- 1Department of Ophthalmology, Otorhinolaryngology and Head and Neck Surgery, Ribeirao Preto Medical School, University of Sao Paulo, Ribeirao Preto, Brazil
- 2Department of Ophthalmology and Otorhinolaryngology, Faculty of Medical Sciences, State University of Campinas (Unicamp), Campinas, Brazil
Oxidative stress (OS) is a major disruption in the physiology of the lacrimal functional unit (LFU). Antioxidant enzymes have dual protective activities: antioxidant and antimicrobial activities. Peroxidases have been indistinctly used as markers of the secretory activity of the LFU and implicated in the pathophysiology, diagnosis and treatment of dry eye disease (DED), even though they comprise a large family of enzymes that includes lactoperoxidase (LPO) and glutathione peroxidase (GPO), among others. Assays to measure and correlate OS with other local LFU phenomena have methodological limitations. Studies implicate molecules and reactions involved in OS as markers of homeostasis, and other studies identify them as part of the physiopathology of diseases. Despite these conflicting concepts and observations, it is clear that OS is influential in the development of DED. Moreover, many antioxidant strategies have been proposed for its treatment, including calorie restriction to nutritional supplementation. This review offers a critical analysis of the biological mechanisms, diagnostic outcomes, drug use, dietary supplements, and life habits that implicate the influence of OS on DED.
Introduction
Oxidative stress (OS) takes part in both the protection and injury to the lacrimal functional unit (LFU) (Bron and Seal 1986; Stern et al., 2004; Davies et al., 2008; Uchino et al., 2012; Wakamatsu et al., 2013). It involves free radicals, which are atoms or molecules with a single unpaired electron composed of atoms of oxygen that react with other molecules donating or taking electrons and usually giving a considerable degree of reactivity to those molecules. The actions of these free radicals, named reactive oxygen species (ROS), are variable and influenced by several biological conditions (Petrides and Nauseef 2000; Droge 2002; Halliwell 2006).
At physiological concentrations, the ROS induces the appropriate level of OS required for a range of cellular processes, such as apoptosis, inflammation, innate immunity, and wound healing, as well as the regulation of transcription factors and their surface receptors (Halliwell 2006). However, excess ROS causes OS to oxidize DNA, protein, and other intracellular molecules. It also induces functional impairment, damage, or even the uncontrolled death of cells exposed to ROS, contributing to the mechanisms of several diseases, including eye diseases (Sies 1991; Halliwell and Chirico 1993; Halliwell 1995; Halliwell 2006; Ung et al., 2017).
The LFU is defined as a system that integrates the ocular surface, the central nervous system (CNS) and the exocrine glands responsible for the tear film. The ocular surface is composed of the cornea, the conjunctiva, and the lids that send sensorial neural inputs to the CNS, and throughout the autonomic nervous system, they provide feedback with stimuli to the exocrine glands, modulating the volume and content of the tear film that wets and nourishes the ocular surface. Among these exocrine glands are the lacrimal gland (LG), Meibomian glands (MG), accessory glands and the epithelial layer of the ocular surface, with their constitutive and regulated secretory and paracrine capacity (Bron and Seal 1986; Halliwell and Gutteridge 1990; Crouch et al., 1991; Droge 2002; Stern et al., 2004; Rocha et al., 2008).
OS is ubiquitous and has been implicated in the protective and pathogenic mechanisms of dry eye (DE) and ocular surface diseases. Therefore, any proposal of therapeutic modulation of those reactions must consider its dual effect (Morrison and Allen 1966; Fridovich 2013; Forman 2016).
As a protector, OS works against microorganisms and other sources of free radicals, maintaining homeostasis and triggering the response to protect against other harmful events that challenge the LFU. In a recent study on tear proteomics, the comparison between healthy and DE individuals revealed that lactoperoxidase (LPO) is the most downregulated enzyme in the DE group (Soria et al., 2017). In parallel, the increase in various proinflammatory proteins in their tears was confirmed in previous studies that used LPO as a biomarker of dysfunction for DE and LFU, as detailed below (Bromberg et al., 1989; Soria et al., 2017).
Throughout evolution, heme peroxidases, similar to myeloperoxidase (MPO), LPO, salivary peroxidase (SPO), and thyroid peroxidase (TPO), were found to be key enzymes for modulating the OS process in the cytoplasmic and secretory fluids (Davies et al., 2008). Antioxidants or free radical scavengers are incorporated into the innate immune arsenal, hormone synthesis and exocrine secretions, generating ROS with antimicrobial actions and counterbalancing the actions of other OS-related enzymes, including the glutathione peroxidases (GPx) family (Morrison and Allen 1966; Petrides and Nauseef 2000; Ihalin et al., 2006; Davies et al., 2008). Recently, a family of six protein isoforms called peroxiredoxins was described, and their structure and function associated with OS were further detailed (Rhee et al., 2012).
As a disease mediator, GPx levels and ROS accumulation have been identified as biomarkers and implicated in the protective mechanisms and damage triggered by the LFU (Wakamatsu et al., 2008). The expression and activity of these markers were associated with DE caused by hormone deficits, systemic autoimmune diseases, microelement deficiency, pollution, and ocular surface disease not necessarily related to systemic conditions (Bakker et al., 2005; Davies et al., 2008; Wakamatsu et al., 2008; Kawashima et al., 2010; Kojima et al., 2012; Uchino et al., 2012; Cejkova and Cejka 2015; Jung et al., 2018).
The present review aims to critically evaluate the apparent paradoxical protective and harmful aspects of ROS and the homeostatic or potentially therapeutic antioxidants associated with the ocular surface as well as LG protection and disease. Moreover, changes in the LFU related to OS, the reliability of the biomarkers, and the evidence supporting antioxidant therapies will be discussed herein.
The Chemistry of Endogenous and Exogenous Reactive Oxygen Species Formation
As previously observed, free radicals composed of oxygen are mainly formed by an electron transfer reaction through several pathways, with the main group of ROS being formed during natural biological processes in aerobic cells. The eight main ROS formation pathways are 1) the mitochondrial electron transport chain; 2) cytochrome P-450 metabolism; 3) the autoxidation of catecholamines; 4) the oxidation of reduced nicotinamide adenine dinucleotide phosphate (NADPH) by NADPH oxidase; 5) the oxidation of xanthine or hypoxanthine by xanthine oxidase; 6) the reduction of molecular oxygen by NO synthase (NOS) isoforms (nNOS or eNOS) under arginine or tetrahydrobiopterin deficiency; 7) peroxisome metabolism and 8) the oxidation of transition heavy metals (Cu+ and Fe2+) (Kruk et al., 2015; Gebicki 2016; Ung et al., 2017; Kruk et al., 2019).
The exogenous sources of ROS include physical factors (sunlight, ionizing radiation, and gamma irradiation), persistent exposure to environmental chemicals (cigarette smoke, xenobiotics, aromatic amines, ozone, and organochlorines), and pathogens (Sies 1991; Sies 2015).
The main exogenous source of ROS formation in the eye is UV radiation. One of the possible causes of ROS formation is by chromophore molecule activation, such as flavins, porphyrin, tryptophan, and melanin, during photochemical reaction processes. Chemically, these reactions involve the electronic excitation of a chromophore to the singlet state, with subsequent conversion to the long-term triplet state. Then, the molecule interacts with an O2 molecule to form 1O2, returning afterward to its ground state. UV exposure can also generate • 2° through a common electron transfer reaction (Kruk et al., 2015).
Historical Perspectives
The biological associations and medical implications of free radicals have drawn the attention of researchers since the 1950’s. They have been associated with the LG and LFU physiology and many diseases, including DE (Forman 2016; Dogru et al., 2018). Several enzymes linked to the redox process were identified in the LG and in tears, including the family of enzymes called catalases, superoxide dismutases and peroxidases.
Peroxidase is a general name for a large enzyme family that has been well preserved in the evolutionary process and is responsible for transforming hydrogen peroxide (H2O2) into water. By doing so, the levels of ROS are reduced in the cells. Data on peroxidase expression or activity in tears or in the LG as a marker of bioactivity of this exocrine gland have been published for more than 4 decades (Morrison and Allen 1966; Crouch et al., 1991) (Figure 1). It was immunologically detected in the LG of cows, and its expression and response to parasympathetic-like stimulation have also been observed in rats (Morrison and Allen 1966; Herzog and Miller 1972a; Herzog and Miller 1972b; Herzog et al., 1976).
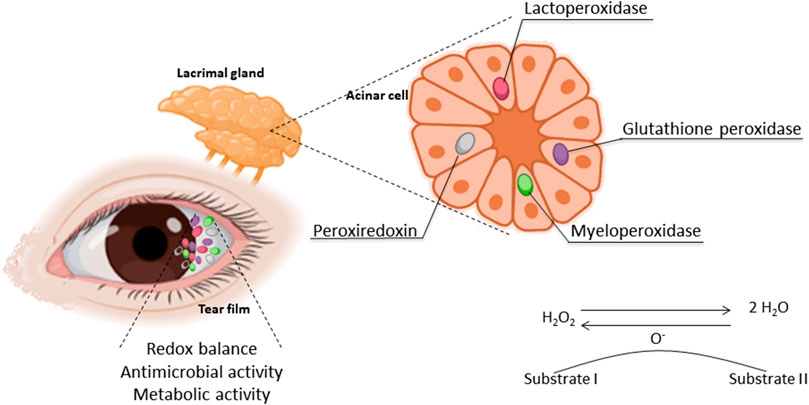
FIGURE 1. Oxidative stress in the LFU: the sources and area where the antioxidant enzymes take place and their activity.
Previous studies identified peroxidase granules in acinar cells to document the viability and maintenance of the secretory activity of those cells in culture conditions (Oliver et al., 1987). Aging has also been observed as a factor in the lowering of LG peroxidase secretion and activity in rats and mice (Bromberg and Welch 1985; Alves et al., 2005b; Rios et al., 2005). These observations made peroxidase activity a widely applied marker of LG bioactivity, not only in aging studies but also in other models investigating DE mechanisms, as detailed in the next section.
Earlier studies provided the opportunity to identify a marker of the structure and function of acinar cells and trace the secretory activity of those exocrine cells, measuring peroxidase in the tear film (Draper et al., 1999; Draper et al., 2003). However, the interpretation of these findings can be questioned. The studies assumed to measure secretory activity, and therefore the enzyme LPO, based on enzymatic assays of peroxidase activity in the tissues or secretion.
LPO is an antioxidant enzyme and member of the heme peroxidase superfamily II or the MPO family. This enzyme is constitutively secreted, which means it is present as a default component and is not determined by external demand or regulated processes. Its secretion occurs not only in tears but also in saliva, milk, and vaginal fluid. It is therefore considered to have a similar origin and structure as salivary peroxidase (SPO) (De et al., 1987; Ihalin et al., 2006). It oxidizes thiol from thiyl free radicals and participates in the host’s defense against bacteria.
Other enzymes associated with antimicrobial, antioxidant, and chemical scavenger functions were found in the LG, tear secretion, and the ocular surface. These include peroxidase isoforms, lactoferrin, and lysozyme, and their expression has been associated with age-related diseases (McGill et al., 1984; De et al., 1987; Gogia et al., 1998; Higuchi et al., 2012; Soria et al., 2017).
Studies performed in 1972 and 1991 failed to identify catalase in the tear film (Herzog and Miller 1972b; Crouch et al., 1991). However, enzymatic investigations using samples of the LG showed that those samples were capable of catalyzing the free radical hydrogen peroxide (H2O2) into water and oxygen in vitro after blocking peroxidase activity. This confirms the presence of catalase in the LG, assuming that it is a constitutive enzyme of this gland but not a substantial part of the secretory elements of the tear film (Herzog and Fahimi 1976). Recently, the presence of catalase was confirmed in the tear film by sensitive immunoassay analysis (Jee et al., 2014). Thus, it is possible to state that catalase is present in the LG, located in the peroxisomes in the cytoplasm, and that it is present in the tear film.
The presence of other soluble peroxidases in the LG and the ocular surface, including GPX, were noted to be abundant and have been described since 1996 (De 1992; Mazumdar et al., 1996).
Superoxide dismutase (SOD) is another family of enzymes capable of O2− dismutation. It is a soluble protein present in the cytosol and nuclei of probably all mammalian cells (McCord and Fridovich 1969; Crapo et al., 1992). Mice with knocked out SOD1 show lacrimal glands that are smaller, inflamed and aging faster. These are associated with deposits of oxidized material in the LG, reduced tear production, secretory vesicles jam in the acinar cells, and keratitis. All manifestations are accentuated with aging (Kojima et al., 2012).
Throughout recent years, other enzymes and hormones have been identified as prompting antioxidant effects, although their mechanisms are not completely clear. Among them are serine protease inhibitor A3K (SERPINA3K) and erythropoietin (EPO), which is suspected to be produced by the LG and has been proven to be protective against corneal epithelial ROS by activating antioxidant enzymes (Gerina 1980; Zhou et al., 2012; Rocha et al., 2013; Lin et al., 2014).
Peroxiredoxin (PRDX) is a family of six antioxidant enzymes expressed in eye tissues, including the ocular surface epithelial basal layers (the cornea, conjunctiva and the limbus). In comparison to healthy subjects (PRDX1, 2, and 5), it has been more frequently expressed in inflammatory conditions of the ocular surface and in the tear film associated with DE, as shown by pterygium biopsies (peroxiredoxin 1 and 2) (Acera et al., 2011; Soria et al., 2013; Jee et al., 2014; Klebe et al., 2014).
Recently, 481 proteins were identified in human tear film. Among them, 18 are agents that protect against OS, including GPX3, and 7 are related to immune defense (Table 1) (de Souza et al., 2006).
