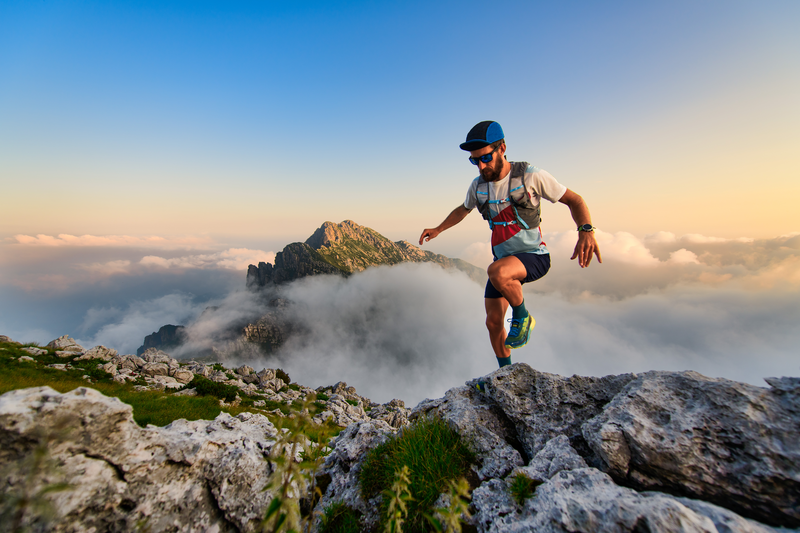
94% of researchers rate our articles as excellent or good
Learn more about the work of our research integrity team to safeguard the quality of each article we publish.
Find out more
ORIGINAL RESEARCH article
Front. Cell Dev. Biol. , 26 January 2022
Sec. Cell Death and Survival
Volume 10 - 2022 | https://doi.org/10.3389/fcell.2022.818513
Background: Remifentanil protects against intestinal ischemia/reperfusion (I/R) injury; however, its exact mechanism remains to be elucidated. The objective of this study was to investigate the underlying molecular mechanism of remifentanil in intestinal I/R injury in mice.
Methods: We evaluated the intestine-protective effect of remifentanil in adult male mice with 45 min superior mesenteric artery occlusion followed by 4 h reperfusion by determining the following: intestinal Chiu’s scores, diamine oxidase, and intestinal fatty acid binding protein in serum; the apoptotic index, lipid peroxidation product malondialdehyde (MDA), and superoxide dismutase (SOD) activity in the intestinal mucosa; and the intestinal mRNA and protein expressions of Bip, CHOP, caspase-12, and cleaved caspase-3, reflecting endoplasmic reticulum (ER) stress. Furthermore, conditional knockout mice, in which the protein disulfide isomerase A3 (PDIA3) gene was deleted from the intestinal epithelium, and SB203580 (a selective p38MAPK inhibitor) were used to determine the role of PDIA3 and p38MAPK in I/R progression and intestinal protection by remifentanil.
Results: Our data showed that intestinal I/R induced obvious oxidative stress and endoplasmic reticulum stress–related cell apoptosis, as evidenced by an increase in the intestinal mucosal malondialdehyde, a decrease in the intestinal mucosal SOD, and an increase in the apoptotic index and the mRNA and protein expression of Bip, CHOP, caspase-12, and cleaved caspase-3. Remifentanil significantly improved these changes. Moreover, the deletion of intestinal epithelium PDIA3 blocked the protective effects of remifentanil. SB203580 also abolished the intestinal protection of remifentanil and downregulated the mRNA and protein expression of PDIA3.
Conclusion: Remifentanil appears to act via p38MAPK to protect the small intestine from intestinal I/R injury by its PDIA3-mediated antioxidant and anti-ER stress properties.
Intestinal ischemia/reperfusion (I/R) injury, a serious clinical event accompanied by high morbidity and mortality, is one of the main causes of multiple organ failure in the perioperative period (Mallick et al., 2004). Unfortunately, effective treatment strategies and drugs for intestinal I/R injury are still lacking.
Remifentanil is a potent ultrashort-acting opioid analgesic agent that is widely used as a general anesthetic (Scott and Perry, 2005; Komatsu et al., 2007). We (Shen et al., 2016) and others (Cho et al., 2013; Sayan-Ozacmak et al., 2015) have previously demonstrated that remifentanil may protect the intestine against intestinal I/R injury. However, the exact protective mechanisms of remifentanil need to be explored further.
Previously, using two-dimensional gel electrophoresis (2DE) followed by matrix-assisted laser desorption/ionization time-of-flight mass spectrometry (MALDI-TOF MS), we identified 16 differentially expressed proteins (>1.5-fold change) in the intestinal mucosa after intestinal I/R (Liu et al., 2010). Protein disulfide isomerase A3 (PDIA3, also known as ERp57, ERp60, and GRP58) is a protein that is markedly downregulated by intestinal I/R injury but upregulated by ischemia preconditioning. PDIA3 is a classic member of the protein disulfide isomerase family and mainly exists in the endoplasmic reticulum (ER) (Frickel et al., 2004). Prior research (Yoo et al., 2017) has also demonstrated that PDIA3 protein levels are significantly altered during transient spinal cord ischemia, which further was identified as a candidate therapeutic agent against spinal cord ischemic damage. Nevertheless, to date, few reports have illustrated the intestinal protective properties of PDIA3 against ischemic challenges.
ER stress can be promoted by various stressors, including hypoxia, acidosis, ATP depletion, oxidative stress, and calcium overload (Ajoolabady et al., 2021; Ren et al., 2021). Furthermore, sustained and severe ER stress leads to ER stress–related cell apoptosis, which is characterized by the upregulation of Bip, CHOP, caspase-12, and cleaved caspase-3 (Folch-Puy et al., 2016). As a multifunctional protein, PDIA3 not only plays an important role in regulating cell apoptosis induced by ER stress but also confers protective effects against oxidative stress (Coe and Michalak, 2010; Yoo et al., 2017; Yoo et al., 2019). Meanwhile, many studies (Cho et al., 2013; Zhao et al., 2013a; Qu et al., 2019; Lewinska et al., 2020; Zhou et al., 2020) have found that remifentanil reduces hypoxia/reoxygenation (H/R) or I/R-mediated oxidative stress. Previous studies (Chen et al., 2016; Chen et al., 2017) have also reported that remifentanil may protect cardiomyocytes from H/R injury by inhibiting ERS-induced apoptosis. Therefore, we explored the mechanisms of remifentanil in intestinal protection and focused on its PDIA3-mediated antioxidant and antiapoptotic properties.
p38 mitogen–activated protein kinase (p38MAPK) is a member of the MAPK family and is activated by a variety of cellular stresses, including intestinal I/R challenge (Fu et al., 2003). The p38MAPK signaling pathway is also involved in intracellular signal transduction pathways that mediate oxidative and ER stress (Imarisio et al., 2017; Han et al., 2020). Upon activation, p38MAPK is translocated to the nucleus, where it is phosphorylated and activates different transcription factors and transactivates target genes. Previously, Zhou et al. (2009) found that PDIA3 expression was decreased by PDIA3 siRNA in human breast cancer cells, but this inhibitory effect was abolished by a p38MAPK-specific inhibitor (SB203580), suggesting that p38MAPK is involved in the regulation of PDIA3 expression. However, further studies are needed to determine whether PDIA3 is regulated by p38MAPK signal transduction during intestinal protection of remifentanil against intestinal I/R injury.
