- Department of Reproductive Medicine, Liuzhou Maternity and Child Healthcare Hospital Affiliated with Women and Children’s Hospital of Guangxi University of Science and Technology, Liuzhou, China
Background: The availability and use of frozen–thawed embryos after controlled ovarian hyperstimulation for assisted reproduction have increased with improvements in vitrification techniques and the rise of gonadotropin-releasing hormone (GnRH) antagonist protocols. Although evidence has shown that frozen–thawed embryo transfers (FETs) result in higher live birth rates than fresh embryo transfers, it is uncertain whether this association exists in cycles employing the GnRH antagonist protocol.
Objective: To test the hypothesis that FETs are more likely to result in a live birth than fresh embryo transfers in a GnRH antagonist protocol cycle and to investigate whether frozen blastocyst transfer increases live birth rates compared to fresh blastocyst transfer.
Design: A retrospective historical cohort study was conducted using data collected from the Department of Reproductive Medicine of Liuzhou Maternity and Child Healthcare Hospital for 1,437 patients who underwent the GnRH antagonist protocol between 1 January 2015, and 31 December 2020. The primary outcome was the live birth rate, which was compared between fresh embryo transfer and FET, and the secondary outcomes were clinical pregnancy rate and miscarriage rate, which were compared between the two groups. Analyses were adjusted to account for the age of the patient, number of embryo transfers, day of embryo transfer, and type of infertility.
Results: Fresh embryo transfers accounted for 1,026 (71.4%) of the 1,437 patients who underwent the GnRH antagonist protocol in our analysis, while FETs accounted for 411 (28.6%). Patients with fresh and frozen–thawed embryos had comparable median body mass index (body mass index; 22.3 [IQR, 24.6–20.0] vs. 22.0 [IQR, 24.5–19.9]). There was a significant difference in the median age of the fresh embryo transfer group (34.0 [IQR, 39.0–30.0]) and the Frozen–thawed embryo transfer group (32.0 [IQR, 37.0–29.0]). Blastocysts were transferred in 14.6% of the fresh embryo transfer cycles and 45.5% of the FET cycles, whereas they account for 10.4% and 13.0% of all patients, respectively. The mean number of embryos transferred was 2 (IQR, 2.0–1.0) for the fresh embryo transfer group and 1 (IQR, 2.0–1.0) for the FET group, with a significant difference in the mean number of embryos transferred. The live birth rate after fresh embryo transfer vs. FET was 28.7% vs. 34.5% (absolute difference, 5.9%; adjusted relative risk [aRR], 1.15 [95% CI, 0.88–1.51]). The clinical pregnancy rates were 39.9% vs. 46.0%, respectively (absolute difference, 6.1%; aRR, 1.10 [95% CI, 0.85–1.43]). The miscarriage rates were 22.5% vs. 23.8%, respectively (absolute difference, 1.3%; aRR, 1.13 [95% CI, 0.75–1.70]).
Conclusion: In this retrospective study of women who underwent assisted reproduction using GnRH antagonists, FETs resulted in a higher live birth rates and clinical pregnancy rates than fresh embryo transfers, which parts of these differences were attributable to embryo stage. However, the interpretation of the findings is limited by the possibility of selection and confounding biases.
Introduction
Human in vitro fertilization (IVF) has become the most common treatment for infertility since its introduction in 1978 (Talaulikar and Arulkumaran, 2013). Since then, numerous assisted reproductive technologies (ARTs) have been developed and refined, including intracytoplasmic sperm injection (ICSI), in vitro embryos, ovulation induction, and cryopreservation. Frozen–thawed embryo transfer (FET) was first used in 1983 to avoid embryo replacement in adverse maternal conditions (Trounson and Mohr, 1983). The use of FET has increased rapidly in recent decades (Shapiro et al., 2014), even though the rate of female infertility has remained unchanged over this time (Mascarenhas et al., 2012). Data from European countries in 2013 showed that FET was used in 27.0% of cycles, a 3.9% increase compared to that in 2012, with substantial variation in utilization between countries (Calhaz-Jorge et al., 2017). The same trend has also been reported in the United States (Litzky et al., 2018), China (Shi et al., 2018), and Japan (Ishihara et al., 2014; Takeshima et al., 2016). Couples with non-male factor infertility have had the most substantial increase in the use of FET (from 48.0% to 72.4%) (Takeshima et al., 2016). The justification for using FET in couples with non-female factor infertility is that it can prevent unexpected total unsuccessful embryo transfers and increase the number of embryos or blastocysts, thereby increasing the chances of having a baby. Additionally, it has been reported that FET may also improve perinatal and neonatal outcomes (Sazonova et al., 2012; Wennerholm et al., 2013; Bhattacharya, 2016).
Previous studies have shown that increasing the number of oocytes can improve the cumulative live birth rate (Polyzos et al., 2018; Law et al., 2019). Therefore, increasing oocyte yield with more embryos is crucial for increasing pregnancy rates. The first IVF therapies were conducted in natural unstimulated IVF cycles, but now gonadotrophins are used to induce multiple follicular developments, and gonadotropin-releasing hormone (GnRH) analogs are used to suppress premature luteinizing hormone (LH) surges in IVF. GnRH antagonists competitively block GnRH receptors in the pituitary gland, resulting in the rapid, reversible suppression of gonadotropin secretion and the avoidance of LH (Hall et al., 1988; Huirne et al., 2007), making them a more logical alternative for preventing premature LH surges in IVF. Additionally, the GnRH antagonist protocol has been shown to significantly improve the flexibility and security of clinical applications, and it has been widely utilized in assisted reproductive treatment cycles around the world because of its advantages (Jing et al., 2020).
Although it has been discovered that frozen blastocyst transfers contribute to a higher live birth rate than fresh blastocyst transfers in ovulatory women with good prognoses (Wei et al., 2019), the difference in live birth rates between the FET and fresh transfer groups in GnRH antagonist cycles is still largely unclear. Some cohort studies discovered that FETs resulted in a significantly higher pregnancy rate than fresh embryo transfers (Roque et al., 2015; Chang et al., 2017), whereas other studies discovered that FETs and fresh embryo transfers resulted in similar pregnancy rates, even when a high-quality embryo was transferred during FET (Veleva et al., 2013). Considering that GnRH antagonists are frequently used in clinics with no adverse side effects (Tata et al., 2018) and that different embryonic stages have different reproductive outcomes in fresh embryo transfer cycles (Carvalho et al., 2017), we hypothesize that in GnRH antagonist cycles, FETs are more likely to result in a live birth than fresh embryo transfers, particularly when blastocyst transfer is performed.