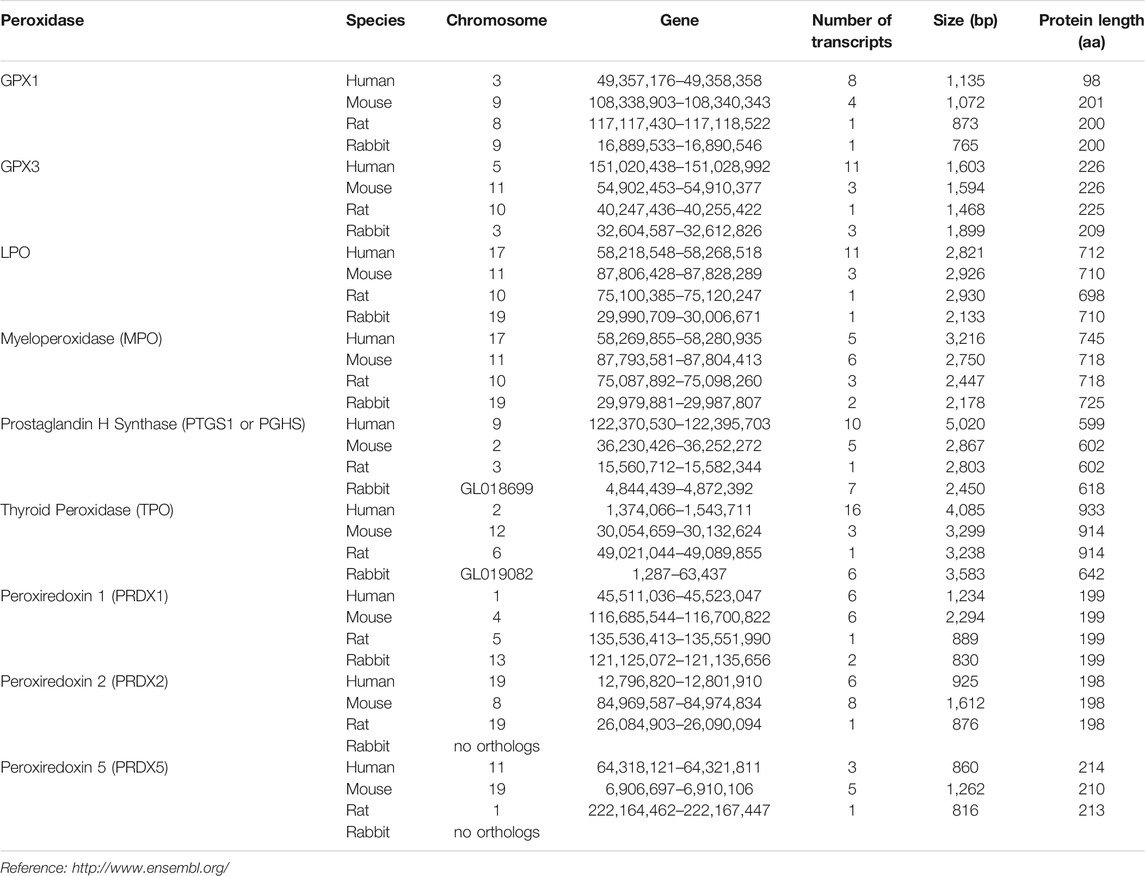
TABLE 1. Genetic source and comparison of antioxidants and free radical scavengers identified in the lacrimal gland and ocular surface cells or tear secretion of human, mouse, rabbit or rat (Flicek 2020).
All these findings lead to two paradoxes: 1) OS is present in the ocular surface simultaneously for protection and harm; 2) increase in the OS biomarker indicates health and healing capacity or tissue degradation and the lack of organ homeostasis.
To deeply understand these paradoxes, it is important to evaluate the biomedical literature in the following topics: 1) available peroxidase assays and limitations; 2) clinical evidence of a relationship between ROS and disease; and 3) potential therapeutic approaches.
Limitations of Each Antioxidant or Oxidative Stress Assay in the Lacrimal Gland, Ocular Surface, and Tear Film
Under physiological conditions, oxidant-antioxidant balance or redox homeostasis is equalized with minimum rest (Droge 2002). Signs of waste can be observed when comparing biologic markers of oxidative stress in aged versus young organisms, as well as on the ocular surface (Batista et al., 2012; Cejka and Cejkova 2015; Forman 2016).
Limitations in tracking the events associated with healthy and pathologic OS using biologic and chemical in vitro and in vivo assays are rarely mentioned. Approximately 0.1% of the O2 reduced during normal cell metabolism generates free radicals. Meanwhile, 1–3% of all electrons in the transport chain “leak” to generate superoxide and contribute to pathologic events instead of contributing to the transformation of oxygen into water (Imlay and Fridovich 1991; Valko et al., 2007).
The limitations begin in the fact that those molecules and their free radical metabolites, including superoxide (O−), nitric oxide (NO), hydrogen peroxide (H2O2), and hydroxyl (OH−), are labile. Therefore, measurement in live tissues, although feasible, is not easy or free from artifacts (McCord 1974; Looms et al., 2002; Hodges et al., 2005).
Currently, the main source of OS and the presence of antioxidants or free radical scavenger enzymes are tested or confirmed in tissues and fluids in different ways, with different specificities (Table 2).
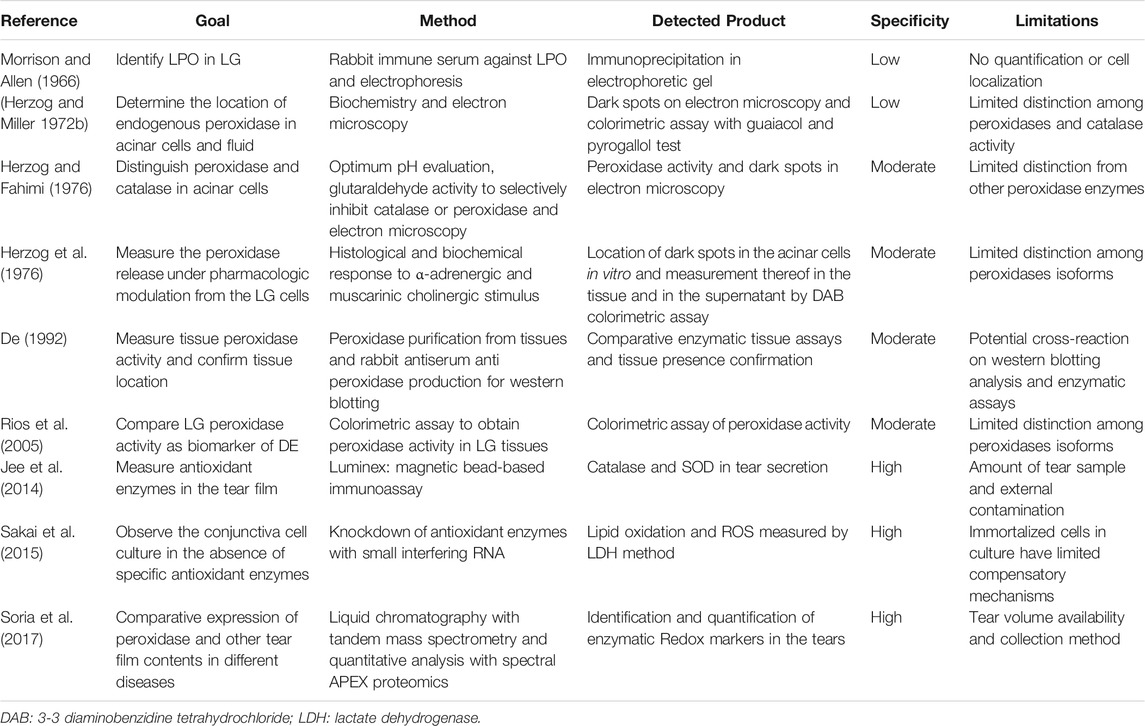
TABLE 2. Assays and their limitations for OS, antioxidant or free radical scavenger expression or activity in the LG, tear film or the ocular surface.
Considering their association with many diseases, a large number of studies have investigated the presence and activity of antioxidant enzymes, the presence of their mRNA and deposits or their residual metabolites of OS reactions as indirect biomarkers of diseases (Marcozzi et al., 2003; Atli et al., 2004; Nogueira et al., 2005; Adachi et al., 2006; Kurimoto et al., 2007; Jorge et al., 2009; Deconte et al., 2011; Knas et al., 2016; Zhang et al., 2017; Seara et al., 2018).
The major concerns are related to the generalization of damage/activity based on biomarker detection and to the nomenclature of the enzymes. “Peroxidase” and “peroxide activity” are terms that have been used disregarding their isoforms/families based on genetic and structural types, specific roles, radical substrates, and cell type expression (Table 2). MPO, LPO and salivary peroxidase belong to the heme peroxidase family. GPO, also expressed in the LG, is part of a different group in terms of both structure and function (Davies et al., 2008; Zamocky et al., 2008; Andrade et al., 2014). Their potential roles in pathologic processes and cross-reactivity in enzymatic assays have not been consistently addressed in current studies (Mansson-Rahemtulla et al., 1988; Thomas et al., 1994; Modulo et al., 2009; Rahimy et al., 2010). A better understanding of their specific roles in healthy conditions and in diseases may help to clarify the paradox of the protective and harmful actions of OS and peroxidases.
Several studies have observed ROS and free radical scavengers limited to the LG, ocular surface tissues and/or tear film. Others did so in association with systemic or environmental diseases as part of the homeostatic, defensive, or pathological mechanisms. We summarized the types of tests in studies involving the LG, ocular surface tissues, and tear film and compared the effects of OS, the amount of metabolites generated, and antioxidant enzyme expression and activity (Table 2). The correlation between the amount of the antioxidant expression at the level of mRNA, protein, their activity in vitro, or target tissues, and the OS reaction assumed to take part in the pathological models of diseases that are commonly thought to exist. However, they are rarely demonstrated.
Table 2 presents correlations whenever adequately tested, which would help to clarify the second paradox related to the OS response capacity based on the stocks of the specific enzyme in the tested condition/model.
Biological Evidence of Reactive Oxygen Species in the Lacrimal Gland and Ocular Surface in Health and Disease Conditions
The LFU includes the LG, ocular surface components and tear film, and it is constantly exposed to triggers of OS. The list includes but is not limited to light, glucose and nitrogen metabolites, high oxygen pressure, pathogens and toxic particles carried in the air or in ocular medications (Brignole-Baudouin et al., 2011b; Torricelli et al., 2011; Alves et al., 2014; Cejkova and Cejka 2015; Lee et al., 2016).
As described above, tissues contain several antioxidants and free radical scavengers, which may control the levels of ROS formation and prevent OS from occurring. Better studied models are those in which LPO presents antimicrobial activity in the tear film as a constitutive enzyme. Additionally, those describing the association between the production of ROS and antioxidant enzyme impairment, diseases associated with DED (such as Sjögren’s syndrome), aging, and hormone and metabolic disturbances such as diabetes mellitus (DM) and hypothyroidism.
LPO antimicrobial activity involves disturbing the stability of bacterial wall proteins, inhibiting microbial respiratory enzymes and interrupting the DNA and RNA synthesis processes of pathogenic microorganisms (Zamocky et al., 2008). These events are more effective against Gram-negative and anaerobic bacteria but also have antifungal and antiviral activities (Carlsson et al., 1983; Yamamoto et al., 1991; Chase and Klebanoff 1992; Lenander-Lumikari 1992; Soukka et al., 1992; Ihalin et al., 2006). LPO transforms ROS into metabolites capable of combating pathogenic agents that interfere with signaling mechanisms related to growth and apoptosis through the PI3K and MAPK pathways (Everse et al., 1991; Daiyasu and Toh 2000; Groeger et al., 2009).
These events are intimately related to LG function and ocular surface protection. However, as a constitutive enzyme of the tear film and potential marker of healthy tear secretion and function, there is a lack of understanding of the role of peroxidases in antimicrobial function versus antioxidant metabolic activities in the LFU. This is due to limitations on bioassays conducted in several studies in the past (listed in Table 2), involving cross-reaction and misleading interpretations, as observed before with other biological fluids (Bonini et al., 2007).
The LPO levels in tears decrease with aging in mice and rats (Bromberg and Welch 1985; Bromberg et al., 1989; Rios et al., 2005). Although it is undisputable that aging deteriorates the LFU, antioxidant and innate defenses while ROS accumulates in the tissues, the assays did not specifically investigate enzyme activity for either purpose.
The LG shows higher peroxidase activity in scavenging H2O2 than pancreatic islets of Langerhans in rats. This suggests that the LG has a better system to respond to oxidative stress and therefore better resistance to toxic and inflammatory agents such as streptozotocin (Cunha et al., 2007).
Regarding the LG in a diabetic rat model, our previous studies revealed that peroxidase activity is higher in the LG tissue of rats with DM induced by streptozotocin than in the LG tissue of the control rats and the insulin- or aspirin-treated DM rats matched for age and sex (Jorge et al., 2009; Modulo et al., 2009). These findings suggest that GPO, a housekeeping enzyme, but not LPO, a secretory enzyme, is responsive to hyperglycemic oxidative damage. However, the presence of higher amounts of lipid peroxidation markers, such as lipofuscin and malondialdehyde (MDA), which are late-phase markers of lipid peroxidation, reveals that higher peroxidase activity is not sufficient to prevent OS.
Not only type 1 DM but also insulin resistance induced by a high-fat diet in rats increased the levels of ROS in salivary glands, parallel to a reduced salivary flow rate. This finding indicated that the parotid gland is the most vulnerable to OS (Knas et al., 2016; Kolodziej et al., 2017).
Moreover, the peroxidase release from the LG of diabetic rats (measured by a method intended to identify LPO) indicated a lower level of LPO in the LG and in the basal region and stimulated tear secretion from those who removed the LG and had fewer secretory granules ex vivo (Shetty et al., 2009). Those studies showed discrepant results on peroxidase activity in the same animal model of DM, where higher OS and higher peroxidase activity are not associated with higher LPO secretion. These findings reveal the need to distinguish the metabolic response to ROS and the effect on secretory activity concerning different peroxidase enzymes and activity in the LG. In DM, the former (high OS) was likely observed in events predominantly associated with GPx versus ROS, and the latter (weaker LG secretory activity) was observed in those events associated with impaired secretory activity related to LPO.
We compared the expression of LPO, GPx and LF proteins by western blotting in the LG of healthy and diabetic rats (after 8 weeks of streptozotocin induction). No significant differences were detected in their expression, which suggests that the expression of those enzymes was not severely affected by DM. Alternatively, they were cross-reacting and hindering the identification of changes in each of those enzymes (data not shown) (De 1992). A better distinction among various antioxidant enzyme expression and activity will be useful to clarify those discrepancies.
A higher activity of GPx in the parotid gland compared to the submandibular gland of individuals with DM was also associated with higher levels of OS (Nogueira et al., 2005; Kolodziej et al., 2017). Recently, additional evidence of the association between DM and DE involving OS was described in high-fat diet-induced DM in mice, where reductions in tear flow and corneal epithelial thickness were observed. In parallel, after 8 weeks, there was an initial increase followed by subsequent declining levels of the following antioxidants in the corneas of diabetic mice with DE: silent information regulator 1 (SIRT1), fork head Box O 3 (FOXO 3), and Mn superoxide dismutase (MnSOD). This suggests that these molecules act in a particular pathway in response to OS related to DE in the cornea (Liu et al., 2015). In human corneal epithelial culture cells and rat corneal cells in vivo challenged with H2O2, the higher expression of catalase and SOD induced by SERPINA3K minimized the OS damage to those epithelial cells (Zhou et al., 2012).
