- 1PerciaVista R&D Co.Ltd, Shiraz, Iran
- 2Department of Oncology, West Kazakhstan Marat Ospanov Medical University, Aktobe, Kazakhstan
- 3Department of Surgery No. 2, West Kazakhstan Medical University, Aktobe, Kazakhstan
- 4Department of Basic Sciences, School of Veterinary Medicine, Shiraz University, Shiraz, Iran
Mesenchymal stromal/stem cell- (MSC-) derived exosomes are gaining popularity for their involvement in tissue repair and repressing various tumors through extensive patterns. Nevertheless, the impact of extracellular vesicles produced by stem cells on tumor formation and progression is controversial and seems to depend on several factors. The utilization of MSCs’ various capabilities in urogenital neoplasms is widely regarded as a potential future therapeutic as well. These genitourinary neoplasms include prostatic neoplasms, ovarian neoplasms, cervical neoplasms, endometrial neoplasms, bladder neoplasms, and renal cell neoplasms. The present study has concentrated on the most recent information on genitourinary neoplasms employing MSCs derived exosomes’ many capabilities, such as delivering effective RNAs, extensive tissue compatibility, and specificity with tumor identification without inherent limitations of cell therapy.
Mesenchymal stromal/stem cell (MSC-) derived exosomes and genitourinary neoplasms
MSCs are a population of multipotent cell lineage that may differentiate into distinct cell types and are found in several organs (Pittenger et al., 1999; Mehrabani et al., 2015). In addition to having the ability to divide themselves repeatedly, they also have the ability to engage directly with numerous immune cells to exhibit anti-inflammatory and immunosuppressive effects (Wang et al., 2014; Tamadon et al., 2015).
Previously exosomes have been widely studied and proposed as a tool for diagnosing various genitourinary neoplasms including ovarian cancer (Taylor and Gercel-Taylor, 2008), endometrial cancer (Maida et al., 2016), cervical cancer (Honegger et al., 2015), and prostate cancer (Vlaeminck-Guillem, 2018). Exosomes, membrane-bound vesicles with a diameter of a few nanometers, are certainly one of the most biocompatible forms of inter-cell messengers for regenerative medicine and medication administration (Hood, 2016).
Exosomes produced from MSCs (MSC-EXOs) are effective therapeutic carriers, and they seem to replicate the wide therapeutic benefits seen in MSCs (Madrigal et al., 2014; Khajehahmadi et al., 2016; Bazoobandi et al., 2020; Xunian and Kalluri, 2020). Previous data have suggested that MSCs may settle within tumors shown by the detection of MSCs integrated into tumors after injection (Kidd et al., 2009).
MSC-EXOs are of growing interest due to their role in tissue healing, which includes reducing inflammation and boosting the recovery of damaged tissue. However, the effect of stem cell-derived extracellular vesicles (EVs) on tumor development and progression is debatable and seems to be influenced by the EV source, the kind of tumor, and the mode of exosome therapy (Bruno et al., 2017; Vakhshiteh et al., 2019). When compared to liposomes of equivalent size, integration of exosomes into tumor cells is 10 times higher, demonstrating the improved selectivity of exosomes for tumor identification (Smyth et al., 2014), and compared to regular cells, tumor cells are more capable of internalizing exosomes (Greco et al., 2016).
The influence of exosomes produced by MSCs on genitourinary neoplasms, such as male, female, and urologic malignancies, has been compiled and presented in this review. The current review includes every study that has examined the use of MSC-derived exosomes in the treatment of urogenital neoplasms, which sums 16 studies. We have also shown how effectively exosomes can be used as potential new treatments in a separate table and figure as well (Table 1 and Figure 1). Extracellular vesicles, mesenchymal stem cells, and urogenital neoplasms were the search terms used in the PubMed data repository on November 2022. The inclusion of related studies was carried out without regarding chronological constraints and we omitted studies that have utilized total extracellular vesicles rather than exosomes specifically. It is worth mentioning the research’ increased publication frequency between 2016 and 2022 indicates that the topic is also receiving more attention.
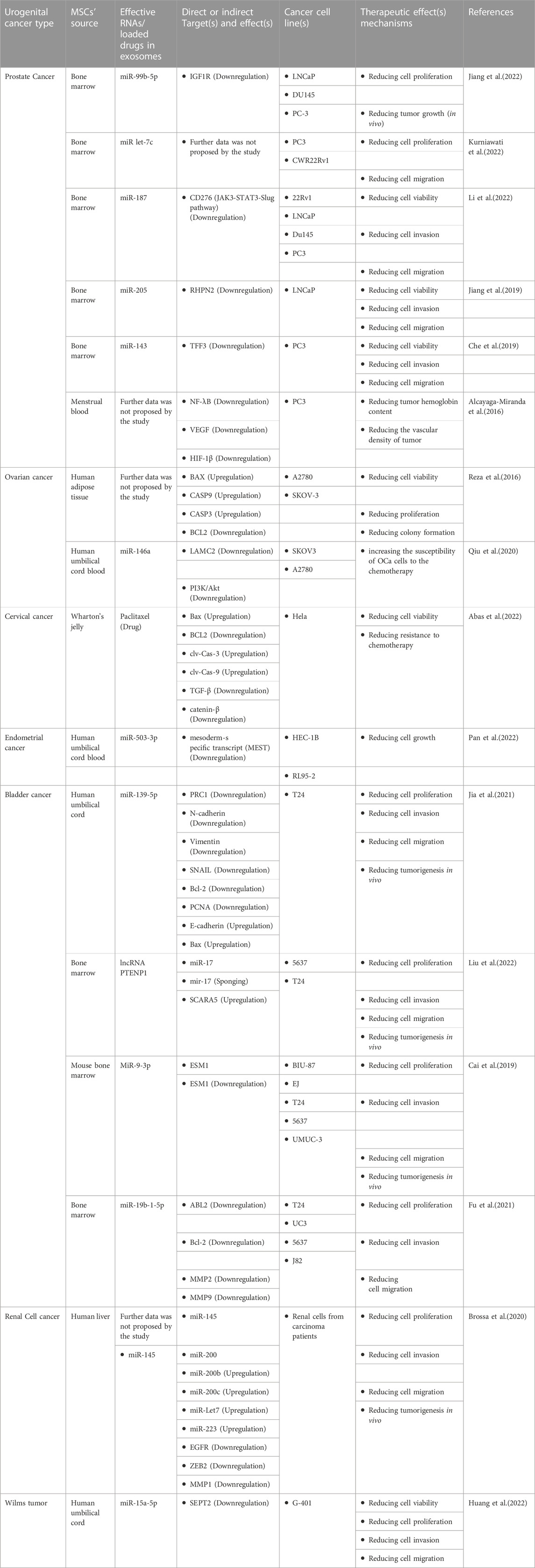
TABLE 1. Mesenchymal stromal/stem cell- (MSC-) derived exosomes and their therapeutic mechanism on genitourinary neoplasms.
