4 family interacting protein 2 might be a protective factor, and its absence inhibits the catalytic function of Nedd4-family E3 ubiquitin ligase and inappropriate TH2 responses during AD pathogenesis (O’Leary et al., 2016). Clinical and experimental data proposed that Sharpin is down-regulated in AD tissues, and Sharpin-silencing could increase FLG expression in vitro, implicating Sharpin as a novel mediator in both inflammation responses and barrier repair (Tang et al., 2018). Furthermore, many E3 ubiquitin ligases in regulating KCs proliferation and maintaining the epithelial tight junction function, such as Trim21 (Yang et al., 2018, 21), March-3 (Leclair et al., 2016, 3), Nedd4-1 (Yan et al., 2021, 1), Nedd4-2 (Raikwar et al., 2010), Chip (Löffek et al., 2010; Katagata, 2011), Otulin (Hoste et al., 2021; Schünke et al., 2021) and Trip (Almeida et al., 2011).
In conclusion, above studies highlight the critical role of ubiquitination in the initiation, progression and outcome of AD, and E3 ubiquitin ligases might be potential therapeutic targets for AD therapy.
Small Ubiquitin-Like Modifier Ylation
SUMOylation is similar to ubiquitination and has approximately 11 kD proteins attached to lysine residues on target proteins covalently (Feligioni et al., 2009). SUMOylation is catalyzed by SUMO-specific enzymes of E1, E2, and E3, and regulates several functional properties, including chromatin organization, transcription, and DNA repair (Flotho and Melchior, 2013).
Increased miR-146a (which targets and represses SUMO1 directly) is found in the inflamed biopsy tissues and serum of AD patients, and its deficiency aggravates inflammation in MC903 (calcipotriol)-induced AD mice (Rebane et al., 2014; Yan et al., 2019a). Besides, protein inhibitor of activated STAT1 (PIAS1, a SUMO E3 ligase) is down-regulated in the peripheral blood mononuclear cells of AD dogs, which exhibits increasing IL-13 concentration and functional insufficiency of Tregs (Majewska et al., 2016; Koury et al., 2019). Several other studies noted that PIAS1 could restrict the differentiation of Tregs (Liu et al., 2010; Yang et al., 2013). It is suggested that miR-146a and PIAS1 might be therapeutic targets for AD management, and deciphering the SUMOylation effects will be required to investigate this further.
Glycosylation
Glycosylation is a highly dynamic and reversible PTMs of proteins, which is catalyzed by a series of enzymes and adhered to abundant glycans. The biosynthesis of glycans is an intricate process requiring the coordinated action of multiple glycosyltransferases and glycosidases to synthesize discrete structures (Agrawal et al., 2017). Glycosylation has been shown to alter the functional activities of proteins and result in autoimmune disorders (Zhou et al., 2021); besides, impaired glycosylation can cause marked serum IgE elevations and severe inflammation (Yang et al., 2014b; Zhang et al., 2014). Several types of glycosylation, including O-linked GlcNAcylation and N-linked glycosylation, have been aroused increasing attention.
Reportedly, the deficit of phosphoglucomutase3 (a critical sugar nucleotide in glycosylation precursor synthesis) results in a prolonged inflammation in AD patients with increasing serum IgE elevations (Yang et al., 2014b; Zhang et al., 2014); its knockdown in T cells tends to produce excessive TH17 and TH2 cytokines (Zhang et al., 2014). Langerhans cells can identify and interact with S. aureus directly through conserving β-N-acetylglucosamine modifications on wall teichoic acid, indicating that β-N-acetylglucosamine is a key trigger in bacterial skin infection (van Dalen et al., 2019). The glycosylation of IgE is important to regulate allergic diseases, which contains seven asparagine N-linked glycosylation sites, providing a compelling diagnostic strategy for AD and other atopic diseases (Shade et al., 2020). These findings highlight the role of glycosylation in the genetic factors, external environment, and immune progression of AD.
Glycation
Protein glycation is a common PTMs, where proteins, lipids, and nucleic acids react non-enzymatically leading to the formation of advanced glycation end products (AGEs) (Hanafy et al., 2021). AGEs exacerbate the inflammatory response through promoting the release of proinflammatory factors and the production of reactive oxygen species; therefore, the accumulation of AGEs is commonly associated with inflammatory and metabolic disorders (Botros et al., 2017; Papagrigoraki et al., 2017). Evidence showed that the increased exogenous AGEs exposure enhances the risk of developing AD and other atopic diseases (Smith et al., 2017).
A previous study found that urinary pentosidine, an AGE formed by sequential glycation and oxidation, tends to be higher in AD patients with acute exacerbation (Tsukahara et al., 2003). Correspondingly, the level of AGEs in corneocytes from AD patients is increasing along with lesion severity (Hong et al., 2020). Additionally, an increasing expression of receptor for AGEs (RAGE) is observed in AD-like mouse models, which results in the release of pro-inflammatory cytokines (Dumitriu et al., 2005; Karuppagounder et al., 2015; Wang et al., 2018). In sum, AGEs provide an attractive addition to novel diagnostic markers in AD, while further studies will be necessary to assess the therapeutic efficacy and safety of AGE-associated inhibitors.
Crosstalk Among PTMS in Atopic Dermatitis
Remarkably, PTMs located within the same protein (especially on histones) can influence each other, and regulate the structure, activity and function of proteins (Narita et al., 2019). They function as molecular switches, and modify the interaction of proteins with DNA, lipids and other proteins (Venne et al., 2014; Narita et al., 2019). Emerging data indicates that there is significant regulatory crosstalk between PTMs during AD pathophysiology, in which the initial PTM serves as an active trigger for the addition or removal of a second PTM (detailed in Table 1) (Lin, 2002; Laarse et al., 2018).
Janus kinase (JAK)-STAT pathway play roles in AD pathogenesis by means of regulating TH2 differentiation, and its activation is caused by the phosphorylation of the two main proteins (JAK and STAT) (Szalus et al., 2020). Interestingly, the lysine-acetylation of STAT could activate STAT phosphorylation directly, except JAK-induced phosphorylation (Krämer et al., 2009; Zhuang, 2013). It is promising that HDAC inhibitors provide synergistic effects on JAK inhibitors in AD treatment (Quintás-Cardama et al., 2012; Rösler et al., 2018; Su et al., 2021).
Increased ERK phosphorylation has been found in an HDAC6-dependent manner in mast cells isolated from AD mice skin tissue (Kwon et al., 2021), suggesting that acetylation is vital for regulating ERK phosphorylation. A similar resolution has been postulated in heart failure (Habibian and Ferguson, 2018). Besides, the activation of NF-κB depends on IκB phosphorylation and subsequent IκB ubiquitin-dependent degradation (Choi et al., 2018; Giridharan and Srinivasan, 2018). Thus, inhibiting IκB protein degradation is one proven approach for reduce immune activation and inflammation in AD treatment.
PIAS4 (an E3-SUMOylation ligase with comparable functions to PIAS1) was previously identified as a significant E3-ubiquitin ligase Trim32 substrate, while the Trim32-deficiency in KCs could result in PIAS4 accumulation and increase global SUMOylation (Albor et al., 2006; Kudryashova et al., 2012). Besides, Trim32 activates NF-κB to induce KCs apoptosis and thus upregulates TH17 versus TH2 immunity, which explains the lower expression of Trim32 observed in AD patients (Albor et al., 2006; Liu et al., 2010; Samaka and Basha, 2020). Indicating that Trim32 may mediate inflammatory responses through SUMOylation and ubiquitination.
Furthermore, acetylation affects protein stability by suppressing ubiquitination and vice versa (Li et al., 2010; Incani et al., 2014; Shimizu et al., 2021). p62 (a ubiquitin binding protein) has a high ubiquitin-binding activity and facilitates protein ubiquitination and degradation (Boyault et al., 2006; Moscat and Diaz-Meco, 2009; Galindo-Moreno et al., 2017; Zientara-Rytter and Subramani, 2019), and it could maintain the autophagic process in homeostasis through inhibiting HDAC6 expression (Yan et al., 2013; Galindo-Moreno et al., 2017). On the other hand, membrane associated Ring-CH-1 (MARCH-1, an E3 ubiquitin ligase) could facilitate the expression of OX40L (a co-stimulatory molecule that could induce TH2 inflammation in AD) and decrease the amount of thymic Treg cells via increasing HDAC11 ubiquitination (Oh et al., 2013; Kishta et al., 2018; Castellanos et al., 2021; Furue and Furue, 2021). These findings suggest that E3 ligases could specifically target HDAC ubiquitination and thus enhance histone acetylation in AD pathophysiology (Scognamiglio et al., 2008).
While the mechanisms of PTMs crosstalk in AD have not been thoroughly investigated, they are also important. Of additional concern is the negative crosstalk between these two PTMs remains poorly understood (Venne et al., 2014; Mondal et al., 2021).
Potential Clues for Atopic Dermatitis Diagnosis and Treatment
The prevalence of AD is increasing worldwide, although estimates in developed countries are stabilizing (Langan et al., 2020). Currently, topical corticosteroids are still the first-line therapy for AD (Cury Martins et al., 2015). If corticosteroids become ineffective or present adverse effects, treatment with phototherapy, systemic immunotherapy, and molecular targeted therapies (such as dupilumab, tralokinumab, baricitinib, and upadacitinib) would be used (Wollenberg et al., 2019; Blauvelt et al., 2021). Given the exceptional performance of biological agents in the treatment of autoimmune diseases, bio-therapeutics may be the future of moderate-to-severe AD treatment.
We emphasized that aberrant PTMs could trigger complex cascades of multi-cellular and multi-factorial pathways in AD pathophysiology, and the diagnostic and prognostic significance of PTMs should however be mentioned. Currently, dermal AGEs and urinary pentosidine have been used as biomarkers for early detection and prognosis estimation in AD (Tsukahara et al., 2003; Hong et al., 2020). With the introduction of skin autofluorescence in AGEs measurement, it raises the possibility of non-invasive tools in assessing both the disease severity and the comorbidity risks in the future (Ying et al., 2021). Besides, specific IgE glycosylation sites appear to be more sensitive biomarkers compared to serum IgE in the early differential diagnosis of atopic diseases (Shade et al., 2019; Shade et al., 2020). Hence, PTMs could work as potential biomarkers for diagnosis and prognosis of AD.
Furthermore, PTMs participate in AD pathophysiology via regulating related transcriptional factors, signaling molecules and proteins; targeting PTMs are prone to improve the synergistic effect of AD treatment. Growing evidence proves that PTMs are key effectors in skin inflammation and TH2 differentiation, which precisely improve the stability and activity of diverse signaling pathways including JAK/STAT, PI3K/AKT/mTOR, and NF-κB signalings (Liu et al., 2014; Choi et al., 2018; Giridharan and Srinivasan, 2018; Huang et al., 2018; Szalus et al., 2020). Besides, activating protein PTMs contributes to the maintenance of epidermal homeostasis, involved in regulating KC proliferation, sustaining tight junction function, and assisting barrier formation. To date, HDAC inhibitors (including vorinostat, romidepsin, belinostat, and panobinostat) have been approved by the FDA for the treatment of cutaneous T-cell lymphoma, peripheral T-cell lymphoma and multiple myeloma, whereas those diseases share similar pathological mechanisms with AD (Yi and McGee, 2021). Although there are no demonstrated PTM targeting approaches for AD treatment currently, some small molecule compounds (such as HADC inhibitors, MAPK inhibitors, and AKT inhibitors) modifying PTMs have shown therapeutic efficacy at the animal and cellular levels (Table 2). While none of these studies have explicitly focused on alleviating AD symptoms, the demonstrated PTMs-related biological effects both in vivo and in vitro are encouraging results that may bring PTMs-related therapeutics to the forefront in AD research.
