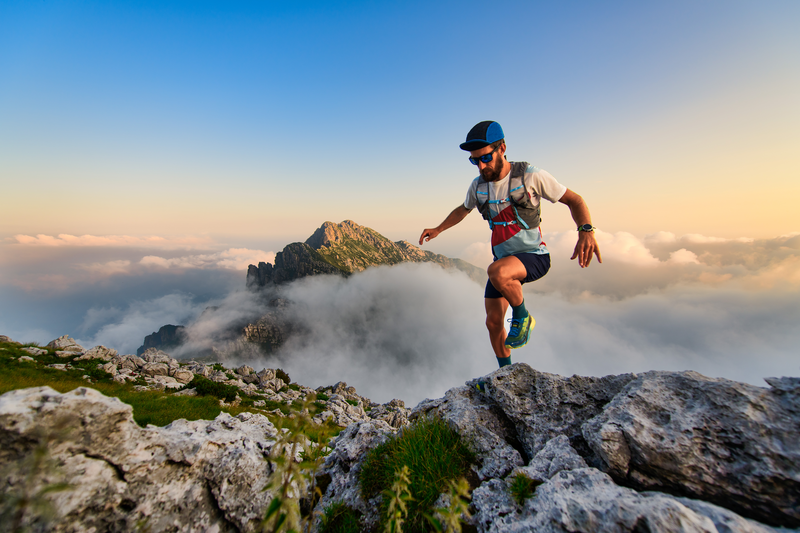
94% of researchers rate our articles as excellent or good
Learn more about the work of our research integrity team to safeguard the quality of each article we publish.
Find out more
MINI REVIEW article
Front. Cell Dev. Biol. , 26 May 2022
Sec. Cancer Cell Biology
Volume 10 - 2022 | https://doi.org/10.3389/fcell.2022.918795
This article is part of the Research Topic Mechanisms of Metastasis from Circulating and Disseminated Tumor Cells View all 8 articles
The extremely poor prognosis for patients with pancreatic ductal adenocarcinoma (PDAC) has remained unchanged for decades. As a hallmark of PDAC histology, the distinct desmoplastic response in the tumor microenvironment is considered a key factor exerting pro- and antitumor effects. Increasing emphasis has been placed on cancer-associated fibroblasts (CAFs), whose heterogeneity and functional diversity is reflected in the numerous subtypes. The myofibroblastic CAFs (myCAFs), inflammatory CAFs (iCAFs) and antigen presenting CAFs (apCAFs) are functionally divergent CAF subtypes with tumor promoting as well as repressing effects. Precise knowledge of the underlying interactions is the basis for a variety of treatment approaches, which are subsumed under the term antistromal therapy. Clinical implementation is still pending due to the lack of benefit—as well as paradoxical preclinical findings. While the prominent significance of CAFs in the immediate environment of the tumor is becoming clear, less is known about the circulating (c)CAFs. cCAFs are of particular interest as they seem not only to be potential new liquid biopsy biomarkers but also to support the survival of circulating tumor cells (CTC) in the bloodstream. In PDAC, CTCs correlate with an unfavorable outcome and can also be employed to monitor treatment response, but the current clinical relevance is limited. In this review, we discuss CTCs, cCAFs, secretomes that include EVs or fragments of collagen turnover as liquid biopsy biomarkers, and clinical approaches to target tumor stroma in PDAC.
Pancreatic ductal adenocarcinoma (PDAC) is one of the most lethal cancers with a 5-year survival rate of <9% over all stages and remains a major challenge for current cancer therapy (Rawla et al., 2019). In the Western world PDAC is predicted to become the second leading cause of cancer-associated death (Malvezzi et al., 2019; Sung et al., 2021). More than 80% of cases are diagnosed at an advanced or metastatic stage, hence most treatment combination regimen rely on chemotherapy (Kuehn, 2020; Wang et al., 2021). A dense fibroblastic stroma is a hallmark of the PDAC microenvironment, which can constitutes up to 90% of the tumor mass (Ansari et al., 2017). The PDAC associated stroma mainly consists of extracellular matrix (ECM) proteins and cancer-associated fibroblasts (CAFs) and functions as a barrier of effective drug penetration (Jacobetz et al., 2013). Furthermore, immune cells, e.g., myeloid cells and lymphocytes, and to a lower extent angiogenic vascular cells are present (Hanahan and Coussens, 2012; Chen and Mellman, 2017; Ren et al., 2018). Considered as critical players in PDAC carcinogenesis CAFs are engaged in a dynamic exchange with cancer cells based on a variety of mechanisms ranging from paracrine secretion to extracellular vesicle (EV) trafficking (von Ahrens et al., 2017; Leca et al., 2016). Given that not only tumor promoting but also tumor repressive effects are mitigated by the tumor-stromal crosstalk, the CAFs in the center are of paramount interest with respect to novel therapeutic approaches (Wang et al., 2021).
The current unmet need for clinically relevant prognostic and predictive biomarkers is considered another reason for the persistently poor prognosis of PDAC patients (Wang et al., 2021). Liquid biopsy has become a powerful non-invasive tool to obtain important tumor related information from body fluids. The number of CTCs correlate with a poor prognosis and can also be used to monitor therapy response (Alix-Panabières and Pantel, 2021). However, the clinical impact of CTC enumeration regarding survival is limited (Ferreira et al., 2016). Meanwhile, the detection of circulating (c)CAFs, as surrogates of the tumor-associated stroma, is a merging novel field in liquid biopsies (Ortiz-Otero et al., 2020a).
In view of their potential use as future liquid biopsy markers and as clinical approaches for tumor stroma targeting, this review summarizes the current knowledge about CTCs, cCAFs and their stroma-derived proteomic signature.
Today, it is well accepted, that CAFs are composed of a large number of cells from different origins—leading to great heterogeneity in terms of phenotype and function, which hinders a consistent classification. Therefore, the key features were summarized in a consensus statement: elongated cells that are negative for lineage-specific epithelial, endothelial, and leukocyte markers and do not harbor mutations of their associated tumor are referred to as CAFs (Sahai et al., 2020). However, the lack of a specific marker compromises accurate tracing of their cellular origin (Nurmik et al., 2020).
Originally, CAFs in PDAC were considered to be derived from tissue-derived fibroblasts and in a majority from pancreatic stellate cells (PSCs) (Erkan et al., 2012; Arina et al., 2016). This paradigm has been challenged by recent findings on the lineage of CAFs. After targeted ablation of PSCs in a PDAC mouse model, it was observed that only a minority of PSCs contribute to the population of total CAFs (Helms et al., 2022). PSCs are mesenchymal tissue-resident cells characterized by vitamin A-containing lipid droplet storage function and expression of fibroblast activation protein α (FAPα) (Erkan et al., 2012; Moir et al., 2015). FAPα exerts proteolytic activity and is linked to ECM degradation and remodeling (Knopf et al., 2015). Upon paracrine stimulation from adjacent tumor cells, quiescent PSCs become activated, then termed CAFs, and increase the production of ECM proteins (Bachem et al., 2005).
However, as part of a versatile multicellular origin, transdifferentiation of epithelial and endothelial cells (Iwano et al., 2002; Zeisberg et al., 2007), as well as adipocytes, pericytes and smooth muscle cells can give rise to CAFs (Dulauroy et al., 2012; Bochet et al., 2013). Moreover, a transgenic mouse model indicated that a relevant subset of CAFs appears to be additionally recruited from bone marrow niche cells and in fact arise from mesenchymal stem cells (MSCs) (Quante et al., 2011) (Figure 1).