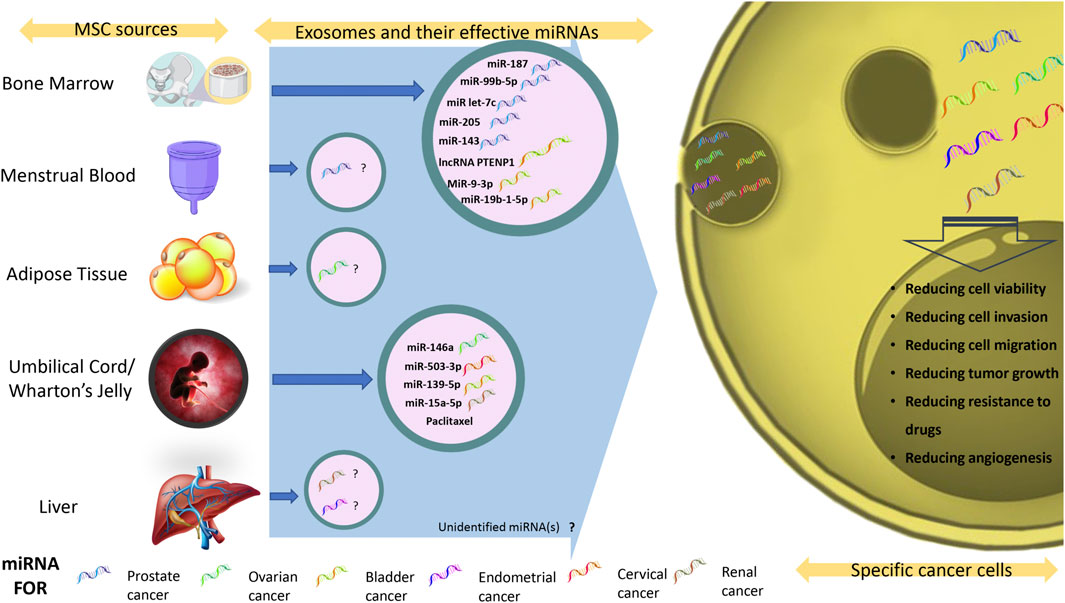
FIGURE 1. Exosomal miRNAs and their therapeutic effects against genitourinary neoplasms. Summary of all available exosomal miRNAs and their effect on genitourinary neoplasms.
Male genitourinary neoplasms and MSCs derived exosomes
Prostate cancer and MSCs derived exosomes
Secreted exosomes from MSCs are associated with their antitumor activity (Yeo et al., 2013). Messenger RNAs (mRNAs), microRNAs (miRNAs), and proteins may all be transported via exosomes (Lässer, 2012). MiRNAs are short, non-coding types of RNAs that often exhibit changes in gene expression in a variety of malignancies like prostate cancer (Sharma and Baruah, 2019).
In a study on patients with prostate cancer (PCa), it was revealed that expressions of hsa-let-7a-5p and hsa-miR-21-5p were elevated in the serum of the high-risk group after radiation therapy but hsa-miR-99b-5p was not substantially different (Malla et al., 2018). Further investigation revealed that miR-99b-5p was increased in exosomes produced from human bone marrow MSCs (hBM-MSCs) but downregulated in neoplastic prostate tissue. The underlying mechanism was proposed as by inhibiting IGF1R, hBM-MSCs-derived exosomes with miR-99b-5p overexpression may ameliorate the progression of PCa which might reveal higher therapeutic and prognostic potency of exosomes (Jiang et al., 2022).
Androgen deprivation therapy resistance and cancer recurrence are two characteristics of the progressive variant of malignant PCa namely Castration-resistant prostate cancer (CRPC) (Landstrom et al., 1994; Divrik et al., 2012). Recent research showed that miR-let-7c has tumor-suppressive properties by inhibiting the multiplication and expansion of CRPC-like cells as well. In CRPC-like cell lines (PC3 and CWR22Rv1), incubation with either intact MSC-EXOs or MSC exosomes with miR let-7c enrichment significantly decreased the proliferation and metastasis tendency of the cells (Kurniawati et al., 2022).
It was discovered that exosomal miR-187 from human bone marrow-derived MSCs (hBM-MSC) inhibited the malignant characteristics of PCa cells. It may be established that miR-187 inhibits the JAK3-STAT3-Slug pathway in PCa by targeting CD276. PCa cell aggressive behaviors were decreased by exosomes from hBM-MSC with overexpression of miR-187, suggesting that these exosomes may represent a feasible therapeutic target (Li et al., 2022).