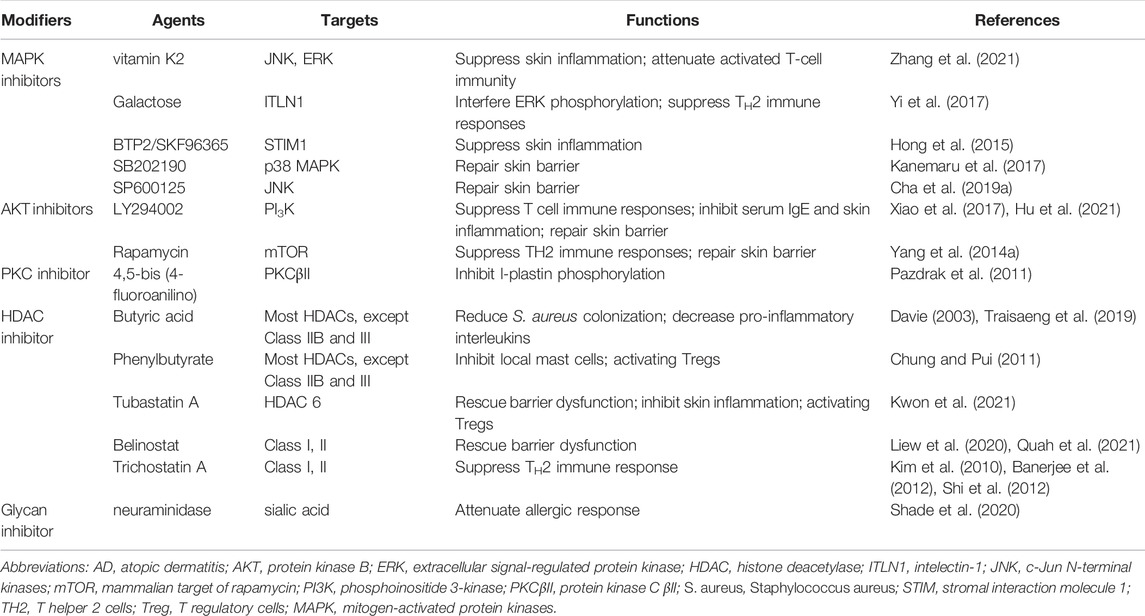
TABLE 2. Potential therapeutic target in AD associated with protein post-translational modifications.
Summary
PTM is one of the later steps in protein biosynthesis, and modulating innate functions of proteins precisely. Characterized protein modifications could cause differentiation of pro-inflammatory T cells, altering inflammatory cytokines, maintaining the normal skin barrier function, increasing sensitivity to allergens, and triggering skin infections (summarized in Figure 2 and Table 3). Targeting these modifications could provide major benefits in AD management. Intriguingly, the interaction among single PTMs sites and synergistic effects among multiple PTMs through which could account for the incredibly complex links involved in the AD pathogenesis to some degree. Nowadays, there were no PTMs-mediated drugs for AD treatment in clinic, although several small molecule compounds function as HDAC inhibitors, MAPKs inhibitors and AKT inhibitors have become available. PTMs crosstalk can integrate diverse signals and vastly increase their regulatory potential in the course of AD disease, while lacks further experimental verification both in vivo and in vitro.
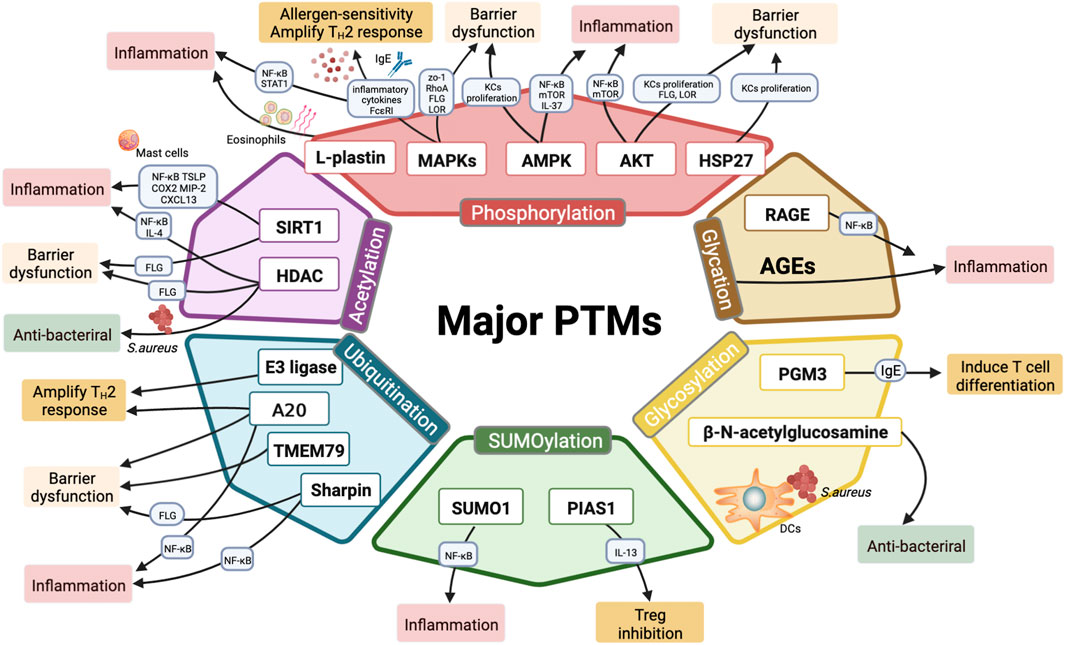
FIGURE 2. Major PTMs involved in the pathogenesis of AD. Six major classes of PTMs involved in AD include phosphorylation, acetylation, ubiquitination, SUMOylation, glycosylation, o-glycosylation, and glycation. Phosphorylation of PTM-related enzymes (MAPKs, AKT and AMPK) and l-plastin may regulate epidermal inflammation and TH2 immune response by modulating inflammatory cytokine secretion (IL-9, IL-25, IL-31, IL-33, TSLP, and IL-37), promoting eosinophils migration, and activating AD-related pathways (such as NF-κB, JAK-STAT, mTOR signaling). Moreover, MAPK p38 phosphorylation upregulates FcεRI and results in allergen-induced hypersensitivity; besides, MAPK phosphorylation inhibits epidermal proteins (FLG, LOR) and tight junction proteins (RhoA, ZO-1), thereby disrupting the barrier functions. Meanwhile, both AKT phosphorylation and AMPK phosphorylation are involved in epidermal barrier function. Phosphorylation of AKT and HSP27 are required for KCs differentiation and epidermis formation. Histone deacetylases (SIRT1, HDAC) play paramount importance in regulating inflammation and maintaining skin barrier functions. Additionally, HDAC inhibitors exhibit antibacterial properties in AD treatment. Multiple E3 ubiquitin ligases are linked to dysregulated T-cell activation and excessive inflammatory infiltration. Ubiquitination editing enzyme A20 maintains skin barrier hemostatic and alleviates inflammation. Other ubiquitination-related proteins (TMEM79, Sharpin) act as mediators in inflammation responses and barrier repair as well. MiR-146 targets SUMO1 to regulate epidermal inflammation, whereas PIAS1 restricts the differentiation of Tregs by elevating IL-13. PGM3 reduces inflammation by inhibiting IgE production and T cell differentiation. β-N-acetylglucosamine modifications promote the identification and the interaction of LCs with S. aureus, thereby triggering bacterial skin infections. AGEs are formed by glycation process and exacerbating inflammatory responses via releasing proinflammatory factors; RAGE, the receptor of AGEs, stimulates skin inflammation via activating NF-κB phosphorylation.
This review provides novel insights regarding the pathogenesis of AD and the development of PTMs-based strategies for inflammatory and allergic diseases therapy. However, the identification of PTMs-related regulators in AD remains in its infancy. Based on advanced proteomics techniques, the intensive studies of PTMs may open up new avenues for the evaluation criteria of AD. We look forward that PTMs-based diagnosis, monitoring and therapeutic approaches will be prevalent in AD patients and play major roles in the future.
Author Contributions
BL and MZ contributed to the conception and design of the article. XM and YR drafted and wrote the manuscript. Q-LC, YB, Y-QL, and JC interpreted the relevant literature. YUL and J-KS revised the figures and tables. YIL and LK evaluated the manuscript. All authors have read and approved the final manuscript.
Funding
This study was supported by a grant from the National Key Research and Development Program of China (No. 2018YFC1705305). It was also supported by the NSFC (Nos 82174383, 82004235, 82004359, 81973860, 81904214, and 81903215), the Shanghai Development Office of TCM [Nos ZY (2018–2020)-FWTX-4010, ZY (2018–2020)-FWTX-1008], and Dermatology Department of Traditional Chinese Medicine, Clinical Key Specialty Construction Project of Shanghai (No. shslczdzk05001), Young Talent Supporting Program of the China Association of Traditional Chinese Medicine [No. CACM-2021-QNRC2-(A10)], Xinglin Youth Scholar of Shanghai University of Traditional Chinese Medicine (No. RY411.33.10), Shanghai Science and Technology Development Funds (Sailing Program) (Nos 21YF1448100, 22YF1441300, and 22YF1450000), Clinical Transformation Incubation Program in Hospital (No. lczh 2021-05), Research Project of Shanghai Clinical Research Plan of SHDC (No. SHDC2020CR4053), Municipal Health Care Commission (No. 20204Y0312), Key Project of Clinical Research from Shanghai Hospital Development Center (NosSHDC2020CR4020 and SHDC12020117).
Conflict of Interest
The authors declare that the research was conducted in the absence of any commercial or financial relationships that could be construed as a potential conflict of interest.
Publisher’s Note
All claims expressed in this article are solely those of the authors and do not necessarily represent those of their affiliated organizations, or those of the publisher, the editors, and the reviewers. Any product that may be evaluated in this article, or claim that may be made by its manufacturer, is not guaranteed or endorsed by the publisher.
Abbreviations
AD, atopic dermatitis; DC, dendritic cell; FLG, filaggrin; IFN-γ, interferon gamma; IgE, immunoglobulin E; IL, interleukin; ILC2, type 2 innate lymphoid cells; LC, langerhans cells; S. aureus, Staphylococcus aureus; TH, T helper; TNF-α, tumor necrosis factor alpha; TSLP, thymic stromal lymphopoietin; KC, keratinocytes; A20, zinc finger protein A20; AD, atopic dermatitis; AGE, advanced glycation end products; AMPK, AMP-activated protein kinase; FLG, filaggrin; LOR, loricrin; HDAC, histone deacetylase; HSP27, hot shock protein 27; IL, interleukin; KC, keratinocytes; LC, langerhans cells; MAPK, mitogen-activated protein kinases; PGM3, phosphoglucomutase3; PIAS1, protein inhibitor of activated STAT1; RAGE, receptor for AGEs; S. aureus, Staphylococcus aureus; SIRT1, sirtuin1; STAT, signal transducer and activator of transcription; SUMO1, small ubiquitin like modifier 1; TH2, T helper 2 cells; TMEM79, transmembrane protein 79; TSLP, thymic stromal lymphopoietin; PTM, post-translational modifications.
