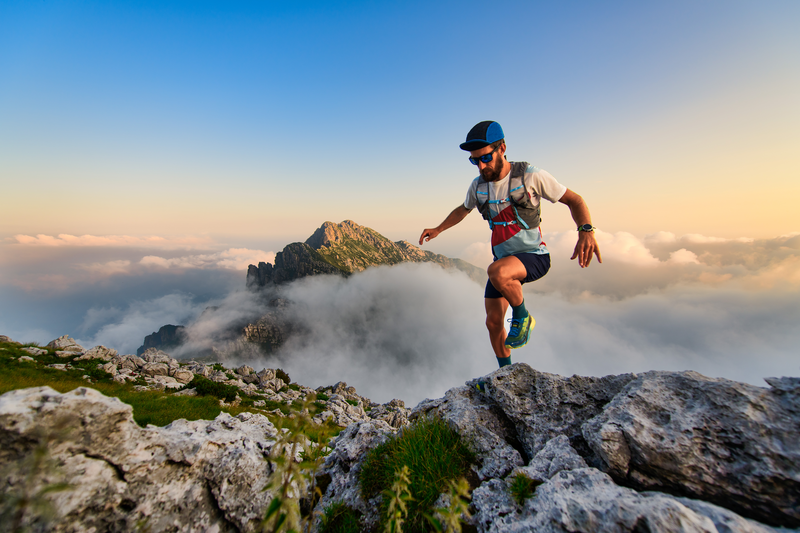
94% of researchers rate our articles as excellent or good
Learn more about the work of our research integrity team to safeguard the quality of each article we publish.
Find out more
OPINION article
Front. Cell Dev. Biol. , 27 April 2022
Sec. Membrane Traffic and Organelle Dynamics
Volume 10 - 2022 | https://doi.org/10.3389/fcell.2022.896618
This article is part of the Research Topic Beclin 1 and Autophagy---in Memory of Beth Levine (1960-2020) View all 5 articles
The prevalence of severe acute respiratory syndrome coronavirus 2 (SARS-CoV-2) infection and the COVID-19 pandemic outbreak are causing overwhelming public health disasters worldwide. At the beginning of the pandemic outbreak, studies in cell cultures revealed that chloroquine and its derivatives, which are commonly used as autophagy inhibitors, significantly suppressed SARS-CoV-2 infection and replication (Liu et al., 2020; Wang et al., 2020). Although the lack of observed benefit in clinical COVID-19 therapies was confirmed through phase III clinical investigation (Borba et al., 2020; Tang et al., 2020), the importance of autophagy during SARS-CoV-2 infection and autophagy-related therapeutic strategies for developing COVID-19 treatment have been generally accepted.
Studies have revealed that SARS-CoV-2 infection hijacks autophagy machinery through different mechanisms (Zhang et al., 2021a; Hayn et al., 2021; Qu et al., 2021; Shang et al., 2021). Different viral proteins activate early autophagy or block late autophagy and autophagic flux and thus lead to the accumulation of autophagosomes, which play essential roles in viral replication and virion egress. Importantly, the mobility group box 1 (HMGB1) is a Beclin1-binding protein and acts as an autophagy effector (Tang et al., 2010), the viral protein ORF3a activates autophagy initiation through HMGB1- and UVRAG-mediated Beclin1 pathways (Qu et al., 2021; Zhang et al., 2022), while inhibiting autophagosome-lysosome fusion and autolysosome maturation by disrupting the VPS39 and HOPS complex (Zhang et al., 2021b; Miao et al., 2021). In summary, ORF3a selectively induces incomplete autophagy during SARS-CoV-2 infection. Interestingly, the homolog ORF3a in SARS-CoV-1 fails to induce autophagy (Qu et al., 2021; Zhang et al., 2022), indicating that this mechanism of autophagy induction specifically occurs during SARS-CoV-2 infection but is not common in other coronavirus infections such as SARS-CoV-1.