The impact of exosomes produced from hBM-MSCs overexpressing miR-205 on the advancement of PCa was investigated, and the findings indicated that the transfer of miR-205 from hBMSCs to PCa cells by modified exosomes reduces multiplication, redistribution, and penetration of PCa cells through adhering to the 3′UTR of RHPN2 which led to its suppression. (Jiang et al., 2019).
According to research, TFF3 expression rises in PCa-related tissues and cells whereas miR-143 expression falls. This particular idea was utilized and it was demonstrated that exosomes from human BM-MSCs with overexpression of miR-143 may prevent PCa cells (PC3) from proliferating, migrating, or invading while promoting apoptosis by suppressing TFF3. This was further shown by the decrease in PCNA, MMP-2, and MMP-9 production levels in cells exposed to exosomal miR-143. Eventually, to elucidate underlying processes, more investigation utilizing in vivo models is suggested (Che et al., 2019).
Exosomes were shown to be responsible for extinguishing NF-κB activity and reducing reactive oxygen species (ROS) formation in vitro, according to research on cells. Also, The PC3 cells used in the angiogenesis plug experiment (in vivo) showed diminished angiogenic characteristics (loss of VEGF and HIF-1 tissue expression) and decreased hemoglobin content within the plugs, which is presumably repercussions of the lowered ROS generation generated by menstrual stem cells derived exosomes as well (Alcayaga-Miranda et al., 2016).
MiR-99b-5p, miR let-7c, miR-187, miR-205, miR-143, and MSC-EXOs have all been claimed to be capable of reducing PCa development. For PCa studies, researchers have preferred to use the PC3 and later the LNCaP cell lines. Also, bone marrow has been the primary source of extracting MSCs in PCa trials. A common route (JAK3-STAT3-Slug pathway), which is regarded to be helpful in reducing tumor development, may also get greater attention in future investigations.
Female genitourinary neoplasms and MSCs derived exosomes
Ovarian cancer and MSCs derived exosomes
Prior research suggested that MSCs are important in preventing the development of Ovarian cancer (OCa) (Khalil et al., 2019). Additionally, mature MSCs play a crucial role in cell signaling as important tumor-targeted conveyance agents by depositing EVs that are highly enriched with miRNAs (O'Brien et al., 2018). In terms of the most prevalent malignancies that result in mortality, OCa is in eighth place (Webb and Jordan, 2017; Wang et al., 2022).
Analysis indicated that overexpression excessive expression of the exosomal miRNA hsa-miR-124-3p resulted in the suppression of many cyclin-dependent kinases (CDKs), including CDK2, CDK4, and CDK6. Likewise, uptake of exosomes led to lower proliferation and colony formation in A2780 cells than exposure to human adipose MSC condition media (hA-MSC-CM) alone, proving that exosomes originating from hA-MSC-CM are significant contributors to the suppression of A2780 cell division. It was supposed that generated vesicles enhanced autophagy by upregulating a variety of pro-apoptotic signaling molecules, including BAX, CASP9, and CASP3, and downregulating the anti-apoptotic protein BCL2 (Reza et al., 2016).
A study found that hUC-MSCs could efficiently transfer miR-146a into released exosomes, increasing the susceptibility of OCa cells to the chemotherapeutic drugs docetaxel and taxane by lowering LAMC2 expression and blocking the PI3K/Akt signaling pathway (a characteristic for many cancer types). By examining the impact of miR-146a inhibitors on cancer cell growth and apoptosis, these findings were further confirmed with reversed consequences as well (Qiu et al., 2020).
The PI3K/Akt signaling pathway has been proven to be intriguing to target in future studies for minimizing resistance to commonly used treatments like chemotherapy, and other therapies may be evaluated in the same trend. As it is noticeable, two preferred cell lines for OCa research have been SKOV3 and A2780.
Cervical neoplasm and MSCs derived exosomes
Exosomes from human umbilical cord Wharton’s jelly MSCs were used in a study to load Paclitaxel and test the exosomes’ effects on cervical cancer (Hela) cell lines. As a consequence, cancer cell death was accelerated by influencing the levels of Bax, BCL2, clv-Cas-3, and clv-Cas-9, and chemoresistance was decreased by affecting epithelial-mesenchymal transition (EMT)-related proteins (such as TGF-β and catenin-β) (Abas et al., 2022).
Endometrial neoplasm and MSCs derived exosomes
In order to ascertain their role in EC cell biological processes, human endometrial cancer (EC) cell lines were co-cultured with Exosomes or treated with upregulated miR-503-3p or suppressed mesoderm-specific transcript (MEST) vectors. MiR-503-3p upregulation or MEST downregulation inhibited the biological activities of EC cells. Additionally, it was revealed that exosomes produced by human umbilical cord mesenchymal stem cell-derived exosomes (hUC-MSC) inhibited the development of EC cells, while exosomes with elevated miR-503-3p content from the same resource exhibited a more pronounced regulatory impact (Pan et al., 2022).
Urologic neoplasms and MSCs derived exosomes
Bladder neoplasm and MSCs derived exosomes
The gene PRC1 may be a novel target for miR-139-5p, according to recent research. Additionally, PRC1’s role as a carcinogen in bladder cancer cells has been confirmed. Results showed that deletion of PRC1 inhibited bladder cancer cells’ ability to proliferate and spread, as well as halted the induction of EMT, which was shown by changes in the production of E-cadherin, and Bax and decreases in the synthesis of N-cadherin, Vimentin, SNAIL, Bcl-2, and PCNA. hUC-MSC-derived exosomes’ effective delivery of miR-139-5p to T24 cells was verified as well. Additionally, the introduction of miR-139-5p from hUC-MSC-derived exosomes resulted in the reduction of bladder carcinogenesis in nude mice as well as the suppression of T24 cell growth, penetration, and expansion (Jia et al., 2021).
