- Institute of Animal Nutrition, Northeast Agricultural University, Harbin, China
The purpose of the experiment was to investigate the Toll-like receptor signaling pathway of quercetin regulating avian beta-defensin (AvBD) in the ileum of Arbor Acre (AA) broilers. Four hundred and eighty one-day-old Arbor Acre broilers with similar body weight, half male and female, were randomly allotted to four treatments; the control treatment and three dietary treatments were fed with the basal diets supplemented with 0, 0.02%, 0.04, and 0.06% quercetin, respectively. The results showed that dietary quercetin supplementation did not significantly influence growth performance (p > 0.05), but significantly decreased the mortality rate of broilers by 85.74%, 85.74, and 71.28%, respectively (p < 0.05, F = 9.06). Compared with control, dietary supplementation with 0.04 and 0.06% quercetin significantly upregulated mRNA expression of total AvBD (p < 0.05), and there were no significant differences in the mRNA expression of AvBD1, AvBD2, and AvBD14 in three quercetin supplementation groups in the ileum of AA broilers (p > 0.05). Dietary supplementation with 0.02 and 0.06% quercetin significantly downregulated the mRNA expression of total Toll-like receptors (p < 0.05). Dietary quercetin supplementation significantly downregulated the mRNA expression of TLR1A, TLR1B, and TLR2A (p < 0.05); however, there were no significant differences in the mRNA expression of TLR2B, TLR5, and TLR15 (p > 0.05). Dietary quercetin supplementation significantly downregulated the mRNA expression of myeloid differentiation primary response protein 88 (MyD88) and TIR domain-containing adaptor protein/MyD88-adaptor-like (TIRAP/MAL) (p < 0.05), 0.02% quercetin significantly downregulated the mRNA expression of tank-binding kinase1 (TBK1), IκB kinase complex-α (IKKα), IKKβ, IKKε, nuclear factor-kappa B (NF-κB), NF-κB inhibitor-alpha (IκBα), IκBα, IκBβ, TNF-receptor-associated factor 3 (TRAF3), and interferons regulatory factor 7 (IRF7) (p < 0.05), 0.04% quercetin significantly downregulated the mRNA expression of IKKβ, IKKε, NF-κB, IκBα, IκBβ, TRAF3, and TRAF6 (p < 0.05), and 0.06% quercetin significantly downregulated the mRNA expression of TBK1 and IKKα (p < 0.05). 0.02% quercetin significantly decreased the relative abundance of Escherichia, Staphylococcus (p < 0.05), and Salmonella (p < 0.01), 0.04% quercetin significantly decreased the relative abundance of Staphylococcus (p < 0.05), Escherichia, and Salmonella (p < 0.01), and 0.06% quercetin significantly decreased the relative abundance of Salmonella (p < 0.05) and Staphylococcus (p < 0.01) in the ileum of AA broilers. These findings suggested that dietary quercetin supplementation regulated the mRNA expression of AvBD, TLR, and the TLR signaling pathways and NF-κB signalling pathways, thereby maintaining the microecological balance of the intestinal tract and decreasing the mortality of broilers, and the optimum addition amount of quercetin is 0.04% under the test conditions.
Introduction
The intestine plays a crucial role in maintaining body immunity that is the first line of defense from exogenous pathogens infecting host cells and tissues of chicken, which is also the largest organ with immune properties (Fisinin and Surai, 2013). Toll-like receptors (TLRs) are evolutionarily conserved pattern recognition receptors that have been identified in chickens, which initiate innate immune responses and regulate the expression of proinflammatory and anti-inflammatory cytokines and avian beta-defensin (AvBD) (St Paul et al., 2013; Yoshimura, 2015; Kang, et al., 2019; Shimizu et al., 2020; Terada et al., 2020). TLR-mediated signaling pathways are involved in regulating intestinal epithelial barrier integrity and promoting the health of chickens (Nighot et al., 2017), which leads to a well-studied signaling cascade initiated by TIR domain-containing signaling adapters, including myeloid differentiation primary response protein 88 (MyD88), TIR domain-containing adaptor inducing IFN-β (TRIF), TIR domain-containing adaptor protein/MyD88-adaptor-like (TIRAP/MAL), and TRIF-related adaptor molecule (TRAM), and these adapters interact with the TIR domain of the TLR (Hamerman et al., 2015). Administration of TLR-Ls alone also may prevent bacterial infections in chickens (Gomis et al., 2003; Taghavi et al., 2008). β-defensin found in birds is an important and versatile component of the innate immune system in all species and belongs to a kind of the antimicrobial peptides (Lynn et al., 2007), which are produced by phagocytic cells, leukocytes, and epithelial cells, has bactericidal activity against a variety of Gram-negative and Gram-positive bacteria, may directly act on immune cells or form a complex with other molecules to regulate immune responses, and controls the interaction between the host and microorganism, thus maintaining host health and reducing the burden of infectious diseases (Mahlapuu et al., 2016; Hall et al., 2017; Meade and O'Farrelly, 2019). Daneshmand et al. (2020) reported that antimicrobial peptides improve the intestinal damage associated with necrotic enteritis, reduce mortality, and restore the microflora balance in the ileum of broilers. The cecum and ileum of broilers are widely concerned, cecum is the most abundant intestinal microflora and the main place for pathogen colonization (Lan et al., 2002), and ileum is the key part of nutrient absorption, with high microbial diversity (Tang et al., 2000). There are few studies related to quercetin, AvBD, and TLRs in the ileum of broilers. It is shown that flavonoids can modify the composition of the microbiota, modulate TLR gene expression, and regulate the TLR-mediated signaling pathways (Pérez-Cano et al., 2014). Quercetin, a major flavonoid, has been indorsed for its antioxidant and anti-inflammatory properties (Comalada et al., 2006; Saeed et al., 2017). The present study was undertaken to confirm the relationship between mortality and AvBD in the ileum of Arbor Acre (AA) broilers. In addition, the effects of quercetin on TLR and signal molecules related to the TLR signaling pathway were explored to elucidate the mechanism by which dietary quercetin supplementation in AA broilers improves health.
Materials and Methods
All procedures used in this study were approved by the Animal Welfare Care and Use Committee of the University. Housing, management, and care of the birds conformed to the guidelines of Agricultural Animal in Agricultural Research and Teaching of Heilongjiang Province (HEI Animal Management Certificate No. 11928).
Materials
Main reagents: quercetin (purity ≥97%) was purchased commercially from Sigma-Aldrich chemical company, United States.
Animals: 1-day-old AA broilers were purchased from Harbin Yinong Poultry Industry, China.
Experimental Design
All the broilers were individually weighed; 480 broilers (1 day old) with similar body weight were randomly divided into four groups with six replicates, with 20 broilers in each replicate (120 broilers per treatment). The assigned groups were as follows: A, normal control broilers fed with basal diet (NC); B, the treatment broilers fed with basal diet of 0.02% quercetin (quercetin 0.02%); C, the treatment broilers fed with basal diet of 0.04% quercetin (quercetin 0.04%); and D, the treatment broilers fed with basal diet of 0.06% quercetin (quercetin 0.06%), and the trial lasted 42 d. Quercetin dehydrate powder was mixed with basal diet and offered in mash form (5mm) after grinding (Table 1).
All the broilers were raised under the same management and environment. Continuous fluorescent light was provided during the experiment. Water and experimental diets were available ad libitum, followed by routine immunization. At the end of the experiment, two chickens per replicate were randomly selected and slaughtered, and the intestinal tract was excised out, washed in ice-cold normal saline, patted dry and frozen in cryopreserved tubes in liquid nitrogen, and stored at −80°C.
Experimental Diets
Corn-soybean meal basal diets were formulated according to Chinese Broiler Feeding Standards (GB/T5916-2020) to meet the nutrient requirements of AA broilers (Table 2).
