Erratum: A Current Overview of the Biological Effects of Combined Space Environmental Factors in Mammals
- 1State Key Laboratory of Radiation Medicine and Protection, Soochow University, Suzhou, China
- 2School of Radiation Medicine and Protection, Suzhou Medical College of Soochow University, Suzhou, China
- 3Collaborative Innovation Center of Radiological Medicine of Jiangsu Higher Education Institutions, Suzhou, China
Distinct from Earth’s environment, space environmental factors mainly include space radiation, microgravity, hypomagnetic field, and disrupted light/dark cycles that cause physiological changes in astronauts. Numerous studies have demonstrated that space environmental factors can lead to muscle atrophy, bone loss, carcinogenesis, immune disorders, vascular function and cognitive impairment. Most current ground-based studies focused on single environmental factor biological effects. To promote manned space exploration, a better understanding of the biological effects of the spaceflight environment is necessary. This paper summarizes the latest research progress of the combined biological effects of double or multiple space environmental factors on mammalian cells, and discusses their possible molecular mechanisms, with the hope of providing a scientific theoretical basis to develop appropriate countermeasures for astronauts.
Introduction
So far, more than 500 astronauts have been sent into space to conduct space exploration missions, who are inevitably faced with a harsh space environment when conducting research activities. Space environmental factors include microgravity, hypomagnetic field, circadian rhythm different from the earth’s environment, space ultraviolet, ionizing radiation, high vacuum, extreme temperature and noise (Thirsk et al., 2009; Horneck et al., 2010). After more than 50 years of space explorations, a large number of studies have proved that the space environment has adverse effects on organisms. Astronauts carrying out space exploration missions may present changes in gene regulation and genomic integrity, as well as molecular and cellular biological changes such as mitochondrial dysfunction. These changes will increase health risks, including muscle atrophy and bone loss, carcinogenesis, immune disorders, changes in vascular function, and cognitive impairment (Williams et al., 2009; Garrett-Bakelman et al., 2019).
At present, studies on single factor biological effects of the space environment mainly focus on space radiation, microgravity, hypomagnetic field and circadian rhythm. There are three main sources of space ionizing radiation: galactic cosmic rays (GCR) containing high-energy protons and heavy ions, solar particle events (SPE) containing medium and high-energy protons, and trapped radiation belt (TRB) dominated by 10 MeV protons and electrons (Simonsen et al., 2020). International Space Station (ISS) orbits the Earth at an altitude of about 350 km, with radiation levels of 0.4–1.0 mSv/days, which is about 100 times the level of radiation on Earth’s surface; for missions beyond low Earth orbit, such as lunar and Mars exploration, astronauts will be more affected by GCR (Yatagai and Ishioka, 2014). In addition, primary space radiation from the universe reacts with the spacecraft bulkhead to generate secondary rays such as neutrons, X-rays and γ-rays, which can produce reactive oxygen species (ROS) more effectively than primary space radiation (Inozemtsev et al., 2018). The main effects of space radiation on organisms are genotoxic effects (such as single-strand and double-strand DNA breaks, chromatin structure destruction, base tautomerism), and other effects including oxidative stress, immune disorders and central nervous system damage (Yatagai and Ishioka, 2014; Kokhan et al., 2019a). Microgravity is another important space environmental factor and is about 10−4–10−6 g in the interplanetary space, which is a state of physical gravity reduction. The change of gravity will affect cell proliferation, differentiation, signal transduction, gene expression and membrane rearrangement (Wuest et al., 2018; Yatagai et al., 2019), resulting in physiological changes at the tissue or organ level during space flight. The effects of microgravity on organisms mainly include changes in gene expression, chromosome aberration, apoptosis, immunosuppression, cardiovascular disease, skeletal muscle atrophy and bone loss (Girardi et al., 2012; Lin et al., 2020; Neelam et al., 2020). The commonly used equipment and models for ground simulation of microgravity include rotating wall vessels (RWV), 2D and 3D clinostat, random positioning machine (RPM), hindlimb unloading (HLU) model, anti-magnetic levitation model and parabolic flying aircraft (Herranz et al., 2012; Herranz et al., 2013; Ghosh et al., 2016; Stervbo et al., 2018; Paul et al., 2021). For humans, the head-down tilt bed rest model is usually used to study the effect of microgravity on bone, muscle, cardiovascular system (Liang et al., 2014; Moreno-Villanueva et al., 2017). There is limited research on the biological effects of the space environments’ hypomagnetic field combined with light/dark cycle dysregulations. The strength of the geomagnetic field (GMF) is about 50 μT, while the intensity of the magnetic field in outer space is very low, called hypomagnetic field (HyMF/HMF, |B| < 5 μT) (Mo et al., 2014; Yang et al., 2018). Mars magnetic field (< 5 μT), lunar magnetic field (< 300 nT) and interstellar space magnetic field (several nT) all belong to hypomagnetic field. Astronauts are inevitably exposed to HMF conditions when performing long-cycle and long-distance space missions. HMF can interfere with a variety of functions of organisms, including gene expression, individual growth, embryonic development, learning and working abilities (Zhang et al., 2021a; Zhang et al., 2021b). The orbit of a spacecraft in low orbit around the earth is about 90 min (Dijk et al., 2001; Thirsk et al., 2009). It means that 16 days and night cycles in 24 h. Such a light-dark cycle pattern is inconsistent with the rhythm of human life on the earth. This seriously affects the biological clock of astronauts, resulting in a decline in sleep quality, appetite, learning and memory capacity (Taillard et al., 2021; von Gall, 2022). During missions to Mars, the light-dark cycle is variable rather than fixed outside the spacecraft and astronauts will be exposed to artificial light rather than the natural light on earth for a long time in the spacecraft. High-fidelity ground simulation studies of Mars missions showed that light exposure patterns during missions to Mars led to melatonin cycle disorder, autonomic nervous system parasympathetic dysfunction, vigilance deficits, shorter total sleep time and lower sleep efficiency (Basner et al., 2013; Vigo et al., 2013; Gemignani et al., 2014). Considering the limited experimental opportunities on spacecrafts or space stations, a number of ground-based studies have been carried out on the effects of single space environmental factor on organisms in order to protect the life and health of astronauts, but the research on the combined biological effects of double or multiple environmental factors is relatively limited. In this paper, the latest research will be reviewed.