As the critical site for cellular and viral protein synthesis inside cells, the endoplasmic reticulum (ER) plays the essential roles in SARS-CoV-2 infection and replication. Cellular protein synthesis is impaired by several viral proteins such as Nsp1 and Nsp14 through multiple strategies, and then efficient viral translation and evasion of innate defenses are enabled through inhibiting cellular translation (Schubert et al., 2020; Finkel et al., 2021; Hsu et al., 2021), and viral proteins rush into the ER compartment and support viral replication, virion assembly and transport. Our recent study showed that SARS-CoV-2 ORF3a localizes to the ER compartment, induces HMGB1 translocation from the nucleus and then recruits HMGB1 to the ER compartment, subsequently ORF3a promotes HMGB1-Beclin1 association and induces autophagy through Beclin1-dependent pathway. Therefore, ORF3a triggers reticulophagy regulator 1 (RETREG1)/FAM134B-mediated autophagy of the endoplasmic reticulum (reticulophagy) during SARS-CoV-2 infection (Figure 1) (Zhang et al., 2022). The degradation of ER membrane proteins is enhanced by ORF3a expression and SARS-CoV-2 infection, while the turnover of mitochondrial membrane proteins is not affected, indicating that ORF3a selectively triggers reticulophagy but not mitophagy during SARS-CoV-2 infection. Mitophagy is triggered by ORF10 and M overexpression, which are localized to mitochondria, to induce MAVS degradation (Li et al., 2022) and suppress antiviral immune responses (Hui et al., 2021) during SARS-CoV-2 infection. In contrast, galectin-8, one of cytosolic lectins, acts as a pattern and/or danger recognition receptor for intracellular pathogens and mediates selective autophagy against bacterial and viral infection (Thurston et al., 2012; Montespan et al., 2017), galectin-8 senses highly glycosylated viral proteins, such as SARS-CoV-2 spike, and then triggers antiviral xenophagy or virophagy, while SARS-CoV-2-encoded 3CLpro cleaves galectin-8 and the adaptor FYCO1 and thus disrupts xenophagy to evade antiviral autophagy (Pablos et al., 2021). These findings indicate that pro-viral autophagy is induced while antiviral autophagy is inhibited through different mechanisms during SARS-CoV-2 infection and replication. Because several viral proteins can also regulate autophagy through other mechanisms, such as ORF3a interacting with heme oxygenase 1 (HMOX1) and probably regulating autophagy under oxidative stress (Zhang et al., 2022), it is interesting to further investigate whether other kinds of autophagy are regulated by SARS-CoV-2 infection.
FIGURE 1. SARS-CoV-2 ORF3a induces reticulophagy and reprograms ER homeostasis. During SARS-CoV-2 infection and replication, ORF3a interacts with HMGB1 and recruits its translocation from nucleus to ER and then promotes HMGB1-Beclin1 association and RETREG1/FAM134B-mediated reticulophagy. As results ① ER integrated proteins and fragments are degraded and recycled through reticulophagy, and ② Nsp3-enriched ER regions are engulfed to form the double-membrane viral replication organelles probably through autophagy-like mechanism and then facilitates viral replication. Consequently ③ reticulophagy induces ER stress, and then triggers inflammatory gene expression and early caspase-12-mediated apoptotic phenotype.
ER stress is induced by ORF3a expression and SARS-CoV-2 infection through HMGB1-dependent RETREG1 -mediated reticulophagy, subsequently triggering acute inflammatory responses and acquisition of an early ER apoptotic phenotype. These results indicate that ORF3a-induced reticulophagy promotes ER turnover and reprograms ER homeostasis during SARS-CoV-2 infection, probably to attenuate inappropriate ER responses to overloaded or unfolded viral proteins, recover from ER stress or repair ER damage. Many viral proteins primarily induce the unfolded protein response (UPR) and ER stress and secondarily trigger autophagy (Sharma et al., 2017; Yuan et al., 2017; Lee et al., 2018); however, ORF3a and SARS-CoV-2 infection induce reticulophagy in a different way. Our studies have excluded the possibility that ORF3a induces reticulophagy in a UPR- or ER stress-dependent manner. The truncated ORF3a construct that fails to interact with HMGB1 fails to induce reticulophagy and ER stress, and ORF3a interaction with HMGB1 alone or ORF3a localization to the ER membrane alone was not sufficient for autophagy induction. On the other hand, FAM134B-mediated reticulophagy is required for ORF3a-induced ER stress, while the activation of ER stress, at least the activating transcription factor 4 (ATF4)-CCAAT/enhancer-binding protein homologous protein (CHOP) arm, is not essential for the autophagy induced by ORF3a overexpression. These results confirmed that ORF3a primarily induced reticulophagy through the HMGB1-ORF3a interaction and ER membrane localization and then secondarily induced ER stress through reticulophagy (Zhang et al., 2022).