Determination of Indexes
Growth Performance
At 0, 21, and 42 days of age, one broiler per replicate was randomly selected from each treatment group and weighed after feed deprivation for 12 h, and growth performance in terms of average daily gain (ADG), average daily feed intake (ADFI), and feed conversion ratio (FCR) per replicate (cage) was recorded and calculated.
Mortality Rate
From day 8 of the trail, the mortality rate of AA broilers in each group was recorded and calculated.
mRNA Expression of Genes
At the end of the experiment, one broiler per replicate from each treatment group was randomly selected and slaughtered, ileum content was individually homogenized, and total RNA was extracted using TRIzol reagent (Invitrogen Corp., Carlsbad, CA, United States), and cDNA was synthesized using a PrimeScript 1st strand cDNA synthesis kit (Takara Bio Inc., Kyoto, Japan). RT-qPCR was performed using the SYBR Premix Ex Taq II, ROX plus (Takara Bio Inc.) and the Prism 7900HT sequence detection system (Applied Biosystems, Foster City, CA, United States). PCR was carried out for 40 cycles (2 min at 50°C, 10 min at 95°C, and 40 cycles of 10 s at 95°C and 1 min at 60°C) (Seo et al., 2016). β-Actin was used as the internal control in this study. According to the gene bank, the primers were designed by Oligo 6.0 software and Primer premier 5.0 software (Tables 3–5).
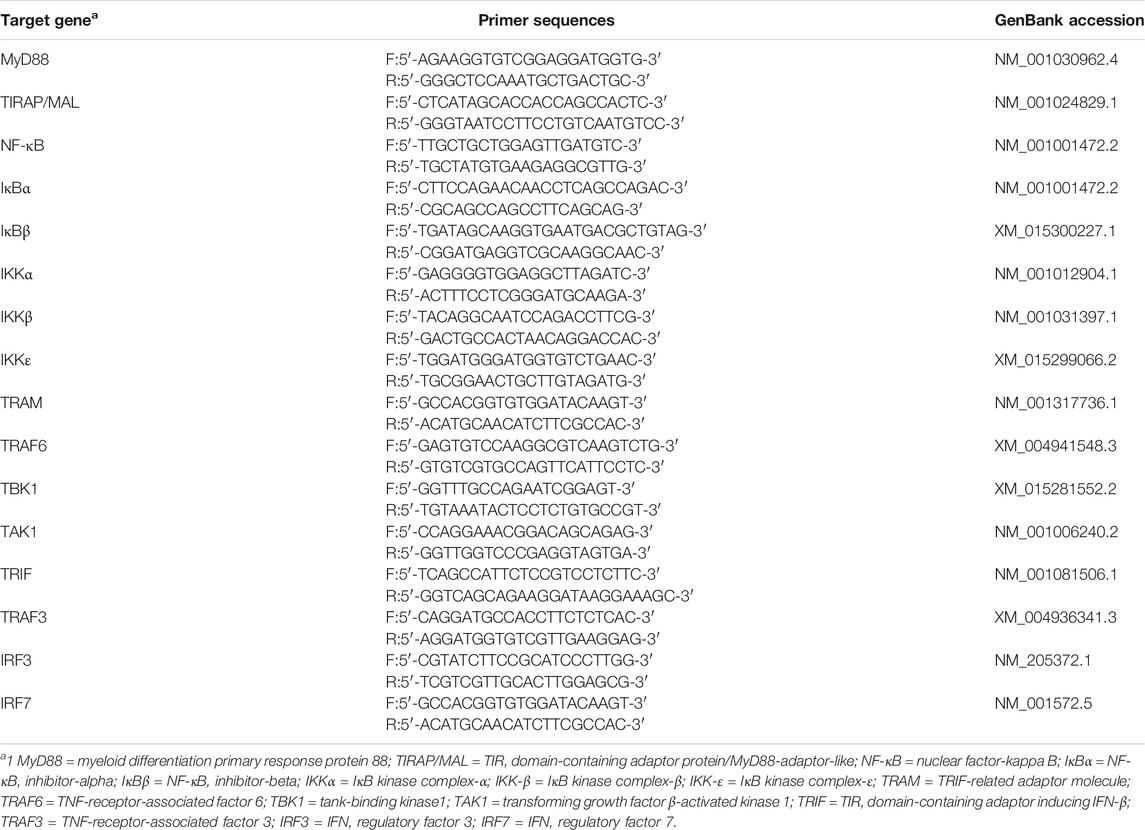
TABLE 5. Primers of genes related to the Toll-like receptors signaling pathway used for mRNA expression.
Whole Metagenomic Sequencing
1 broiler from each treatment group was randomly selected and slaughtered, DNA was extracted from the ileum content, and illumina TruSeq libraries were prepared from genomic DNA and sequenced on Illumina HiSeq systems 4,000 by Majorbio (China). Paired-end reads (2 × 150 bp) were generated, resulting in between 8 and 15 GB per sample (between 40 and 73 million paired reads). Briefly, to measure the abundance of known functional microbial genes in the ileum samples, reads from whole metagenomic sequencing were aligned to the Kyoto Encyclopedia of Genes and Genomes (KEGG) database using Majorbio (www.majorbio.com).
Data Analysis
The experimental data were analyzed by the general linear model using SPSS 20.0 statistical package for Windows (2010, SPSS Inc., Chicago, IL 60606–6307). Difference and interaction among treatments were examined by one-way ANOVA with Duncan’s test. Calculated △Ct (corrected sample) = mean value of the target gene–mean value of the internal reference gene; △△ Ct = △Ct–mean value of the control group. Values of p < 0.05 mean a significantly statistical difference, and all the results were expressed as “mean ± standard deviation.”
Results
Effects of Quercetin on Growth Performance in AA Broilers
Compared with control, there were no significant differences in ADG, ADFI, and FCR among groups during the 42-day study (p > 0.05) (Table 6).
Effects of Quercetin on Mortality Rate in AA Broilers
Maximum mortality rate (4.84%) was observed in the control group, and compared with control, dietary supplementation with 0.02%, 0.04, and 0.06% quercetin significantly decreased the mortality rate by 85.74%, 85.74, and 71.28%, respectively (p < 0.05, F = 9.06) (Table 7).
Effects of Quercetin on the mRNA Expression of Avian Beta-Defensins in the Ileum of AA Broilers
Dietary supplementation with 0.04 and 0.06% quercetin significantly upregulated the mRNA expression of total AvBD (p < 0.05, F = 4.27); however, quercetin did not significantly affect the mRNA expression of AvBD1 (p = 0.12, F = 2.22), AvBD2 (p = 0.49, F = 0.84), and AvBD14 (p = 0.43, F = 0.96) in the ileum of AA broilers, compared with control. In addition, 0.02% quercetin significantly upregulated the mRNA expression of AvBD3, AvBD4, AvBD6, and AvBD11 and downregulated the mRNA expression of AvBD12 and AvBD13 (p < 0.05). 0.04% quercetin significantly upregulated the mRNA expression of AvBD7, AvBD9, and AvBD10 (p < 0.05). 0.06% quercetin significantly upregulated the mRNA expression of AvBD5, AvBD8, AvBD10, AvBD11, and AvBD13 (p < 0.05). These results indicated that dietary quercetin supplementation regulated the mRNA expression of AvBD in the ileum of AA broilers (Table 8).