Exosomal long non-coding RNA (lncRNA) PTENP1, which is released by hBM-MSCs, inhibits the aggressive phenotypes of Bladder cancer cells (5637 and T24) by controlling the miR-17/SCARA5 axis. In order to promote the expression of SCARA5, exosomal lncRNA PTENP1 sponges miR-17 to prevent bladder cancer cell malignant behaviors as the mechanism (Liu et al., 2022).
Recently researchers used mouse BM-MSC-generated exosomes to suppress the survivability, metastasis, and invasion of bladder cancer cells while promoting apoptosis. MiR-9-3p was shown to be an efficient agent that targets ESM1 and has been shown to have anti-oncogenic effects on bladder neoplasia-specific cell lines (BIU-87, EJ, T24, 5637, and UMUC-3) and BALB/c nude mice model of bladder cancer. As the underlying mechanism, following the treatment of Exo-miR-nine to three, the protein expression levels of proliferation-associated proteins (Ki67 and PCNA) and invasion-associated indicators (MMP-2 and MMP-9) dropped significantly (Cai et al., 2019).
ABL2 is up-regulated while miR-19b-1-5p is down-regulated in bladder cancer tissues and cell lines, according to another research. Recently, the research revealed that BMSCs-derived exosomal miR-19b-1-5p could inhibit bladder cancer cell lines (T24, UC3, 5637, and J82) from migrating, proliferating, or invading while promoting cell death via inhibiting ABL2, Bcl-2, MMP2 and MMP9 (Fu et al., 2021).
The most favored cell lines for bladder tumor therapy with MSC-EXOs have been T24 and 5637. Cell proliferation, invasion, migration, and further in vivo experiments after using exosomes were the most applied confirming methods in these researches. While a study has revealed that targeting miRNAs may be successful instead of utilizing them to target other cellular markers, it is also considered a further step toward cell-free treatment. This specific strategy seems feasible in the future as the functions of exosomal miRNAs are becoming more understood.
Renal cell neoplasm and MSCs derived exosomes
Stem cells with mesenchymal origin have shown promising results in renal cell neoplasm as well. In a study, researchers examined the impact of EVs produced from human liver stem cells (hL-SCs) with mesenchymal origin both in vitro and in vivo. In SCID mice, pretreatment of renal cancer stem cells with EVs prior to subcutaneous infusion slowed tumor initiation. By lowering tumor angiogenesis and triggering tumor cell death, hL-SCs-derived EVs dramatically reduced subcutaneous tumor development too. In tumor explants that had received treatment from EVs, upregulation of the miR-200 family members, miR-Let7, and miR-223 was obsereved, and the delivery of mir-145 by EVs was considered to be effective. Their target genes, such as EGFR, ZEB2, and MMP1, were inhibited with diminishing impacts on cell survival, invasion, and proliferation in transfected cancer stem cells eventually (Brossa et al., 2020).
Wilms tumor and MSCs derived exosomes
Wilms tumor (WT), the most common kidney carcinoma in children, is an embryonic carcinoma without a definitive cure (Millar et al., 2017). According to a recent study, Septin 2 (SEPT2) loss-of-function in G-401 cells (WT cells) reduced proliferation, migration, and invasion while inducing apoptosis emphasizing the role of SEPT2 on tumorigenesis in WT. They proposed that SEPT2 could be targeted by miR-15a-5p-loaded hUC-MSCs derived exosomes that inhibited WT development significantly as a new means of therapeutics (Huang et al., 2022).
Conclusion
Exosomes are single-membrane vesicles with a similar structure as cells and are generally considered the size between 30 and 200 nm (Chernyshev et al., 2015), a density of ∼1.1–1.2 g/ml (Raposo et al., 1996) and enrichment with marker proteins of CD81, CD63, and CD9 (Escola et al., 1998; Hemler, 2003). Exosomes also transport extracellular RNAs in active form to neighboring cells and tissues (Ratajczak et al., 2006). Small non-coding RNAs (ncRNAs), small nuclear RNAs (snRNAs), miRNAs, transfer RNAs (tRNAs), YRNAs, vault RNAs, repetitive element RNAs, and fragmented RNAs, including 3′mRNA remnants, appear to be abundant in exosomes (Shurtleff et al., 2017; Wei et al., 2017).
MSC-EXOs have recently been revealed to have the ability to repair tissue injuries and may be used to cure cancer (Keshtkar et al., 2018). Exosomes are smaller, less complex, and less immunogenic than their producing cells because they have fewer membrane-bound polypeptides (Lou et al., 2017). Thus, they do not cause tumor development or acute immunological rejection (Chen et al., 2011). Exosomes may be produced in significant quantities by MSCs in vitro (Yeo et al., 2013) and the overall safety of exosomes has been shown in vivo by several animal studies (Sun et al., 2016). This topic of stem cell therapy has received a great deal of interest, which may be attributed to all these aspects.
Future insights
There are still unexplored areas that need to be studied in the future for even better outcomes. As (Jiang et al., 2022) stated, some already known downstream targets of miR-99b-5p like FGFR3 (Ding et al., 2021) and MFG-E8 (Lu et al., 2021) have the potential to be examined as potential therapeutics in the future (Kurniawati et al., 2022). also has shown the effectiveness of miR let-7c exosomes but the direct targets have not been detected by the study.
Other studies provided a distinctive insight as well. Although (Alcayaga-Miranda et al., 2016) did not provide a specific effective factor in exosomes, they utilized menstrual blood as a source of MSCs (Mens-SCs) which may possess superior qualities compared to other sources like bone marrow which seems to be preferred by researchers of this field by now. Menstrual blood stem cells secreted EVs may be more favorable for therapeutic use than whole cells either because of their ready-to-use potential, improved treatment efficacy, the introduction of fewer antigens, and prevention of immune responses. However, due to their fast clearance from the body, continuous treatment in substantial amounts is required (Bozorgmehr et al., 2020).