Autophagy may exert either pro-viral or antiviral effects during different viral infection. Studies have revealed that autophagy induction is essential for the infection and replication of SARS-CoV-2 and coronaviruses (Maier and Britton, 2012; Carmona-Gutierrez et al., 2020; Miller et al., 2020). HMGB1 depletion or RETREG1 depletion significantly suppresses the level of SARS-CoV-2 gene expression, indicating that HMGB1-mediated reticulophagy is essential for SARS-CoV-2 infection or replication. Why does the replication of SARS-CoV-2 and coronaviruses require autophagy and reticulophagy? First, coronaviruses replicate in double-membrane vacuoles named viral replication organelles (RO) (Wolff et al., 2020a), which are likely autophagy-related vacuoles or are generated through autophagy-like machinery. As reported by several groups, ORF3a expression and SARS-CoV-2 infection trigger incomplete autophagy (Zhang et al., 2021b; Miao et al., 2021; Qu et al., 2021; Su et al., 2021) and then cause the accumulation of autophagosomes and immature autolysosomes, which may promote RO formation or provide membrane sources for the double-membrane vacuoles. Second, several viral proteins are localized in the ER compartment (Santerre et al., 2021) and remodel ER morphology to facilitate viral assembly and transport. Third, reticulophagy and ER stress may exert pro-viral responses to promote viral replication and assembly (Zhang et al., 2022). Finally, recent studies have shown that SARS-CoV-2 ORF3a blocks autophagosome-lysosome fusion and promotes viral egress through lysosomal exocytosis (Chen et al., 2021; Miao et al., 2021), emphasizing that the subversion of autophagic or lysosomal vacuole translocation is important for virion transport and release. These findings indicate that autophagy and autophagy-related vacuoles play essential roles in the replication of SARS-CoV-2 and coronaviruses through multiple mechanisms.
Interestingly, the induction of reticulophagy by SARS-CoV-2 infection is opposite to that of flaviviruses (e.g., Zika). Zika virus NS2B3 cleaves RETREG1/FAM134B to suppress reticulophagy and remodel ER morphology and then establishes ER-localized viral replication compartments (Lennemann and Coyne, 2017; Evans et al., 2020), which are mainly derived from the ER through the formation of intermediate single-membrane structures (Wolff et al., 2020b). In contrast, SARS-CoV-2 directly induced the formation of double-membrane RO structures derived from the ER, probably through an autophagy-like mechanism, and then replicated in this kind of autophagosome-like vacuole (Knoops et al., 2008; Wolff et al., 2020a). Therefore, reticulophagy and ER turnover exhibit the opposite effects in the replication of SARS-CoV-2 and Zika virus. However, it remains unknown whether reticulophagy is commonly induced by infection with coronaviruses for the formation of viral replication organelles.
In summary, SARS-CoV-2 infection induces RETREG1-mediated reticulophagy through ORF3a interacting and recruiting HMGB1-Beclin1 complexes to the ER compartment and then reprograms ER homeostasis, probably through both ER morphology and responses to ER stress, to facilitate viral replication and trigger the proinflammatory responses. These findings represent novel insights into the induction and function of reticulophagy in SARS-CoV-2 infection and provide important targets for developing autophagy-related COVID-19 treatment.
XL and EK designed the article and wrote the manuscript.
This work is supported by grants from the National Natural Science Foundation of China (81871643 and 32061143008) to EK and the National Natural Science Foundation of China (81971928) to XL.
The authors declare that the research was conducted in the absence of any commercial or financial relationships that could be construed as a potential conflict of interest.
All claims expressed in this article are solely those of the authors and do not necessarily represent those of their affiliated organizations, or those of the publisher, the editors and the reviewers. Any product that may be evaluated in this article, or claim that may be made by its manufacturer, is not guaranteed or endorsed by the publisher.
