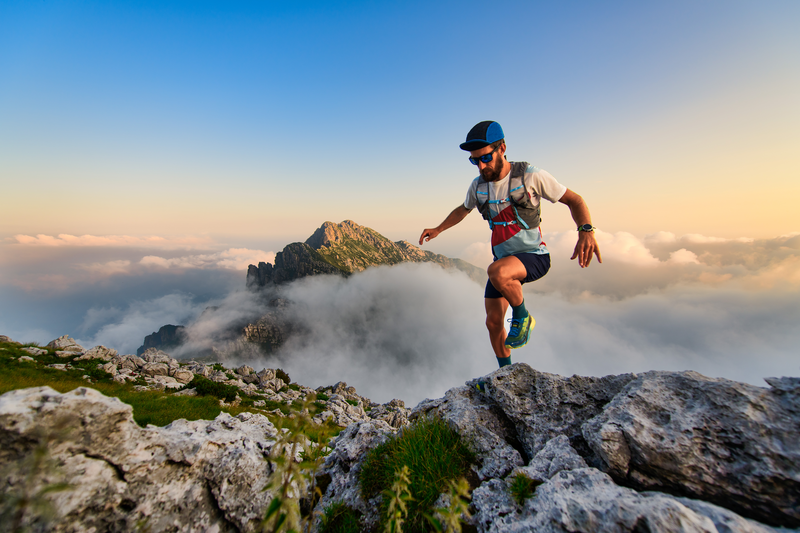
94% of researchers rate our articles as excellent or good
Learn more about the work of our research integrity team to safeguard the quality of each article we publish.
Find out more
BRIEF RESEARCH REPORT article
Front. Cell Dev. Biol. , 18 May 2022
Sec. Cancer Cell Biology
Volume 10 - 2022 | https://doi.org/10.3389/fcell.2022.876510
This article is part of the Research Topic Therapy Resistance in Tumor Microenvironment: Metabolic Reprogramming and Purinergic Signaling View all 6 articles
ATP and adenosine are key constituents of the tumor niche where they exert opposite and complementary roles. ATP can be released in response to cell damage or actively released by tumor cells and subsequently degraded into adenosine, which accumulates within the tumor microenvironment. Notably, while ATP promotes immune eradicating responses mainly via the P2X7 receptor (P2X7R), extracellular adenosine acts as a potent immune suppressor and facilitates neovascularization thanks to the A2A receptor (A2AR). To date, studies exploring the interplay between P2X7R and A2AR in the tumor microenvironment are as yet missing. Here, we show that, in C57/bl6 P2X7 null mice inoculated with B16-F10 melanoma cells, several pro-inflammatory cytokines, including interleukin 1 beta (IL-1β), tumor necrosis factor alpha (TNF-α), interleukin 6 (IL-6), interleukin 12 (IL-12), interleukin 17 (IL-17), interferon gamma (IFN-γ) were significantly decreased, while the immune suppressant transforming growth factor beta (TGF-β) was almost three-fold increased. Interestingly, tumors growing in P2X7-null mice upregulated tumor-associated and splenic A2AR, suggesting that immunosuppression linked to lack of the P2X7R might depend upon A2AR overexpression. Immunohistochemical analysis showed that tumor cells’ A2AR expression was increased, especially around necrotic areas, and that vascular endothelial growth factor (VEGF) and the endothelial marker CD31 were upregulated. A2AR antagonist SCH58261 treatment reduced tumor growth similarly in the P2X7 wild type or null mice strain. However, SCH58261 reduced VEGF only in the P2X7 knock out mice, thus supporting the hypothesis of an A2AR-mediated increase in vascularization observed in the P2X7-null host. SCH58261 administration also significantly reduced intratumor TGF-β levels, thus supporting a key immune suppressive role of A2AR in our model. Altogether, these results indicate that in the absence of host P2X7R, the A2AR favors tumor growth via immune suppression and neovascularization. This study shows a novel direct correlation between P2X7R and A2AR in oncogenesis and paves the way for new combined therapies promoting anti-cancer immune responses and reducing tumor vascularization.
The P2X7 receptor (P2X7R) is an ATP gated ion channel known to be central in inflammation for its ability to activate the NLRP3 inflammasome and trigger IL-1β release (Di Virgilio et al., 2017; Orioli et al., 2017; Adinolfi et al., 2018; Pelegrin, 2021). In the context of cancer, P2X7R has been assigned multiple and often contrasting roles as a driver of cancer cell growth (Di Virgilio et al., 2018; Scarpellino et al., 2019) and metastatic dissemination (Di Virgilio et al., 2016; Ulrich et al., 2018; Pegoraro et al., 2021), or as a promoter of immune-mediated tumor eradication (Adinolfi et al., 2015; Adinolfi et al., 2019; De Marchi et al., 2019; Lara et al., 2020). Interestingly, approaches based on either P2X7R antagonism or agonism have proved effective in reducing tumor growth, thus leaving many open questions on the mechanisms underlying P2X7R activity in cancer (Adinolfi et al., 2012; Amoroso et al., 2015; Douguet et al., 2021; Drill et al., 2021). The abundance of P2X7R ligand extracellular ATP (eATP) is a well-recognized characteristic of the tumor microenvironment (TME) (Di Virgilio et al., 2018; De Marchi et al., 2020). The TME is also rich in the ATP hydrolysis derivative extracellular adenosine (eADO) (Boison and Yegutkin, 2019). These two molecules exert opposing actions on the immune system, as, while eATP (via P2X7R) is pro-inflammatory and promotes anti-tumor immune response (Kepp et al., 2021), adenosine acts as an immunosuppressant, thus facilitating tumor immune escape (Leone and Emens, 2018; Young et al., 2018; Allard et al., 2020; Antonioli et al., 2021). However, the effects of both eATP and eADO are not limited to activity on immune cells as often through the same receptors expressed by either tumor cells or surrounding stroma they also promote cancer growth, vascularization and metastasis (Di Virgilio et al., 2016; Borea et al., 2018; Scarpellino et al., 2019; Arnaud-Sampaio et al., 2020; Sitkovsky, 2020).