Furthermore, the difficulty of differentiating hBM-MSC is a limiting problem since it necessitates invasive surgery and donors’ ethical concerns, even though hBM-MSCs have received significant emphasis and dominating research (Chen et al., 2019). Mens-SCs can be delivered in huge volumes of cells by intravenous injection, they are safe and dependable (Wang et al., 2014), have shown no signs of tumor development or toxicity, and are capable of significantly slowing tumor development (Wang et al., 2017). Thus, they can be utilized more frequently in the future.
The majority of research has shown how miRNAs may improve tumorigenesis status, as demonstrated by Table 1. However, it has been hypothesized by (Liu et al., 2022) that miRNAs themselves may potentially be targeted. Similar therapeutic benefits may be obtained by employing lncRNAs, much as when miRNAs are first used for delivery. This concept is potentially beneficial and is one that might be adopted in the future.
In the end, it is noteworthy to highlight that different exosome cargos, including proteins, lipids, or RNAs, may contribute to the identified alleviating effects on cancer cells or tumors. Future research should be able to adequately demonstrate the effectiveness of a single exosome cargo if that is the focus of the investigation.
Author contributions
AS, SB, NM, and FR conceived and designed the format of the manuscript. AS drafted and edited the manuscript. SB, NM, and FR reviewed the manuscript. All authors contributed to the critical reading and discussion of the manuscript. All authors have read and agreed to the published version of the manuscript.
Conflict of interest
Author AS is employed by PerciaVista R&D Co.Ltd.
The remaining authors declare that the research was conducted in the absence of any commercial or financial relationships that could be construed as a potential conflict of interest.
Publisher’s note
All claims expressed in this article are solely those of the authors and do not necessarily represent those of their affiliated organizations, or those of the publisher, the editors and the reviewers. Any product that may be evaluated in this article, or claim that may be made by its manufacturer, is not guaranteed or endorsed by the publisher.
Abbreviations
CDKs, cyclin-dependent kinases; PCa, Prostate cancer; CRPC, Castration-resistant prostate cancer; EC, Endometrial cancer; EVs, Extracellular vesicles; EXO, Exosome; hA-MSC-CM, human adipose tissue-derived mesenchymal stromal/stem cell condition media; hBM-MSC, Human bone marrow-derived mesenchymal stromal/stem cell; hL-SCs, Human liver stem cells; hUC-MSC, human umbilical cord-derived mesenchymal stromal/stem cell; lncRNA, Long non-coding RNA; Men-SCs, Menstrual blood-derived stem cells; MEST, mesoderm-specific transcript; miRNA, microRNA; mir, microRNA; MSC, Mesenchymal stromal/stem cell; MSC-EXO, Mesenchymal stromal/stem cell-derived exosome; OCa, Ovarian cancer; ROS, Reactive oxygen species; SEPT2, Septin 2; WT, Wilms tumor.
References
Abas, B. I., Demirbolat, G. M., and Cevik, O. (2022). Wharton jelly-derived mesenchymal stem cell exosomes induce apoptosis and suppress EMT signaling in cervical cancer cells as an effective drug carrier system of paclitaxel. PLoS One 17, e0274607. doi:10.1371/journal.pone.0274607
Alcayaga-Miranda, F., Gonzalez, P. L., Lopez-Verrilli, A., Varas-Godoy, M., Aguila-Diaz, C., Contreras, L., et al. (2016). Prostate tumor-induced angiogenesis is blocked by exosomes derived from menstrual stem cells through the inhibition of reactive oxygen species. Oncotarget 7, 44462–44477. doi:10.18632/oncotarget.9852
Bazoobandi, S., Tanideh, N., Rahmanifar, F., Zare, S., Koohi-Hosseinabadi, O., Razeghian-Jahromi, I., et al. (2020). Preventive effects of intrauterine injection of bone marrow-derived mesenchymal stromal cell-conditioned media on uterine fibrosis immediately after endometrial curettage in rabbit. Stem Cells Int. 2020, 8849537. doi:10.1155/2020/8849537
Bozorgmehr, M., Gurung, S., Darzi, S., Nikoo, S., Kazemnejad, S., Zarnani, A. H., et al. (2020). Endometrial and menstrual blood mesenchymal stem/stromal cells: Biological properties and clinical application. Front. Cell Dev. Biol. 8, 497. doi:10.3389/fcell.2020.00497
Brossa, A., Fonsato, V., Grange, C., Tritta, S., Tapparo, M., Calvetti, R., et al. (2020). Extracellular vesicles from human liver stem cells inhibit renal cancer stem cell-derived tumor growth in vitro and in vivo. Int. J. Cancer 147, 1694–1706. doi:10.1002/ijc.32925
Bruno, S., Tapparo, M., Collino, F., Chiabotto, G., Deregibus, M. C., Soares Lindoso, R., et al. (2017). Renal regenerative potential of different extracellular vesicle populations derived from bone marrow mesenchymal stromal cells. Tissue Eng. Part A 23, 1262–1273. doi:10.1089/ten.TEA.2017.0069
Cai, H., Yang, X., Gao, Y., Xu, Z., Yu, B., Xu, T., et al. (2019). Exosomal MicroRNA-9-3p secreted from BMSCs downregulates ESM1 to suppress the development of bladder cancer. Mol. Ther. Nucleic Acids 18, 787–800. doi:10.1016/j.omtn.2019.09.023
Che, Y., Shi, X., Shi, Y., Jiang, X., Ai, Q., Shi, Y., et al. (2019). Exosomes derived from miR-143-overexpressing MSCs inhibit cell migration and invasion in human prostate cancer by downregulating TFF3. Mol. Ther. Nucleic Acids 18, 232–244. doi:10.1016/j.omtn.2019.08.010