Combined Biological Effects of Space Environmental Factors in Mammals
Space Radiation and Microgravity
Research of the biological effects of space environmental factors usually takes space radiation and microgravity as characteristic factors. Many studies have discussed the combined effects of space radiation and microgravity in mammals of the two factors to explain how space environment can cause the physiological changes in astronauts, including the effects on bones, nervous system, circulatory system and reproductive system, as demonstrated in Table 1. The negative effects of microgravity on bone are obvious, and most of the studies about microgravity and radiation also focus on their combined effect on bone formation and functions. It was found that high LET iron ion radiation (0.5 or 2 Gy) aggravated the decrease of bone strength caused by lumbar decommissioning in a HLU model of 4-month-old male C57BL/6J mice, and partially prevented the recovery of cancellous bone microstructure caused by bone unloading, resulting in the loss of bone integrity (Alwood et al., 2010). Proton irradiation (1 Gy) combined with HLU was also reported to reduce the bone strength and mechanical properties of the femur and tibia in 15-week-old female C57BL/6 mice (Lloyd et al., 2012). Low dose X-rays (25 mGy) combined with HLU could reduce the trabecular mass of 10-week-old male C57BL/6J mice, but there was no significant difference compared with the experimental group treated with HLU alone, while the decrease of bone surface area and femoral cortical thickness only appeared in the HLU combined radiation group, and it was found that the loss of cortical bone and the decrease of trabecular mass were caused by the absorption of osteoclasts, which are multinucleate giant cells formed by the fusion of mononuclear macrophages (Farley et al., 2020). The effect of high LET radiation combined with partial weightlessness on bones was also studied. Female BALB/cByJ 4-month-old mice were exposed to low dose and high LET silicon ion radiation (0.17 Gy single radiation, 0.5 Gy single radiation and fractionated radiation) under partial weightlessness (G/6 to simulate lunar gravity). It was noted that the combination of the two factors had a negative effect on the maintenance of bone mass by both reducing bone formation and increasing bone resorption, and the impaired bone formation response was related to the inhibited Wnt signaling (Macias et al., 2016), the increased Runx2 and decreased Caspase-3 mRNA expression (Dong et al., 2021). On the whole, most studies showed that microgravity-induced bone loss and impaired bone mechanical properties were aggravated by ionizing radiation exposure.
The combined effect of microgravity and radiation also has an effect on the nervous system, usually in an antagonistic manner. In the striatum, the content of 5-hydroxytryptamine (5-HT) metabolite 5-hydroxyindoleacetic acid (5-HIAA) increased under the action of HLU simulated microgravity alone, and γ-ray irradiation (3 Gy) combined with microgravity could normalize the content of 5-HIAA (Shtemberg et al., 2014). In the prefrontal cortex (PFC) and amygdala of Long-Evans rats, the combined effects of radiation (whole body γ-irradiation of 3 Gy and carbon-ion head irradiation of 1.5 Gy) and microgravity (12 days) led to their antagonistic effects on 5-HT neurotransmitters. Moreover, irradiation was dominant to reduce 5-HT metabolism (Kokhan et al., 2019a). Neural readaptation occurred in 6-month-old female C57BL/6J mice treated with low dose and low dose rate γ-rays (0.04 Gy, 0.01 cGy/h) and HLU for 21 days, which is a dynamic process involving pathways regulating neuronal function and structure and leads to delayed neurological sequelae (Overbey et al., 2019). γ-ray irradiation (4.5 Gy) can antagonize the stress response of the hypothalamus-pituitary-adrenocortical (HPA) axis and macrophages induced by microgravity in male Wistar rats, and aggravate T cell dysfunction, resulting in HPA axis and immune dysfunction (Zhu et al., 2020). Simulated microgravity and ionizing radiation (γ-ray whole-body exposure of 3 Gy and proton head exposure of 1.5 Gy) also antagonized the psychological and emotional state and cognitive ability of 6-week-old male Wistar rats, which was found to be related to the changes of monoamine content in PFC, hippocampus and hypothalamus (Kokhan et al., 2017). Further research showed that combined microgravity and radiation affected the conversion rates of 5-HT and dopamine (DA) in PFC, hippocampus and striatum of 3-month-old male Wistar rats. This reduced the adverse effects induced by microgravity and radiation alone, such as the recovery of the impaired motor activity and long-term contextual memory, and the suppressed orientation and exploratory behavior. It is speculated that 5-HT receptor 5-HT2a in PFC and DA receptor D2 in hippocampus are involved (Kokhan et al., 2019b). In brains of 6-month-old female C57BL/6J mice treated with HLU model (21 days) combined with low dose rate radiation (122 keV/μm, γ-rays of 40 mGy), it was found that the level of specific oxidation marker 4-HNE protein in nerve increased and the level of superoxide dismutase (SOD) decreased. It is estimated that the increase of neuronal damage caused by long-term exposure to simulated microgravity and low dose rate radiation may be related to the increase of oxidative pressure and the decrease of antioxidant defense ability (Mao et al., 2016). In addition, it was also discovered that oxidative stress in brains of 6-month-old female B6.129S6-CYBBM mice knocked out of NADPH oxidase Nox2 gene was inhibited by 0.04 Gy γ-rays and HLU simulated microgravity. It is speculated that Nox2 may be involved in space environment-induced oxidative stress (Mao et al., 2017).
Circulatory and immune systems were also found to be affected by the combined effect of microgravity and radiation. 6-month-old female C57BL/6J mice after continuous low-dose γ-irradiation (a total dose of 0.04 Gy) and HLU (21 days) treatment showed persistent low-grade inflammation and decreased red blood cell quality, which is suggestive of anemia (Paul et al., 2021). When 8- to 12-week-old male C57BL/6 mice were simultaneously exposed to chronic γ-radiation (a total dose of 0.5 Gy) and HLU simulated microgravity for a month, the proportion of immune cells in their thymus altered, but not in the spleen (Sadhukhan et al., 2021). Compared with the action of HLU alone, proton radiation of 2 Gy combined with HLU could significantly reduce the number of spleen T lymphocytes and cytotoxic T lymphocytes in 6–8-week-old female ICR mice, and the activation of T lymphocytes was inhibited and their proliferation was significantly decreased (Sanzari et al., 2013). The level of endothelial nitric oxide synthase (eNOS) in the retina of 6-month-old male C57BL/6J mice increased significantly after 50 cGy proton radiation combined with HLU treatment, the B cell count in retinal vessels decreased significantly, and the NK cell count increased (Mao et al., 2019). Compared with HLU (15 days) and γ-irradiation of 200 cGy (84 cGy/min) alone, the combined effect of them did not further reduce the endothelium-dependent vasodilation function of gastrocnemius artery in 16-week-old C57BL/6J male mice, but increased the mRNA expression of eNOS and decreased the vasodilation mediated by endothelium-derived relaxing factor NO, suggesting that the NOS signal pathway may be uncoupled. The mechanism of vasodilation has shifted from a mechanism that mainly depends on NO to a mechanism that may be more dependent on PGI2 signals. It is also concluded that the damage of endothelium-dependent vasodilation mediated by microgravity and radiation is closely related to the changes of trabecular volume and microstructure. It speculates that NO couples vascular signals to bone remodeling (Prisby et al., 2016). There are also studies on the effects of high LET radiation combined with microgravity on vasodilation. The researchers treated 16-week-old male C57BL/6 mice with iron ion beam irradiation of 1 Gy (10 cGy/min) and HLU (13–16 days). It was found that the combined effect could further damage the endothelium-dependent vasodilation function of gastrocnemius artery through NOS signal pathway, and had a negative effect on eNOS, xanthine oxidase XO and SOD-1 protein levels. In addition, it was also found that the decrease of endothelium-dependent vasodilation was associated with lower cancellous bone volume fraction (Ghosh et al., 2016). 21 days after 6-month-old female C57BL/6J mice were exposed to HLU and γ-rays (0.01 cGy/h, 0.04 Gy), GSH:GSSG ratio, SAM:SAH ratio and inflammation marker CD-2 protein content decreased. These results suggest that exposure to low dose rate and low dose radiation combined with microgravity can reduce the potential of DNA methylation in heart tissue (Seawright et al., 2017).
