- 1Department of Thoracic Surgery, First Affiliated Hospital of China Medical University, Shenyang, China
- 2Department of Geriatric Surgery, First Affiliated Hospital of China Medical University, Shenyang, China
Reactive oxygen species (ROS) play a crucial role in the regulation of tumor occurrence and development. As a main source of ROS, NADPH oxidases are key enzymes that mediate electron transport within intracellular membranes. Of the NOX members that have been reported to be dysregulated in a wide variety of tumors, NOX4 is the member to be most frequently expressed. Numerous studies have elucidated that NOX4 gets involved in the regulation of tumor proliferation, metastasis, therapy resistance, tumor-stromal interaction and dysregulated tumor metabolism. In this review, we primarily discussed the biological function of NOX4 in tumorigenesis and progression of multiple cancer models, including its role in activating oncogenic signaling pathways, rewiring the metabolic phenotype and mediating immune response. Besides, the development of NOX4 inhibitors has also been unraveled. Herein, we discussed the interplay between NOX4 and tumorigenesis, proposing NOX4 as a promising therapeutic target waiting for further exploration.
Introduction
The NOX family of NADPH oxidases are enzymes that mediate electron transport via intracellular membranes (Brandes et al., 2014a). NOX family was initially discovered in the membrane of phagocytes (Bedard et al., 2007). Currently, six members of the NOX family has been identified, including NOX1, NOX3, NOX4, NOX5, DUOX1, and DUOX2 (Vermot et al., 2021). These enzymes share common structure of six transmembrane domains and a C-terminus with a NADPH binding region, with each member exhibiting a specific tissue distribution (Bedard et al., 2007). The regulatory mechanisms for activation of each NOX member are diverse. Various mechanisms have been reported to regulate NOXs activity including post-translational modifications, lipids, calcium level, etc (Rossary et al., 2007; Brandes et al., 2014b; Douda et al., 2015). NOXs also function as reactive oxygen species- (ROS-) producing enzymes to regulate a series of biological function, comprising redox-dependent signaling pathways, oxygen sensor, metabolic reprograming, and immune defense (Bedard and Krause, 2007; Leto et al., 2009). Moreover, downstream ROS production are key regulators of cell differentiation, transformation, growth and death, which are actively engaged in the occurrence and development of multiple cancers (Klaunig, 2018).
Several NOX members have been found to be dysregulated in diverse cancer models, with NOX4 being the member most frequently expressed. Numerous studies have shown that NOX4 plays a crucial role in tumorigenesis and tumor development by supporting cancer cell transformation, proliferation, migration, invasion, and epithelial–mesenchymal transition (EMT). To date, NOX4 has been observed to participate in multiple malignancies, including lung cancer, renal cell cancer (RCC), colorectal cancer (CRC), gastric cancer (GC), pancreatic cancer, glioblastoma, and ovarian cancer, etc (Zeng et al., 2016; Meitzler et al., 2017; Shanmugasundaram et al., 2017; Du et al., 2018; Liu et al., 2021; Shen et al., 2020).
NOX4, ROS, and Cancer
The sources of ROS are the electron transport chain, producing ROS as a byproduct, as well as NOXs. Besides, endoplasmic reticulum membranes and peroxisomes express enzymes that can generate H2O2 are also considered as an indispensable supply of intracellular ROS (Brieger et al., 2012). In multiple cancer models, elevated ROS generation has been detected in tumor cells resulting from hypoxic environment, increased metabolic rates or altered redox-related gene expression (Zhang et al., 2016; Wu et al., 2021). Moreover, elevated ROS production also play diverse roles in tumor development, which can activate oncogenic signaling pathway, drive DNA damage and genetic instability, reprogram metabolic phenotype and mediate immune response (Yang et al., 2013; Schieber and Chandel, 2014; Moloney and Cotter, 2018). ROS could regulate a variety of signaling pathways, including PI3K/AKT, hypoxia-inducible factor-1α (HIF-1α), c-myc, NF-κB, and STAT3, and other molecules, thus linking to growth, metastasis, angiogenesis and chemoresistance of tumor (Zhang et al., 2014; Prasad et al., 2017).
NOX family members are transmembrane proteins that share the capacity to transport electrons across the biological membrane and to produce superoxide and other downstream ROS (Bedard et al., 2007). Conclusively, oxygen is regarded as electron acceptor and superoxide is the product of the electron transfer reaction. Thus, the main biological function of NOX family member is considered as ROS production. NOX4, the most frequently expressed member of NOX family, thus has a potential role in activating diverse signaling pathways and mediating metabolic plasticity through manipulating tumoral ROS level to participate in tumor occurrence and development (Vermot et al., 2021). Therefore, the diverse function of NOX4 and downstream ROS production during tumor progression make it a promising therapeutic target, and it is imperative to thoroughly understand the molecular mechanisms in different cancer models (Figure 1).