In another DE model induced by hormone impairment (hypothyroidism induced by thiamazole in rats), lipid peroxidation was detected in ocular surface tissues and tear film (Drozhzhyna G.I. & G.I. 2019). This finding agreed with previous work in which male rats with hypothyroidism presented lower tear secretion, lower peroxidase activity, and increased levels of malondialdehyde in the LG (Dias et al., 2007). Both studies also confirm the associated repercussions in the tissues integrated by the LFU.
Considering the sex differences in the LFU in health and disease, the possible modulation of ROS by sex hormones and their implications on DE, it is interesting to observe that the LG of a female hamster has three times more peroxidase activity than the male LG. Further studies revealed that it could be suppressed by androgen (Paliwal and De 2006; Dowling and Simmons 2009; Sullivan et al., 2017). To confirm these findings in rats, we compared the activity of peroxidase between the LG homogenates in the male and female rat by a colorimetric test, but no difference was found (Table 3). To investigate whether peroxidase enzyme levels would change and could overlap with the activity of the other, we used western blotting and specific antibodies (Santa Cruz Biotechnologies, Santa Cruz, CA, United States) to compare the expression of three enzymes involved in OS in the LFU, GPx3, LPO, and LF. All of them presented similar levels in the LG of 8-week old female and male Wistar rats (data not shown). Thus, these results limit our understanding of the role of sex hormones in oxidative stress enzymes in DE and LFU diseases.

TABLE 3. Peroxidase activity in Wistar rats LG. The comparison included eight-week-old males and females (n = 7/group). The assay was conducted with a colorimetric assay (Amplex Red; Molecular Probes, Eugene, OR, United States) and read at a spectrophotometer (SpectroMax M2, Molecular Devices, Sunnyvale, CA, United States) after 30 min. Comparisons were performed by Student’s t test for the two groups and ANOVA for the three groups. The p value was significant when <0.05.
Nonobese diabetic (NOD) mice have been used in studies to understand DM and Sjögren’s syndrome-related DE (SSDE). Their characteristics reveal that females are more likely to develop DM and males are more likely to develop LG but not salivary gland (SG) inflammation (Toda et al., 1999; de Paiva and Rocha 2015). The possible role of antioxidant enzymes was not explored in the LG of NOD mice. However, the expression of antioxidant enzymes (including SOD, catalase and GPx) in various tissues, such as lung and pancreatic beta cells, is higher in males. This suggests that they have better protection against pancreatic destruction (Cornelius et al., 1993). Moreover, transgenic overexpression of thioredoxin antioxidant enzyme in pancreatic beta cells prevented the onset of DM in NOD mice (Yamamoto et al., 2008). Whether sex differences in SSDE NOD mice have any relationship with OS is still unknown.
The commensal microbiota has a protective and anti-inflammatory effect on the ocular surface and the LG compared to germ-free conditions in wild-type C57BL/6J and CD25 knockout mice, which is a model for SSDE (Wang et al., 2018; Zaheer et al., 2018). The mechanisms of action of the gut microflora in such events are not clearly known. Only five key communication routes are described as hypotheses that relate ocular surface diseases and gut dysbiosis: myeloid cell migration (altered migration of dendritic cells or macrophages to the lymph nodes, ocular surface and the lacrimal gland to prime T cells or promote pro-inflammatory cytokines secretion); effector lymphocyte imprint (Treg/TH17 imbalance in ocular surface and LG); molecular mimicry (B cells autoantibodies production mediated by microbial-derived antigens); metabolite circulation (the reduction of short-chain fatty acids microbial metabolites, which affect the tear secretion) and neuropeptide circulation (gut-derived neuropeptides influence tear secretion). However, as mentioned above, mechanical investigations are necessary to further elucidate these five key communication routes and their potential overlap (Thaiss et al., 2016; Moon et al., 2020). The effect of the microbiota inducing a balanced level of OS to modulate the innate immune system and promote homeostasis in the other organs and systems is considered part of the protection mechanism. Indeed, dysbiosis is a deviation in the communication of the gut-brain axis that induces exaggerated inflammation and OS (Luca et al., 2019).
The best and most long-standing agreement among studies regarding the LFU and OS is seen in aging models. The LG of old mice and rats present lower peroxidase activity and lower stimulated peroxidase secretion than their younger counterparts (8 weeks old) (Bromberg et al., 1989; Alves et al., 2005b; Rios et al., 2005). These observations are also in agreement with functional impairment of secretion, reduction of molecules associated with secretory control such as Rab3d, accumulation of degenerative and oxidative stress markers such as lipofuscin, and reduction of vitamin E (an antioxidant) in the LG of aged rats (Batista et al., 2012). Functional changes were prevented in aging rats with 35% calorie restriction (CR), which also preserved the tissue structure, reduced inflammatory cell infiltration, and attenuated the markers of oxidative damage to the LG (Kawashima et al., 2010). In autoimmune mouse models, 40% CR attenuated the inflammatory process in the SG, extended their lifespan, and, in healthy primates, delayed degenerative diseases (Chandrasekar et al., 1995; Colman et al., 2009). The mechanism is attributed to OS reduction in metabolic and inflammatory processes (Heilbronn and Ravussin 2003). In SOD knockout mice, functional impairment and increases in several degenerative parameters are associated with aging. This included OS and inflammatory markers in the LG and tear film, manifested as early as 50 weeks of age, indicating that antioxidant enzymes and OS have a key role in the causative events related to age-related DE (Kojima et al., 2012). Observations that ROS trigger DE pathology were also identified in a mev-1 conditional transgenic mouse (Tet-mev-1), in which mitochondrial oxidative damage led to DE through LG damage (Uchino et al., 2012). Those last observations indicating OS as a causative factor are relevant to the understanding of DE physiopathology, avoiding the chicken and egg dilemma or the belief that ROS are only a side effect of other causes of DE. These findings could support the goal of therapeutic strategies focused on antioxidant and OS prevention.
Meanwhile, OS can be considered an adjuvant factor in the mechanism of DE and ocular surface diseases. Although studies identified the suppression of antioxidant activities as triggers of DE, the action of genetic knockout enzymes may produce other nontracked effects associated with it. Moreover, all other models, including DM, hormone deficiency, and aging, present several competing factors, such as impaired neural transmission and metabolism, hypotrophism, and osmotic imbalance (Rocha et al., 2003; Alves et al., 2005a; Alves et al., 2005b; Kojima et al., 2012; Uchino et al., 2012; Dias et al., 2015). Therefore, it is unlikely that OS induced by a deficiency in antioxidant enzymes in the LFU is the only cause of DE in major conditions such as aging, DM, and hormone deficiency. It is more plausible that OS is an adjuvant factor.
Clinical Evidence of Reactive Oxygen Species as a Cause of Dry Eye
Sex differences in LG and DE diseases, with a higher prevalence in females, are remarkable characteristics that are well reviewed (Sullivan et al., 2017). The investigation of OS in the female sex revealed that peroxidase activity in the tear film is enhanced in response to serum levels of estrogen, and it is also attenuated by oral contraceptives (Liberati et al., 2002). Moreover, peroxidase activity in tears is similar between young men and women and diminishes at a later age in men compared to women, being therefore higher in middle-aged men compared to menopausal women (Marcozzi et al., 2003). Of equal importance, several environmental and behavioral factors may influence sexual comparative analyses of the antioxidant capacity of tears, namely, smoking habits and exercise frequency (Gus et al., 2006).
Sjögren’s Syndrome (SS) is a systemic autoimmune disease more prevalent in women, with an unknown cause and no cure, that causes DE and dry mouth due to the inflammation of exocrine glands (Akpek et al., 2011). Major biomarkers of SS are autoantibodies that protect against intracellular ribosomal proteins, including anti-Ro52/60, also called SSa (Vitali et al., 2002). It was demonstrated that Ro52/SSa is a signaling molecule sensitive to H2O2 that is capable of translocating from the cytoplasm to the nucleus in the MAP kinase (MAPK) pathway (Nobuhara et al., 2007). Other studies associate the autoantibodies of SS (SSa and SSb) with cross-reactivity of viral proteins. There is plausibility for the hypothesis that excessive OS and overexpression of signaling molecules such as MAPK lead to cytotoxicity and inflammation. Subsequent cytolysis induces exposure and immune sensitization to intracellular molecules and the production of autoantibodies as part of the complex mechanism in the target tissues of SS disease (e.g., exocrine glands) (Figure 2) (Baboonian et al., 1989; Scofield and Harley 1991; Garcia-Carrasco et al., 2006).
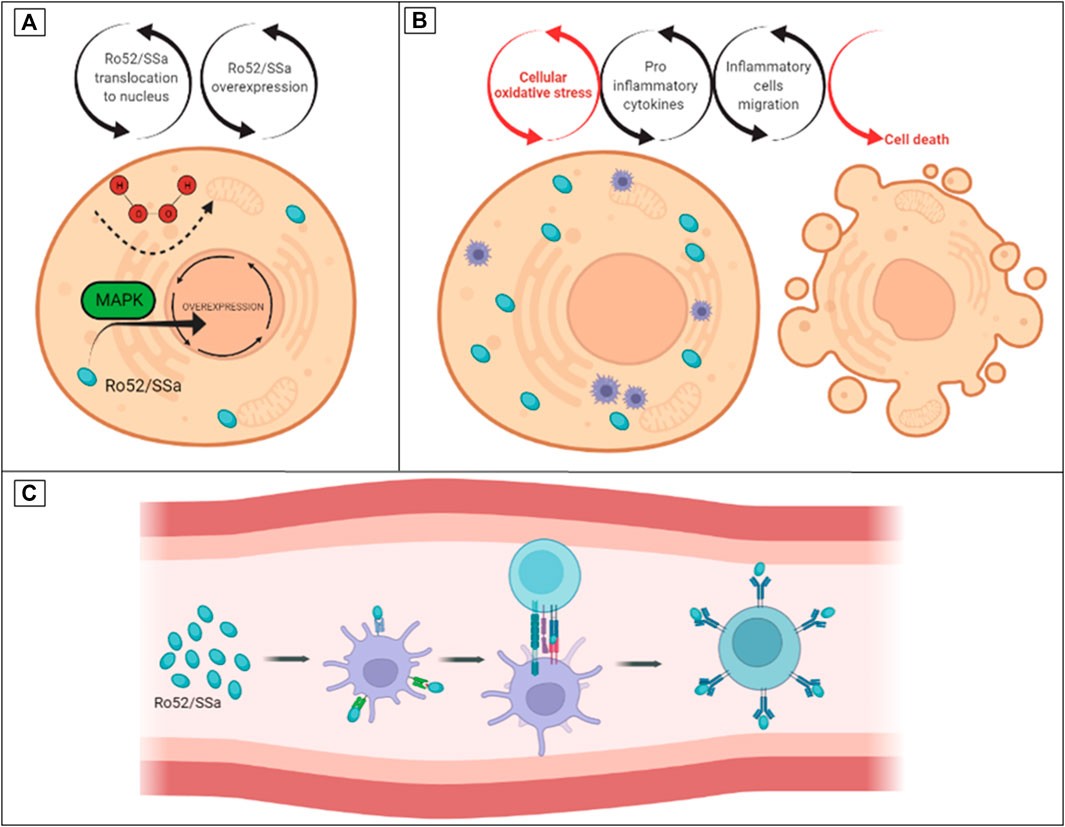
FIGURE 2. The hypothesis of turning Ro52/SSa from an oxidative stress intracellular signaling molecule to an auto antigen in SS. (A) Ro52/SSa translocation to the nucleus by H2O2 stimuli. (B) Overexpression of Ro52/SSa led to cytotoxicity and inflammation. (C) Immune sensitization and production of anti-Ro autoantibodies.
Markers of OS, such as 8-hydroxy-2′-deoxyguanosine (8-OHdG) and hex-anoyl-lysine (HEL), present higher levels in the saliva of SS individuals than in other salivary dysfunctions (Ryo et al., 2006). These observations were later confirmed and accompanied by higher levels of the antioxidant thioredoxin in the saliva of SS individuals compared to the control group (Kurimoto et al., 2007). In systemic sclerosis, another autoimmune disease commonly associated with SS, impaired peroxidase activity and reduced salivary levels of SOD are related to reduced unstimulated salivary flow. In individuals with normal unstimulated salivary flow, the values were similar to those of the control group (Zalewska et al., 2014). In rheumatoid arthritis (RA), blood levels of antioxidant enzymes and OS markers vary among studies (Quinonez-Flores et al., 2016). Together, these observations indicate the association of OS and inflammatory processes in autoimmune diseases. Discrepancies among studies may reflect differences along the disease time-course.
Tear proteomics was capable of identifying eighteen antioxidant enzymes in the tears of healthy volunteers (de Souza et al., 2006). Impression cytology analysis revealed that the conjunctival epithelial cells in patients with SSDE express lower amounts of SOD, GPx, and catalase than in healthy controls (Cejkova et al., 2008). However, tears of SS individuals have higher average levels of proteins than non-SS DE and controls. Tear proteomics also revealed that proteins related to OS (including SOD) and inflammatory activities presented higher levels in SS (Li et al., 2014). Differences in antioxidant enzyme expression, such as SOD, in SS individuals in comparison to controls may reflect aspects of the examined tissue, the time course of SS disease, and the methods utilized. Nonetheless, these differences indicate the fragility of using those biomarkers to understand complex aspects of OS in the LFU.
Lipid peroxidation markers such as HEL and 4-hydroxy-2-nonenal (4-HNE) were identified in the conjunctiva. The former was also identified in the tears of SS individuals. It was positively correlated with poor scores in clinical ocular surface findings and with the number of inflammatory cells harvested by brush cytology (Wakamatsu et al., 2013). These observations were later confirmed in a large sample of non-SS individuals who were compared to controls using other lipid peroxidation markers, suggesting that they are not present solely in SS (Choi et al., 2016b).
The mentioned findings indicate that OS markers are present in the saliva and tears of SS individuals and may take part in the mechanism of the disease. At the time when SOD and catalase were identified in joints and shown to protect synovial fluid degradation by ROS during inflammatory processes, therapeutic approaches against OS were suggested (McCord 1974). The actual stage of protection provided by antioxidants will be discussed in the next section.
