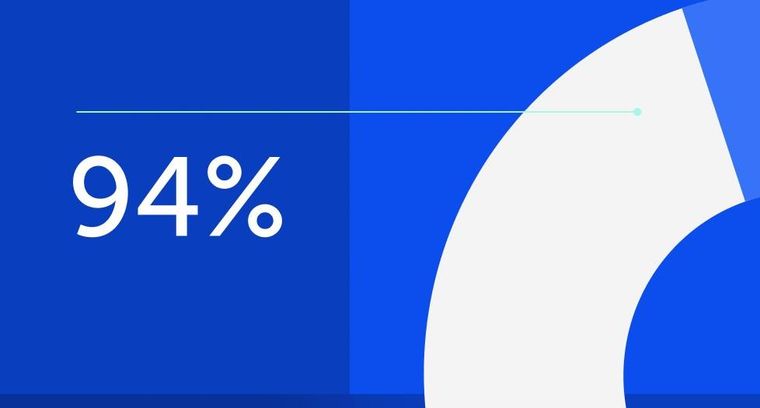
94% of researchers rate our articles as excellent or good
Learn more about the work of our research integrity team to safeguard the quality of each article we publish.
Find out more
REVIEW article
Front. Cell Dev. Biol., 20 June 2022
Sec. Epigenomics and Epigenetics
Volume 10 - 2022 | https://doi.org/10.3389/fcell.2022.831215
This article is part of the Research TopicHeterogeneity in Cells: Identification of Markers and Functional DifferencesView all 4 articles
CD4+ T cells differentiate towards different subpopulations through the regulation of lineage-specific cytokines and transcription factors, which flexibly respond to various immune challenges. However, considerable work has demonstrated that the CD4+ T-cell differentiation mechanism is complex and not limited to transcription factors and cytokines. Long noncoding RNAs (lncRNAs) are RNA molecules with lengths exceeding 200 base pairs that regulate various biological processes and genes. LncRNAs have been found to conciliate the plasticity of CD4+ T-cell differentiation. Then, we focused on lncRNAs involved in CD4+ T-cell differentiation and enlisted some molecular thought into the plasticity and functional heterogeneity of CD4+ T cells. Furthermore, elucidating how lncRNAs modulate CD4+ T-cell differentiation in disparate immune diseases may provide a basis for the pathological mechanism of immune-mediated diseases.
CD4+ T cells possess vital properties in immunomodulation, mediating adaptive immune responses by differentiating into various subpopulations, such as T-helper 1 (Th1), Th2, Th17, Th9, and Th22 cells; regulatory T cells (Tregs); and T follicular helper (Tfh) cells. Th1 cells function to destroy endocellular viruses and bacteria, whereas Th2 cells target extracellular pathogens and are necessary to fight parasitic infections (Saravia et al., 2019). Similar to Th2 cells, Th9 cells are involved in fighting parasitic infections and inducing allergic diseases (Sehra et al., 2015). Additionally, Th17 cells stimulate protective immunity against extracellular bacteria and fungi, and Th22 cells express specific interleukin (IL)-22 subsets and are distinguished from Th17 cells, which also produce IL-22 (Saravia et al., 2019). Tregs figure prominently in maintaining the status of immune balance and preventing autoimmune diseases and transplant rejection. Tfh cells, another subgroup of CD4+ T cells, activate B cells and regulate humoral immune responses synergistically, and are a crucial class of T cells in germinal centers (Crotty, 2019; Niogret et al., 2021).
Long noncoding RNAs (lncRNAs) are noncoding RNA molecules with lengths of greater than 200 base pairs and a structure similar to that of mRNA, although without the ability to encode protein. Importantly, lncRNAs are characterized by cell-specific than protein-coding genes and are involved in multiple biological processes such as regulating epigenetic inheritance, cell cycle, and cell differentiation (Cabili et al., 2011). Moreover, lncRNAs exert their various functions via a variety of mechanisms, including regulation of transcription through chromatin, modulation of protein activity, direct participation in post-transcriptional regulation of mRNA, and regulation of microRNAs (miRNAs) through sponging (Thomson and Dinger, 2016; Akhade et al., 2017). Abnormal lncRNA expression can influence the emergence, development, and prognosis of various immune diseases by controlling CD4+ T-cell differentiation. Thus, further analyses of lncRNAs may facilitate the elucidation of the molecular mechanisms of differentiation plasticity in CD4+ T cells.
Here, we review the functional plasticity of CD4+ T-cell subsets and how their effector function is influenced by the transcriptional regulatory network triggered by T cells, which includes lncRNAs. Comprehending the modulation mechanisms and identifying the participants in CD4+ T-cell plasticity may raise our awareness of the pathogenesis of immune-related diseases and facilitate the identification of novel therapeutic targets.
Naïve CD4+ T cells are stimulated to differentiate into Th1 cells mainly by the cytokines interferon (IFN)-γ and IL-12. Signal transducer and activator of transcription (STAT) 1 is activated by IFN-γ signaling, which stimulates the production of T-bet, a transcription factor of the Th1 lineage. IL-12 further drives T-bet expression through STAT4. T-bet enhances the Th1 phenotype by promoting IFN-γ and IL-12 expression (Zhang et al., 2014). Furthermore, Th2 cells can be induced to differentiate by IL-4 and IL-2. IL-4 accelerates IL-4 transcription and the production of the transcription factors musculoaponeurotic fibrosarcoma (c-MAF) and GATA3 by activating STAT6. Among these factors, c-MAF promotes IL-4 expression and shuts down IFN-γ generation, whereas GATA3 makes Th2-cell differentiation primarily by stimulating the induction of IL-5 and IL-13. IL-2 induces Th2 cytokine production by activating STAT5 (Zhu, 2015). The Th1/Th2 balance is critical for the workings of the immune system, and when that balance is disrupted in favor of either side, disease can occur.
The lncRNA transcript Tmevpg1, also called NeST or Ifng-AS1, was originally discovered in the context of Theiler’s virus infection, and the researchers found that mice without Tmevpg1 expression could not control intracranial viral infection (Collier et al., 2012). Tmevpg1, an essential gene involved in specific expression of the IFN-γ gene (Ifng), is located near and co-expressed with Ifng (Collier et al., 2012). Several studies have suggested that transcription of Tmevpg1 is to rely on STAT4 and T-bet (Collier et al., 2012; Collier et al., 2014). Mechanistically, the epigenetic remodeling of Tmevpg1 is induced by T-bet, resulting in the aggregation of transcription factors NF-κB and Ets-1 at this site (Collier et al., 2014). Furthermore, T-bet completed specific expression of Ifng in Th1 lineage by epigenetic remodeling of Tmevpg1 specific enhancers and Ifng specific enhancers. Thus, Tmevpg1 transcription is Th1 selective. GATA3-AS1 is a divergent lncRNA contiguous to GATA3 that has been shown to be essential for efficient transcription of IL-5 and IL-13 genes and for GATA3 transcription under a Th2 bias. GATA3-AS1 forms permissive chromatin markers at the GATA3-AS1-GATA3 locus via H3K27 acetylation and H3K4 di-/trimethylation. In addition, GATA3-AS1 binds the MLL methyltransferase component to form an R-loop at the GATA3/GATA3-AS1 locus, thereby leading MLL methyltransferase to the gene sites (Gibbons et al., 2018). Accordingly, the lncRNA GATA3-AS1 promotes Th2 lineage development through SATA3. Moreover, lincR-Ccr2-5′AS regulates the expression of chemokine in mice, including Ccr1, Ccr3, Ccr2, and Ccr5. LincR-Ccr2-5′AS has been shown to be regulated by GATA-3 and, lincR-Ccr2-5′AS together with GATA-3, regulates Th2 cell-specific gene expression and is critical for Th2 cell migration (Hu et al., 2013).
Locus control region (LCR) plays a major role in the regulation of Th2 cytokine genes (Koh et al., 2010). In humans, the gene encoding an lncRNA cluster overlaps the RAD50 gene (encoding a double-strand break repair protein) and is adjacent to the TH2 LCR in mice, so it is named TH2-LCR lncRNA (Spurlock et al., 2015). The TH2-LCR lncRNA cluster consists of four transcripts, all of which are Th2-lineage specific and co-expressed with IL-4, IL-5, and IL-13 but not RAD50 (Spurlock et al., 2015). One function of TH2-LCR lncRNA is to promote transcription of IL-4 and IL-13 by promoting the formation of chromatin marker H3K4Me3. However, the mechanism of IL-5 transcription is still unclear (Spurlock et al., 2015). TH2-LCR is necessary for Th2 cytokine production and positively regulates Th2 subset formation. Additionally, chromatin-related lncRNA linc-MAF-4 targeting the Th1 subpopulation is inversely correlated with the expression of c-MAF, a Th2-associated transcription factor encoded by the MAF gene. Linc-MAF-4 negatively regulates MAF transcription by using chromatin modifier LSD1 and Zeste homolog 2 (EZH2) to regulate the enzyme activity of EZH2 on the MAF promoter (Ranzani et al., 2015). Accordingly, linc-MAF-4 may suppress Th2-cell differentiation.