Effect of Quercetin on the Expression of Toll-Like Receptors in the Ileum of AA Broilers
Dietary supplementation with 0.02 and 0.06% quercetin significantly downregulated the mRNA expression of total TLR (p < 0.01, F = 33.5) in the ileum of AA broilers, compared with control. Dietary quercetin supplementation significantly downregulated the mRNA expression of TLR1A (p = 0.00, F = 28.85), TLR1B (p = 0.00, F = 42.40), and TLR2A (p = 0.00, F = 34.43); however, there were no significant differences in the mRNA expression of TLR2B (p = 0.06, F = 2.92) and TLR5 (p = 0.24, F = 1.54). Besides, 0.02% quercetin significantly downregulated the mRNA expression of TLR4 and TLR21 (p < 0.05); 0.04% quercetin significantly upregulated the mRNA expression of TLR4 and TLR7 (p < 0.05), and 0.06% quercetin significantly downregulated the mRNA expression of TLR3 (p < 0.05). These results indicated that dietary quercetin supplementation regulated the mRNA expression of TLR in the ileum of AA broilers (Table 9).
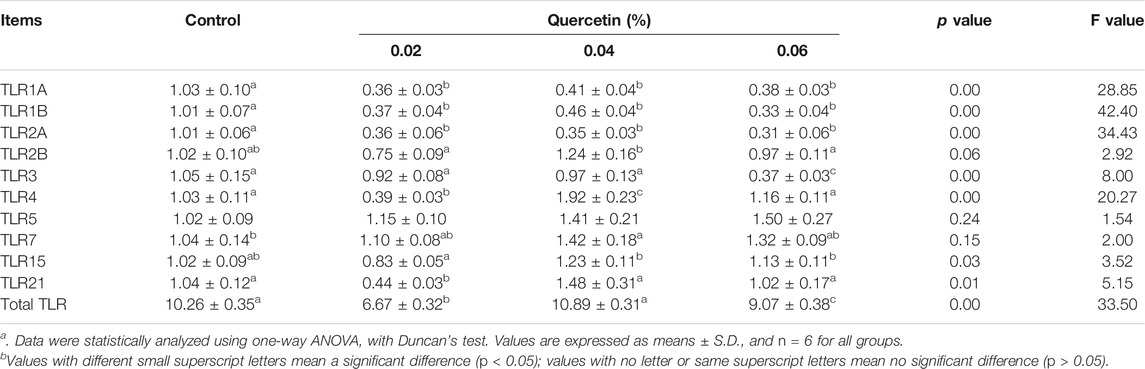
TABLE 9. Effect of quercetin on the mRNA expression of Toll-like receptors in the ileum of AA broilers.
Effect of Quercetin on the Expression of Genes Related to the Toll-Like Receptor Signaling Pathway in the Ileum of AA Broilers
Dietary quercetin supplementation significantly downregulated the mRNA expression of MyD88 (p = 0.00, F = 15.98) and TIRAP/MAL (p = 0.00, F = 25.75); however, there were no significant differences in the mRNA expression of interferons regulatory factor 3 (IRF3) (p = 0.20, F = 1.68), TRIF, transforming growth factor β-activated kinase 1 (TAK1) (p = 0.20, F = 1.68), and TRAM (p = 0.38, F = 1.09) in the ileum of AA broilers, compared with control. 0.02% quercetin significantly downregulated the mRNA expression of tank-binding kinase1 (TBK1), nuclear factor-kappa B (NF-κB), NF-κB inhibitor-alpha (IκBα), IκBβ, IκB kinase complex-α (IKKα), IKKβ, IKKε, TNF-receptor-associated factor 3 (TRAF3), and IRF7 (p < 0.05), 0.04% quercetin significantly downregulated the mRNA expression of NF-κB, IκBα, IκBβ, IKKβ, IKKε, TRAF3, and TRAF6 (p < 0.05), and 0.06% quercetin significantly downregulated the mRNA expression of TBK1 and IKKα (p < 0.05). These results indicated that dietary quercetin supplementation regulated TLR signalling pathways and NF-κB signalling pathways in the ileum of AA broilers (Table 10).
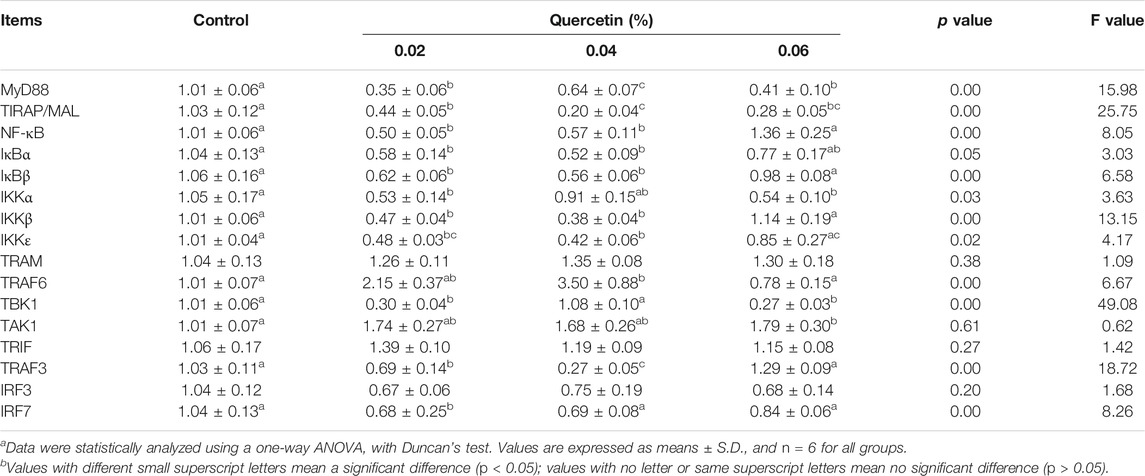
TABLE 10. Effect of quercetin on the mRNA expression of genes related to the Toll-like receptor signaling pathway in the ileum of AA broilers.
Effect of Quercetin on Microbial Diversity in the Ileum of AA Broilers
Quercetin had no significant effect on the Shannon index in the ileum of AA broilers (p > 0.05) (Figure 1).
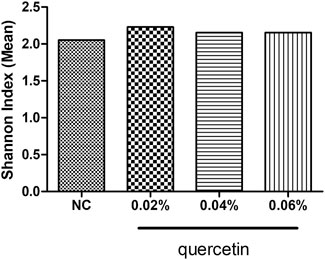
FIGURE 1. Effect of quercetin on microbial diversity in the ileum of AA broilers, NC (normal control broilers) and quercetin (0.02%, 0.04, and 0.06%) groups. Data were statistically analyzed using one-way ANOVA with Duncan’s test. Values are expressed as means ± S.D., and n = 1 for all groups. Values not marked mean no significant difference was seen (p > 0.05).
Effect of Quercetin on the Relative Abundance of Microbes in the Ileum of AA Broilers at the Genus and Family Level
At the genus level, compared with control, 0.02% quercetin significantly decreased the relative abundance of Escherichia, Staphylococcus (p < 0.05), and Salmonella (p < 0.01), 0.04% quercetin significantly decreased the relative abundance of Staphylococcus (p < 0.05), Escherichia, and Salmonella (p < 0.01), and 0.06% quercetin significantly decreased the relative abundance of Salmonella (p < 0.05) and Staphylococcus (p < 0.01) in the ileum of AA broilers (Figure 2).