In the TME, eADO accumulation causes suppression of immune effector functions and promotes tumor progression (Allard et al., 2020). These effects are mediated via A2A and A2B receptors (A2AR, A2BR), which are expressed by immune stromal and tumor cells. These receptors are both coupled to Gs protein and differ for their ligand binding affinity as the A2AR has a high (Kd: 310 nM), while A2BR has a low affinity (Kd 15 μM) (Yegutkin, 2008). The A2AR, being ubiquitously expressed in the body, especially in the immune system, has emerged as the major adenosine receptor subtype in downregulating inflammation induced by injury, ischemia or infection in a wide variety of diseases (Hasko and Pacher, 2008), in a cyclic AMP (cAMP)/protein kinase A (PKA)-dependent manner, regulating the activity of various transcriptions factors (Nemeth et al., 2003; De Ponti et al., 2007; Ansari et al., 2017; Ahmad et al., 2018). In the context of cancer, the A2AR has been widely studied, emerging as a crucial mediator of eADO effects in the TME (Ohta et al., 2006; Boison and Yegutkin, 2019). Its activation potently suppresses both B and T cells’ effector activity while promoting the accumulation of regulatory T cells (Tregs), which are known to be immunosuppressive (Sorrentino et al., 2013; Antonioli et al., 2021). Furthermore, stimulation of A2AR, on macrophages populating the tumor lesion, promotes the differentiation of M2-like macrophages, which are the major responsible for the accumulation of vascular endothelial growth factor (VEGF) in the TME and the subsequential angiogenesis (Sitkovsky et al., 2014; Allard et al., 2020). Importantly, A2AR not only promotes the establishment of a pro-tumorigenic environment driving macrophage differentiation but also mediates the direct effects of eADO on tumor cells, as shown by the finding that in animal models, tumor cell A2AR promotes invasiveness, motility and proliferation (Beavis et al., 2013; Koszalka et al., 2016; Allard et al., 2019; Kamai et al., 2021).
The eATP and eADO levels are strictly intertwined. In the extracellular milieu, eADO is generated starting from eATP via two ectoenzymes, CD39 and CD73, which are also upregulated in the tumor lesion, favoring cancer growth through eADO-mediated immune suppression (Allard et al., 2020; Battastini et al., 2021). We have recently demonstrated that, in an implanted murine melanoma model, P2X7R genetic knockdown or pharmacological antagonism modulates CD39 and CD73 levels on Tregs, CD4+ effector lymphocytes, macrophage and dendritic cells (De Marchi et al., 2019). Changes in ectonucleotidases expression correlate with alterations in eATP levels (De Marchi et al., 2019), strongly suggesting that P2X7R expression and function affect the eATP/eADO axis in the TME. Independent studies also indicated a key role of the P2X7R in anti-tumor, CD39-targeting therapies (Li et al., 2019; Yan et al., 2020). Despite this wealth of studies, to our knowledge, no studies demonstrating a correlation between P2X7R and A2AR activity in cancer have been carried out so far. Here we show that the A2AR is upregulated in melanoma bearing P2X7R-null mice and highlight a pivotal role for the A2AR in immune suppression and neovascularization promoted by the absence of the P2X7R. We thus demonstrate, for the first time, a P2X7R-A2AR centered crosstalk in tumorigenesis.
Mouse B16-F10 (ATCC CRL-6475) melanoma cell lines were purchased from Sigma-Aldrich and periodically tested with MycoAlertTM kit (Lonza, Switzerland). B16-F10 were grown in RPMI-1640 medium (Sigma-Aldrich) plus non-essential amino acids (Sigma-Aldrich), FBS (10%), penicillin (100 U/ml), and streptomycin (100 mg/ml) all purchased from Euroclone, Milan, Italy.
In vivo experiments were performed with a p2x7−/− mice in the C57bl/6 strain and corresponding wild type controls (Adinolfi et al., 2015; De Marchi et al., 2019). Based on calculations performed with the G-power software (Faul et al., 2007) on previously published data (De Marchi et al., 2019), a minimal sample size of six animals per group was chosen to achieve a predicted power of 0.85 with an effect size of 1.78 using a two-tailed t-test. C57bl/6 mice were fed with standard rodent chow diet and water ad libitum and were given 1 week to acclimatize after their delivery to the animal facility. The animals were maintained at 22–24°C with a 12 h light cycle in a specific pathogen-free environment. B16-F10 cells (2.5 × 105) were subcutaneously injected into the right flank of each mouse. The animals were weighed and randomised in groups of 3–4 per cage, thanks to the free software available at random.org. Mice present in each cage received the same treatment and the operator was blinded to the group of allocation. Tumors were measured with a calliper, and their volume calculated according to the following equation: volume = π/6 [w1 × (w2)2], were w1 = major diameter and w2 = minor diameter. A2AR antagonist SCH58261 (Tocris, bio-techne) or placebo (sterile PBS containing 0.005% DMSO) were intra-peritoneum injected every 3 days after first tumor mass detection (day five from inoculum). The volume of drug administered was based on body weight of each mouse. SCH58261 dose (1 mg/kg) and administration schedule were similar to those previously described in literature (Beavis et al., 2013; Young et al., 2016; Ma et al., 2017). Mice blood samples were collected from the submandibular vein and complemented with 1.5 mg/ml EDTA, immediately before sacrifice (post inoculum day 14). Tumors were post-mortem excised and processed for further analysis. Mice plasma was collected by centrifugation of blood (1,000 × g, 10 min at 4°C). Halt Protease and Phosphatase Inhibitor Cocktail (Thermo Scientific) was added to the samples before storage at −80°C. All animal procedures were approved by the Organism for the animal wellbeing (OPBA) of the University of Ferrara and the Italian Ministry of Health in compliance with EU Directive 2010/63/EU and Italian D.Lgs 26/2014.
Cytokines levels were evaluated within diluted plasma (1:2) either with mouse interleukin (IL)-1β, tumor necrosis factor (TNF)-α, transforming growth factor (TGF)-β1 (Boster, distributed by Tema Ricerca, Bologna, Italy), interferon (IFN)-γ (R&D, bio-techne) ELISA kits or with Luminex mouse multi-cytokine assay kit (R&D, bio-techne) as per manufacturer’s instructions. Tumor mass were homogenised in lysis buffer (300 μM sucrose, 1 mM K2HPO4, 1 mM MgSO4, 5.5 mM glucose, 20 mM HEPES (pH 7.4), HaltTM Protease and Phosphatase inhibitor cocktail, EDTA-free 100 × (Thermo Fisher), IGEPAL CA-630 0.5%) and loaded for VEGF and TGF-β1 analysis in a 1:5 ratio with mouse ELISA kits (Boster, distributed by Tema Ricerca, Bologna, Italy).