The lncRNA AW112010 potentiates inflammatory T-cell differentiation, which exhibits high expression in Th1 and Th17 cells and low expression in Th2 cells. Studies have shown that AW112010 interacts with the histone demethylase KDM5A to reduce H3K4me3 at the IL-10 gene locus, inhibit IL-10 expression, and promote inflammatory T-cell differentiation (Yang et al., 2020). Collectively, Tmevpg1, GATA3-AS1 and Th2-LCR are examples of lncRNAs that regulate the expression of adjacent protein-coding genes. These genes are frequently co-expressed with their corresponding lncRNAs. By contrast, the lncRNA lincR-Ccr2-5′AS affects the expression of adjacent genes, demonstrating that some lncRNAs can also facilitate the transcription of adjacent related genes rather than just being limited to co-expression. LncRNAs can also participate in transcriptional regulation through chromatin, including histone modification, DNA methylation, and chromatin remodeling. As described above, linc-MAF-4 recruits the chromatin modifiers to regulate MAF transcription, GATA3-AS1 recruits target gene sites through histone modification enzymes, and AW112010 inhibits IL-10 expression by decreasing H3K4me3 at the IL-10 gene locus. These findings indicate that lncRNA modulates the open or closed state of chromatin, which is determined by epigenetic factors.
The lncRNA nuclear paraspeckle assembly transcript 1 (NEAT1) is an important component of paraspeckles, a substructure that is widely found in mammalian nuclei (Fox and Lamond, 2010). Paraspeckles can regulate gene expression, and NEAT1 is the skeleton that determines whether paraspeckles can form (Clemson et al., 2009). Previous studies have shown that NEAT1 is up-regulated in many cancers, and its high expression often leads to poor prognosis of patients (Chakravarty et al., 2014; Chen et al., 2015; Ma et al., 2016). NEAT1 also plays a role in CD4+ T-cell differentiation. Studies have shown that NEAT1 can enhance the level of STAT6 by repressing STAT6 ubiquitination and degradation, thereby increasing Th2-cell proliferation (Huang et al., 2021). Systemic lupus erythematosus (SLE) is a systemic autoimmune disease. The transformation of Th1/Th2 cell balance to Th2 is considered to be one of the key processes in the pathogenesis of SLE (Marder, 2019). Notably, NEAT1 is highly expressed in peripheral blood mononuclear cells (PBMCs) of patients with SLE and negatively correlated with the Th1/Th2 ratio, thus influencing the progression of systemic lupus erythematosus (Jiang et al., 2021). The mechanism of action of NEAT1 reflects another function of lncRNAs, which bind to specific proteins and regulate protein activity. This transcriptional regulation may involve either a cis or trans mechanism, and the target proteins are usually transcription factors (Long et al., 2017).
The lncRNA plasmacytoma variant translocation 1 (PVT1) is encoded by PVT1 gene, and is usually highly expressed in various malignant tumor tissues or cells, while the expression level is low in normal tissues (Jin et al., 2019). PVT1 is a potential predictor of cancer progression and patient prognosis (Jin et al., 2019). LncRNA PVT1 has been shown to function in the differentiation of CD4+ T cells through miRNAs. In many patients with asthma, chronic airway inflammation is caused by Th2 cells (Hammad and Lambrecht, 2021). In ozone-induced exacerbation of asthma, PVT1 activates the asthma-related phosphatidylinositol 3-kinase (PI3K)/AKT/mammalian target of rapamycin (mTOR) pathway by inhibiting miR-15a-5p, leading to a decrease in Th1/Th2 ratio, thereby promoting the progression of asthma. Specifically, IL-4, IL-10, and GATA3 proteins levels increased, whereas IFN-γ, IL-2, and T-bet levels decreased (Wei et al., 2020). PVT1 has also been shown to exhibit activity in other pathways. Depletion of PVT1 in Th1 cells results in reduced IFN-γ secretion and Th1 cell dysfunction. This process also reduces the glycolysis gene transcription factor Myc expression and inhibits cell proliferation (Fu et al., 2020). Sjögren’s syndrome (SS) is a chronic inflammatory autoimmune disease involving exocrine glands (Fox, 2005). Excessive proliferation and activation of CD4+ T cells in salivary gland tissue is a hallmark of SS (Fu et al., 2020). LncRNA PVT1 upregulation maintained Myc expression in CD4+ T cells of SS patients, while inhibition of glycolysis attenuated SS-like autoimmune response (Fu et al., 2020). PVT1 may be a potential therapeutic target for SS. LncRNA growth arrest specific 5 (GAS5) is a well-known tumor suppressor (Gao et al., 2017). According to report, GAS5 is associated with T-cell differentiation in immune-related diseases (Song et al., 2021). Allergic rhinitis (AR) is a well-documented allergic disease mediated by Th2 cells (Ji et al., 2021). The lncRNA GAS5 is overexpressed in nasal mucus and nasal mucosal epithelial cells of AR patients. This lncRNA may inhibit Th1-cell differentiation by downregulating the expression of EZH2 and T-bet, and promote the differentiation of CD4+ T cells into Th2. LncRNA GAS5 destroys the balance of Th1/Th2, leading to the occurrence of AR (Zhu et al., 2020a). Another study explored the mechanisms through which GAS5 enhances Th2-cell differentiation in AR, and found that GAS5 and CircHIPK3 induced GATA-3 upregulation by regulating the common target miR-495, promote Th2-cell differentiation and aggravate AR (Zhu et al., 2020b). LncRNA GAS5 may be a potential biomarker for the development and progression of AR. In distinct clinical contexts, both PVT1 and GAS5 play roles in Th1- and Th2-cell development. As a result, we speculate that the same lncRNAs may perform distinct or comparable activities in different settings by mediating multiple pathways.
The lncRNA metastasis-associated lung adenocarcinoma transcript 1 (MALAT1) is found in early non-small-cell lung cancer (NSCLC) and can predict metastasis and patient prognosis in early NSCLC (Li et al., 2018). In bronchial asthma, lncRNA MALAT1 inhibits the expression of miR-155 in CD4+ T cells through sponging and acts in conjunction with the CTLA-4 gene to alter T-cell activity. As a result, the Th1/Th2 balance is biased toward the Th2 lineage, thereby promoting the development of bronchial asthma (Liang and Tang, 2020). LncRNA taurine upregulated 1 (TUG1) is one of the earliest lncRNAs found to be associated with human disease. It is abnormally expressed in many malignant tumors and is involved in the development of many cancers and cardiovascular diseases (Guo et al., 2020). A recent study found the role of lncRNA TUG1 in promoting Th2-cell differentiation in asthma. LncRNA TUG1 and B7-H3 were upregulated in peripheral blood of asthmatic children, while miR-29c was downregulated. The activity of B7-H3 in Th2-cell differentiation was inhibited by miR-29c. LncRNA TUG1 regulates the expression of B7-H3 through sponge miR-29c and, thus promoting Th2-cell differentiation (Sun et al., 2021). This suggests that lncRNA TUG1 may be involved in the acute attack of asthma. To summarize, the lncRNAs PVT1, GAS5, MALAT1, and TUG1 can bind with corresponding miRNAs to regulate Th-cell differentiation, highlighting the roles of lncRNAs in the regulation of miRNAs. Indeed, some lncRNAs carry sequences complementary to certain miRNA sequences, enabling them to bind miRNAs like sponges and thereby interrupt miRNAs from interacting with their target mRNAs.