Similar findings were observed in another study about the combined effect of microgravity and radiation on the reproductive system. HLU combined with 0.25–2 Gy carbon-ion radiation was utilized to treat 10-week-old male Swiss Webster mice. It was found that the combined action of HLU and carbon ion radiation promoted the expression of p53, Bax and PCNA in spermatogenic cells and increased cell apoptosis, while reduced sperm count and survival rate and aggravated sperm DNA damage in mice. It is speculated that one of the reasons for the decline of male fertility in space environment is sperm DNA damage (Li et al., 2013). Compared with normal gravity, the number of spermatozoa, primary spermatocytes and spermatogonia of male Kunming mice reduced significantly under microgravity simulated by HLU and carbon ion irradiation (0.4–1 Gy). Radiation at the dose of 0.8 Gy or above combined with microgravity can significantly up-regulate the ratio of Bax/Bcl-xL. It is assumed that the aggravation of germ cell injury is caused by the increase of apoptosis caused by mitochondrial apoptosis pathway (Liu et al., 2013). These results suggest that the combined effects of microgravity and radiation have obvious adverse effects on the male reproductive system, usually in a synergistic manner, while there are few studies on the female reproductive system.
The research of microgravity combined radiation is increasing gradually, and some effects as well as the underlying mechanisms were demonstrated. However, the research of the combined effect is still in the preliminary stage, and the mechanism underlying most phenomena is still lacking. There are also shortcomings in the field. Space radiation environment is dominated by GCR particles at a relatively low dose rate (about 1.84 mSv/day) during manned interplanetary flights (Zeitlin et al., 2013). However, most of radiation types used in current research are photons delivered as acute irradiation, while there are few studies employing protons and heavy ions that are delivered as chronic irradiation. Many studies have shown that acute and chronic exposures cause different biological effects. For example, acute and chronic radiation exposure have dissimilar effects on CNS, which in turn affects the behavioral and cognitive performance on mice (Holden et al., 2021), as well as the phenotype of immune cells and genomic response (Kovalchuk et al., 2004; Chaudhry et al., 2012; Sadhukhan et al., 2021). Therefore, the dose rate of radiation should be taken into account in the study of the combined effects of radiation. Due to analog limitations of studying spaceflight environmental factors on Earth, space radiation co-exists with the microgravity during space exploration mission duration. Current ground-based studies mostly treat the samples by ionizing radiation and simulated microgravity in a sequential manner, which is quite different from the real space environment and the yielded results are limited in explaining the biological effects observed in space. Thus, further research into high fidelity modeling schemes for spaceflight is still required.
Hypomagnetic Field and Microgravity
The magnetic and gravitational fields are a natural part of a habitable environment on Earth, and both inevitably change in space. Numerous studies have shown that microgravity can cause bone loss and muscle atrophy, yet, the combined effect between hypomagnetic field and microgravity are limited (Table 2). Studies have shown that HMF (< 300 nT) can aggravate bone loss and change the biomechanical properties of the femur in HLU male Sprague-Dawley (SD) rats. At the same time, an obvious expression of RANKL (Receptor Activator of Nuclear Factor Kappa B Ligand) and increase of serum iron concentration were observed in the trabeculae of the femur. Elevated expression of RANKL is the main cause of bone loss (Jia et al., 2014). It was also found in HLU 8-week-old male C57BL/6 mice that HMF (<300 nT) promoted bone loss of femur and had a negative effect on the biomechanical properties of the femur, and the accumulation of iron in serum, liver, spleen and bone of mice was significantly higher than that of microgravity group and HMF group (Yang et al., 2018). It is speculated that iron overload causes oxidative stress and promotes bone loss. Compared with HLU combined with GMF and reloading for a period of time, the bone mineral content of femur and tibia of 6-week-old male C57BL/6 mice reloaded with HLU combined with HMF (B < 300 nT) was lower, the microstructure and mechanical properties of femur were worse, the unbalanced bone remodeling of the tibia was less ideal, and the content of iron in serum, tibia, liver and spleen was higher. The iron content in bone, liver and spleen of mice treated with iron chelated agent deferoxamine (DFO) decreased. DFO can significantly reduce the bone loss induced by microgravity in the HMF reload group, implying that HMF inhibits the recovery of bone loss induced by microgravity. It may be due to the increase of ferritin Hepcidin expression and the decrease of membrane transporter FPN expression, which causes iron accumulation to return to physiological level (Xue et al., 2020b).
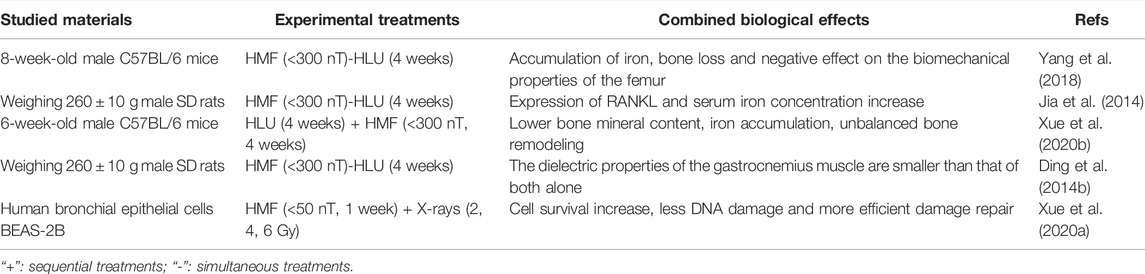
TABLE 2. Studies reporting combined biological effects of hypomagnetic field and microgravity in mammals.
In addition, a small amount of the research is dedicated to the combined effect of microgravity and hypomagnetic field on the dielectric properties of tissue, as demonstrated in Table 2. There was no significant change in the dielectric properties of the gastrocnemius muscle of male SD rats treated with HLU for 4 weeks, but the electrical conductivity of gastrocnemius muscle was increased when the intensity of HMF was lower than that of 300 nT alone. At the same time, it is found that the combined effect of HLU and HMF on the dielectric properties of the gastrocnemius muscle is smaller than that of both alone, rather than a simple additive relationship (Ding et al., 2014b). HLU and HMF alone or in combination have different effects on the dielectric properties of the spleen, whole blood and testis of male SD rats, and the degree of change varies with different tissues (Ding et al., 2014a). These experiments suggest that the dielectric properties of biological tissues in the space environment are different from those in normal conditions, which are worthy of further study, so as to expand the application of biological physiology and pathology detection and monitoring in the space environment.