Tumor or organs were homogenised in lysis buffer (300 μM sucrose, 1 mM K2HPO4, 1 mM MgSO4, 5.5 mM glucose, 20 mM HEPES (pH 7.4), HaltTM Protease and Phosphatase inhibitor cocktail, EDTA-free 100 × (Thermo Fisher), IGEPAL CA-630 0.5%). The lysates were loaded in 4–12% NuPAGE Bis-Tris precast gels (Thermo Scientific). Following electrophoretic separation we perfomed transfer blotting on nitrocellulose membranes (Amersham Protran, GE Healthcare, United States); which were subsequently incubated overnight at 4°C with primary antibodies as follows: anti-P2X7 antibody (P8232, Sigma-Aldrich) was diluted 1:300 (1.3 μg/ml), anti-A2AR antibody (sc-32261 Santa Cruz Biotechnology, Heidelberg, Germany) was diluted 1:500 (0.4 μg/ml), anti-myosin II antibody (M8064 Sigma-Aldrich) was diluted 1:1000 (1.5 μg/ml), anti-GAPDH antibody (#6C5-sc32233, Santa Cruz Biotechnology) was diluted 1:1000 (0.1 μg/ml) and anti-β actin antibody (#E-ab-20031, Elabscience) was diluted 1:2500 (0.4 μg/ml). Goat anti-rabbit (170-6515, BIORAD) or goat anti-mouse (170-6516, BIORAD), HRP-conjugated antibodies were applied at a 1:3000 dilution (0.5 μg/ml) as secondary antibodies. Protein bands were visualised by ECL HRP Chemiluminescent Substrate WESTAR RC ULTRA 2.0 or WESTARN NOVA 2.0 (Cyanagen Srl, Bologna, Italy) with a Licor C-Digit Model 3600. Densitometric analysis was carried out with ImageJ software, and data were normalised on the appropriate housekeeping protein (myosin II, GAPDH or β actin).
Excised tumors were fixed in Bouin (Sigma-Aldrich) for 7 h at 4°C, dehydrated in cold-graded ethanol series, cleared in xylene, and embedded in paraffin. For immunohistochemistry of A2AR and CD31, serial 7-μm-thick sections were rehydrated, washed in TBS (150 mM NaCl, 50 mM Tris, pH 7.6), incubated for 1 h in blocking solution (TBS 8% FBS, 5% BSA 0.2% TRITON X-100) and subsequently for 16 h at 4°C in blocking solution containing primary antibodies (anti-A2AR mouse antibody, sc-32261 Santa Cruz Biotechnology, at 1:100 (2 μg/ml) and anti-CD31 rat monoclonal antibody, ER-MP12 Thermo Scientific, 1:20 (50 μg/ml)). For detection of A2AR and CD31, heat-induced epitope retrieval was performed using Rodent Decloaker (RD913M Biocare Medical). Slides were then washed twice in TBS plus 0.025% Triton X-100, and endogenous peroxidase activity was blocked by a 10-min incubation at room temperature in PeroxAbolish Solution (PXA969L Biocare Medical). Sections were then incubated for 1 h at room temperature in the blocking solution containing the diluted secondary antibodies [HRP-conjugated goat anti-mouse IgG, P0447, Dako, 1:100 (10 μg/ml), or rabbit anti-rat IgG, P0448, Dako 1:100 (2.5 μg/ml)]. Slides were washed twice in TBS, and peroxidase activity was detected with Liquid DAB Substrate Chromogen System (Dako). Nuclear counterstaining was obtained with Mayer hematoxylin. Following dehydration, sections were mounted with EUKITT (Kindler GmbH), and images acquired thanks to NIS-elements software with a Nikon Eclipse 90i digital microscope (Nikon Instruments, Europe). The percentage of positive cells was quantified using the QuPath free software for immunohistochemical analysis (Bankhead et al., 2017) as previously described (Tattersall et al., 2021).
All data are shown as mean ± standard error of the mean (SEM). Significance was calculated assuming equal standard deviations and variance with a two-tailed Student’s t-test, performed with the GraphPad Prism software V.6.0 (GraphPad, San Diego, Ca, United States). p-Values lower than 0.05 were considered statistically significant.
In recent years we and others have demonstrated that, in mice lacking the P2X7R, solid and liquid tumors show an increased growth due to reduced immune cell infiltration and lower eATP levels (Adinolfi et al., 2015; Hofman et al., 2015; De Marchi et al., 2019). However, an in-depth analysis of the systemic levels of several pro or anti-inflammatory cytokines in tumor-bearing P2X7R null mice has never been carried out. Figure 1 shows that, in parallel with an accelerated tumor growth (Figures 1A–C), P2X7R null mice also show a generalized reduction of systemic pro-inflammatory cytokines (Figures 1D–I) as well as an increase of the immune suppressant TGF-β (Figure 1J). Notably, blood concentration of IL-6 (Figure 1F), IL-12 (Figure 1G), IL-17 (Figure 1H), and IFN-γ (Figure 1I) was strikingly decreased (more than halved), suggesting a substantial impairment of anti-tumoral immune responses. On the contrary, blood TGF-β levels were almost triplicated (Figure 1J). Taken together, these data strongly suggest that the increased tumor progression observed in P2X7R null mice could be linked to a downmodulation of the tumor-eradicating immune response.
FIGURE 1. C57bl/6 mice were inoculated into the right flank with B16-F10 cells in wild type and P2X7-null mice. (A) Tumor volume was in vivo assessed at the indicated time points. (B) Ex-vivo tumor volume assessed by calliper. (C) Representative pictures of tumors from wild type and P2X7 null mice at post-inoculum day 14 (n = 6 per group). (D–J) Levels of plasma cytokines of tumor-bearing C57bl/6 mice inoculated with B16 cells. IL-1β (D), TNF-α (E), IL-6 (F), IL-12 (G), IL-17 (H), IFN-γ (I) and TGF-β (J) were evaluated in plasma samples obtained at post-inoculum day 14 (n = 4 per group). Data are shown as the mean ± SEM. *p < 0.05, **p < 0.01 and ***p < 0.001.