Borba, M. G. S., Val, F. F. A., Sampaio, V. S., Alexandre, M. A. A., Melo, G. C., Brito, M., et al. (2020). Effect of High vs Low Doses of Chloroquine Diphosphate as Adjunctive Therapy for Patients Hospitalized with Severe Acute Respiratory Syndrome Coronavirus 2 (SARS-CoV-2) Infection. JAMA Netw. Open 3 (4), e208857. doi:10.1001/jamanetworkopen.2020.8857
Carmona-Gutierrez, D., Bauer, M. A., Zimmermann, A., Kainz, K., Hofer, S. J., Kroemer, G., et al. (2020). Digesting the Crisis: Autophagy and Coronaviruses. Mic 7 (5), 119–128. doi:10.15698/mic2020.05.715
Chen, D., Zheng, Q., Sun, L., Ji, M., Li, Y., Deng, H., et al. (2021). ORF3a of SARS-CoV-2 Promotes Lysosomal Exocytosis-Mediated Viral Egress. Developmental Cel 56 (23), 3250–3263. doi:10.1016/j.devcel.2021.10.006
Evans, A. S., Lennemann, N. J., and Coyne, C. B. (2020). BPIFB3 Regulates Endoplasmic Reticulum Morphology to Facilitate Flavivirus Replication. J. Virol. 94 (9). doi:10.1128/JVI.00029-20
Finkel, Y., Gluck, A., Nachshon, A., Winkler, R., Fisher, T., Rozman, B., et al. (2021). SARS-CoV-2 Uses a Multipronged Strategy to Impede Host Protein Synthesis. Nature 594 (7862), 240–245. doi:10.1038/s41586-021-03610-3
Hayn, M., Hirschenberger, M., Koepke, L., Nchioua, R., Straub, J. H., Klute, S., et al. (2021). Systematic Functional Analysis of SARS-CoV-2 Proteins Uncovers Viral Innate Immune Antagonists and Remaining Vulnerabilities. Cel Rep. 35 (7), 109126. doi:10.1016/j.celrep.2021.109126
Hsu, J. C., Laurent-Rolle, M., Pawlak, J. B., Wilen, C. B., and Cresswell, P. (2021). Translational Shutdown and Evasion of the Innate Immune Response by SARS-CoV-2 NSP14 Protein. Proc. Natl. Acad. Sci. U S A. 118 (24). doi:10.1073/pnas.2101161118
Hui, X., Zhang, L., Cao, L., Huang, K., Zhao, Y., Zhang, Y., et al. (2021). SARS-CoV-2 Promote Autophagy to Suppress Type I Interferon Response. Sig Transduct Target. Ther. 6 (1), 180. doi:10.1038/s41392-021-00574-8
Knoops, K., Kikkert, M., Worm, S. H. E. v. d., Zevenhoven-Dobbe, J. C., van der Meer, Y., Koster, A. J., et al. (2008). SARS-coronavirus Replication Is Supported by a Reticulovesicular Network of Modified Endoplasmic Reticulum. Plos Biol. 6 (9), e226. doi:10.1371/journal.pbio.0060226
Lee, Y.-R., Kuo, S.-H., Lin, C.-Y., Fu, P.-J., Lin, Y.-S., Yeh, T.-M., et al. (2018). Dengue Virus-Induced ER Stress Is Required for Autophagy Activation, Viral Replication, and Pathogenesis Both In Vitro and In Vivo. Sci. Rep. 8 (1), 489. doi:10.1038/s41598-017-18909-3
Lennemann, N. J., and Coyne, C. B. (2017). Dengue and Zika Viruses Subvert Reticulophagy by NS2B3-Mediated Cleavage of FAM134B. Autophagy 13 (2), 322–332. doi:10.1080/15548627.2016.1265192
Li, X., Hou, P., Ma, W., Wang, X., Wang, H., Yu, Z., et al. (2022). SARS-CoV-2 ORF10 Suppresses the Antiviral Innate Immune Response by Degrading MAVS through Mitophagy. Cell Mol Immunol 19 (1), 67–78. doi:10.1038/s41423-021-00807-4
Liu, J., Cao, R., Xu, M., Wang, X., Zhang, H., Hu, H., et al. (2020). Hydroxychloroquine, a Less Toxic Derivative of Chloroquine, Is Effective in Inhibiting SARS-CoV-2 Infection In Vitro. Cell Discov 6, 16. doi:10.1038/s41421-020-0156-0