Based on the aforementioned studies, we hypothesized that remifentanil could upregulate PDIA3 expression by activating p38MAPK to inhibit intestinal I/R-induced oxidative and ER stress.
The current study was approved by the Animal Care Committee at Sun Yat-sen University (Guangzhou, China), and the animal experimental protocols were performed in accordance with the National Institutes of Health guidelines. Adult male C57BL/6N mice (8–12 weeks old, 21–30 g) were obtained from the Animal Center of Sun Yat-sen University (Guangzhou, China). Flox mice (PDIA3 flox/flox) and conditional knockout (cKO) mice (PDIA3 flox/flox; pVillin-Cre) were purchased from Cyagen Biosciences Inc (Guangzhou, China).
All mice were anesthetized with pentobarbital (30 mg/kg, intraperitoneal injection), and the intestinal I/R model was established as described previously (Li et al., 2017a). Briefly, a 2-cm midline laparotomy was performed, and the small intestine was exteriorized. Then, the superior mesenteric artery (SMA) was identified and occluded for 45 min with an atraumatic microvascular clip, followed by removal of the clip for 4 h of reperfusion. Body temperature was maintained at 37.5 ± .5°C with warming pads during the experimental period.
Remifentanil was dissolved in 154 mM NaCl (normal saline). A selective p38MAPK inhibitor SB203580 was dissolved in dimethyl sulfoxide (DMSO), and the concentration was 40 μg/ml (Abhilasha et al., 2019). All drugs were administered via intraperitoneal injections (i.p.).
Experiment 1 To explore the role of PDIA3 in the intestinal protection of remifentanil.
Flox mice (PDIA3 flox/flox) were randomly assigned to FS, FIR, and FRF groups and cKO mice (PDIA3 flox/flox; pVillin-Cre) were randomly assigned to CS, CIR, and CRF groups (n = 5 each) as follows (Figure 1A):
1. FS: normal saline (10 ml/kg, i.p., 5 min before sham surgical preparation) + sham surgical preparation (isolation of SMA without occlusion).
2. FIR: normal saline + intestinal I/R (SMA occlusion for 45 min followed by 4 h reperfusion).
3. FRF: remifentanil (.1 μg/ml, 10 ml/kg, i.p., 5 min before I/R) + intestinal I/R.
4. CS: normal saline + sham surgical preparation.
5. CIR: normal saline + intestinal I/R.
6. CRF: remifentanil + intestinal I/R.
FIGURE 1. Experimental protocols. (A) Experiment 1. (B) Experiment 2. NS = normal saline; RF = remifentanil; SB203580, the selective p38MAPK inhibitor; DMSO, the solvent of SB203580.
Experiment 2 To explore the role of p38MAPK in the intestinal protection of remifentanil.
Wild mice were randomly assigned to one of seven groups (n = 8 each) as follows (Figure 1B):
1. Sham: normal saline (10 ml/kg, i.p., 20 min before sham surgical preparation) + normal saline (10 ml/kg, i.p., 5 min before sham surgical preparation) + sham surgical preparation.
2. IR: normal saline + normal saline + intestinal I/R.
3. RF: normal saline + remifentanil (.1 μg/ml, 10 ml/kg, i.p., 5 min before I/R) + intestinal I/R.
4. SIR: SB203580 (selective p38MAPK inhibitor, 40 μg/ml, 10 ml/kg, i.p., 20 min before I/R) + normal saline + intestinal I/R.
5. SRF: SB203580 + remifentanil + intestinal I/R.
6. DIR: DMSO (the vehicle control of SB203580, 2%, 10 ml/kg, i.p., 20 min before I/R) + normal saline + intestinal I/R.
7. DRF: DMSO + remifentanil + intestinal I/R.
At the end of the reperfusion period, blood was sampled by eyeball extirpation and centrifuged at 3,500 rpm for 15 min at 4°C, and the clear supernatants were collected for subsequent measurements of serum biochemical markers. A 10-cm segment of the ileum was harvested from the ileocecal valve and divided into two segments. The first 1 cm was fixed in 4% formaldehyde and embedded in paraffin for histological analysis and evaluation of apoptosis of the intestinal mucosal epithelium. The remaining intestinal fragment (9 cm long) was irrigated with cold normal saline. The intestinal mucosa was dried with filter paper and preserved at −80°C after being scraped off gently.
The segment of the small intestine was stained using hematoxylin and eosin. The stained (4 μm) sections were evaluated independently by two pathologists who were blinded to the study groups according to Chiu’s scores for the intestine, as previously described (Shen et al., 2016).
To further assess intestinal injury, circulating DAO and iFABP levels were measured. The markers were measured using ELISA kits (Enzyme-Linked Biotechnology Co., Ltd., Shanghai, China) according to the manufacturer’s instructions. The results were expressed in pg/ml.
The lipid peroxidation product, MDA, in the intestinal mucosa was measured using ELISA kits (Enzyme-Linked Biotechnology Co., Ltd., Shanghai, China). The results were expressed as nmol/g. SOD activity was evaluated by inhibition of nitroblue tetrazolium reduction by superoxide anion generated by the xanthine/xanthine oxidase system using a commercial assay kit (Enzyme-Linked Biotechnology Co., Ltd., Shanghai, China). The results were expressed as ng/g.
The ileal segments were fixed in 4% formaldehyde and embedded in paraffin. To assess apoptosis of the intestinal mucosal epithelial cells, the tissue sections were stained using the TUNEL method, as described previously (Shen et al., 2016). Cells with obvious dark brown staining of the nucleus and nuclear membrane were considered TUNEL-positive. Five randomly chosen observation fields were examined on each slide by two pathologists who were blinded to the study groups. The apoptotic index (TUNEL-positive cell number/total cell number × 100%) was calculated for each slide.