Potential Therapeutic Approaches and the Role of Nutrition
There are controversies regarding biomarker identification and the role of OS as a trigger and not only a side effect or associated event of tissue damage and LFU dysfunction in patients with DE. However, many studies have emphasized the preventive and therapeutic approaches of antioxidants for patients with DE (Table 4 and Table 5).
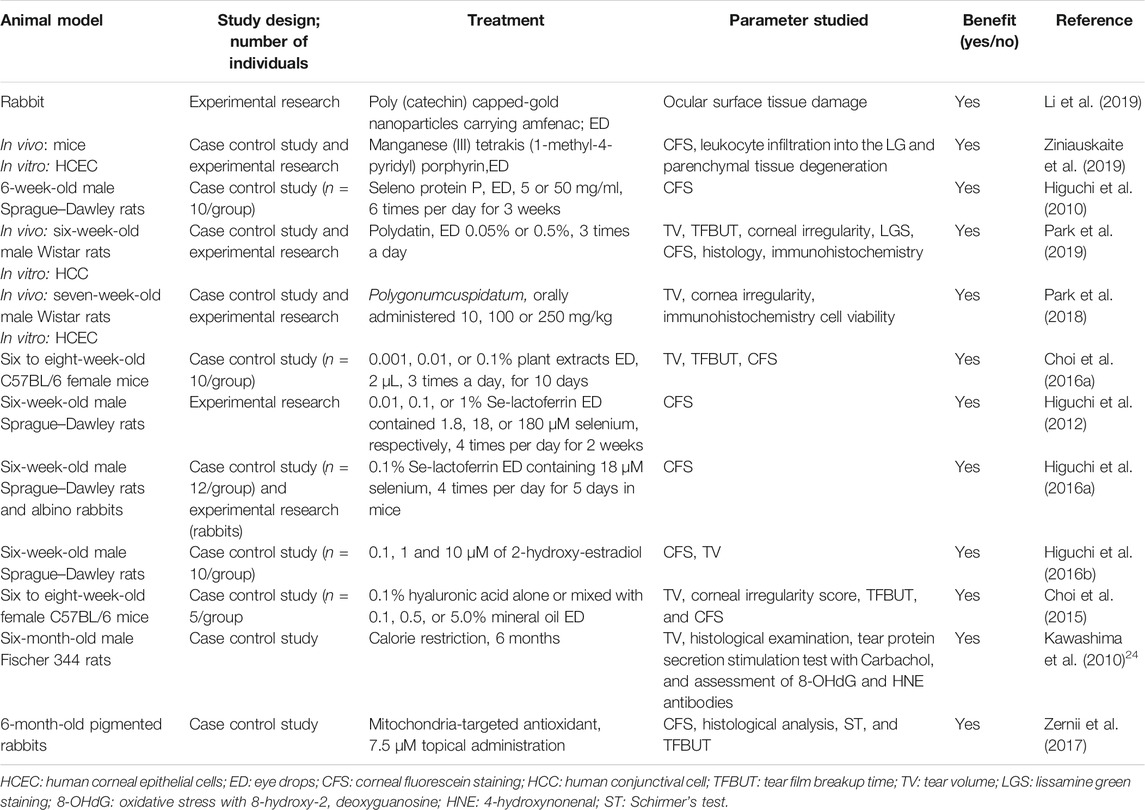
TABLE 4. Preventive and therapeutic antioxidant strategies for DE disease in animal models published between 2010 and 2019 were searched for “antioxidant” and “dry eye” in PubMed.
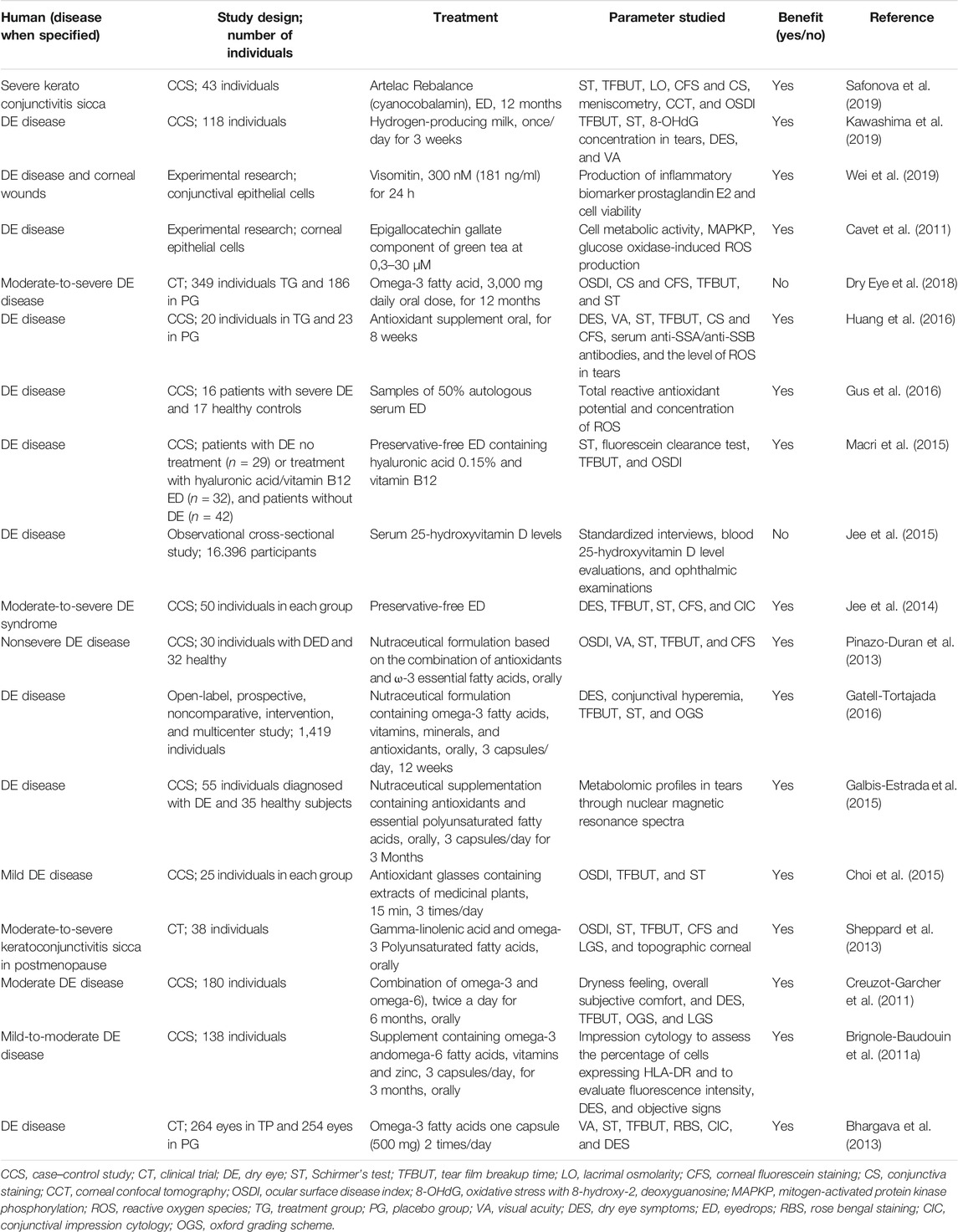
TABLE 5. Preventive and therapeutic antioxidant strategies for DE disease in humans published between 2010 and 2019 were searched for “antioxidant” and “dry eye” in PubMed.
Strategies to revert or treat DE with antioxidant therapies are becoming more common since 2010, based on the growing number of publications (Seen and Tong 2018; Higuchi 2019).
Regarding animal models, different treatment approaches have been reported, including many chemical substances. Plant extracts diluted in ethanol at different concentrations and applied topically three times a day for 10 days in mice showed that at 0.1%, it improved scopolamine-induced DE parameters (Choi et al., 2016a). Different strategies of administering selenium by topical eye drops to different DE animal models revealed improved parameters of DE and reduced markers of OS, which was also observed in corneal epithelial cells in vitro. Examples of these strategies include LG ablation, exposure to tobacco fume in rats and short-term desiccation in rabbits (Higuchi et al., 2012; Higuchi et al., 2016a). The beneficial results of the three studies are attributed to the associated enhancement of GPx activity.
The presence of antioxidants and ROS in 50% human autologous serum eye drop (ASED) samples was investigated. They were found to be similar among individuals with an ocular surface disease and with healthy controls and were stable in 3 months (Gus et al., 2016). This is an interesting observation, since ASED have been used for tear replacement and corneal wound healing for decades. However, their components and ubiquitous mechanisms of action have been seen for a long time (Fox et al., 1984; Leite et al., 2006). In humans, DE and non-DE individuals were compared after 1 month of vitamin B12/hyaluronic acid eye drop use before cataract surgery (Macri et al., 2015). A conjunctival biopsy during the surgical treatment provided tissue to evaluate OS markers by immunohistochemistry. The parameters were compared among the groups and correlated with DE clinical findings. Although a benefit was demonstrated, the lack of a placebo group, the use of lubricant or a vehicle, and the short period of follow-up all limit the conclusion that an antioxidant was a determinant for the obtained results (Macri et al., 2015).
The efficacy of drugs to reduce OS in DE involves careful examination, since the simple change or suppression of benzalkonium chloride (BAK) preservative can reduce the OS in the ocular surface. The BAK suppression in corticosteroid and cyclosporine A eye drops for 30 days was able to reduce the expression of inflammatory markers, increase the presence of antioxidant enzymes, and improve DE parameters in individuals with severe DE compared to controls using preserved formulas of the same eye drops (Jee et al., 2014). On the ocular surface, BAK is itself degraded into H2O2, leading to OS, inflammation, and tissue damage (Ingram et al., 2004; Baffa et al., 2008; Marques et al., 2015).
Oral treatment with capsules containing vitamins, omega-3, and other antioxidant elements, such as micronutrients, has been tested in DE individuals. The prescription of those supplements as a complementary DE therapy, despite a very modest increase in some of the parameters tested, is encouraged by the minimal risk of side effects (Galbis-Estrada et al., 2015; Gatell-Tortajada 2016; Huang et al., 2016). However, many of those studies have limited evidence-based benefits because of the lack of a placebo group, the limited follow-up period, and other uncontrolled factors that may have favored supplementary therapy. Additionally, they were not confirmed by a large randomized clinical trial (Rosenberg and Asbell 2010; Galbis-Estrada et al., 2015; Gatell-Tortajada 2016; Huang et al., 2016; Dry Eye et al., 2018).
In the same way, the use of glasses containing a plant extract of a combination of medicinal antioxidants for 8 weeks, 3 times a day (15 min/each), compared to a group who used placebo glasses, presented improvement in comparison to baseline on DE parameters and subjective analyses of the discomfort measured by the Ocular surface disease index (OSDI) score. This also suggests some statistical effect, such as returning to the mean (Choi Won et al., 2015).
Among life habits, the most beneficial to reduce OS and LFU inflammation, although with little robust data in human studies, is calorie restriction (CR) (Tsubota et al., 2012). The simplest explanation is that in reducing glucose and carbohydrate intake and calorie burning, ROS formation as side products of energy generation will be reduced. Subsequently, the cascade of tissue scavengers, inflammation, and cytotoxicity will be diminished, preserving function and postponing the aging process. The appropriate time to introduce this strategy and level of restriction in implementing an effective antioxidant therapeutic strategy is unknown. As mentioned above, most of the studies with animals induce CR of 30% or more and very early in life. However, Dr. Kazuo Tsubota reported his personal experience with benefits observed in his middle age (Tsubota et al., 2010).
Another lifestyle habit with potential beneficial antioxidant effects for DE is physical exercise. Although exercise is largely recommended, excessive exercise and a higher caloric demand are less studied factors that may have a dual effect of optimizing the scavenger mechanisms and inducing OS. As of now, the boundaries are unknown (Kruk et al., 2015; Jones et al., 2017).
The pathogenic microbiota that aggravates the signs and symptoms of chronic diseases is called dysbiosis. It is associated with DE in patients with DM and SS, where a more diverse microbiota reduces the severity of inflammation and OS in the target organs of individuals with those diseases (de Paiva et al., 2016; Luca et al., 2019). These observations reinforce the implications of environmental factors such as the influence of nutritional deficiencies in the development of OS associated with DE. They also reinforce the potential benefit of microbiota manipulation in reducing the impact of those diseases.
Based on the above, the simplest way to prevent OS related to DE is consuming calorie-restricted healthy meals that are filled with micronutrients and vitamins capable of promoting microbiota diversity, working as ROS scavengers and antioxidants, and limiting the extension of OS in the LFU and in the entire body (Figure 3). However, there are individuals and populations deprived of micronutrients and vitamins in a phenomenon defined as “hidden hunger.” The term is used to differentiate the cases from chronic hunger, where the diets are limited by macronutrients and calories (Gödecke et al., 2018). In fact, the aging population and those with chronic diseases are estimated to comprise approximately two billion people, and the deprivation of such micronutrients and vitamins increases their vulnerability regarding ROS scavenging and antioxidant processes. This may impact DE prevalence and severity, as indicated above (2018).
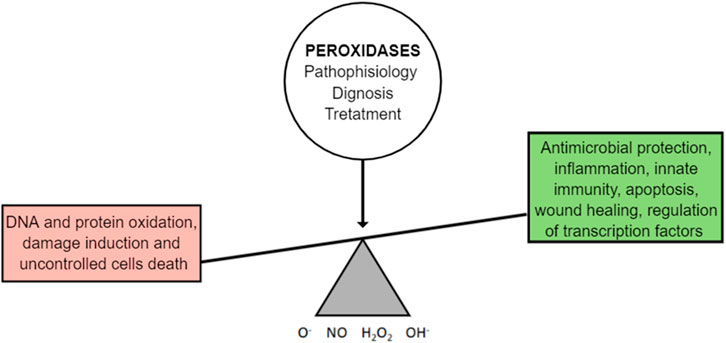
FIGURE 3. OS is an integrated mechanism of the biological functions and the mechanisms of the diseases. It involves caloric restriction and body synergism with its microflora.