FIGURE 1. Cancer-associated fibroblasts (CAFs) have multiple origins and arise from pancreatic stellate cells (PSCs), mesenchymal stem cells (MSCs), adipocytes, pericytes, and smooth muscle cells. Myofibroblastic CAFs (myCAFs), inflammatory CAFs (iCAFs), antigen-presenting CAFs (apCAFs) and Meflin-positive CAFs are the major CAF subtypes and reflect functional diversity. As potential biomarkers, circulating tumor cells (CTCs), circulating (c)CAFs, stromal fragments, and extracellular vesicles (EVs) are an emerging area of research in liquid biopsy and offer future clinical implications.
The different subtypes appear to have partially contrasting effects on tumor biology, so accurate knowledge of these subpopulations is crucial for understanding and developing therapeutic approaches.
This section highlights shortly the most important CAF subtypes currently described. However, numerous other CAF subtypes have been identified in recent years. Summarizing them all is beyond the scope of this overview—yet they have recently been described and reviewed (Awaji and Singh, 2019; Neuzillet et al., 2019; Pereira et al., 2019; Garcia et al., 2020; Helms et al., 2020; Huang and Brekken, 2020).
Single cell resolution studies, including single-cell RNA sequencing, have provided valuable insights into the composition and properties of stromal cells (Öhlund et al., 2017; Elyada et al., 2019; Biffi et al., 2019). In a 3D-coculture system of PSCs and PDAC cells Öhlund et al. described two distinct subtypes, myofibroblastic CAFs (myCAFs) and inflammatory CAFs (iCAFs), thereby confirming their functional heterogeneity (Öhlund et al., 2017) (Figure 1). In addition, these subtypes seem to differ in their spatial distribution. MyCAFs are FAPα-positive cells with high expression of α-smooth muscle actin (αSMA) and are in direct contact with cancer cells (Öhlund et al., 2017). Activated by the proximity to cancer cells, myCAFs increase their collagen I synthesis (Öhlund et al., 2017). However, counterintuitively, depletion of αSMA-positive CAFs impaired survival in mice despite reduction in total collagen content (Özdemir et al., 2014). Thus, these results imply a possible antitumor activity of myCAFs (Özdemir et al., 2014).
The iCAF subtype is located more distant from tumor cells and showed an upregulation of leukemia inhibitory factor (LIF), C-X-C Motif Chemokine Ligand 1 (CXCL1), and Interleukin 6 (IL-6) and IL-11 (Öhlund et al., 2017). In a PDAC mouse model, inhibition of LIF and genetic depletion of its receptor resulted in increased sensitivity to gemcitabine treatment and impaired tumor growth (Shi et al., 2019). Further studies have identified additional markers for iCAFs including CXCL12, IL6, and IL8 as wells as TNFα and NF-κB (Elyada et al., 2019). By secreting pro-inflammatory cytokines and mediating immune evasion, iCAFs are widely regarded as promoters of tumor growth in PDAC (Biffi et al., 2019).
Previous work has illustrated that myCAFs and iCAFs can switch subtypes, thereby indicating a high level of plasticity. These subpopulations are therefore conceptualized as functional states on a continuum rather than endpoints of differentiation. Through JAK/STAT activation, IL-1 promotes the development of inflammatory CAFs. Whereas TGFβ counteracts the JAK/STAT signaling cascade by suppressing expression of Il1r1, thereby mediating a transition to the myCAF type (Biffi et al., 2019). Moreover, inhibition of the TGFβ pathway resulted in elevated expression of iCAF marker genes (Biffi et al., 2019).
Recently scRNA-seq of tumors in a PDAC mouse model revealed a novel CAF subtype that expresses MHC class II family genes (Elyada et al., 2019). The upregulated signaling pathways and genes, e.g., histocompatibility 2, class II antigen A (H2-Aa) and H2-Ab1, are typically associated with antigen-presenting cells (APCs), hence this subtype was referred to as antigen-presenting CAFs (apCAFs) (Elyada et al., 2019).
Conceptually, another classification proposal for this complex, growing landscape of different CAF subtypes is to divide CAFs into cancer-promoting CAFs (pCAFs) and cancer-restraining CAFs (rCAFs) (Kalluri, 2016; Kobayashi et al., 2019; Mizutani et al., 2019). However, the definition of pCAFs and rCAFs still needs to be clarified (Kalluri, 2016). Inclusion of the subgroups described above, such as iCAFs, apCAFs, and myCAFs, in this classification is also challenging. This is partly due to the multiple functions of their markers, exemplified by the controversial role of alpha-SMA in the progression of PDAC (Özdemir et al., 2014). Mizutani et al. discovered a potential marker for rCAFs, namely meflin, a glycosylphosphatidyl inositol (GPI)-anchored protein specifically expressed in MSCs (Mizutani et al., 2019). The Meflin-positive CAF subtype is defined by its positivity for Meflin, Platelet-derived growth factor receptor-α (PDGFRα), Glioma-associated oncogene homologue 1 (Gli1) with low expression of αSMA (Mizutani et al., 2019). The infiltration of Meflin-positive CAFs in human PDAC tissue samples appears to have prognostic value as it was correlated with beneficial outcome (Mizutani et al., 2019). This observation was also supported in a meflin-deficient PDAC mouse model. Thus, ablation of meflin promoted tumor growth along with a poorly differentiated histology (Mizutani et al., 2019).
Knowledge of CAF subtypes in PDAC is clearly increasing, but their precise implications for treatment response and overall survival (OS) has yet to be determined.
Liquid biopsy has become a powerful non-invasive tool to obtain important tumor related information from body fluids. Most clinically relevant data has been collected from the analyses of circulating tumor DNA (ctDNA) and circulating tumor cells (CTCs). The presence of CTCs in the bloodstream of cancer patients not only correlate with a poor prognosis but can also be used to monitor the therapy response and collect tumor material for molecular analyses (Alix-Panabières and Pantel, 2021). Whereas numerous studies on the biological and clinical role of CTC in PDAC exist today, the detection of cCAFs is a merging novel field (Ao et al., 2015; Ortiz-Otero et al., 2020a).
CTCs in the blood of cancer patients are a rare subset of the most malignant tumor cells, representing the whole tumor burden (Yeo et al., 2022). In non-resectable PDAC patients, the gain of tumor tissue is often difficult. CTCs might therefore become an important alternative tool due to its potential utility as diagnostic, prognostic, and predictive biomarker (Pantel and Alix-Panabières, 2019; Yeo et al., 2022). Circulating epithelial cells have been found in pancreatic precancerous lesions such as intraductal papillary mucinous neoplasm (IPMN) indicative of highly migratory capabilities of pancreatic cells even in early tumor setting (Franses et al., 2018).
There is no gold-standard CTC detection enrichment method yet for PDAC, and low detection rates, small cohorts and a focus on locally advanced and metastatic stages hinder valuable comparisons between the existing studies that have assessed CTC detection in peripheral blood of PDAC patients (Gall et al., 2019; Lee et al., 2019; Yeo et al., 2022). Previous studies have included between 14 and 172 PDAC patients each, with reported CTC rates between 7 and 42% for operable patients and 19 and 48% for metastatic patients (Ferreira et al., 2016; Effenberger et al., 2018; Martini et al., 2019; Yeo et al., 2022). Many of these studies have proven that CTCs are independent predictors of bad prognosis and could facilitate a better stratification of patients than classical parameters (TNM classification, imaging methods, CA-19-9 levels) (Ferreira et al., 2016; Effenberger et al., 2018; Martini et al., 2019). Two meta analyses, comprising more than 600 patients each, have proved the association of CTC positivity and worse survival (Han et al., 2014; Ma et al., 2014).