The above experiments suggest that HMF can exacerbate microgravity-induced bone loss and this process may be caused by iron accumulation. In addition, HMF combined with microgravity can affect the dielectric properties of tissues and organs. These studies provide a basis for the role of GMF and HMF in the recovery of bone loss and muscle atrophy induced by microgravity. Beyond these, there is little research on other systems. HMF can affect the function of multiple systems in an organism, especially in the central nervous system (Zhang et al., 2021b). Similarly, microgravity can affect the central nervous system while the combined effects of HMF and microgravity is yet to be elucidated.
Multiple Space Environmental Factors
To simulate the space environment as accurately as possible, some researchers have carried out studies on the combined biological effects greater than two environmental factors in mammals. They used circadian rhythm changes (45 min bright: 45 min dark) combined with HLU and 4 Gy X-ray irradiation to study the combined effects on the hindlimb bones of rats. It was found that the combination of the three factors could significantly reduce bone biomechanical properties, bone mineral density and bone trabecular parameters, resulting in bone loss. However, there was no significant difference between the HLU group and the combined three-factor group. The results showed that the change of circadian rhythm had no significant effect on bone parameters (Zhang et al., 2018). Other researchers placed SD rats in a new type of cage that could simulate circadian rhythm disorder (12 h bright: 12 h dark), microgravity (HLU model), noise (65 dB) and claustrophobic environment. It was found that the rats had depressive behavior, with upregulated expression of splice-related proteins, but downregulated expression of oxidative phosphorylation, glutamatergic and GABA-ergic synaptic related proteins and post-synaptic density protein 95 (PSD-95) proteins (Min et al., 2021). It is speculated that these differentially expressed proteins may be markers of mental disorders. However, the health effects caused by the combination of circadian rhythm changes and other space environmental factors have not been reported.
Combined Biological Effects of Space Environmental Factors in Human Cells
Space Radiation and Microgravity
According to the classic Target Theory, nuclear DNA damage induced by space radiation plays a key role in the cell fate determination in cells exposed to space radiation. Whether microgravity affects radiation-induced DNA damage and subsequent cell fate depends on the cell type and growth condition (Lu et al., 2017). For example, the interaction between these two environmental factors inducing chromosome aberration (CA) has been controversial. Microgravity simulated by RWV had no effect on the CA of human lymphocytes induced by X-rays or proton beams (0–6 Gy) (Manti et al., 2005). However, the CA of human fibroblasts or human lymphoblastic TK6 cells exposed to radiation (1.5 Gy X-rays or 0.5 Gy carbon-ion beams) and clinostat simulated microgravity (24 h) increased (Hada et al., 2019; Yamanouchi et al., 2020), and the CA of human lymphocytes exposed to X-rays (1.5 Gy) and clinostat simulated microgravity also increased (Mosesso et al., 2001). In addition, the combined effect of microgravity and space radiation on DNA damage repair is also controversial. Under simulated microgravity, the efficiency of DNA damage repair of human lymphocytes exposed to 5 Gy γ-rays decreased (Mognato et al., 2009). However, when human fibroblasts irradiated by 5 Gy or 10 Gy X-rays were carried into space by the space shuttle Columbia carrying out the STS-65 mission, there is no significant difference between the DNA damage repair efficiency of cells under real space microgravity and ground gravity (Horneck et al., 1996). In another study employing human lymphoblastoid TK6 cells, it was found that microgravity simulated by RWV reduced the level of γ-ray irradiation-induced apoptosis while enhanced genomic damage as manifested by HPRT mutant frequency and the micronucleus formation (Canova et al., 2005). However, Dang et al. found that microgravity simulated by RWV aggravated carbon ion radiation-induced cell apoptosis mediated by a ROS-sensitive signaling pathway in human B lymphoblast HMy2.CIR cells (Dang et al., 2014). As demonstrated in Table 3, it can be concluded that microgravity may present synergistic, additive or antagonistic effect on DNA and cellular damage induced by ionizing radiation.
Several studies have also tried to clarify the underlying molecular mechanisms of cellular damage caused by microgravity combined with radiation. Human peripheral blood lymphocytes (PBL) were incubated in microgravity simulated by RWV after exposure to X-rays or γ-rays (1 or 2 Gy). Compared with irradiated PBL incubated in normal gravity, the mutation frequency of HPRT in microgravity increased, but the transcription of DNA repair-related genes did not change significantly, indicating that the increased mutation frequency had nothing to do with transcriptional impairment. It may be related to the decreased activity of DNA damage sensing proteins (Mognato and Celotti, 2005). Ikeda et al. (2019) maintained human fibroblasts under 3D clinostat simulated microgravity and exposed them to synchronized 1 Gy carbon-ion radiation. It was found the expression of cell cycle inhibitory genes decreased, while the expression of cell cycle promoting genes increased, which may lead to failure of cell cycle checkpoint block and increased genomic instability. By analyzing the mRNA and miRNA expression profiles of human PBL treated by γ-ray irradiation (0.2 or 2 Gy) and microgravity stimulated by RWV for 4 h or 24 h, it was found that microgravity reduced the number of radiation-responsive miRNAs and changed the gene expression of DNA damage repair pathway, suggesting that simulated microgravity can affect the radiation-induced DNA damage response (Girardi et al., 2012). RWV simulated microgravity and 2 Gy γ-ray irradiation were shown to additively change the expression patterns of RNAs while synergistically altering their expression levels in human lymphoblast TK6 cells, among which miRNAs and lncRNAs were predicted to regulate immune/inflammatory response through their target genes (Fu et al., 2020). Tan et al. (2020) suggested that 3D clinostat simulated microgravity for 48 h and 2 Gy X-rays could additively inhibit the proliferation and induce the apoptotic and DNA double-strand breaks of human lung epithelial BEAS-2B cells, possibly due to the upregulation of RAC2
Considering the above-mentioned studies, the effects of radiation quality, types of samples, biological endpoints and even sample collection time points all show an impact on the research results, which should be carefully considered in the follow-up studies. Though various progresses have been achieved, the specific molecular targets mediating combined biological effects of microgravity and radiation remain to be clarified. In addition, the studies on the combined effect of space radiation and microgravity on cells are mainly focused on genetic material, and there is a lack of studies on phenotypic microstructure or submicron structure effects.
Space Radiation and Hypomagnetic Field
In the deep space environment, astronauts will be more susceptible to space radiation-induced adverse effects after leaving the protection of the earth’s magnetic field, which highlights the importance of the combined biological effect study of hypomagnetic field and space radiation. By studying DNA damage and repair of immortalized human bronchial epithelial BEAS-2B cells exposed to HMF (<50 nT) after 2 Gy X-ray irradiation, Xue et al. (2020) found increased survival, less DNA damage and more efficient DNA damage repair, suggesting potential application of HMF in radiation protection. This was also the first that identified that HMF participates in the process of DNA damage repair induced by irradiation.
Future Challenges
Human exploration of space is rapidly developing, which requires deeper studies and understanding of space medicine and space biology. This paper summarizes the research progress on the combined biological effects of space environmental factors and the underlying mechanism in mammalian cells and provides suggestions for the further study of the harmful physiological effects of space environment.