Tumor immune suppression, in analogy to that observed in the P2X7R null melanoma model, has been associated with the accumulation of eADO in the TME and the ensuing activation of the A2AR (Ohta et al., 2006; Ohta and Sitkovsky, 2014). Moreover, decreased eATP concentration accompanied by increased CD73 and CD39 expression strongly support eADO accumulation in the TME of P2X7R null mice. However, P2X7R and A2AR reciprocal crosstalk in the TME was never been investigated before. Therefore, we analyzed A2AR expression levels in tumors growing in either WT or P2X7 null mice observing a significant increase of tumor A2AR expression (Figure 2A). Interestingly, A2AR expression was also higher in the spleens, but not in the livers, from p2x7−/− mice whether in tumor-bearing or tumor free than WT mice (Figures 2B–E).
FIGURE 2. (A–E) Western Blot and relative quantification of A2AR in tumor (A), spleen (B,D) and liver (C,E) derived from wild type and P2X7 null tumor-bearing (A–C) or non-tumor-bearing (D,E) C57bl/6 mice (n = 3–13 per group). (F–H) Immunohistochemistry (F,G) and relative quantification (H) of A2AR in tumor derived from wild type (F) and P2X7 null (G) C57bl/6 mice (wild type n = 4, P2X7 null n = 8). Pictures are obtained with a 40X objective. Data are shown as the mean ± SEM.*p < 0.05 and **p < 0.01.
Immunohistochemical analysis confirmed overexpression of A2AR in tumors from P2X7R-null mice (Figures 2F–H), mainly on tumor cells (Figures 2F,G) and in the periphery of necrotic sites (Figures 3A,B). This observation leds us to investigate tumor levels of the angiogenetic factor VEGF. VEGF is upregulated in hypoxic regions associated with necrosis, and can be secreted in response to A2AR stimulation. Figure 3 shows a significantly higher intra-mass accumulation of VEGF in tumors growing in P2X7R-null mice than WT controls (Figure 3C). The high VEGF tumor levels were paralleled by an augmented expression of the endothelial marker CD31 (Figures 3D–F), indicating increased intratumor blood vessel formation.
FIGURE 3. (A,B) Immunohistochemistry of A2AR in tumors derived from wild type (A) and P2X7 null (B) C57bl/6 mice. Pictures are obtained with a 20X objective. (C) Intra-tumor levels of VEGF (wild type n = 5, P2X7 null n = 4). (D–F) Immunohistochemistry (D,E) and relative quantification (F) of CD31 in tumor derived from wild type and P2X7 null C57bl/6 mice (n = 4 per group). Data are shown as the mean ± SEM. *p < 0.05 and **p < 0.01.
To better understand the role played by A2AR in tumor progression in P2X7R-null mice, we administered the A2AR antagonist SCH58261 to p2x7−/− and WT tumor-bearing mice. This drug was administered by intraperitoneal injections at a 1 mg/kg dose every 3 days from the first appearance of the tumor mass. The A2AR antagonist reduced tumor growth in both mice strains (Figures 4A,B), thus demonstrating the central role of A2AR in the B16-F10 melanoma model. Interestingly, SCH58261 treatment did not affect the tumor levels of A2AR, which remained significantly higher in P2X7R-null versus WT mice (Figures 4C,D). On the other hand, the level of P2X7R expressed by tumor cells increased in P2X7 null mice following A2AR antagonism (Figures 4E,F), thus further supporting an interdependency of the two receptors in the TME. It has to be noted that B16-F10 cells injected in P2X7R-null mice still express P2X7R and are, therefore, responsible for the detection of the protein shown in Figures 4E,F. A2AR antagonism also reduced VEGF levels only in P2X7 devoid hosts (Figure 4G). On the other hand, SCH58261 treatment significantly reduced TGF-β levels in tumors growing in both mice strains, further emphasizing the leading role of A2AR in generating an immune-suppressed TME.
FIGURE 4. C57bl/6 mice were inoculated into the right flank with B16-F10 cells in wild type and P2X7 null mice. A2AR antagonist SCH58261 (1 mg/kg) or placebo (sterile PBS containing 0.005% DMSO) were intra-peritoneum injected every 3 days after first tumor mass detection (day 5 from inoculum). (A) Ex-vivo tumor volume assessed by calliper. (B) Representative pictures of tumors derived from treated wild type and P2X7 null mice at post inoculum day 14 (n = 7–11 per group). (C–F) A2AR (C) and P2X7R (E) expression by Western Blot and relative quantification (D,F) (n = 5–13 per group). VEGF (G) and TGF-β (H) were evaluated in tumor masses obtained at post inoculum day 14 (n = 3–6 per group). Data are shown as the mean ± SEM. *p < 0.05, **p < 0.01 and ***p < 0.001.
The TME is the privileged site of host-tumor interaction and, as such, a key determinant of cancer progression and metastatic spreading. Properties of the TME are also dictated by its features, such as hypoxia that can determine tumor vascularization causing the release of VEGF. Over the last few years, the abundance of eATP was unexpectedly identified as a prominent TME characteristic (Di Virgilio et al., 2018). eATP accumulates into the TME as a consequence of cell death or injury and of non-lytic release from tumor and host cells (Di Virgilio et al., 2018). In the TME, eATP ligates its specific cognate receptors (P2Y and P2X), and it is rapidly metabolised by plasma membrane-expressed or soluble ecto-nucleotidases. The semi-final degradation product of eATP is eADO (the final being inosine), a potent immunosuppressive molecule acting at A2AR (Sitkovsky et al., 2014; Vijayan et al., 2017; Boison and Yegutkin, 2019). Thus, eATP is central in the immunoregulation of the TME because, on the one hand, it is an immunostimulant mediator acting at P2Y and P2X receptors, and on the other hand it supports immunosuppression by generating eADO that activates A2AR. Converging evidence supports the view that the P2X7R is the P2 receptor most heavily involved in host-tumor interaction in the TME (Lara et al., 2020). eATP and the P2X7R are “partners in crime” in the TME as eATP ligates P2X7R and triggers P2X7R-mediated responses, among which release of ATP-itself, controlling the concentration of its own agonist (De Marchi et al., 2019). We recently demonstrated a substantial reduction in eATP levels in tumor-bearing mice devoid of P2X7R. This reduction was also accompanied by a significant increase of ectonucleotidases expression by tumor-infiltrating immune cells, in particular Tregs, thus suggesting an upregulation of eADO levels in the TME of mice lacking P2X7R (De Marchi et al., 2019).