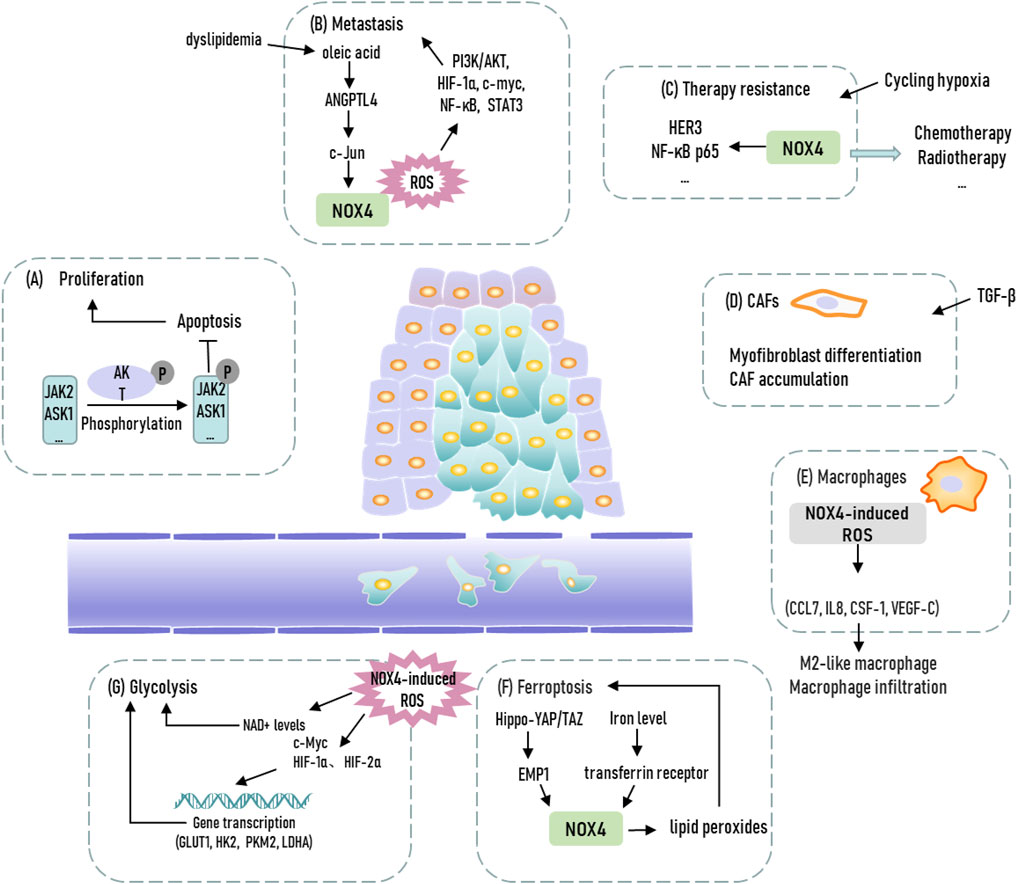
FIGURE 1. Representation of biological functions of NOX4 in cancer. JAK2, Janus kinase 2; ASK1, apoptosis signal-regulating kinase 1; ANGPTL4, Angiopoetin-like 4; CAFs, cancer-associated fibroblasts; EMP1, epithelial membrane protein 1; GLUT1, glucose transporter 1; HK2, hexokinase 2; PKM2, pyruvate kinase isoform 2; LDHA, lactate dehydrogenase A.
Role of NOX4 in Diverse Malignances
Lung Cancer
Recent statistics have revealed that lung cancer is the second most frequently diagnosed cancer and the primary cause of cancer-related morality worldwide, comprising both small cell lung cancer and non-small cell lung cancer (NSCLC) (Mao et al., 2016; Sung et al., 2021). Even though significant advances have been achieved in targeted therapy and immunotherapy of NSCLC, which accounts for the majority of lung cancers, exploiting promising therapeutic targets for lung cancer treatment is still urgently needed to improve the prognosis of these patients (Nagano et al., 2019). NOX4 is abundantly expressed in NSCLC tissues and contribute to tumor development through diverse oncogenic mechanisms (Zhang et al., 2019). For instance, NOX4 could reprogram the metabolic phenotype of NSCLC cells to enhance glycolysis and pentose phosphate pathway in A549 and H460 cells. Specifically, NOX4 upregulates the expression of c-Myc, a crucial transcriptional factor for activating glycolytic enzymes, including glucose transporter 1, hexokinase 2, pyruvate kinase isoform 2 and lactate dehydrogenase A, to support the glycolytic phenotype of A549 cells via ROS/PI3K/Akt activation (Zeng et al., 2016; Fang et al., 2019). Nuclear factor erythroid 2-related factor 2 (Nrf2) mediates the modulation of redox adaptation in NOX4-overexpressed NSCLC cells. NOX4-induced ROS maintains the stability of Nrf2 protein by preventing ROS-related proteasomal degradation via PI3K activation (Wu et al., 2017). Besides, NOX4-induced ROS could also elevate cytokine production via PI3K/Akt signaling-dependent manner to increase tumor-associated macrophage infiltration and exert pro-tumor function in NSCLC (Zhang et al., 2019). Cisplatin-based chemotherapy has been regarded as a traditional and primary treatment to prevent the relapse of NSCLC and improve the clinical outcomes of NSCLC patients. However, cisplatin resistance remains a huge obstacle for therapeutic response in NSCLC patients. In NSCLC, EF-hand domain-containing protein D2 (EFHD2) could upregulate NOX4-induced ROS production of A549 and H1299 cells, which ultimately increases transporter ATP-binding cassette subfamily C member 1 expression to elevate cisplatin efflux. A non-steroidal anti-inflammatory drug ibuprofen, which can degrade EFHD2 and thus inhibit EFHD2 expression, could improve the therapeutic response of NSCLC to cisplatin via inhibiting NOX4-ROS-ABCC1 axis (Fan et al., 2020). Hence, NOX4 inhibition may serve as a promising treatment for NSCLC patients.