Maier, H., and Britton, P. (2012). Involvement of Autophagy in Coronavirus Replication. Viruses 4 (12), 3440–3451. doi:10.3390/v4123440
Miao, G., Zhao, H., Li, Y., Ji, M., Chen, Y., Shi, Y., et al. (2021). ORF3a of the COVID-19 Virus SARS-CoV-2 Blocks HOPS Complex-Mediated Assembly of the SNARE Complex Required for Autolysosome Formation. Developmental Cel 56 (4), 427–442. doi:10.1016/j.devcel.2020.12.010
Miller, K., McGrath, M. E., Hu, Z., Ariannejad, S., Weston, S., Frieman, M., et al. (2020). Coronavirus Interactions with the Cellular Autophagy Machinery. Autophagy 16 (12), 2131–2139. doi:10.1080/15548627.2020.1817280
Montespan, C., Marvin, S. A., Austin, S., Burrage, A. M., Roger, B., Rayne, F., et al. (2017). Multi-layered Control of Galectin-8 Mediated Autophagy during Adenovirus Cell Entry through a Conserved PPxY Motif in the Viral Capsid. Plos Pathog. 13 (2), e1006217. doi:10.1371/journal.ppat.1006217
Pablos, I., Machado, Y., de Jesus, H. C. R., Mohamud, Y., Kappelhoff, R., Lindskog, C., et al. (2021). Mechanistic Insights into COVID-19 by Global Analysis of the SARS-CoV-2 3CLpro Substrate Degradome. Cel Rep. 37 (4), 109892. doi:10.1016/j.celrep.2021.109892
Qu, Y., Wang, X., Zhu, Y., Wang, W., Wang, Y., Hu, G., et al. (2021). ORF3a-Mediated Incomplete Autophagy Facilitates Severe Acute Respiratory Syndrome Coronavirus-2 Replication. Front. Cel Dev. Biol. 9, 716208. doi:10.3389/fcell.2021.716208
Santerre, M., Arjona, S. P., Allen, C. N., Shcherbik, N., and Sawaya, B. E. (2021). Why Do SARS-CoV-2 NSPs rush to the ER? J. Neurol. 268 (6), 2013–2022. doi:10.1007/s00415-020-10197-8
Schubert, K., Karousis, E. D., Jomaa, A., Scaiola, A., Echeverria, B., Gurzeler, L.-A., et al. (2020). SARS-CoV-2 Nsp1 Binds the Ribosomal mRNA Channel to Inhibit Translation. Nat. Struct. Mol. Biol. 27 (10), 959–966. doi:10.1038/s41594-020-0511-8
Shang, C., Zhuang, X., Zhang, H., Li, Y., Zhu, Y., Lu, J., et al. (2021). Inhibition of Autophagy Suppresses SARS-CoV-2 Replication and Ameliorates Pneumonia in hACE2 Transgenic Mice and Xenografted Human Lung Tissues. J. Virol. 95 (24), e0153721. doi:10.1128/JVI.01537-21
Sharma, M., Bhattacharyya, S., Sharma, K. B., Chauhan, S., Asthana, S., Abdin, M. Z., et al. (2017). Japanese Encephalitis Virus Activates Autophagy through XBP1 and ATF6 ER Stress Sensors in Neuronal Cells. J. Gen. Virol. 98 (5), 1027–1039. doi:10.1099/jgv.0.000792
Su, W. Q., Yu, X. J., and Zhou, C. M. (2021). SARS-CoV-2 ORF3a Induces Incomplete Autophagy via the Unfolded Protein Response. Viruses 13 (12). doi:10.3390/v13122467
Tang, D., Kang, R., Livesey, K. M., Cheh, C.-W., Farkas, A., Loughran, P., et al. (2010). Endogenous HMGB1 Regulates Autophagy. J. Cel Biol 190 (5), 881–892. doi:10.1083/jcb.200911078
Tang, W., Cao, Z., Han, M., Wang, Z., Chen, J., Sun, W., et al. (2020). Hydroxychloroquine in Patients with Mainly Mild to Moderate Coronavirus Disease 2019: Open Label, Randomised Controlled Trial. BMJ 369, m1849. doi:10.1136/bmj.m1849