References
Acevedo, N., Benfeitas, R., Katayama, S., Bruhn, S., Andersson, A., Wikberg, G., et al. (2020). Epigenetic Alterations in Skin Homing CD4+CLA+ T Cells of Atopic Dermatitis Patients. Sci. Rep. 10, 18020. doi:10.1038/s41598-020-74798-z
Agrawal, P., Fontanals-Cirera, B., Sokolova, E., Jacob, S., Vaiana, C. A., Argibay, D., et al. (2017). A Systems Biology Approach Identifies FUT8 as a Driver of Melanoma Metastasis. Cancer Cell 31, 804–819. e7. doi:10.1016/j.ccell.2017.05.007
Agrawal, R., Wisniewski, J. A., and Woodfolk, J. A. (2011). “The Role of Regulatory T Cells in Atopic Dermatitis,” in Current Problems in Dermatology. Editor T. Shiohara (Basel: Karger), 112–124. doi:10.1159/000323305
Alashkar Alhamwe, B., Alhamdan, F., Ruhl, A., Potaczek, D. P., and Renz, H. (2020). The Role of Epigenetics in Allergy and Asthma Development. Curr. Opin. Allergy & Clin. Immunol. 20, 48–55. doi:10.1097/aci.0000000000000598
Alaskhar Alhamwe, B., Khalaila, R., Wolf, J., von Bülow, V., Harb, H., Alhamdan, F., et al. (2018). Histone Modifications and Their Role in Epigenetics of Atopy and Allergic Diseases. Allergy Asthma Clin. Immunol. 14, 39. doi:10.1186/s13223-018-0259-4
Albor, A., El-Hizawi, S., Horn, E. J., Laederich, M., Frosk, P., Wrogemann, K., et al. (2006). The Interaction of Piasy with Trim32, an E3-Ubiquitin Ligase Mutated in Limb-Girdle Muscular Dystrophy Type 2H, Promotes Piasy Degradation and Regulates UVB-Induced Keratinocyte Apoptosis through NFκB. J. Biol. Chem. 281, 25850–25866. doi:10.1074/jbc.M601655200
Almeida, S., Ryser, S., Obarzanek-Fojt, M., Hohl, D., and Huber, M. (2011). The TRAF-Interacting Protein (TRIP) Is a Regulator of Keratinocyte Proliferation. J. Investigative Dermatology 131, 349–357. doi:10.1038/jid.2010.329
Banerjee, A., Trivedi, C. M., Damera, G., Jiang, M., Jester, W., Hoshi, T., et al. (2012). Trichostatin A Abrogates Airway Constriction, but Not Inflammation, in Murine and Human Asthma Models. Am. J. Respir. Cell Mol. Biol. 46, 132–138. doi:10.1165/rcmb.2010-0276oc
Banerjee Mustafi, S., Chakraborty, P. K., Dwivedi, S. K. D., Ding, K., Moxley, K. M., Mukherjee, P., et al. (2017). BMI1, a New Target of CK2α. Mol. Cancer 16, 56. doi:10.1186/s12943-017-0617-8
Barbarot, S., Auziere, S., Gadkari, A., Girolomoni, G., Puig, L., Simpson, E. L., et al. (2018). Epidemiology of Atopic Dermatitis in Adults: Results from an International Survey. Allergy 73, 1284–1293. doi:10.1111/all.13401
Biniek, K., Levi, K., and Dauskardt, R. H. (2012). Solar UV Radiation Reduces the Barrier Function of Human Skin. Proc. Natl. Acad. Sci. U.S.A. 109, 17111–17116. doi:10.1073/pnas.1206851109
Blauvelt, A., Teixeira, H. D., Simpson, E. L., Costanzo, A., De Bruin-Weller, M., Barbarot, S., et al. (2021). Efficacy and Safety of Upadacitinib vs Dupilumab in Adults with Moderate-To-Severe Atopic Dermatitis. JAMA Dermatol 157, 1047. doi:10.1001/jamadermatol.2021.3023
Botros, N., Sluik, D., van Waateringe, R. P., de Vries, J. H. M., Geelen, A., and Feskens, E. J. M. (2017). Advanced Glycation End-Products (AGEs) and Associations with Cardio-Metabolic, Lifestyle, and Dietary Factors in a General Population: the NQplus Study. Diabetes Metab. Res. Rev. 33, e2892. doi:10.1002/dmrr.2892
Boyault, C., Gilquin, B., Zhang, Y., Rybin, V., Garman, E., Meyer-Klaucke, W., et al. (2006). HDAC6-p97/VCP Controlled Polyubiquitin Chain Turnover. EMBO J. 25, 3357–3366. doi:10.1038/sj.emboj.7601210
Buerger, C., Shirsath, N., Lang, V., Berard, A., Diehl, S., Kaufmann, R., et al. (2017). Inflammation Dependent mTORC1 Signaling Interferes with the Switch from Keratinocyte Proliferation to Differentiation. PLoS ONE 12, e0180853. doi:10.1371/journal.pone.0180853
Callard, R. E., and Harper, J. I. (2007). The Skin Barrier, Atopic Dermatitis and Allergy: a Role for Langerhans Cells? Trends Immunol. 28, 294–298. doi:10.1016/j.it.2007.05.003
Castellanos, C. A., Ren, X., Gonzalez, S. L., Li, H. K., Schroeder, A. W., Liang, H.-E., et al. (2021). Lymph Node-Resident Dendritic Cells Drive T H 2 Cell Development Involving MARCH1. Sci. Immunol. 6, eabh0707. doi:10.1126/sciimmunol.abh0707
Cedeno-Laurent, F., Singer, E. M., Wysocka, M., Benoit, B. M., Vittorio, C. C., Kim, E. J., et al. (2015). Improved Pruritus Correlates with Lower Levels of IL-31 in CTCL Patients under Different Therapeutic Modalities. Clin. Immunol. 158, 1–7. doi:10.1016/j.clim.2015.02.014
Cha, K.-J., Kashif, A., Hong, M. H., Kim, G., Lee, J.-S., and Kim, I. S. (2019a). Poncirus Trifoliata (L.) Raf. Extract Inhibits the Development of Atopic Dermatitis-like Lesions in Human Keratinocytes and NC/Nga Mice. Int. J. Med. Sci. 16, 1116–1122. doi:10.7150/ijms.34323
Cha, K.-J., Song, C.-S., Lee, J.-S., Kashif, A., Hong, M. H., Kim, G., et al. (2019b). Chaenomeles Sinensis Koehne Extract Suppresses the Development of Atopic Dermatitis-like Lesions by Regulating Cytokine and Filaggrin Expression in NC/Nga Mice. Int. J. Med. Sci. 16, 1604–1613. doi:10.7150/ijms.37854
Chen, L., Grabowski, K. A., Xin, J.-p., Coleman, J., Huang, Z., Espiritu, B., et al. (2004). IL-4 Induces Differentiation and Expansion of Th2 Cytokine-Producing Eosinophils. J. Immunol. 172, 2059–2066. doi:10.4049/jimmunol.172.4.2059
Chen, M., Amado, N., Tan, J., Reis, A., Ge, M., Abreu, J. G., et al. (2020). TMEM79/MATTRIN Defines a Pathway for Frizzled Regulation and Is Required for Xenopus Embryogenesis. eLife 9, e56793. doi:10.7554/elife.56793
Choi, J. H., Lee, G. H., Jin, S. W., Kim, J. Y., Hwang, Y. P., Han, E. H., et al. (2021). Impressic Acid Ameliorates Atopic Dermatitis-like Skin Lesions by Inhibiting ERK1/2-Mediated Phosphorylation of NF-Κb and STAT1. IJMS 22, 2334. doi:10.3390/ijms22052334
Choi, Y. Y., Kim, M. H., Lee, H., Jo, S. Y., and Yang, W. M. (2018). (R)-(+)-pulegone Suppresses Allergic and Inflammation Responses on 2,4-Dinitrochlorobenzene-Induced Atopic Dermatitis in Mice Model. J. Dermatological Sci. 91, 292–300. doi:10.1016/j.jdermsci.2018.06.002
Chung, Y.-L., and Pui, N. N. M. (2011). Phenylbutyrate Suppresses Distinct Skin Reactions that Are Enhanced by Blockade of Epidermal Growth Factor Receptor Signaling. J. Dermatological Sci. 64, 163–173. doi:10.1016/j.jdermsci.2011.08.007
Ciechanover, A. (2015). The Unravelling of the Ubiquitin System. Nat. Rev. Mol. Cell Biol. 16, 322–324. doi:10.1038/nrm3982
Collison, A., Hatchwell, L., Verrills, N., Wark, P. A. B., de Siqueira, A. P., Tooze, M., et al. (2013). The E3 Ubiquitin Ligase Midline 1 Promotes Allergen and Rhinovirus-Induced Asthma by Inhibiting Protein Phosphatase 2A Activity. Nat. Med. 19, 232–237. doi:10.1038/nm.3049
Crane, E. D., Wong, W., Zhang, H., O’Neil, G., and Crane, J. D. (2021). AMPK Inhibits mTOR-Driven Keratinocyte Proliferation after Skin Damage and Stress. J. Investigative Dermatology 141, 2170–2177. e3. doi:10.1016/j.jid.2020.12.036
Cury Martins, J., Martins, C., Aoki, V., Gois, A. F., Ishii, H. A., and da Silva, E. M. (2015). Topical Tacrolimus for Atopic Dermatitis. Cochrane Database Syst. Rev. 2015 (7), CD009864. doi:10.1002/14651858.CD009864.pub2
Davie, J. R. (2003). Inhibition of Histone Deacetylase Activity by Butyrate. J. Nutr. 133, 2485S–2493S. doi:10.1093/jn/133.7.2485s
Devos, M., Mogilenko, D. A., Fleury, S., Gilbert, B., Becquart, C., Quemener, S., et al. (2019). Keratinocyte Expression of A20/TNFAIP3 Controls Skin Inflammation Associated with Atopic Dermatitis and Psoriasis. J. Investigative Dermatology 139, 135–145. doi:10.1016/j.jid.2018.06.191
Ding, X., Willenborg, S., Bloch, W., Wickström, S. A., Wagle, P., Brodesser, S., et al. (2020). Epidermal Mammalian Target of Rapamycin Complex 2 Controls Lipid Synthesis and Filaggrin Processing in Epidermal Barrier Formation. J. Allergy Clin. Immunol. 145, 283–300. e8. doi:10.1016/j.jaci.2019.07.033
Dong, J., Segawa, R., Mizuno, N., Hiratsuka, M., and Hirasawa, N. (2016). Inhibitory Effects of Nicotine Derived from Cigarette Smoke on Thymic Stromal Lymphopoietin Production in Epidermal Keratinocytes. Cell. Immunol. 302, 19–25. doi:10.1016/j.cellimm.2016.01.001
Dong, L., Du, H., Zhang, M., Xu, H., Pu, X., Chen, Q., et al. (2022). Anti‐inflammatory Effect of Rhein on Ulcerative Colitis via Inhibiting PI3K /Akt/mTOR Signaling Pathway and Regulating Gut Microbiota. Phytotherapy Res. 36, 2081–2094. doi:10.1002/ptr.7429
Dumitriu, I. E., Baruah, P., Valentinis, B., Voll, R. E., Herrmann, M., Nawroth, P. P., et al. (2005). Release of High Mobility Group Box 1 by Dendritic Cells Controls T Cell Activation via the Receptor for Advanced Glycation End Products. J. Immunol. 174, 7506–7515. doi:10.4049/jimmunol.174.12.7506
Fang, D., Elly, C., Gao, B., Fang, N., Altman, Y., Joazeiro, C., et al. (2002). Dysregulation of T Lymphocyte Function in Itchy Mice: a Role for Itch in TH2 Differentiation. Nat. Immunol. 3, 281–287. doi:10.1038/ni763
Feketea, G., and Tsabouri, S. (2017). Common Food Colorants and Allergic Reactions in Children: Myth or Reality? Food Chem. 230, 578–588. doi:10.1016/j.foodchem.2017.03.043
Feligioni, M., Nishimune, A., and Henley, J. M. (2009). Protein SUMOylation Modulates Calcium Influx and Glutamate Release from Presynaptic Terminals. Eur. J. Neurosci. 29, 1348–1356. doi:10.1111/j.1460-9568.2009.06692.x
Feng, Y., Madungwe, N. B., da Cruz Junho, C. V., and Bopassa, J. C. (2017). Activation of G Protein-Coupled Oestrogen Receptor 1 at the Onset of Reperfusion Protects the Myocardium against Ischemia/reperfusion Injury by Reducing Mitochondrial Dysfunction and Mitophagy. Br. J. Pharmacol. 174, 4329–4344. doi:10.1111/bph.14033
Flotho, A., and Melchior, F. (2013). Sumoylation: A Regulatory Protein Modification in Health and Disease. Annu. Rev. Biochem. 82, 357–385. doi:10.1146/annurev-biochem-061909-093311
Fujita, H., Inoue, Y., Seto, K., Komitsu, N., and Aihara, M. (2013). Interleukin-37 Is Elevated in Subjects with Atopic Dermatitis. J. Dermatological Sci. 69, 173–175. doi:10.1016/j.jdermsci.2012.11.001
Furue, M., and Furue, M. (2021). OX40L-OX40 Signaling in Atopic DermatitisOX40L–OX40 Signaling in Atopic Dermatitis. Jcm 10, 2578. doi:10.3390/jcm10122578
G'Sell, R. T., Gaffney, P. M., and Powell, D. W. (2015). Review: A20-Binding Inhibitor of NF-Κb Activation 1 Is a Physiologic Inhibitor of NF-Κb: A Molecular Switch for Inflammation and Autoimmunity. Arthritis & Rheumatology 67, 2292–2302. doi:10.1002/art.39245
Galindo-Moreno, M., Giráldez, S., Sáez, C., Japón, M. Á., Tortolero, M., and Romero, F. (2017). Both p62/SQSTM1-HDAC6-dependent Autophagy and the Aggresome Pathway Mediate CDK1 Degradation in Human Breast Cancer. Sci. Rep. 7, 10078. doi:10.1038/s41598-017-10506-8
Gandhi, N. A., Bennett, B. L., Graham, N. M. H., Pirozzi, G., Stahl, N., and Yancopoulos, G. D. (2016). Targeting Key Proximal Drivers of Type 2 Inflammation in Disease. Nat. Rev. Drug Discov. 15, 35–50. doi:10.1038/nrd4624
Garcin, G., Guiraud, I., Lacroix, M., Genthon, C., Rialle, S., Joujoux, J.-M., et al. (2015). AMPK/HuR-Driven IL-20 Post-Transcriptional Regulation in Psoriatic Skin. J. Investigative Dermatology 135, 2732–2741. doi:10.1038/jid.2015.282
Giansanti, P., Strating, J. R. P. M., Defourny, K. A. Y., Cesonyte, I., Bottino, A. M. S., Post, H., et al. (2020). Dynamic Remodelling of the Human Host Cell Proteome and Phosphoproteome upon Enterovirus Infection. Nat. Commun. 11, 4332. doi:10.1038/s41467-020-18168-3
Giridharan, S., and Srinivasan, M. (2018). Mechanisms of NF-Κb P65 and Strategies for Therapeutic Manipulation. JIR V11, 407–419.