Renal Cell Cancer
RCC, which commonly exhibits loss of Von Hippel-Lindau (VHL) suppressor gene, represents the majority of cases with kidney cancer (D'Avella et al., 2020; Sung et al., 2021). Higher NOX4 expression has been found to be correlated with unfavorable survival in RCC (Kaushik et al., 2020). Precious study has revealed that NOX4 expression in RCC tissues mediates the expression and activity of hypoxia-inducible factor 2α (HIF-2α), a crucial transcriptional factor of tumor glycolysis and various malignant behavior, to support renal tumorigenesis (Maranchie and Zhan, 2005; Hoefflin et al., 2020; Singhal et al., 2021). It is worth noting that NOX4 could alter the distribution of HIF-2α via redox adaptation. Specifically, NOX4 silencing in 786-0 and RCC4 NS cells could reduce nuclear accumulation of HIF-2α under both normal and hypoxic oxygen conditions, indicating NOX4 as an alternative activating signal for HIF-2α translocation (Gregg et al., 2014). It is demonstrated that NOX4 also serves as a mitochondrial energetic sensor engaged in reprogramming tumor metabolism for drug resistance. Of note, NOX4 has an ATP-binding motif to directly bind to ATP, which negatively regulates NOX4 activity in VHL-deficient RCC. Further, NOX4-induced ROS in 786-O and A498 cells could reduce the acetylation of pyruvate kinase-M2 isoform from being degraded mediated by P300/CBP-related factor. NOX4 inhibition, through pyruvate kinase-M2, sensitizes 786-O and A498 cells to drug-induced cell death in xenograft models and ex vivo cultures (Shanmugasundaram et al., 2017). Recent study also elucidated that NOX4 as a renal-enriched ROS-generating enzyme essential for lipid peroxidation and ferroptosis in RCC. The expression of NOX4 can be induced by activation of Hippo-YAP/TAZ pathway to further enhance lipid peroxidation, which mediates susceptibility of RCC4 and 786O cells to ferroptosis (Yang et al., 2019; Yang and Chi, 2019). Besides, NOX4 could increase hypoxia-induced IL-6 and IL-8 production in RCC, linking NOX4 to inflammation-induced RCC metastasis and making NOX4 a therapeutic target to reduce IL-6- and IL-8-induced inflammation and invasion in RCC (Fitzgerald et al., 2012). According to these observations, NOX4 blockade might aid the development of therapeutic intervention of RCC, especially in TAZ-activated CRC cells.
Gastrointestinal Cancer
GC is the fifth most frequently diagnosed malignancies and fourth common cause of cancer-related deaths globally (Sung et al., 2021). NOX4 exhibits upregulated expression in GC tumor tissue compared with adjacent normal tissues (Du et al., 2019). Moreover, anoikis-resistant MKN-45 and AGS cells exhibit enhanced malignant phenotypes, which can be attenuated by NOX4 blockade. This can be explained by the finding that detachment from the ECM drives NOX4 overexpression, and NOX4-induced ROS could directly upregulate EGFR expression, further increasing anoikis resistance of GC cells (Du et al., 2018). Besides, NOX4 mediates the upregulation of GLI1, a transcription regulator for the Hedgehog signaling pathway (Doheny et al., 2020). NOX4 overexpression could promote MKN-45 and AGS cell proliferation via activation of the GLI1 pathway, while GLI1 knockdown reverses the malignant phenotype induced by NOX4 overexpression (Tang et al., 2018). Thus, NOX4 is a promising therapeutic target for blockage of GC development.
CRC represents the third most frequent cancer, but second in terms of mortality (Sung et al., 2021). Upregulation of NOX4 expression has been found to be strongly correlated with myofibroblastic cancer-associated fibroblasts (CAFs). In fibroblasts, NOX4 inhibition abrogates ROS production, blocking myofibroblast differentiation and CAF accumulation. NOX4 inhibition can revert the myofibroblastic-CAF phenotype, which indicates NOX4 inhibition as a stromal-targeted approach for CRC (Hanley et al., 2018). Besides, CRC development are highly correlated with metabolic disorders, like dyslipidemia (Chen et al., 2021). It is demonstrated that NOX4 regulate oleic acid (OA)-drived CRC metastasis. OA-induced ANGPTL4 upregulates NOX4 expression via the activation of c-Jun, which further increase ROS production to promote SW480, and HT-29 cell metastasis (Shen et al., 2020). Targeting this ANGPTL4/NOX4 axis may provide promising therapeutic opportunity for dyslipidemia-associated CRC metastasis. Recent study has investigated the role of NOX4 in the survivin-associated adaptive response in colorectal cells. NOX4 inhibition induces the reversal of HCT116 cell TP53 WT and HCT116 cell Mut adaptive responses from pro-survival to radio-sensitization (Murley et al., 2018). Besides, the anti-tumor effect of silver nanoparticles (AgNP) has also been investigated in CRC tumors. AgNPs induce ROS production and endoplasmic reticulum stress responses through NOX4, leading to HCT116 cell apoptosis (Quan et al., 2021). All these evidences prove that NOX4 blockade could be a potential target in CRC treatment.
Pancreatic Cancer
Pancreatic cancer ranks seventh in terms of mortality globally with a usually poor prognosis, while pancreatic ductal adenocarcinoma (PDAC) accounts for more than 80% of pancreatic cancer cases (Sung et al., 2021). In the membrane of endoplasmic reticulum (ER), high NADPH levels upregulates NOX4 expression. Peroxiredoxin 4 (PRDX4), an antioxidant protein expressed in the ER, is essential for growth and survival of MIAPaCa-2 and PANC-1 cells depending on NOX4-induced ROS production (Jain et al., 2021). In response to hypoxia, HIF-1α could activate NOX4 to elevate the methylation modification of histone H3, thus increasing the transcriptional upregulation of EMT-related marker to enhance metastasis. NOX4 blockade impairs the activation of the HPAC and Panc1 cell survival kinase AKT by attenuating phosphorylation of AKT (Li et al., 2021). NOX4 activates phosphorylation of Janus kinase 2 to exert anti-apoptotic effects. NOX4 activation could impair activity of protein tyrosine phosphatases to increase Janus kinase 2 to impede PaCa cell apoptosis (Lee et al., 2007). NOX4-induced ROS can also protect tumor cells from apoptosis via AKT-dependent phosphorylation of apoptosis signal-regulating kinase 1, thus NOX4 blockade resulting in cell apoptosis (Mochizuki et al., 2006). Oncogenic Kras and inactivation of p16 regulate the expression of NOX4 in PDAC, which could enhance glycolysis by increasing supplement of NAD+. NAD+ functions as a primary substrate for GAPDH-mediated glycolysis to strengthen PDAC growth (Ju et al., 2017). In cachectic muscles, tumor-induced SIRT1 loss leads to the activation of NF-κB, which upregulates NOX4 expression to exaggerate pancreatic tumor-induced cachexia. Therefore, targeting the Sirt1-NOX4 axis in cachectic muscles may provide a promising opportunity to overcome cachexia in patients with pancreatic cancer.