Due to the large heterogeneity seen in PDAC CTCs, physical enrichment techniques combined with optimized CTC detection seem promising (Kalluri and Neilson, 2003; Kalluri and Weinberg, 2009; Martini et al., 2019). Another promising approach for increasing CTC detection rates is to analyze the tumor-draining blood—as CTCs are released directly into the tumor-draining portal vein before entering the liver as a first capillary bed (Arnoletti et al., 2017; Song et al., 2020; White et al., 2021; Arnoletti et al., 2022).
CTCs can be found as single cells or cell clusters (multicellular CTC aggregates called CTC clusters). The CTC cluster can be composed of only CTCs (homotypic clusters) or admixed of CTCs and immune or stromal cells (heterotypic clusters) (Aceto, 2020; Wrenn et al., 2021). Although CTC clusters are much more rarely seen than single CTCs, their presence has been associated with higher metastatic potential in animal models (Cheung et al., 2016; Liu et al., 2019; Taftaf et al., 2021) and indicated worse prognosis than single CTCs (Hou et al., 2012; Aceto et al., 2014). Also, among PDAC patients, clusters have been identified (Wu et al., 2018). The heterotypic clusters can be composed of fibroblasts, endothelial cells, white blood cells, or platelets (Aceto et al., 2014). In a lung cancer mouse model, the viability of CTC was higher if they formed heterotypic clusters with fibroblasts (Duda et al., 2010). Importantly, depletion of CAFs resulted in reduced metastasis, implying their essential role in CTC survival and metastatic capacity (Duda et al., 2010).
In future personalized-medicine approaches, characterization and functional testing of CTCs and clusters might lead to benefits for patient management and uncover new therapies by identifying the specific CTC subpopulations for metastatic progression (Yeo et al., 2022).
In various tumor entities including PDAC, cCAFs have been detected (Ortiz-Otero et al., 2020a). These rare cells can be found in a heterotypic cluster with CTCs or alone (Ao et al., 2015; Sharma et al., 2021). In general, patients at a metastatic stage showed a significantly higher amount of cCAFs in the blood compared to patients with localized tumors (Ao et al., 2015). In a cohort of 34 patients with metastatic breast cancer, cCAFs were found in 88.2%, with numbers varying notably, ranging from 0 to 117 per 7.5 ml of EDTA blood. In contrast, cCAFs were present in only 23.1% of the 13 patients with localized cancer, and the maximum count per 7.5 ml of blood did not exceed 2 (Ao et al., 2015). The potential use of cCAFs as a predictive biomarker is also supported by the observation that cCAFs were not present in healthy donors and an increased cCAF count correlated with poor prognosis (Ao et al., 2015; Ortiz-Otero et al., 2020a).
Previous studies have employed different enrichment methods and surface markers to detect cCAFs, thereby limiting their comparability and predictions on the occurrence of cCAFs in different tumor types (Ao et al., 2015; Ortiz-Otero et al., 2020a). Ao et al. first exploited the physical properties of cCAFs by applying a size-based microfilter to enrich cCAFs from peripheral blood samples of patients with breast cancer (Ao et al., 2015). Subsequently, the classification of cCAFs was conducted by the combined detection of FAPα and αSMA. As an additional morphological condition, the spindle-shaped form of the captured cells, a feature typical of CAFs, was verified (Ao et al., 2015). Another study analyzed the blood of patients with prostate cancer using the CellSearchTM system. Fibroblast-like cells, defined as CK- and CD45-negative and vimentin-positive populations, were observed in patients with metastatic but not localized disease stage in this study (Jones et al., 2013). High expression of vimentin is indeed found on fibroblasts. Nevertheless it should be taken into account that other cells of mesenchymal origin and tumor cells undergoing EMT also express vimentin (Kalluri and Weinberg, 2009).
The studies by Ortiz-Ortero et al. covered the most extensive variety of different tumor types, including six patients with metastatic PDAC (Ortiz-Otero et al., 2020a). In their approach, mononuclear cells were isolated using Ficoll density centrifugation, followed by positive enrichment based on anti-fibroblast magnetic beads and identification by αSMA staining (Ortiz-Otero et al., 2020a).
As local CAFs are of paramount importance in the tumor microenvironment (Sahai et al., 2020), it is reasonable to speculate that cCAFs alone or in a heterotypic cluster with CTCs might also have analogous biological significance, e.g., facilitating metastasis (Ortiz-Otero et al., 2020b). This remains a hypothesis, as there is much less known about cCAFs than CTCs, although initial data are promising.
Since cCAF are rare and a heterogeneous population of cells, a combined detection approach including CTCs might be beneficial to increase sensitivity. Furthermore, it is conceivable to exploit other PDAC specific stromal characteristics: ECM proteins as hyaluronan (HA) along with collagen types I, III, and IV, are surrogates for desmoplasmic expanse in PDAC (Whatcott et al., 2015). This abundant accumulation of ECM caused by the increased activity of CAFs is also subject to enzyme-mediated turnover, e.g., by matrix metalloproteinases, the fragments of which may, in turn, be released into the bloodstream. Several studies have explored the value of these blood-detectable stromal fragments as potential biomarkers in PDAC (Willumsen et al., 2013; Willumsen et al., 2019; Chen et al., 2020; Nissen et al., 2021). In a cohort of 972 participants, including 809 patients with PDAC, serum levels of HA and the N-terminal propeptide of type III collagen (PRO-C3) were measured and compared with healthy subjects. Levels of HA and PRO-C3 were significantly elevated in patients with PDAC compared to healthy subjects or patients with benign diseases, including IPMN (Chen et al., 2020). Another study demonstrated the association between a higher PRO-C3 value and worse OS. Moreover, the higher ratio of fragment C3M, the MMP-mediated degradation product of type III collagen, to PRO-C3 was associated with longer OS and can be interpreted as a sign of a decreased fibrotic activity (Willumsen et al., 2019).
However, the crosstalk of CAFs and PDAC cancer cells occurs not only by paracrine mechanisms but also through EV trafficking. When CAFs are exposed to unfavorable pathophysiological conditions, such as lipid deficiency, macrophage co-cultivation and hypoxia, they secrete EVs bearing the complex annexin A6/LDL receptor-related protein 1/thrombospondin 1 (ANXA6/LRP1/TSP1) (Leca et al., 2016). Uptake of this complex by cancer cells resulted in increased tumor aggressiveness. Interestingly, Leca et al. detected CAF-derived ANXA6-positive EVs exclusively in the serum of PDAC patients compared with healthy subjects and subsequently showed that their occurrence was linked to shorter OS (Leca et al., 2016).
In the future, information on the fibrotic activity or aggressiveness of PDAC obtained by liquid biopsy could be an effective tool for PDAC subclassification and treatment stratification. Blood-based measurements of stromal fragments or CAF-derived EVs could be a valuable contribution to this purpose.
Stromal and CAF depletion as a therapeutic concept in PDAC is an intense area of research with numerous ongoing preclinical and translational studies (Jiang et al., 2020; Geng et al., 2021). From the plethora of potentially actionable pathways and targets, a few have been highlighted below for their potential clinical implications in the near future.