Materials and Methods
Participants
This retrospective cohort study was conducted at the Department of Reproductive Medicine of Liuzhou Maternity and Child Healthcare Hospital, which is affiliated with the Women and Children’s Hospital of Guangxi University of Science and Technology, and it included all women who underwent controlled ovarian hyperstimulation (COH) and their first IVF/ICSI cycle between January 2015 and December 2020. The following were the inclusion criteria: first IVF/ICSI cycles with at least one embryo available for transfer at either the cleavage or blastocyst stage and planned COH using the GnRH antagonist protocol. The exclusion criteria were cycles with preimplantation genetic testing for aneuploidy (PGT-A), donor oocytes, donor embryos, miscarriages, adverse pregnancy history, uterine anomalies, or incomplete records. Patient information, such as age, infertility diagnosis, infertility type, body mass index (BMI), embryo transfer day, and the number of embryos transferred, was recorded for each cycle.
COH Protocols
GnRH antagonist protocol: Patients received 150–300 IU/day on day 2 or day 3 of their menstrual cycle until a trigger occurred. The ovarian response was monitored by transvaginal ultrasonography and serum hormone levels, and the r-FSH dosage was adjusted. The patients also received 0.25 mg of a GnRH antagonist (Cetrorelix, Merck Serono, France) daily until the leading follicles reached a mean diameter of 14 mm, which was considered a trigger. When three follicles reached a mean diameter of 17 mm or two follicles reached a mean diameter of 18 mm, 5,000–10,000 IU of recombinant human chorionic gonadotropin (r-HCG, Merck, Darmstadt, Germany) was administered to induce the final maturation of the oocytes. Vaginal ultrasound-guided oocyte retrieval was performed 36 h after HCG rejection. After oocyte retrieval, routine IVF or ICSI was performed based on sperm quality. Luteal phase support was initiated on day 1 after oocyte retrieval by injecting 60 mg of progesterone (Xianju, Zhejiang, China).
Embryo Transfer
Fresh Embryo Transplantation
Embryo transplantation was carried out 3 days after oocyte retrieval or with blastocyst transplantation 5 days after oocyte retrieval. Fresh cycles were canceled if patients had an endometrial thickness <7 mm, severe ovarian hyperstimulation syndrome (OHSS; E2 > 5,000 pg/ml on the trigger day and the number of oocytes acquired >20), no available embryos, or other personal reasons.
Frozen Embryo Transplantation
The endometrial preparations in the subsequent FET cycles were programmed using artificial cycles, downregulation + artificial cycles, natural cycles, and induced ovulation cycles, depending on the specific circumstances of the different patients. Artificial cycles were applied to patients with irregular menstrual cycles or polycystic ovary syndrome (PCOS) using exogenous estrogen and progesterone. Oral estradiol (Progynova, Bayer Healthcare, Germany) was administered at 2–3 mg TID (the drug dosage was adjusted according to endometrium thickness), and a transvaginal ultrasound examination was performed 5 days later. GnRH-agonist was used for downregulation in patients with endometriosis, adenomyosis, or uterine fibroids before artificial cycles were applied. An intramuscular injection of Diphereline was used for downregulation when the endometrium was less than 5 mm on day 2 of the menstrual cycle. Thereafter, an artificial cycle was performed after the deregulation standard was attained. Natural cycles were suitable for patients with spontaneous ovulation and regular menstrual cycles. We performed a vaginal ultrasound examination on these patients on days 8–10 of menstruation. An intramuscular injection of 10,000 IU of HCG was administered when the dominant follicles were ≥18 mm and the endometrial thickness was ≥7 mm; FETs were then performed after 3 or 5 days. For ovulation cycles, oral clomiphene or letrozole was administered on day 3 or 5 of their menstrual cycle for a total of 5 days, and HCG was injected to induce ovulation when the dominant follicles were ≥15 mm and the endometrial thickness was ≥7 mm.
The follicle and endometrial thickness were assessed using vaginal ultrasound, and embryo or blastocyst transplantation was performed 3–5 days later under abdominal ultrasound guidance.
Outcome Measures
In this study, we compared the primary outcome (live birth rate) and the secondary outcomes (clinical pregnancy rate and miscarriage rate) of fresh embryo/blastocyst transfers and frozen–thawed embryo/blastocyst transfers. The primary outcome was defined as the live birth of one or more infants. Clinical pregnancy was defined as an intrauterine gestational sac visible on ultrasound, miscarriage, or termination in the absence of ultrasound data. Miscarriage was defined as pregnancy loss before 20 weeks of gestation.
Statistical Analyses
All statistical analyses were performed using the Statistical Package for the Social Sciences (SPSS) version 26 (SPSS Inc., Chicago, IL, United States). Medians and interquartile ranges (IQRs) were calculated for baseline and patient characteristics that were not normally distributed. Log-binomial regression models were used to calculate the relative risks (RRs) and 95% confidence intervals (CIs) for the primary and secondary outcomes. Findings from the analyses of secondary endpoints should be interpreted as exploratory because multiple comparisons can produce type 1 errors.
Crude and adjusted analyses were performed to account for the age of the patients, number of embryo transfers, day of embryo transfer, and type of infertility. Sensitivity analyses for the embryo stage for all outcomes. The fresh and frozen–thawed cycles and the embryo stage were evaluated using log-binomial regression interactions. Statistical significance was set at p < 0.05.
Results
Study Population
Figure 1 shows that 21,008 cycles were excluded for the following reasons: nonavailability of embryo/other COH protocols/not the initial cycle (n = 20,411), adverse pregnancy history/miscarriages/uterine anomalies (n = 216), PGT-A (n = 53), at risk of OHSS (n = 38), and incomplete records (n = 290). The remaining 1,437 cycles, including 1,026 fresh embryo transfers and 411 FETs, met the eligibility criteria.