Throughout this review, current research on the combined biological effects of space environmental factors needs to be improved in the following aspects: 1) Except for the study of microgravity combined with space radiation, there are very few studies on the combined effects of other space environmental factors. 2) It is still uncertain which specific genes or signaling pathways mediate the coupling of the biological effects of the different space environmental factors, leading to hesitation in moving forward in both diagnosis and targeted treatment of related diseases. 3) It is speculated that radiation-induced non-targeted effect may play a significant role in the biological outcomes induced by low-dose and low-dose rate space radiation (Shuryak et al., 2021). Therefore, influence of other space environmental factors on the radiation-induced non-targeted effect remains to be clarified. 4) No full-scale ground analogs that can completely model the spaceflight environment (LEO or beyond LEO), including microgravity, hypomagnetic field, disrupted light/dark cycles, space radiation, and noise, while the biological experimental opportunities basing on spacecrafts or space stations are very limited.
In conclusion, there is an urgent need to build a ground-based research platform for synchronized spaceflight factors space environment simulation, in order to carry out biological experiments at the cell, tissue and individual levels, and to enrich the knowledge of the combined biological effects of space environmental factors on Earth as well as their interactive mechanisms, in the hope of providing a theoretical basis for ensuring the health and safety of astronauts.
Author Contributions
Conceptualization, YX and WH; writing—original draft preparation, YX and WH; writing—review and editing, WP and WH; supervision, WH. All authors have read and approved the final version of the manuscript.
Funding
This work was supported by the National Natural Science Foundation of China (Nos 32071243 and 82103786), Collaborative Innovation Center of Radiological Medicine of Jiangsu Higher Education Institutions, and a project funded by the Priority Academic Program Development of Jiangsu Higher Education Institutions (PAPD).
Conflict of Interest
The authors declare that the research was conducted in the absence of any commercial or financial relationships that could be construed as a potential conflict of interest.
Publisher’s Note
All claims expressed in this article are solely those of the authors and do not necessarily represent those of their affiliated organizations, or those of the publisher, the editors and the reviewers. Any product that may be evaluated in this article, or claim that may be made by its manufacturer, is not guaranteed or endorsed by the publisher.
Acknowledgments
We would like to thank Prof. Guangming Zhou, School of Radiation Medicine and Protection of Soochow University, for his constructive suggestions when preparing this publication.
References
Alwood, J. S., Yumoto, K., Mojarrab, R., Limoli, C. L., Almeida, E. A. C., Searby, N. D., et al. (2010). Heavy Ion Irradiation and Unloading Effects on Mouse Lumbar Vertebral Microarchitecture, Mechanical Properties and Tissue Stresses. Bone 47 (2), 248–255. doi:10.1016/j.bone.2010.05.004
Basner, M., Dinges, D. F., Mollicone, D., Ecker, A., Jones, C. W., Hyder, E. C., et al. (2013). Mars 520-d mission Simulation Reveals Protracted Crew Hypokinesis and Alterations of Sleep Duration and Timing. Proc. Natl. Acad. Sci. U.S.A. 110 (7), 2635–2640. doi:10.1073/pnas.1212646110
Canova, S., Fiorasi, F., Mognato, M., Grifalconi, M., Reddi, E., Russo, A., et al. (2005). "Modeled Microgravity" Affects Cell Response to Ionizing Radiation and Increases Genomic Damage. Radiat. Res. 163 (2), 191–199. doi:10.1667/rr3304
Chaudhry, M. A., Omaruddin, R. A., Kreger, B., de Toledo, S. M., and Azzam, E. I. (2012). Micro RNA Responses to Chronic or Acute Exposures to Low Dose Ionizing Radiation. Mol. Biol. Rep. 39 (7), 7549–7558. doi:10.1007/s11033-012-1589-9
Dang, B., Yang, Y., Zhang, E., Li, W., Mi, X., Meng, Y., et al. (2014). Simulated Microgravity Increases Heavy Ion Radiation-Induced Apoptosis in Human B Lymphoblasts. Life Sci. 97 (2), 123–128. doi:10.1016/j.lfs.2013.12.008
Dijk, D.-J., Neri, D. F., Wyatt, J. K., Ronda, J. M., Riel, E., Ritz-De Cecco, A., et al. (2001). Sleep, Performance, Circadian Rhythms, and Light-Dark Cycles during Two Space Shuttle Flights. Am. J. Physiology-Regulatory, Integr. Comp. Physiol. 281, R1647–R1664. doi:10.1152/ajpregu.2001.281.5.r1647
Ding, C., Jia, B., Liu, X., Lv, Y., Wang, J., and Shang, P. (2014a). Dielectric Properties of Rat Whole Blood, Spleen and Testis Were Changed by Hypo-Magnetic Field and Hind-Limb Unloading. Space Med. Med. Eng. 27 (3), 157–163. doi:10.16289/j.cnki.1002-0837.2014.03.008
Ding, C., Jia, B., Liu, X., Lv, Y., Wang, J., and Shang, P. (2014b). Effects of Hypo-Magnetic Field and Simulated Microgravity on Dielectric Properties of Gastrocnemius in Rats. Space Med. Med. Eng. 27 (1), 1–5. doi:10.16289/j.cnki.1002-0837.2014.01.011
Dong, J., Wang, H., Li, G., Wang, K., Tan, Y., Zhang, L., et al. (2021). The Combined Effects of Simulated Microgravity and X-ray Radiation on MC3T3-E1 Cells and Rat Femurs. NPJ Microgravity 7 (1), 3. doi:10.1038/s41526-021-00131-1
Farley, A., Gnyubkin, V., Vanden-Bossche, A., Laroche, N., Neefs, M., Baatout, S., et al. (2020). Unloading-Induced Cortical Bone Loss Is Exacerbated by Low-Dose Irradiation during a Simulated Deep Space Exploration Mission. Calcif Tissue Int. 107 (2), 170–179. doi:10.1007/s00223-020-00708-0
Fu, H., Su, F., Zhu, J., Zheng, X., and Ge, C. (2020). Effect of Simulated Microgravity and Ionizing Radiation on Expression Profiles of miRNA, lncRNA, and mRNA in Human Lymphoblastoid Cells. Life Sci. Space Res. 24, 1–8. doi:10.1016/j.lssr.2019.10.009