In the current study, we deepened these observations focusing on another key actor of the adenosinergic pathway in cancer: the A2AR (Sitkovsky, 2020). Indeed, A2AR role in causing tumor immune suppression in hypoxic tumors is a consolidated notion that recently lead to several clinical trials exploring the anti-cancer therapeutic efficacy of the administration of A2AR antagonists as a stand-alone treatment or in combination with immune checkpoints inhibitors (Boison and Yegutkin, 2019; Fong et al., 2020; Franco et al., 2021; Augustin et al., 2022). Interestingly, in the TME A2AR activation has been reported to reduce the production of those tumor eradicating cytokines IL-6, IL-17, and IFN-γ that in the present study we found downmodulated in tumor-bearing p2x7−/− mice (Augustin et al., 2022). We also observed a reduction of other pro-inflammatory cytokines (IL-1β, TNF-α, and IL-12) related to P2X7R activation and, in general, to innate and type 1 immune response (Correa et al., 2017; Orioli et al., 2017). This immune, cancer-promoting scenario prompted us to further analyse A2AR levels in B16-F10 melanoma-bearing p2x7−/− and WT mice model. Our data show that in P2X7-null mice A2AR expression was increased in the tumor cells and in immunocompetent tissues such as the spleen. However, A2AR expression was also increased in the spleen of non-tumor-bearing P2X7-null mice, strongly suggesting that P2X7R deletion is a prime driver of A2AR upregulation in the immune compartment. In support of this hypothesis, no increased A2AR expression was found in liver and other non-immune tissues from p2x7−/− mice (not shown). Of interest, A2AR inhibition was able to increase P2X7R expression by B16-F10 cells, thus suggesting that the compensatory mechanism leading to upregulation of one of the two receptors when the other is downmodulated or blocked might be reciprocal.
A2AR is also known to promote neovascularisation and immune suppression via VEGF and TGF-β release (Steingold and Hatfield, 2020) both upregulated in melanoma-bearing p2x7−/− mice. Moreover, to further stress the pivotal function of the eADO signalling in the tumor hypoxic response, A2AR expression in the p2x7−/− mice was enhanced in peri-necrotic areas, together with an enhanced staining by the endothelial marker CD31. Of note, A2AR inhibition normalised intra-tumor VEGF levels only in P2X7-null mice, thus further supporting the hypothesis that the pro-angiogenic phenotype observed in this model was mainly dependent upon A2AR activity. Finally, A2AR blockade inhibited TGF-β release, thus further reducing immune suppression in the TME.
In conclusion, our data unveil a new A2AR-dependent compensatory mechanism emerging in the absence of host P2X7R. Although the underlying molecular mechanisms warrant further future investigation, the upregulated A2AR in P2X7-null mice promotes tumor growth by favoring immune suppression and neovascularization. Therefore, targeting both receptors has the potential to prove more efficacious than a stand-alone therapeutic strategy. Finally, based on previous studies, a combination of P2X7R and A2AR directed drugs could also prove beneficial in association with traditional chemotherapy (Pegoraro et al., 2020; Sitkovsky, 2020) or irradiation (Huang et al., 2020; Zanoni et al., 2022).
Complete raw data sets presented in this study will be made available upon reasonable request to the corresponding author.
The animal study was reviewed and approved by Organismo Preposto al Benessere Animale (OPBA, organism for animal wellbeing), University of Ferrara and Italian Ministry of Health.
EDM performed most of the experimental work. AP helped with in vivo and immunohistochemistry experiments. RT helped with Western blotting analysis. FDV, SM, EDM, and AP participated in experimental design and manuscript preparation. EA designed and supervised the study, participated in vivo experimentation, wrote and gave final approval to the manuscript.
This work was funded from the Italian Association for Cancer Research (AIRC) through grants IG16812 and IG22837 to EA and IG13025 and IG18581 to FDV.
FDV is a member of the Scientific Advisory Board of Biosceptre Ltd., a Biotech Company involved in the development of P2X7-targeted therapies.
The remaining authors declare that the research was conducted in the absence of any commercial or financial relationships that could be construed as a potential conflict of interest.
All claims expressed in this article are solely those of the authors and do not necessarily represent those of their affiliated organizations, or those of the publisher, the editors and the reviewers. Any product that may be evaluated in this article, or claim that may be made by its manufacturer, is not guaranteed or endorsed by the publisher.
The authors are grateful to Marzia Scarletti and Paola Chiozzi for technical support, and to Anna Scialdone and Letizia Alfieri for fruitful discussion.