Nab-paclitaxel was the first drug with antistromal activity to show benefit in clinically relevant studies (Von Hoff et al., 2011; Von Hoff et al., 2013). Furthermore, the HA expression is considered a negative prognostic marker in PDAC and is therefore an attractive target for drug intervention (Whatcott et al., 2015). The benefit of HA degradation by pegvorhyaluronidase-alfa has been evaluated in multiple clinical trials (Hingorani et al., 2016; Infante et al., 2018). After initially promising results, it failed to improve survival in a large phase III trial (Van Cutsem et al., 2020). Several other anti-fibrotic agents such as halofuginone have demonstrated in preclinical studies that modulation of the TME toward a lower stromal content enhances the effect of antineoplastic agents (Elahi-Gedwillo et al., 2019). However, clinical benefit has yet to be proven.
PDAC peritumoral stromal response is significantly regulated by the Sonic Hedgehog (Shh) pathway, yet Shh inhibitors have failed in clinical trials (De Jesus-Acosta et al., 2020). Inhibition of Shh led to a reduction in αSMA-positive CAFs, but paradoxically to more aggressive tumors, underscoring the distinct and partially protective functions of the recently identified CAF subtypes in PDAC (Rhim et al., 2014).
Given that CAFs in part fuel tumor growth and form physical barriers impeding effective drug delivery, these cells appeared to be promising targets for therapeutic approaches (Jacobetz et al., 1136; Vonlaufen et al., 2008). An interesting target is the marker FAPα, which has been identified as a promoter of tumor growth, as its genetic deletion resulted in delayed tumor development and attenuation of metastasis (Lo et al., 2017). Nevertheless, inhibition of FAPα-positive CAFs by bispecific antibodies or chimeric antigen receptor T-cells, however, has shown no clinical value so far (Hingorani et al., 2016).
Evasion of cancer immunity and therapy resistance involves intimately also the interaction of CAFs with immune and tumor cells, which can be partially reversed by augmenting T-cell responses (Feig et al., 2013). The inhibition of CXCL12 secreted by FAPα-positive CAFs via CXCR4 antagonists increased cytotoxic T-cell infiltration and promoted an anti-α-PD-L1-mediated immune response in PDAC mouse models (Feig et al., 2013). In a phase II trial (NCT02826486), a CXCR-4 inhibitor in combination with PD-1-inhibition and chemotherapy led to an increase in survival in metatastic PDAC patients (Bockorny et al., 2020). Furthermore, via secretion of pro-inflammatory cytokines, including Il-6, iCAFs are considered a driving oncogenic force in PDAC (Geng et al., 2021). High IL-6 levels in the serum of PDAC patients are associated with cachexia and a worse prognosis (Okada et al., 1998; Rupert et al., 2021). Dual blockade of IL-6 and the immune checkpoint PD-1 acts synergistically and is hypothesized to overcome thereby immune resistance (Mace et al., 2018). The clinical utility of an IL-6 inhibitor is currently being evaluated in a combination therapy in advanced PDAC (NCT02767557).
Tumor promoting crosstalk between PDAC cancer cells and CAFs is mediated by a ligand for HER3 and HER4 receptors, neuroregulin-1, which is secreted by both cell types (Ogier et al., 2018). Its inhibition showed reduced migration and tumor growth in PDAC mouse models (Ogier et al., 2018). In an ongoing phase I/II, an anti-HER2xHER3 bispecific antibody has shown promising efficacy in neuroregulin-1 fusion-positive PDAC patients (NCT02912949).
The fact that the antifibrotic mechanisms of action and CAF heterogeneity is still rather poorly understood, exemplifies the ongoing cumbersome search for the ideal targeted therapy in PDAC.
Within the PDAC microenvironment, CAFs predominantly imprint intrastromal cellular crosstalk and exert both tumor-promoting and tumor-suppressive effects (Wang et al., 2021). Moreover, their biological functions are as diverse as their heterogeneous origins. The more we know about this biological complexity, the more we can use partial aspects, such as blood circulation of CAFs and release of stromal fragments, for liquid biopsy approaches to establish predictive markers in PDAC (Willumsen et al., 2019; Ortiz-Otero et al., 2020a). The most promising approach for liquid biopsies in PDAC might indeed be a combined approach of CTC, cCAF and other stromal fragment detection to increase sensitivity and maximize the chance of translation liquid biopsy techniques in clinical routine with therapeutic relevance (Willumsen et al., 2019; Ortiz-Otero et al., 2020a; Yeo et al., 2022). The steps toward clinical application of antistromal therapies, in addition to nab-paclitaxel, have been marked by many setbacks (Van Cutsem et al., 2020; Geng et al., 2021). However, the numerous preclinical and ongoing clinical trials are encouraging (Geng et al., 2021).
All authors listed have made a substantial, direct, and intellectual contribution to the work and approved it for publication.
Funded by: Foundation for the University Medical Center Hamburg-Eppendorf (JG and CN).
The authors declare that the research was conducted in the absence of any commercial or financial relationships that could be construed as a potential conflict of interest.
All claims expressed in this article are solely those of the authors and do not necessarily represent those of their affiliated organizations, or those of the publisher, the editors and the reviewers. Any product that may be evaluated in this article, or claim that may be made by its manufacturer, is not guaranteed or endorsed by the publisher.
Aceto, N., Bardia, A., Miyamoto, D. T., Donaldson, M. C., Wittner, B. S., Spencer, J. A., et al. (2014). Circulating Tumor Cell Clusters Are Oligoclonal Precursors of Breast Cancer Metastasis. Cell 158 (5), 1110–1122. doi:10.1016/j.cell.2014.07.013
Aceto, N. (2020). Bring along Your Friends: Homotypic and Heterotypic Circulating Tumor Cell Clustering to Accelerate Metastasis. Biomed. J. 43 (1), 18–23. doi:10.1016/j.bj.2019.11.002