Garrett-Bakelman, F. E., Darshi, M., Green, S. J., Gur, R. C., Lin, L., Macias, B. R., et al. (2019). The NASA Twins Study: A Multidimensional Analysis of a Year-Long Human Spaceflight. Science 364 (6436), 8650. doi:10.1126/science.aau8650
Gemignani, A., Piarulli, A., Menicucci, D., Laurino, M., Rota, G., Mastorci, F., et al. (2014). How Stressful Are 105days of Isolation? Sleep EEG Patterns and Tonic Cortisol in Healthy Volunteers Simulating Manned Flight to Mars. Int. J. Psychophysiology 93 (2), 211–219. doi:10.1016/j.ijpsycho.2014.04.008
Ghosh, P., Behnke, B. J., Stabley, J. N., Kilar, C. R., Park, Y., Narayanan, A., et al. (2016). Effects of High-LET Radiation Exposure and Hindlimb Unloading on Skeletal Muscle Resistance Artery Vasomotor Properties and Cancellous Bone Microarchitecture in Mice. Radiat. Res. 185 (3), 257–266. doi:10.1667/RR4308.1
Girardi, C., De Pittà, C., Casara, S., Sales, G., Lanfranchi, G., Celotti, L., et al. (2012). Analysis of miRNA and mRNA Expression Profiles Highlights Alterations in Ionizing Radiation Response of Human Lymphocytes under Modeled Microgravity. Plos One 7 (2), e31293. doi:10.1371/journal.pone.0031293
Hada, M., Ikeda, H., Rhone, J., Beitman, A., Plante, I., Souda, H., et al. (2019). Increased Chromosome Aberrations in Cells Exposed Simultaneously to Simulated Microgravity and Radiation. Ijms 20 (1), 43. doi:10.3390/ijms20010043
Herranz, R., Larkin, O. J., Dijkstra, C. E., Hill, R. J., Anthony, P., Davey, M. R., et al. (2012). Microgravity Simulation by Diamagnetic Levitation: Effects of a strong Gradient Magnetic Field on the Transcriptional Profile of Drosophila melanogaster. BMC Genomics 13, 52. doi:10.1186/1471-2164-13-52
Herranz, R., Anken, R., Boonstra, J., Braun, M., Christianen, P. C. M., de Geest, M., et al. (2013). Ground-based Facilities for Simulation of Microgravity: Organism-specific Recommendations for Their Use, and Recommended Terminology. Astrobiology 13 (1), 1–17. doi:10.1089/ast.2012.0876
Holden, S., Perez, R., Hall, R., Fallgren, C. M., Ponnaiya, B., Garty, G., et al. (2021). Effects of Acute and Chronic Exposure to a Mixed Field of Neutrons and Photons and Single or Fractionated Simulated Galactic Cosmic Ray Exposure on Behavioral and Cognitive Performance in Mice. Radiat. Res. 196 (1), 31–39. doi:10.1667/RADE-20-00228.1
Horneck, G., Rettberg, P., Baumstark-Khan, C., Rink, H., Kozubek, S., Schäfer, M., et al. (1996). DNA Repair in Microgravity: Studies on Bacteria and Mammalian Cells in the Experiments REPAIR and KINETICS. J. Biotechnol. 47 (2-3), 99–112. doi:10.1016/0168-1656(96)01382-x
Horneck, G., Klaus, D. M., and Mancinelli, R. L. (2010). Space Microbiology. Microbiol. Mol. Biol. Rev. 74 (1), 121–156. doi:10.1128/MMBR.00016-09
Ikeda, H., Muratani, M., Hidema, J., Hada, M., Fujiwara, K., Souda, H., et al. (2019). Expression Profile of Cell Cycle-Related Genes in Human Fibroblasts Exposed Simultaneously to Radiation and Simulated Microgravity. Ijms 20 (19), 4791. doi:10.3390/ijms20194791
Inozemtsev, K. O., Kushin, V. V., Strádi, A., Ambrožová, I., Kodaira, S., Szabó, J., et al. (2018). Measurement of Different Components of Secondary Radiation Onboard International Space Station by Means of Passive Detectors. Radiat. Prot. Dosimetry 181 (4), 412–417. doi:10.1093/rpd/ncy043
Jia, B., Xie, L., Zheng, Q., Yang, P.-f., Zhang, W.-j., Ding, C., et al. (2014). A Hypomagnetic Field Aggravates Bone Loss Induced by Hindlimb Unloading in Rat Femurs. Plos ONE 9 (8), e105604. doi:10.1371/journal.pone.0105604
Kokhan, V. S., Kudrin, V. S., and Shtemberg, A. S. (2019a). Serotonin and Noradrenaline Metabolism in the Brain of Rats under the Combined Action of Radiation and Hypogravity in a Ground-Based Experiment. Neurochem. J. 13 (1), 57–61. doi:10.1134/s1819712419010100
Kokhan, V. S., Lebedeva-Georgievskaya, K. B., Kudrin, V. S., Bazyan, A. S., Maltsev, A. V., and Shtemberg, A. S. (2019b). An Investigation of the Single and Combined Effects of Hypogravity and Ionizing Radiation on Brain Monoamine Metabolism and Rats' Behavior. Life Sci. Space Res. 20, 12–19. doi:10.1016/j.lssr.2018.11.003
Kokhan, V. S., Matveeva, M. I., Bazyan, A. S., Kudrin, V. S., Mukhametov, A., and Shtemberg, A. S. (2017). Combined Effects of Antiorthostatic Suspension and Ionizing Radiation on the Behaviour and Neurotransmitters Changes in Different Brain Structures of Rats. Behav. Brain Res. 320, 473–483. doi:10.1016/j.bbr.2016.10.032
Kovalchuk, O., Ponton, A., Filkowski, J., and Kovalchuk, I. (2004). Dissimilar Genome Response to Acute and Chronic Low-Dose Radiation in Male and Female Mice. Mutat. Research/Fundamental Mol. Mech. Mutagenesis 550 (1-2), 59–72. doi:10.1016/j.mrfmmm.2004.02.007
Li, H. Y., Zhang, H., Miao, G. Y., Xie, Y., Sun, C., Di, C. X., et al. (2013). Simulated Microgravity Conditions and Carbon Ion Irradiation Induce Spermatogenic Cell Apoptosis and Sperm DNA Damage. Biomed. Environ. Sci. 26 (9), 726–734. doi:10.3967/0895-3988.2013.09.003
Liang, X., Zhang, L., Shen, H., Chen, X., Wan, Y., Li, L., et al. (2014). Effects of a 45-day Head-Down Bed Rest on the Diurnal Rhythms of Activity, Sleep, and Heart Rate. Biol. Rhythm Res. 45 (4), 591–601. doi:10.1080/09291016.2014.882093
Lin, X., Zhang, K., Wei, D., Tian, Y., Gao, Y., Chen, Z., et al. (2020). The Impact of Spaceflight and Simulated Microgravity on Cell Adhesion. Ijms 21 (9), 3031. doi:10.3390/ijms21093031
Liu, Y., Zhang, L. W., Zhang, H., Wu, Z. H., Wang, Z. H., Wang, Y. B., et al. (2013). Risk of Simulated Microgravity on Testicular Injury Induced by High-LET Carbon-Ion Beams in Mice. Nucl. Sci. Tech. 24 (6), 64–69. doi:10.13538/j.1001-8042/nst.2013.06.002