Grewe, M., Bruijnzeel-Koomen, C. A. F. M., Schöpf, E., Thepen, T., Langeveld-Wildschut, A. G., Ruzicka, T., et al. (1998). A Role for Th1 and Th2 Cells in the Immunopathogenesis of Atopic Dermatitis. Immunol. Today 19, 359–361. doi:10.1016/S0167-5699(98)01285-7
Guttman-Yassky, E., Suárez-Fariñas, M., Chiricozzi, A., Nograles, K. E., Shemer, A., Fuentes-Duculan, J., et al. (2009). Broad Defects in Epidermal Cornification in Atopic Dermatitis Identified through Genomic Analysis. J. Allergy Clin. Immunol. 124, 1235–1244. e58. doi:10.1016/j.jaci.2009.09.031
Hönzke, S., Wallmeyer, L., Ostrowski, A., Radbruch, M., Mundhenk, L., Schäfer-Korting, M., et al. (2016). Influence of Th2 Cytokines on the Cornified Envelope, Tight Junction Proteins, and β-Defensins in Filaggrin-Deficient Skin Equivalents. J. Investigative Dermatology 136, 631–639. doi:10.1016/j.jid.2015.11.007
Habibian, J., and Ferguson, B. (2018). The Crosstalk between Acetylation and Phosphorylation: Emerging New Roles for HDAC Inhibitors in the Heart. IJMS 20, 102. doi:10.3390/ijms20010102
Hanafy, B. I., Cave, G. W. V., Barnett, Y., and Pierscionek, B. K. (2021). Nanoceria Prevents Glucose-Induced Protein Glycation in Eye Lens Cells. Nanomaterials 11, 1473. doi:10.3390/nano11061473
Harirchian, P., Lee, J., Hilz, S., Sedgewick, A. J., Perez White, B. E., Kesling, M. J., et al. (2019). A20 and ABIN1 Suppression of a Keratinocyte Inflammatory Program with a Shared Single-Cell Expression Signature in Diverse Human Rashes. J. Investigative Dermatology 139, 1264–1273. doi:10.1016/j.jid.2018.10.046
Hong, C.-H., Chang, K.-L., Wang, H.-J., Yu, H.-S., and Lee, C.-H. (2015). IL-9 Induces IL-8 Production via STIM1 Activation and ERK Phosphorylation in Epidermal Keratinocytes: A Plausible Mechanism of IL-9R in Atopic Dermatitis. J. Dermatological Sci. 78, 206–214. doi:10.1016/j.jdermsci.2015.03.004
Hong, C.-H., Lee, C.-H., Yu, H.-S., and Huang, S.-K. (2016). Benzopyrene, a Major Polyaromatic Hydrocarbon in Smoke Fume, Mobilizes Langerhans Cells and Polarizes Th2/17 Responses in Epicutaneous Protein Sensitization through the Aryl Hydrocarbon Receptor. Int. Immunopharmacol. 36, 111–117. doi:10.1016/j.intimp.2016.04.017
Hong, J. Y., Kim, M. J., Hong, J. K., Noh, H. H., Park, K. Y., Lee, M. K., et al. (2020). In Vivo quantitative Analysis of Advanced Glycation End Products in Atopic Dermatitis-Possible Culprit for the Comorbidities? Exp. Dermatol 29, 1012–1016. doi:10.1111/exd.14167
Hoste, E., Lecomte, K., Annusver, K., Vandamme, N., Roels, J., Maschalidi, S., et al. (2021). OTULIN Maintains Skin Homeostasis by Controlling Keratinocyte Death and Stem Cell Identity. Nat. Commun. 12, 5913. doi:10.1038/s41467-021-25944-2
Hou, T., Sun, X., Zhu, J., Hon, K.-L., Jiang, P., Chu, I. M.-T., et al. (2020). IL-37 Ameliorating Allergic Inflammation in Atopic Dermatitis through Regulating Microbiota and AMPK-mTOR Signaling Pathway-Modulated Autophagy Mechanism. Front. Immunol. 11, 752. doi:10.3389/fimmu.2020.00752
Howell, M. D., Gallo, R. L., Boguniewicz, M., Jones, J. F., Wong, C., Streib, J. E., et al. (2006). Cytokine Milieu of Atopic Dermatitis Skin Subverts the Innate Immune Response to Vaccinia Virus. Immunity 24, 341–348. doi:10.1016/j.immuni.2006.02.006
Hu, X. Q., Tang, Y., Ju, Y., Zhang, X. Y., Yan, J. J., Wang, C. M., et al. (2021). Scratching Damages Tight Junctions through the Akt-Claudin 1 axis in Atopic Dermatitis. Clin. Exp. Dermatol. 46, 74–81. doi:10.1111/ced.14380
Huang, X., Liu, G., Guo, J., and Su, Z. (2018). The PI3K/AKT Pathway in Obesity and Type 2 Diabetes. Int. J. Biol. Sci. 14, 1483–1496. doi:10.7150/ijbs.27173
Incani, F., Serra, M. L., Meloni, A., Cossu, C., Saba, L., Cabras, T., et al. (2014). AIRE Acetylation and Deacetylation: Effect on Protein Stability and Transactivation Activity. J. Biomed. Sci. 21, 85. doi:10.1186/s12929-014-0085-z
Ishikawa, J., Narita, H., Kondo, N., Hotta, M., Takagi, Y., Masukawa, Y., et al. (2010). Changes in the Ceramide Profile of Atopic Dermatitis Patients. J. Investigative Dermatology 130, 2511–2514. doi:10.1038/jid.2010.161
Jackson, B., Peyrollier, K., Pedersen, E., Basse, A., Karlsson, R., Wang, Z., et al. (2011). RhoA Is Dispensable for Skin Development, but Crucial for Contraction and Directed Migration of Keratinocytes. MBoC 22, 593–605. doi:10.1091/mbc.e09-10-0859
Jang, Y., Jeong, S. H., Park, Y.-H., Bae, H. C., Lee, H., Ryu, W.-I., et al. (2013). UVB Induces HIF-1α-dependent TSLP Expression via the JNK and ERK Pathways. J. Investigative Dermatology 133, 2601–2608. doi:10.1038/jid.2013.203
Janssens, M., van Smeden, J., Gooris, G. S., Bras, W., Portale, G., Caspers, P. J., et al. (2012). Increase in Short-Chain Ceramides Correlates with an Altered Lipid Organization and Decreased Barrier Function in Atopic Eczema Patients. J. Lipid Res. 53, 2755–2766. doi:10.1194/jlr.P030338
Jia, Q.-N., and Zeng, Y.-P. (2020). Rapamycin Blocks the IL-13-induced Deficiency of Epidermal Barrier Related Proteins via Upregulation of miR-143 in HaCaT Keratinocytes. Int. J. Med. Sci. 17, 2087–2094. doi:10.7150/ijms.45765
Jonak, C., Mildner, M., Klosner, G., Paulitschke, V., Kunstfeld, R., Pehamberger, H., et al. (2011). The hsp27kD Heat Shock Protein and P38-MAPK Signaling Are Required for Regular Epidermal Differentiation. J. Dermatological Sci. 61, 32–37. doi:10.1016/j.jdermsci.2010.10.009
Jungersted, J. M., Scheer, H., Mempel, M., Baurecht, H., Cifuentes, L., Høgh, J. K., et al. (2010). Stratum Corneum Lipids, Skin Barrier Function and Filaggrin Mutations in Patients with Atopic Eczema. Allergy 65, 911–918. doi:10.1111/j.1398-9995.2010.02326.x
Kanemaru, K., Nakamura, Y., Totoki, K., Fukuyama, T., Shoji, M., Kaneko, H., et al. (2017). Phospholipase Cδ1 Regulates P38 MAPK Activity and Skin Barrier Integrity. Cell Death Differ. 24, 1079–1090. doi:10.1038/cdd.2017.56
Karuppagounder, V., Arumugam, S., Thandavarayan, R. A., Pitchaimani, V., Sreedhar, R., Afrin, R., et al. (2015). Modulation of HMGB1 Translocation and RAGE/NFκB Cascade by Quercetin Treatment Mitigates Atopic Dermatitis in NC/Nga Transgenic Mice. Exp. Dermatol 24, 418–423. doi:10.1111/exd.12685
Katagata, Y. (2011). Hsp40 Regulates the Amount of Keratin Proteins via Ubiquitin-Proteasome Pathway in Cultured Human Cells. Int. J. Mol. Med. 29 (2), 165–168. doi:10.3892/ijmm.2011.826
Kauppinen, A., Suuronen, T., Ojala, J., Kaarniranta, K., and Salminen, A. (2013). Antagonistic Crosstalk between NF-Κb and SIRT1 in the Regulation of Inflammation and Metabolic Disorders. Cell. Signal. 25, 1939–1948. doi:10.1016/j.cellsig.2013.06.007
Kim, B. E., Howell, M. D., Guttman, E., Gilleaudeau, P. M., Cardinale, I. R., Boguniewicz, M., et al. (2011). TNF-Α Downregulates Filaggrin and Loricrin through C-Jun N-Terminal Kinase: Role for TNF-α Antagonists to Improve Skin Barrier. J. Investigative Dermatology 131, 1272–1279. doi:10.1038/jid.2011.24
Kim, B. S., Wang, K., Siracusa, M. C., Saenz, S. A., Brestoff, J. R., Monticelli, L. A., et al. (2014). Basophils Promote Innate Lymphoid Cell Responses in Inflamed Skin. J. I. 193, 3717–3725. doi:10.4049/jimmunol.1401307
Kim, J., Kim, J., Cho, D., and Park, H. (2016). Molecular Mechanisms of Cutaneous Inflammatory Disorder: Atopic Dermatitis. IJMS 17, 1234. doi:10.3390/ijms17081234
Kim, T.-H., Jung, J.-A., Kim, G.-D., Jang, A.-H., Cho, J.-J., Park, Y. S., et al. (2010). The Histone Deacetylase Inhibitor, Trichostatin A, Inhibits the Development of 2,4-Dinitrofluorobenzene-Induced Dermatitis in NC/Nga Mice. Int. Immunopharmacol. 10, 1310–1315. doi:10.1016/j.intimp.2010.08.004
Kim, Y., Kim, K., Park, D., Lee, E., Lee, H., Lee, Y.-S., et al. (2012). Histone Deacetylase 3 Mediates Allergic Skin Inflammation by Regulating Expression of MCP1 Protein. J. Biol. Chem. 287, 25844–25859. doi:10.1074/jbc.m112.348284
Kishta, O. A., Sabourin, A., Simon, L., McGovern, T., Raymond, M., Galbas, T., et al. (20182018). March1 E3 Ubiquitin Ligase Modulates Features of Allergic Asthma in an Ovalbumin-Induced Mouse Model of Lung Inflammation. J. Immunol. Res. 2018, 1–17. doi:10.1155/2018/3823910
Koury, J., Ramirez, A., Xie, C., Harb, J., Dong, C., Maki, C., et al. (2019). Phosphodiesterase 4D, miR-203 and Selected Cytokines in the Peripheral Blood Are Associated with Canine Atopic Dermatitis. PLoS ONE 14, e0218670. doi:10.1371/journal.pone.0218670
Krämer, O. H., Knauer, S. K., Greiner, G., Jandt, E., Reichardt, S., Gührs, K.-H., et al. (2009). A Phosphorylation-Acetylation Switch Regulates STAT1 Signaling. Genes Dev. 23, 223–235. doi:10.1101/gad.479209