The causes of hidden hunger involve socioeconomic parameters, cultural habits, modern methods of food production, storage, and processing, and individuals’ health status (Bawa and Anilakumar 2013; Faustino et al., 2016; Faustino et al., 2018; Gödecke et al., 2018; Trono 2019). Genetic modification to produce food crop biofortification enriched for Fe, Zn, and vitamin A is an initiative to revert the global imbalance between an excessive caloric diet versus micronutrient malnutrition. It can be a nutritional approach to the antioxidant strategy of preventing OS in chronic diseases and aging, including DE (Kumar et al., 2019). To date, the impact of dietary micronutrient deficiency on OS associated with DE is unknown. Better biomarkers of OS population studies are necessary to clarify the extent of this association.
In summary, we agree with previous reports that the antioxidant therapeutic approach for DE is not a simple task and that current strategies are limited and require further studies (Seen and Tong 2018).
Conclusion
In conclusion, it is clear that OS and the expression of the antioxidant elements in the LFU is a growing research field for understanding the mechanisms of DE, the ocular surface response to environmental aggression, and autoimmune mechanisms such as those observed in SS. Biomarkers are still limited in identifying disease mechanisms due to lack of specificity and differences in tissues and species expression, as well as in the duration of diseases. In several models reporting the association of DE and OS, the egg and chicken effect inhibits conclusions about the presence of OS as the cause or as part of the observed impacts, therefore not allowing us to identify exact targets for therapy. Thus far, we do not have among OS markers or antioxidant agents the reliable elements for determining DE diagnoses or exerting DE cures. Therapeutic approaches involve antioxidant therapy and supplementation with micronutrients. The trend of excessive calorie intake associated with micronutrient deficiency may contribute to OS and the associated diseases, supporting nutritional strategies for reducing OS in DE. In the future, the growing understanding of the protective versus the damaging effects of OS in the LFU and the cross-talk between the antioxidant and inflammatory pathways may improve our knowledge of the DE mechanisms and the DE therapy itself.
Method of Literature Search
A search in the PubMed and Scielo databases was conducted between 2010 and 2020. The search terms of the investigation were “aging,” “antimicrobial,” “antioxidant,” “diabetes mellitus,” “dry eye,” “exocrine gland,” “hormone,” “insulin,” “lacrimal gland,” “lactoperoxidase,” “ocular surface,” “oxidative stress,” “peroxidase,” “saliva,” “sex,” “superoxide dismutase,” “tears” and “tear film.” Our search was extensive, with articles written in English and not limited to the year of publication. All abstracts were screened, and relevant articles were included in this review.
Author Contributions
All authors listed have made a substantial, direct, and intellectual contribution to the work and approved it for publication.
Funding
The study was supported by grants from the following Brazilian governmental institutions: Fundação de Amparo a Pesquisa do Estado de São Paulo (FAPESP) (n°2010/50470-5, 2015/20580-7 and 2014/22451-7) (São Paulo, SP, Brazil); Conselho Nacional de Desenvolvimento Científico e Tecnológico (CNPq) (no: 474450/2012-0) (Brasilia, DF, Brazil); CAPES (Coordenação de Aperfeiçoamento de Pessoal de Nível Superior) (Finance Code 001) (Brasília, DF, Brazil); Fundação de Apoio ao Ensino, Pesquisa e Assistência do Hospital das Clinicas da Faculdade de Medicina de Ribeirão Preto da Universidade de São Paulo (FAEPA) (669/2018) (Ribeirão Preto, SP. Brazil); and Research Core of Ocular Physiopathology and Therapeutics from University of São Paulo (NAP-FTO) (n°12.1.25431.01.7) (Ribeirão Preto, SP. Brazil).
Conflict of Interest
The authors declare that the research was conducted in the absence of any commercial or financial relationships that could be construed as a potential conflict of interest.
Publisher’s Note
All claims expressed in this article are solely those of the authors and do not necessarily represent those of their affiliated organizations, or those of the publisher, the editors and the reviewers. Any product that may be evaluated in this article, or claim that may be made by its manufacturer, is not guaranteed or endorsed by the publisher.
References
Acera, A., Suárez, T., Rodríguez-Agirretxe, I., Vecino, E., and Durán, J. A. (2011). Changes in Tear Protein Profile in Patients with Conjunctivochalasis. Cornea 30, 42–49. doi:10.1097/ico.0b013e3181dea7d7
Adachi, J., Kumar, C., Zhang, Y., Olsen, J. V., and Mann, M. (2006). The Human Urinary Proteome Contains More Than 1500 Proteins, Including a Large Proportion of Membrane Proteins. Genome Biol. 7, R80. doi:10.1186/gb-2006-7-9-r80
Akpek, E. K., Lindsley, K. B., Adyanthaya, R. S., and Swamy, R. (2011). AN Baer & PJ McDonnellTreatment of Sjögren's Syndrome-Associated Dry Eye an Evidence-Based Review. Ophthalmology 118, 1242–1252.
Alves, M., Novaes, P., Morraye, Mde. A., Reinach, P. S., and Rocha, E. M. (2014). Is Dry Eye an Environmental Disease? Arq Bras Oftalmol 77, 193–200. doi:10.5935/0004-2749.20140050
Alves, M., Calegari, V. C., Cunha, D. A., Saad, M. J. A., Velloso, L. A., and Rocha, E. M. (2005a). Increased Expression of Advanced Glycation End-Products and Their Receptor, and Activation of Nuclear Factor Kappa-B in Lacrimal Glands of Diabetic Rats. Diabetologia 48, 2675–2681. doi:10.1007/s00125-005-0010-9
Alves, M., Cunha, D. A., Calegari, V. C., Saad, M. J. A., Boschero, A. C., Velloso, L. A., et al. (2005b). Nuclear Factor-Κb and Advanced Glycation End-Products Expression in Lacrimal Glands of Aging Rats. J. Endocrinol. 187, 159–166. doi:10.1677/joe.1.06209
Andrade, A. S., Salomon, T. B., Behling, C. S., Mahl, C. D., Hackenhaar, F. S., Putti, J., et al. (2014). Alpha-lipoic Acid Restores Tear Production in an Animal Model of Dry Eye. Exp. Eye Res. 120, 1–9. doi:10.1016/j.exer.2013.12.014
Andrade Cunha, D., de Alves, M. C., Stoppiglia, L. F., Jorge, A. G., Módulo, C. M., Carneiro, E. M., et al. (2007). Extra-pancreatic Insulin Production in RAt Lachrymal Gland after Streptozotocin-Induced Islet β-cells Destruction. Biochim. Biophys. Acta (Bba) - Gen. Subjects 1770, 1128–1135. doi:10.1016/j.bbagen.2007.05.002
Atli, T., Keven, K., Avci, A., Kutlay, S., Turkcapar, N., Varli, M., et al. (2004). Oxidative Stress and Antioxidant Status in Elderly Diabetes Mellitus and Glucose Intolerance Patients. Arch. Gerontol. Geriatr. 39, 269–275. doi:10.1016/j.archger.2004.04.065
Baboonian, C., Venables, P. J., Booth, J., Williams, D. G., Roffe, L. M., and Maini, R. N. (1989). Virus Infection Induces Redistribution and Membrane Localization of the Nuclear Antigen La (SS-B): a Possible Mechanism for Autoimmunity. Clin. Exp. Immunol. 78, 454–459.
Baffa, L. d. P., Ricardo, J. R. d. S., Dias, A. C., Módulo, C. M., Braz, A. M., Paula, J. S. d., et al. (2008). Tear Film and Ocular Surface Alterations in Chronic Users of Antiglaucoma Medications. Arq. Bras. Oftalmol. 71, 18–21. doi:10.1590/s0004-27492008000100004
Bakker, W. W., van Dael, C. M. L., Pierik, L. J. W. M., van Wijk, J. A. E., Nauta, J., Borghuis, T., et al. (2005). Altered Activity of Plasma Hemopexin in Patients with Minimal Change Disease in Relapse. Pediatr. Nephrol. 20, 1410–1415. doi:10.1007/s00467-005-1936-3
Batista, T. M., Tomiyoshi, L. M., Dias, A. C., Roma, L. P., Módulo, C. M., Malki, L. T., et al. (2012). Age-dependent Changes in Rat Lacrimal Gland Anti-oxidant and Vesicular Related Protein Expression Profiles. Mol. Vis. 18, 194–202.
Bawa, A. S., and Anilakumar, K. R. (2013). Genetically Modified Foods: Safety, Risks and Public Concerns-A Review. J. Food Sci. Technol. 50, 1035–1046. doi:10.1007/s13197-012-0899-1
Bhargava, R., Kumar, P., Kumar, M., Mehra, N., and Mishra, A. (2013). A Randomized Controlled Trial of omega-3 Fatty Acids in Dry Eye Syndrome. Int. J. Ophthalmol. 6, 811–816. doi:10.3980/j.issn.2222-3959.2013.06.13
Bonini, M. G., Siraki, A. G., Bhattacharjee, S., and Mason, R. P. (2007). Glutathione-induced Radical Formation on Lactoperoxidase Does Not Correlate with the Enzyme's Peroxidase Activity. Free Radic. Biol. Med. 42, 985–992. doi:10.1016/j.freeradbiomed.2006.12.026
Brignole-Baudouin, F., Baudouin, C., Aragona, P., Rolando, M., Labetoulle, M., Pisella, P. J., et al. (2011a). A Multicentre, Double-Masked, Randomized, Controlled Trial Assessing the Effect of Oral Supplementation of omega-3 and omega-6 Fatty Acids on a Conjunctival Inflammatory Marker in Dry Eye Patients. Acta Ophthalmol. 89, e591–e597. doi:10.1111/j.1755-3768.2011.02196.x
Brignole-Baudouin, F., Riancho, L., Liang, H., Nakib, Z., and Baudouin, C. (2011b). In Vitro comparative Toxicology of Polyquad-Preserved and Benzalkonium Chloride-Preserved Travoprost/timolol Fixed Combination and Latanoprost/timolol Fixed Combination. J. Ocul. Pharmacol. Ther. 27, 273–280. doi:10.1089/jop.2010.0111
Bromberg, B. B., Cripps, M. M., and Welch, M. H. (1989). Peroxidase Secretion by Lacrimal Glands from Juvenile F344 Rats. Invest. Ophthalmol. Vis. Sci. 30, 562–568.
Bromberg, B. B., and Welch, M. H. (1985). Lacrimal Protein Secretion: Comparison of Young and Old Rats. Exp. Eye Res. 40, 313–320. doi:10.1016/0014-4835(85)90015-6
Bron, A. J., and Seal, D. V. (1986). The Defences of the Ocular Surface. Trans. Ophthalmol. Soc. U K 105 (Pt 1), 18–25.
Carlsson, J., Iwami, Y., and Yamada, T. (1983). Hydrogen Peroxide Excretion by Oral Streptococci and Effect of Lactoperoxidase-Thiocyanate-Hydrogen Peroxide. Infect. Immun. 40, 70–80. doi:10.1128/iai.40.1.70-80.1983
Cavet, M. E., Harrington, K. L., Vollmer, T. R., Ward, K. W., and Zhang, J. Z. (2011). Anti-inflammatory and Anti-oxidative Effects of the green tea Polyphenol Epigallocatechin Gallate in Human Corneal Epithelial Cells. Mol. Vis. 17, 533–542.
Cejka, C., and Cejkova, J. (2015). Oxidative Stress to the Cornea, Changes in Corneal Optical Properties, and Advances in Treatment of Corneal Oxidative Injuries. Oxid Med. Cell Longev 2015, 591530. doi:10.1155/2015/591530
Cejková, J., Ardan, T., Simonová, Z., Cejka, C., Malec, J., Brunová, D., et al. (2008). Decreased Expression of Antioxidant Enzymes in the Conjunctival Epithelium of Dry Eye (Sjögren's Syndrome) and its Possible Contribution to the Development of Ocular Surface Oxidative Injuries. Histol. Histopathol 23, 1477–1483. doi:10.14670/HH-23.1477
Cejkova, J., and Cejka, C. (2015). The Role of Oxidative Stress in Corneal Diseases and Injuries. Histol. Histopathol 30, 893–900.