RNA was extracted from the epithelium of the small intestine using TRIzol reagent (Invitrogen). cDNA was generated using the HiScript II Q RT SuperMix for qPCR (R222-01; Vazyme, Nanjing, China). RT-PCR analysis was performed on cDNA with the ChamQ universal SYBR qPCR Master Mix (Q711-02; Vazyme, Nanjing, China), and reactions were run on the Bio-Rad CFX96 Real-Time System (Bio-Rad, Hercules, CA). Gene expression was displayed as the fold increase, and β-actin was used as the endogenous standard.
The primer sequences used were as follows: PDIA3, forward 5′- AGAGGCTTGCCCCTGAGTAT-3′, reverse 5′-GCTGACAATTCCATCAGCAGT -3′; Bip, forward 5′-TGTCGCCCTCAGACCAGAA-3′, reverse 5′-GAACACA CCGACGCAGGAATA-3′; CHOP, forward 5′-GCAGCGACAGAGCCAGAATA-3′, reverse 5′-ATGTGCGTGTGACCTCTGTT-3′; caspase-12, forward 5′-GACAT GCTGGATGGGGTTTT-3′, reverse 5′-CCTCTACTTTTCTCTTGGATTCTGA-3′; and β-actin, forward 5′-AGCCATGTACGTAGCCATCC-3′, reverse 5′-GCTGTG GTGGTGAAGCTGTA-3′.
The intestinal mucosa was harvested and suspended in lysis buffer. The insoluble cell debris was removed by centrifugation (12,000 rpm for 15 min at 4°C). The supernatants were collected, and the protein concentration of the extract was determined using a bicinchoninic acid protein assay kit (Kangcheng BioTech, Shanghai, China). The samples were then boiled for 10 min, loaded onto polyacrylamide gels for electrophoresis, and then transferred onto polyvinylidene fluoride membranes for immunoblotting. To prevent nonspecific binding, the membranes were first blocked with 5% (w/v) nonfat milk in Tris-buffered saline–Tween 20 for 1 h at room temperature and then incubated with antibodies against p-p38 (1:1,000, 4511S, Cell Signaling Technology, Danvers, United States), p38 (1:1000, 8690S, Cell Signaling Technology, Danvers, United States), PDIA3 (1:2000, ab13506, Abcam, Cambridge, United Kingdom), Bip (1:1000, 3177S, Cell Signaling Technology, Danvers, United States), CHOP (1:2500, 2895, Cell Signaling Technology, Danvers, United States), caspase-12 (1:200, sc-21747, Santa Cruz Biotechnology, Oregon, United States), cleaved caspase-3 (1:1,000, 9664S, Cell Signaling Technology, Danvers, United States), or β-actin (1:1,000, 4,970, Cell Signaling Technology, Danvers, United States) overnight at 4°C, followed by horseradish peroxidase (HRP)–labeled goat anti-mouse IgG (1:5,000, SA00001-1, Proteintech, Chicago, United States) or HRP-labeled goat anti-rabbit IgG (1:5,000, SA00001-2, Proteintech, Chicago, United States) or HRP-labeled goat anti-rat IgG (1:5,000, SA00001-15, Proteintech, Chicago, United States) for 1 h at room temperature. The blots were visualized using the LI-COR Odyssey imaging system (Shen et al., 2016) and quantified using ImageJ software (National Institutes of Health, Bethesda, MD, United States).
Data were presented as the mean ± standard deviation (SD) and analyzed with GraphPad Prism 8.0 software (La Jolla, CA, United States). The significance of the data was evaluated using one-way ANOVA (Tukey’s post hoc multiple comparison test for multiple groups). Differences were considered statistically significant at p < .05.
Representative mucosal morphological changes are presented in Figures 2A,B. Normal-appearing morphology with structural integrity of the villi and glands was revealed in the sham-operated mice (FS, CS, and sham groups in Figures 2A,B). In contrast, 45 min of ischemia followed by 4 h of reperfusion caused acute intestinal damage with marked edema and mucosal villi disruption, infiltration of inflammatory cells, and increased gaps between epithelial cells (FIR, CIR, and IR groups in Figures 2A,B). Mild injury to the intestinal mucosal tissues was observed in the FRF and RF groups (Figures 2A,B). Mucosal injury was quantified according to Chiu’s scores (Figures 2C,F). Chiu’s scores were significantly higher in the injury groups than those in the sham-operation group (FIR vs. FS and IR vs. sham, p < .05) and were attenuated by remifentanil (FRF vs. FIR and RF vs. IR, p < .05).
FIGURE 2. Ischemia/reperfusion injury in the intestine. Groups are the same as in Figure 1. Histopathologic changes of the intestinal mucosa were assessed by hematoxylin and eosin staining (A,B), and intestinal injury was evaluated using Chiu’s scores (C,F) under light microscopy (×200, scale bar: 100 μm), diamine oxidase activity (DAO) (D,G), and intestinal fatty acid–binding protein (iFABP) (E,H) in serum. In the FS, CS, and sham groups, normal intestinal villi and glands are seen and shown by black arrows. In the FIR, CIR, CRF, IR, SIR, SRF, and DIR groups, injured intestinal gland, disintegrated intestinal villi, and significantly increased gap between epithelial cells are observed and shown by red arrows, indicating severe mucosal damage. In the FRF, RF, and DRF groups, no significant edema in intestinal villi is seen, and extension of the subepithelial space (Gruenhagen space) was developed, which are shown by blue arrows, indicating slight mucosal damage. Data are expressed as mean ± SD, n = 5–8. The results were compared by ANOVA with Tukey’s post hoc test. *P < .05.
As an index of small intestinal mucosal injury, the serum levels of DAO (Figures 2D,G) and iFABP (Figures 2E,H) in the injury groups were markedly higher than those in the sham-operation group (FIR vs. FS and IR vs. Sham, p < .05) and were reduced by remifentanil (FRF vs. FIR and RF vs. IR, p < .05).
Accompanying mucosal morphological changes, changes in Chiu’s scores and serum DAO and iFABP concentrations further indicated that remifentanil could protect the intestine against intestinal I/R injury.
As shown in Figures 3A,B, TUNEL-positive mucosal epithelial cells had nuclei that were stained dark brown. Detailed descriptions of mucosal epithelial apoptosis in different groups are showed in the legend of Figure 3. The apoptotic indices among the different groups (Figures 3C,D) were significantly higher in the injury model groups than those in the sham-operation groups (FIR vs. FS and IR vs. Sham, p < .05). Administration of remifentanil markedly decreased the apoptotic index (FRF vs. FIR and RF vs. IR, p < .05).