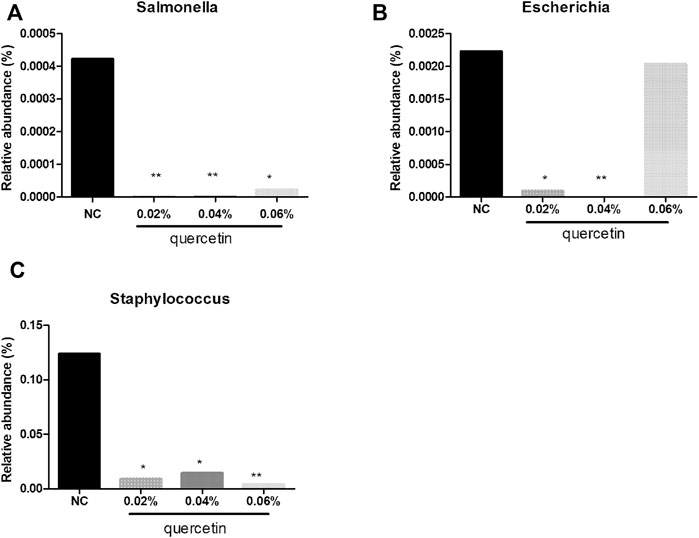
FIGURE 2. Effect of quercetin on microbial relative abundance in the ileum of AA broilers at the genus level, NC (normal control broilers) and quercetin (0.02%, 0.04, and 0.06%) groups. (A) Effect of quercetin on Salmonella relative abundance in the ileum of AA broilers at the genus level; (B) Effect of quercetin on Escherichia relative abundance in the ileum of AA broilers at the genus level; (C) Effect of quercetin on Staphylococcus relative abundance in the ileum of AA broilers at the genus level. Data were statistically analyzed using one-way ANOVA with Duncan’s test. Values are expressed as means ± S.D., and n = 1 for all groups. *p < 0.05 and **p < 0.01.
At the family level, compared with control, 0.02% quercetin significantly decreased the relative abundance of Coriobacteriaceae and Desulfovibrionaceae (p < 0.01), 0.04% quercetin significantly decreased the relative abundance of Enterobacteriaceae, Coriobacteriaceae, and Desulfovibrionaceae (p < 0.01), and 0.06% quercetin significantly decreased the relative abundance of Enterobacteriaceae, Coriobacteriaceae (p < 0.05), Lachnospiraceae, and Desulfovibrionaceae (p < 0.01) in the ileum of AA broilers (Figure 3).
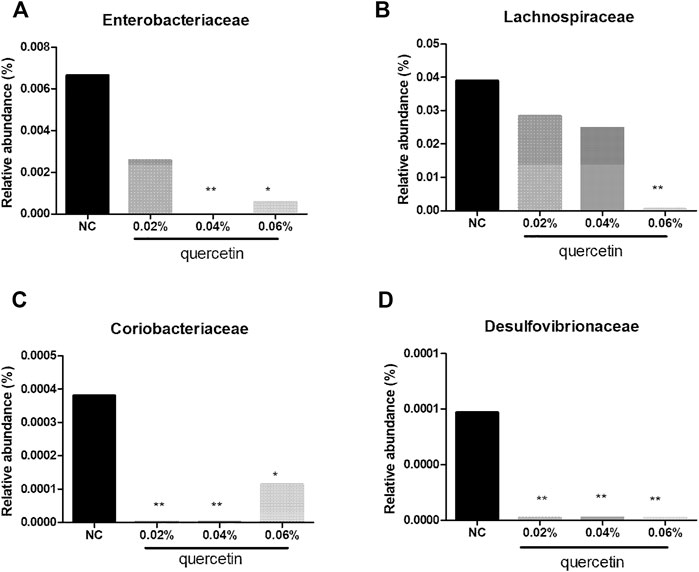
FIGURE 3. Effect of quercetin on microbial relative abundance in the ileum of AA broilers at the family level, NC (normal control broilers) and quercetin (0.02%, 0.04, and 0.06%) groups. (A) Effect of quercetin on Enterobacteriaceae relative abundance in the ileum of AA broilers at the family level; (B) Effect of quercetin on Lachnospiraceae relative abundance in the ileum of AA broilers at the family level; (C) Effect of quercetin on Coriobacteriaceae relative abundance in the ileum of AA broilers at the family level; (D) Effect of quercetin on Desulfovibrionaceae relative abundance in the ileum of AA broilers at the family level. Data were statistically analyzed using one-way ANOVA with Duncan’s test. Values are expressed as means ± S.D., and n = 1 for all groups. *p < 0.05 and **p < 0.01.
Estimate of the Optimal Supplementation of Dietary Quercetin in AA Broilers
Through the quadratic curve regression model, the quadratic regression equation of quercetin supplementation level and mortality, total AvBD, and total TLR expression in AA broiler diets was established, and the optimal range of quercetin supplementation was calculated by considering the following data comprehensively. The optimum addition amount of quercetin is 0.04% under the test conditions (Table 11).
Discussion
The present study showed that dietary quercetin supplementation significantly upregulated the mRNA expression of total AvBD and significantly downregulated the mRNA expression of total TLR and regulated the TLR signaling pathway in the ileum of AA broilers (p < 0.05). The current study was the first report regarding the effects and mechanism of quercetin on the mRNA expression of AvBD in the ileum of AA broilers.
Flavonoids exhibited different effects on growth in chicken. Dietary supplementation with 0.02%, 0.04, and 0.06% quercetin had no significant effect on ADG, ADFI, and FCR in AA broilers during the 42d study (p > 0.05). Similar results were reported by Sayed et al. (2015), who studied 10%, 20, and 30% buckwheat (rich bioactive compounds including flavones and flavonoids) supplementation for 4 weeks had no effect on body weight gain, feed intake, and FCR (p > 0.05) in broilers. However, these results were different from those of Hassan et al. (2018), who found that dietary rutin supplementation with 1 g/kg diet for 42d significantly increased body weight gain and decreased FCR (p < 0.001), and there were no linear or quadratic effects on either feed intake or the relative growth rate (p > 0.05). The variation resulted from the difference in types of flavonoids and experimental animals. Gut microbiota is composed of trillions of bacteria, which play an important role in animal metabolism. Desulfovibrioceae, Rhodospirillum, and Lacetospirillum are positively correlated with obesity in mice, and quercetin and resveratrol reduced the relative abundance of these bacteria (Zhao et al., 2017). The previous research of our lab found that quercetin reduced the relative abundance of Desulfovibrio desulphuricans, Miridae, and Spirilloidea in the ileum of broilers (data not shown) and the blood lipid content of AA broilers, increased the secretion of leptin and adiponectin, activated the phosphatidylinositol 3-kinase/protein kinase B (PI3K/PKB) signaling pathway, thereby improving lipid metabolism, and ultimately reduced the abdominal fat deposition in broilers (Ying et al., 2020). Combined with the results of this study, it was indicated that quercetin improved lipid metabolism and reduced fat deposition of broilers, which may result from regulating some of the obesity-related flora in the intestine. That is, quercetin interfered with lipid metabolism by reducing the abundance of intestinal flora such as Laospirillaceae, which is positively related to obesity, thereby reduced fat deposition, and then, played an antiobesity role. This also explained why quercetin has no significant effect on the growth performance of AA broilers.