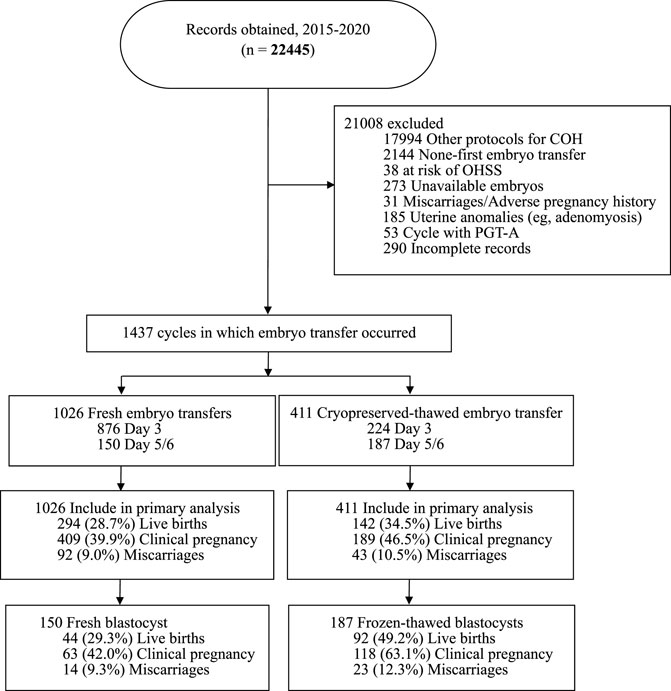
FIGURE 1. Outline of the selection process, transfer cycle type, embryo stage at transfer and outcomes of embryo transfer cycles included in this study.
Study Group Characteristics
Patients with fresh and frozen–thawed embryos had comparable median BMIs (22.3 [IQR, 24.6–20.0] vs. 22.0 [IQR, 24.5–19.9]). Patient BMI data were missing in 28 fresh embryo transfer cycles and 10 FET cycles. There was a significant difference in the median age of the fresh embryo transfer group (34.0 [IQR, 39.0–30.0]) and the FET group (32.0 [IQR, 37.0–29.0]). Blastocysts were transferred in 14.6% of the fresh embryo transfer cycles and 45.5% of the FET cycles, whereas they account for 10.4% and 13.0% of all patients, respectively. These data were not normally distributed; the mean number of embryos transferred was 2 (IQR, 2.0–1.0) for the fresh embryo transfer group and 1 (IQR, 2.0–1.0) for the FET group, as presented in Table 1.
Primary infertility was more common in the FET group (42.3% [174/411]) than in the fresh embryo transfer group (34.2% [351/1,026]). There was no significant difference in intracytoplasmic sperm injection utilization between the fresh embryo transfer group (19.4%) and the FET group (15.6%). Meanwhile, we excluded a small number of cycles for which this variable was not recorded (Table 1).
Primary Outcome
As shown in Table 2, there was a significant difference in the live birth rate after fresh embryo transfer compared to FET (28.7% vs. 34.5%, respectively; absolute difference, 5.9%; unadjusted RR, 1.21 [95% CI, 1.02–1.42]). In addition, after adjusting for patient age, type of infertile, the diagnoses of infertility, and number of embryo transfer did not change the significance of the difference (aRR, 1.33 [95% CI, 1.024–1.716]; data not presented), whereas we observed no significant difference in both live birth rates and clinical pregnancy rates after adjusting for the effect of embryo stage (adjusted RR [aRR], 1.15 [95% CI, 0.88–1.51]), suggesting that parts of these differences were attributable to embryo stage.
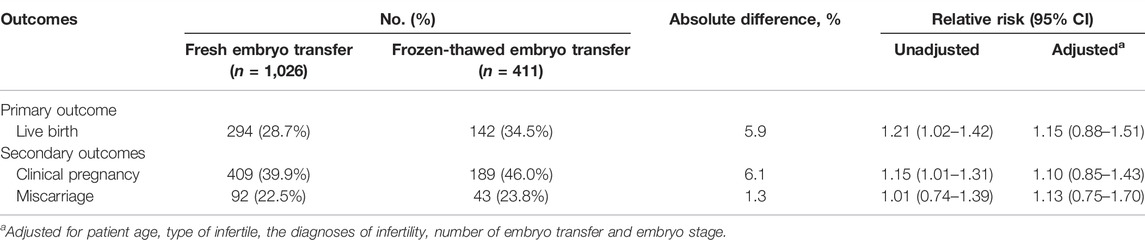
TABLE 2. Live birth, clinical pregnancy, and miscarriage rates in fresh vs. frozen-thawed embryo transfer cycle.
Secondary Outcomes
After fresh embryo transfer, the clinical pregnancy rate was 39.9%, which was statistically lower than the 46.0% rate after FET (absolute difference, 6.1%; RR, 1.15 [95% CI, 1.01–1.39]). Similarly, there was not statistically significant when the clinical pregnancy rates were adjusted for embryo stage (aRR, 1.10 [95% CI, 0.85–1.43]).
The miscarriage rate was 22.5% after fresh embryo transfer and 23.8% after FET, with no statistically significant difference between the two groups (absolute difference, 1.3%; RR, 1.01 [95% CI, 0.74–1.39]; aRR, 1.13 [95% CI, 0.75–1.70]).
Cycle Using Blastocyst
The baseline demographics and clinical characteristics of the fresh and frozen cleavage-stage embryo or blastocyst groups are shown in Supplementary eTable S1 and Table 3, respectively. 23.5% of cycles (337/1,437) underwent blastocyst transfer. Of those, 10.4% (150/1,437) were fresh blastocyst transfer group and 13.0% (187/1,437) were frozen-thawed transfer group.