Chandrasekar, B., McGuff, H. S., Aufdermorte, T. B., Troyer, D. A., Talal, N., and Fernandes, G. (1995). Effects of Calorie Restriction on Transforming Growth Factor β1 and Proinflammatory Cytokines in Murine Sjogren's Syndrome. Clin. Immunol. immunopathology 76, 291–296. doi:10.1006/clin.1995.1128
Chase, M. J., and Klebanoff, S. J. (1992). Viricidal Effect of Stimulated Human Mononuclear Phagocytes on Human Immunodeficiency Virus Type 1. Proc. Natl. Acad. Sci. 89, 5582–5585. doi:10.1073/pnas.89.12.5582
Choi Jung Han, J. H., Kim, J. H., Li, Z., Oh, H. J., Ahn, K. Y., and Yoon, K. C. (2015). Efficacy of the Mineral Oil and Hyaluronic Acid Mixture Eye Drops in Murine Dry Eye. Korean J. Ophthalmol. 29, 131–137. doi:10.3341/kjo.2015.29.2.131
Choi, W., Lee, J. B., Cui, L., Li, Y., Li, Z., Choi, J. S., et al. (2016a). Therapeutic Efficacy of Topically Applied Antioxidant Medicinal Plant Extracts in a Mouse Model of Experimental Dry Eye. Oxid Med. Cell Longev 2016, 4727415. doi:10.1155/2016/4727415
Choi, W., Lian, C., Ying, L., Kim, G. E., You, I. C., Park, S. H., et al. (2016b). Expression of Lipid Peroxidation Markers in the Tear Film and Ocular Surface of Patients with Non-sjogren Syndrome: Potential Biomarkers for Dry Eye Disease. Curr. Eye Res. 41, 1143–1149. doi:10.3109/02713683.2015.1098707
Choi Won, W., Kim, J. C., Kim, W. S., Oh, H. J., Yang, J. M., Lee, J. B., et al. (2015). Clinical Effect of Antioxidant Glasses Containing Extracts of Medicinal Plants in Patients with Dry Eye Disease: A Multi-Center, Prospective, Randomized, Double-Blind, Placebo-Controlled Trial. PLoS One 10, e0139761. doi:10.1371/journal.pone.0139761
Colman, R. J., Anderson, R. M., Johnson, S. C., Kastman, E. K., Kosmatka, K. J., Beasley, T. M., et al. (2009). Caloric Restriction Delays Disease Onset and Mortality in Rhesus Monkeys. Science 325, 201–204. doi:10.1126/science.1173635
Cornelius, J. G., Luttge, B. G., and Peck, A. B. (1993). Antioxidant Enzyme Activities in IDD-Prone and IDD-Resistant Mice: a Comparative Study. Free Radic. Biol. Med. 14, 409–420. doi:10.1016/0891-5849(93)90090-h
Crapo, J. D., Oury, T., Rabouille, C., Slot, J. W., and Chang, L. Y. (1992). Copper,zinc Superoxide Dismutase Is Primarily a Cytosolic Protein in Human Cells. Proc. Natl. Acad. Sci. 89, 10405–10409. doi:10.1073/pnas.89.21.10405
Creuzot-Garcher, C., Baudouin, C., Labetoulle, M., Pisella, P.-J., Mouriaux, F., Meddeb-Ouertani, A., et al. (2011). Évaluation de l'efficacité du Nutrilarm, complément nutritionnel à base d'acides gras essentiels polyinsaturés oméga 3 et oméga 6, versus placebo chez des patients atteints de sécheresse oculaire bilatérale modérée et traitée. J. Français d'Ophtalmologie 34, 448–455. doi:10.1016/j.jfo.2011.01.018
Crouch, R. K., Goletz, P., Snyder, A., and Coles, W. H. (1991). Antioxidant Enzymes in Human Tears. J. Ocul. Pharmacol. Ther. 7, 253–258. doi:10.1089/jop.1991.7.253
Daiyasu, H., and Toh, H. (2000). Molecular Evolution of the Myeloperoxidase Family. J. Mol. Evol. 51, 433–445. doi:10.1007/s002390010106
Davies, M. J., Hawkins, C. L., Pattison, D. I., and Rees, M. D. (2008). Mammalian Heme Peroxidases: from Molecular Mechanisms to Health Implications. Antioxid. Redox Signaling 10, 1199–1234. doi:10.1089/ars.2007.1927
De Amit Roy, P. K., Banerjee, R. K., and Banerjee, R. (1987). Immunological Characterization of Soluble Peroxidases from Rat Tissues Including Preputial Gland. Mol. Cell Biochem 77, 127–134. doi:10.1007/bf00221921
de Paiva, C. S., Jones, D. B., Stern, M. E., Bian, F., Moore, Q. L., Corbiere, S., et al. (2016). Altered Mucosal Microbiome Diversity and Disease Severity in Sjögren Syndrome. Sci. Rep. 6, 23561. doi:10.1038/srep23561
de Paiva, C. S., and Rocha, E. M. (2015). Sjögren Syndrome. Curr. Opin. Ophthalmol. 26, 517–525. doi:10.1097/icu.0000000000000208
De, P. K. (1992). Tissue Distribution of Constitutive and Induced Soluble Peroxidase in Rat. Purification and Characterization from Lacrimal Gland. Eur. J. Biochem. 206, 59–67. doi:10.1111/j.1432-1033.1992.tb16901.x
de Souza, G. A., Godoy, L. M., and Mann, M. (2006). Identification of 491 Proteins in the Tear Fluid Proteome Reveals a Large Number of Proteases and Protease Inhibitors. Genome Biol. 7, R72. doi:10.1186/gb-2006-7-8-r72
Deconte, S. R., Oliveira, R. J. d. S., Calábria, L. K., Oliveira, V. N. d., Gouveia, N. M. d., Moraes, A. d. S., et al. (2011). Alterations of Antioxidant Biomarkers and Type I Collagen Deposition in the Parotid Gland of Streptozotocin-Induced Diabetic Rats. Arch. Oral Biol. 56, 744–751. doi:10.1016/j.archoralbio.2011.01.005
Dias, A. C., Batista, T. M., Roma, L. P., Módulo, C. M., Malki, L. T., Dias, L. C., et al. (2015). Insulin Replacement Restores the Vesicular Secretory Apparatus in the Diabetic Rat Lacrimal Gland. Arq Bras Oftalmol 78, 158–163. doi:10.5935/0004-2749.20150041
Dias, A. C., Mo´dulo, C. M., Jorge, A. l. G., Braz, A. M., Jorda∼o, A. A., Filho, R. B., et al. (2007). Influence of Thyroid Hormone on Thyroid Hormone Receptor β-1 Expression and Lacrimal Gland and Ocular Surface Morphology. Invest. Ophthalmol. Vis. Sci. 48, 3038–3042. doi:10.1167/iovs.06-1309
Dogru, M., Kojima, T., Simsek, C., and Tsubota, K. (2018). Potential Role of Oxidative Stress in Ocular Surface Inflammation and Dry Eye Disease. Invest. Ophthalmol. Vis. Sci. 59, DES163–DES168. doi:10.1167/iovs.17-23402
Dowling, D. K., and Simmons, L. W. (2009). Reactive Oxygen Species as Universal Constraints in Life-History Evolution. Proc. R. Soc. B. 276, 1737–1745. doi:10.1098/rspb.2008.1791
Draper, C. E., Singh, J., and Adeghate, E. (2003). Effects of Age on Morphology, Protein Synthesis and Secretagogue-Evoked Secretory Responses in the Rat Lacrimal Gland. Mol. Cell Biochem 248, 7–16. doi:10.1023/a:1024159529257
Draper, C. E., Adeghate, E. A., Singh, J., and Pallot, D. J. (1999). Evidence to Suggest Morphological and Physiological Alterations of Lacrimal Gland Acini with Ageing. Exp. Eye Res. 68, 265–276. doi:10.1006/exer.1998.0605
Dröge, W. (2002). Free Radicals in the Physiological Control of Cell Function. Physiol. Rev. 82, 47–95. doi:10.1152/physrev.00018.2001
Drozhzhyna, G., Pavlovskii, M., and Pavlovskaia, G. (2019). Peroxidation Processes in Ocular Surface Tissues in Experimental Hypothyroidism. Oftalmol Zh 81, 64–68. doi:10.31288/oftalmolzh201946468
Dry Eye, A., Asbell, P. A., Maguire, M. G., Pistilli, M., Ying, G. S., Szczotka-Flynn, L. B., et al. (2018). n-3 Fatty Acid Supplementation for the Treatment of Dry Eye Disease. N. Engl. J. Med. 378, 1681–1690. doi:10.1056/NEJMoa1709691
Everse, J., Everse, K. E., and Grisham, M. B. (1991). Peroxidases in Chemistry and Biology. Boca Raton, FL: CRC Press.
Faustino, J. F., Ribeiro-Silva, A., Dalto, R. F., Souza, M. M., Furtado, J. M., Rocha, Gde. M., et al. (2016). Vitamin A and the Eye: an Old Tale for Modern Times. Arq Bras Oftalmol 79, 56–61. doi:10.5935/0004-2749.20160018
Flicek, P. (2020). e!Ensembl. Available at: https://www.ensembl.org/index.html.
Forman, H. J. (2016). Redox Signaling: An Evolution from Free Radicals to Aging. Free Radic. Biol. Med. 97, 398–407. doi:10.1016/j.freeradbiomed.2016.07.003
Fox, R. I., Chan, R., Michelson, J. B., Belmont, J. B., and Michelson, P. E. (1984). Beneficial Effect of Artificial Tears Made with Autologous Serum in Patients with Keratoconjunctivitis Sicca. Arthritis Rheum. 27, 459–461. doi:10.1002/art.1780270415
Fridovich, I. (2013). Oxygen: How Do We Stand it? Med. Princ Pract. 22, 131–137. doi:10.1159/000339212
Galbis-Estrada, C., Pinazo-Durán, M. D., Martínez-Castillo, S., Morales, J. M., Monleón, D., and Zanon-Moreno, V. (2015). A Metabolomic Approach to Dry Eye Disorders. The Role of Oral Supplements with Antioxidants and omega 3 Fatty Acids. Mol. Vis. 21, 555–567.
García-Carrasco, M., Fuentes-Alexandro, S., Escárcega, R. O., Salgado, G., Riebeling, C., and Cervera, R. (2006). Pathophysiology of Sjögren's Syndrome. Arch. Med. Res. 37, 921–932. doi:10.1016/j.arcmed.2006.08.002
Gatell-Tortajada, J. (2016). Oral Supplementation with a Nutraceutical Formulation Containing omega-3 Fatty Acids, Vitamins, Minerals, and Antioxidants in a Large Series of Patients with Dry Eye Symptoms: Results of a Prospective Study. Clin. Interv. Aging 11, 571–578. doi:10.2147/CIA.S98102
Gebicki, J. M. (2016). Oxidative Stress, Free Radicals and Protein Peroxides. Arch. Biochem. Biophys. 595, 33–39. doi:10.1016/j.abb.2015.10.021
Gerina, L. S. (1980). Role of Salivary Glands in Extrarenal Production of Erythropoietin. Fiziol Zh SSSR Im I M Sechenova 66, 1412–1417.
Gödecke, T., Stein, A. J., and Qaim, M. (2018). The Global burden of Chronic and Hidden Hunger: Trends and Determinants. Glob. Food Security 17, 21–29. doi:10.1016/j.gfs.2018.03.004
Gogia, R., Richer, S. P., and Rose, R. C. (1998). Tear Fluid Content of Electrochemically Active Components Including Water Soluble Antioxidants. Curr. Eye Res. 17, 257–263. doi:10.1076/ceyr.17.3.257.5213
Groeger, G., Quiney, C., and Cotter, T. G. (2009). Hydrogen Peroxide as a Cell-Survival Signaling Molecule. Antioxid. Redox Signaling 11, 2655–2671. doi:10.1089/ars.2009.2728
Gus, P. I., Marinho, D., Zelanis, S., Belló-Klein, A., Locatelli, C., Nicola, F., et al. (2016). A Case-Control Study on the Oxidative Balance of 50% Autologous Serum Eye Drops. Oxid Med. Cell Longev 2016, 9780193. doi:10.1155/2016/9780193
Gus, P. I., Belló-Klein, A., Llesuy, S., Quinto, G. G., Matos, G. H., and Bechara, S. J. (2006). Potencial antioxidativo da lágrima de adultos jovens. Arq. Bras. Oftalmol. 69, 565–570. doi:10.1590/s0004-27492006000400020
Halliwell, B., and Chirico, S. (1993). Lipid Peroxidation: its Mechanism, Measurement, and Significance. Am. J. Clin. Nutr. 57, 715S–725S. doi:10.1093/ajcn/57.5.715s
Halliwell, B., and Gutteridge, J. M. C. (1990). The Antioxidants of Human Extracellular Fluids. Arch. Biochem. Biophys. 280, 1–8. doi:10.1016/0003-9861(90)90510-6
Halliwell, B. (1995). Oxygen Radicals, Nitric Oxide and Human Inflammatory Joint Disease. Ann. Rheum. Dis. 54, 505–510. doi:10.1136/ard.54.6.505
Halliwell, B. (2006). Reactive Species and Antioxidants. Redox Biology Is a Fundamental Theme of Aerobic Life. Plant Physiol. 141, 312–322. doi:10.1104/pp.106.077073
Heilbronn, L. K., and Ravussin, E. (2003). Calorie Restriction and Aging: Review of the Literature and Implications for Studies in Humans. Am. J. Clin. Nutr. 78, 361–369. doi:10.1093/ajcn/78.3.361
Herzog, V., and Fahimi, H. D. (1976). Intracellular Distinction between Peroxidase and Catalase in Exocrine Cells of Rat Lacrimal Gland: a Biochemical and Cytochemical Study. Histochemistry 46, 273–286. doi:10.1007/bf02464417
Herzog, V., and Miller, F. (1972a). Endogenous Peroxidase in the Lacrimal Gland of the Rat and its Differentiation against Injected Catalase and Horseradish-Peroxidase. Histochemie 30, 235–246. doi:10.1007/bf00277594
Herzog, V., and Miller, F. (1972b). The Localization of Endogenous Peroxidase in the Lacrimal Gland of the Rat during Postnatal Development. J. Cell Biol 53, 662–680. doi:10.1083/jcb.53.3.662
Herzog, V., Sies, H., and Miller, F. (1976). Exocytosis in Secretory Cells of Rat Lacrimal Gland. Peroxidase Release from Lobules and Isolated Cells upon Cholinergic Stimulation. J. Cell Biol 70, 692–706. doi:10.1083/jcb.70.3.692
Higuchi, A., Oonishi, E., Kawakita, T., and Tsubota, K. (2016b). Evaluation of Treatment for Dry Eye with 2-hydroxyestradiol Using a Dry Eye Rat Model. Mol. Vis. 22, 446–453.