Chen, L., Qu, J., and Xiang, C. (2019). The multi-functional roles of menstrual blood-derived stem cells in regenerative medicine. Stem Cell Res. Ther. 10, 1. doi:10.1186/s13287-018-1105-9
Chen, T. S., Arslan, F., Yin, Y., Tan, S. S., Lai, R. C., Choo, A. B., et al. (2011). Enabling a robust scalable manufacturing process for therapeutic exosomes through oncogenic immortalization of human ESC-derived MSCs. J. Transl. Med. 9, 47. doi:10.1186/1479-5876-9-47
Chernyshev, V. S., Rachamadugu, R., Tseng, Y. H., Belnap, D. M., Jia, Y., Branch, K. J., et al. (2015). Size and shape characterization of hydrated and desiccated exosomes. Anal. Bioanal. Chem. 407, 3285–3301. doi:10.1007/s00216-015-8535-3
Ding, M., Liu, B., Chen, X., Ouyang, Z., Peng, D., and Zhou, Y. (2021). MiR-99b-5p suppressed proliferation of human osteoblasts by targeting FGFR3 in osteoporosis. Hum. Cell 34, 1398–1409. doi:10.1007/s13577-021-00567-3
Divrik, R. T., Turkeri, L., Sahin, A. F., Akdogan, B., Ates, F., Cal, C., et al. (2012). Prediction of response to androgen deprivation therapy and castration resistance in primary metastatic prostate cancer. Urol. Int. 88, 25–33. doi:10.1159/000334539
Escola, J. M., Kleijmeer, M. J., Stoorvogel, W., Griffith, J. M., Yoshie, O., and Geuze, H. J. (1998). Selective enrichment of tetraspan proteins on the internal vesicles of multivesicular endosomes and on exosomes secreted by human B-lymphocytes. J. Biol. Chem. 273, 20121–20127. doi:10.1074/jbc.273.32.20121
Fu, D., Liu, B., Jiang, H., Li, Z., Fan, C., and Zang, L. (2021). Bone marrow mesenchymal stem cells-derived exosomal microRNA-19b-1-5p reduces proliferation and raises apoptosis of bladder cancer cells via targeting ABL2. Genomics 113, 1338–1348. doi:10.1016/j.ygeno.2021.03.011
Greco, K. A., Franzen, C. A., Foreman, K. E., Flanigan, R. C., Kuo, P. C., and Gupta, G. N. (2016). PLK-1 silencing in bladder cancer by siRNA delivered with exosomes. Urology 91, 241 e1–e7. doi:10.1016/j.urology.2016.01.028
Hemler, M. E. (2003). Tetraspanin proteins mediate cellular penetration, invasion, and fusion events and define a novel type of membrane microdomain. Annu. Rev. Cell Dev. Biol. 19, 397–422. doi:10.1146/annurev.cellbio.19.111301.153609
Honegger, A., Schilling, D., Bastian, S., Sponagel, J., Kuryshev, V., Sultmann, H., et al. (2015). Dependence of intracellular and exosomal microRNAs on viral E6/E7 oncogene expression in HPV-positive tumor cells. PLoS Pathog. 11, e1004712. doi:10.1371/journal.ppat.1004712
Hood, J. L. (2016). Post isolation modification of exosomes for nanomedicine applications. Nanomedicine (Lond) 11, 1745–1756. doi:10.2217/nnm-2016-0102
Huang, H., Zhong, P., Zhang, J., Chen, X., Chen, J., Lin, T., et al. (2022). Human umbilical cord-mesenchymal stem cells-derived exosomes carrying microRNA-15a-5p possess therapeutic effects on Wilms tumor via regulating septin 2. Bioengineered 13, 6136–6149. doi:10.1080/21655979.2022.2037379
Jia, Y., Ding, X., Zhou, L., Zhang, L., and Yang, X. (2021). Mesenchymal stem cells-derived exosomal microRNA-139-5p restrains tumorigenesis in bladder cancer by targeting PRC1. Oncogene 40, 246–261. doi:10.1038/s41388-020-01486-7
Jiang, S., Chen, H., He, K., and Wang, J. (2022). Human bone marrow mesenchymal stem cells-derived exosomes attenuated prostate cancer progression via the miR-99b-5p/IGF1R axis. Bioengineered 13, 2004–2016. doi:10.1080/21655979.2021.2009416
Jiang, S., Mo, C., Guo, S., Zhuang, J., Huang, B., and Mao, X. (2019). Human bone marrow mesenchymal stem cells-derived microRNA-205-containing exosomes impede the progression of prostate cancer through suppression of RHPN2. J. Exp. Clin. Cancer Res. 38, 495. doi:10.1186/s13046-019-1488-1
Keshtkar, S., Azarpira, N., and Ghahremani, M. H. (2018). Mesenchymal stem cell-derived extracellular vesicles: Novel frontiers in regenerative medicine. Stem Cell Res. Ther. 9, 63. doi:10.1186/s13287-018-0791-7
Khajehahmadi, Z., Mehrabani, D., Ashraf, M. J., Rahmanifar, F., Tanideh, N., Tamadon, A., et al. (2016). Healing effect of conditioned media from bone marrow-derived stem cells in thioacetamide-induced liver fibrosis of rat. J. Med. Sci. 16, 7–15. doi:10.3923/jms.2016.7.15
Khalil, C., Moussa, M., Azar, A., Tawk, J., Habbouche, J., Salameh, R., et al. (2019). Anti-proliferative effects of mesenchymal stem cells (MSCs) derived from multiple sources on ovarian cancer cell lines: An in-vitro experimental study. J. Ovarian Res. 12, 70. doi:10.1186/s13048-019-0546-9
Kidd, S., Spaeth, E., Dembinski, J. L., Dietrich, M., Watson, K., Klopp, A., et al. (2009). Direct evidence of mesenchymal stem cell tropism for tumor and wounding microenvironments using in vivo bioluminescent imaging. Stem Cells 27, 2614–2623. doi:10.1002/stem.187
Kurniawati, I., Liu, M. C., Hsieh, C. L., Do, A. D., and Sung, S. Y. (2022). Targeting castration-resistant prostate cancer using mesenchymal stem cell exosomes for therapeutic MicroRNA-let-7c delivery. Front. Biosci. (Landmark Ed. 27, 256. doi:10.31083/j.fbl2709256
Landstrom, M., Damber, J. E., and Bergh, A. (1994). Prostatic tumor regrowth after initially successful castration therapy may be related to a decreased apoptotic cell death rate. Cancer Res. 54, 4281–4284.