Alix-Panabières, C., and Pantel, K. (2021). Liquid Biopsy: From Discovery to Clinical Application. Cancer Discov. 11 (4), 858–873. doi:10.1158/2159-8290.cd-20-1311
Ansari, D., Carvajo, M., Bauden, M., and Andersson, R. (2017). Pancreatic Cancer Stroma: Controversies and Current Insights. Scand. J. Gastroenterol. 52 (6-7), 641–646. doi:10.1080/00365521.2017.1293726
Ao, Z., Shah, S. H., Machlin, L. M., Parajuli, R., Miller, P. C., Rawal, S., et al. (2015). Identification of Cancer-Associated Fibroblasts in Circulating Blood from Patients with Metastatic Breast Cancer. Cancer Res. 75 (22), 4681–4687. doi:10.1158/0008-5472.can-15-1633
Arina, A., Idel, C., Hyjek, E. M., Alegre, M.-L., Wang, Y., Bindokas, V. P., et al. (2016). Tumor-associated Fibroblasts Predominantly Come from Local and Not Circulating Precursors. Proc. Natl. Acad. Sci. U.S.A. 113 (27), 7551–7556. doi:10.1073/pnas.1600363113
Arnoletti, J. P., Reza, J., Rosales, A., Monreal, A., Fanaian, N. i., Whisner, S., et al. (2022). Pancreatic Ductal Adenocarcinoma (PDAC) Circulating Tumor Cells Influence Myeloid Cell Differentiation to Support Their Survival and Immunoresistance in Portal Vein Circulation. PLoS One 17 (3), e0265725. doi:10.1371/journal.pone.0265725
Arnoletti, J. P., Zhu, X., Almodovar, A. J. O., Veldhuis, P. P., Sause, R., Griffith, E., et al. (2017). Portal Venous Blood Circulation Supports Immunosuppressive Environment and Pancreatic Cancer Circulating Tumor Cell Activation. Pancreas 46 (1), 116–123. doi:10.1097/mpa.0000000000000667
Awaji, M., and Singh, R. K. (2019). Cancer-Associated Fibroblasts' Functional Heterogeneity in Pancreatic Ductal Adenocarcinoma. Cancers (Basel) 11 (3), 290. doi:10.3390/cancers11030290
Bachem, M. G., Schünemann, M., Ramadani, M., Siech, M., Beger, H., Buck, A., et al. (2005). Pancreatic Carcinoma Cells Induce Fibrosis by Stimulating Proliferation and Matrix Synthesis of Stellate Cells. Gastroenterology 128 (4), 907–921. doi:10.1053/j.gastro.2004.12.036
Biffi, G., Oni, T. E., Spielman, B., Hao, Y., Elyada, E., Park, Y., et al. (2019). IL1-Induced JAK/STAT Signaling Is Antagonized by TGFβ to Shape CAF Heterogeneity in Pancreatic Ductal Adenocarcinoma. Cancer Discov. 9 (2), 282–301. doi:10.1158/2159-8290.cd-18-0710
Bochet, L., Lehuédé, C., Dauvillier, S., Wang, Y. Y., Dirat, B., Laurent, V., et al. (2013). Adipocyte-derived Fibroblasts Promote Tumor Progression and Contribute to the Desmoplastic Reaction in Breast Cancer. Cancer Res. 73 (18), 5657–5668. doi:10.1158/0008-5472.can-13-0530
Bockorny, B., Semenisty, V., Macarulla, T., Borazanci, E., Wolpin, B. M., Stemmer, S. M., et al. (2020). BL-8040, a CXCR4 Antagonist, in Combination with Pembrolizumab and Chemotherapy for Pancreatic Cancer: the COMBAT Trial. Nat. Med. 26 (6), 878–885. doi:10.1038/s41591-020-0880-x
Chen, D. S., and Mellman, I. (2017). Elements of Cancer Immunity and the Cancer-Immune Set Point. Nature 541 (7637), 321–330. doi:10.1038/nature21349
Chen, I. M., Willumsen, N., Dehlendorff, C., Johansen, A. Z., Jensen, B. V., Hansen, C. P., et al. (2020). Clinical Value of Serum Hyaluronan and Propeptide of Type III Collagen in Patients with Pancreatic Cancer. Int. J. Cancer 146 (10), 2913–2922. doi:10.1002/ijc.32751
Cheung, K. J., Padmanaban, V., Silvestri, V., Schipper, K., Cohen, J. D., Fairchild, A. N., et al. (2016). Polyclonal Breast Cancer Metastases Arise from Collective Dissemination of Keratin 14-expressing Tumor Cell Clusters. Proc. Natl. Acad. Sci. U. S. A. 113 (7), E854–E863. doi:10.1073/pnas.1508541113
De Jesus-Acosta, A., Sugar, E. A., O’Dwyer, P. J., Ramanathan, R. K., Von Hoff, D. D., Rasheed, Z., et al. (2020). Phase 2 Study of Vismodegib, a Hedgehog Inhibitor, Combined with Gemcitabine and Nab-Paclitaxel in Patients with Untreated Metastatic Pancreatic Adenocarcinoma. Br. J. Cancer 122 (4), 498–505. doi:10.1038/s41416-019-0683-3
Duda, D. G., Duyverman, A. M. M. J., Kohno, M., Snuderl, M., Steller, E. J. A., Fukumura, D., et al. (2010). Malignant Cells Facilitate Lung Metastasis by Bringing Their Own Soil. Proc. Natl. Acad. Sci. U.S.A. 107 (50), 21677–21682. doi:10.1073/pnas.1016234107
Dulauroy, S., Di Carlo, S. E., Langa, F., Eberl, G., and Peduto, L. (2012). Lineage Tracing and Genetic Ablation of ADAM12+ Perivascular Cells Identify a Major Source of Profibrotic Cells during Acute Tissue Injury. Nat. Med. 18 (8), 1262–1270. doi:10.1038/nm.2848
Effenberger, K. E., Schroeder, C., Hanssen, A., Wolter, S., Eulenburg, C., Tachezy, M., et al. (2018). Improved Risk Stratification by Circulating Tumor Cell Counts in Pancreatic Cancer. Clin. Cancer Res. 24 (12), 2844–2850. doi:10.1158/1078-0432.ccr-18-0120
Elahi-Gedwillo, K. Y., Carlson, M., Zettervall, J., and Provenzano, P. P. (2019). Antifibrotic Therapy Disrupts Stromal Barriers and Modulates the Immune Landscape in Pancreatic Ductal Adenocarcinoma. Cancer Res. 79 (2), 372–386. doi:10.1158/0008-5472.can-18-1334
Elyada, E., Bolisetty, M., Laise, P., Flynn, W. F., Courtois, E. T., Burkhart, R. A., et al. (2019). Cross-Species Single-Cell Analysis of Pancreatic Ductal Adenocarcinoma Reveals Antigen-Presenting Cancer-Associated Fibroblasts. Cancer Discov. 9 (8), 1102–1123. doi:10.1158/2159-8290.cd-19-0094
Erkan, M., Adler, G., Apte, M. V., Bachem, M. G., Buchholz, M., Detlefsen, S., et al. (2012). StellaTUM: Current Consensus and Discussion on Pancreatic Stellate Cell Research. Gut 61 (2), 172–178. doi:10.1136/gutjnl-2011-301220
Feig, C., Jones, J. O., Kraman, M., Wells, R. J. B., Deonarine, A., Chan, D. S., et al. (2013). Targeting CXCL12 from FAP-Expressing Carcinoma-Associated Fibroblasts Synergizes with Anti-PD-L1 Immunotherapy in Pancreatic Cancer. Proc. Natl. Acad. Sci. U.S.A. 110 (50), 20212–20217. doi:10.1073/pnas.1320318110
Ferreira, M. M., Ramani, V. C., and Jeffrey, S. S. (2016). Circulating Tumor Cell Technologies. Mol. Oncol. 10 (3), 374–394. doi:10.1016/j.molonc.2016.01.007