FIGURE 3. TUNEL assay of the intestinal mucosal cell apoptosis by light microscopy (×200 and ×400, scale bar: 100 μm). (A,B) The intestinal mucosal cell apoptosis changes assessed by TUNEL staining among the groups. Groups are the same as in Figure 1. Apoptotic nuclei are stained dark brown. In the FS, CS, and sham groups, few apoptotic epithelial cells are observed at the villous. In the FIR, CIR, CRF, IR, SIR, SRF, and DIR groups, notable destruction of the villi is evident, and apoptotic enterocytes are present in the upper regions of the villi. In addition, large numbers of detached epithelial cells with dark brown nuclei are seen in the enteric cavity. In the FRF, RF, and DRF groups, the damage of villi is slight, and there are fewer apoptotic cells than in the FIR and IR groups. (C,D) Apoptotic indices among the groups. Data are expressed as mean ± SD, n = 5–8. The results were compared by ANOVA with Tukey’s post hoc test. *P < .05.
As shown in Figures 4B–D,F–H, after 4 h of reperfusion, the mRNA levels of the ER stress–related genes Bip, CHOP, and caspase-12 were significantly upregulated, indicating enhanced ER stress in the intestinal tissues (FIR vs. FS and IR vs. Sham, p < .05). These mRNA levels were markedly reduced by remifentanil (FRF vs. FIR and RF vs. IR, p < .05).
FIGURE 4. Changes in intestinal mRNA expression of the relevant proteins. Groups are the same as in Figure 1. The changes in PDIA3, Bip, CHOP, and caspase-12 mRNA levels in the intestine are shown in (A–H) (n = 5–6). Data are expressed as mean ± SD. The results were compared by ANOVA with Tukey’s post hoc test. *P < .05.
Representative Western blot bands of relevant proteins in the intestinal mucosa are shown in Figures 5A,B. Quantitative changes in protein concentrations (Figures 5C–M) are shown by densitometry analysis from Western blotting assay. Compared to the sham-operation group, there was a notable upregulation in the protein expression of Bip, CHOP, caspase-12, and cleaved caspase-3 after I/R injury (FIR vs. FS and IR vs. Sham, p < .05). In addition, remifentanil significantly decreased the expressions of these proteins (FRF vs. FIR and RF vs. IR, p < .05).
FIGURE 5. Changes in relevant intestinal protein expression. Groups are the same as in Figure 1. Representative Western blot bands (A,B) show p-p38MAPK, p38MAPK, PDIA3, Bip, CHOP, caspase-12, cleaved caspase-3 and β-actin expression in the intestinal mucosa. Quantitative changes in protein concentrations (C–M) are shown by densitometry analysis from the Western blotting assay (n = 5–6). Data are expressed as mean ± SD. The results were compared by ANOVA with Tukey’s post hoc test. *P < .05.
Accompanying the changes in intestinal mucosal epithelial apoptosis (Figure 3) and mRNA (Bip, CHOP, and caspase-12) expressions (Figure 4), the changes in protein (Bip, CHOP, caspase-12, and cleaved caspase-3) expressions (Figure 5) further indicated that remifentanil protected the intestine against intestinal I/R injury by inhibiting ER stress–related cell apoptosis.
As shown in Figures 6A,C, MDA levels in the intestinal mucosa after I/R injury were significantly increased (FIR vs. FS and IR vs. sham, p < .05). Conversely, the SOD activity obviously decreased as shown in Figures 6B,D (FIR vs. FS and IR vs. sham, p < .05). In addition, remifentanil significantly reduced MDA levels and notably enhanced SOD levels in the intestinal mucosa (FRF vs. FIR and RF vs. IR, p < .05). These changes in intestinal mucosal MDA and SOD concentrations demonstrated that remifentanil could alleviate oxidative stress induced by intestinal I/R.
FIGURE 6. Changes in intestinal mucosa malondialdehyde (MDA) (A,C) and superoxide dismutase (SOD) (B,D). Groups are the same as in Figure 1. Data are expressed as mean ± SD, n = 5–8. The results were compared by ANOVA with Tukey’s post hoc test. *P < .05.
As shown in Figures 2C–E, Chiu’s scores and serum DAO and iFABP concentrations in the CRF group were similar to those in the FIR and CIR groups (CRF vs. FIR and CRF vs. CIR, p > .05) and significantly higher than those in the FRF group (CRF vs. FRF, p < .05), indicating that the deletion of PDIA3 from intestinal mucosa completely abrogated the intestinal protective effect of remifentanil.
As shown in Figures 3C, 4B–D–D, 5D–G–G, the apoptotic index and mRNA (Bip, CHOP, and caspase-12) and protein (Bip, CHOP, caspase-12, and cleaved caspase-3) expressions in the CRF group were markedly higher than those in the FRF group (CRF vs. FRF, p < .05), and similar to those in the FIR and CIR groups (CRF vs. FIR and CRF vs. CIR, p > .05), indicating that remifentanil upregulated PDIA3 expression to protect against intestinal I/R injury via inhibition of ER stress–related cell apoptosis.
As shown in Figures 6A,B, the intestinal mucosal MDA and SOD concentrations in the CRF group were similar to those in the FIR and CIR groups (CRF vs. FIR and CRF vs. CIR, p > .05). There was an obvious increase in intestinal mucosal MDA and a marked decrease in the intestinal mucosal SOD in the CRF group compared to those in the FRF group (CRF vs. FRF, p < .05), suggesting that the antioxidant stress property of PDIA3 contributed to the intestinal protection of remifentanil.
Meanwhile, there were no statistical differences in the abovementioned variables in comparison between the FS and CS groups and between the FIR and CIR groups (FS vs. CS and FIR vs. CIR, p > .05).
As showed in Figures 2F–H–H, 3D, 4F–H–H, 5J–MJ–M5J–M, 6C–D–D, there was an obvious increase in Chiu’s scores, serum DAO and iFABP concentration, apoptotic index, mRNA (Bip, CHOP, and caspase-12) protein (Bip, CHOP, caspase-12, and cleaved caspase-3) expressions, and intestinal mucosal MDA concentration, with a marked decrease in intestinal mucosal SOD, in the SRF group compared with those in the RF and DRF groups (SRF vs. RF and SRF vs. DRF, p < .05). These variables in the SRF group were similar to those in the IR, SIR, and DIR groups (SRF vs. IR, SRF vs. SIR, and SRF vs. DIR, p > .05). These results indicate that pretreatment with SB203580 (a selective p38MAPK inhibitor) abolished the protective effects of remifentanil on intestinal I/R injury.
As shown in Figures 4, 5, the p-p38MAPK protein expression and the mRNA and protein expression of PDIA3 in the intestinal mucosa were significantly lower in the SRF than those in the RF and DRF groups (SRF vs. RF and SRF vs. DRF, p < .05), and similar to those in the IR, SIR, and DIR groups (SRF vs. IR, SRF vs. SIR, and SRF vs. DIR, p > .05), suggesting that activation of p38MAPK may upregulate PDIA3 expression, contributing to the protective effect of remifentanil.