Thurston, T. L. M., Wandel, M. P., von Muhlinen, N., Foeglein, Á., and Randow, F. (2012). Galectin 8 Targets Damaged Vesicles for Autophagy to Defend Cells against Bacterial Invasion. Nature 482 (7385), 414–418. doi:10.1038/nature10744
Wang, M., Cao, R., Zhang, L., Yang, X., Liu, J., Xu, M., et al. (2020). Remdesivir and Chloroquine Effectively Inhibit the Recently Emerged Novel Coronavirus (2019-nCoV) In Vitro. Cell Res 30 (3), 269–271. doi:10.1038/s41422-020-0282-0
Wolff, G., Limpens, R. W. A. L., Zevenhoven-Dobbe, J. C., Laugks, U., Zheng, S., de Jong, A. W. M., et al. (2020). A Molecular Pore Spans the Double Membrane of the Coronavirus Replication Organelle. Science 369 (6509), 1395–1398. doi:10.1126/science.abd3629
Wolff, G., Melia, C. E., Snijder, E. J., and Bárcena, M. (2020). Double-Membrane Vesicles as Platforms for Viral Replication. Trends Microbiol. 28 (12), 1022–1033. doi:10.1016/j.tim.2020.05.009
Yuan, P., Dong, L., Cheng, Q., Wang, S., Li, Z., Sun, Y., et al. (2017). Prototype Foamy Virus Elicits Complete Autophagy Involving the ER Stress-Related UPR Pathway. Retrovirology 14 (1), 16. doi:10.1186/s12977-017-0341-x
Zhang, X., Yang, Z., Pan, T., Long, X., Sun, Q., Wang, P.-H., et al. (2022). SARS-CoV-2 ORF3a Induces RETREG1/FAM134B-dependent Reticulophagy and Triggers Sequential ER Stress and Inflammatory Responses during SARS-CoV-2 Infection. Autophagy, 1–17. doi:10.1080/15548627.2022.2039992
Zhang, Y., Chen, Y., Li, Y., Huang, F., Luo, B., Yuan, Y., et al. (2021). The ORF8 Protein of SARS-CoV-2 Mediates Immune Evasion through Down-Regulating MHC-Iota. Proc. Natl. Acad. Sci. U S A. 118 (23), e2024202118. doi:10.1073/pnas.2024202118
Keywords: reticulophagy, SARS-CoV-2, replication, endoplasmic reticulum, ER stress
Citation: Li X and Kuang E (2022) Reticulophagy Reprograms the Endoplasmic Reticulum for SARS-CoV-2 Replication. Front. Cell Dev. Biol. 10:896618. doi: 10.3389/fcell.2022.896618
Received: 16 March 2022; Accepted: 11 April 2022;
Published: 27 April 2022.
Edited by:
Jorge E. Azevedo, Universidade do Porto, PortugalReviewed by:
Rafael Kroon Campos, University of Texas Medical Branch at Galveston, United StatesCopyright © 2022 Li and Kuang. This is an open-access article distributed under the terms of the Creative Commons Attribution License (CC BY). The use, distribution or reproduction in other forums is permitted, provided the original author(s) and the copyright owner(s) are credited and that the original publication in this journal is cited, in accordance with accepted academic practice. No use, distribution or reproduction is permitted which does not comply with these terms.
*Correspondence: Ersheng Kuang, a3VhbmdlcnNoQG1haWwuc3lzdS5lZHUuY24=
Disclaimer: All claims expressed in this article are solely those of the authors and do not necessarily represent those of their affiliated organizations, or those of the publisher, the editors and the reviewers. Any product that may be evaluated in this article or claim that may be made by its manufacturer is not guaranteed or endorsed by the publisher.
Research integrity at Frontiers
Learn more about the work of our research integrity team to safeguard the quality of each article we publish.