Krebs, E. G., and Beavo, J. A. (1979). Phosphorylation-Dephosphorylation of Enzymes. Annu. Rev. Biochem. 48, 923–959. doi:10.1146/annurev.bi.48.070179.004423
Kudryashova, E., Kramerova, I., and Spencer, M. J. (2012). Satellite Cell Senescence Underlies Myopathy in a Mouse Model of Limb-Girdle Muscular Dystrophy 2H. J. Clin. Invest. 122, 1764–1776. doi:10.1172/JCI59581
Kwon, Y., Choi, Y., Kim, M., Jeong, M. S., Jung, H. S., and Jeoung, D. (2021). HDAC6 and CXCL13 Mediate Atopic Dermatitis by Regulating Cellular Interactions and Expression Levels of miR-9 and SIRT1. Front. Pharmacol. 12, 691279. doi:10.3389/fphar.2021.691279
Kwon, Y. T., and Ciechanover, A. (2017). The Ubiquitin Code in the Ubiquitin-Proteasome System and Autophagy. Trends Biochem. Sci. 42, 873–886. doi:10.1016/j.tibs.2017.09.002
Löffek, S., Wöll, S., Höhfeld, J., Leube, R. E., Has, C., Bruckner-Tuderman, L., et al. (2010). The Ubiquitin Ligase CHIP/STUB1 Targets Mutant Keratins for Degradation. Hum. Mutat. 31, 466–476. doi:10.1002/humu.21222
Laarse, S. A. M., Leney, A. C., and Heck, A. J. R. (2018). Crosstalk between Phosphorylation and O‐Glc NA Cylation: Friend or Foe. FEBS J. 285, 3152–3167. doi:10.1111/febs.14491
Langan, S. M., Irvine, A. D., and Weidinger, S. (2020). Atopic Dermatitis. Lancet 396, 345–360. doi:10.1016/S0140-6736(20)31286-1
Laplante, M., and Sabatini, D. M. (2012). mTOR Signaling in Growth Control and Disease. Cell 149, 274–293. doi:10.1016/j.cell.2012.03.017
Laughter, M. R., Maymone, M. B. C., Mashayekhi, S., Arents, B. W. M., Karimkhani, C., Langan, S. M., et al. (2021). The Global Burden of Atopic Dermatitis: Lessons from the Global Burden of Disease Study 1990-2017*. Br. J. Dermatol 184, 304–309. doi:10.1111/bjd.19580
Lazarski, C. A., Ford, J., Katzman, S. D., Rosenberg, A. F., and Fowell, D. J. (2013). IL-4 Attenuates Th1-Associated Chemokine Expression and Th1 Trafficking to Inflamed Tissues and Limits Pathogen Clearance. PLoS ONE 8, e71949. doi:10.1371/journal.pone.0071949
Leclair, H. M., André-Grégoire, G., Treps, L., Azzi, S., Bidère, N., and Gavard, J. (2016). The E3 Ubiquitin Ligase MARCH3 Controls the Endothelial Barrier. FEBS Lett. 590, 3660–3668. doi:10.1002/1873-3468.12417
Lee, C.-H., Hong, C.-H., Yu, W.-T., Chuang, H.-Y., Huang, S.-K., Chen, G.-S., et al. (2012). Mechanistic Correlations between Two Itch Biomarkers, Cytokine Interleukin-31 and Neuropeptide β-endorphin, via STAT3/calcium axis in Atopic Dermatitis. Br. J. Dermatology 167, 794–803. doi:10.1111/j.1365-2133.2012.11047.x
Lee, J.-H., Kim, J.-S., Park, S.-Y., and Lee, Y.-J. (2016). Resveratrol Induces Human Keratinocyte Damage via the Activation of Class III Histone Deacetylase, Sirt1. Oncol. Rep. 35, 524–529. doi:10.3892/or.2015.4332
Leon Carrion, S., Sutter, C. H., and Sutter, T. R. (2014). Combined Treatment with Sodium Butyrate and PD153035 Enhances Keratinocyte Differentiation. Exp. Dermatol 23, 211–214. doi:10.1111/exd.12333
Li, K., Wang, R., Lozada, E., Fan, W., Orren, D. K., and Luo, J. (2010). Acetylation of WRN Protein Regulates its Stability by Inhibiting Ubiquitination. PLoS ONE 5, e10341. doi:10.1371/journal.pone.0010341
Liew, W. C., Sundaram, G. M., Quah, S., Lum, G. G., Tan, J. S. L., Ramalingam, R., et al. (2020). Belinostat Resolves Skin Barrier Defects in Atopic Dermatitis by Targeting the Dysregulated miR-335:SOX6 axis. J. Allergy Clin. Immunol. 146, 606–620. e12. doi:10.1016/j.jaci.2020.02.007
Lim, X., Tan, S. H., Koh, W. L. C., Chau, R. M. W., Yan, K. S., Kuo, C. J., et al. (2013). Interfollicular Epidermal Stem Cells Self-Renew via Autocrine Wnt Signaling. Science 342, 1226–1230. doi:10.1126/science.1239730
Lin, H.-K. (2002). Phosphorylation-dependent Ubiquitylation and Degradation of Androgen Receptor by Akt Require Mdm2 E3 Ligase. EMBO J. 21, 4037–4048. doi:10.1093/emboj/cdf406
Liu, M., Li, C.-M., Chen, Z.-F., Ji, R., Guo, Q.-H., Li, Q., et al. (2014). Celecoxib Regulates Apoptosis and Autophagy via the PI3K/Akt Signaling Pathway in SGC-7901 Gastric Cancer Cells. Int. J. Mol. Med. 33, 1451–1458. doi:10.3892/ijmm.2014.1713
Liu, Q., Zhang, S., Sun, Z., Guo, X., and Zhou, H. (2019). E3 Ubiquitin Ligase Nedd4 Is a Key Negative Regulator for Non-canonical Inflammasome Activation. Cell Death Differ. 26, 2386–2399. doi:10.1038/s41418-019-0308-7
Liu, Y., Lagowski, J. P., Gao, S., Raymond, J. H., White, C. R., and Kulesz-Martin, M. F. (2010). Regulation of the Psoriatic Chemokine CCL20 by E3 Ligases Trim32 and Piasy in Keratinocytes. J. Investigative Dermatology 130, 1384–1390. doi:10.1038/jid.2009.416
Liu, Y., Wang, Z., De La Torre, R., Barling, A., Tsujikawa, T., Hornick, N., et al. (2017). Trim32 Deficiency Enhances Th2 Immunity and Predisposes to Features of Atopic Dermatitis. J. Investigative Dermatology 137, 359–366. doi:10.1016/j.jid.2016.09.020
Loeser, S., and Penninger, J. M. (2007a). The Ubiquitin E3 Ligase Cbl-B in T Cells Tolerance and Tumor Immunity. Cell Cycle 6, 2478–2485. doi:10.4161/cc.6.20.4797
Loeser, S., and Penninger, J. M. (2007b). The Ubiquitin E3 Ligase Cbl-B in T Cells Tolerance and Tumor Immunity. Cell Cycle 6, 2478–2485. doi:10.4161/cc.6.20.4797
Lou, H., Lu, J., Choi, E. B., Oh, M. H., Jeong, M., Barmettler, S., et al. (2017). Expression of IL-22 in the Skin Causes Th2-Biased Immunity, Epidermal Barrier Dysfunction, and Pruritus via Stimulating Epithelial Th2 Cytokines and the GRP Pathway. J. I. 198, 2543–2555. doi:10.4049/jimmunol.1600126
Ma, L., Xue, H.-B., Guan, X.-H., Shu, C.-M., Wang, F., Zhang, J.-H., et al. (2014). The Imbalance of Th17 Cells and CD4+ CD25high Foxp3+ Treg Cells in Patients with Atopic Dermatitis. J. Eur. Acad. Dermatol Venereol. 28, 1079–1086. doi:10.1111/jdv.12288
Madonna, S., Scarponi, C., Pallotta, S., Cavani, A., and Albanesi, C. (2012). Anti-apoptotic Effects of Suppressor of Cytokine Signaling 3 and 1 in Psoriasis. Cell Death Dis. 3, e334. doi:10.1038/cddis.2012.69
Maggi, L., Montaini, G., Mazzoni, A., Rossettini, B., Capone, M., Rossi, M. C., et al. (2017). Human Circulating Group 2 Innate Lymphoid Cells Can Express CD154 and Promote IgE Production. J. Allergy Clin. Immunol. 139, 964–976. e4. doi:10.1016/j.jaci.2016.06.032
Majewska, A., Gajewska, M., Dembele, K., Maciejewski, H., Prostek, A., and Jank, M. (2016). Lymphocytic, Cytokine and Transcriptomic Profiles in Peripheral Blood of Dogs with Atopic Dermatitis. BMC Vet. Res. 12, 174. doi:10.1186/s12917-016-0805-6
Margolis, D. J., Gupta, J., Apter, A. J., Hoffstad, O., Papadopoulos, M., Rebbeck, T. R., et al. (2014). Exome Sequencing of Filaggrin and Related Genes in African-American Children with Atopic Dermatitis. J. Investigative Dermatology 134, 2272–2274. doi:10.1038/jid.2014.126
Meephansan, J., Komine, M., Tsuda, H., Karakawa, M., Tominaga, S.-i., and Ohtsuki, M. (2013). Expression of IL-33 in the Epidermis: The Mechanism of Induction by IL-17. J. Dermatological Sci. 71, 107–114. doi:10.1016/j.jdermsci.2013.04.014
Mercurio, L., Albanesi, C., and Madonna, S. (2021). Recent Updates on the Involvement of PI3K/AKT/mTOR Molecular Cascade in the Pathogenesis of Hyperproliferative Skin Disorders. Front. Med. 8, 665647. doi:10.3389/fmed.2021.665647
Ming, M., Zhao, B., Shea, C. R., Shah, P., Qiang, L., White, S. R., et al. (2015). Loss of Sirtuin 1 (SIRT1) Disrupts Skin Barrier Integrity and Sensitizes Mice to Epicutaneous Allergen Challenge. J. Allergy Clin. Immunol. 135, 936–945. e4. doi:10.1016/j.jaci.2014.09.035
Mohapatra, B., Ahmad, G., Nadeau, S., Zutshi, N., An, W., Scheffe, S., et al. (2013a). Protein Tyrosine Kinase Regulation by Ubiquitination: Critical Roles of Cbl-Family Ubiquitin Ligases. Biochimica Biophysica Acta (BBA) - Mol. Cell Res. 1833, 122–139. doi:10.1016/j.bbamcr.2012.10.010
Mohapatra, B., Ahmad, G., Nadeau, S., Zutshi, N., An, W., Scheffe, S., et al. (2013b). Protein Tyrosine Kinase Regulation by Ubiquitination: Critical Roles of Cbl-Family Ubiquitin Ligases. Biochimica Biophysica Acta (BBA) - Mol. Cell Res. 1833, 122–139. doi:10.1016/j.bbamcr.2012.10.010
Mondal, S., Wang, S., Zheng, Y., Sen, S., Chatterjee, A., and Thompson, P. R. (2021). Site-specific Incorporation of Citrulline into Proteins in Mammalian Cells. Nat. Commun. 12, 45. doi:10.1038/s41467-020-20279-w
Mooney, E. C., and Sahingur, S. E. (2021). The Ubiquitin System and A20: Implications in Health and Disease. J. Dent. Res. 100, 10–20. doi:10.1177/0022034520949486