The decrease of mortality was observed in broilers supplemented with quercetin (p < 0.05), and similar results were reported by Kalia et al. (2018), who examined the effects of H. rhamnoides aqueous extract (100, 150, 200, 300, 400, and 800 mg/kg body weight for 42d) on the mortality of broilers, which is abundant in bioactive plant molecules including flavonoids, vitamins, carotenoids, and organic acid. Fan et al. (2019) reported that rabbit neutrophil peptide-1 (NP-1) is a multifunctional cationic peptide with broad-spectrum antimicrobial activities, which increased duodenal Lactobacillus and adjusted the balance and quality of the intestinal microflora, thus improving chicken health. In birds, AvBDs are the biggest cluster of host defense peptide (HDPs), and HDPs potentially control a wide range of pathogens without resistance; moreover, they play important roles in adaptive immunity, wound healing, and sperm fertilization and may also be used as alternatives to antibiotics (Lynn et al., 2007; Hazlett and Wu, 2010; Zhang et al., 2016). Fourteen kinds of AvBDs are present in chickens which play an important role in the innate host defence against viruses, Gram-positive and Gram-negative bacteria, and parasites in the digestive system, reproductive system, and immune function in poultry (Lynn et al., 2007; Zhang and Sunkara, 2014; Cheng et al., 2015). AvBD is an effective tool for maintaining health and reducing the burden of infectious disease in livestock species (Meade and O’Farrelly, 2019). Infectious bronchitis virus (IBV) infection induces the mRNA expression of AvBD2, 4, 5, 6, 9, and 12, which suggests that AvBDs may play significant roles in resisting IBV replication in chickens (Xu et al., 2015). There are few studies that focus on the flavonoids in promoting AvBD expression. The black tea extract and the aflavin derivatives induced the secretion of the antimicrobial peptides human β-defensin 1(hBD-1), hBD-2, and hBD-4 in oral epithelial cells to protect the oral cavity against periodontal disease (Bedran et al., 2013). Zizania latifolia has been used as a health food in Asian countries, which may upregulate the mRNA expression of hBD in HaCaT cells and, thus, inhibit S. aureus infection through the TLR2 signaling pathway (Kang et al., 2018). In the present study, compared with control, dietary supplementation with 0.04 and 0.06% quercetin significantly upregulated the mRNA expression of total AvBD (p < 0.05) in the ileum of AA broilers. The high expression of AvBD6 in the proximal digestive tract and broad antimicrobial activity indicate that AvBD6 plays an important role in chicken innate host (Van Dijk et al., 2006). The present results showed that dietary quercetin supplementation significantly upregulated the mRNA expression of AvBD10 (0.04 and 0.06%, p < 0.05) and AvBD11 (0.02 and 0.06%, p < 0.05), and these findings are consistent with the results of Laptev et al. (2019); it was shown that phytobiotic intebio enriched with essential oils (90 g/t for 42d) significantly upregulated the mRNA expression of AvBD10 (p < 0.05) in the early stage of Salmonella infection (at 1 dpi); however, in the late stage of Salmonella infection (23dpi), the mRNA expression of AvBD11 was significantly lowered, and it suggested that the essential oils inhibited the earlier inflammatory reaction. Hong et al. (2012) demonstrated that AvBD10 induction was detected in response to Salmonella of poultry. In the current study, compared with control, dietary quercetin supplementation did not influence the mRNA expression of AvBD1, AvBD2, AvBD8, and AvBD14 (p > 0.05); however, dietary quercetin supplementation significantly upregulated the mRNA expression of AvBD3, AvBD4, AvBD5, AvBD6, AvBD7, AvBD8, AvBD9, AvBD10, AvBD11, and AvBD13 (p < 0.05) and significantly downregulated the mRNA expression of AvBD12 (p < 0.05). Lee et al. (2016) reported synthetic linear AvBD8 and AvBD10 peptides have weak lytic activity against Escherichia coli (Semple and Dorin, 2012). The abovementioned results revealed that quercetin reduced the mortality rate of broilers by promoting the expression of intestinal AvBDs, thereby promoting the health of broilers.
TLRs are type I trans-membrane proteins present either on the cell surface or in the intracellular compartments and the important components of the innate immune system, which are responsible for recognizing and responding to microbial components and endogenous molecules that are released by metabolism (Jin and Lee, 2008; Kang and Lee, 2011). Ten kinds of TLRs in chicken have been identified since the first report of the Toll protein in fruit fly (Anderson et al., 1985). There are two types of TLR signaling pathways, including the MyD88-dependent pathway and the TRIF-dependent pathway, respectively. Almost all the TLRs are activated in the MyD88-dependent response except for TLR3 (Kawai et al., 1999; Takeuchi et al., 2000; Kawai and Akira, 2007; Keestra et al., 2013; Warden et al., 2018). Flavonoids may regulate the expression of the TLR and TLR signaling pathway. Nawab et al. (2019) reported that curcumin downregulates the gene expression of TLR4 and associated downstream molecules such as NF-κB, MyD88, and TNF-α in laying hens. EGCG is the most abundant polyphenol in green tea, accounting for more than 50% of total polyphenols, and it effectively attenuated the acute lung injury induced by paraquat pesticides (PQ) via inhibiting the activation of NF-κB and upregulating TLR 2, 4, and 9 as well as their adaptors MyD88 and TRAF6 in mice (Oliveira et al., 2016; Shen et al., 2017). Quercetin regulated the TLR signaling pathway and inhibited NF-κB activity only via TAK1 in a dose-dependent manner, thus alleviating neuropathic pain (Ji et al., 2017). Cheng et al. (2017) showed that baicalin downregulated the expression of TLR4 and inhibited NF-κB activation in lipopolysaccharide (LPS)-induced liver inflammation of chicken via negatively regulating inflammatory mediators. The current study showed that dietary supplementation with 0.02%, 0.04, and 0.06% quercetin significantly downregulated the mRNA expression of TLR1A, TLR1B, TLR2A, and total TLR in the ileum of AA broilers (p < 0.05). In addition, the mRNA expression of TLR4 and TLR21 (0.02% quercetin supplementation, p < 0.05) and TLR3 (0.06% quercetin supplementation, p < 0.05) was significantly downregulated, and 0.04% quercetin significantly upregulated the mRNA expression of TLR4 and TLR7 (p < 0.05). Dietary quercetin supplementation effectively prevented inflammation via inhibiting the activation of NF-κB and downregulating total TLR, TLR1A, TLR1B, TLR2A, and TLR2B2, as well as their adaptors MyD88 and TIRAP/MAL (p < 0.05), in the ileum of AA broilers. 0.02% quercetin significantly downregulated the mRNA expression of TBK1, NF-κB, IκBα, IκBβ, IKKα, IKKβ, IKKε, TRAF3, and IRF7 (p < 0.05); 0.04% quercetin significantly downregulated the mRNA expression of NF-κB, IκBα, IκBβ, IKKβ, IKKε, TRAF3, and TRAF6 (p < 0.05), and 0.06% quercetin significantly downregulated the mRNA expression of TBK1 and IKKα (p < 0.05). The previous research of our lab found that dietary quercetin supplementation significantly increased the mRNA expression of TNF-α, TRAF2, nuclear factor-kappa-Bp65 subunit (NF-κBp65), and IFNγ (p < 0.05) and decreased the mRNA expression of IκBα (p < 0.05); that is, quercetin improved immune function via the NF-κB signaling pathway triggered by TNF-α (Yang et al., 2020). These results indicated that flavonoids played an anti-inflammatory role by inhibiting the expression of TLR and signal molecules related to TLR signaling pathways and NF-κB signalling pathways, thereby improving immunity and promoting health. The inflammatory process is a potential life-saving response to microbial challenge, which is ultimately to prevent tissue and the host from damage (Swaggerty et al., 2016). Under commercial farming models, broilers are exposed to a series of potential pathogens, with higher mRNA expression of proinflammatory factors in peripheral blood neutrophils, resisting necrotic enteritis induced by Salmonella, Eimeria tenella, and Clostridium perfringens (Swaggerty et al., 2014; Swaggerty et al., 2015). All the results showed that the inflammatory response plays an important role in enhancing the resistance of chicken to common pathogen infections, and a moderate inflammatory response is beneficial to the health of poultry.