Franses, J. W., Basar, O., Kadayifci, A., Yuksel, O., Choz, M., Kulkarni, A. S., et al. (2018). Improved Detection of Circulating Epithelial Cells in Patients with Intraductal Papillary Mucinous Neoplasms. Oncologist 23 (1), 121–127. doi:10.1634/theoncologist.2017-0234
Gall, T. M. H., Belete, S., Khanderia, E., Frampton, A. E., and Jiao, L. R. (2019). Circulating Tumor Cells and Cell-Free DNA in Pancreatic Ductal Adenocarcinoma. The Am. J. of Pathology 189 (1), 71–81. doi:10.1016/j.ajpath.2018.03.020
Garcia, P. E., Scales, M. K., Allen, B. L., and Pasca di Magliano, M. (2020). Pancreatic Fibroblast Heterogeneity: From Development to Cancer. Cells 9 (11), 2464. doi:10.3390/cells9112464
Geng, X., Chen, H., Zhao, L., Hu, J., Yang, W., Li, G., et al. (2021). Cancer-Associated Fibroblast (CAF) Heterogeneity and Targeting Therapy of CAFs in Pancreatic Cancer. Front. Cell Dev. Biol. 9, 655152. doi:10.3389/fcell.2021.655152
Han, L., Chen, W., and Zhao, Q. (2014). Prognostic Value of Circulating Tumor Cells in Patients with Pancreatic Cancer: a Meta-Analysis. Tumor Biol. 35 (3), 2473–2480. doi:10.1007/s13277-013-1327-5
Hanahan, D., and Coussens, L. M. (2012). Accessories to the Crime: Functions of Cells Recruited to the Tumor Microenvironment. Cancer Cell 21 (3), 309–322. doi:10.1016/j.ccr.2012.02.022
Helms, E. J., Berry, M. W., Chaw, R. C., DuFort, C. C., Sun, D., Onate, M. K., et al. (2022). Mesenchymal Lineage Heterogeneity Underlies Nonredundant Functions of Pancreatic Cancer-Associated Fibroblasts. Cancer Discov. 12 (2), 484–501. doi:10.1158/2159-8290.cd-21-0601
Helms, E., Onate, M. K., and Sherman, M. H. (2020). Fibroblast Heterogeneity in the Pancreatic Tumor Microenvironment. Cancer Discov. 10 (5), 648–656. doi:10.1158/2159-8290.cd-19-1353
Hingorani, S. R., Harris, W. P., Beck, J. T., Berdov, B. A., Wagner, S. A., Pshevlotsky, E. M., et al. (2016). Phase Ib Study of PEGylated Recombinant Human Hyaluronidase and Gemcitabine in Patients with Advanced Pancreatic Cancer. Clin. Cancer Res. 22 (12), 2848–2854. doi:10.1158/1078-0432.ccr-15-2010
Hou, J.-M., Krebs, M. G., Lancashire, L., Sloane, R., Backen, A., Swain, R. K., et al. (2012). Clinical Significance and Molecular Characteristics of Circulating Tumor Cells and Circulating Tumor Microemboli in Patients with Small-Cell Lung Cancer. Jco 30 (5), 525–532. doi:10.1200/jco.2010.33.3716
Huang, H., and Brekken, R. A. (2020). Recent Advances in Understanding Cancer-Associated Fibroblasts in Pancreatic Cancer. Am. J. of Physiology-Cell Physiology 319 (2), C233–C243. doi:10.1152/ajpcell.00079.2020
Infante, J. R., Korn, R. L., Rosen, L. S., LoRusso, P., Dychter, S. S., Zhu, J., et al. (2018). Phase 1 Trials of PEGylated Recombinant Human Hyaluronidase PH20 in Patients with Advanced Solid Tumours. Br. J. Cancer 118 (2), 153–161. doi:10.1038/bjc.2017.327
Iwano, M., Plieth, D., Danoff, T. M., Xue, C., Okada, H., and Neilson, E. G. (2002). Evidence that Fibroblasts Derive from Epithelium during Tissue Fibrosis. J. Clin. Invest. 110 (3), 341–350. doi:10.1172/jci0215518
Jacobetz, M. A., Chan, D. S., Neesse, A., Bapiro, T. E., Cook, N., Frese, K. K., et al. Hyaluronan Impairs Vascular Function and Drug Delivery in a Mouse Model of Pancreatic Cancer. Gut. 62 (1), 112–120. doi:10.1136/gutjnl-2012-302529
Jacobetz, M. A., Chan, D. S., Neesse, A., Bapiro, T. E., Cook, N., Frese, K. K., et al. (2013). Hyaluronan Impairs Vascular Function and Drug Delivery in a Mouse Model of Pancreatic Cancer. Gut 62 (1), 112–120. doi:10.1136/gutjnl-2012-302529
Jiang, B., Zhou, L., Lu, J., Wang, Y., Liu, C., You, L., et al. (2020). Stroma-Targeting Therapy in Pancreatic Cancer: One Coin with Two Sides? Front. Oncol. 10, 576399. doi:10.3389/fonc.2020.576399
Jones, M. L., Siddiqui, J., Pienta, K. J., and Getzenberg, R. H. (2013). Circulating Fibroblast-like Cells in Men with Metastatic Prostate Cancer. Prostate 73 (2), 176–181. doi:10.1002/pros.22553
Kalluri, R., and Neilson, E. G. (2003). Epithelial-mesenchymal Transition and its Implications for Fibrosis. J. Clin. Invest. 112 (12), 1776–1784. doi:10.1172/jci200320530
Kalluri, R. (2016). The Biology and Function of Fibroblasts in Cancer. Nat. Rev. Cancer 16 (9), 582–598. doi:10.1038/nrc.2016.73
Kalluri, R., and Weinberg, R. A. (2009). The Basics of Epithelial-Mesenchymal Transition. J. Clin. Invest. 119 (6), 1420–1428. doi:10.1172/jci39104
Knopf, J. D., Tholen, S., Koczorowska, M. M., De Wever, O., Biniossek, M. L., and Schilling, O. (2015). The Stromal Cell-Surface Protease Fibroblast Activation Protein-α Localizes to Lipid Rafts and Is Recruited to Invadopodia. Biochim. Biophys. Acta 1853 (10 Pt A), 2515–2525. doi:10.1016/j.bbamcr.2015.07.013
Kobayashi, H., Enomoto, A., Woods, S. L., Burt, A. D., Takahashi, M., and Worthley, D. L. (2019). Cancer-associated Fibroblasts in Gastrointestinal Cancer. Nat. Rev. Gastroenterol. Hepatol. 16 (5), 282–295. doi:10.1038/s41575-019-0115-0
Kuehn, B. M. (2020). Looking to Long-Term Survivors for Improved Pancreatic Cancer Treatment. JAMA 324 (22), 2242–2244. doi:10.1001/jama.2020.21717
Leca, J., Martinez, S., Lac, S., Nigri, J., Secq, V., Rubis, M., et al. (2016). Cancer-associated Fibroblast-Derived Annexin A6+ Extracellular Vesicles Support Pancreatic Cancer Aggressiveness. J. Clin. Invest. 126 (11), 4140–4156. doi:10.1172/jci87734
Lee, J. S., Park, S. S., Lee, Y. K., Norton, J. A., and Jeffrey, S. S. (2019). Liquid Biopsy in Pancreatic Ductal Adenocarcinoma: Current Status of Circulating Tumor Cells and Circulating tumorDNA. Mol. Oncol. 13 (8), 1623–1650. doi:10.1002/1878-0261.12537
Liu, X., Taftaf, R., Kawaguchi, M., Chang, Y.-F., Chen, W., Entenberg, D., et al. (2019). Homophilic CD44 Interactions Mediate Tumor Cell Aggregation and Polyclonal Metastasis in Patient-Derived Breast Cancer Models. Cancer Discov. 9 (1), 96–113. doi:10.1158/2159-8290.cd-18-0065
Lo, A., Li, C. P., Buza, E. L., Blomberg, R., Govindaraju, P., Avery, D., et al. (2017). Fibroblast Activation Protein Augments Progression and Metastasis of Pancreatic Ductal Adenocarcinoma. JCI Insight 2 (19), e92232. doi:10.1172/jci.insight.92232