Glioblastoma
Glioblastoma is one of the most lethal and common brain tumors, with the 2-years overall survival of glioblastoma patients exhibiting only 25% (Aldape et al., 2003; van Tellingen et al., 2015). Increased NOX4 expression has been observed in glioblastoma tissues (Shono et al., 2008). Cycling hypoxia is an environmental cue for participating in tumor progression. Cycling hypoxic glioblastoma cells exhibit significantly upregulated NOX4 expression and ROS production compared with normoxic cells (Hsieh et al., 2012). Cycling hypoxia drives NOX4 expression to promote resistance to radiotherapy in glioblastoma. Ferroptosis is an important type of programmed cell death and intensively related to iron-associated lipid peroxidation and intracellular homeostasis, which is involved in occurrence and development of tumor. In glioblastoma, increased iron level induced by pseudolaric acid B treatment via upregulation of transferrin receptor could upregulate NOX4 expression, thus leading to accumulated lipid peroxides (Wang et al., 2018). NOX4 also participates in the regulation of TGF-β1-drived metabolic rewiring of glioblastoma cells (Su et al., 2021a). NOX4-induced ROS production further increases HIF-1α nuclear accumulation and stability to enhance aerobic glycolysis and EMT program of U87 and A172 cells. NOX4-induced ROS also increases FOXM1 transcription to mediate HIF-1α stabilization to enhance aerobic glycolysis (Su et al., 2021b). These findings have elucidated NOX4 as a crucial factor for supporting malignant behaviors of glioblastoma cells.
NOX4 and Other Malignancies
NOX4 also participates in the regulation of other cancer types, including prostate cancer, gallbladder cancer, thyroid cancer, and ovarian cancer (Sampson et al., 2018; Helfinger et al., 2019; Pan et al., 2020; Liu et al., 2021). In human prostate cancer, NOX4 mediates TGFβ1-induced activation of primary fibroblasts to acquire a CAF-associated phenotype. NOX4 blockade could diminish CAF-associated marker expression and migrative capabilities of CAFs (Sampson et al., 2018). NOX4 is highly upregulated in gallbladder cancer cells and gallbladder CAFs, which is associated with malignant behaviors and poor prognosis. Specifically, gallbladder CAFs elevate vasculogenic mimicry formation and tumor growth through increasing NOX4 expression via activating IL-6-JAK-STAT3 signaling (Pan et al., 2020). In thyroid cancer, knockdown of NOX4 and p22phox in hypoxia reduces ROS level, thus destabilizing HIF1α to restraining glycolysis and cell growth of TPC-1 cells (Helfinger et al., 2019). NOX4 also mediates the resistance to chemotherapy and radiotherapy in ovarian cancer cells. NOX4 blockade could significantly elevate response of A2780 and OVCAR3 cells to chemotherapy and radiotherapy by downregulating HER3 and NF-κB p65 (Liu et al., 2021). Taken together, NOX4 displays great significance in wide variety of cancers, indicating great potentials of NOX4 inhibitors as anti-tumor therapy.
Prospects of NOX4 Inhibitors in Cancer Therapy
With the acknowledgements to the role of NOX4 in tumor occurrence and development, NOX4 is an emerging therapeutic target for development of anti-tumor drug. NOX4 inhibitors, including GKT137831, fulvene-5, and diphenyleneiodonium (DPI), are being extensively studied. Currently, GKT137831 is the only NOX4 inhibitors that has been tested in clinical trials. GKT137831 can revert the myofibroblastic-CAF phenotype and promote CD8+ T cell infiltration (Hanley et al., 2018). GKT137831 also attenuates prostate cancer cell-driven fibroblast activation to mediate tumor stromal interactions (Sampson et al., 2018). In NSCLC, NOX4 blockade by GKT137831 could reduce pro-tumoral M2-like macrophage and immune infiltration to limit tumor growth (Zhang et al., 2019). In tumors with high CAF levels, GKT137831 can increase immunotherapy response. Moreover, immunotherapy resistance can be impeded by NOX4 blockade and favor prognosis in multiple cancers (Ford et al., 2020). Fulvene-5 has been confirmed to be a potential NOX4 inhibitor. In hemangioma, fulvene-5 impairs NOX4 activity and inhibits hemangioma growth in vivo (Bhandarkar et al., 2009). Fulvene-5 could revert adaptive responses of CRC cells from pro-survival to radio-sensitization (Murley et al., 2018). As a pan NOX inhibitor, DPI inhibits phosphorylation of STAT3 and STAT5, and drives PARP1 cleavage in JAK2V617F-positive cells (Lima et al., 2021). DPI can also eliminate ROS production to inhibit tumor progression in ovarian, prostate and lung cancer (Xia et al., 2007; Ma et al., 2021). Even though NOX4 inhibitors have been evaluated in multiple cancer types for its anti-tumor activity in vitro and vivo, it is still required to explore deeper to identify the cancer types that can be effectively treated with NOX4 inhibitors. Additionally, clinical trials should be accelerated to evaluate the therapeutic effects of more novel NOX4 inhibitors in different cancer types. Besides, it is necessary to exploit potential biomarkers that sensitize tumor cells for NOX4 blockade. Given the role of NOX4 in ROS production, combination therapies with ROS scavenger should be further explored.