Meanwhile, SB203580 or DMSO alone had no effect on the abovementioned variables in the comparison between the SIR or DIR group and IR group, or between the DRF and RF groups (SIR vs. IR, DIR vs. IR, and DRF vs. RF, p > .05).
This study presents three important findings. First, a single dose of remifentanil (1 μg/kg) administered intraperitoneally before intestinal ischemia protected the intestine from I/R injury by reducing oxidative and ER stress. Second, we generated pVillin-Cre–mediated PDIA3 cKO mice (PDIA3 flox/flox; pVillin-Cre), in which the PDIA3 gene was deleted specifically in the intestinal epithelium, and demonstrated that PDIA3 with antioxidant and anti-ER stress properties played a key role in the intestinal protection of remifentanil. Third, after applying a selective p38MAPK inhibitor (SB203580), remifentanil appeared to protect against intestinal I/R injury via p38MAPK to enhance PDIA3 expression.
Several studies have provided evidence that remifentanil can mediate protective effects against intestinal I/R injury. One study (Sayan-Ozacmak et al., 2015) showed that pretreatment with remifentanil can remarkably attenuate the intestinal I/R injury, possibly by lowering lipid peroxidation and leukocyte infiltration. Another study (Cho et al., 2013) demonstrated that a single bolus of remifentanil inhibited local oxidative stress and systemic inflammation and protected against I/R injury in the small intestine. We (Shen et al., 2016) previously found that remifentanil preconditioning attenuated both intestinal I/R injury in vivo and IEC-6 cell OGD/R injury in vitro by inhibiting intestinal mucosal epithelial cell apoptosis via δ- and μ-opioid receptors but not δ-opioid receptors. A growing body of literature has shown that both oxidative and ER stress contribute significantly to epithelial damage during intestinal I/R injury (Bi et al., 2020; Ding et al., 2020; Hartmann et al., 2017; Liu et al., 2019). As a result, oxidative stress in the ER caused by intestinal I/R accelerates the accumulation of unfolded and misfolded proteins, ultimately leading to apoptosis (Jiang et al., 2020; Yin et al., 2020; Yoo et al., 2019). Consistently, in the current study, we found that remifentanil significantly suppressed intestinal I/R-induced oxidative stress, as evidenced by decreased intestinal mucosal MDA and increased intestinal mucosal SOD levels, as well as downregulated ER stress, as evidenced by the decreased mRNA and protein expressions of Bip, CHOP, caspase-12, and cleaved caspase-3 in the intestine.
PDIA3 facilitates isomerization of the disulfide bond in nascent and denatured proteins with a thioredoxin-like domain, thereby playing an important role in ER stress (Hatahet and Ruddock, 2009; Kozlov et al., 2010). In the present study, our results showed that PDIA3 was upregulated after remifentanil administration, which blocked the ER stress–associated apoptotic pathway, as evidenced by a distinctly elevated expression of Bip, CHOP, caspase-12, and cleaved caspase-3 in the intestinal epithelium. These results are mostly consistent with those of a previous study (Yoo et al., 2019) which demonstrated that exogenous PDIA3 protein (Tat-PDIA3) could protect against ischemic damage, likely by attenuating ER stress–induced apoptosis through CHOP. As expected, our results showed that remifentanil significantly promoted PDIA3 upregulation to decrease the lipid peroxidation product MDA while restoring SOD activity in the intestinal mucosa. The antioxidative effect of PDIA3 in the current study concurred with the findings of (Yoo et al., 2017; Yoo et al., 2019), who found that PDIA3 attenuated oxidative damage by ameliorating ischemia-induced lipid peroxidation and reducing antioxidant enzymes.
Growing evidence demonstrates that the p38MAPK family plays an important role in intracellular signal transduction in response to extracellular stimuli, including intestinal I/R challenge (Fu, Xing, et al., 2003; Fu, Yang, et al., 2003; Guo et al., 2016; Li et al., 2021; Li, Xu, et al., 2017; Liu et al., 2020; Ming et al., 2019; Murayama et al., 2006; Santén et al., 2009; Yusof et al., 2009; Zheng et al., 2005). Dexmedetomidine (Liu et al., 2020), propofol (Li et al., 2021), and 6-gingerol (Li, Xu, et al., 2017) have been shown to protect against intestinal I/R injury via p38MAPK inhibition, wherein p38MAPK activation conferred detrimental effects on intestinal I/R progression. However, in our current study, phosphorylation of p38MAPK was significantly downregulated after 45 min of ischemia followed by 4 h of reperfusion but was obviously enhanced by remifentanil treatment, as confirmed by Western blot analysis. Moreover, pretreatment with the p38MAPK-specific inhibitor SB203580 abolished p38MAPK activation and reversed the intestinal protective effects of remifentanil. This finding is partly consistent with that of previous studies. Previous studies (Zhao, Liu, et al., 2013; Zhu et al., 2014) found that hypoxic postconditioning mediated neuroprotection against transient global cerebral ischemia in rats and mice by increasing the phosphorylation of p38MAPK, which may be attributable to mitochondrial translocation of Bcl-2–related protein Bcl-xL. Another study (Guan et al., 2014) provided evidence that cerebral ischemic preconditioning induces ischemic tolerance by activating the p38MAPK signaling pathway in the rat brain. Taken together, these results suggest that remifentanil-induced p38MAPK signaling pathway activation plays an important role in mitigating intestinal I/R injury.
Controversy continues with respect to the functions of p38MAPK phosphorylation. We speculate that these two factors might have contributed to this. First, the duration of ischemia and/or reperfusion varies, meaning that samples from different time points were collected, leading to different levels of phosphorylated p38MAPK. Second, different subtypes of p38MAPK, such as p38α, β, δ, and γ, with different or even opposite functions (Beenstock et al., 2014; Grossi et al., 2014), may be activated at different time points during the progression of I/R.
A previous study (Zhou et al., 2009) found that PDIA3 expression was decreased by PDIA3 siRNA in human breast cancer cells, and this inhibitory effect was abolished by a p38MAPK-specific inhibitor (SB203580), suggesting that the expression of PDIA3 requires the p38 MAPK pathway. In contrast, our study found that activation of p38MAPK promoted PDIA3 expression, as evidenced by the notable downregulation of the mRNA and protein expression of PDIA3 after p38MAPK-specific inhibitor administration. The relationship between p38MAPK and PDIA3 is an interesting area for future studies.
Our study had several limitations. First, it was not designed to determine the effects of remifentanil on long-term mouse survival and gut function, nor was the relationship between opioid receptor activation and p38MAPK/PDIA3 upregulation investigated. Also, only one dose of remifentanil was used to investigate its intestinal protective effects and explore the relevant mechanism. Nevertheless, more doses should be used to further investigate the dose–effect relationship. In addition, no in vitro experiment has been performed to further confirm the ER stress–associated mechanism of remifentanil against intestinal I/R injury. Therefore, more in vitro studies potentially using RNA interference technology may help elucidate this.