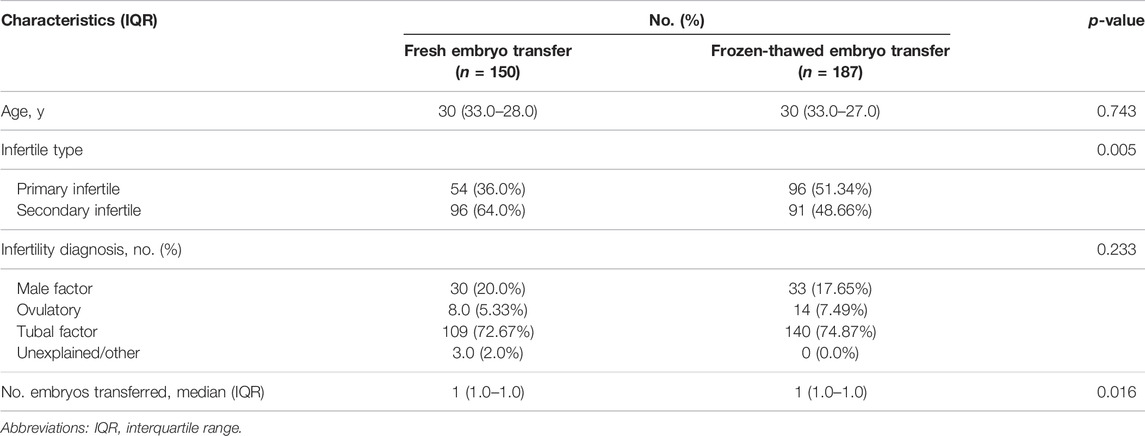
TABLE 3. Baseline patient characteristics of women with fresh blastocyst transfers and frozen–thawed blastocyst transfers.
As shown in Figure 2 and Supplementary eTable S3, the FET group had a statistically higher live birth rate for blastocyst transfers than the fresh embryo transfer group (49.2% vs. 29.3%, respectively; absolute difference, 19.9%; aRR, 2.30 [95% CI, 1.45–3.65], which were adjusted to account for the age of patients, type of infertility, diagnosis of infertility, and number of embryo transfers), although the absolute difference in outcomes was smaller than that observed when all fresh embryo transfers were compared to all FETs.
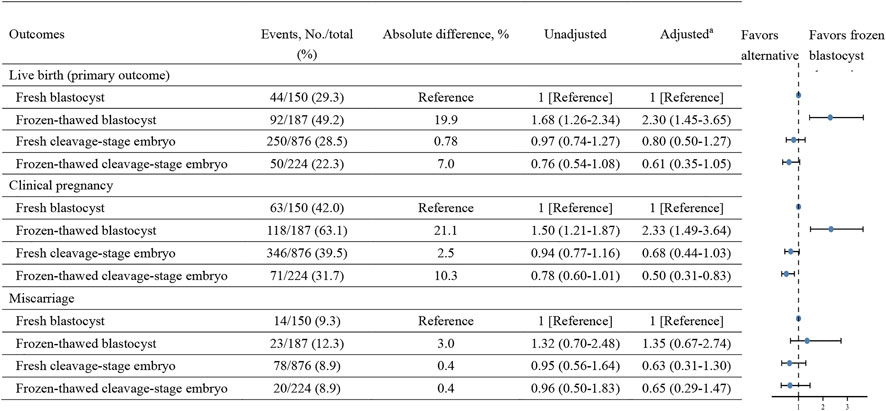
FIGURE 2. Liver birth, clinical pregnancy, and miscarriage rates in fresh and frozen-thawed embryo transfers with cleavage-stage embryo and blastocyst.
The frozen–thawed blastocyst transfer group also had a statistically significantly higher clinical pregnancy rate than the fresh blastocyst transfer group (63.1% vs. 42.0%, respectively; absolute difference, 21.1%; aRR, 2.33 [95% CI, 1.49–3.65]). There was no significant difference in the miscarriage rate between fresh blastocyst cycles and frozen–thawed blastocyst cycles (9.3% vs. 12.3%, respectively; absolute difference, 3.0%; aRR, 1.35 [95% CI, 0.67–2.74]).
In contrast, live birth rates, clinical pregnancy rates and miscarriage rates did not differ when fresh cleavage-stage embryo transfer group compared with frozen-thawed cleavage-stage embryo group (Supplementary eTable S2). Our results also revealed that there were no statistically significant differences in live birth rates, clinical pregnancy rates, or miscarriage rates between the fresh or frozen–thawed cleavage-stage embryo transfer group and the fresh blastocyst transfer group (Figure 2).
Discussion
This study demonstrated that, in cycles using the GnRH antagonist protocol, FETs resulted in statistically significantly higher live birth rates than fresh embryo transfer, which parts of these differences were attributable to embryo stage. When comparing fresh blastocyst transfers with frozen-thawed blastocyst transfers, the frozen blastocyst transfer group demonstrated a statistically significantly higher live birth rate and clinical pregnancy rate. This finding is consistent with previous findings that demonstrated higher live birth rates after FET in cycles involving ovarian stimulation.
The proposed mechanisms for improving pregnancy rates using frozen–thawed embryos rather than fresh embryos in GnRH antagonist cycles have demonstrated the possibility that poor endometrial receptivity (ER) is more likely to occur in fresh embryo transfer cycles after controlled ovarian stimulation than in FETs, in which the embryos can be cryopreserved and transferred to a more receptive endometrium (Weinerman and Mainigi, 2014). In addition, the dysregulation of the steroidogenesis-associated gene has been observed in women with endometriosis (Kao et al., 2003; Borghese et al., 2008), suggesting that a disordered endometrium could be exacerbated by high steroid levels, which justifies the FET strategy. Furthermore, using a gestational carrier in fresh embryo transfer or FET can improve IVF outcomes, suggesting that the uterus has a significant effect (Murugappan et al., 2018). Conversely, Insogna et al. (2021) and Miravet-Valenciano et al. (2017) argued that IVF outcomes remain significantly different between fresh embryo transfer and FET cycles despite the recipient uterus not being exposed to superphysiological levels of estrogen or gonadotropins in donor oocyte cycles. Therefore, a disordered endometrium cannot explain the differences observed in the study population.