Conclusion
Precious studies have clearly introduced NOX4 as a key regulator of cancer occurrence and development in multiple cancer models. Multiple underlying mechanisms by which NOX4-induced ROS production have been elucidated. NOX4-induced ROS production could activate various oncogenic signaling pathway, rewire metabolic phenotype of tumors and reprogram the tumor stroma. Tumor microenvironment is a complex and dynamic network, which contains a heterogeneous composition of immune cells, endothelial cells, and fibroblasts, etc. Worth noticing, NOX4 is a key interface to tumor-stromal cell interactions. For instance, NOX4 inhibitor could restrain fibroblast activation resulting from stimulation of surrounding tumor cells, indicating the value of NOX4 blockade as a stromal-targeted strategies. Currently, it is well-recognized that tumor cells co-opt specific immune checkpoints to regulate resistance to immunotherapy (Pardoll, 2012). Specifically, αPD-1/PD-L1 immune-checkpoint immunotherapy have displayed enormous potential in a wide variety of cancer types (Ai et al., 2020). Thus, exploring underlyingly molecular mechanisms of resistance to immunotherapy is critical for developing effective anti-tumor strategies. NOX4 blockade has shown its potential for enhancing the efficacy of immunotherapy, elucidating the therapeutic potential of NOX4 inhibitor in combination with immunotherapy (Ford et al., 2020). Taken together, NOX4, involved in a variety of biological process in cancers, has promising prospective awaiting for further exploration.
Author Contributions
SG and SW—writing and elaborating the figures. MS —writing and reviewing the final version.
Conflict of Interest
The authors declare that the research was conducted in the absence of any commercial or financial relationships that could be construed as a potential conflict of interest.
Publisher’s Note
All claims expressed in this article are solely those of the authors and do not necessarily represent those of their affiliated organizations, or those of the publisher, the editors and the reviewers. Any product that may be evaluated in this article, or claim that may be made by its manufacturer, is not guaranteed or endorsed by the publisher.
References
Ai, L., Xu, A., and Xu, J. (2020). Roles of PD-1/pd-L1 Pathway: Signaling, Cancer, and beyond. Adv. Exp. Med. Biol. 1248, 33–59. doi:10.1007/978-981-15-3266-5_3
Aldape, K. D., Okcu, M. F., Bondy, M. L., and Wrensch, M. (2003). Molecular Epidemiology of Glioblastoma. Cancer J. 9, 99–106. doi:10.1097/00130404-200303000-00005
Bedard, K., and Krause, K.-H. (2007). The NOX Family of ROS-Generating NADPH Oxidases: Physiology and Pathophysiology. Physiol. Rev. 87, 245–313. doi:10.1152/physrev.00044.2005
Bedard, K., Lardy, B., and Krause, K. (2007). NOX Family NADPH Oxidases: Not Just in Mammals. Biochimie 89, 1107–1112. doi:10.1016/j.biochi.2007.01.012
Bhandarkar, S. S., Jaconi, M., Fried, L. E., Bonner, M. Y., Lefkove, B., and Govindarajan, B. (2009). Fulvene-5 Potently Inhibits NADPH Oxidase 4 and Blocks the Growth of Endothelial Tumors in Mice. J. Clin. Invest. 119, 2359–2365. doi:10.1172/JCI33877
Brandes, R. P., Weissmann, N., and Schröder, K. (2014b). Nox Family NADPH Oxidases in Mechano-Transduction: Mechanisms and Consequences. Antioxid. Redox Signal 20, 887–898. doi:10.1089/ars.2013.5414
Brandes, R. P., Weissmann, N., and Schröder, K. (2014a). Nox Family NADPH Oxidases: Molecular Mechanisms of Activation. Free Radic. Biol. Med. 76, 208–226. doi:10.1016/j.freeradbiomed.2014.07.046
Brieger, K., Schiavone, S., Miller, F. J., and Krause, K. H. (2012). Reactive Oxygen Species: from Health to Disease. Swiss Med. Wkly. 142, w13659. doi:10.4414/smw.2012.13659
Chen, H., Zheng, X., Zong, X., Li, Z., Li, N., Hur, J., et al. (2021). Metabolic Syndrome, Metabolic Comorbid Conditions and Risk of Early-Onset Colorectal Cancer. Gut 70, 1147–1154. Epub 2020 Oct 9. doi:10.1136/gutjnl-2020-321661
D'Avella, C., Abbosh, P., Pal, S. K., and Geynisman, D. M. (2020). Mutations in Renal Cell Carcinoma. Urologic Oncol. Seminars Orig. Investigations 38, 763–773. doi:10.1016/j.urolonc.2018.10.027
Doheny, D., Manore, S. G., Wong, G. L., and Lo, H.-W. (2020). Hedgehog Signaling and Truncated GLI1 in Cancer. Cells 9, 2114. doi:10.3390/cells9092114
Douda, D. N., Khan, M. A., Grasemann, H., and Palaniyar, N. (2015). SK3 Channel and Mitochondrial ROS Mediate NADPH Oxidase-independent NETosis Induced by Calcium Influx. Proc. Natl. Acad. Sci. U.S.A. 112, 2817–2822. doi:10.1073/pnas.1414055112
Du, S., Miao, J., Lu, X., Shi, L., Sun, J., Xu, E., et al. (2019). NADPH Oxidase 4 Is Correlated with Gastric Cancer Progression and Predicts a Poor Prognosis. Am. J. Transl. Res. 11, 3518–3530. eCollection 2019.