Lässer, C. (2012). Exosomal RNA as biomarkers and the therapeutic potential of exosome vectors. Expert Opin. Biol. Ther. 12 (1), S189–S197. doi:10.1517/14712598.2012.680018
Li, C., Sun, Z., Song, Y., and Zhang, Y. (2022). Suppressive function of bone marrow-derived mesenchymal stem cell-derived exosomal microRNA-187 in prostate cancer. Cancer Biol. Ther. 23, 1–14. doi:10.1080/15384047.2022.2123675
Liu, S. C., Cao, Y. H., Chen, L. B., Kang, R., Huang, Z. X., and Lu, X. S. (2022). BMSC-derived exosomal lncRNA PTENP1 suppresses the malignant phenotypes of bladder cancer by upregulating SCARA5 expression. Cancer Biol. Ther. 23, 1–13. doi:10.1080/15384047.2022.2102360
Lou, G., Chen, Z., Zheng, M., and Liu, Y. (2017). Mesenchymal stem cell-derived exosomes as a new therapeutic strategy for liver diseases. Exp. Mol. Med. 49, e346. doi:10.1038/emm.2017.63
Lu, Y., Liu, L., Pan, J., Luo, B., Zeng, H., Shao, Y., et al. (2021). MFG-E8 regulated by miR-99b-5p protects against osteoarthritis by targeting chondrocyte senescence and macrophage reprogramming via the NF-κB pathway. Cell Death Dis. 12, 533. doi:10.1038/s41419-021-03800-x
Madrigal, M., Rao, K. S., and Riordan, N. H. (2014). A review of therapeutic effects of mesenchymal stem cell secretions and induction of secretory modification by different culture methods. J. Transl. Med. 12, 260. doi:10.1186/s12967-014-0260-8
Maida, Y., Takakura, M., Nishiuchi, T., Yoshimoto, T., and Kyo, S. (2016). Exosomal transfer of functional small RNAs mediates cancer-stroma communication in human endometrium. Cancer Med. 5, 304–314. doi:10.1002/cam4.545
Malla, B., Aebersold, D. M., and Dal Pra, A. (2018). Protocol for serum exosomal miRNAs analysis in prostate cancer patients treated with radiotherapy. J. Transl. Med. 16, 223. doi:10.1186/s12967-018-1592-6
Mehrabani, D., Rahmanifar, F., Mellinejad, M., Tamadon, A., Dianatpour, M., Zare, S., et al. (2015). Isolation, culture, characterization, and adipogenic differentiation of heifer endometrial mesenchymal stem cells. Comp. Clin. Pathol. 24, 1159–1164.
Millar, A. J. W., Cox, S., and Davidson, A. (2017). Management of bilateral Wilms tumours. Pediatr. Surg. Int. 33, 461–469. doi:10.1007/s00383-016-4047-2
O'Brien, K. P., Khan, S., Gilligan, K. E., Zafar, H., Lalor, P., Glynn, C., et al. (2018). Employing mesenchymal stem cells to support tumor-targeted delivery of extracellular vesicle (EV)-encapsulated microRNA-379. Oncogene 37, 2137–2149. doi:10.1038/s41388-017-0116-9
Pan, Y., Wang, X., Li, Y., Yan, P., and Zhang, H. (2022). Human umbilical cord blood mesenchymal stem cells-derived exosomal microRNA-503-3p inhibits progression of human endometrial cancer cells through downregulating MEST. Cancer Gene Ther. 29, 1130–1139. doi:10.1038/s41417-021-00416-3
Pittenger, M. F., Mackay, A. M., Beck, S. C., Jaiswal, R. K., Douglas, R., Mosca, J. D., et al. (1999). Multilineage potential of adult human mesenchymal stem cells. Science 284, 143–147.