Moscat, J., and Diaz-Meco, M. T. (2009). p62 at the Crossroads of Autophagy, Apoptosis, and Cancer. Cell 137, 1001–1004. doi:10.1016/j.cell.2009.05.023
Murphy, K. M., and Reiner, S. L. (2002). The Lineage Decisions of Helper T Cells. Nat. Rev. Immunol. 2, 933–944. doi:10.1038/nri954
Naeem, A. S., Tommasi, C., Cole, C., Brown, S. J., Zhu, Y., Way, B., et al. (2017). A Mechanistic Target of Rapamycin Complex 1/2 (mTORC1)/V-Akt Murine Thymoma Viral Oncogene Homolog 1 (AKT1)/cathepsin H axis Controls Filaggrin Expression and Processing in Skin, a Novel Mechanism for Skin Barrier Disruption in Patients with Atopic Dermatitis. J. Allergy Clin. Immunol. 139, 1228–1241. doi:10.1016/j.jaci.2016.09.052
Nakatsuji, T., Chen, T. H., Two, A. M., Chun, K. A., Narala, S., Geha, R. S., et al. (2016). Staphylococcus aureus Exploits Epidermal Barrier Defects in Atopic Dermatitis to Trigger Cytokine Expression. J. Investigative Dermatology 136, 2192–2200. doi:10.1016/j.jid.2016.05.127
Nakatsuji, T., and Gallo, R. L. (2019). The Role of the Skin Microbiome in Atopic Dermatitis. Ann. Allergy, Asthma & Immunol. 122, 263–269. doi:10.1016/j.anai.2018.12.003
Narita, T., Weinert, B. T., and Choudhary, C. (2019). Functions and Mechanisms of Non-histone Protein Acetylation. Nat. Rev. Mol. Cell Biol. 20, 156–174. doi:10.1038/s41580-018-0081-3
Naruke, A., Nakano, R., Nunomura, J., Suwabe, Y., Nakano, M., Namba, S., et al. (2021). Tpl2 Contributes to IL-1β-induced IL-8 Expression via ERK1/2 Activation in Canine Dermal Fibroblasts. PLoS ONE 16, e0259489. doi:10.1371/journal.pone.0259489
Niiyama, S., Yoshino, T., Matsukuma, S., and Mukai, H. (2016). Heat Shock Protein 27 kDa Content in Stratum Corneum Correlates with Severity of Atopic Dermatitis. Acta Derm. Venerol. 96, 976–977. doi:10.2340/00015555-2436
Nitulescu, G., Van De Venter, M., Nitulescu, G., Ungurianu, A., Juzenas, P., Peng, Q., et al. (2018). The Akt Pathway in Oncology Therapy and beyond (Review). Int. J. Oncol. 53 (6), 2319–2331. doi:10.3892/ijo.2018.4597
Nograles, K. E., Zaba, L. C., Shemer, A., Fuentes-Duculan, J., Cardinale, I., Kikuchi, T., et al. (2009). IL-22-producing "T22" T Cells Account for Upregulated IL-22 in Atopic Dermatitis Despite Reduced IL-17-producing TH17 T Cells. J. Allergy Clin. Immunol. 123, 1244–1252. e2. doi:10.1016/j.jaci.2009.03.041
Noh, J. Y., Shin, J. U., Park, C. O., Lee, N., Jin, S., Kim, S. H., et al. (2016). Thymic Stromal Lymphopoietin Regulates Eosinophil Migration via Phosphorylation Ofl-Plastin in Atopic Dermatitis. Exp. Dermatol 25, 880–886. doi:10.1111/exd.13111
Novak, N. (2012). An Update on the Role of Human Dendritic Cells in Patients with Atopic Dermatitis. J. Allergy Clin. Immunol. 129, 879–886. doi:10.1016/j.jaci.2012.01.062
O'Shaughnessy, R. F. L., Welti, J. C., Cooke, J. C., Avilion, A. A., Monks, B., Birnbaum, M. J., et al. (2007). AKT-Dependent HspB1 (Hsp27) Activity in Epidermal Differentiation. J. Biol. Chem. 282, 17297–17305. doi:10.1074/jbc.M610386200
Ogura, M., Endo, K., Suzuki, T., and Homma, Y. (2021). Prenylated Quinolinecarboxylic Acid Compound-18 Prevents Sensory Nerve Fiber Outgrowth through Inhibition of the Interleukin-31 Pathway. PLoS ONE 16, e0246630. doi:10.1371/journal.pone.0246630
Oh, J., Wu, N., Baravalle, G., Cohn, B., Ma, J., Lo, B., et al. (2013). MARCH1-mediated MHCII Ubiquitination Promotes Dendritic Cell Selection of Natural Regulatory T Cells. J. Exp. Med. 210, 1069–1077. doi:10.1084/jem.20122695
O’Leary, C. E., Riling, C. R., Spruce, L. A., Ding, H., Kumar, S., Deng, G., et al. (2016). Ndfip-mediated Degradation of Jak1 Tunes Cytokine Signalling to Limit Expansion of CD4+ Effector T Cells. Nat. Commun. 7, 11226. doi:10.1038/ncomms11226
Pan, Y., Wen, X., Hao, D., Wang, Y., Wang, L., He, G., et al. (2020). The Role of IL-37 in Skin and Connective Tissue Diseases. Biomed. Pharmacother. 122, 109705. doi:10.1016/j.biopha.2019.109705
Papagrigoraki, A., Maurelli, M., del Giglio, M., Gisondi, P., and Girolomoni, G. (2017). Advanced Glycation End Products in the Pathogenesis of Psoriasis. IJMS 18, 2471. doi:10.3390/ijms18112471
Parisi, J., Corrêa, M., and Gil, C. (2019). Lack of Endogenous Annexin A1 Increases Mast Cell Activation and Exacerbates Experimental Atopic Dermatitis. Cells 8, 51. doi:10.3390/cells8010051
Park, J.-H., Ahn, E.-K., Ko, H.-J., Lee, J. Y., Hwang, S.-M., Ko, S., et al. (2019). Korean Red Ginseng Water Extract Alleviates Atopic Dermatitis-like Inflammatory Responses by Negative Regulation of Mitogen-Activated Protein Kinase Signaling Pathway In Vivo. Biomed. Pharmacother. 117, 109066. doi:10.1016/j.biopha.2019.109066
Park, J.-H., Kim, M.-S., Jeong, G.-S., and Yoon, J. (2015). Xanthii Fructus Extract Inhibits TNF-Α/ifn-γ-Induced Th2-Chemokines Production via Blockade of NF-Κb, STAT1 and P38-MAPK Activation in Human Epidermal Keratinocytes. J. Ethnopharmacol. 171, 85–93. doi:10.1016/j.jep.2015.05.039
Park, S. H., Lee, C. H., Lee, J. Y., Yang, H., Kim, J. H., Park, J. H. Y., et al. (2020). Topical Application of 7,3′,4′-Trihydroxyisoflavone Alleviates Atopic Dermatitis-like Symptoms in NC/Nga Mice. Planta Med. 86, 190–197. doi:10.1055/a-1068-7983
Patrick, G. J., Archer, N. K., and Miller, L. S. (2021). Which Way Do We Go? Complex Interactions in Atopic Dermatitis Pathogenesis. J. Investigative Dermatology 141, 274–284. doi:10.1016/j.jid.2020.07.006
Pazdrak, K., Young, T. W., Straub, C., Stafford, S., and Kurosky, A. (2011). Priming of Eosinophils by GM-CSF Is Mediated by Protein Kinase CβII-Phosphorylated L-Plastin. J. I. 186, 6485–6496. doi:10.4049/jimmunol.1001868
Perretti, M., and Gavins, F. N. E. (2003). Annexin 1: An Endogenous Anti-inflammatory Protein. Physiology 18, 60–64. doi:10.1152/nips.01424.2002
Pinto, S. M., Subbannayya, Y., Rex, D. A. B., Raju, R., Chatterjee, O., Advani, J., et al. (2018). A Network Map of IL-33 Signaling Pathway. J. Cell Commun. Signal. 12, 615–624. doi:10.1007/s12079-018-0464-4
Potaczek, D. P., Harb, H., Michel, S., Alhamwe, B. A., Renz, H., and Tost, J. (2017). Epigenetics and Allergy: from Basic Mechanisms to Clinical Applications. Epigenomics 9, 539–571. doi:10.2217/epi-2016-0162
Quah, S., Subramanian, G., and Sampath, P. (2021). Repurposing Belinostat for Alleviation of Atopic Dermatitis. Dermatol Ther. (Heidelb) 11, 655–660. doi:10.1007/s13555-021-00527-7
Quintás-Cardama, A., Kantarjian, H., Estrov, Z., Borthakur, G., Cortes, J., and Verstovsek, S. (2012). Therapy with the Histone Deacetylase Inhibitor Pracinostat for Patients with Myelofibrosis. Leukemia Res. 36, 1124–1127. doi:10.1016/j.leukres.2012.03.003
Rösler, B., Wang, X., Keating, S. T., Joosten, L. A. B., Netea, M. G., and van de Veerdonk, F. L. (2018). HDAC Inhibitors Modulate Innate Immune Responses to Micro-organisms Relevant to Chronic Mucocutaneous Candidiasis. Clin. Exp. Immunol. 194, 205–219. doi:10.1111/cei.13192
Raikwar, N. S., Vandewalle, A., and Thomas, C. P. (2010). Nedd4-2 Interacts with Occludin to Inhibit Tight Junction Formation and Enhance Paracellular Conductance in Collecting Duct Epithelia. Am. J. Physiology-Renal Physiology 299, F436–F444. doi:10.1152/ajprenal.00674.2009
Rebane, A., Runnel, T., Aab, A., Maslovskaja, J., Rückert, B., Zimmermann, M., et al. (2014). MicroRNA-146a Alleviates Chronic Skin Inflammation in Atopic Dermatitis through Suppression of Innate Immune Responses in Keratinocytes. J. Allergy Clin. Immunol. 134, 836–847. e11. doi:10.1016/j.jaci.2014.05.022
Robertson, E. D., Weir, L., Romanowska, M., Leigh, I. M., and Panteleyev, A. A. (2012). ARNT Controls the Expression of Epidermal Differentiation Genes through HDAC- and EGFR-dependent Pathways. J. Cell Sci. jcs 125 (Pt 14), 095125. doi:10.1242/jcs.095125
Rodríguez, E., Baurecht, H., Herberich, E., Wagenpfeil, S., Brown, S. J., Cordell, H. J., et al. (2009). Meta-analysis of Filaggrin Polymorphisms in Eczema and Asthma: Robust Risk Factors in Atopic Disease. J. Allergy Clin. Immunol. 123, 1361–1370. e7. doi:10.1016/j.jaci.2009.03.036
Roesner, L. M., Floess, S., Witte, T., Olek, S., Huehn, J., and Werfel, T. (2015). Foxp3+regulatory T Cells Are Expanded in Severe Atopic Dermatitis Patients. Allergy 70, 1656–1660. doi:10.1111/all.12712
Ruf, M. T., Andreoli, A., Itin, P., Pluschke, G., and Schmid, P. (2014). Ribosomal Protein S6 Is Hyperactivated and Differentially Phosphorylated in Epidermal Lesions of Patients with Psoriasis and Atopic Dermatitis. Br. J. Dermatol 171, 1533–1536. doi:10.1111/bjd.13248