Du, S., Miao, J., Zhu, Z., Xu, E., Shi, L., Ai, S., et al. (2018). NADPH Oxidase 4 Regulates Anoikis Resistance of Gastric Cancer Cells through the Generation of Reactive Oxygen Species and the Induction of EGFR. Cell. Death Dis. 9, 948. doi:10.1038/s41419-018-0953-7
Fan, C. C., Tsai, S. T., Lin, C. Y., Chang, L. C., Yang, J. C., Chen, G. Y., et al. (2020). EFHD2 Contributes to Non-small Cell Lung Cancer Cisplatin Resistance by the Activation of NOX4-ROS-ABCC1 axis. Redox Biol. 34, 101571. doi:10.1016/j.redox.2020.101571
Fang, Y., Shen, Z.-Y., Zhan, Y.-Z., Feng, X.-C., Chen, K.-L., Li, Y.-S., et al. (2019). CD36 Inhibits β-catenin/c-myc-mediated Glycolysis through Ubiquitination of GPC4 to Repress Colorectal Tumorigenesis. Nat. Commun. 10, 3981. doi:10.1038/s41467-019-11662-3
Fitzgerald, J. P., Nayak, B., Shanmugasundaram, K., Friedrichs, W., Sudarshan, S., Eid, A. A., et al. (2012). Nox4 Mediates Renal Cell Carcinoma Cell Invasion through Hypoxia-Induced Interleukin 6- and 8- Production. PLoS One 7, e30712. doi:10.1371/journal.pone.0030712
Ford, K., Hanley, C. J., Mellone, M., Szyndralewiez, C., Heitz, F., Wiesel, P., et al. (2020). NOX4 Inhibition Potentiates Immunotherapy by Overcoming Cancer-Associated Fibroblast-Mediated CD8 T-Cell Exclusion from Tumors. Cancer Res. 80, 1846–1860. Epub 2020 Mar 2. doi:10.1158/0008-5472.CAN-19-3158
Gregg, J. L., Turner, R. M., Chang, G., Joshi, D., Zhan, Y., Chen, L., et al. (2014). NADPH Oxidase NOX4 Supports Renal Tumorigenesis by Promoting the Expression and Nuclear Accumulation of HIF2alpha. Cancer Res. 74, 3501–3511. doi:10.1158/0008-5472.CAN-13-2979
Hanley, C. J., Mellone, M., Ford, K., Thirdborough, S. M., Mellows, T., Frampton, S. J., et al. (2018). Targeting the Myofibroblastic Cancer-Associated Fibroblast Phenotype through Inhibition of NOX4. J. Natl. Cancer Inst. 110, 109–120. doi:10.1093/jnci/djx121
Helfinger, V., Palfi, K., Weigert, A., and Schröder, K. (2019). The NADPH Oxidase Nox4 Controls Macrophage Polarization in an NFkappaB-dependent Manner. Oxid. Med. Cell. Longev. 2019, 3264858. doi:10.1155/2019/3264858
Hoefflin, R., Harlander, S., Schäfer, S., Metzger, P., Kuo, F., Schönenberger, D., et al. (2020). HIF-1α and HIF-2α Differently Regulate Tumour Development and Inflammation of Clear Cell Renal Cell Carcinoma in Mice. Nat. Commun. 11, 4111. doi:10.1038/s41467-020-17873-3
Hsieh, C. H., Wu, C. P., Lee, H. T., Liang, J. A., Yu, C. Y., and Lin, Y. J. (2012). NADPH Oxidase Subunit 4 Mediates Cycling Hypoxia-Promoted Radiation Resistance in Glioblastoma Multiforme. Free Radic. Biol. Med. 53, 649–658. doi:10.1016/j.freeradbiomed.2012.06.009
Jain, P., Dvorkin-Gheva, A., Mollen, E., Malbeteau, L., Xie, M., Jessa, F., et al. (2021). NOX4 Links Metabolic Regulation in Pancreatic Cancer to Endoplasmic Reticulum Redox Vulnerability and Dependence on PRDX4. Sci. Adv. 7, eabf7114. doi:10.1126/sciadv.abf7114
Ju, H. Q., Ying, H., Tian, T., Ling, J., Fu, J., Lu, Y., et al. (2017). Mutant Kras- and P16-Regulated NOX4 Activation Overcomes Metabolic Checkpoints in Development of Pancreatic Ductal Adenocarcinoma. Nat. Commun. 8, 14437. doi:10.1038/ncomms14437
Kaushik, D., Ashcraft, K. A., Wang, H., Shanmugasundaram, K., Shah, P. K., Gonzalez, G., et al. (2020). Nuclear NADPH Oxidase-4 Associated with Disease Progression in Renal Cell Carcinoma. Transl. Res. 223, 1–14. doi:10.1016/j.trsl.2020.05.009
Klaunig, J. E. (2019). Oxidative Stress and Cancer. Cpd 24, 4771–4778. doi:10.2174/1381612825666190215121712
Lee, J. K., Edderkaoui, M., Truong, P., Ohno, I., Jang, K. T., Berti, A., et al. (2007). NADPH Oxidase Promotes Pancreatic Cancer Cell Survival via Inhibiting JAK2 Dephosphorylation by Tyrosine Phosphatases. Gastroenterology 133, 1637–1648. doi:10.1053/j.gastro.2007.08.022
Leto, T. L., Morand, S., Hurt, D., and Ueyama, T. (2009). Targeting and Regulation of Reactive Oxygen Species Generation by Nox Family NADPH Oxidases. Antioxid. Redox Signal 11, 2607–2619. doi:10.1089/ars.2009.2637
Li, H., Peng, C., Zhu, C., Nie, S., Qian, X., Shi, Z., et al. (2021). Hypoxia Promotes the Metastasis of Pancreatic Cancer through Regulating NOX4/KDM5A-Mediated Histone Methylation Modification Changes in a HIF1A-independent Manner. Clin. Epigenetics 13, 18. doi:10.1186/s13148-021-01016-6
Lima, K., Lopes, L. R., and Machado-Neto, J. A. (2021). Exploring Redox Vulnerabilities in JAK2V617F-Positive Cellular Models. Hematol. Transfus. Cell. Ther. 43, 430–436. doi:10.1016/j.htct.2020.08.006
Liu, W.-J., Huang, Y.-X., Wang, W., Zhang, Y., Liu, B.-J., Qiu, J.-G., et al. (2021). NOX4 Signaling Mediates Cancer Development and Therapeutic Resistance through HER3 in Ovarian Cancer Cells. Cells 10, 1647. doi:10.3390/cells10071647