LncRNA FOXD3-AS1 was found to be downregulated in nasal mucosa of patients with AR, while IL-25, which promotes Th2-cell differentiation, was upregulated. Overexpression of the lncRNA FOXD3-AS1 inhibits IL-25 expression in nasal epithelial cells and prevents the occurrence of the Th2 immune response (Zhang et al., 2020). LncRNA FOXD3-AS1 has the potential to serve as a therapeutic target for AR. Additionally, the lncRNA Dreg1 is intimately linked to the expression of GATA3 during Th2-cell differentiation and participates in the establishment of GATA3 expression (Chan et al., 2021), suggesting that Dreg1 may promote Th2-cell differentiation. The expression of LncRNA028466 decreased after inoculation with recombinant Echinococcus granulosus antigen P29 vaccine, and studies have shown that LncRNA028466 can promote Th2-cell differentiation and the secretion of IL-10 and IL-4, but inhibits the secretion of Th1-related cytokines, IFN-γ and IL-2 (Wang et al., 2021). Cystic echinococcosis is a chronic parasitic disease for which there is still no commercial vaccine due to the complex host-parasite relationship. However, some effective antigens have been used as candidate vaccines for cystic echinococcosis prevention, such as recombinant Echinococcus granulosus antigen P29 (Wang et al., 2021). LncRNA028466 may be involved in recombinant Echinococcus granulosus antigen P29 vaccination-mediated immune response by regulating the differentiation of CD4+ T cells during echinococcus granulosus infection (Wang et al., 2021). Finally, various knockdown and overexpression experiments indicate that lncRNAs play a key role in CD4+ T-cell differentiation. However, the characterization of these phenomena requires further study of the mechanisms by which they affect CD4+ T-cell differentiation (Figure 1).
FIGURE 1. The figure depicts the role of lncRNA in the development of Th1 and Th2 cells. Th1 cells are regulated by light yellow lncRNA, and Th2 cells are regulated by red lncRNA. Most lncRNAs affect Th1 and Th2 differentiation at different stages to promote or inhibit cell differentiation.
Th17 cells are defined by their ability to produce the cytokine IL-17 as well as express the transcription factor RAR-related orphan receptor γ (RORγt). IL-6 and transforming growth factor (TGF)-β regulate RORγt expression via STAT3 and Smad proteins, respectively, promoting differentiation into the Th17 lineage (Chang et al., 2020). IL-21 also activates STAT3 as a surrogate cytokine. Alternatively, the STAT3 pathway can also be activated by IL-23, promoting Th17 lineage maintenance, but not commitment (Zhang, 2018).
The lncRNA DNA-damage-inducible transcript 4 (lncDDIT4) represses Th17-cell differentiation through the targeting effect of DDIT4, a cytoplasmic protein that is transcriptionally upregulated in the backdrop of DNA damage and is important for T cell survival and proliferation (Reuschel et al., 2015). DDIT4, located upstream of lncDDIT4, is a candidate target for cis regulation of lncDDIT4. The PI3K-AKT-mTORC1-S6K axis has been reported to actively regulate Th17 differentiation (Kurebayashi et al., 2012). Furthermore, lncDDIT4 targets DDIT4, which activates the tuberous sclerosis complex 1/2 complex to inhibit the mTOR pathway, ultimately inhibiting IL-17 production (Zhang et al., 2018). The lncRNA 1700040D17Rik was obtained from a mouse spleen cDNA library prepared from experimental autoimmune encephalomyelitis (EAE) model mice, and its expression is thought to affect Th17-cell differentiation. EAE mouse model is an ideal animal model for human multiple sclerosis (MS). MS is an inflammatory demyelinating disease of the central nervous system, the pathogenesis of which is associated with increased Th1 and Th17 lineages (Moser et al., 2020). Moreover, 1700040D17Rik expression has been shown to be markedly decreased in EAE model mice but upregulated in mice treated with RHIL23R-CHR, an antagonist of endogenous IL-23, which functions to maintain Th17-cell differentiation. Functional studies have shown that 1700040D17Rik is located near the RORγt gene and may affect the expression of RORγt in cis-mode and inhibit Th17-cell differentiation (Guo et al., 2017). LncRNA 1700040D17Rik may be a potential therapeutic target or biomarker for MS.
NEAT1 is also involved in Th17-cell differentiation. Rheumatoid arthritis (RA) is a chronic autoimmune disease dominated by inflammatory synovitis, and the increase of Th17 cells is closely related to RA. Therefore, inhibition of Th17 cell differentiation as a therapeutic target may be a potential strategy for RA (Shui et al., 2019). Studies have shown that NEAT1 expression is upregulated in PBMC of RA patients and in vitro induced Th17 cells. By knocking down NEAT1, the arthritic degree of type II collagen induced arthritis in mice was alleviated. Binding of NEAT1 to STAT3 prevents ubiquitination-mediated protein degradation and positively regulates STAT3 levels, thereby facilitating the regulation of Th17-cell differentiation. However, NEAT1 knockdown alone suppresses Th17-cell differentiation, whereas simultaneous NEAT1 knockdown and overexpression of STAT3 showed opposite effects; these findings reveal that NEAT1 can be a helper molecule rather than a decisive factor in Th17 differentiation (Shui et al., 2019). As described above, lncRNA GAS5 promotes Th2-cell differentiation. Studies have found that GAS5 also plays a role in Th17-cell differentiation and is linked to the occurrence and progression of autoimmune disorders (Wu et al., 2019). Immune thrombocytopenia (ITP) is a common autoimmune disease in which increased differentiation of Th17 cells accelerates ITP progression (Li et al., 2020). GAS5 expression is downregulated in PMBC of ITP patients and spleen tissues of ITP mice, while overexpression of GAS5 in CD4+ T cells results in downregulated RORγt protein expression and suppresses IL-17 secretion. GAS5 inhibits Th17 differentiation by promoting STAT3 ubiquitination, alleviates ITP, and provides a possible therapeutic target for ITP (Li et al., 2020). Similarly, the lincRNA XLOC000261, which is associated with Crohn’s disease, appears to negatively regulate RORγt protein expression (Braga-Neto et al., 2020). The functions of lncRNAs to bind transcription factors or regulate transcription as enhancer RNA are reflected in the above. We discovered that the same lncRNA could specifically transcription in different environments and participate in different signaling pathways, thus leading to the differentiation of CD4+ T cells in the direction of promoting disease, through NEAT1 promoting of Th2 and Th17 cell differentiation in SLE and RA, respectively. GAS5 is unique in that its expression is downregulated in ITP but upregulated in AR. In diverse conditions, an lncRNA can be specifically transcribed or repressed, facilitating disease progression.
LncRNA Opa-interacting protein 5 antisense transcript 1 (OIP5-AS1) was increased in peripheral blood lymphocytes of MS patients. By targeting miR-140-5p, lncRNA OIP5-AS1 stimulates the RhoA/Rho kinase 2 (ROCK2) pathway, boosting Th17 subsets and accelerating EAE development in vivo. RhoA, a component of the Rho GTPase family, and its downstream factor ROCK2 are negatively modulated by miR-140-5p. Moreover, miR-140-5p inversely regulates the Th17 cell ratio and IL-17A expression, whereas OIP5-AS1 reverses this effect (Liu R. et al., 2021). The role of OIP5-AS1 in the development of MS may provide some candidate targets for the diagnosis and treatment of MS. LncRNA Gm15575 accumulates specifically in Th17 cells and spleen cells of mice with EAE, through sponging miR-686, Gm15575 promotes chemokine C-C motif chemokine ligand 7 (CCL7) expression (Bian et al., 2020). CCL7, a constituent of the chemokine C-C family, stimulates Th cells to produce cytokines and positively regulates Th17-cell differentiation; accordingly, the lncRNA Gm15575 works on the pathogenesis of EAE (Bian et al., 2020). Inflammatory bowel disease (IBD) is a chronic autoimmune disease in which the imbalance between Th17/Treg cells is one of the causes. Th17 cells promote tissue inflammation, and Treg cells inhibit autoimmunity in IBD (Yan et al., 2020). The lncRNA intersectin 1–2 (Lnc-ITSN1-2) is strongly expressed in the intestinal mucosa and PBMCs of patients with IBD and has been shown to actively regulate IL-23R expression by sponging miR-125a (Nie and Zhao, 2020). Previous studies have shown that IL-23R can regulate the Janus kinase (JAK)/STAT pathway (Floss et al., 2015; Hummel et al., 2017) and the proliferation and differentiation of Th1 and Th17 cells is enhanced by IL-23R through regulation of JAK2/STAT3 and nuclear factor (NF)-κB pathways (Nie and Zhao, 2020). Lnc-ITSN1-2 may provide a new perspective for the pathogenesis of IBD. Finally, lncRNA is important for Th cell function in both homeostasis and disease. LncRNAs can serve as guides for proteins to be recruited at specific DNA sites in both cis and trans. LncRNAs, on the other hand, may act as decoys, preventing proteins from contacting DNA. The diversity of lncRNAs functions determines that they may act on many network nodes of CD4+ T-cell differentiation, and they are present before, during and after transcription.
Knockdown of Lnc-DC, which exists only in conventional human dendritic cells (DCs), inhibits DC differentiation and decreases the capacity of DCs to activate T cells. Moreover, Lnc-DC can bind to STAT3 and activate transcription (Wang et al., 2014). Because STAT3 is an important transcription factor in the Th17 lineage, we hypothesized that lnc-DC may be involved in Th17-cell differentiation (Figure 2).