Collectively, our study provides evidence that remifentanil promotes PDIA3 expression by activating p38MAPK to inhibit intestinal I/R-induced oxidative and ER stress. PDIA3, a therapeutic candidate protein, may be a new target for the prevention and treatment of intestinal injury after intestinal I/R.
The original contributions presented in the study are included in the article/Supplementary Material; further inquiries can be directed to the corresponding authors.
The animal study was reviewed and approved by the Animal Care Committee at Sun Yat-sen University (Guangzhou, China).
SW and WH conceived and designed the study. JS, YZ, QH, QD, and KL were involved in the whole experiment. JS wrote the manuscript. All authors edited the manuscript and approved the submitted version.
This work was supported by the grants from the National Natural Science Foundation of China (No. 81801887, to JS).
The authors declare that the research was conducted in the absence of any commercial or financial relationships that could be construed as a potential conflict of interest.
All claims expressed in this article are solely those of the authors and do not necessarily represent those of their affiliated organizations, or those of the publisher, the editors, and the reviewers. Any product that may be evaluated in this article, or claim that may be made by its manufacturer, is not guaranteed or endorsed by the publisher.
We thank Yi-Hong Ling (Sun Yat-sen University Cancer Center) for his help with histological analysis.
Abhilasha, K. V., Sumanth, M. S., Chaithra, V. H., Jacob, S. P., Thyagarajan, A., Sahu, R. P., et al. (2019). p38 MAP-Kinase Inhibitor Protects against Platelet-Activating Factor-Induced Death in Mice. Free Radic. Biol. Med. 143, 275–287. doi:10.1016/j.freeradbiomed.2019.08.019
Ajoolabady, A., Wang, S., Kroemer, G., Klionsky, D., Uversky, V., Sowers, J., et al. (2021). ER Stress in Cardiometabolic Diseases: from Molecular Mechanisms to Therapeutics. Endocr. Rev. 42 (6), 839–871. doi:10.1210/endrev/bnab006
Beenstock, J., Ben-Yehuda, S., Melamed, D., Admon, A., Livnah, O., Ahn, N. G., et al. (2014). The P38β Mitogen-Activated Protein Kinase Possesses an Intrinsic Autophosphorylation Activity, Generated by a Short Region Composed of the α-G Helix and MAPK Insert. J. Biol. Chem. 289, 23546–23556. doi:10.1074/jbc.m114.578237
Bi, J., Zhang, J., Ren, Y., Du, Z., Li, T., Wang, T., et al. (2020). Irisin Reverses Intestinal Epithelial Barrier Dysfunction during Intestinal Injury via Binding to the Integrin αVβ5 Receptor. J. Cel Mol Med 24, 996–1009. doi:10.1111/jcmm.14811
Chen, L., Chen, M., Du, J., Wan, L., Zhang, L., and Gu, E. (2016). Hyperglycemia Attenuates Remifentanil Postconditioning-Induced Cardioprotection against Hypoxia/reoxygenation Injury in H9c2 Cardiomyoblasts. J. Surg. Res. 203, 483–490. doi:10.1016/j.jss.2016.03.052
Chen, M., Liu, Q., Chen, L., Zhang, L., and Gu, E. (2017). Remifentanil Postconditioning Ameliorates Histone H3 Acetylation Modification in H9c2 Cardiomyoblasts after Hypoxia/reoxygenation via Attenuating Endoplasmic Reticulum Stress. Apoptosis 22, 662–671. doi:10.1007/s10495-017-1347-5
Cho, S. S., Rudloff, I., Berger, P. J., Irwin, M. G., Nold, M. F., Cheng, W., et al. (2013). Remifentanil Ameliorates Intestinal Ischemia-Reperfusion Injury. BMC Gastroenterol. 13, 69. doi:10.1186/1471-230x-13-69
Coe, H., and Michalak, M. (2010). ERp57, a Multifunctional Endoplasmic Reticulum Resident Oxidoreductase. Int. J. Biochem. Cel Biol. 42, 796–799. doi:10.1016/j.biocel.2010.01.009
Ding, R., Wu, W., Sun, Z., and Li, Z. (2020). AMP-activated Protein Kinase: An Attractive Therapeutic Target for Ischemia-Reperfusion Injury. Eur. J. Pharmacol. 888, 173484. doi:10.1016/j.ejphar.2020.173484
Folch-Puy, E., Panisello, A., Oliva, J., Lopez, A., Castro Benítez, C., Adam, R., et al. (2016). Relevance of Endoplasmic Reticulum Stress Cell Signaling in Liver Cold Ischemia Reperfusion Injury. Int. J. Mol. Sci. 17. doi:10.3390/ijms17060807
Frickel, E.-M., Frei, P., Bouvier, M., Stafford, W. F., Helenius, A., Glockshuber, R., et al. (2004). ERp57 Is a Multifunctional Thiol-Disulfide Oxidoreductase. J. Biol. Chem. 279, 18277–18287. doi:10.1074/jbc.m314089200
Fu, X.-B., Xing, F., Yang, Y., Sun, T., and Guo, B. (2003a). Activation of Phosphorylating-P38 Mitogen-Activated Protein Kinase and its Relationship with Localization of Intestinal Stem Cells in Rats after Ischemia-Reperfusion Injury. Wjg 9, 2036–2039. doi:10.3748/wjg.v9.i9.2036
Fu, X.-B., Yang, Y., Sun, T., Chen, W., Li, J., and Sheng, Z. (2003b). Rapid Mitogen-Activated Protein Kinase by Basic Fibroblast Growth Factor in Rat Intestine after Ischemia/reperfusion Injury. Wjg 9, 1312–1317. doi:10.3748/wjg.v9.i6.1312
Grossi, V., Peserico, A., Tezil, T., and Simone, C. (2014). p38α MAPK Pathway: A Key Factor in Colorectal Cancer Therapy and Chemoresistance. Wjg 20, 9744–9758. doi:10.3748/wjg.v20.i29.9744
Guan, J., Li, H., Lv, T., Chen, D., Yuan, Y., and Qu, S. (2014). Bone Morphogenic Protein-7 Contributes to Cerebral Ischemic Preconditioning Induced-Ischemic Tolerance by Activating P38 Mitogen-Activated Protein Kinase Signaling Pathway. Inflammation 37, 1289–1296. doi:10.1007/s10753-014-9856-7