Ma, X.-L., Li, Y.-Y., Zhang, J., Huang, J.-W., Jia, H.-Y., Liu, L., et al. (2014). Prognostic Role of Circulating Tumor Cells in Patients with Pancreatic Cancer: a Meta-Analysis. Asian Pac. J. of Cancer Prev. 15 (15), 6015–6020. doi:10.7314/apjcp.2014.15.15.6015
Mace, T. A., Shakya, R., Pitarresi, J. R., Swanson, B., McQuinn, C. W., Loftus, S., et al. (2018). IL-6 and PD-L1 Antibody Blockade Combination Therapy Reduces Tumour Progression in Murine Models of Pancreatic Cancer. Gut 67 (2), 320–332. doi:10.1136/gutjnl-2016-311585
Malvezzi, M., Carioli, G., Bertuccio, P., Boffetta, P., Levi, F., La Vecchia, C., et al. (2019). European Cancer Mortality Predictions for the Year 2019 with Focus on Breast Cancer. Ann. of Oncol. 30 (5), 781–787. doi:10.1093/annonc/mdz051
Martini, V., Timme-Bronsert, S., Fichtner-Feigl, S., Hoeppner, J., and Kulemann, B. (2019). Circulating Tumor Cells in Pancreatic Cancer: Current Perspectives. Cancers (Basel) 11 (11), 1659. doi:10.3390/cancers11111659
Mizutani, Y., Kobayashi, H., Iida, T., Asai, N., Masamune, A., Hara, A., et al. (2019). Meflin-Positive Cancer-Associated Fibroblasts Inhibit Pancreatic Carcinogenesis. Cancer Res. 79 (20), 5367–5381. doi:10.1158/0008-5472.can-19-0454
Moir, J. A. G., Mann, J., and White, S. A. (2015). The Role of Pancreatic Stellate Cells in Pancreatic Cancer. Surg. Oncol. 24 (3), 232–238. doi:10.1016/j.suronc.2015.05.002
Neuzillet, C., Tijeras‐Raballand, A., Ragulan, C., Cros, J., Patil, Y., Martinet, M., et al. (2019). Inter‐ and Intra‐tumoural Heterogeneity in Cancer‐associated Fibroblasts of Human Pancreatic Ductal Adenocarcinoma. J. Pathol. 248 (1), 51–65. doi:10.1002/path.5224
Nissen, N. I., Kehlet, S., Johansen, A. Z., Chen, I. M., Karsdal, M., Johansen, J. S., et al. (2021). Noninvasive Prognostic Biomarker Potential of Quantifying the Propeptides of Type XI Collagen Alpha‐1 Chain ( PRO‐C11 ) in Patients with Pancreatic Ductal Adenocarcinoma. Int. J. Cancer 149 (1), 228–238. doi:10.1002/ijc.33551
Nurmik, M., Ullmann, P., Rodriguez, F., Haan, S., and Letellier, E. (2020). In Search of Definitions: Cancer‐associated Fibroblasts and Their Markers. Int. J. Cancer 146 (4), 895–905. doi:10.1002/ijc.32193
Ogier, C., Colombo, P.-E., Bousquet, C., Canterel-Thouennon, L., Sicard, P., Garambois, V., et al. (2018). Targeting the NRG1/HER3 Pathway in Tumor Cells and Cancer-Associated Fibroblasts with an Anti-neuregulin 1 Antibody Inhibits Tumor Growth in Pre-clinical Models of Pancreatic Cancer. Cancer Lett. 432, 227–236. doi:10.1016/j.canlet.2018.06.023
Öhlund, D., Handly-Santana, A., Biffi, G., Elyada, E., Almeida, S. A., Ponz-Sarvise, M., et al. (2017). Distinct Populations of Inflammatory Fibroblasts and Myofibroblasts in Pancreatic Cancer. J. Exp. Med. 214 (3), 579–596. doi:10.1084/jem.20162024
Okada, S., Okusaka, T., Ishii, H., Kyogoku, A., Yoshimori, M., Kajimura, N., et al. (1998). Elevated Serum Interleukin-6 Levels in Patients with Pancreatic Cancer. Jpn. J. of Clin. Oncol. 28 (1), 12–15. doi:10.1093/jjco/28.1.12
Ortiz-Otero, N., Clinch, A. B., Hope, J., Wang, W., Reinhart-King, C. A., and King, M. R. (2020). Cancer Associated Fibroblasts Confer Shear Resistance to Circulating Tumor Cells during Prostate Cancer Metastatic Progression. Oncotarget 11 (12), 1037–1050. doi:10.18632/oncotarget.27510
Ortiz-Otero, N., Marshall, J. R., Lash, B., and King, M. R. (2020). Chemotherapy-induced Release of Circulating-Tumor Cells into the Bloodstream in Collective Migration Units with Cancer-Associated Fibroblasts in Metastatic Cancer Patients. BMC Cancer 20 (1), 873. doi:10.1186/s12885-020-07376-1
Özdemir, B. C., Pentcheva-Hoang, T., Carstens, L. J., Zheng, X., Wu, C-C., Simpson, R. V., et al. (2014). Depletion of Carcinoma-Associated Fibroblasts and Fibrosis Induces Immunosuppression and Accelerates Pancreas Cancer with Reduced Survival. Cancer Cell 25 (6), 719–734. doi:10.1016/j.ccr.2014.04.005
Pantel, K., and Alix-Panabières, C. (2019). Liquid Biopsy and Minimal Residual Disease - Latest Advances and Implications for Cure. Nat. Rev. Clin. Oncol. 16 (7), 409–424. doi:10.1038/s41571-019-0187-3
Pereira, B. A., Vennin, C., Papanicolaou, M., Chambers, C. R., Herrmann, D., Morton, J. P., et al. (2019). CAF Subpopulations: A New Reservoir of Stromal Targets in Pancreatic Cancer. Trends Cancer 5 (11), 724–741. doi:10.1016/j.trecan.2019.09.010
Quante, M., Tu, S. P., Tomita, H., Gonda, T., Wang, S. S. W., Takashi, S., et al. (2011). Bone Marrow-Derived Myofibroblasts Contribute to the Mesenchymal Stem Cell Niche and Promote Tumor Growth. Cancer Cell 19 (2), 257–272. doi:10.1016/j.ccr.2011.01.020
Rawla, P., Sunkara, T., and Gaduputi, V. (2019). Epidemiology of Pancreatic Cancer: Global Trends, Etiology and Risk Factors. World J. Oncol. 10 (1), 10–27. doi:10.14740/wjon1166
Ren, B., Cui, M., Yang, G., Wang, H., Feng, M., You, L., et al. (2018). Tumor Microenvironment Participates in Metastasis of Pancreatic Cancer. Mol. Cancer 17 (1), 108. doi:10.1186/s12943-018-0858-1
Rhim, A. D., Oberstein, P. E., Thomas, D. H., Mirek, E. T., Palermo, C. F., Sastra, S. A., et al. (2014). Stromal Elements Act to Restrain, rather Than Support, Pancreatic Ductal Adenocarcinoma. Cancer Cell 25 (6), 735–747. doi:10.1016/j.ccr.2014.04.021
Rupert, J. E., Narasimhan, A., Jengelley, D. H. A., Jiang, Y., Liu, J., Au, E., et al. (2021). Tumor-derived IL-6 and Trans-signaling Among Tumor, Fat, and Muscle Mediate Pancreatic Cancer Cachexia. J. Exp. Med. 218 (6), e20190450. doi:10.1084/jem.20190450
Sahai, E., Astsaturov, I., Cukierman, E., DeNardo, D. G., Egeblad, M., Evans, R. M., et al. (2020). A Framework for Advancing Our Understanding of Cancer-Associated Fibroblasts. Nat. Rev. Cancer 20 (3), 174–186. doi:10.1038/s41568-019-0238-1
Sharma, U., Medina-Saenz, K., Miller, P. C., Troness, B., Spartz, A., Sandoval-Leon, A., et al. (2021). Heterotypic Clustering of Circulating Tumor Cells and Circulating Cancer-Associated Fibroblasts Facilitates Breast Cancer Metastasis. Breast Cancer Res. Treat. 189 (1), 63–80. doi:10.1007/s10549-021-06299-0