FIGURE 2. LncRNAs found in Th17 cells at various phases of development. LncRNAs influence Th17 cell development in a variety of ways. The lncRNA GAS5 inhibits the transcription factor STAT3 expression, as indicated by the dashed lines.
CD25 and Foxp3, a genealogy-specific transcription factor, are markers of Tregs. TGF-β drives the downstream transcription factors Smad2 and Smad3 to promote Foxp3 transcription (Sekiya et al., 2016). Additionally, IL-2 promotes Treg production through STAT5 and co-induces Foxp3 expression with TGF-β (Josefowicz et al., 2012). In addition, TGF-β also regulates the balance between Th17 cells and Tregs (Konkel et al., 2017; Liu et al., 2018).
The lncRNA Foxp3-specific lncRNA anticipatory of Tregs (Flatr), a member of the core Treg lncRNA transcriptome, is highly conserved and aggregated in sensitized Tregs. Flatr is expressed earlier than Foxp3, existing upstream of Foxp3, but depends on Foxp3 after its initial expression. In mice, defects in the Flatr gene result in minor Treg-induced damage. Accordingly, Flatr may have applications as a biomarker but does not appear to have a major functional role in mediating Foxp3 expression (Brajic et al., 2018). Oral lichen planus (OLP) is one of the common chronic inflammatory oral mucosal diseases, and Th17 response plays an important role in this disease (Monteiro et al., 2015). The lncRNA DQ786243 is expressed increasingly in peripheral blood CD4+ T cells of OLP patients and is positively correlated with Foxp3 expression. Although the number of Tregs in OLP patients is higher than in normal subjects, their activity appears to be reduced. Overexpression of DQ786243 was found to regulate miR-146a through Foxp3, miR-146a subsequently inhibits NF-κB signaling by blocking IL1R-associated kinase expression and tumor necrosis factor receptor-associated factor (TRAF) 6, increasing the proportion and inhibitory function of Tregs in patients with OLP (Wang et al., 2019b). LncRNA DQ786243 may provide a possible treatment pathway for OLP. Tregs not only regulate immune tolerance but also play vital roles in transplantation immunity. In the event of the rejection period, the expression of PVT1 is downregulated in Tregs and is inversely associated with miR-146a expression. In Tregs overexpressing PVT1, the expression of miR-146a is inhibited; this leads to upregulation of TRAF6, thereby enhancing the autophagy and inhibitory functions of Tregs and contributing to alleviation of transplant rejection (Lu et al., 2021). PVT1 may be a novel therapeutic strategy for transplant rejection. The lncRNA Foxp3 intergene noncoding RNA (Flicr) is located adjacent to Foxp3, expressed specifically in mature Tregs and negatively regulated Foxp3 expression. Flicr regulates Foxp3 expression by modifying chromatin accessibility at the conserved noncoding sequence 3/accessible region 5 region of Foxp3 (Zemmour et al., 2017). The influence of Flicr is especially evident when IL-2 is in deficiency. It can also be shown that IL-2 contributes to the phenotypic stability of Tregs (Zemmour et al., 2017). Therefore, Flicr impacts Treg differentiation by reducing Foxp3 expression and may therefore also reduce the phenotypic stability of Tregs. Like many lncRNAs, Flicr affects autoimmune diseases through subtle molecular mechanisms (Sawant and Vignali, 2014). LncRNA-Smad3 inhibits the differentiation of Tregs by mediating the expression of Smad3, the downstream signal transduction molecule of TGF-β. Notably, histone deacetylase 1 (HDAC1) combines with the promoter region of the Smad3 gene to silence Smad3 transcription. LncRNA-Smad3 inhibits the expression of Smad3 by recruiting HDAC1, thereby affecting Treg differentiation (Xia et al., 2017). As a result, subtle changes in lncRNA expression levels may alter the Treg gene network, which cause the shift to inflammatory cell differentiation. In other words, lncRNA has a wide range of control over the growth and function of CD4+ T cells. LncRNAs are structurally plastic and fold into complex structures to bind to DNA, RNA, and proteins. Furthermore, lncRNAs may have some advantages because they do not require protein translation and respond faster (Figure 3).
FIGURE 3. Treg differentiation is influenced by LncRNAs. Most lncRNAs operate as activators or inhibitors at different stages of Treg differentiation. The expression of lncRNA Flatr is dependent on Foxp3, but has no significant effect on Foxp3 expression.
TGF-β promotes the differentiation of naïve T cells into Tregs, whereas TGF-β induces the differentiation of Th17 cells when combined with pro-inflammatory factors such as IL-6 and IL-21. The dynamic balance of Tregs and Th17 cells is an important mechanism that functions to maintain immune homeostasis.
LncRNA maternally expressed gene 3 (MEG3) has been reported to be lost or reduced in many human tumors, and MEG3 changes in various cancers suggest that it has the possibility to diagnose and evaluate the prognosis of cancer (Al-Rugeebah et al., 2019). One lncRNA may play roles in Th17 divergence through absorbing distinct miRNAs in various disease models. LncRNA MEG3 is highly expressed in CD4+ T cells of ITP patients, resulting in an imbalance in the Treg/Th17 ratio through sponging miR-125a-5p; this then promotes Th17-cell differentiation and causes an immune imbalance in ITP patients (Li et al., 2016). MEG3 may be a target for restoring Th17/Treg immune balance (Wang et al., 2019a). Aplastic anemia (AA) is a life-threatening autoimmune disease, in which the main mechanism is hematopoietic tissue damage caused by T cell hyperfunctio (Wang et al., 2019a). The expression of MEG3 in CD4+ T cells in AA patients is much lower than in normal individuals, although the expression of miR-23a is significantly higher. Importantly, low MEG3 expression leads to excessive CD4+ T-cell differentiation into Th1 and Th17 cells, which significantly increases the levels of RORγt and T-bet and IL-17a and enhances the levels of IL-17a and IFN-γ, thereby exacerbating the symptoms of AA (Wang et al., 2019a). MEG3 overexpression reverses this effect by absorbing miR-23a (Wang et al., 2019a). LncRNA MEG3 also has some roles in asthma. The expression level of MEG3 in CD4+ T cells in peripheral blood of asthmatic patients increased, while the miR-17 level decreased. MEG3 upregulates RORγt through sponge miR-17, tilting Treg/Th17 balance toward Th17 in asthmatic patients (Qiu et al., 2019). MEG3 may also be a potential therapeutic target for immune-related diseases in the future.
Necrotizing enterocolitis (NEC) is a severe inflammatory bowel necrosis that occurs mainly in premature infants. The imbalance of Th17/Treg is increasingly associated with NEC (Gao et al., 2021). The lncRNA LINC01512 is downregulated in NEC patients, while melatonin upregulates LINC01512 through adenosine monophosphate-activated protein kinase (AMPK) signaling and interacts with sirtiun 1 (SIRT1), a regulator of various cellular and bodily processes. Furthermore, LINC01512 promotes Treg differentiation and inhibits Th17 differentiation by enhancing SIRT1 expression, thereby administering to correct the imbalance of Treg/Th17 ratio (Gao et al., 2021). The above melatonin-induced pathway may be a novel way to suppress inflammation.
Th17/Treg imbalance plays an important role in the pathogenesis of pneumonia (Chi et al., 2021). In children with pneumonia, GAS5 expression is downregulated, and the proportion of Th17 cells increases. Conversely, overexpression of GAS5 can correct the imbalance in Tregs/Th17 cells. Specifically, GAS5 reduces the binding of miR-217 with its target gene STAT5 by sponging miR-217, leading to upregulation of STAT5 expression and promoting the differentiation of Tregs (Chi et al., 2021). This mechanism may provide insights into the development of novel approaches for treating pediatric pneumonia.
The lncRNA MALAT1 is downregulated in the acute stage of EAE mice, similar to the trend observed for MALAT1 expression in the brain tissues of patients with MS. Low expression of MALAT1 leads to differentiation of CD4+ T cells into Th1 and Th17 cells, and increases the secretion of IFN-γ and IL-17, while reducing Treg differentiation (Masoumi et al., 2019). Although the molecular mechanism has not been reported, changes at the cellular level and cytokine level may also explain this phenomenon to some extent. LncRNA MALAT1 may be a potential target for future therapeutic interventions in inflammatory demyelination. (Figure 2; Figure 3).
Many immune diseases are caused by a Th17/Treg imbalance. Although the differentiation process of Th17 and Treg cells is irreversible, the phenotype of CD4+ T cells can be controlled. LncRNA analysis provides compelling evidence to explain the plasticity of CD4+ T-cell development from a molecular perspective, which aids in the discovery of new therapeutic targets for immune diseases.