Adinolfi, E., Raffaghello, L., Giuliani, A. L., Cavazzini, L., Capece, M., Chiozzi, P., et al. (2012). Expression of P2X7 Receptor Increases In VivoTumor Growth. Cancer Res. 72 (12), 2957–2969. doi:10.1158/0008-5472.CAN-11-1947
Adinolfi, E., Capece, M., Franceschini, A., Falzoni, S., Giuliani, A. L., Rotondo, A., et al. (2015). Accelerated Tumor Progression in Mice Lacking the ATP Receptor P2X7. Cancer Res. 75 (4), 635–644. doi:10.1158/0008-5472.CAN-14-1259
Adinolfi, E., Giuliani, A. L., De Marchi, E., Pegoraro, A., Orioli, E., and Di Virgilio, F. (2018). The P2X7 Receptor: A Main Player in Inflammation. Biochem. Pharmacol. 151, 234–244. doi:10.1016/j.bcp.2017.12.021
Adinolfi, E., De Marchi, E., Orioli, E., Pegoraro, A., and Di Virgilio, F. (2019). Role of the P2X7 Receptor in Tumor-Associated Inflammation. Curr. Opin. Pharmacol. 47, 59–64. doi:10.1016/j.coph.2019.02.012
Ahmad, S. F., Ansari, M. A., Nadeem, A., Bakheet, S. A., Mohammad, R., and Attia, S. M. (2018). Immune Alterations in CD8+ T Cells Are Associated with Neuronal C-C and C-X-C Chemokine Receptor Regulation through Adenosine A2A Receptor Signaling in a BTBR T+ Itpr3tf/J Autistic Mouse Model. Mol. Neurobiol. 55 (3), 2603–2616. doi:10.1007/s12035-017-0548-9
Allard, B., Cousineau, I., Allard, D., Buisseret, L., Pommey, S., Chrobak, P., et al. (2019). Adenosine A2A Receptor Promotes Lymphangiogenesis and Lymph Node Metastasis. Oncoimmunology 8 (8), 1601481. doi:10.1080/2162402X.2019.1601481
Allard, B., Allard, D., Buisseret, L., and Stagg, J. (2020). The Adenosine Pathway in Immuno-Oncology. Nat. Rev. Clin. Oncol. 17 (10), 611–629. doi:10.1038/s41571-020-0382-2
Amoroso, F., Capece, M., Rotondo, A., Cangelosi, D., Ferracin, M., Franceschini, A., et al. (2015). The P2X7 Receptor is a Key Modulator of the PI3K/GSK3β/VEGF Signaling Network: Evidence in Experimental Neuroblastoma. Oncogene 34 (41), 5240–5251. doi:10.1038/onc.2014.444
Ansari, M. A., Attia, S. M., Nadeem, A., Bakheet, S. A., Raish, M., Khan, T. H., et al. (2017). Activation of Adenosine A2A Receptor Signaling Regulates the Expression of Cytokines Associated with Immunologic Dysfunction in BTBR T+ Itpr3Tf/J Mice. Mol. Cell Neurosci. 82, 76–87. doi:10.1016/j.mcn.2017.04.012
Antonioli, L., Fornai, M., Pellegrini, C., D’Antongiovanni, V., Turiello, R., Morello, S., et al. (2021). Adenosine Signaling in the Tumor Microenvironment. Adv. Exp. Med. Biol. 1270, 145–167. doi:10.1007/978-3-030-47189-7_9
Arnaud-Sampaio, V. F., Rabelo, I. L. A., Ulrich, H., and Lameu, C. (2020). The P2X7 Receptor in the Maintenance of Cancer Stem Cells, Chemoresistance and Metastasis. Stem Cel Rev Rep 16 (2), 288–300. doi:10.1007/s12015-019-09936-w
Augustin, R. C., Leone, R. D., Naing, A., Fong, L., Bao, R., and Luke, J. J. (2022). Next Steps for Clinical Translation of Adenosine Pathway Inhibition in Cancer Immunotherapy. J. Immunother. Cancer 10 (2), e004089. doi:10.1136/jitc-2021-004089
Bankhead, P., Loughrey, M. B., Fernández, J. A., Dombrowski, Y., McArt, D. G., Dunne, P. D., et al. (2017). QuPath: Open Source Software for Digital Pathology Image Analysis. Sci. Rep. 7 (1), 16878. doi:10.1038/s41598-017-17204-5
Battastini, A. M. O., Figueiró, F., Leal, D. B. R., Doleski, P. H., and Schetinger, M. R. C. (2021). CD39 and CD73 as Promising Therapeutic Targets: What Could Be the Limitations? Front. Pharmacol. 12, 633603. doi:10.3389/fphar.2021.633603
Beavis, P. A., Divisekera, U., Paget, C., Chow, M. T., John, L. B., Devaud, C., et al. (2013). Blockade of A 2A Receptors Potently Suppresses the Metastasis of CD73+ Tumors. Proc. Natl. Acad. Sci. U.S.A. 110 (36), 14711–14716. doi:10.1073/pnas.1308209110
Boison, D., and Yegutkin, G. G. (2019). Adenosine Metabolism: Emerging Concepts for Cancer Therapy. Cancer Cell 36 (6), 582–596. doi:10.1016/j.ccell.2019.10.007
Borea, P. A., Gessi, S., Merighi, S., Vincenzi, F., and Varani, K. (2018). Pharmacology of Adenosine Receptors: The State of the Art. Physiol. Rev. 98 (3), 1591–1625. doi:10.1152/physrev.00049.2017
Correa, G., Almeida Lindenberg, C. d., Moreira-Souza, A. C. d. A., Savio, L. E. B., Takiya, C. M., Marques-da-Silva, C., et al. (2017). Inflammatory Early Events Associated to the Role of P2X7 Receptor in Acute Murine Toxoplasmosis. Immunobiology 222 (4), 676–683. doi:10.1016/j.imbio.2016.12.007
De Marchi, E., Orioli, E., Pegoraro, A., Sangaletti, S., Portararo, P., Curti, A., et al. (2019). The P2X7 Receptor Modulates Immune Cells Infiltration, Ectonucleotidases Expression and Extracellular ATP Levels in the Tumor Microenvironment. Oncogene 38, 3636–3650. doi:10.1038/s41388-019-0684-y
De Marchi, E., Orioli, E., Pegoraro, A., Adinolfi, E., and Di Virgilio, F. (2020). Detection of Extracellular ATP in the Tumor Microenvironment, Using the pmeLUC Biosensor. Methods Mol. Biol. 2041, 183–195. doi:10.1007/978-1-4939-9717-6_13
De Ponti, C., Carini, R., Alchera, E., Nitti, M. P., Locati, M., Albano, E., et al. (2007). Adenosine A2A Receptor-Mediated, Normoxic Induction of HIF-1 through PKC and PI-3k-dependent Pathways in Macrophages. J. Leukoc. Biol. 82 (2), 392–402. doi:10.1189/jlb.0107060