Guo, W.-l., Wang, J., Liu, C., Yang, F.-b., and Li, S.-w. (2016). Expression of P38 Mitogen-Activated Protein Kinase (p38MAPK) and Pathological Change in Intussusception. Pediatr. Int. 58, 881–886. doi:10.1111/ped.12928
Han, F., Jiang, H., Qu, W., and Rui, Y. J. (2020). KLF11 Protects Chondrocytes via Inhibiting P38 MAPK Signaling Pathway. Eur. Rev. Med. Pharmacol. Sci. 24, 6505–6516. doi:10.26355/eurrev_202006_21634
Hartmann, R. M., Licks, F., Schemitt, E. G., Colares, J. R., do Couto Soares, M., Zabot, G. P., et al. (2017). Protective Effect of Glutamine on the Main and Adjacent Organs Damaged by Ischemia-Reperfusion in Rats. Protoplasma 254, 2155–2168. doi:10.1007/s00709-017-1102-3
Hatahet, F., and Ruddock, L. W. (2009). Protein Disulfide Isomerase: a Critical Evaluation of its Function in Disulfide Bond Formation. Antioxid. Redox Signaling 11, 2807–2850. doi:10.1089/ars.2009.2466
Imarisio, C., Alchera, E., Bangalore Revanna, C., Valente, G., Follenzi, A., Trisolini, E., et al. (2017). Oxidative and ER Stress-dependent ASK1 Activation in Steatotic Hepatocytes and Kupffer Cells Sensitizes Mice Fatty Liver to Ischemia/reperfusion Injury. Free Radic. Biol. Med. 112, 141–148. doi:10.1016/j.freeradbiomed.2017.07.020
Jiang, S., Fan, Q., Xu, M., Cheng, F., Li, Z., Ding, G., et al. (2020). Hydrogen-rich saline Protects Intestinal Epithelial Tight junction Barrier in Rats with Intestinal Ischemia-Reperfusion Injury by Inhibiting Endoplasmic Reticulum Stress-Induced Apoptosis Pathway. J. Pediatr. Surg. 55, 2811–2819. doi:10.1016/j.jpedsurg.2020.01.061
Komatsu, R., Turan, A. M., Orhan-Sungur, M., McGuire, J., Radke, O. C., and Apfel, C. C. (2007). Remifentanil for General Anaesthesia: a Systematic Review. Anaesthesia 62, 1266–1280. doi:10.1111/j.1365-2044.2007.05221.x
Kozlov, G., Määttänen, P., Thomas, D. Y., and Gehring, K. (2010). A Structural Overview of the PDI Family of Proteins. FEBS J. 277, 3924–3936. doi:10.1111/j.1742-4658.2010.07793.x
Lewinska, A., Adamczyk-Grochala, J., Bloniarz, D., Horeczy, B., Zurek, S., Kurowicki, A., et al. (2020). Remifentanil Preconditioning Protects against Hypoxia-Induced Senescence and Necroptosis in Human Cardiac Myocytes In Vitro. Aging 12, 13924–13938. doi:10.18632/aging.103604
Li, L., Shu, F., Wang, X. Q., Wang, F., Cai, L., Zhao, X., et al. (2021). Propofol Alleviates Intestinal Ischemia/reperfusion Injury in Rats through P38 MAPK/NF-κB Signaling Pathway. Eur. Rev. Med. Pharmacol. Sci. 25, 1574–1581. doi:10.26355/eurrev_202102_24867
Li, Y., Wen, S., Yao, X., Liu, W., Shen, J., Deng, W., et al. (2017a). MicroRNA-378 Protects against Intestinal Ischemia/reperfusion Injury via a Mechanism Involving the Inhibition of Intestinal Mucosal Cell Apoptosis. Cell Death Dis 8, e3127. doi:10.1038/cddis.2017.508
Li, Y., Xu, B., Xu, M., Chen, D., Xiong, Y., Lian, M., et al. (2017b). 6-Gingerol Protects Intestinal Barrier from Ischemia/reperfusion-Induced Damage via Inhibition of P38 MAPK to NF-Κb Signalling. Pharmacol. Res. 119, 137–148. doi:10.1016/j.phrs.2017.01.026
Liu, D.-Q., Chen, S.-P., Sun, J., Wang, X.-M., Chen, N., Zhou, Y.-Q., et al. (2019). Berberine Protects against Ischemia-Reperfusion Injury: A Review of Evidence from Animal Models and Clinical Studies. Pharmacol. Res. 148, 104385. doi:10.1016/j.phrs.2019.104385
Liu, K.-X., Li, C., Li, Y.-S., Yuan, B.-l., Xu, M., Xia, Z., et al. (2010). Proteomic Analysis of Intestinal Ischemia/reperfusion Injury and Ischemic Preconditioning in Rats Reveals the Protective Role of Aldose Reductase. Proteomics 10, 4463–4475. doi:10.1002/pmic.201000078
Liu, X.-M., Chen, Q.-H., Hu, Q., Liu, Z., Wu, Q., Liang, S.-S., et al. (2020). Dexmedetomidine Protects Intestinal Ischemia-Reperfusion Injury via Inhibiting P38 MAPK Cascades. Exp. Mol. Pathol. 115, 104444. doi:10.1016/j.yexmp.2020.104444
Mallick, I. H., Yang, W., Winslet, M. C., and Seifalian, A. M. (2004). REVIEW: Ischemia-Reperfusion Injury of the Intestine and Protective Strategies against Injury. Dig. Dis. Sci. 49, 1359–1377. doi:10.1023/b:ddas.0000042232.98927.91
Ming, Y.-C., Chao, H.-C., Chu, S.-M., and Luo, C.-C. (2019). Heparin-binding Epidermal Growth Factor-like Growth Factor (HB-EGF) Protected Intestinal Ischemia-Reperfusion Injury through JNK and p38/MAPK-dependent Pathway for Anti-apoptosis. Pediatr. Neonatal. 60, 332–336. doi:10.1016/j.pedneo.2018.08.003
Murayama, T., Tanabe, M., Matsuda, S., Shimazu, M., Kamei, S., Wakabayashi, G., et al. (2006). JNK (C-Jun NH2 Terminal Kinase) and P38 during Ischemia Reperfusion Injury in the Small Intestine. Transplantation 81, 1325–1330. doi:10.1097/01.tp.0000209167.48030.6b
Qu, Z. J., Qu, Z. J., Zhou, H. B., Xu, C. S., Zhang, D. Z., and Wang, G. (2019). Protective Effect of Remifentanil on Myocardial Ischemia-Reperfusion Injury through Fas Apoptosis Signaling Pathway. Eur. Rev. Med. Pharmacol. Sci. 23, 5980–5986. doi:10.26355/eurrev_201907_18344
Ren, J., Bi, Y., Sowers, J. R., Hetz, C., and Zhang, Y. (2021). Endoplasmic Reticulum Stress and Unfolded Protein Response in Cardiovascular Diseases. Nat. Rev. Cardiol. 18, 499–521. doi:10.1038/s41569-021-00511-w