Th9, as a class of newly discovered CD4+ Th cells, expresses the transcription factor PU.1 and the cytokine IL-9 (Chen et al., 2019). Although no studies have found that lncRNAs participate in the differentiation of this subgroup, IL-9 and the lncRNA Gm13568 have been shown to be correlated in MS and EAE (Liu X. et al., 2021). Therefore, lncRNAs may also be involved in Th9-cell differentiation, waiting for us to explore.
Tfh cells are also a relatively new Th cell type, and their differentiation mainly depends on the pedigree-defined transcription factor Bcl6 expression as well as the other important transcription factors Maf and STAT3 (STAT3 and STAT4 in humans) (Crotty, 2014; Vinuesa et al., 2016). One study demonstrated that the lncRNA MALAT1 is overexpressed in T cell subsets but is not a critical regulator of T cells; furthermore, MALAT1 has been shown to be nonessential for Tfh-cell differentiation (Yao et al., 2018). Although lncRNAs have not yet been shown to regulate Tfh-cell differentiation, relevant sequencing studies have indicated that many lncRNAs are relevant to Tfh pedigree transcription factors and cytokines (Deng et al., 2021; Zhang et al., 2021). Therefore, the roles and specific mechanisms of lncRNAs in Tfh-cell differentiation are waiting for further study.
Several RNA-based therapies have been developed, such as small interfering RNA (siRNA), antisense oligonucleotides (ASOs), and short hairpin RNA (shRNA). Currently, the US Food and Drug Administration and/or the European Medicines Agency have approved various RNA-based treatments. Fomivirsen was the first ASO to be approved to target the cytomegalovirus IE-2 mRNA (Stein and Castanotto, 2017). Patisiran was licensed in 2018 for the treatment of hereditary thyroxine amyloidosis, the first RNAi-based medication (Adams et al., 2018). In recent years, Givosiran, Viltolarsen, Inclisiran, and others have been approved (Winkle et al., 2021). Nedosiran for primary type 1 hyperoxaluria, primary type 2 hyperoxaluria, renal illness, and urinary disease, Alicaforsen for Crohn’s disease, and additional RNA treatments are in phase II or III clinical trials. However, no lncRNA-based therapy has been approved for use in humans. The variety of functions of lncRNAs indicates a variety of therapeutic targets. Natural antisense transcription (NAT), in which lncRNA is transcribed into coding genes in the antisense direction and negatively regulates them in the cis, is a promising path to pursue (Winkle et al., 2021). In the experimental stage, NAT has showed encouraging outcomes. “AntigoNATs” are ASOs that target NATs. In one investigation, upregulation of the SCN1A healthy allele using antagoNATs targeting SCN1ANAT alleviated Dravet syndrome (Hsiao et al., 2016). AntagoNATs were found to upregulate brain-derived neurotrophic factor, a protein linked to memory formation, in another study (Modarresi et al., 2012). These findings point to the potential of lncRNA-based treatments. Committing to researching the regulation mechanism of lncRNA may, to some extent, redefine the controller and regulator of Th-cell fate and provide a new direction for therapeutic intervention in immune diseases. Simultaneously, we must realize that genetic medications are difficult to administer precisely targeted and easy to provoke immune responses and other adverse effects, which is a severe challenge.
In conclusion, lncRNAs play an important role in CD4+ T-cell differentiation, revealing the complexities of CD4+ T-cell development and function at the molecular level. Because the specific molecular mechanism of some lncRNAs in the differentiation process of CD4+ T cells is unknown, we should continue to investigate their molecular mechanism and related undiscovered lncRNAs. Meanwhile, investigating the potential role of lncRNA in CD4+ T cells can help us better understand the role of the non-coding transcriptome in adaptive immunity. Thus, determining the pathways by which lncRNAs regulate CD4+ T-cell development and plasticity can help us better understand the immune response regulation mechanisms, as well as identify the causes of CD4+ T-cell functional alterations in illnesses and identify new therapeutic targets.
CL and YZ wrote the manuscript. ZM prepared the figure. HY supervised and revised the manuscript. All authors contributed to the article and approved the submitted version.
This research is supported by a grant from the Science and Technology Department of Jilin Province (20190201140JC).
The authors declare that the research was conducted in the absence of any commercial or financial relationships that could be construed as a potential conflict of interest.
All claims expressed in this article are solely those of the authors and do not necessarily represent those of their affiliated organizations, or those of the publisher, the editors and the reviewers. Any product that may be evaluated in this article, or claim that may be made by its manufacturer, is not guaranteed or endorsed by the publisher.
We would like to thank Editage (www.editage.cn) for English language editing.
Adams, D., Gonzalez-Duarte, A., O’Riordan, W. D., Yang, C.-C., Ueda, M., Kristen, A. V., et al. (2018). Patisiran, an RNAi Therapeutic, for Hereditary Transthyretin Amyloidosis. N. Engl. J. Med. 379 (1), 11–21. doi:10.1056/NEJMoa1716153
Akhade, V. S., Pal, D., and Kanduri, C. (2017). Long Noncoding RNA: Genome Organization and Mechanism of Action. Adv. Exp. Med. Biol. 1008, 47–74. doi:10.1007/978-981-10-5203-3_2
Al-Rugeebah, A., Alanazi, M., and Parine, N. R. (2019). MEG3: an Oncogenic Long Non-coding RNA in Different Cancers. Pathol. Oncol. Res. 25 (3), 859–874. doi:10.1007/s12253-019-00614-3
Bian, Z., Lei, W., Li, Q., Xue, W., Gao, Y., Zeng, Y., et al. (2020). Gm15575 Functions as a ceRNA to Up-Regulate CCL7 Expression through Sponging miR-686 in Th17 Cells. Mol. Immunol. 125, 32–42. doi:10.1016/j.molimm.2020.06.027
Braga-Neto, M. B., Gaballa, J. M., Bamidele, A. O., Sarmento, O. F., Svingen, P., Gonzalez, M., et al. (2020). Deregulation of Long Intergenic Non-coding RNAs in CD4+ T Cells of Lamina Propria in Crohn's Disease through Transcriptome Profiling. J. Crohns Colitis 14 (1), 96–109. doi:10.1093/ecco-jcc/jjz109
Brajic, A., Franckaert, D., Burton, O., Bornschein, S., Calvanese, A. L., Demeyer, S., et al. (2018). The Long Non-coding RNA Flatr Anticipates Foxp3 Expression in Regulatory T Cells. Front. Immunol. 9, 1989. doi:10.3389/fimmu.2018.01989
Cabili, M. N., Trapnell, C., Goff, L., Koziol, M., Tazon-Vega, B., Regev, A., et al. (2011). Integrative Annotation of Human Large Intergenic Noncoding RNAs Reveals Global Properties and Specific Subclasses. Genes Dev. 25 (18), 1915–1927. doi:10.1101/gad.17446611
Chakravarty, D., Sboner, A., Nair, S. S., Giannopoulou, E., Li, R., Hennig, S., et al. (2014). The Oestrogen Receptor Alpha-Regulated lncRNA NEAT1 Is a Critical Modulator of Prostate Cancer. Nat. Commun. 5, 5383. doi:10.1038/ncomms6383
Chan, W. F., Coughlan, H. D., Iannarella, N., Smyth, G. K., Johanson, T. M., Keenan, C. R., et al. (2021). Identification and Characterization of the Long Noncoding RNA Dreg1 as a Novel Regulator of Gata3. Immunol. Cell Biol. 99 (3), 323–332. doi:10.1111/imcb.12408
Chang, D., Xing, Q., Su, Y., Zhao, X., Xu, W., Wang, X., et al. (2020). The Conserved Non-coding Sequences CNS6 and CNS9 Control Cytokine-Induced Rorc Transcription during T Helper 17 Cell Differentiation. Immunity 53 (3), 614–626. e614. doi:10.1016/j.immuni.2020.07.012
Chen, J., Guan, L., Tang, L., Liu, S., Zhou, Y., Chen, C., et al. (2019). T Helper 9 Cells: A New Player in Immune-Related Diseases. DNA Cell Biol. 38 (10), 1040–1047. doi:10.1089/dna.2019.4729
Chen, X., Kong, J., Ma, Z., Gao, S., and Feng, X. (2015). Up Regulation of the Long Non-coding RNA NEAT1 Promotes Esophageal Squamous Cell Carcinoma Cell Progression and Correlates with Poor Prognosis. Am. J. Cancer Res. 5 (9), 2808–2815.