Higuchi, A. (2019). Development of New Pharmaceutical Candidates with Antioxidant Activity for the Treatment of Corneal Disorders. Suppl 38, S45–S49. doi:10.1097/ico.0000000000002072
Higuchi, A., Inoue, H., Kaneko, Y., Oonishi, E., and Tsubota, K. (2016a). Selenium-binding Lactoferrin Is Taken into Corneal Epithelial Cells by a Receptor and Prevents Corneal Damage in Dry Eye Model Animals. Sci. Rep. 6, 36903. doi:10.1038/srep36903
Higuchi, A., Inoue, H., Kawakita, T., Ogishima, T., and Tsubota, K. (2012). Selenium Compound Protects Corneal Epithelium against Oxidative Stress. PLoS One 7, e45612. doi:10.1371/journal.pone.0045612
Higuchi, A., Takahashi, K., Hirashima, M., Kawakita, T., and Tsubota, K. (2010). Selenoprotein P Controls Oxidative Stress in Cornea. PLoS One 5, e9911. doi:10.1371/journal.pone.0009911
Hodges, R. R., Shatos, M. A., Tarko, R. S., Vrouvlianis, J., Gu, J., and Dartt, D. A. (2005). Nitric Oxide and cGMP Mediate α1D-Adrenergic Receptor-Stimulated Protein Secretion and P42/p44 MAPK Activation in Rat Lacrimal Gland. Invest. Ophthalmol. Vis. Sci. 46, 2781–2789. doi:10.1167/iovs.05-0022
Huang, J. Y., Yeh, P. T., and Hou, Y. C. (2016). A Randomized, Double-Blind, Placebo-Controlled Study of Oral Antioxidant Supplement Therapy in Patients with Dry Eye Syndrome. Clin. Ophthalmol. 10, 813–820. doi:10.2147/OPTH.S106455
Ihalin, R., Loimaranta, V., and Tenovuo, J. (2006). Origin, Structure, and Biological Activities of Peroxidases in Human Saliva. Arch. Biochem. Biophys. 445, 261–268. doi:10.1016/j.abb.2005.07.004
Imlay, J. A., and Fridovich, I. (1991). Assay of Metabolic Superoxide Production in Escherichia coli. J. Biol. Chem. 266, 6957–6965. doi:10.1016/s0021-9258(20)89596-9
Ingram, P. R., Pitt, A. R., Wilson, C. G., Olejnik, O., and Spickett, C. M. (2004). A Comparison of the Effects of Ocular Preservatives on Mammalian and Microbial ATP and Glutathione Levels. Free Radic. Res. 38, 739–750. doi:10.1080/10715760410001712773
Jee, D., Park, M., Lee, H. J., Kim, M. S., and Kim, E. C. (2015). Comparison of Treatment with Preservative-free versus Preserved Sodium Hyaluronate 0.1% and Fluorometholone 0.1% Eyedrops after Cataract Surgery in Patients with Preexisting Dry-Eye Syndrome. J. Cataract Refract Surg. 41, 756–763. doi:10.1016/j.jcrs.2014.11.034
Jee, D., Park, S. H., Kim, M. S., and Kim, E. C. (2014). Antioxidant and Inflammatory Cytokine in Tears of Patients with Dry Eye Syndrome Treated with Preservative-free versus Preserved Eye Drops. Invest. Ophthalmol. Vis. Sci. 55, 5081–5089. doi:10.1167/iovs.14-14483
Jones, L., Downie, L. E., Korb, D., Benitez-Del-Castillo, J. M., Dana, R., Deng, S. X., et al. (2017). TFOS DEWS II Management and Therapy Report. Ocul. Surf. 15, 575–628. doi:10.1016/j.jtos.2017.05.006
Jorge, A. G., Módulo, C. M., Dias, A. C., Braz, A. M., Filho, R. B., Jordão, A. A., et al. (2009). Aspirin Prevents Diabetic Oxidative Changes in Rat Lacrimal Gland Structure and Function. Endocr 35, 189–197. doi:10.1007/s12020-009-9151-9
Jung, S. J., Mehta, J. S., and Tong, L. (2018). Effects of Environment Pollution on the Ocular Surface. Ocul. Surf. 16, 198–205. doi:10.1016/j.jtos.2018.03.001
Kawashima, M., Kawakita, T., Okada, N., Ogawa, Y., Murat, D., Nakamura, S., et al. (2010). Calorie Restriction: A New Therapeutic Intervention for Age-Related Dry Eye Disease in Rats. Biochem. Biophysical Res. Commun. 397, 724–728. doi:10.1016/j.bbrc.2010.06.018
Kawashima, M., Tsuno, S., Matsumoto, M., and Tsubota, K. (2019). Hydrogen-producing Milk to Prevent Reduction in Tear Stability in Persons Using Visual Display Terminals. Ocul. Surf. 17, 714–721. doi:10.1016/j.jtos.2019.07.008
Klebe, S., Callahan, T., and Power, J. H. (2014). Peroxiredoxin I and II in Human Eyes. J. Histochem. Cytochem. 62, 85–96. doi:10.1369/0022155413508409
Knas, M., Maciejczyk, M., Daniszewska, I., Klimiuk, A., Matczuk, J., Kolodziej, U., et al. (2016). Oxidative Damage to the Salivary Glands of Rats with Streptozotocin-Induced Diabetes-Temporal Study: Oxidative Stress and Diabetic Salivary Glands. J. Diabetes Res. 2016, 4583742.
Kojima, T., Wakamatsu, T. H., Dogru, M., Ogawa, Y., Igarashi, A., Ibrahim, O. M. A., et al. (2012). Age-Related Dysfunction of the Lacrimal Gland and Oxidative Stress. Am. J. Pathol. 180, 1879–1896. doi:10.1016/j.ajpath.2012.01.019
Kolodziej, U., Maciejczyk, M., Niklinska, W., Waszkiel, D., Zendzian-Piotrowska, M., Zukowski, P., et al. (2017). Chronic High-Protein Diet Induces Oxidative Stress and Alters the Salivary Gland Function in Rats. Arch. Oral Biol. 84, 6–12.
Kruk, J., Aboul-Enein, H. Y., Kładna, A. J. E., and Bowser, J. E. (2019). Oxidative Stress in Biological Systems and its Relation with Pathophysiological Functions: the Effect of Physical Activity on Cellular Redox Homeostasis. Free Radic. Res. 53, 497–521. doi:10.1080/10715762.2019.1612059
Kruk, J., Kubasik-Kladna, K., and Aboul-Enein, H. (2015). The Role Oxidative Stress in the Pathogenesis of Eye Diseases: Currnt Status and a Dual Role of Physical Activity. Mrmc 16, 241–257. doi:10.2174/1389557516666151120114605
Kumar, S., Palve, A., Joshi, C., and Srivastava, R. K. (2019). Crop Biofortification for Iron (Fe), Zinc (Zn) and Vitamin A with Transgenic Approaches. Heliyon 5, e01914.
Kurimoto, C., Kawano, S., Tsuji, G., Hatachi, S., Jikimoto, T., Sugiyama, D., et al. (2007). Thioredoxin May Exert a Protective Effect against Tissue Damage Caused by Oxidative Stress in Salivary Glands of Patients with Sjögren's Syndrome. J. Rheumatol. 34, 2035–2043.
Lee, H. S., Cui, L., Li, Y., Choi, J. S., Choi, J. H., Li, Z., et al. (2016). Influence of Light Emitting Diode-Derived Blue Light Overexposure on Mouse Ocular Surface. PLoS One 11, e0161041. doi:10.1371/journal.pone.0161041
Leite, S. C., de Castro, R. S., Alves, M., Cunha, D. A., Correa, M. E. P., da Silveira, L. A., et al. (2006). Risk Factors and Characteristics of Ocular Complications, and Efficacy of Autologous Serum Tears after Haematopoietic Progenitor Cell Transplantation. Bone Marrow Transpl. 38, 223–227. doi:10.1038/sj.bmt.1705426
Lenander-Lumikari, M. (1992). Inhibition of Candida Albicans by the peroxidase/SCN−/H2O2system. Oral Microbiol. Immunol. 7, 315–320. doi:10.1111/j.1399-302x.1992.tb00595.x
Li, B., Sheng, M., Li, J., Yan, G., Lin, A., Li, M., et al. (2014). Tear Proteomic Analysis of Sjögren Syndrome Patients with Dry Eye Syndrome by Two-Dimensional-Nano-Liquid Chromatography Coupled with Tandem Mass Spectrometry. Sci. Rep. 4, 5772. doi:10.1038/srep05772
Li, Y.-J., Luo, L.-J., Harroun, S. G., Wei, S.-C., Unnikrishnan, B., Chang, H.-T., et al. (2019). Synergistically Dual-Functional Nano Eye-Drops for Simultaneous Anti-inflammatory and Anti-oxidative Treatment of Dry Eye Disease. Nanoscale 11, 5580–5594. doi:10.1039/c9nr00376b
Liberati, V., de Feo, G., Madia, F., and Marcozzi, G. (2002). Effect of Oral Contraceptives on Lacrimal Fluid Peroxidase Activity in Women. Ophthalmic Res. 34, 251–253. doi:10.1159/000063882
Lin, Z., Zhou, Y., Wang, Y., Zhou, T., Li, J., Luo, P., et al. (2014). Serine Protease Inhibitor A3K Suppressed the Formation of Ocular Surface Squamous Metaplasia in a Mouse Model of Experimental Dry Eye. Invest. Ophthalmol. Vis. Sci. 55, 5813–5820. doi:10.1167/iovs.13-13546
Liu, H., Sheng, M., Liu, Y., Wang, P., Chen, Y., Chen, L., et al. (2015). Expression of SIRT1 and Oxidative Stress in Diabetic Dry Eye. Int. J. Clin. Exp. Pathol. 8, 7644–7653.
Looms, D. K., Tritsaris, K., and Dissing, S. (2002). Nitric Oxide-Induced Signalling in Rat Lacrimal Acinar Cells. Acta Physiol. Scand. 174, 109–115. doi:10.1046/j.1365-201x.2002.00935.x
Luca, M., Di Mauro, M., Di Mauro, M., and Luca, A. (2019). Gut Microbiota in Alzheimer's Disease, Depression, and Type 2 Diabetes Mellitus: The Role of Oxidative Stress. Oxid Med. Cell Longev 2019, 4730539. doi:10.1155/2019/4730539
Macri, A., Scanarotti, C., Bassi, A. M., Giuffrida, S., Sangalli, G., Traverso, C. E., et al. (2015). Evaluation of Oxidative Stress Levels in the Conjunctival Epithelium of Patients with or without Dry Eye, and Dry Eye Patients Treated with Preservative-free Hyaluronic Acid 0.15 % and Vitamin B12 Eye Drops. Graefes Arch. Clin. Exp. Ophthalmol. 253, 425–430. doi:10.1007/s00417-014-2853-6
Mansson-Rahemtulla, B., Rahemtulla, F., Baldone, D. C., Pruitt, K. M., and Hjerpe, A. (1988). Purification and Characterization of Human Salivary Peroxidase. Biochemistry 27, 233–239. doi:10.1021/bi00401a035
Marcozzi, G., Liberati, V., Madia, F., Centofanti, M., and de Feo, G. (2003). Age- and Gender-Related Differences in Human Lacrimal Fluid Peroxidase Activity. Ophthalmologica 217, 294–297. doi:10.1159/000070638
Marques, D. L., Alves, M., Modulo, C. M., Silva, L. E. C. M. d., Reinach, P., and Rocha, E. M. (2015). Lacrimal Osmolaritu and Ocular Surface in Experimental Model of Dry Eye Caused by Toxicity. Rev. Bras Oftalmol 74, 68–72. doi:10.5935/0034-7280.20150016
Mazumdar, A., Chatterjee, R., Adak, S., Ghosh, A., Mondal, C., and Banerjee, R. K. (1996). Characterization of Sheep Lacrimal-Gland Peroxidase and its Major Physiological Electron Donor. Biochem. J. 314 (Pt 2), 413–419. doi:10.1042/bj3140413
McCord, J. M. (1974). Free Radicals and Inflammation: protection of Synovial Fluid by Superoxide Dismutase. Science 185, 529–531. doi:10.1126/science.185.4150.529
McCord, J. M., and Fridovich, I. (1969). Superoxide Dismutase. J. Biol. Chem. 244, 6049–6055. doi:10.1016/s0021-9258(18)63504-5
McGill, J. I., Liakos, G. M., Goulding, N., and Seal, D. V. (1984). Normal Tear Protein Profiles and Age-Related Changes. Br. J. Ophthalmol. 68, 316–320. doi:10.1136/bjo.68.5.316
Módulo, C. M., Jorge, A. G., Dias, A. C., Braz, A. M., Bertazolli-Filho, R., Jordão, A. A., et al. (2009). Influence of Insulin Treatment on the Lacrimal Gland and Ocular Surface of Diabetic Rats. Endocr 36, 161–168. doi:10.1007/s12020-009-9208-9
Moon, J., Yoon, C. H., Choi, S. H., and Kim, M. K. (2020). Can Gut Microbiota Affect Dry Eye Syndrome? Ijms 21 (22), 8443. doi:10.3390/ijms21228443
Morrison, M., and Allen, P. Z. (1966). Lactoperoxidase: Identification and Isolation from Harderian and Lacrimal Glands. Science 152, 1626–1628. doi:10.1126/science.152.3729.1626
Nobuhara, Y., Kawano, S., Kageyama, G., Sugiyama, D., Saegusa, J., and Kumagai, S. (2007). Is SS-A/Ro52 a Hydrogen Peroxide-Sensitive Signaling Molecule? Antioxid. Redox Signaling 9, 385–391. doi:10.1089/ars.2006.1480
Nogueira, F. N., Carvalho, A. M., Yamaguti, P. M., and Nicolau, J. (2005). Antioxidant Parameters and Lipid Peroxidation in Salivary Glands of Streptozotocin-Induced Diabetic Rats. Clinica Chim. Acta 353, 133–139. doi:10.1016/j.cccn.2004.11.004
Oliver, C., Waters, J. F., Tolbert, C. L., and Kleinman, H. K. (1987). Growth of Exocrine Acinar Cells on a Reconstituted Basement Membrane Gel. In Vitro Cell Dev Biol 23, 465–473. doi:10.1007/bf02628416
Paliwal, A., and De, P. K. (2006). Marked Sexual Dimorphism of Lacrimal Gland Peroxidase in Hamster: Repression by Androgens and Estrogens. Biochem. Biophysical Res. Commun. 341, 1286–1293. doi:10.1016/j.bbrc.2006.01.095
Park, B., Jo, K., Lee, T. G., Hyun, S. W., Kim, J. S., and Kim, C. S. (2019). Polydatin Inhibits NLRP3 Inflammasome in Dry Eye Disease by Attenuating Oxidative Stress and Inhibiting the NF-Κb Pathway. Nutrients 11, 11. doi:10.3390/nu11112792
Park, B., Lee, I. S., Hyun, S. W., Jo, K., Lee, T. G., Kim, B. J. S., et al. (2018). The Protective Effect of Polygonum Cuspidatum (PCE) Aqueous Extract in a Dry Eye Model. Nutrients 10, 1550. doi:10.3390/nu10101550
Petrides, P. E., and Nauseef, W. M. (2000). The Peroxidase Multigene Family of Enzymes. Berlin: Springer.