Ruvinsky, I., and Meyuhas, O. (2006). Ribosomal Protein S6 Phosphorylation: from Protein Synthesis to Cell Size. Trends Biochem. Sci. 31, 342–348. doi:10.1016/j.tibs.2006.04.003
Ryu, W.-I., Lee, H., Kim, J. H., Bae, H. C., Ryu, H. J., and Son, S. W. (2015). IL-33 Induces Egr-1-dependent TSLP Expression via the MAPK Pathways in Human Keratinocytes. Exp. Dermatol 24, 857–863. doi:10.1111/exd.12788
Salva, K. A., Reeder, M. J., Lloyd, R., and Wood, G. S. (2017). c-CBL E3 Ubiquitin Ligase Expression Increases across the Spectrum of Benign and Malignant T-Cell Skin Diseases. Am. J. Dermatopathol. 39, 731–737. doi:10.1097/dad.0000000000000780
Samaka, R. M., Basha, M. A., and El Beih, D. (2020). Role of TRIM Proteins in Inflammatory Skin Diseases. Egypt. J. Hosp. Med. 81, 2462–2467. doi:10.21608/ejhm.2020.133965
Sanford, J. A., Zhang, L.-J., Williams, M. R., Gangoiti, J. A., Huang, C.-M., and Gallo, R. L. (2016). Inhibition of HDAC8 and HDAC9 by Microbial Short-Chain Fatty Acids Breaks Immune Tolerance of the Epidermis to TLR Ligands. Sci. Immunol. 1, eaah4609. doi:10.1126/sciimmunol.aah4609
Sasaki, T., Shiohama, A., Kubo, A., Kawasaki, H., Ishida-Yamamoto, A., Yamada, T., et al. (2013). A Homozygous Nonsense Mutation in the Gene for Tmem79, a Component for the Lamellar Granule Secretory System, Produces Spontaneous Eczema in an Experimental Model of Atopic Dermatitis. J. Allergy Clin. Immunol. 132, 1111–1120. e4. doi:10.1016/j.jaci.2013.08.027
Saunders, S. P., Goh, C. S. M., Brown, S. J., Palmer, C. N. A., Porter, R. M., Cole, C., et al. (2013). Tmem79/Matt Is the Matted Mouse Gene and Is a Predisposing Gene for Atopic Dermatitis in Human Subjects. J. Allergy Clin. Immunol. 132, 1121–1129. doi:10.1016/j.jaci.2013.08.046
Schünke, H., Göbel, U., Dikic, I., and Pasparakis, M. (2021). OTULIN Inhibits RIPK1-Mediated Keratinocyte Necroptosis to Prevent Skin Inflammation in Mice. Nat. Commun. 12, 5912. doi:10.1038/s41467-021-25945-1
Scognamiglio, A., Nebbioso, A., Manzo, F., Valente, S., Mai, A., and Altucci, L. (2008). HDAC-Class II Specific Inhibition Involves HDAC Proteasome-dependent Degradation Mediated by RANBP2fic Inhibition Involves HDAC Proteasome-dependent Degradation Mediated by RANBP2. Biochimica Biophysica Acta (BBA) - Mol. Cell Res. 1783, 2030–2038. doi:10.1016/j.bbamcr.2008.07.007
Shade, K.-T. C., Conroy, M. E., Washburn, N., Kitaoka, M., Huynh, D. J., Laprise, E., et al. (2020). Sialylation of Immunoglobulin E Is a Determinant of Allergic Pathogenicity. Nature 582, 265–270. doi:10.1038/s41586-020-2311-z
Shade, K.-T., Conroy, M. E., and Anthony, R. M. (2019). “IgE Glycosylation in Health and Disease,” in Fc Mediated Activity Of Antibodies Current Topics in Microbiology and Immunology. Editors J. V. Ravetch,, and F. Nimmerjahn (Cham, 77–93. doi:10.1007/82_2019_151
Shaw, R. J. (2009). LKB1 and AMP-Activated Protein Kinase Control of mTOR Signalling and Growth. Acta Physiol. 196, 65–80. doi:10.1111/j.1748-1716.2009.01972.x
Shen, H., Sha, Y., Huang, J., Mao, A. Q., Zhang, T., Wu, M. Y., et al. (2021). The Roles of AMPK-Mediated Autophagy and Mitochondrial Autophagy in a Mouse Model of Imiquimod-Induced Psoriasis. Am. J. Transl. Res. 13, 12626–12637.
Shi, Y.-L., Gu, J., Park, J.-Y., Xu, Y.-P., Yu, F.-S., Zhou, L., et al. (2012). Histone Deacetylases Inhibitor Trichostatin A Ameliorates DNFB-Induced Allergic Contact Dermatitis and Reduces Epidermal Langerhans Cells in Mice. J. Dermatological Sci. 68, 99–107. doi:10.1016/j.jdermsci.2012.09.001
Shimizu, K., Gi, M., Suzuki, S., North, B. J., Watahiki, A., Fukumoto, S., et al. (2021). Interplay between Protein Acetylation and Ubiquitination Controls MCL1 Protein Stability. Cell Rep. 37, 109988. doi:10.1016/j.celrep.2021.109988
Silverberg, J. I., Barbarot, S., Gadkari, A., Simpson, E. L., Weidinger, S., Mina-Osorio, P., et al. (2021). Atopic Dermatitis in the Pediatric Population. Ann. Allergy, Asthma & Immunol. 126, 417–428. e2. doi:10.1016/j.anai.2020.12.020
Smith, P. K., Masilamani, M., Li, X.-M., and Sampson, H. A. (2017). The False Alarm Hypothesis: Food Allergy Is Associated with High Dietary Advanced Glycation End-Products and Proglycating Dietary Sugars that Mimic Alarmins. J. Allergy Clin. Immunol. 139, 429–437. doi:10.1016/j.jaci.2016.05.040
Song, Z., Deng, X., Chen, W., Xu, J., Chen, S., Zhong, H., et al. (2015). Toll-like Receptor 2 Agonist Pam3CSK4 Up-Regulates FcεRI Receptor Expression on Monocytes from Patients with Severe Extrinsic Atopic Dermatitis. J. Eur. Acad. Dermatol Venereol. 29, 2169–2176. doi:10.1111/jdv.13172
Su, M., Gong, X., and Liu, F. (2021). An Update on the Emerging Approaches for Histone Deacetylase (HDAC) Inhibitor Drug Discovery and Future Perspectives. Expert Opin. Drug Discov. 16, 745–761. doi:10.1080/17460441.2021.1877656
Sugaya, M. (2020). The Role of Th17-Related Cytokines in Atopic Dermatitis. IJMS 21, 1314. doi:10.3390/ijms21041314
Sundberg, J. P., Pratt, C. H., Goodwin, L. P., Silva, K. A., Kennedy, V. E., Potter, C. S., et al. (2020). Keratinocyte-specific Deletion of SHARPIN Induces Atopic Dermatitis-like Inflammation in Mice. PLoS ONE 15, e0235295. doi:10.1371/journal.pone.0235295
Szalus, K., Trzeciak, M., and Nowicki, R. J. (2020). JAK-STAT Inhibitors in Atopic Dermatitis from Pathogenesis to Clinical Trials Results. Microorganisms 8, 1743. doi:10.3390/microorganisms8111743
Tang, L., Wang, J., Zhu, J., and Liang, Y. (2018). Down‐regulated SHARPIN May Accelerate the Development of Atopic Dermatitis through Activating Interleukin‐33/ST 2 Signalling. Exp. Dermatol 27, 1328–1335. doi:10.1111/exd.13784
Theivanthiran, B., Kathania, M., Zeng, M., Anguiano, E., Basrur, V., Vandergriff, T., et al. (2015). The E3 Ubiquitin Ligase Itch Inhibits P38α Signaling and Skin Inflammation through the Ubiquitylation of Tab1. Sci. Signal 8, ra22. doi:10.1126/scisignal.2005903
Thrash, B. R., Menges, C. W., Pierce, R. H., and McCance, D. J. (2006). AKT1 Provides an Essential Survival Signal Required for Differentiation and Stratification of Primary Human Keratinocytes. J. Biol. Chem. 281, 12155–12162. doi:10.1074/jbc.M512116200
Traisaeng, S., Herr, D. R., Kao, H.-J., Chuang, T.-H., and Huang, C.-M. (2019). A Derivative of Butyric Acid, the Fermentation Metabolite of Staphylococcus Epidermidis, Inhibits the Growth of a Staphylococcus aureus Strain Isolated from Atopic Dermatitis Patients. Toxins 11, 311. doi:10.3390/toxins11060311
Trautmann, A., Akdis, M., Kleemann, D., Altznauer, F., Simon, H.-U., Graeve, T., et al. (2000). T Cell-Mediated Fas-Induced Keratinocyte Apoptosis Plays a Key Pathogenetic Role in Eczematous Dermatitis. J. Clin. Invest. 106, 25–35. doi:10.1172/JCI9199
Tsuji, G., Okiyama, N., Villarroel, V. A., and Katz, S. I. (2015). Histone Deacetylase 6 Inhibition Impairs Effector CD8 T-Cell Functions during Skin Inflammation. J. Allergy Clin. Immunol. 135, 1228–1239. doi:10.1016/j.jaci.2014.10.002
Tsuji, S., Uehori, J., Matsumoto, M., Suzuki, Y., Matsuhisa, A., Toyoshima, K., et al. (2001). Human Intelectin Is a Novel Soluble Lectin that Recognizes Galactofuranose in Carbohydrate Chains of Bacterial Cell Wall. J. Biol. Chem. 276, 23456–23463. doi:10.1074/jbc.m103162200
Tsukahara, H., Shibata, R., Ohta, N., Sato, S., Hiraoka, M., Ito, S., et al. (2003). High Levels of Urinary Pentosidine, an Advanced Glycation End Product, in Children with Acute Exacerbation of Atopic Dermatitis: Relationship with Oxidative Stress. Metabolism 52, 1601–1605. doi:10.1016/s0026-0495(03)00310-x
van Dalen, R., De La Cruz Diaz, J. S., Rumpret, M., Fuchsberger, F. F., van Teijlingen, N. H., Hanske, J., et al. (2019). Langerhans Cells Sense Staphylococcus aureus Wall Teichoic Acid through Langerin to Induce Inflammatory Responses. mBio 10. doi:10.1128/mbio.00330-19
Venne, A. S., Kollipara, L., and Zahedi, R. P. (2014). The Next Level of Complexity: Crosstalk of Posttranslational Modifications. Proteomics 14, 513–524. doi:10.1002/pmic.201300344
Verdin, E., and Ott, M. (2015). 50 Years of Protein Acetylation: from Gene Regulation to Epigenetics, Metabolism and beyond. Nat. Rev. Mol. Cell Biol. 16, 258–264. doi:10.1038/nrm3931
Wabnitz, G. H., Köcher, T., Lohneis, P., Stober, C., Konstandin, M. H., Funk, B., et al. (2007). Costimulation Induced Phosphorylation of L-Plastin Facilitates Surface Transport of the T Cell Activation Molecules CD69 and CD25. Eur. J. Immunol. 37, 649–662. doi:10.1002/eji.200636320