Ma, M., Shi, F., Zhai, R., Wang, H., Li, K., Xu, C., et al. (2021). TGF-β Promote Epithelial-Mesenchymal Transition via NF‐κB/NOX4/ROS Signal Pathway in Lung Cancer Cells. Mol. Biol. Rep. 48, 2365–2375. Epub 2021 Apr 1. doi:10.1007/s11033-021-06268-2
Mao, Y., Yang, D., He, J., and Krasna, M. J. (2016). Epidemiology of Lung Cancer. Surg. Oncol. Clin. N. Am. 25, 439–445. doi:10.1016/j.soc.2016.02.001
Maranchie, J. K., and Zhan, Y. (2005). Nox4 is critical for hypoxia-inducible factor 2-alpha transcriptional activity in von Hippel-Lindau-deficient renal cell carcinoma. Cancer Res. 65, 9190–9193. doi:10.1158/0008-5472.CAN-05-2105
Meitzler, J. L., Makhlouf, H. R., Antony, S., Wu, Y., Butcher, D., Jiang, G., et al. (2017). Decoding NADPH Oxidase 4 Expression in Human Tumors. Redox Biol. 13, 182–195. doi:10.1016/j.redox.2017.05.016
Mochizuki, T., Furuta, S., Mitsushita, J., Shang, W. H., Ito, M., Yokoo, Y., et al. (2006). Inhibition of NADPH Oxidase 4 Activates Apoptosis via the AKT/apoptosis Signal-Regulating Kinase 1 Pathway in Pancreatic Cancer PANC-1 Cells. Oncogene 25, 3699–3707. doi:10.1038/sj.onc.1209406
Moloney, J. N., and Cotter, T. G. (2018). ROS Signalling in the Biology of Cancer. Seminars Cell. & Dev. Biol. 80, 50–64. Epub 2017 Jun 3. doi:10.1016/j.semcdb.2017.05.023
Murley, J. S., Arbiser, J. L., Weichselbaum, R. R., and Grdina, D. J. (2018). ROS Modifiers and NOX4 Affect the Expression of the Survivin-Associated Radio-Adaptive Response. Free Radic. Biol. Med. 123, 39–52. doi:10.1016/j.freeradbiomed.2018.04.547
Nagano, T., Tachihara, M., and Nishimura, Y. (2019). Molecular Mechanisms and Targeted Therapies Including Immunotherapy for Non-small Cell Lung Cancer. Ccdt 19, 595–630. doi:10.2174/1568009619666181210114559
Pan, M. S., Wang, H., Ansari, K. H., Li, X. P., Sun, W., and Fan, Y. Z. (2020). Gallbladder Cancer-Associated Fibroblasts Promote Vasculogenic Mimicry Formation and Tumor Growth in Gallbladder Cancer via Upregulating the Expression of NOX4, a Poor Prognosis Factor, through IL-6-JAK-STAT3 Signal Pathway. J. Exp. Clin. Cancer Res. 39, 234. doi:10.1186/s13046-020-01742-4
Pardoll, D. M. (2012). The Blockade of Immune Checkpoints in Cancer Immunotherapy. Nat. Rev. Cancer 12, 252–264. doi:10.1038/nrc3239
Prasad, S., Gupta, S. C., and Tyagi, A. K. (2017). Reactive Oxygen Species (ROS) and Cancer: Role of Antioxidative Nutraceuticals. Cancer Lett. 387, 95–105. Epub 2016 Mar 29. doi:10.1016/j.canlet.2016.03.042
Quan, J.-H., Gao, F. F., Chu, J.-Q., Cha, G.-H., Yuk, J.-M., Wu, W., et al. (2021). Silver Nanoparticles Induce Apoptosis via NOX4-Derived Mitochondrial Reactive Oxygen Species and Endoplasmic Reticulum Stress in Colorectal Cancer Cells. Nanomedicine 16, 1357–1375. doi:10.2217/nnm-2021-0098
Rossary, A., Arab, K., and Steghens, J.-P. (2007). Polyunsaturated Fatty Acids Modulate NOX 4 Anion Superoxide Production in Human Fibroblasts. Biochem. J. 406, 77–83. doi:10.1042/BJ20061009
Sampson, N., Brunner, E., Weber, A., Puhr, M., Schäfer, G., Szyndralewiez, C., et al. (2018). Inhibition of Nox4-dependent ROS Signaling Attenuates Prostate Fibroblast Activation and Abrogates Stromal-Mediated Protumorigenic Interactions. Int. J. Cancer 143, 383–395. doi:10.1002/ijc.31316
Schieber, M., and Chandel, N. S. (2014). ROS Function in Redox Signaling and Oxidative Stress. Curr. Biol. 24, R453–R462. doi:10.1016/j.cub.2014.03.034
Shanmugasundaram, K., Nayak, B. K., Friedrichs, W. E., Kaushik, D., Rodriguez, R., and Block, K. (2017). NOX4 Functions as a Mitochondrial Energetic Sensor Coupling Cancer Metabolic Reprogramming to Drug Resistance. Nat. Commun. 8, 997. doi:10.1038/s41467-017-01106-1
Shen, C. J., Chang, K. Y., Lin, B. W., Lin, W. T., Su, C. M., Tsai, J. P., et al. (2020). Oleic Acid-Induced NOX4 Is Dependent on ANGPTL4 Expression to Promote Human Colorectal Cancer Metastasis. Theranostics 10, 7083–7099. doi:10.7150/thno.44744
Shono, T., Yokoyama, N., Uesaka, T., Kuroda, J., Takeya, R., Yamasaki, T., et al. (2008). Enhanced Expression of NADPH Oxidase Nox4 in Human Gliomas and its Roles in Cell Proliferation and Survival. Int. J. Cancer 123, 787–792. doi:10.1002/ijc.23569
Singhal, R., Mitta, S. R., Das, N. K., Kerk, S. A., Sajjakulnukit, P., Solanki, S., et al. (2021). HIF-2α Activation Potentiates Oxidative Cell Death in Colorectal Cancers by Increasing Cellular Iron. J. Clin. Invest. 131, e143691. doi:10.1172/JCI143691