Di Virgilio, F., Falzoni, S., Giuliani, A. L., and Adinolfi, E. (2016). P2 Receptors in Cancer Progression and Metastatic Spreading. Curr. Opin. Pharmacol. 29, 17–25. doi:10.1016/j.coph.2016.05.001
Di Virgilio, F., Dal Ben, D., Sarti, A. C., Giuliani, A. L., and Falzoni, S. (2017). The P2X7 Receptor in Infection and Inflammation. Immunity 47 (1), 15–31. doi:10.1016/j.immuni.2017.06.020
Di Virgilio, F., Sarti, A. C., Falzoni, S., De Marchi, E., and Adinolfi, E. (2018). Extracellular ATP and P2 Purinergic Signalling in the Tumour Microenvironment. Nat. Rev. Cancer 18, 601–618. doi:10.1038/s41568-018-0037-0
Douguet, L., Janho Dit Hreich, S., Benzaquen, J., Seguin, L., Juhel, T., Dezitter, X., et al. (2021). A Small-Molecule P2RX7 Activator Promotes Anti-tumor Immune Responses and Sensitizes Lung Tumor to Immunotherapy. Nat. Commun. 12 (1), 653. doi:10.1038/s41467-021-20912-2
Drill, M., Jones, N. C., Hunn, M., O’Brien, T. J., and Monif, M. (2021). Antagonism of the ATP-Gated P2X7 Receptor: a Potential Therapeutic Strategy for Cancer. Purinergic Signal. 17 (2), 215–227. doi:10.1007/s11302-021-09776-9
Faul, F., Erdfelder, E., Lang, A.-G., and Buchner, A. (2007). G*Power 3: A Flexible Statistical Power Analysis Program for the Social, Behavioral, and Biomedical Sciences. Behav. Res. Methods 39 (2), 175–191. doi:10.3758/bf03193146
Fong, L., Hotson, A., Powderly, J. D., Sznol, M., Heist, R. S., Choueiri, T. K., et al. (2020). Adenosine 2A Receptor Blockade as an Immunotherapy for Treatment-Refractory Renal Cell Cancer. Cancer Discov. 10 (1), 40–53. doi:10.1158/2159-8290.CD-19-0980
Franco, R., Rivas-Santisteban, R., Navarro, G., and Reyes-Resina, I. (2021). Adenosine Receptor Antagonists to Combat Cancer and to Boost Anti-cancer Chemotherapy and Immunotherapy. Cells 10 (11), 2831. doi:10.3390/cells10112831
Hasko, G., and Pacher, P. (2008). A2A Receptors in Inflammation and Injury: Lessons Learned from Transgenic Animals. J. Leukoc. Biol. 83 (3), 447–455. doi:10.1189/jlb.0607359
Hofman, P., Cherfils-Vicini, J., Bazin, M., Ilie, M., Juhel, T., Hébuterne, X., et al. (2015). Genetic and Pharmacological Inactivation of the Purinergic P2RX7 Receptor Dampens Inflammation but Increases Tumor Incidence in a Mouse Model of Colitis-Associated Cancer. Cancer Res. 75 (5), 835–845. doi:10.1158/0008-5472.CAN-14-1778
Huang, J., Zhang, D., Bai, Y., Yang, P., Xing, L., and Yu, J. (2020). A2AR Antagonism with DZD2269 Augments Antitumor Efficacy of Irradiation in Murine Model. J. Cancer 11 (12), 3685–3692. doi:10.7150/jca.43966
Kamai, T., Kijima, T., Tsuzuki, T., Nukui, A., Abe, H., Arai, K., et al. (2021). Increased Expression of Adenosine 2A Receptors in Metastatic Renal Cell Carcinoma Is Associated with Poorer Response to Anti-vascular Endothelial Growth Factor Agents and Anti-PD-1/Anti-CTLA4 Antibodies and Shorter Survival. Cancer Immunol. Immunother. 70 (7), 2009–2021. doi:10.1007/s00262-020-02843-x
Kepp, O., Bezu, L., Yamazaki, T., Di Virgilio, F., Smyth, M. J., Kroemer, G., et al. (2021). ATP and Cancer Immunosurveillance. EMBO J. 40 (13), e108130. doi:10.15252/embj.2021108130
Koszalka, P., Gołuńska, M., Urban, A., Stasiłojć, G., Stanisławowski, M., Majewski, M., et al. (2016). Specific Activation of A3, A2A and A1 Adenosine Receptors in CD73-Knockout Mice Affects B16F10 Melanoma Growth, Neovascularization, Angiogenesis and Macrophage Infiltration. PLoS One 11 (3), e0151420. doi:10.1371/journal.pone.0151420
Lara, R., Adinolfi, E., Harwood, C. A., Philpott, M., Barden, J. A., Di Virgilio, F., et al. (2020). P2X7 in Cancer: From Molecular Mechanisms to Therapeutics. Front. Pharmacol. 11, 793. doi:10.3389/fphar.2020.00793
Leone, R. D., and Emens, L. A. (2018). Targeting Adenosine for Cancer Immunotherapy. J. Immunotherapy Cancer 6 (1), 57. doi:10.1186/s40425-018-0360-8
Li, X.-Y., Moesta, A. K., Xiao, C., Nakamura, K., Casey, M., Zhang, H., et al. (2019). Targeting CD39 in Cancer Reveals an Extracellular ATP- and Inflammasome-Driven Tumor Immunity. Cancer Discov. 9 (12), 1754–1773. doi:10.1158/2159-8290.CD-19-0541
Ma, S.-R., Deng, W.-W., Liu, J.-F., Mao, L., Yu, G.-T., Bu, L.-L., et al. (2017). Blockade of Adenosine A2A Receptor Enhances CD8+ T Cells Response and Decreases Regulatory T Cells in Head and Neck Squamous Cell Carcinoma. Mol. Cancer 16 (1), 99. doi:10.1186/s12943-017-0665-0
Nemeth, Z. H., Leibovich, S. J., Deitch, E. A., Sperlágh, B., Virág, L., Vizi, E. S., et al. (2003). Adenosine Stimulates CREB Activation in Macrophages via a P38 MAPK-Mediated Mechanism. Biochem. Biophys. Res. Commun. 312 (4), 883–888. doi:10.1016/j.bbrc.2003.11.006
Ohta, A., and Sitkovsky, M. (2014). Extracellular Adenosine-Mediated Modulation of Regulatory T Cells. Front. Immunol. 5, 304. doi:10.3389/fimmu.2014.00304
Ohta, A., Gorelik, E., Prasad, S. J., Ronchese, F., Lukashev, D., Wong, M. K. K., et al. (2006). A2A Adenosine Receptor Protects Tumors from Antitumor T Cells. Proc. Natl. Acad. Sci. U.S.A. 103 (35), 13132–13137. doi:10.1073/pnas.0605251103
Orioli, E., De Marchi, E., Giuliani, A. L., and Adinolfi, E. (2017). P2X7 Receptor Orchestrates Multiple Signalling Pathways Triggering Inflammation, Autophagy and Metabolic/Trophic Responses. Curr. Med. Chem. 24 (21), 2261–2275. doi:10.2174/0929867324666170303161659