Lloyd, S. A., Bandstra, E. R., Willey, J. S., Riffle, S. E., Tirado-Lee, L., Nelson, G. A., et al. (2012). Effect of Proton Irradiation Followed by Hindlimb Unloading on Bone in Mature Mice: a Model of Long-Duration Spaceflight. Bone 51 (4), 756–764. doi:10.1016/j.bone.2012.07.001
Lu, T., Zhang, Y., Kidane, Y., Feiveson, A., Stodieck, L., Karouia, F., et al. (2017). Cellular Responses and Gene Expression Profile Changes Due to Bleomycin-Induced DNA Damage in Human Fibroblasts in Space. Plos One 12 (3), e0170358. doi:10.1371/journal.pone.0170358
Macias, B. R., Lima, F., Swift, J. M., Shirazi-Fard, Y., Greene, E. S., Allen, M. R., et al. (2016). Simulating the Lunar Environment: Partial Weightbearing and High-LET Radiation-Induce Bone Loss and Increase Sclerostin-Positive Osteocytes. Radiat. Res. 186 (3), 254–263. doi:10.1667/RR13579.1
Manti, L., Durante, M., Cirrone, G., Grossi, G., Lattuada, M., Pugliese, M., et al. (2005). Modelled Microgravity Does Not Modify the Yield of Chromosome Aberrations Induced by High-Energy Protons in Human Lymphocytes. Int. J. Radiat. Biol. 81 (2), 147–155. doi:10.1080/09553000500091188
Mao, X. W., Boerma, M., Rodriguez, D., Campbell-Beachler, M., Jones, T., Stanbouly, S., et al. (2018). Combined Effects of Low-Dose Proton Radiation and Simulated Microgravity on the Mouse Retina and the Hematopoietic System. Radiat. Res. 192 (3), 241–250. doi:10.1667/RR15219.1
Mao, X. W., Nishiyama, N. C., Campbell-Beachler, M., Gifford, P., Haynes, K. E., Gridley, D. S., et al. (2017). Role of NADPH Oxidase as a Mediator of Oxidative Damage in Low-Dose Irradiated and Hindlimb-Unloaded Mice. Radiat. Res. 188 (4), 392–399. doi:10.1667/RR14754.1
Mao, X. W., Nishiyama, N. C., Pecaut, M. J., Campbell-Beachler, M., Gifford, P., Haynes, K. E., et al. (2016). Simulated Microgravity and Low-Dose/Low-Dose-Rate Radiation Induces Oxidative Damage in the Mouse Brain. Radiat. Res. 185 (6), 647–657. doi:10.1667/RR14267.1
Min, R., Chen, Z., Wang, Y., Deng, Z., Zhang, Y., and Deng, Y. (2021). Quantitative Proteomic Analysis of Cortex in the Depressive-like Behavior of Rats Induced by the Simulated Complex Space Environment. J. Proteomics 237, 104144. doi:10.1016/j.jprot.2021.104144
Mo, W., Liu, Y., and He, R. (2014). Hypomagnetic Field, an Ignorable Environmental Factor in Space? Sci. China Life Sci. 57 (7), 726–728. doi:10.1007/s11427-014-4662-x
Mognato, M., and Celotti, L. (2005). Modeled Microgravity Affects Cell Survival and HPRT Mutant Frequency, but Not the Expression of DNA Repair Genes in Human Lymphocytes Irradiated with Ionising Radiation. Mutat. Research/Fundamental Mol. Mech. Mutagenesis 578 (1-2), 417–429. doi:10.1016/j.mrfmmm.2005.06.011
Mognato, M., Girardi, C., Fabris, S., and Celotti, L. (2009). DNA Repair in Modeled Microgravity: Double Strand Break Rejoining Activity in Human Lymphocytes Irradiated with γ-rays. Mutat. Research/Fundamental Mol. Mech. Mutagenesis 663 (1-2), 32–39. doi:10.1016/j.mrfmmm.2009.01.002
Moreno-Villanueva, M., Wong, M., Lu, T., Zhang, Y., and Wu, H. (2017). Interplay of Space Radiation and Microgravity in DNA Damage and DNA Damage Response. NPJ Microgravity 3, 14. doi:10.1038/s41526-017-0019-7
Mosesso, P., Schuber, M., Seibt, D., Schmitz, C., Fiore, M., Schinoppi, A., et al. (2001). X-ray-induced Chromosome Aberrations in Human Lymphocytes In Vitro Are Potentiated under Simulated Microgravity Conditions (Clinostat). Phys. Med. 17 Suppl 1 (Suppl. 1), 264–266.
Neelam, S., Richardson, B., Barker, R., Udave, C., Gilroy, S., Cameron, M. J., et al. (2020). Changes in Nuclear Shape and Gene Expression in Response to Simulated Microgravity Are LINC Complex-dependent. Ijms 21 (18), 6762. doi:10.3390/ijms21186762
Overbey, E. G., Paul, A. M., da Silveira, W. A., Tahimic, C. G. T., Reinsch, S. S., Szewczyk, N., et al. (2019). Mice Exposed to Combined Chronic Low-Dose Irradiation and Modeled Microgravity Develop Long-Term Neurological Sequelae. Ijms 20 (17), 4094. doi:10.3390/ijms20174094
Paul, A. M., Overbey, E. G., da Silveira, W. A., Szewczyk, N., Nishiyama, N. C., Pecaut, M. J., et al. (2021). Immunological and Hematological Outcomes Following Protracted Low Dose/low Dose Rate Ionizing Radiation and Simulated Microgravity. Sci. Rep. 11 (1), 11452. doi:10.1038/s41598-021-90439-5
Prisby, R. D., Alwood, J. S., Behnke, B. J., Stabley, J. N., McCullough, D. J., Ghosh, P., et al. (2016). Effects of Hindlimb Unloading and Ionizing Radiation on Skeletal Muscle Resistance Artery Vasodilation and its Relation to Cancellous Bone in Mice. J. Appl. Physiol. 120 (2), 97–106. doi:10.1152/japplphysiol.00423.2015
Sadhukhan, R., Majumdar, D., Garg, S., Landes, R. D., McHargue, V., Pawar, S. A., et al. (2021). Simultaneous Exposure to Chronic Irradiation and Simulated Microgravity Differentially Alters Immune Cell Phenotype in Mouse Thymus and Spleen. Life Sci. Space Res. 28, 66–73. doi:10.1016/j.lssr.2020.09.004
Sanzari, J. K., Romero-Weaver, A. L., James, G., Krigsfeld, G., Lin, L., Diffenderfer, E. S., et al. (2013). Leukocyte Activity Is Altered in a Ground Based Murine Model of Microgravity and Proton Radiation Exposure. Plos One 8 (8), e71757. doi:10.1371/journal.pone.0071757
Seawright, J. W., Samman, Y., Sridharan, V., Mao, X. W., Cao, M., Singh, P., et al. (2017). Effects of Low-Dose Rate γ-irradiation Combined with Simulated Microgravity on Markers of Oxidative Stress, DNA Methylation Potential, and Remodeling in the Mouse Heart. Plos One 12 (7), e0180594. doi:10.1371/journal.pone.0180594
Shtemberg, A. S., Lebedeva-Georgievskaya, K. B., Matveeva, M. I., Kudrin, V. S., Narkevich, V. B., Klodt, P. M., et al. (2014). Effect of Space Flight Factors Simulated in Ground-Based Experiments on the Behavior, Discriminant Learning, and Exchange of Monoamines in Different Brain Structures of Rats. Biol. Bull. Russ. Acad. Sci. 41 (2), 161–167. doi:10.1134/s1062359014020095