The previous studies in our lab found that quercetin significantly regulated cecal flora of chickens. Wang found that quercetin reduced the number of Pseudomonas aeruginosa, Helicobacter pylori, Salmonella, Staphylococcus aureus, Escherichia coli, Clostridium perfringens, and Campylobacter jejuni in cecum of AA broilers (Wang, 2018). Quercetin also increased the number of bifidobacteria and reduced the abundance of Escherichia coli, Staphylococcus aureus, Salmonella, Pseudomonas aeruginosa, Streptococcus faecalis, and Enterococcus in cecum of laying hens (Teng, 2015). In addition, quercetin reduced the number of the total Escherichia coli and increased the number of Bifidobacteria and Lactobacillus in cecum of laying hens (Jin, 2013). In the present study, 0.02% quercetin significantly decreased the relative abundance of Escherichia, Staphylococcus (p < 0.05), and Salmonella (p < 0.01), 0.04% quercetin significantly decreased the relative abundance of Staphylococcus (p < 0.05), Escherichia, and Salmonella (p < 0.01), and 0.06% quercetin significantly decreased the relative abundance of Salmonella (p < 0.05) and Staphylococcus (p < 0.01) in the ileum of AA broilers. Together with the abovementioned results, quercetin significantly regulated the intestinal flora of chickens, which reduced the colonization of harmful bacteria and promoted the colonization of beneficial bacteria in the intestinal tract, thereby improving the microecological balance of the intestinal tract, thus promoting the healthy growth of broilers. The potential effects of quercetin on reducing the morality rate of broilers mediates the innate immune responses by modulating the expression of TLRs, and there are three primary levels: 1) by regulating the expression of AvBD, 2) by regulating the TLR signaling pathways, and 3) by adjusting the composition of microbiota (Figure 4).
Conclusion
In conclusion, quercetin enhances immunity by regulating the expression of AvBD, TLRs, and the TLR signaling pathways and NF-κB signalling pathways, to achieve the purpose of anti-inflammatory effect, and maintains the balance of intestinal flora by modulating the composition of the microbiota, so as to promote the body health and reduced mortality rate of broilers, and the optimum addition amount of quercetin is 0.04% under the test conditions.
Data Availability Statement
Publicly available datasets were analyzed in this study. This data can be found here: https://www.ncbi.nlm.nih.gov/bioproject/808675/BioProject ID PRJNA808675. The original contributions presented in the study are publicly available. This data can be found here: https://www.ncbi.nlm.nih.gov/biosample/26101061/SAMN26101061, https://www.ncbi.nlm.nih.gov/biosample/26101062/SAMN26101062, https://www.ncbi.nlm.nih.gov/biosample/26101063/SAMN26101063, https://www.ncbi.nlm.nih.gov/biosample/26101064/SAMN26101064, https://www.ncbi.nlm.nih.gov/biosample/26101065/SAMN26101065, https://www.ncbi.nlm.nih.gov/biosample/26101066/SAMN26101066, https://www.ncbi.nlm.nih.gov/biosample/26101067/SAMN26101067, https://www.ncbi.nlm.nih.gov/biosample/26101068/SAMN26101068, https://www.ncbi.nlm.nih.gov/biosample/26101069/SAMN26101069, https://www.ncbi.nlm.nih.gov/biosample/26101070/SAMN26101070, https://www.ncbi.nlm.nih.gov/biosample/26101071/SAMN26101071, https://www.ncbi.nlm.nih.gov/biosample/26101072/SAMN26101072.
Ethics Statement
The animal study was reviewed and approved by the Animal Welfare Care and Use Committee of the University. Housing, management, and care of the birds conformed to the guidelines of Agricultural Animal in Agricultural Research and Teaching of Heilongjiang Province (HEI Animal Management Certificate No. 11928).
Author Contributions
LY participated in the design of the study, data interpretation, and manuscript writing. HW performed the experiments and analysis and participated in data interpretation. SZ, HL, MD, BW, SW, YM, and FX performed the experiments. YL supervised the work, evaluated the data, wrote the manuscript, and corrected the manuscript for publication. All authors approved the final version of the manuscript.
Funding
This project was supported by the National Science Foundation of China (32072749).
Conflict of Interest
The authors declare that the research was conducted in the absence of any commercial or financial relationships that could be construed as a potential conflict of interest.
Publisher’s Note
All claims expressed in this article are solely those of the authors and do not necessarily represent those of their affiliated organizations, or those of the publisher, the editors, and the reviewers. Any product that may be evaluated in this article, or claim that may be made by its manufacturer, is not guaranteed or endorsed by the publisher.
References
Anderson, K. V., Bokla, L., and Nüsslein-Volhard, C. (1985). Establishment of Dorsal-Ventral Polarity in the drosophila Embryo: The Induction of Polarity by the Toll Gene Product. Cell 42 (3), 791–798. doi:10.1016/0092-8674(85)90275-2
Bedran, T. B. L., Feghali, K., Zhao, L., Palomari Spolidorio, D. M., and Grenier, D. (2013). Green tea Extract and its Major Constituent, Epigallocatechin-3-Gallate, Induce Epithelial Beta-Defensin Secretion and Prevent Beta-Defensin Degradation by Porphyromonas Gingivalis. J. Periodont Res. 49 (5), 615–623. doi:10.1111/jre.12142
Cheng, P., Wang, T., Li, W., Muhammad, I., Wang, H., Sun, X., et al. (2017). Baicalin Alleviates Lipopolysaccharide-Induced Liver Inflammation in Chicken by Suppressing TLR4-Mediated NF-Κb Pathway. Front. Pharmacol. 8, 547. doi:10.3389/fphar.2017.00547
Cheng, Y., Prickett, M. D., Gutowska, W., Kuo, R., Belov, K., and Burt, D. W. (2015). Evolution of the Avian β-defensin and Cathelicidin Genes. BMC Evol. Biol. 15 (1), 188. doi:10.1186/s12862-015-0465-3
Comalada, M., Ballester, I., Bailón, E., Sierra, S., Xaus, J., Gálvez, J., et al. (2006). Inhibition of Pro-inflammatory Markers in Primary Bone Marrow-Derived Mouse Macrophages by Naturally Occurring Flavonoids: Analysis of the Structure-Activity Relationship. Biochem. Pharmacol. 72, 1010–1021. doi:10.1016/j.bcp.2006.07.016
Daneshmand, A., Kermanshahi, H., Sekhavati, M. H., Javadmanesh, A., Ahmadian, M., Alizadeh, M., et al. (2020). Effects of cLFchimera Peptide on Intestinal Morphology, Integrity, Microbiota, and Immune Cells in Broiler Chickens Challenged with Necrotic Enteritis. Sci. Rep. 10 (1), 17704. doi:10.1038/s41598-020-74754-x
Fan, C., Wu, J., Xu, L., Bai, L., Yang, H., Yan, C., et al. (2019). A Mutated Rabbit Defensin NP-1 Produced by Chlorella Ellipsoidea Can Improve the Growth Performance of Broiler Chickens. Sci. Rep. 9 (1), 12778. doi:10.1038/s41598-019-49252-4
Fisinin, V. I., Surai, P., and Surai, P. (2013). Gut Immunity in Birds: Facts and Reflections. S-h. Biol. 4, 3–25. doi:10.15389/agrobiology.2013.4.3eng
Gomis, S., Babiuk, L., Godson, D. L., Allan, B., Thrush, T., Townsend, H., et al. (2003). Protection of Chickens against Escherichia coli Infections by DNA Containing CpG Motifs. Infect. Immun. 71, 857–863. doi:10.1128/IAI.71.2.85710.1128/iai.71.2.857-863.2003
Hall, T. J., McQuillan, C., Finlay, E. K., O’Farrelly, C., Fair, S., and Meade, K. G. (2017). Comparative Genomic Identification and Validation of β-defensin Genes in the Ovis aries Genome. BMC Genomics 18, 278. doi:10.1186/s12864-017-3666-x
Hamerman, J. A., Pottle, J., Ni, M., He, Y., Zhang, Z.-Y., and Buckner, J. H. (2015). Negative Regulation of TLR Signaling in Myeloid Cells-Implications for Autoimmune Diseases. Immunol. Rev. 269, 212–227. doi:10.1111/imr.12381
Hassan, F., Roushdy, E., Kishawy, A., Zaglool, A., Tukur, H., and Saadeldin, I. (2018). Growth Performance, Antioxidant Capacity, Lipid-Related Transcript Expression and the Economics of Broiler Chickens Fed Different Levels of Rutin. Animals 9 (1), 7. doi:10.3390/ani9010007
Hazlett, L., and Wu, M. (2010). Defensins in Innate Immunity. Cell Tissue Res 343 (1), 175–188. doi:10.1007/s00441-010-1022-4
Hong, Y. H., Song, W., Lee, S. H., and Lillehoj, H. S. (2012). Differential Gene Expression Profiles of β-defensins in the Crop, Intestine, and Spleen Using a Necrotic Enteritis Model in 2 Commercial Broiler Chicken Lines. Poult. Sci. 91 (5), 1081–1088. doi:10.3382/ps.2011-01948
Ji, C., Xu, Y., Han, F., Sun, D., Zhang, H., Li, X., et al. (2017). Quercetin Alleviates thermal and Cold Hyperalgesia in a Rat Neuropathic Pain Model by Inhibiting Toll-like Receptor Signaling. Biomed. Pharmacother. 94, 652–658. doi:10.1016/j.biopha.2017.07.145
Jin, F. (2013). “Effect of Quercetin on Growth Performance, Nutrient Digestion and Absorption of Laying Hens,” (Harbin, China: Northeast Agricultural University). Doctoral Dissertation.