Su, X., Yang, Y., Guo, C., Zhang, R., Sun, S., Wang, Y., et al. (2021a). NOX4-Derived ROS Mediates TGF-Beta1-Induced Metabolic Reprogramming during Epithelial-Mesenchymal Transition through the PI3K/AKT/HIF-1alpha Pathway in Glioblastoma. Oxid. Med. Cell. Longev. 2021, 5549047. doi:10.1155/2021/5549047
Su, X., Yang, Y., Yang, Q., Pang, B., Sun, S., Wang, Y., et al. (2021b). NOX4-derived ROS-Induced Overexpression of FOXM1 Regulates Aerobic Glycolysis in Glioblastoma. BMC Cancer 21, 1181. doi:10.1186/s12885-021-08933-y
Sung, H., Ferlay, J., Siegel, R. L., Laversanne, M., Soerjomataram, I., Jemal, A., et al. (2021). Global Cancer Statistics 2020: GLOBOCAN Estimates of Incidence and Mortality Worldwide for 36 Cancers in 185 Countries. CA Cancer J. Clin. 71, 209–249. doi:10.3322/caac.21660
Tang, C. T., Lin, X. L., Wu, S., Liang, Q., Yang, L., Gao, Y. J., et al. (2018). NOX4-driven ROS Formation Regulates Proliferation and Apoptosis of Gastric Cancer Cells through the GLI1 Pathway. Cell. Signal 46, 52–63. doi:10.1016/j.cellsig.2018.02.007
van Tellingen, O., Yetkin-Arik, B., de Gooijer, M. C., Wesseling, P., Wurdinger, T., and de Vries, H. E. (2015). Overcoming the Blood-Brain Tumor Barrier for Effective Glioblastoma Treatment. Drug Resist. Updat. 19, 1–12. Epub 2015 Mar 6. doi:10.1016/j.drup.2015.02.002
Vermot, A., Petit-Härtlein, I., Smith, S. M. E., and Fieschi, F. (2021). NADPH Oxidases (NOX): An Overview from Discovery, Molecular Mechanisms to Physiology and Pathology. Antioxidants (Basel) 10, 890. doi:10.3390/antiox10060890
Wang, Z., Ding, Y., Wang, X., Lu, S., Wang, C., He, C., et al. (2018). Pseudolaric Acid B Triggers Ferroptosis in Glioma Cells via Activation of Nox4 and Inhibition of xCT. Cancer Lett. 428, 21–33. doi:10.1016/j.canlet.2018.04.021
Wu, Q., Yao, B., Li, N., Ma, L., Deng, Y., Yang, Y., et al. (2017). Nrf2 Mediates Redox Adaptation in NOX4-Overexpressed Non-small Cell Lung Cancer Cells. Exp. Cell. Res. 352, 245–254. doi:10.1016/j.yexcr.2017.02.014
Wu, Z., Zuo, M., Zeng, L., Cui, K., Liu, B., Yan, C., et al. (2021). OMA1 Reprograms Metabolism under Hypoxia to Promote Colorectal Cancer Development. EMBO Rep. 22, e50827. doi:10.15252/embr.202050827
Xia, C., Meng, Q., Liu, L.-Z., Rojanasakul, Y., Wang, X.-R., and Jiang, B.-H. (2007). Reactive Oxygen Species Regulate Angiogenesis and Tumor Growth through Vascular Endothelial Growth Factor. Cancer Res. 67, 10823–10830. doi:10.1158/0008-5472.CAN-07-0783
Yang, W.-H., and Chi, J.-T. (2019). Hippo Pathway Effectors YAP/TAZ as Novel Determinants of Ferroptosis. Mol. Cell. Oncol. 7, 1699375. doi:10.1080/23723556.2019.1699375
Yang, W. H., Ding, C. C., Sun, T., Rupprecht, G., Lin, C. C., Hsu, D., et al. (2019). The Hippo Pathway Effector TAZ Regulates Ferroptosis in Renal Cell Carcinoma. Cell. Rep. 28, 2501–2508. doi:10.1016/j.celrep.2019.07.107
Yang, Y., Bazhin, A. V., Werner, J., and Karakhanova, S. (2013). Reactive Oxygen Species in the Immune System. Int. Rev. Immunol. 32, 249–270. doi:10.3109/08830185.2012.755176
Zeng, C., Wu, Q., Wang, J., Yao, B., Ma, L., Yang, Z., et al. (2016). NOX4 Supports Glycolysis and Promotes Glutamine Metabolism in Non-small Cell Lung Cancer Cells. Free Radic. Biol. Med. 101, 236–248. doi:10.1016/j.freeradbiomed.2016.10.500
Zhang, C., Lan, T., Hou, J., Li, J., Fang, R., Yang, Z., et al. (2014). NOX4 Promotes Non-small Cell Lung Cancer Cell Proliferation and Metastasis through Positive Feedback Regulation of PI3K/Akt Signaling. Oncotarget 5, 4392–4405. doi:10.18632/oncotarget.2025
Zhang, J., Wang, X., Vikash, V., Ye, Q., Wu, D., Liu, Y., et al. (20162016). ROS and ROS-Mediated Cellular Signaling. Oxidative Med. Cell. Longev. 2016, 1–18. doi:10.1155/2016/4350965
Keywords: reactive oxygen species, tumor microenevironment, NOX4, cancer, metabolism
Citation: Gong S, Wang S and Shao M (2022) NADPH Oxidase 4: A Potential Therapeutic Target of Malignancy. Front. Cell Dev. Biol. 10:884412. doi: 10.3389/fcell.2022.884412
Received: 26 February 2022; Accepted: 27 April 2022;
Published: 11 May 2022.
Edited by:
Chunxiao Liu, University of Texas MD Anderson Cancer Center, United StatesReviewed by:
Ana Cipak Gasparovic, Rudjer Boskovic Institute, CroatiaCopyright © 2022 Gong, Wang and Shao. This is an open-access article distributed under the terms of the Creative Commons Attribution License (CC BY). The use, distribution or reproduction in other forums is permitted, provided the original author(s) and the copyright owner(s) are credited and that the original publication in this journal is cited, in accordance with accepted academic practice. No use, distribution or reproduction is permitted which does not comply with these terms.
*Correspondence: Mingrui Shao, smrcmu@sina.com