Pinazo-Duran, M., Galbis-Estrada, C., Pons-Vázquez, S., Cantu-Dibildox, J., Marco-Ramirez, C., and Benitez-del-Castillo, F. (2013). Effects of a Nutraceutical Formulation Based on the Combination of Antioxidants and ω-3 Essential Fatty Acids in the Expression of Inflammation and Immune Response Mediators in Tears from Patients with Dry Eye Disorders. Cia 8, 139–148. doi:10.2147/cia.s40640
Quiñonez-Flores, C. M., González-Chávez, S. A., Del Río Nájera, D., and Pacheco-Tena, C. (2016). Oxidative Stress Relevance in the Pathogenesis of the Rheumatoid Arthritis: A Systematic Review. Biomed. Res. Int. 2016, 6097417. doi:10.1155/2016/6097417
Rahimy, E., Pitcher, J. D., Pangelinan, S. B., Chen, W., Farley, W. J., Niederkorn, J. Y., et al. (2010). Spontaneous Autoimmune Dacryoadenitis in Aged CD25KO Mice. Am. J. Pathol. 177, 744–753. doi:10.2353/ajpath.2010.091116
Rhee, S. G., Woo, H. A., Kil, I. S., and Bae, S. H. (2012). Peroxiredoxin Functions as a Peroxidase and a Regulator and Sensor of Local Peroxides. J. Biol. Chem. 287, 4403–4410. doi:10.1074/jbc.r111.283432
Ríos, J. D., Horikawa, Y., Chen, L.-L., Kublin, C. L., Hodges, R. R., Dartt, D. A., et al. (2005). Age-dependent Alterations in Mouse Exorbital Lacrimal Gland Structure, Innervation and Secretory Response. Exp. Eye Res. 80, 477–491. doi:10.1016/j.exer.2004.10.012
Rocha, E. M., Cotrim, A. P., Zheng, C., Riveros, P. P., Baum, B. J., and Chiorini, J. (2013). Recovery of Radiation-Induced Dry Eye and Corneal Damage by Pre Treatment with Adenoviral Vector-Mediated Transfer of Erythropoietin to the Salivary Glands in Mice. Hum Gene Ther. 24, 417–423. doi:10.1089/hum.2012.111
Rocha, E. M., Alves, M., Rios, J. D., and Dartt, D. A. (2008). The Aging Lacrimal Gland: Changes in Structure and Function. Ocul. Surf. 6, 162–174. doi:10.1016/s1542-0124(12)70177-5
Rocha, E. M., Carvalho, C. R. O., Saad, M. J. A., and Velloso, L. A. (2003). The Influence of Ageing on the Insulin Signalling System in Rat Lacrimal and Salivary Glands. Acta Ophthalmol. Scand. 81, 639–645. doi:10.1111/j.1395-3907.2003.00162.x
Rosenberg, E. S., and Asbell, P. A. (2010). Essential Fatty Acids in the Treatment of Dry Eye. Ocul. Surf. 8, 18–28. doi:10.1016/s1542-0124(12)70214-8
Ryo, K., Yamada, H., Nakagawa, Y., Tai, Y., Obara, K., Inoue, H., et al. (2006). Possible Involvement of Oxidative Stress in Salivary Gland of Patients with Sjögren's Syndrome. Pathobiology 73, 252–260. doi:10.1159/000098211
Safonova, T. N., Gladkova, O. V., and Boev, V. I. (2019). Oxidative Stress Correction in the Treatment of Severe Keratoconjunctivitis Sicca in Patients with Sjorgen's syndrome[Oxidative Stress Correction in the Treatment of Severe Keratoconjunctivitis Sicca in Patients with Sjorgen's Syndrome]. Vestn. Oftal'mol. 135, 59–66. doi:10.17116/oftalma201913501159
Sakai, O., Uchida, T., Imai, H., Ueta, T., and Amano, S. (2015). Role of Glutathione Peroxidase 4 in Conjunctival Epithelial Cells. Invest. Ophthalmol. Vis. Sci. 56, 538–543. doi:10.1167/iovs.14-15463
Scofield, R. H., and Harley, J. B. (1991). Autoantigenicity of Ro/SSA Antigen Is Related to a Nucleocapsid Protein of Vesicular Stomatitis Virus. Proc. Natl. Acad. Sci. 88, 3343–3347. doi:10.1073/pnas.88.8.3343
Seara, F. A. C., Maciel, L., Barbosa, R. A. Q., Rodrigues, N. C., Silveira, A. L. B., Marassi, M. P., et al. (2018). Cardiac Ischemia/reperfusion Injury Is Inversely Affected by Thyroid Hormones Excess or Deficiency in Male Wistar Rats. PLoS One 13, e0190355. doi:10.1371/journal.pone.0190355
Seen, S., and Tong, L. (2018). Dry Eye Disease and Oxidative Stress. Acta Ophthalmol. 96, e412–e420. doi:10.1111/aos.13526
Sheppard, J. D., Singh, R., McClellan, A. J., Weikert, M. P., Scoper, S. V., Joly, T. J., et al. (2013). Long-term Supplementation with N-6 and N-3 PUFAs Improves Moderate-To-Severe Keratoconjunctivitis Sicca. Cornea 32, 1297–1304. doi:10.1097/ico.0b013e318299549c
Shetty, R., Saeed, T., Rashed, H., Adeghate, E., and Singh, J. (2009). Effect of Diabetes Mellitus on Acinar Morphology, Peroxidase Concentration, and Release in Isolated Rat Lacrimal Glands. Curr. Eye Res. 34, 905–911. doi:10.3109/02713680903184268
Sies, H. (1991). Oxidative Stress: from Basic Research to Clinical Application. Am. J. Med. 91, 31S–38S. doi:10.1016/0002-9343(91)90281-2
Sies, H. (2015). Oxidative Stress: a Concept in Redox Biology and Medicine. Redox Biol. 4, 180–183. doi:10.1016/j.redox.2015.01.002
Soria, J., Acera, A., Merayo-LLoves, J., Durán, J. A., González, N., Rodriguez, S., et al. (2017). Tear Proteome Analysis in Ocular Surface Diseases Using Label-free LC-MS/MS and Multiplexed-Microarray Biomarker Validation. Sci. Rep. 7, 17478. doi:10.1038/s41598-017-17536-2
Soria, J., Durán, J. A., Etxebarria, J., Merayo, J., González, N., Reigada, R., et al. (2013). Tear Proteome and Protein Network Analyses Reveal a Novel Pentamarker Panel for Tear Film Characterization in Dry Eye and Meibomian Gland Dysfunction. J. Proteomics 78, 94–112. doi:10.1016/j.jprot.2012.11.017
Soukka, T., Tenovuo, J., and Lenander-Lumikari, M. (1992). Fungicidal Effect of Human Lactoferrin againstCandida Albicans. FEMS Microbiol. Lett. 90, 223–228. doi:10.1111/j.1574-6968.1992.tb05156.x
Stern, M. E., Gao, J., Siemasko, K. F., Beuerman, R. W., and Pflugfelder, S. C. (2004). The Role of the Lacrimal Functional Unit in the Pathophysiology of Dry Eye. Exp. Eye Res. 78, 409–416. doi:10.1016/j.exer.2003.09.003
Sullivan, D. A., Rocha, E. M., Aragona, P., Clayton, J. A., Ding, J., Golebiowski, B., et al. (2017). TFOS DEWS II Sex, Gender, and Hormones Report. Ocul. Surf. 15, 284–333. doi:10.1016/j.jtos.2017.04.001
Thaiss, C. A., Zmora, N., Levy, M., and Elinav, E. (2016). The Microbiome and Innate Immunity. Nature 535 (7610), 65–74. doi:10.1038/nature18847
Thomas, E. L., Jefferson, M. M., Joyner, R. E., Cook, G. S., and King, C. C. (1994). Leukocyte Myeloperoxidase and Salivary Lactoperoxidase: Identification and Quantitation in Human Mixed Saliva. J. Dent Res. 73, 544–555. doi:10.1177/00220345940730021001
Toda, I., Sullivan, B. D., Rocha, E. M., Da Silveira, L. A., Wickham, L. A., and Sullivan, D. A. (1999). Impact of Gender on Exocrine Gland Inflammation in Mouse Models of Sjögren's Syndrome. Exp. Eye Res. 69, 355–366. doi:10.1006/exer.1999.0715
Torricelli, A. A. M., Novaes, P., Matsuda, M., Alves, M. R., and Monteiro, M. L. R. (2011). Ocular Surface Adverse Effects of Ambient Levels of Air Pollution. Arq. Bras. Oftalmol. 74, 377–381. doi:10.1590/s0004-27492011000500016
Trono, D. (2019). Carotenoids in Cereal Food Crops: Composition and Retention throughout Grain Storage and Food Processing. Basel: Plants, 8.
Tsubota, K., Kawashima, M., Inaba, T., Dogru, M., Matsumoto, Y., Ishida, R., et al. (2012). The Antiaging Approach for the Treatment of Dry Eye. Suppl 31, S3–S8. doi:10.1097/ico.0b013e31826a05a8
Tsubota, K., Kawashima, M., Inaba, T., Dogru, M., Ogawa, Y., Nakamura, S., et al. (2010). The Era of Antiaging Ophthalmology Comes of Age: Antiaging Approach for Dry Eye Treatment. Ophthalmic Res. 44, 146–154. doi:10.1159/000316594
Uchino, Y., Kawakita, T., Miyazawa, M., Ishii, T., Onouchi, H., Yasuda, K., et al. (2012). Oxidative Stress Induced Inflammation Initiates Functional Decline of Tear Production. PLoS One 7, e45805. doi:10.1371/journal.pone.0045805
Ung, L., Pattamatta, U., Carnt, N., Wilkinson-Berka, J. L., Liew, G., and White, A. J. R. (2017). Oxidative Stress and Reactive Oxygen Species: a Review of Their Role in Ocular Disease. Clin. Sci. (Lond) 131, 2865–2883. doi:10.1042/cs20171246
Valko, M., Leibfritz, D., Moncol, J., Cronin, M. T. D., Mazur, M., and Telser, J. (2007). Free Radicals and Antioxidants in normal Physiological Functions and Human Disease. Int. J. Biochem. Cell Biol. 39, 44–84. doi:10.1016/j.biocel.2006.07.001
Vitali, C., Bombardieri, S., Jonsson, R., Moutsopoulos, H., Alexander, E., Carsons, S., et al. (2002). Classification Criteria for Sjogren's Syndrome: a Revised Version of the European Criteria Proposed by the American-European Consensus Group. Ann. Rheum. Dis. 61, 554–558. doi:10.1136/ard.61.6.554
Wakamatsu, T. H., Dogru, M., Matsumoto, Y., Kojima, T., Kaido, M., Ibrahim, O. M. A., et al. (2013). Evaluation of Lipid Oxidative Stress Status in Sjögren Syndrome Patients. Invest. Ophthalmol. Vis. Sci. 54, 201–210. doi:10.1167/iovs.12-10325
Wakamatsu, T. H., Dogru, M., and Tsubota, K. (2008). Tearful Relations: Oxidative Stress, Inflammation and Eye Diseases. Arq. Bras. Oftalmol. 71, 72–79. doi:10.1590/s0004-27492008000700015
Wang, C., Zaheer, M., Bian, F., Quach, D., Swennes, A. G., Britton, R. A., et al. (2018). Sjögren-Like Lacrimal Keratoconjunctivitis in Germ-free Mice. Int. J. Mol. Sci. 19, 19. doi:10.3390/ijms19020565
Wei, Y., Troger, A., Spahiu, V., Perekhvatova, N., Skulachev, M., Petrov, A., et al. (2019). The Role of SKQ1 (Visomitin) in Inflammation and Wound Healing of the Ocular Surface. Ophthalmol. Ther. 8, 63–73. doi:10.1007/s40123-018-0158-2
Yamamoto, K., Miyoshi-Koshio, T., Utsuki, Y., Mizuno, S., and Suzuki, K. (1991). Virucidal Activity and Viral Protein Modification by Myeloperoxidase: a Candidate for Defense Factor of Human Polymorphonuclear Leukocytes against Influenza Virus Infection. J. Infect. Dis. 164, 8–14. doi:10.1093/infdis/164.1.8
Yamamoto, M., Yamato, E., Shu-Ichi, T., Tashiro, F., Ikegami, H., Yodoi, J., et al. (2008). Transgenic Expression of Antioxidant Protein Thioredoxin in PancreaticβCells Prevents Progression of Type 2 Diabetes Mellitus. Antioxid. Redox Signaling 10, 43–50. doi:10.1089/ars.2007.1586
Zaheer, M., Wang, C., Bian, F., Yu, Z., Hernandez, H., de Souza, R. G., et al. (2018). Protective Role of Commensal Bacteria in Sjögren Syndrome. J. Autoimmun. 93, 45–56. doi:10.1016/j.jaut.2018.06.004
Zalewska, A., Knaś, M., Gińdzieńska-Sieśkiewicz, E., Waszkiewicz, N., Klimiuk, A., Litwin, K., et al. (2014). Salivary Antioxidants in Patients with Systemic Sclerosis. J. Oral Pathol. Med. 43, 61–68. doi:10.1111/jop.12084
Zamocky, M., Jakopitsch, C., Furtmüller, P. G., Dunand, C., and Obinger, C. (2008). The Peroxidase-Cyclooxygenase Superfamily: Reconstructed Evolution of Critical Enzymes of the Innate Immune System. Proteins 72, 589–605. doi:10.1002/prot.21950
Zernii, E. Y., Gancharova, O. S., Baksheeva, V. E., Golovastova, M. O., Kabanova, E. I., Savchenko, M. S., et al. (2017). Mitochondria-Targeted Antioxidant SkQ1 Prevents Anesthesia-Induced Dry Eye Syndrome. Oxid Med. Cell Longev 2017, 9281519. doi:10.1155/2017/9281519
Zhang, Y., Catts, V. S., and Shannon Weickert, C. (2017). Lower Antioxidant Capacity in the Prefrontal Cortex of Individuals with Schizophrenia. Aust. N. Z. J. Psychiatry 52 (7), 690–698. doi:10.1177/0004867417728805
Zhou, T., Zong, R., Zhang, Z., Zhu, C., Pan, F., Xiao, X., et al. (2012). SERPINA3K Protects against Oxidative Stress via Modulating ROS Generation/degradation and KEAP1-NRF2 Pathway in the Corneal Epithelium. Invest. Ophthalmol. Vis. Sci. 53, 5033–5043. doi:10.1167/iovs.12-9729
Keywords: lacrimal gland, ocular surface, oxidative stres, dry eye, lacrimal functional unit (LFU)
Citation: Lemos CN, Silva LECMd, Faustino JF, Fantucci MZ, Murashima AdAB, Adriano L, Alves M and Rocha EM (2022) Oxidative Stress in the Protection and Injury of the Lacrimal Gland and the Ocular Surface: are There Perspectives for Therapeutics?. Front. Cell Dev. Biol. 10:824726. doi: 10.3389/fcell.2022.824726
Received: 29 November 2021; Accepted: 16 February 2022;
Published: 11 March 2022.
Edited by:
Claudia Fiorillo, University of Florence, ItalyReviewed by:
Mee Kum Kim, Seoul National University, South KoreaChuanqing Ding, SightGlass Vision, United States
Copyright © 2022 Lemos, Silva, Faustino, Fantucci, Murashima, Adriano, Alves and Rocha. This is an open-access article distributed under the terms of the Creative Commons Attribution License (CC BY). The use, distribution or reproduction in other forums is permitted, provided the original author(s) and the copyright owner(s) are credited and that the original publication in this journal is cited, in accordance with accepted academic practice. No use, distribution or reproduction is permitted which does not comply with these terms.
*Correspondence: Camila Nunes Lemos, camilanl.usp@gmail.com