Santén, S., Mihaescu, A., Laschke, M. W., Menger, M. D., Wang, Y., Jeppsson, B., et al. (2009). p38 MAPK Regulates Ischemia-Reperfusion-Induced Recruitment of Leukocytes in the colon. Surgery 145, 303–312. doi:10.1016/j.surg.2008.10.011
Sayan-Ozacmak, H., Ozacmak, V. H., Turan, I., Barut, F., and Hanci, V. (2015). Pretreatment with Remifentanil Protects against the Reduced-Intestinal Contractility Related to the Ischemia and Reperfusion Injury in Rat. Braz. J. Anesthesiology (English Edition) 65, 483–490. doi:10.1016/j.bjane.2013.09.007
Scott, L. J., and Perry, C. M. (2005). Spotlight on Remifentanil for General Anaesthesia1. CNS drugs 19, 1069–1074. doi:10.2165/00023210-200519120-00010
Shen, J.-T., Li, Y.-S., Xia, Z.-Q., Wen, S.-H., Yao, X., Yang, W.-J., et al. (2016). Remifentanil Preconditioning Protects the Small Intestine against Ischemia/reperfusion Injury via Intestinal δ- and μ-opioid Receptors. Surgery 159, 548–559. doi:10.1016/j.surg.2015.07.028
Yin, M., Li, Z.-H., Wang, C., Li, Y., Zhang, H., Du, H.-B., et al. (2020). Stellate Ganglion Blockade Repairs Intestinal Mucosal Barrier through Suppression of Endoplasmic Reticulum Stress Following Hemorrhagic Shock. Int. J. Med. Sci. 17, 2147–2154. doi:10.7150/ijms.47662
Yoo, D. Y., Cho, S. B., Jung, H. Y., Kim, W., Choi, G.-M., Won, M.-H., et al. (2017). Tat-protein Disulfide-Isomerase A3: a Possible Candidate for Preventing Ischemic Damage in the Spinal Cord. Cel Death Dis 8–e3075. doi:10.1038/cddis.2017.473
Yoo, D. Y., Cho, S. B., Jung, H. Y., Kim, W., Lee, K. Y., Kim, J. W., et al. (2019). Protein Disulfide-Isomerase A3 Significantly Reduces Ischemia-Induced Damage by Reducing Oxidative and Endoplasmic Reticulum Stress. Neurochem. Int. 122, 19–30. doi:10.1016/j.neuint.2018.11.002
Yusof, M., Kamada, K., Kalogeris, T., Gaskin, F. S., and Korthuis, R. J. (2009). Hydrogen Sulfide Triggers Late-phase Preconditioning in Postischemic Small Intestine by an NO- and P38 MAPK-dependent Mechanism. Am. J. Physiology-Heart Circulatory Physiol. 296, H868–H876. doi:10.1152/ajpheart.01111.2007
Zhao, G., Shen, X., Nan, H., Yan, L., Zhao, H., Yu, J., et al. (2013a). Remifentanil Protects Liver against Ischemia/reperfusion Injury through Activation of Anti-apoptotic Pathways. J. Surg. Res. 183, 827–834. doi:10.1016/j.jss.2013.02.058
Zhao, L., Liu, X., Liang, J., Han, S., Wang, Y., Yin, Y., et al. (2013b). Phosphorylation of P38 MAPK Mediates Hypoxic Preconditioning-Induced Neuroprotection against Cerebral Ischemic Injury via Mitochondria Translocation of Bcl-xL in Mice. Brain Res. 1503, 78–88. doi:10.1016/j.brainres.2013.01.051
Zheng, S.-Y., Fu, X., Xu, J., Zhao, J., Sun, T., and Chen, W. (2005). Inhibition of P38 Mitogen-Activated Protein Kinase May Decrease Intestinal Epithelial Cell Apoptosis and Improve Intestinal Epithelial Barrier Function after Ischemia- Reperfusion Injury. Wjg 11, 656–660. doi:10.3748/wjg.v11.i5.656
Zhou, Q.-M., Zhang, H., Lu, Y.-Y., Wang, X.-F., and Su, S.-B. (2009). Curcumin Reduced the Side Effects of Mitomycin C by Inhibiting GRP58-Mediated DNA Cross-Linking in MCF-7 Breast Cancer Xenografts. Cancer Sci. 100, 2040–2045. doi:10.1111/j.1349-7006.2009.01297.x
Zhou, Q., Song, J., Wang, Y., and Lin, T. (2020). Remifentanil Attenuates Cardiac Dysfunction, Lipid Peroxidation and Immune Disorder in Rats with Isoproterenol-Induced Myocardial Injury via JNK/NF-KB P65 Inhibition. Ann. Transl Med. 8, 551. doi:10.21037/atm-20-3134
Keywords: remifentanil, PDIA3, p38MAPK, oxidative stress, endoplasmic reticulum stress
Citation: Shen J, Zhan Y, He Q, Deng Q, Li K, Wen S and Huang W (2022) Remifentanil Promotes PDIA3 Expression by Activating p38MAPK to Inhibit Intestinal Ischemia/Reperfusion-Induced Oxidative and Endoplasmic Reticulum Stress. Front. Cell Dev. Biol. 10:818513. doi: 10.3389/fcell.2022.818513
Received: 19 November 2021; Accepted: 06 January 2022;
Published: 26 January 2022.
Edited by:
Jun Ren, Fudan University, ChinaReviewed by:
Ne Natalie Wu, Fudan University, ChinaCopyright © 2022 Shen, Zhan, He, Deng, Li, Wen and Huang. This is an open-access article distributed under the terms of the Creative Commons Attribution License (CC BY). The use, distribution or reproduction in other forums is permitted, provided the original author(s) and the copyright owner(s) are credited and that the original publication in this journal is cited, in accordance with accepted academic practice. No use, distribution or reproduction is permitted which does not comply with these terms.
*Correspondence: Shihong Wen, d3NoaWhAbWFpbC5zeXN1LmVkdS5jbg==; Wenqi Huang, aHVhbmd3ZW5xaTg2QGFsaXl1bi5jb20=
†These authors have contributed equally to this work
Disclaimer: All claims expressed in this article are solely those of the authors and do not necessarily represent those of their affiliated organizations, or those of the publisher, the editors and the reviewers. Any product that may be evaluated in this article or claim that may be made by its manufacturer is not guaranteed or endorsed by the publisher.
Research integrity at Frontiers
Learn more about the work of our research integrity team to safeguard the quality of each article we publish.