Embryo quality may be another possible explanation for the advantage of FET over fresh embryo transfer because embryos must be subjected to rigorous selection before freezing (Ginström Ernstad et al., 2019). In contrast, Oktay et al. (2015) suggested that frozen embryo cycles most likely constitute a selection of low-quality embryos because high-quality embryos would have been transferred during the initial fresh transfer. Thus, a prospective cohort study that attempted to control embryo quality by including only initial high-quality embryos that were transferred in fresh or frozen–thawed cycles demonstrated that IVF outcomes can be improved by employing the freeze-all strategy in cases without progesterone elevation (Sacks et al., 2018). Similarly, only the initial transfer in the fresh or frozen–thawed group was analyzed in this study to address the question of embryo quality, and FETs were found to have a higher likelihood of live birth than fresh embryo transfers in patients who used the GnRH antagonist protocol.
Although using blastocysts in fresh cycles is beneficial, it is still uncertain whether or not the stage of embryo development affects live birth and pregnancy rates because they both have advantages and disadvantages (Glujovsky et al., 2016). The in vivo environment is likely to be superior to the in vitro environment, where a low developmental rate of embryos cultured past the cleavage stage (i.e., only 30%–50% of embryos developed into blastocysts) resulted in a high incidence of cycle cancellation (Marek et al., 1999; Racowsky et al., 2000). In addition, in vitro culture that goes beyond embryonic genomic activation can cause embryo damage (Martins et al., 2017). However, the uterine environment is assumed to stress the cleavage-stage embryo because the uterus provides a different nutritional environment from the oviduct (Baart et al., 2006), and late embryo transfer (late on day 3 or early on day 4) is believed to be more analogous to natural cycles (Brown et al., 2016). The morphological criteria used for selecting the highest implantation potential are anticipated to be more accurate than those for selecting cleavage-stage embryos if the culture duration is extended for an additional 2–3 days (days 5–6) (Machtinger and Racowsky, 2013). Indeed, many published studies have shown that fresh blastocyst stage transfers result in higher live birth rates than fresh cleavage-stage transfers (Papanikolaou et al., 2008; Glujovsky et al., 2016; Carvalho et al., 2017). However, our results suggest that fresh blastocyst transfer is not superior to fresh cleavage-stage embryo transfer in terms of live birth or pregnancy rates in women undergoing the GnRH antagonist protocol. This finding corroborated recent findings from a meta-analysis of randomized controlled trials that found no difference in live birth rates, clinical pregnancy rates, or miscarriage rates when blastocyst transfers were compared to cleavage-stage embryo transfers (Martins et al., 2017). Nevertheless, similar to the findings reported by Daimin et al. in a study on ovulatory women (Wei et al., 2019), FET was found to be associated with higher live birth or pregnancy rates in women who underwent the GnRH antagonist protocol when the fresh blastocyst cycles were compared to the frozen–thawed blastocyst cycles, indicating that some physiological differences do exist between fresh and frozen–thawed blastocyst transfers. Although the underlying mechanism is still unclear, the substantial increase in pregnancy rates could be due to a physical change in stiffness in human embryos that were previously cryopreserved (Murayama et al., 2006; Ko et al., 2008; Yanez et al., 2016).
Pregnancy loss cannot explain the differences in live birth and clinical pregnancy rates following fresh embryo transfers or FETs because the miscarriage rates were similar in both groups. Thus, these findings suggest that the frozen–thawed process boosted the implantation potential of embryos obtained from women who had been on the GnRH antagonist protocol. Although there was no difference in miscarriage rates between the fresh and frozen–thawed blastocyst transfer groups, the CIs were large, indicating that further investigation is required.
The findings of this study have several strengths. First, the cost of a successful cycle in the GnRH antagonist protocol is high, ranging from ¥6,757.39 to ¥153,327.20 (Pan et al., 2019). Although the GnRH antagonist protocol is better than other protocols in terms of psychosocial and physical well-being during the first ART treatment (Toftager et al., 2018), these data, considering the significant financial investment, may influence physician recommendation or patients’ decision-making regarding a COH protocol and a fresh embryo transfer vs. FET a priori for convenience. Thus, future research into the cost-effectiveness of fresh embryo transfer vs. FET in this population would be beneficial in guiding practice. Second, recent studies have suggested that the freeze-all policy results in low perinatal morbidity/mortality rates, few birth defects, low birth weight, short gestational age, and additional antepartum hemorrhage (Maheshwari et al., 2012; Roy et al., 2014). However, our findings suggest that frozen–thawed blastocyst transfer results in a significantly higher live birth rate than fresh blastocyst transfer, which may provide useful guidance when deciding whether to pursue a fresh blastocyst transfer or a frozen–thawed blastocyst transfer when using the GnRH antagonist protocol.
However, some limitations must also be considered, particularly its retrospective design, which allows associations between exposures and outcomes to be detected but does not address causality. Second, incomplete data for race and BMI, both of which are known to contribute to miscarriage, may have skewed the results. Additionally, the study was conducted only in a single center, and the sample size was relatively small, limiting the generalizability of the findings. Third, although each clinic’s data were validated by the medical director, it is still possible that certain diseases, such as endometriosis, were underdiagnosed, resulting in women being categorized as having unexplained infertility.
Conclusion
In this retrospective cohort study of women who underwent ART using the GnRH antagonist protocol, FETs were found to result in higher live birth rates and clinical pregnancy rates than fresh embryo transfers, which parts of these differences were attributable to embryo stage. However, the interpretation of the findings is limited by its retrospective nature. Furthermore, a large, multi-institution collaborative trial is required to determine whether the embryo stage during transfer affects the live birth rate of initial fresh embryo transfers or FETs.
Data Availability Statement
The raw data supporting the conclusion of this article will be made available by the authors, without undue reservation.
Ethics Statement
The studies involving human participants were reviewed and approved by the Ethics Committee of Liuzhou maternity and Child Healthcare Hospital. Written informed consent for participation was not required for this study in accordance with the national legislation and the institutional requirements.
Author Contributions
WH, LF, and JL took part in the study design; CY and NT collected and analyzed data. XW and YT interpreted the results; WH, LF, and JL wrote the manuscript. All authors read, revised and approved the final manuscript.
Funding
This work was financially supported by Self-funded by the Health Commission of Guangxi Zhuang autonomous Region (grant number Z20210585) (to WH).
Conflict of Interest
The authors declare that the research was conducted in the absence of any commercial or financial relationships that could be construed as a potential conflict of interest.