Chi, X., Guo, Y., Zhang, L., Zhang, J., Du, Y., Zhao, W., et al. (2021). Long Non-coding RNA GAS5 Regulates Th17/Treg Imbalance in Childhood Pneumonia by Targeting miR-217/STAT5. Cell. Immunol. 364, 104357. doi:10.1016/j.cellimm.2021.104357
Clemson, C. M., Hutchinson, J. N., Sara, S. A., Ensminger, A. W., Fox, A. H., Chess, A., et al. (2009). An Architectural Role for a Nuclear Noncoding RNA: NEAT1 RNA Is Essential for the Structure of Paraspeckles. Mol. Cell 33 (6), 717–726. doi:10.1016/j.molcel.2009.01.026
Collier, S. P., Collins, P. L., Williams, C. L., Boothby, M. R., and Aune, T. M. (2012). Cutting Edge: Influence of Tmevpg1, a Long Intergenic Noncoding RNA, on the Expression of Ifng by Th1 Cells. J. Immunol. 189 (5), 2084–2088. doi:10.4049/jimmunol.1200774
Collier, S. P., Henderson, M. A., Tossberg, J. T., and Aune, T. M. (2014). Regulation of the Th1 Genomic Locus fromIfngthroughTmevpg1by T-Bet. J. Immunol. 193 (8), 3959–3965. doi:10.4049/jimmunol.1401099
Crotty, S. (2019). T Follicular Helper Cell Biology: A Decade of Discovery and Diseases. Immunity 50 (5), 1132–1148. doi:10.1016/j.immuni.2019.04.011
Crotty, S. (2014). T Follicular Helper Cell Differentiation, Function, and Roles in Disease. Immunity 41 (4), 529–542. doi:10.1016/j.immuni.2014.10.004
Deng, L., Wang, P., Qu, Z., and Liu, N. (2021). The Construction and Analysis of ceRNA Network and Immune Infiltration in Kidney Renal Clear Cell Carcinoma. Front. Genet. 12, 667610. doi:10.3389/fgene.2021.667610
Floss, D. M., Schröder, J., Franke, M., and Scheller, J. (2015). Insights into IL-23 Biology: From Structure to Function. Cytokine & Growth Factor Rev. 26 (5), 569–578. doi:10.1016/j.cytogfr.2015.07.005
Fox, A. H., and Lamond, A. I. (2010). Paraspeckles. Cold Spring Harb. Perspect. Biol. 2 (7), a000687. doi:10.1101/cshperspect.a000687
Fox, R. I. (2005). Sjögren's Syndrome. Lancet 366 (9482), 321–331. doi:10.1016/s0140-6736(05)66990-5
Fu, J., Shi, H., Wang, B., Zhan, T., Shao, Y., Ye, L., et al. (2020). LncRNA PVT1 Links Myc to Glycolytic Metabolism upon CD4+ T Cell Activation and Sjögren's Syndrome-like Autoimmune Response. J. Autoimmun. 107, 102358. doi:10.1016/j.jaut.2019.102358
Gao, X., Liu, W., Gao, P., Li, S., Chen, Z., and Ma, F. (2021). Melatonin-induced lncRNA LINC01512 Prevents Treg/Th17 Imbalance by Promoting SIRT1 Expression in Necrotizing Enterocolitis. Int. Immunopharmacol. 96, 107787. doi:10.1016/j.intimp.2021.107787
Gao, Z.-Q., Wang, J.-f., Chen, D.-H., Ma, X.-S., Wu, Y., Tang, Z., et al. (2017). Long Non-coding RNA GAS5 Suppresses Pancreatic Cancer Metastasis through Modulating miR-32-5p/PTEN axis. Cell Biosci. 7, 66. doi:10.1186/s13578-017-0192-0
Gibbons, H. R., Shaginurova, G., Kim, L. C., Chapman, N., Spurlock, C. F., and Aune, T. M. (2018). Divergent lncRNA GATA3-AS1 Regulates GATA3 Transcription in T-Helper 2 Cells. Front. Immunol. 9, 2512. doi:10.3389/fimmu.2018.02512
Guo, C., Qi, Y., Qu, J., Gai, L., Shi, Y., and Yuan, C. (2020). Pathophysiological Functions of the lncRNA TUG1. Cpd 26 (6), 688–700. doi:10.2174/1381612826666191227154009
Guo, W., Lei, W., Yu, D., Ge, Y., Chen, Y., Xue, W., et al. (2017). Involvement of lncRNA-1700040D17Rik in Th17 Cell Differentiation and the Pathogenesis of EAE. Int. Immunopharmacol. 47, 141–149. doi:10.1016/j.intimp.2017.03.014
Hammad, H., and Lambrecht, B. N. (2021). The Basic Immunology of Asthma. Cell 184 (6), 1469–1485. doi:10.1016/j.cell.2021.02.016
Hsiao, J., Yuan, T. Y., Tsai, M. S., Lu, C. Y., Lin, Y. C., Lee, M. L., et al. (2016). Upregulation of Haploinsufficient Gene Expression in the Brain by Targeting a Long Non-coding RNA Improves Seizure Phenotype in a Model of Dravet Syndrome. EBioMedicine 9, 257–277. doi:10.1016/j.ebiom.2016.05.011
Hu, G., Tang, Q., Sharma, S., Yu, F., Escobar, T. M., Muljo, S. A., et al. (2013). Expression and Regulation of Intergenic Long Noncoding RNAs during T Cell Development and Differentiation. Nat. Immunol. 14 (11), 1190–1198. doi:10.1038/ni.2712
Huang, S., Dong, D., Zhang, Y., Chen, Z., Geng, J., and Zhao, Y. (2021). Long Non-coding RNA Nuclear Paraspeckle Assembly Transcript 1 Promotes Activation of T Helper 2 Cells via Inhibiting STAT6 Ubiquitination. Hum. Cell 34 (3), 800–807. doi:10.1007/s13577-021-00496-1
Hummel, T. M., Ackfeld, T., Schönberg, M., Ciupka, G., Schulz, F., Oberdoerster, A., et al. (2017). Synthetic Deletion of the Interleukin 23 Receptor (IL-23R) Stalk Region Led to Autonomous IL-23R Homodimerization and Activation. Mol. Cell Biol. 37 (17). doi:10.1128/mcb.00014-17
Ji, K.-Y., Jung, D. H., Pyun, B.-J., Kim, Y. J., Lee, J. Y., Choi, S., et al. (2021). Angelica gigas Extract Ameliorates Allergic Rhinitis in an Ovalbumin-Induced Mouse Model by Inhibiting Th2 Cell Activation. Phytomedicine 93, 153789. doi:10.1016/j.phymed.2021.153789
Jiang, Y., Zhao, Y., and Mo, X. (2021). Expression of lncRNA NEAT1 in Peripheral Blood Mononuclear Cells of Patients with Systemic Lupus Erythematosus and its Correlation with Th1/Th2 Balance. Int. J. Clin. Exp. Pathol. 14 (5), 646–652.