Wang, Y., Zhang, Y., Peng, G., and Han, X. (2018). Glycyrrhizin Ameliorates Atopic Dermatitis-like Symptoms through Inhibition of HMGB1. Int. Immunopharmacol. 60, 9–17. doi:10.1016/j.intimp.2018.04.029
Wang, Z., Yoo, Y. J., De La Torre, R., Topham, C., Hanifin, J., Simpson, E., et al. (2021). Inverse Correlation of TRIM32 and Protein Kinase C ζ in T Helper Type 2-Biased Inflammation. J. Investigative Dermatology 141, 1297–1307. e3. doi:10.1016/j.jid.2020.09.021
Weidinger, S., and Novak, N. (2016). Atopic Dermatitis. Lancet 387, 1109–1122. doi:10.1016/s0140-6736(15)00149-x
Winge, M. C. G., Bilcha, K. D., Liedén, A., Shibeshi, D., Sandilands, A., Wahlgren, C.-F., et al. (2011). Novel Filaggrin Mutation but No Other Loss-Of-Function Variants Found in Ethiopian Patients with Atopic Dermatitis. Br. J. Dermatology 165, 1074–1080. doi:10.1111/j.1365-2133.2011.10475.x
Winget, J. M., Finlay, D., Mills, K. J., Huggins, T., Bascom, C., Isfort, R. J., et al. (2016). Quantitative Proteomic Analysis of Stratum Corneum Dysfunction in Adult Chronic Atopic Dermatitis. J. Investigative Dermatology 136, 1732–1735. doi:10.1016/j.jid.2016.03.037
Wollenberg, A., Howell, M. D., Guttman-Yassky, E., Silverberg, J. I., Kell, C., Ranade, K., et al. (2019). Treatment of Atopic Dermatitis with Tralokinumab, an anti-IL-13 mAb. J. Allergy Clin. Immunol. 143, 135–141. doi:10.1016/j.jaci.2018.05.029
Wu, C. L., Qiang, L., Han, W., Ming, M., Viollet, B., and He, Y. Y. (2013). Role of AMPK in UVB-Induced DNA Damage Repair and Growth Control. Oncogene 32, 2682–2689. doi:10.1038/onc.2012.279
Wu, J.-L., Chiang, M.-F., Hsu, P.-H., Tsai, D.-Y., Hung, K.-H., Wang, Y.-H., et al. (2017). O-GlcNAcylation Is Required for B Cell Homeostasis and Antibody Responses. Nat. Commun. 8, 1854. doi:10.1038/s41467-017-01677-z
Xiao, D.-Q., Xu, P.-H., Deng, H., Chen, L.-S., Ding, X.-A., Bai, M., et al. (2017). The Characterization and Clinical Significance of PI3K/Akt Signaling Pathway Activation in the Peripheral T Cells of Pediatric Patients with Atopic Dermatitis. Int. J. Clin. Exp. Med. 10, 2904–2910.
Yan, F., Meng, W., Ye, S., Zhang, X., Mo, X., Liu, J., et al. (2019a). MicroRNA-146a as a Potential Regulator Involved in the Pathogenesis of Atopic Dermatitis. Mol. Med. Rep. 20 (5), 4645–4653. doi:10.3892/mmr.2019.10695
Yan, F., Zhang, J., Li, X., Mo, X., Liu, J., Ye, S., et al. (2019b2019). Therapeutic Effects of Chinese Herbal Formula (PTQX) on NC/Nga Mice with Atopic Dermatitis-like Skin Lesions. Evidence-Based Complementary Altern. Med. 2019, 1–13. doi:10.1155/2019/8359252
Yan, J., Seibenhener, M. L., Calderilla-Barbosa, L., Diaz-Meco, M.-T., Moscat, J., Jiang, J., et al. (2013). SQSTM1/p62 Interacts with HDAC6 and Regulates Deacetylase Activity. PLoS ONE 8, e76016. doi:10.1371/journal.pone.0076016
Yan, S., Ripamonti, R., Kawabe, H., Ben-Yehuda Greenwald, M., and Werner, S. (2022). NEDD4-1 Is a Key Regulator of Epidermal Homeostasis and Wound Repair. J. Investigative Dermatology 142, 1703–1713. S0022202X21024040. doi:10.1016/j.jid.2021.09.033
Yang, F., Tanaka, M., Wataya-Kaneda, M., Yang, L., Nakamura, A., Matsumoto, S., et al. (2014a). Topical Application of Rapamycin Ointment amelioratesDermatophagoides Farinabody Extract-Induced Atopic Dermatitis in NC/Nga Mice. Exp. Dermatol 23, 568–572. doi:10.1111/exd.12463
Yang, H., Zhang, W., Pan, H., Feldser, H. G., Lainez, E., Miller, C., et al. (2012). SIRT1 Activators Suppress Inflammatory Responses through Promotion of P65 Deacetylation and Inhibition of NF-Κb Activity. PLoS ONE 7, e46364. doi:10.1371/journal.pone.0046364
Yang, L., Fliegauf, M., and Grimbacher, B. (2014b). Hyper-IgE Syndromes. Curr. Opin. Pediatr. 26, 697–703. doi:10.1097/mop.0000000000000158
Yang, L., Jin, L., Ke, Y., Fan, X., Zhang, T., Zhang, C., et al. (2018). E3 Ligase Trim21 Ubiquitylates and Stabilizes Keratin 17 to Induce STAT3 Activation in Psoriasis. J. Investigative Dermatology 138, 2568–2577. doi:10.1016/j.jid.2018.05.016
Yang, L., and Yan, Y. (2022). Emerging Roles of Post-Translational Modifications in Skin Diseases: Current Knowledge, Challenges and Future Perspectives. JIR Vol. 15, 965–975. doi:10.2147/jir.s339296
Yang, N., Zhao, B., Rasul, A., Qin, H., Li, J., and Li, X. (2013). PIAS1-modulated Smad2/4 Complex Activation Is Involved in Zinc-Induced Cancer Cell Apoptosis. Cell Death Dis. 4, e811. doi:10.1038/cddis.2013.333
Yew, Y. W., Thyssen, J. P., and Silverberg, J. I. (2019). A Systematic Review and Meta-Analysis of the Regional and Age-Related Differences in Atopic Dermatitis Clinical Characteristics. J. Am. Acad. Dermatology 80, 390–401. doi:10.1016/j.jaad.2018.09.035
Yi, J. Z., and McGee, J. S. (2021). Epigenetic‐modifying Therapies: An Emerging Avenue for the Treatment of Inflammatory Skin Diseases. Exp. Dermatol 30, 1167–1176. doi:10.1111/exd.14334
Yi, L., Cheng, D., Zhang, K., Huo, X., Mo, Y., Shi, H., et al. (2017). Intelectin Contributes to Allergen-Induced IL-25, IL-33, and TSLP Expression and Type 2 Response in Asthma and Atopic Dermatitis. Mucosal Immunol. 10, 1491–1503. doi:10.1038/mi.2017.10
Ying, L., Shen, Y., Zhang, Y., Wang, Y., Liu, Y., Yin, J., et al. (2021). Association of Advanced Glycation End Products with Lower-Extremity Atherosclerotic Disease in Type 2 Diabetes Mellitus. Front. Cardiovasc. Med. 8, 696156. doi:10.3389/fcvm.2021.696156
Yosipovitch, G., Misery, L., Proksch, E., Metz, M., Ständer, S., and Schmelz, M. (2019). Skin Barrier Damage and Itch: Review of Mechanisms, Topical Management and Future Directions. Acta Derm. Venereol. 99, 1201–1209. doi:10.2340/00015555-3296
Zeze, N., Kido-Nakahara, M., Tsuji, G., Maehara, E., Sato, Y., Sakai, S., et al. (2022). Role of ERK Pathway in the Pathogenesis of Atopic Dermatitis and its Potential as a Therapeutic Target. IJMS 23, 3467. doi:10.3390/ijms23073467
Zhang, M., Miura, T., Suzuki, S., Chiyotanda, M., Tanaka, S., Sugiyama, K., et al. (2021). Vitamin K2 Suppresses Proliferation and Inflammatory Cytokine Production in Mitogen-Activated Lymphocytes of Atopic Dermatitis Patients through the Inhibition of Mitogen-Activated Protein Kinases. Biol. Pharm. Bull. 44, 7–17. doi:10.1248/bpb.b20-00079
Zhang, Y., Yu, X., Ichikawa, M., Lyons, J. J., Datta, S., Lamborn, I. T., et al. (2014). Autosomal Recessive Phosphoglucomutase 3 (PGM3) Mutations Link Glycosylation Defects to Atopy, Immune Deficiency, Autoimmunity, and Neurocognitive Impairment. J. Allergy Clin. Immunol. 133, 1400–1409. e5. doi:10.1016/j.jaci.2014.02.013
Zheng, B. W., Wang, B. Y., Xiao, W. L., Sun, Y. J., Yang, C., and Zhao, B. T. (2022). Different Molecular Weight Hyaluronic Acid Alleviates Inflammation Response in DNFB-Induced Mice Atopic Dermatitis and LPS-Induced RAW 264.7 Cells. Life Sci. 301, 120591. doi:10.1016/j.lfs.2022.120591
Zhou, X., Motta, F., Selmi, C., Ridgway, W. M., Gershwin, M. E., and Zhang, W. (2021). Antibody Glycosylation in Autoimmune Diseases. Autoimmun. Rev. 20, 102804. doi:10.1016/j.autrev.2021.102804
Zhuang, S. (2013). Regulation of STAT Signaling by Acetylation. Cell. Signal. 25, 1924–1931. doi:10.1016/j.cellsig.2013.05.007
Keywords: atopic dermatitis, post-translational modifications, skin disorders, therapeutic potential, epigenetics
Citation: Ma X, Ru Y, Luo Y, Kuai L, Chen Q-L, Bai Y, Liu Y-Q, Chen J, Luo Y, Song J-K, Zhou M and Li B (2022) Post-Translational Modifications in Atopic Dermatitis: Current Research and Clinical Relevance. Front. Cell Dev. Biol. 10:942838. doi: 10.3389/fcell.2022.942838
Received: 16 May 2022; Accepted: 16 June 2022;
Published: 07 July 2022.
Edited by:
Guanghong Jia, University of Missouri, United StatesReviewed by:
Ke Yang, Shanghai Jiao Tong University, ChinaKwonik O. H., Hallym University, South Korea
Copyright © 2022 Ma, Ru, Luo, Kuai, Chen, Bai, Liu, Chen, Luo, Song, Zhou and Li. This is an open-access article distributed under the terms of the Creative Commons Attribution License (CC BY). The use, distribution or reproduction in other forums is permitted, provided the original author(s) and the copyright owner(s) are credited and that the original publication in this journal is cited, in accordance with accepted academic practice. No use, distribution or reproduction is permitted which does not comply with these terms.
*Correspondence: Mi Zhou, dmlla3kyODY2QDE2My5jb20=; Bin Li, MTg5MzA1NjgxMjlAMTYzLmNvbQ==
†These authors have contributed equally to this work