Shuryak, I., Sachs, R. K., and Brenner, D. J. (2021). Quantitative Modeling of Carcinogenesis Induced by Single Beams or Mixtures of Space Radiations Using Targeted and Non-targeted Effects. Sci. Rep. 11 (1), 23467. doi:10.1038/s41598-021-02883-y
Simonsen, L. C., Slaba, T. C., Guida, P., and Rusek, A. (2020). NASA's First Ground-Based Galactic Cosmic Ray Simulator: Enabling a new era in Space Radiobiology Research. Plos Biol. 18 (5), e3000669. doi:10.1371/journal.pbio.3000669
Stervbo, U., Roch, T., Kornprobst, T., Sawitzki, B., Grütz, G., Wilhelm, A., et al. (2018). Gravitational Stress during Parabolic Flights Reduces the Number of Circulating Innate and Adaptive Leukocyte Subsets in Human Blood. Plos One 13 (11), e0206272. doi:10.1371/journal.pone.0206272
Taillard, J., Sagaspe, P., Philip, P., and Bioulac, S. (2021). Sleep Timing, Chronotype and Social Jetlag: Impact on Cognitive Abilities and Psychiatric Disorders. Biochem. Pharmacol. 191, 114438. doi:10.1016/j.bcp.2021.114438
Tan, S., Pei, W., Huang, H., Zhou, G., and Hu, W. (2020). Additive Effects of Simulated Microgravity and Ionizing Radiation in Cell Death, Induction of ROS and Expression of RAC2 in Human Bronchial Epithelial Cells. NPJ Microgravity 6 (1), 34. doi:10.1038/s41526-020-00123-7
Thirsk, R., Kuipers, A., Mukai, C., and Williams, D. (2009). The Space-Flight Environment: the International Space Station and beyond. Can. Med. Assoc. J. 180 (12), 1216–1220. doi:10.1503/cmaj.081125
Vigo, D. E., Tuerlinckx, F., Ogrinz, B., Wan, L., Simonelli, G., Bersenev, E., et al. (2013). Circadian Rhythm of Autonomic Cardiovascular Control during Mars500 Simulated mission to Mars. Aviation, Space Environ. Med. 84 (10), 1023–1028. doi:10.3357/asem.3612.2013
von Gall, C. (2022). The Effects of Light and the Circadian System on Rhythmic Brain Function. Ijms 23 (5), 2778. doi:10.3390/ijms23052778
Williams, D., Kuipers, A., Mukai, C., and Thirsk, R. (2009). Acclimation during Space Flight: Effects on Human Physiology. Can. Med. Assoc. J. 180 (13), 1317–1323. doi:10.1503/cmaj.090628
Wuest, S. L., Gantenbein, B., Ille, F., and Egli, M. (2018). Electrophysiological Experiments in Microgravity: Lessons Learned and Future Challenges. NPJ Microgravity 4, 7. doi:10.1038/s41526-018-0042-3
Xue, X., Ali, Y. F., Liu, C., Hong, Z., Luo, W., Nie, J., et al. (2020a). Geomagnetic Shielding Enhances Radiation Resistance by Promoting DNA Repair Process in Human Bronchial Epithelial Cells. Ijms 21 (23), 9304. doi:10.3390/ijms21239304
Xue, Y., Yang, J., Luo, J., Ren, L., Shen, Y., Dong, D., et al. (2020b). Disorder of Iron Metabolism Inhibits the Recovery of Unloading‐Induced Bone Loss in Hypomagnetic Field. J. Bone Miner Res. 35 (6), 1163–1173. doi:10.1002/jbmr.3949
Yamanouchi, S., Rhone, J., Mao, J.-H., Fujiwara, K., Saganti, P. B., Takahashi, A., et al. (2020). Simultaneous Exposure of Cultured Human Lymphoblastic Cells to Simulated Microgravity and Radiation Increases Chromosome Aberrations. Life 10 (9), 187. doi:10.3390/life10090187
Yang, J., Meng, X., Dong, D., Xue, Y., Chen, X., Wang, S., et al. (2018). Iron Overload Involved in the Enhancement of Unloading-Induced Bone Loss by Hypomagnetic Field. Bone 114, 235–245. doi:10.1016/j.bone.2018.06.012
Yatagai, F., Honma, M., Dohmae, N., and Ishioka, N. (2019). Biological Effects of Space Environmental Factors: A Possible Interaction between Space Radiation and Microgravity. Life Sci. Space Res. 20, 113–123. doi:10.1016/j.lssr.2018.10.004
Yatagai, F., and Ishioka, N. (2014). Are Biological Effects of Space Radiation Really Altered under the Microgravity Environment? Life Sci. Space Res. 3, 76–89. doi:10.1016/j.lssr.2014.09.005
Zhang, B., Wang, L., Zhan, A., Wang, M., Tian, L., Guo, W., et al. (2021a). Long-term Exposure to a Hypomagnetic Field Attenuates Adult Hippocampal Neurogenesis and Cognition. Nat. Commun. 12 (1), 1174. doi:10.1038/s41467-021-21468-x
Zhang, Y. N., Shi, W. G., Li, H., Hua, J. R., Feng, X., Wei, W. J., et al. (2018). Bone Loss Induced by Simulated Microgravity, Ionizing Radiation And/or Ultradian Rhythms in the Hindlimbs of Rats. Biomed. Environ. Sci. 31 (2), 126–135. doi:10.3967/bes2018.015
Zhang, Z., Xue, Y., Yang, J., Shang, P., and Yuan, X. (2021b). Biological Effects of Hypomagnetic Field: Ground‐Based Data for Space Exploration. Bioelectromagnetics 42 (6), 516–531. doi:10.1002/bem.22360
Zhu, H., Zhang, L., Qian, M., Shi, T., Fan, F., Li, W., et al. (20202020). Microgravity versus Microgravity and Irradiation: Investigating the Change of Neuroendocrine-Immune System and the Antagonistic Effect of Traditional Chinese Medicine Formula. Biomed. Res. Int. 2020, 1–9. doi:10.1155/2020/2641324
Keywords: microgravity, space radiation, hypomagnetic field, space exploration, combined biological effect
Citation: Xu Y, Pei W and Hu W (2022) A Current Overview of the Biological Effects of Combined Space Environmental Factors in Mammals. Front. Cell Dev. Biol. 10:861006. doi: 10.3389/fcell.2022.861006
Received: 24 January 2022; Accepted: 23 March 2022;
Published: 12 April 2022.
Edited by:
Afshin Beheshti, Space Biosciences Research of NASA Ames Research Center, United StatesReviewed by:
Michael Lebert, University of Erlangen Nuremberg, GermanyAmber M. Paul, Embry–Riddle Aeronautical University, United States
Copyright © 2022 Xu, Pei and Hu. This is an open-access article distributed under the terms of the Creative Commons Attribution License (CC BY). The use, distribution or reproduction in other forums is permitted, provided the original author(s) and the copyright owner(s) are credited and that the original publication in this journal is cited, in accordance with accepted academic practice. No use, distribution or reproduction is permitted which does not comply with these terms.
*Correspondence: Weiwei Pei, wwpei@suda.edu.cn; Wentao Hu, wthu@suda.edu.cn