Jin, M. S., and Lee, J.-O. (2008). Structures of the Toll-like Receptor Family and its Ligand Complexes. Immunity 29 (2), 182–191. doi:10.1016/j.immuni.2008.07.007
Kalia, S., Bharti, V. K., Giri, A., Kumar, B., Arora, A., and Balaje, S. S. (2018). Hippophae Rhamnoides as Novel Phytogenic Feed Additive for Broiler Chickens at High Altitude Cold Desert. Sci. Rep. 8 (1), 5954. doi:10.1038/s41598-018-24409-9
Kang, B. Y., Lee, S.-S., Bang, M.-H., Jeon, H., Kim, H., and Chung, D. K. (2018). Water-based Extracts of Zizania Latifolia Inhibit Staphylococcus aureus Infection through the Induction of Human Beta-Defensin 2 Expression in HaCaT Cells. J. Microbiol. 56 (12), 910–916. doi:10.1007/s12275-018-8307-9
Kang, J. Y., and Lee, J.-O. (2011). Structural Biology of the Toll-like Receptor Family. Annu. Rev. Biochem. 80 (1), 917–941. doi:10.1146/annurev-biochem-052909-141507
Kang, Y., Nii, T., Isobe, N., and Yoshimura, Y. (2019). Effects of the Routine Multiple Vaccinations on the Expression of Innate Immune Molecules and Induction of Histone Modification in Ovarian Cells of Layer Chicks. Poult. Sci. 98, 5127–5136. doi:10.3382/ps/pez214
Kawai, T., Adachi, O., Ogawa, T., Takeda, K., and Akira, S. (1999). Unresponsiveness of MyD88-Deficient Mice to Endotoxin. Immunity 11 (1), 115–122. doi:10.1016/s1074-7613(00)80086-2
Kawai, T., and Akira, S. (2007). TLR Signaling. Semin. Immunol. 19 (1), 24–32. doi:10.1016/j.smim.2006.12.004
Keestra, A. M., de Zoete, M. R., Bouwman, L. I., Vaezirad, M. M., and van Putten, J. P. M. (2013). Unique Features of Chicken Toll-like Receptors. Develop. Comp. Immunol. 41 (3), 316–323. doi:10.1016/j.dci.2013.04.009
Lan, P. T. N., Hayashi, H., Sakamoto, M., and Benno, Y. (2002). Phylogenetic Analysis of Cecal Microbiota in Chicken by the Use of 16S rDNA Clone Libraries. Microbiol. Immunol. 46 (6), 371–382. doi:10.1111/j.1348-0421.2002.tb02709.x
Laptev, G. Y., Filippova, V. A., Kochish, I. I., Yildirim, E. A., Ilina, L. A., Dubrovin, A. V., et al. (2019). Examination of the Expression of Immunity Genes and Bacterial Profiles in the Caecum of Growing Chickens Infected with Salmonella Enteritidis and Fed a Phytobiotic. animals 9 (9), 615. doi:10.3390/ani9090615
Lee, M. O., Jang, H.-J., Rengaraj, D., Yang, S.-Y., Han, J. Y., Lamont, S. J., et al. (2016). Tissue Expression and Antibacterial Activity of Host Defense Peptides in Chicken. BMC Vet. Res. 12 (1), 231. doi:10.1186/s12917-016-0866-6
Lynn, D. J., Higgs, R., Lloyd, A. T., O’Farrelly, C., Hervé-Grépinet, V., Nys, Y., et al. (2007). Avian Beta-Defensin Nomenclature: a Community Proposed Update. Immunol. Lett. 110 (1), 86–89. doi:10.1016/j.imlet.2007.03.007
Mahlapuu, M., Håkansson, J., Ringstad, L., and Björn, C. (2016). Antimicrobial Peptides: an Emerging Category of Therapeutic Agents. Front. Cel. Infect. Microbiol. 6. doi:10.3389/fcimb.2016.00194
Meade, K. G., and O'Farrelly, C. (2019). β-Defensins: Farming the Microbiome for Homeostasis and Health. Front. Immunol. 9, 3072. doi:10.3389/fimmu.2018.03072
Nawab, A., Li, G., An, L., wu, J., Chao, L., Xiao, M., et al. (2019). Effect of Curcumin Supplementation on TLR4 Mediated Non-specific Immune Responses in Liver of Laying Hens under High-Temperature Conditions. J. Therm. Biol. 84, 384–397. doi:10.1016/j.jtherbio.2019.07.003
Nighot, M., Al-Sadi, R., Guo, S., Rawat, M., Nighot, P., Watterson, M. D., et al. (2017). Lipopolysaccharide-induced Increase in Intestinal Epithelial Tight Permeability Is Mediated by Toll-like Receptor 4/myeloid Differentiation Primary Response 88 (MyD88) Activation of Myosin Light Chain Kinase Expression. Am. J. Pathol. 187, 2698–2710. doi:10.1016/j.ajpath.2017.08.005
Oliveira, M. R. d., Nabavi, S. F., Daglia, M., Rastrelli, L., and Nabavi, S. M. (2016). Epigallocatechin Gallate and Mitochondria-A story of Life and Death. Pharmacol. Res. 104, 70–85. doi:10.1016/j.phrs.2015.12.027
Pérez-Cano, F., Massot-Cladera, M., Rodríguez-Lagunas, M., and Castell, M. (2014). Flavonoids Affect Host-Microbiota Crosstalk through TLR Modulation. Antioxidants 3, 649–670. doi:10.3390/antiox3040649
Saeed, M., Naveed, M., Arain, M. A., Arif, M., Abd El-Hack, M. E., Alagawany, M., et al. (2017). Quercetin: Nutritional and Beneficial Effects in Poultry. World's Poult. Sci. J. 73, 355–364. doi:10.1017/s004393391700023x
Sayed, M., Islam, M., Haque, M., Shah, M., Ahmed, R., Siddiqui, M., et al. (2015). Dietary Effects of Chitosan and Buckwheat (Fagopyrum Esculentum) on the Performance and Serum Lipid Profile of Broiler Chicks. SA J. Sci. 45 (4), 429–440. doi:10.4314/sajas.v45i4.9
Semple, F., and Dorin, J. R. (2012). SS-Defensins: Multifunctional Modulators of Infection, Inflammation and More? J. Innate Immun. 4, 337–348. doi:10.1159/000336619
Seo, E., Oh, Y. S., and Jun, H.-S. (2016). Psoralea Corylifolia L. Seed Extract Attenuates Nonalcoholic Fatty Liver Disease in High-Fat Diet-Induced Obese Mice. Nutrients 8 (2), 83. doi:10.3390/nu8020083
Shen, H., Wu, N., Liu, Z., Zhao, H., and Zhao, M. (2017). Epigallocatechin-3-gallate Alleviates Paraquat-Induced Acute Lung Injury and Inhibits Upregulation of Toll-like Receptors. Life Sci. 170, 25–32. doi:10.1016/j.lfs.2016.11.021
Shimizu, M., Nii, T., Isobe, N., and Yoshimura, Y. (2020). Effects of Avian Infectious Bronchitis with Newcastle Disease and Marek's Disease Vaccinations on the Expression of Toll-like Receptors and Avian β-defensins in the Kidneys of Broiler Chicks. Poult. Sci. 99 (12), 7092–7100. doi:10.1016/j.psj.2020.08.071