Publisher’s Note
All claims expressed in this article are solely those of the authors and do not necessarily represent those of their affiliated organizations, or those of the publisher, the editors and the reviewers. Any product that may be evaluated in this article, or claim that may be made by its manufacturer, is not guaranteed or endorsed by the publisher.
Supplementary Material
The Supplementary Material for this article can be found online at: https://www.frontiersin.org/articles/10.3389/fcell.2022.884677/full#supplementary-material
References
Baart, E. B., Martini, E., van den Berg, I., Macklon, N. S., Galjaard, R.-J. H., Fauser, B. C. J. M., et al. (2006). Preimplantation Genetic Screening Reveals a High Incidence of Aneuploidy and Mosaicism in Embryos from Young Women Undergoing IVF. Hum. Reprod. 21 (1), 223–233. doi:10.1093/humrep/dei291
Bhattacharya, S. (2016). Maternal and Perinatal Outcomes after Fresh versus Frozen Embryo Transfer-What Is the Risk-Benefit Ratio? Fertil. Sterility 106 (2), 241–243. doi:10.1016/j.fertnstert.2016.06.038
Borghese, B., Mondon, F., Noel, J.-C., Fayt, I., Mignot, T.-M., Vaiman, D., et al. (2008). Research Resource: Gene Expression Profile for Ectopic versus Eutopic Endometrium Provides New Insights into Endometriosis Oncogenic Potential. Mol. Endocrinol. 22 (11), 2557–2562. doi:10.1210/me.2008-0322
Brown, J., Daya, S., and Matson, P. (2016). Day Three versus Day Two Embryo Transfer Following In Vitro Fertilization or Intracytoplasmic Sperm Injection. Cochrane Database Syst. Rev. 12 (12), Cd004378. doi:10.1002/14651858.CD004378.pub3
Calhaz-Jorge, C., De Geyter, C., Calhaz-Jorge, C., De Geyter, C., Kupka, M. S., de Mouzon, J., et al. (2017). Assisted Reproductive Technology in Europe, 2013: Results Generated from European Registers by ESHRE. Hum. Reprod. 32 (10), 1957–1973. doi:10.1093/humrep/dex264
Carvalho, B. R., Barbosa, M. W., Bonesi, H., Gomes, D. B., Cabral, Í. O., Barbosa, A. C., et al. (2017). Embryo Stage of Development Is Not Decisive for Reproductive Outcomes in Frozen-Thawed Embryo Transfer Cycles. JBRA Assist. Reprod. 21 (1), 23–26. doi:10.5935/1518-0557.20170007
Chang, J.-C., Chen, M.-J., Guu, H.-F., Chen, Y.-F., Yi, Y.-C., Kung, H.-F., et al. (2017). Does the "Freeze-All" Policy Allow for a Better Outcome in Assisted Reproductive Techniques Than the Use of Fresh Embryo Transfers? - A Retrospective Study on Cumulative Live Birth Rates. Taiwanese J. Obstet. Gynecol. 56 (6), 775–780. doi:10.1016/j.tjog.2017.10.013
Ginström Ernstad, E., Wennerholm, U. B., Khatibi, A., Petzold, M., and Bergh, C. (2019). Neonatal and Maternal Outcome after Frozen Embryo Transfer: Increased Risks in Programmed Cycles. Am. J. Obstet. Gynecol. 221 (2), 126–e18. doi:10.1016/j.ajog.2019.03.010
Glujovsky, D., Farquhar, C., Quinteiro Retamar, A. M., Alvarez Sedo, C. R., and Blake, D. (2016). Cleavage Stage versus Blastocyst Stage Embryo Transfer in Assisted Reproductive Technology. Cochrane Database Syst. Rev. 6, Cd002118. doi:10.1002/14651858.CD002118.pub5
Hall, J. E., Brodie, T. D., Badger, T. M., Rivier, J., Vale, W., Conn, P. M., et al. (1988). Evidence of Differential Control of FSH and LH Secretion by Gonadotropin-Releasing Hormone (GnRH) from the Use of a GnRH Antagonist*. J. Clin. Endocrinol. Metab. 67 (3), 524–531. doi:10.1210/jcem-67-3-524
Huirne, J. A., Homburg, R., and Lambalk, C. B. (2007). Are GnRH Antagonists Comparable to Agonists for Use in IVF? Hum. Reprod. 22 (11), 2805–2813. doi:10.1093/humrep/dem270
Insogna, I. G., Lanes, A., Lee, M. S., Ginsburg, E. S., and Fox, J. H. (2021). Association of Fresh Embryo Transfers Compared with Cryopreserved-Thawed Embryo Transfers with Live Birth Rate Among Women Undergoing Assisted Reproduction Using Freshly Retrieved Donor Oocytes. Jama 325 (2), 156–163. doi:10.1001/jama.2020.23718
Ishihara, O., Araki, R., Kuwahara, A., Itakura, A., Saito, H., and Adamson, G. D. (2014). Impact of Frozen-Thawed Single-Blastocyst Transfer on Maternal and Neonatal Outcome: an Analysis of 277,042 Single-Embryo Transfer Cycles from 2008 to 2010 in Japan. Fertil. Sterility 101 (1), 128–133. doi:10.1016/j.fertnstert.2013.09.025
Jing, M., Lin, C., Zhu, W., Tu, X., Chen, Q., Wang, X., et al. (2020). Cost-effectiveness Analysis of GnRH-Agonist Long-Protocol and GnRH-Antagonist Protocol for In Vitro Fertilization. Sci. Rep. 10 (1), 8732. doi:10.1038/s41598-020-65558-0
Kao, L. C., Germeyer, A., Tulac, S., Lobo, S., Yang, J. P., Taylor, R. N., et al. (2003). Expression Profiling of Endometrium from Women with Endometriosis Reveals Candidate Genes for Disease-Based Implantation Failure and Infertility. Endocrinology 144 (7), 2870–2881. doi:10.1210/en.2003-0043
Ko, C. S., Ding, D. C., Chu, T. W., Chu, Y. N., Chen, I. C., Chen, W. H., et al. (2008). Changes to the Meiotic Spindle and Zona Pellucida of Mature Mouse Oocytes Following Different Cryopreservation Methods. Anim. Reprod. Sci. 105 (3-4), 272–282. doi:10.1016/j.anireprosci.2007.03.010