Shi, Y., Gao, W., Lytle, N. K., Huang, P., Yuan, X., Dann, A. M., et al. (2019). Targeting LIF-Mediated Paracrine Interaction for Pancreatic Cancer Therapy and Monitoring. Nature 569 (7754), 131–135. doi:10.1038/s41586-019-1130-6
Song, B. G., Kwon, W., Kim, H., Lee, E. M., Han, Y. M., Kim, H., et al. (2020). Detection of Circulating Tumor Cells in Resectable Pancreatic Ductal Adenocarcinoma: A Prospective Evaluation as a Prognostic Marker. Front. Oncol. 10, 616440. doi:10.3389/fonc.2020.616440
Sung, H., Ferlay, J., Siegel, R. L., Laversanne, M., Soerjomataram, I., Jemal, A., et al. (2021). Global Cancer Statistics 2020: GLOBOCAN Estimates of Incidence and Mortality Worldwide for 36 Cancers in 185 Countries. CA A Cancer J. Clin. 71 (3), 209–249. doi:10.3322/caac.21660
Taftaf, R., Liu, X., Singh, S., Jia, Y., Dashzeveg, N. K., Hoffmann, A. D., et al. (2021). ICAM1 Initiates CTC Cluster Formation and Trans-endothelial Migration in Lung Metastasis of Breast Cancer. Nat. Commun. 12 (1), 4867. doi:10.1038/s41467-021-25189-z
Van Cutsem, E., Tempero, M. A., Sigal, D., Oh, D.-Y., Fazio, N., Macarulla, T., et al. (2020). Randomized Phase III Trial of Pegvorhyaluronidase Alfa with Nab-Paclitaxel Plus Gemcitabine for Patients with Hyaluronan-High Metastatic Pancreatic Adenocarcinoma. Jco 38 (27), 3185–3194. doi:10.1200/jco.20.00590
von Ahrens, D., Bhagat, T. D., Nagrath, D., Maitra, A., and Verma, A. (2017). The Role of Stromal Cancer-Associated Fibroblasts in Pancreatic Cancer. J. Hematol. Oncol. 10 (1), 76. doi:10.1186/s13045-017-0448-5
Von Hoff, D. D., Ervin, T., Arena, F. P., Chiorean, E. G., Infante, J., Moore, M., et al. (2013). Increased Survival in Pancreatic Cancer with Nab-Paclitaxel Plus Gemcitabine. N. Engl. J. Med. 369 (18), 1691–1703. doi:10.1056/NEJMoa1304369
Von Hoff, D. D., Ramanathan, R. K., Borad, M. J., Laheru, D. A., Smith, L. S., Wood, T. E., et al. (2011). Gemcitabine Plusnab-Paclitaxel Is an Active Regimen in Patients with Advanced Pancreatic Cancer: A Phase I/II Trial. Jco 29 (34), 4548–4554. doi:10.1200/jco.2011.36.5742
Vonlaufen, A., Phillips, P. A., Xu, Z., Goldstein, D., Pirola, R. C., Wilson, J. S., et al. (2008). Pancreatic Stellate Cells and Pancreatic Cancer Cells: An Unholy Alliance: Figure 1. Cancer Res. 68 (19), 7707–7710. doi:10.1158/0008-5472.can-08-1132
Wang, S., Zheng, Y., Yang, F., Zhu, L., Zhu, X.-Q., Wang, Z.-F., et al. (2021). The Molecular Biology of Pancreatic Adenocarcinoma: Translational Challenges and Clinical Perspectives. Sig Transduct. Target Ther. 6 (1), 249. doi:10.1038/s41392-021-00659-4
Whatcott, C. J., Diep, C. H., Jiang, P., Watanabe, A., LoBello, J., Sima, C., et al. (2015). Desmoplasia in Primary Tumors and Metastatic Lesions of Pancreatic Cancer. Clin. Cancer Res. 21 (15), 3561–3568. doi:10.1158/1078-0432.ccr-14-1051
White, M. G., Lee, A., Vicente, D., Hall, C., Kim, M. P., Katz, M. H. G., et al. (2021). Measurement of Portal Vein Blood Circulating Tumor Cells Is Safe and May Correlate with Outcomes in Resected Pancreatic Ductal Adenocarcinoma. Ann. Surg. Oncol. 28 (8), 4615–4622. doi:10.1245/s10434-020-09518-y
Willumsen, N., Ali, S. M., Leitzel, K., Drabick, J. J., Yee, N., Polimera, H. V., et al. (2019). Collagen Fragments Quantified in Serum as Measures of Desmoplasia Associate with Survival Outcome in Patients with Advanced Pancreatic Cancer. Sci. Rep. 9 (1), 19761. doi:10.1038/s41598-019-56268-3
Willumsen, N., Bager, C. L., Leeming, D. J., Smith, V., Karsdal, M. A., Dornan, D., et al. (2013). Extracellular Matrix Specific Protein Fingerprints Measured in Serum Can Separate Pancreatic Cancer Patients from Healthy Controls. BMC Cancer 13 (1), 554. doi:10.1186/1471-2407-13-554
Wrenn, E., Huang, Y., and Cheung, K. (2021). Collective Metastasis: Coordinating the Multicellular Voyage. Clin. Exp. Metastasis 38 (4), 373–399. doi:10.1007/s10585-021-10111-0
Wu, G., Zhu, R., Li, Y., Zhao, Y., and Dai, M. (2018). Prognostic Significance of Circulating Tumor Microemboli in Patients with Pancreatic Ductal Adenocarcinoma. Oncol. Lett. 15 (5), 7376–7382. doi:10.3892/ol.2018.8264
Yeo, D., Bastian, A., Strauss, H., Saxena, P., Grimison, P., and Rasko, J. E. J. (2022). Exploring the Clinical Utility of Pancreatic Cancer Circulating Tumor Cells. Int. J. Mol. Sci. 23 (3), 1671. doi:10.3390/ijms23031671
Keywords: pancreatic cancer, liquid biopsy, cancer-associated fibroblasts (CAFs), tumor microenvironment, circulating tumor cells (CTCs)
Citation: Götze J, Nitschke C, Uzunoglu FG, Pantel K, Sinn M and Wikman H (2022) Tumor-Stroma Interaction in PDAC as a New Approach for Liquid Biopsy and its Potential Clinical Implications. Front. Cell Dev. Biol. 10:918795. doi: 10.3389/fcell.2022.918795
Received: 12 April 2022; Accepted: 05 May 2022;
Published: 26 May 2022.
Edited by:
Roberto Piñeiro, Health Research Institute of Santiago de Compostela (IDIS), SpainReviewed by:
Richard Beatson, University College London, United KingdomCopyright © 2022 Götze, Nitschke, Uzunoglu, Pantel, Sinn and Wikman. This is an open-access article distributed under the terms of the Creative Commons Attribution License (CC BY). The use, distribution or reproduction in other forums is permitted, provided the original author(s) and the copyright owner(s) are credited and that the original publication in this journal is cited, in accordance with accepted academic practice. No use, distribution or reproduction is permitted which does not comply with these terms.
*Correspondence: Harriet Wikman, aC53aWttYW5AdWtlLmRl
Disclaimer: All claims expressed in this article are solely those of the authors and do not necessarily represent those of their affiliated organizations, or those of the publisher, the editors and the reviewers. Any product that may be evaluated in this article or claim that may be made by its manufacturer is not guaranteed or endorsed by the publisher.
Research integrity at Frontiers
Learn more about the work of our research integrity team to safeguard the quality of each article we publish.