St. Paul, M., Brisbin, J. T., Abdul-Careem, M. F., and Sharif, S. (2013). Immunostimulatory Properties of Toll-like Receptor Ligands in Chickens. Vet. Immunol. Immunopathology 152, 191–199. doi:10.1016/j.vetimm.2012.10.013
Swaggerty, C. L., McReynolds, J. L., Byrd, J. A., Pevzner, I. Y., Duke, S. E., Genovese, K. J., et al. (2016). Selection for Pro-inflammatory Mediators Produces Chickens More Resistant to Clostridium Perfringens-Induced Necrotic Enteritis. Poult. Sci. 95 (2), 370–374. doi:10.3382/ps/pev348
Swaggerty, C. L., Pevzner, I. Y., and Kogut, M. H. (2015). Selection for Pro-inflammatory Mediators Produces Chickens More Resistant to Eimeria Tenella. Poult. Sci. 94 (1), 37–42. doi:10.3382/ps/peu053
Swaggerty, C. L., Pevzner, I. Y., and Kogut, M. H. (2014). Selection for Pro-inflammatory Mediators Yields Chickens with Increased Resistance against Salmonella enterica Serovar Enteritidis. Poult. Sci. 93 (3), 535–544. doi:10.3382/ps.2013-03559
Taghavi, A., Allan, B., Mutwiri, G., Van Kessel, A., Willson, P., Babiuk, L., et al. (2008). Protection of Neonatal Broiler Chicks against Salmonella Typhimurium Septicemia by DNA Containing CpG Motifs. Avian Dis. 52, 398–406. doi:10.1637/8196-121907-Reg
Takeuchi, O., Takeda, K., Hoshino, K., Adachi, O., Ogawa, T., and Akira, S. (2000). Cellular Responses to Bacterial Cell wall Components Are Mediated through MyD88-dependent Signaling Cascades. Int. Immunol. 12 (1), 113–117. doi:10.1093/intimm/12.1.113
Tang, L., Li, D., Jiang, J., and Zheng, C. (2000). “Determination and Comparison of Ileum Apparent Metabolic Energy of Broilers from Different Sources of Corn,” in Proceedings of the 6th National Member Congress and the 8th Academic Symposium of animal nutrition branch of Chinese society of animal husbandry and veterinary medicine 10, 7–11.
Teng, N. (2015). “Vivo and In Vitro Study on the Antibacterial Effect of Quercetin,” (Harbin, China: Northeast Agricultural University). Doctoral Dissertation.
Terada, T., Nii, T., Isobe, N., and Yoshimura, Y. (2020). Effects of Probiotics Lactobacillus Reuteri and Clostridium Butyricum on the Expression of Toll-like Receptors, Pro- and Anti-inflammatory Cytokines, and Antimicrobial Peptides in Broiler Chick Intestine. J. Poult. Sci. 57, 310–318. doi:10.2141/jpsa.0190098
Van Dijk, A., Veldhuizen, E. J., Kalkhove, S. I., Tjeerdsma-van Bokhoven, J. L., Romijn, R. A., and Haagsman, H. P. (2006). The Beta-Defensin Gallinacin-6 Is Expressed in the Chicken Digestive Tract and Has Antimicrobial Activity against Food-Borne Pathogens. Antimicrob. Agents Chemother. 51 (3), 912–922. doi:10.1128/aac.0056810.1128/AAC.00568-06
Wang, S. (2018). “The Effect and Mechanism of Quercetin on Protein Digestion and Utilization of AA Broilers,” (Harbin, China: Northeast Agricultural University). Doctoral Dissertation.
Warden, A. S., Azzam, M., DaCosta, A., Mason, S., Blednov, Y. A., Messing, R. O., et al. (2019). Toll-like Receptor 3 Activation Increases Voluntary Alcohol Intake in C57BL/6J Male Mice. Brain Behav. Immun. 77 (77), 55–65. doi:10.1016/j.bbi.2018.12.004
Xu, Y., Zhang, T., Xu, Q., Han, Z., Liang, S., Shao, Y., et al. (2015). Differential Modulation of Avian β-defensin and Toll-like Receptor Expression in Chickens Infected with Infectious Bronchitis Virus. Appl. Microbiol. Biotechnol. 99 (21), 9011–9024. doi:10.1007/s00253-015-6786-8
Yang, J. X., Maria, T. C., Zhou, B., Xiao, F. L., Wang, M., Mao, Y. J., et al. (2020). Quercetin Improves Immune Function in Arbor Acre Broilers through Activation of NF-Κb Signaling Pathway. Poult. Sci. 99 (4), 2305. doi:10.1016/j.psj.2019.12.02110.1016/j.psj.2020.03.003
Ying, L., Chaudhry, M. T., Xiao, F., Mao, Y., Wang, M., Wang, B., et al. (2020). The Effects and Mechanism of Quercetin Dietary Supplementation in Streptozotocin-Induced Hyperglycemic Arbor Acre Broilers. Oxidative Med. Cell Longevity 2020, 1–11. doi:10.1155/2020/9585047
Yoshimura, Y. (2015). Avian β-defensins Expression for the Innate Immune System in Hen Reproductive Organs. Poult. Sci. 94, 804–809. doi:10.3382/ps/peu021
Zhang, G., and Sunkara, L. (2014). Avian Antimicrobial Host Defense Peptides: from Biology to Therapeutic Applications. Pharmaceuticals 7 (3), 220–247. doi:10.3390/ph7030220
Zhang, L., Lu, L., Li, S., Zhang, G., Ouyang, L., Robinson, K., et al. (2016). 1,25-Dihydroxyvitamin-D3 Induces Avian β-Defensin Gene Expression in Chickens. PLOS ONE 11 (5), e0154546. doi:10.1371/journal.pone.0154546
Keywords: quercetin, avian beta-defensin, Toll-like receptor, ileum, broilers
Citation: Ying L, Wu H, Zhou S, Lu H, Ding M, Wang B, Wang S, Mao Y, Xiao F and Li Y (2022) Toll-Like Receptors Signaling Pathway of Quercetin Regulating Avian Beta-Defensin in the Ileum of Broilers. Front. Cell Dev. Biol. 10:816771. doi: 10.3389/fcell.2022.816771
Received: 17 November 2021; Accepted: 31 January 2022;
Published: 23 February 2022.
Edited by:
Silvia Selleri, CHU Sainte-Justine, CanadaReviewed by:
Sheikh Fayaz Ahmad, King Saud University, Saudi ArabiaMengzhi Wang, Yangzhou University, China
Copyright © 2022 Ying, Wu, Zhou, Lu, Ding, Wang, Wang, Mao, Xiao and Li. This is an open-access article distributed under the terms of the Creative Commons Attribution License (CC BY). The use, distribution or reproduction in other forums is permitted, provided the original author(s) and the copyright owner(s) are credited and that the original publication in this journal is cited, in accordance with accepted academic practice. No use, distribution or reproduction is permitted which does not comply with these terms.
*Correspondence: Yao Li, bGl5YW9sendAMTYzLmNvbQ==