Law, Y. J., Zhang, N., Venetis, C. A., Chambers, G. M., and Harris, K. (2019). The Number of Oocytes Associated with Maximum Cumulative Live Birth Rates Per Aspiration Depends on Female Age: a Population Study of 221 221 Treatment Cycles. Hum. Reprod. 34 (9), 1778–1787. doi:10.1093/humrep/dez100
Litzky, J. F., Boulet, S. L., Esfandiari, N., Zhang, Y., Kissin, D. M., Theiler, R. N., et al. (2018). Effect of Frozen/thawed Embryo Transfer on Birthweight, Macrosomia, and Low Birthweight Rates in US Singleton Infants. Am. J. Obstet. Gynecol. 218 (4), 433–e10. doi:10.1016/j.ajog.2017.12.223
Machtinger, R., and Racowsky, C. (2013). Morphological Systems of Human Embryo Assessment and Clinical Evidence. Reprod. BioMedicine Online 26 (3), 210–221. doi:10.1016/j.rbmo.2012.10.021
Maheshwari, A., Pandey, S., Shetty, A., Hamilton, M., and Bhattacharya, S. (2012). Obstetric and Perinatal Outcomes in Singleton Pregnancies Resulting from the Transfer of Frozen Thawed versus Fresh Embryos Generated through In Vitro Fertilization Treatment: a Systematic Review and Meta-Analysis. Fertil. Sterility 98 (2), 368–377. e1-9. doi:10.1016/j.fertnstert.2012.05.019
Marek, D., Langley, M., Gardner, D. K., Confer, N., Doody, K. M., and Doody, K. J. (1999). Introduction of Blastocyst Culture and Transfer for All Patients in an In Vitro Fertilization Program. Fertil. Sterility 72 (6), 1035–1040. doi:10.1016/s0015-0282(99)00409-4
Martins, W. P., Nastri, C. O., Rienzi, L., van der Poel, S. Z., Gracia, C., and Racowsky, C. (2017). Blastocyst vs Cleavage-Stage Embryo Transfer: Systematic Review and Meta-Analysis of Reproductive Outcomes. Ultrasound Obstet. Gynecol. 49 (5), 583–591. doi:10.1002/uog.17327
Mascarenhas, M. N., Flaxman, S. R., Boerma, T., Vanderpoel, S., and Stevens, G. A. (2012). National, Regional, and Global Trends in Infertility Prevalence since 1990: a Systematic Analysis of 277 Health Surveys. Plos Med. 9 (12), e1001356. doi:10.1371/journal.pmed.1001356
Miravet-Valenciano, J., Ruiz-Alonso, M., Gómez, E., and Garcia-Velasco, J. A. (2017). Endometrial Receptivity in Eutopic Endometrium in Patients with Endometriosis: it Is Not Affected, and Let Me Show You Why. Fertil. Sterility 108 (1), 28–31. doi:10.1016/j.fertnstert.2017.06.002
Murayama, Y., Mizuno, J., Kamakura, H., Fueta, Y., Nakamura, H., Akaishi, K., et al. (2006). Mouse Zona Pellucida Dynamically Changes its Elasticity during Oocyte Maturation, Fertilization and Early Embryo Development. Hum. Cel 19 (4), 119–125. doi:10.1111/j.1749-0774.2006.00019.x
Murugappan, G., Farland, L. V., Missmer, S. A., Correia, K. F., Anchan, R. M., and Ginsburg, E. S. (2018). Gestational Carrier in Assisted Reproductive Technology. Fertil. Sterility 109 (3), 420–428. doi:10.1016/j.fertnstert.2017.11.011
Oktay, K., Turan, V., Bedoschi, G., Pacheco, F. S., and Moy, F. (2015). Fertility Preservation Success Subsequent to Concurrent Aromatase Inhibitor Treatment and Ovarian Stimulation in Women with Breast Cancer. Jco 33 (22), 2424–2429. doi:10.1200/jco.2014.59.3723
Pan, W., Tu, H., Jin, L., Hu, C., Li, Y., Wang, R., et al. (2019). Decision Analysis about the Cost-Effectiveness of Different In Vitro Fertilization-Embryo Transfer Protocol under Considering Governments, Hospitals, and Patient. Medicine (Baltimore) 98 (19), e15492. doi:10.1097/md.0000000000015492
Papanikolaou, E. G., Kolibianakis, E. M., Tournaye, H., Venetis, C. A., Fatemi, H., Tarlatzis, B., et al. (2008). Live Birth Rates after Transfer of Equal Number of Blastocysts or Cleavage-Stage Embryos in IVF. A Systematic Review and Meta-Analysis. Hum. Reprod. 23 (1), 91–99. doi:10.1093/humrep/dem339
Polyzos, N. P., Drakopoulos, P., Parra, J., Pellicer, A., Santos-Ribeiro, S., Tournaye, H., et al. (2018). Cumulative Live Birth Rates According to the Number of Oocytes Retrieved after the First Ovarian Stimulation for In Vitro Fertilization/intracytoplasmic Sperm Injection: a Multicenter Multinational Analysis Including ∼15,000 Women. Fertil. Sterility 110 (4), 661–670. doi:10.1016/j.fertnstert.2018.04.039
Racowsky, C., Jackson, K. V., Cekleniak, N. A., Fox, J. H., Hornstein, M. D., and Ginsburg, E. S. (2000). The Number of Eight-Cell Embryos Is a Key Determinant for Selecting Day 3 or Day 5 Transfer. Fertil. Sterility 73 (3), 558–564. doi:10.1016/s0015-0282(99)00565-8
Roque, M., Valle, M., Guimarães, F., Sampaio, M., and Geber, S. (2015). Freeze-all Policy: Fresh vs. Frozen-Thawed Embryo Transfer. Fertil. Sterility 103 (5), 1190–1193. doi:10.1016/j.fertnstert.2015.01.045
Roy, T. K., Bradley, C. K., Bowman, M. C., and McArthur, S. J. (2014). Single-embryo Transfer of Vitrified-Warmed Blastocysts Yields Equivalent Live-Birth Rates and Improved Neonatal Outcomes Compared with Fresh Transfers. Fertil. Sterility 101 (5), 1294–1301. doi:10.1016/j.fertnstert.2014.01.046