Jin, K., Wang, S., Zhang, Y., Xia, M., Mo, Y., Li, X., et al. (2019). Long Non-coding RNA PVT1 Interacts with MYC and its Downstream Molecules to Synergistically Promote Tumorigenesis. Cell. Mol. Life Sci. 76 (21), 4275–4289. doi:10.1007/s00018-019-03222-1
Josefowicz, S. Z., Lu, L.-F., and Rudensky, A. Y. (2012). Regulatory T Cells: Mechanisms of Differentiation and Function. Annu. Rev. Immunol. 30, 531–564. doi:10.1146/annurev.immunol.25.022106.141623
Koh, B. H., Hwang, S. S., Kim, J. Y., Lee, W., Kang, M.-J., Lee, C. G., et al. (2010). Th2 LCR Is Essential for Regulation of Th2 Cytokine Genes and for Pathogenesis of Allergic Asthma. Proc. Natl. Acad. Sci. U.S.A. 107 (23), 10614–10619. doi:10.1073/pnas.1005383107
Konkel, J. E., Zhang, D., Zanvit, P., Chia, C., Zangarle-Murray, T., Jin, W., et al. (2017). Transforming Growth Factor-β Signaling in Regulatory T Cells Controls T Helper-17 Cells and Tissue-specific Immune Responses. Immunity 46 (4), 660–674. doi:10.1016/j.immuni.2017.03.015
Kurebayashi, Y., Nagai, S., Ikejiri, A., Ohtani, M., Ichiyama, K., Baba, Y., et al. (2012). PI3K-Akt-mTORC1-S6K1/2 Axis Controls Th17 Differentiation by Regulating Gfi1 Expression and Nuclear Translocation of RORγ. Cell Rep. 1 (4), 360–373. doi:10.1016/j.celrep.2012.02.007
Li, J.-Q., Hu, S.-Y., Wang, Z.-Y., Lin, J., Jian, S., Dong, Y.-C., et al. (2016). Long Non-coding RNA MEG3 Inhibits microRNA-125a-5p Expression and Induces Immune Imbalance of Treg/Th17 in Immune Thrombocytopenic Purpura. Biomed. Pharmacother. 83, 905–911. doi:10.1016/j.biopha.2016.07.057
Li, J., Tian, J., Lu, J., Wang, Z., Ling, J., Wu, X., et al. (2020). LncRNA GAS5 Inhibits Th17 Differentiation and Alleviates Immune Thrombocytopenia via Promoting the Ubiquitination of STAT3. Int. Immunopharmacol. 80, 106127. doi:10.1016/j.intimp.2019.106127
Li, Z.-X., Zhu, Q.-N., Zhang, H.-B., Hu, Y., Wang, G., and Zhu, Y.-S. (2018). MALAT1: a Potential Biomarker in Cancer. Cancer Manag. Res. 10, 6757–6768. doi:10.2147/cmar.S169406
Liang, Z., and Tang, F. (2020). The Potency of lncRNA MALAT1/miR-155/CTLA4 axis in Altering Th1/Th2 Balance of Asthma. Biosci. Rep. 40 (2). doi:10.1042/bsr20190397
Liu, M., Li, S., and Li, M. O. (2018). TGF-β Control of Adaptive Immune Tolerance: A Break from Treg Cells. Bioessays 40 (11), 1800063. doi:10.1002/bies.201800063
Liu, R., Li, Y., Zhou, H., Wang, H., Liu, D., Wang, H., et al. (2021a). WITHDRAWN: OIP5-AS1 Facilitates Th17 Differentiation and EAE Severity by Targeting miR-140-5p to Regulate RhoA/ROCK2 Signaling Pathway. Life Sci. 2021, 119108. doi:10.1016/j.lfs.2021.119108
Liu, X., Zhou, F., Wang, W., Chen, G., Zhang, Q., Lv, R., et al. (2021b). IL-9-triggered lncRNA Gm13568 Regulates Notch1 in Astrocytes through Interaction with CBP/P300: Contribute to the Pathogenesis of Experimental Autoimmune Encephalomyelitis. J. Neuroinflammation 18 (1), 108. doi:10.1186/s12974-021-02156-5
Long, Y., Wang, X., Youmans, D. T., and Cech, T. R. (2017). How Do lncRNAs Regulate Transcription? Sci. Adv. 3 (9), eaao2110. doi:10.1126/sciadv.aao2110
Lu, J., Wang, X., Zhang, B., Li, P., Du, X., and Qi, F. (2021). The lncRNA PVT1 Regulates Autophagy in Regulatory T Cells to Suppress Heart Transplant Rejection in Mice by Targeting miR-146a. Cell. Immunol. 367, 104400. doi:10.1016/j.cellimm.2021.104400
Ma, Y., Liu, L., Yan, F., Wei, W., Deng, J., and Sun, J. (2016). Enhanced Expression of Long Non-coding RNA NEAT1 Is Associated with the Progression of Gastric Adenocarcinomas. World J. Surg. Onc 14 (1), 41. doi:10.1186/s12957-016-0799-3
Marder, W. (2019). Update on Pregnancy Complications in Systemic Lupus Erythematosus. Curr. Opin. Rheumatol. 31 (6), 650–658. doi:10.1097/bor.0000000000000651
Masoumi, F., Ghorbani, S., Talebi, F., Branton, W. G., Rajaei, S., Power, C., et al. (2019). Malat1 Long Noncoding RNA Regulates Inflammation and Leukocyte Differentiation in Experimental Autoimmune Encephalomyelitis. J. Neuroimmunol. 328, 50–59. doi:10.1016/j.jneuroim.2018.11.013
Modarresi, F., Faghihi, M. A., Lopez-Toledano, M. A., Fatemi, R. P., Magistri, M., Brothers, S. P., et al. (2012). Inhibition of Natural Antisense Transcripts In Vivo Results in Gene-specific Transcriptional Upregulation. Nat. Biotechnol. 30 (5), 453–459. doi:10.1038/nbt.2158
Monteiro, B. V. d. B., Pereira, J. d. S., Nonaka, C. F. W., Godoy, G. P., da Silveira, É. J. D., and Miguel, M. C. d. C. (2015). Immunoexpression of Th17-Related Cytokines in Oral Lichen Planus. Appl. Immunohistochem. Mol. Morphol. 23 (6), 409–415. doi:10.1097/pai.0000000000000096
Moser, T., Akgün, K., Proschmann, U., Sellner, J., and Ziemssen, T. (2020). The Role of TH17 Cells in Multiple Sclerosis: Therapeutic Implications. Autoimmun. Rev. 19 (10), 102647. doi:10.1016/j.autrev.2020.102647
Nie, J., and Zhao, Q. (2020). Lnc-ITSN1-2, Derived from RNA Sequencing, Correlates with Increased Disease Risk, Activity and Promotes CD4+ T Cell Activation, Proliferation and Th1/Th17 Cell Differentiation by Serving as a ceRNA for IL-23R via Sponging miR-125a in Inflammatory Bowel Disease. Front. Immunol. 11, 852. doi:10.3389/fimmu.2020.00852
Niogret, J., Berger, H., Rebe, C., Mary, R., Ballot, E., Truntzer, C., et al. (2021). Follicular Helper-T Cells Restore CD8+-dependent Antitumor Immunity and Anti-PD-l1/pd-1 Efficacy. J. Immunother. Cancer 9 (6), e002157. doi:10.1136/jitc-2020-002157
Qiu, Y.-y., Wu, Y., Lin, M.-j., Bian, T., Xiao, Y.-l., and Qin, C. (2019). LncRNA-MEG3 Functions as a Competing Endogenous RNA to Regulate Treg/Th17 Balance in Patients with Asthma by Targeting microRNA-17/RORγt. Biomed. Pharmacother. 111, 386–394. doi:10.1016/j.biopha.2018.12.080
Ranzani, V., Rossetti, G., Panzeri, I., Arrigoni, A., Bonnal, R. J. P., Curti, S., et al. (2015). The Long Intergenic Noncoding RNA Landscape of Human Lymphocytes Highlights the Regulation of T Cell Differentiation by Linc-MAF-4. Nat. Immunol. 16 (3), 318–325. doi:10.1038/ni.3093
Reuschel, E. L., Wang, J., Shivers, D. K., Muthumani, K., Weiner, D. B., Ma, Z., et al. (2015). REDD1 Is Essential for Optimal T Cell Proliferation and Survival. PLoS One 10 (8), e0136323. doi:10.1371/journal.pone.0136323
Saravia, J., Chapman, N. M., and Chi, H. (2019). Helper T Cell Differentiation. Cell Mol. Immunol. 16 (7), 634–643. doi:10.1038/s41423-019-0220-6
Sawant, D. V., and Vignali, D. A. A. (2014). Once a Treg, Always a Treg? Immunol. Rev. 259 (1), 173–191. doi:10.1111/imr.12173
Sehra, S., Yao, W., Nguyen, E. T., Glosson-Byers, N. L., Akhtar, N., Zhou, B., et al. (2015). TH9 Cells Are Required for Tissue Mast Cell Accumulation during Allergic Inflammation. J. Allergy Clin. Immunol. 136 (2), 433–440. e431. doi:10.1016/j.jaci.2015.01.021
Sekiya, T., Nakatsukasa, H., Lu, Q., and Yoshimura, A. (2016). Roles of Transcription Factors and Epigenetic Modifications in Differentiation and Maintenance of Regulatory T Cells. Microbes Infect. 18 (6), 378–386. doi:10.1016/j.micinf.2016.02.004
Shui, X., Chen, S., Lin, J., Kong, J., Zhou, C., and Wu, J. (2019). Knockdown of lncRNA NEAT1 Inhibits Th17/CD4+ T Cell Differentiation through Reducing the STAT3 Protein Level. J. Cell. Physiology 234 (12), 22477–22484. doi:10.1002/jcp.28811