Pegoraro, A., Orioli, E., De Marchi, E., Salvestrini, V., Milani, A., Di Virgilio, F., et al. (2020). Differential Sensitivity of Acute Myeloid Leukemia Cells to Daunorubicin Depends on P2X7A versus P2X7B Receptor Expression. Cel Death Dis. 11 (10), 876. doi:10.1038/s41419-020-03058-9
Pegoraro, A., De Marchi, E., Ferracin, M., Orioli, E., Zanoni, M., Bassi, C., et al. (2021). P2X7 Promotes Metastatic Spreading and Triggers Release of miRNA-Containing Exosomes and Microvesicles from Melanoma Cells. Cell Death Dis. 12 (12), 1088. doi:10.1038/s41419-021-04378-0
Pelegrin, P. (2021). P2X7 Receptor and the NLRP3 Inflammasome: Partners in Crime. Biochem. Pharmacol. 187, 114385. doi:10.1016/j.bcp.2020.114385
Scarpellino, G., Genova, T., and Munaron, L. (2019). Purinergic P2X7 Receptor: A Cation Channel Sensitive to Tumor Microenvironment. Recent Pat Anticancer Drug Discov. 14 (1), 32–38. doi:10.2174/1574892814666190116122256
Sitkovsky, M. V., Hatfield, S., Abbott, R., Belikoff, B., Lukashev, D., and Ohta, A. (2014). Hostile, Hypoxia-A2-Adenosinergic Tumor Biology as the Next Barrier to Overcome for Tumor Immunologists. Cancer Immunol. Res. 2 (7), 598–605. doi:10.1158/2326-6066.CIR-14-0075
Sitkovsky, M. V. (2020). Lessons from the A2A Adenosine Receptor Antagonist-Enabled Tumor Regression and Survival in Patients with Treatment-Refractory Renal Cell Cancer. Cancer Discov. 10 (1), 16–19. doi:10.1158/2159-8290.CD-19-1280
Sorrentino, R., Pinto, A., and Morello, S. (2013). The Adenosinergic System in Cancer. Oncoimmunology 2 (1), e22448. doi:10.4161/onci.22448
Steingold, J. M., and Hatfield, S. M. (2020). Targeting Hypoxia-A2A Adenosinergic Immunosuppression of Antitumor T Cells during Cancer Immunotherapy. Front. Immunol. 11, 570041. doi:10.3389/fimmu.2020.570041
Tattersall, L., Shah, K. M., Lath, D. L., Singh, A., Down, J. M., De Marchi, E., et al. (2021). The P2RX7B Splice Variant Modulates Osteosarcoma Cell Behaviour and Metastatic Properties. J. Bone Oncol. 31, 100398. doi:10.1016/j.jbo.2021.100398
Ulrich, H., Ratajczak, M. Z., Schneider, G., Adinolfi, E., Orioli, E., Ferrazoli, E. G., et al. (2018). Kinin and Purine Signaling Contributes to Neuroblastoma Metastasis. Front. Pharmacol. 9, 500. doi:10.3389/fphar.2018.00500
Vijayan, D., Young, A., Teng, M. W. L., and Smyth, M. J. (2017). Erratum: Targeting Immunosuppressive Adenosine in Cancer. Nat. Rev. Cancer 17 (12), 765. doi:10.1038/nrc.2017.110
Yan, J., Li, X.-Y., Roman Aguilera, A., Xiao, C., Jacoberger-Foissac, C., Nowlan, B., et al. (2020). Control of Metastases via Myeloid CD39 and NK Cell Effector Function. Cancer Immunol. Res. 8 (3), 356–367. doi:10.1158/2326-6066.CIR-19-0749
Yegutkin, G. G. (2008). Nucleotide- and Nucleoside-Converting Ectoenzymes: Important Modulators of Purinergic Signalling cascade. Biochim. Biophys. Acta Mol. Cel Res. 1783 (5), 673–694. doi:10.1016/j.bbamcr.2008.01.024
Young, A., Ngiow, S. F., Barkauskas, D. S., Sult, E., Hay, C., Blake, S. J., et al. (2016). Co-inhibition of CD73 and A2AR Adenosine Signaling Improves Anti-tumor Immune Responses. Cancer Cell 30 (3), 391–403. doi:10.1016/j.ccell.2016.06.025
Young, A., Ngiow, S. F., Gao, Y., Patch, A.-M., Barkauskas, D. S., Messaoudene, M., et al. (2018). A2AR Adenosine Signaling Suppresses Natural Killer Cell Maturation in the Tumor Microenvironment. Cancer Res. 78 (4), 1003–1016. doi:10.1158/0008-5472.CAN-17-2826
Keywords: P2X7, A2AR, ATP, cancer, immunosuppression, VEGF
Citation: De Marchi E, Pegoraro A, Turiello R, Di Virgilio F, Morello S and Adinolfi E (2022) A2A Receptor Contributes to Tumor Progression in P2X7 Null Mice. Front. Cell Dev. Biol. 10:876510. doi: 10.3389/fcell.2022.876510
Received: 15 February 2022; Accepted: 05 April 2022;
Published: 18 May 2022.
Edited by:
Shyamala Maheswaran, Massachusetts General Hospital and Harvard Medical School, United StatesReviewed by:
Sheikh Fayaz Ahmad, King Saud University, Saudi ArabiaCopyright © 2022 De Marchi, Pegoraro, Turiello, Di Virgilio, Morello and Adinolfi. This is an open-access article distributed under the terms of the Creative Commons Attribution License (CC BY). The use, distribution or reproduction in other forums is permitted, provided the original author(s) and the copyright owner(s) are credited and that the original publication in this journal is cited, in accordance with accepted academic practice. No use, distribution or reproduction is permitted which does not comply with these terms.
*Correspondence: Elena Adinolfi, ZWxlbmEuYWRpbm9sZmlAdW5pZmUuaXQ=
Disclaimer: All claims expressed in this article are solely those of the authors and do not necessarily represent those of their affiliated organizations, or those of the publisher, the editors and the reviewers. Any product that may be evaluated in this article or claim that may be made by its manufacturer is not guaranteed or endorsed by the publisher.
Research integrity at Frontiers
Learn more about the work of our research integrity team to safeguard the quality of each article we publish.