Sacks, D., Sacks, D., Baxter, B., Campbell, B. C. V., Carpenter, J. S., Cognard, C., et al. (2018). Multisociety Consensus Quality Improvement Revised Consensus Statement for Endovascular Therapy of Acute Ischemic Stroke. AJNR Am. J. Neuroradiol 39 (6), E61–E32. doi:10.3174/ajnr.A5638
Sazonova, A., Kallen, K., Thurin-Kjellberg, A., Wennerholm, U.-B., and Bergh, C. (2012). Obstetric Outcome in Singletons after In Vitro Fertilization with Cryopreserved/thawed Embryos. Hum. Reprod. 27 (5), 1343–1350. doi:10.1093/humrep/des036
Shapiro, B. S., Daneshmand, S. T., Garner, F. C., Aguirre, M., and Hudson, C. (2014). Clinical Rationale for Cryopreservation of Entire Embryo Cohorts In Lieu of Fresh Transfer. Fertil. Sterility 102 (1), 3–9. doi:10.1016/j.fertnstert.2014.04.018
Shi, Y., Sun, Y., Hao, C., Zhang, H., Wei, D., Zhang, Y., et al. (2018). Transfer of Fresh versus Frozen Embryos in Ovulatory Women. N. Engl. J. Med. 378 (2), 126–136. doi:10.1056/nejmoa1705334
Takeshima, K., Jwa, S. C., Saito, H., Nakaza, A., Kuwahara, A., Ishihara, O., et al. (2016). Impact of Single Embryo Transfer Policy on Perinatal Outcomes in Fresh and Frozen Cycles-Analysis of the Japanese Assisted Reproduction Technology Registry between 2007 and 2012. Fertil. Sterility 105 (2), 337–346. e3. doi:10.1016/j.fertnstert.2015.10.002
Talaulikar, V. S., and Arulkumaran, S. (2013). Maternal, Perinatal and Long-Term Outcomes after Assisted Reproductive Techniques (ART): Implications for Clinical Practice. Eur. J. Obstet. Gynecol. Reprod. Biol. 170 (1), 13–19. doi:10.1016/j.ejogrb.2013.04.014
Tata, B., Mimouni, N. E. H., Barbotin, A.-L., Malone, S. A., Loyens, A., Pigny, P., et al. (2018). Elevated Prenatal Anti-müllerian Hormone Reprograms the Fetus and Induces Polycystic Ovary Syndrome in Adulthood. Nat. Med. 24 (6), 834–846. doi:10.1038/s41591-018-0035-5
Toftager, M., Sylvest, R., Schmidt, L., Bogstad, J., Løssl, K., Prætorius, L., et al. (2018). Quality of Life and Psychosocial and Physical Well-Being Among 1,023 Women during Their First Assisted Reproductive Technology Treatment: Secondary Outcome to a Randomized Controlled Trial Comparing Gonadotropin-Releasing Hormone (GnRH) Antagonist and GnRH Agonist Protocols. Fertil. Sterility 109 (1), 154–164. doi:10.1016/j.fertnstert.2017.09.020
Trounson, A., and Mohr, L. (1983). Human Pregnancy Following Cryopreservation, Thawing and Transfer of an Eight-Cell Embryo. Nature 305 (5936), 707–709. doi:10.1038/305707a0
Veleva, Z., Orava, M., Nuojua-Huttunen, S., Tapanainen, J. S., and Martikainen, H. (2013). Factors Affecting the Outcome of Frozen-Thawed Embryo Transfer. Hum. Reprod. 28 (9), 2425–2431. doi:10.1093/humrep/det251
Wei, D., Liu, J.-Y., Sun, Y., Shi, Y., Zhang, B., Liu, J.-Q., et al. (2019). Frozen versus Fresh Single Blastocyst Transfer in Ovulatory Women: a Multicentre, Randomised Controlled Trial. The Lancet 393 (10178), 1310–1318. doi:10.1016/s0140-6736(18)32843-5
Weinerman, R., and Mainigi, M. (2014). Why We Should Transfer Frozen Instead of Fresh Embryos: the Translational Rationale. Fertil. Sterility 102 (1), 10–18. doi:10.1016/j.fertnstert.2014.05.019
Wennerholm, U.-B., Henningsen, A.-K. A., Romundstad, L. B., Bergh, C., Pinborg, A., Skjaerven, R., et al. (2013). Perinatal Outcomes of Children Born after Frozen-Thawed Embryo Transfer: a Nordic Cohort Study from the CoNARTaS Group. Hum. Reprod. 28 (9), 2545–2553. doi:10.1093/humrep/det272
Keywords: frozen-thawed transfer, fresh transfer, assisted reproduction, blastocyst, cleavage-stage embryo
Citation: Fan L, Tang N, Yao C, Wei X, Tang Y, Li J and Huang W (2022) Association Between Fresh Embryo Transfers and Frozen–Thawed Embryo Transfers Regarding Live Birth Rates Among Women Undergoing Long Gonadotropin-Releasing Hormone Antagonist Protocols. Front. Cell Dev. Biol. 10:884677. doi: 10.3389/fcell.2022.884677
Received: 26 February 2022; Accepted: 11 April 2022;
Published: 28 April 2022.
Edited by:
Jennifer R. Wood, University of Nebraska System, United StatesReviewed by:
Ana Belen Perez Oliva, Biomedical Research Institute of Murcia (IMIB), SpainDavid McCulloh, NYU Grossman School of Medicine, United States
Copyright © 2022 Fan, Tang, Yao, Wei, Tang, Li and Huang. This is an open-access article distributed under the terms of the Creative Commons Attribution License (CC BY). The use, distribution or reproduction in other forums is permitted, provided the original author(s) and the copyright owner(s) are credited and that the original publication in this journal is cited, in accordance with accepted academic practice. No use, distribution or reproduction is permitted which does not comply with these terms.
*Correspondence: Jingjing Li, NzQwN2xpamluZ2ppbmdAMTYzLmNvbQ==; Wenjie Huang, YTE3Mzc3NTUyMjQyQDE2My5jb20=