Song, J., Wang, T., Chen, Y., and Cen, R. (2021). Long Non‐coding RNA Growth Arrest‐specific 5 and its Targets, microRNA‐21 and microRNA‐140, Are Potential Biomarkers of Allergic Rhinitis. Clin. Lab. Anal. 35 (10), e23938. doi:10.1002/jcla.23938
Spurlock, C. F., Tossberg, J. T., Guo, Y., Collier, S. P., Crooke, P. S., and Aune, T. M. (2015). Expression and Functions of Long Noncoding RNAs during Human T Helper Cell Differentiation. Nat. Commun. 6, 6932. doi:10.1038/ncomms7932
Stein, C. A., and Castanotto, D. (2017). FDA-approved Oligonucleotide Therapies in 2017. Mol. Ther. 25 (5), 1069–1075. doi:10.1016/j.ymthe.2017.03.023
Sun, H., Wang, T., Zhang, W., Dong, H., Gu, W., Huang, L., et al. (2021). LncRNATUG1 Facilitates Th2 Cell Differentiation by Targeting the miR-29c/B7-H3 Axis on Macrophages. Front. Immunol. 12, 631450. doi:10.3389/fimmu.2021.631450
Thomson, D. W., and Dinger, M. E. (2016). Endogenous microRNA Sponges: Evidence and Controversy. Nat. Rev. Genet. 17 (5), 272–283. doi:10.1038/nrg.2016.20
Vinuesa, C. G., Linterman, M. A., Yu, D., and MacLennan, I. C. M. (2016). Follicular Helper T Cells. Annu. Rev. Immunol. 34, 335–368. doi:10.1146/annurev-immunol-041015-055605
Wang, C., Yang, S.-H., Niu, N., Tao, J., Du, X.-C., Yang, J.-H., et al. (2021). lncRNA028466 Regulates Th1/Th2 Cytokine Expression and Associates with Echinococcus Granulosus Antigen P29 Immunity. Parasites Vectors 14 (1), 295. doi:10.1186/s13071-021-04795-2
Wang, J., Liu, X., Hao, C., Lu, Y., Duan, X., Liang, R., et al. (2019a). MEG3 Modulates TIGIT Expression and CD4 + T Cell Activation through Absorbing miR-23a. Mol. Cell Biochem. 454 (1-2), 67–76. doi:10.1007/s11010-018-3453-2
Wang, J., Zhai, X., Guo, J., Li, Y., Yang, Y., Wang, L., et al. (2019b). Long Non-coding RNA DQ786243 Modulates the Induction and Function of CD4+ Treg Cells through Foxp3-miR-146a-NF-Κb axis: Implications for Alleviating Oral Lichen Planus. Int. Immunopharmacol. 75, 105761. doi:10.1016/j.intimp.2019.105761
Wang, P., Xue, Y., Han, Y., Lin, L., Wu, C., Xu, S., et al. (2014). The STAT3-Binding Long Noncoding RNA Lnc-DC Controls Human Dendritic Cell Differentiation. Science 344 (6181), 310–313. doi:10.1126/science.1251456
Wei, Y., Han, B., Dai, W., Guo, S., Zhang, C., Zhao, L., et al. (2020). Exposure to Ozone Impacted Th1/Th2 Imbalance of CD4+ T Cells and Apoptosis of ASMCs Underlying Asthmatic Progression by Activating lncRNA PVT1-miR-15a-5p/miR-29c-3p Signaling. Aging 12 (24), 25229–25255. doi:10.18632/aging.104124
Winkle, M., El-Daly, S. M., Fabbri, M., and Calin, G. A. (2021). Noncoding RNA Therapeutics - Challenges and Potential Solutions. Nat. Rev. Drug Discov. 20 (8), 629–651. doi:10.1038/s41573-021-00219-z
Wu, G.-C., Hu, Y., Guan, S.-Y., Ye, D.-Q., and Pan, H.-F. (2019). Differential Plasma Expression Profiles of Long Non-coding RNAs Reveal Potential Biomarkers for Systemic Lupus Erythematosus. Biomolecules 9 (6), 206. doi:10.3390/biom9060206
Xia, M., Liu, J., Liu, S., Chen, K., Lin, H., Jiang, M., et al. (2017). Ash1l and Lnc-Smad3 Coordinate Smad3 Locus Accessibility to Modulate iTreg Polarization and T Cell Autoimmunity. Nat. Commun. 8, 15818. doi:10.1038/ncomms15818
Yan, J.-b., Luo, M.-m., Chen, Z.-y., and He, B.-h. (2020). The Function and Role of the Th17/Treg Cell Balance in Inflammatory Bowel Disease. J. Immunol. Res. 2020, 1–8. doi:10.1155/2020/8813558
Yang, X., Bam, M., Becker, W., Nagarkatti, P. S., and Nagarkatti, M. (2020). Long Noncoding RNA AW112010 Promotes the Differentiation of Inflammatory T Cells by Suppressing IL-10 Expression through Histone Demethylation. J. Immunol. 205 (4), 987–993. doi:10.4049/jimmunol.2000330
Yao, Y., Guo, W., Chen, J., Guo, P., Yu, G., Liu, J., et al. (2018). Long Noncoding RNA Malat1 Is Not Essential for T Cell Development and Response to LCMV Infection. RNA Biol. 15 (12), 1477–1486. doi:10.1080/15476286.2018.1551705
Zemmour, D., Pratama, A., Loughhead, S. M., Mathis, D., and Benoist, C. (2017). Flicr , a Long Noncoding RNA, Modulates Foxp3 Expression and Autoimmunity. Proc. Natl. Acad. Sci. U.S.A. 114 (17), E3472–e3480. doi:10.1073/pnas.1700946114
Zhang, F., Liu, G., Li, D., Wei, C., and Hao, J. (2018). DDIT4 and Associated lncDDIT4 Modulate Th17 Differentiation through the DDIT4/TSC/mTOR Pathway. J. Immunol. 200 (5), ji1601689–1626. doi:10.4049/jimmunol.1601689
Zhang, H., Zhu, X., Liu, X., Wang, Y., and Liu, Y. (2020). Long Non-coding RNA FOXD3-AS1 Regulates the Expression and Secretion of IL-25 in Nasal Epithelial Cells to Inhibit Th2 Type Immunoreaction in Allergic Rhinitis. Mol. Cell Biochem. 473 (1-2), 239–246. doi:10.1007/s11010-020-03825-4
Zhang, S. (2018). The Role of Transforming Growth Factorβin T Helper 17 Differentiation. Immunology 155 (1), 24–35. doi:10.1111/imm.12938
Zhang, Y., Zhang, Y., Gu, W., and Sun, B. (2014). TH1/TH2 Cell Differentiation and Molecular Signals. Adv. Exp. Med. Biol. 841, 15–44. doi:10.1007/978-94-017-9487-9_2
Zhang, Z., Tang, Y., Liu, Y., Zhuang, H., Lin, E., Xie, L., et al. (2021). A Novel Immune-Related lncRNA-Based Model for Survival Prediction in Clear Cell Renal Cell Carcinoma. J. Immunol. Res. 2021, 1–37. doi:10.1155/2021/9921466
Zhu, J. (2015). T Helper 2 (Th2) Cell Differentiation, Type 2 Innate Lymphoid Cell (ILC2) Development and Regulation of Interleukin-4 (IL-4) and IL-13 Production. Cytokine 75 (1), 14–24. doi:10.1016/j.cyto.2015.05.010
Zhu, X., Wang, X., Wang, Y., and Zhao, Y. (2020a). Exosomal Long Non-coding RNA GAS5 Suppresses Th1 Differentiation and Promotes Th2 Differentiation via Downregulating EZH2 and T-Bet in Allergic Rhinitis. Mol. Immunol. 118, 30–39. doi:10.1016/j.molimm.2019.11.009
Keywords: long noncoding RNA, plasticity, CD4+ T-cell differentiation, immune modulation, immune diseases
Citation: Liu C, Zhang Y, Ma Z and Yi H (2022) Long Noncoding RNAs as Orchestrators of CD4+ T-Cell Fate. Front. Cell Dev. Biol. 10:831215. doi: 10.3389/fcell.2022.831215
Received: 09 December 2021; Accepted: 13 May 2022;
Published: 20 June 2022.
Edited by:
Damjan Glavač, University of Ljubljana, SloveniaReviewed by:
Guangwei Liu, Beijing Normal University, ChinaCopyright © 2022 Liu, Zhang, Ma and Yi. This is an open-access article distributed under the terms of the Creative Commons Attribution License (CC BY). The use, distribution or reproduction in other forums is permitted, provided the original author(s) and the copyright owner(s) are credited and that the original publication in this journal is cited, in accordance with accepted academic practice. No use, distribution or reproduction is permitted which does not comply with these terms.
*Correspondence: Huanfa Yi, eWlodWFuZmFAamx1LmVkdS5jbg==
†These authors have contributed equally to this work and share first authorship
Disclaimer: All claims expressed in this article are solely those of the authors and do not necessarily represent those of their affiliated organizations, or those of the publisher, the editors and the reviewers. Any product that may be evaluated in this article or claim that may be made by its manufacturer is not guaranteed or endorsed by the publisher.
Research integrity at Frontiers
Learn more about the work of our research integrity team to safeguard the quality of each article we publish.