Qiu, L., Wang, J., Chen, M., Chen, F., and Tu, W. (2020). Exosomal microRNA-146a derived from mesenchymal stem cells increases the sensitivity of ovarian cancer cells to docetaxel and taxane via a LAMC2-mediated PI3K/Akt axis. Int. J. Mol. Med. 46, 609–620. doi:10.3892/ijmm.2020.4634
Raposo, G., Nijman, H. W., Stoorvogel, W., Liejendekker, R., Harding, C. V., Melief, C. J., et al. (1996). B lymphocytes secrete antigen-presenting vesicles. J. Exp. Med. 183, 1161–1172. doi:10.1084/jem.183.3.1161
Ratajczak, J., Miekus, K., Kucia, M., Zhang, J., Reca, R., Dvorak, P., et al. (2006). Embryonic stem cell-derived microvesicles reprogram hematopoietic progenitors: Evidence for horizontal transfer of mRNA and protein delivery. Leukemia 20, 847–856. doi:10.1038/sj.leu.2404132
Reza, A., Choi, Y. J., Yasuda, H., and Kim, J. H. (2016). Human adipose mesenchymal stem cell-derived exosomal-miRNAs are critical factors for inducing anti-proliferation signalling to A2780 and SKOV-3 ovarian cancer cells. Sci. Rep. 6, 38498. doi:10.1038/srep38498
Sharma, N., and Baruah, M. M. (2019). The microRNA signatures: Aberrantly expressed miRNAs in prostate cancer. Clin. Transl. Oncol. 21, 126–144. doi:10.1007/s12094-018-1910-8
Shurtleff, M. J., Yao, J., Qin, Y., Nottingham, R. M., Temoche-Diaz, M. M., Schekman, R., et al. (2017). Broad role for YBX1 in defining the small noncoding RNA composition of exosomes. Proc. Natl. Acad. Sci. U. S. A. 114, E8987–E8995. doi:10.1073/pnas.1712108114
Smyth, T. J., Redzic, J. S., Graner, M. W., and Anchordoquy, T. J. (2014). Examination of the specificity of tumor cell derived exosomes with tumor cells in vitro. Biochim. Biophys. Acta 1838, 2954–2965. doi:10.1016/j.bbamem.2014.07.026
Sun, L., Xu, R., Sun, X., Duan, Y., Han, Y., Zhao, Y., et al. (2016). Safety evaluation of exosomes derived from human umbilical cord mesenchymal stromal cell. Cytotherapy 18, 413–422. doi:10.1016/j.jcyt.2015.11.018
Tamadon, A., Mehrabani, D., Rahmanifar, F., Jahromi, A. R., Panahi, M., Zare, S., et al. (2015). Induction of spermatogenesis by bone marrow-derived mesenchymal stem cells in busulfan-induced azoospermia in hamster. Int. J. stem cells 8, 134–145. doi:10.15283/ijsc.2015.8.2.134
Taylor, D. D., and Gercel-Taylor, C. (2008). MicroRNA signatures of tumor-derived exosomes as diagnostic biomarkers of ovarian cancer. Gynecol. Oncol. 110, 13–21. doi:10.1016/j.ygyno.2008.04.033
Vakhshiteh, F., Atyabi, F., and Ostad, S. N. (2019). Mesenchymal stem cell exosomes: A two-edged sword in cancer therapy. Int. J. Nanomedicine 14, 2847–2859. doi:10.2147/IJN.S200036
Vlaeminck-Guillem, V. (2018). Extracellular vesicles in prostate cancer carcinogenesis, diagnosis, and management. Front. Oncol. 8, 222. doi:10.3389/fonc.2018.00222
Wang, X., Jiang, L., and Liu, Q. (2022). miR-18a-5p derived from mesenchymal stem cells-extracellular vesicles inhibits ovarian cancer cell proliferation, migration, invasion, and chemotherapy resistance. J. Transl. Med. 20, 258. doi:10.1186/s12967-022-03422-7
Wang, X. J., Xiang, B. Y., Ding, Y. H., Chen, L., Zou, H., Mou, X. Z., et al. (2017). Human menstrual blood-derived mesenchymal stem cells as a cellular vehicle for malignant glioma gene therapy. Oncotarget 8, 58309–58321. doi:10.18632/oncotarget.17621
Wang, Y., Chen, X., Cao, W., and Shi, Y. (2014). Plasticity of mesenchymal stem cells in immunomodulation: Pathological and therapeutic implications. Nat. Immunol. 15, 1009–1016. doi:10.1038/ni.3002
Webb, P. M., and Jordan, S. J. (2017). Epidemiology of epithelial ovarian cancer. Best. Pract. Res. Clin. Obstet. Gynaecol. 41, 3–14. doi:10.1016/j.bpobgyn.2016.08.006
Wei, Z., Batagov, A. O., Schinelli, S., Wang, J., Wang, Y., El Fatimy, R., et al. (2017). Coding and noncoding landscape of extracellular RNA released by human glioma stem cells. Nat. Commun. 8, 1145. doi:10.1038/s41467-017-01196-x
Xunian, Z., and Kalluri, R. (2020). Biology and therapeutic potential of mesenchymal stem cell-derived exosomes. Cancer Sci. 111, 3100–3110. doi:10.1111/cas.14563
Keywords: Mesenchymal Stromal/Stem Cell, MSCs, Exosomes, miRNAs, Urogenital neoplasms, Genitourinary neoplasms, Reproductive neoplasms, Reproductive cancers
Citation: Salehpour A, Balmagambetova S, Mussin N, Kaliyev A and Rahmanifar F (2023) Mesenchymal stromal/stem cell-derived exosomes and genitourinary cancers: A mini review. Front. Cell Dev. Biol. 10:1115786. doi: 10.3389/fcell.2022.1115786
Received: 04 December 2022; Accepted: 14 December 2022;
Published: 04 January 2023.
Edited by:
Reza Shirazi, UNSW Sydney, AustraliaReviewed by:
Ulanbek Zhanbyrbekuly, Astana Medical University, KazakhstanMohammad Saied Salehi, Shiraz University of Medical Sciences, Iran
Sahar Almasi-Turk, Bushehr University of Medical Sciences, Iran
Copyright © 2023 Salehpour, Balmagambetova, Mussin, Kaliyev and Rahmanifar. This is an open-access article distributed under the terms of the Creative Commons Attribution License (CC BY). The use, distribution or reproduction in other forums is permitted, provided the original author(s) and the copyright owner(s) are credited and that the original publication in this journal is cited, in accordance with accepted academic practice. No use, distribution or reproduction is permitted which does not comply with these terms.
*Correspondence: Farhad Rahmanifar, cmFobWFuaWZhckBzaGlyYXp1LmFjLmly, Zi5yYWhtYW5pZmFyQHlhaG9vLmNvbQ==
†ORCID: Aria Salehpour, orcid.org/0000-0002-6592-5202; Saule Balmagambetova, orcid.org/0000-0003-4080-5383; Nadiar Mussin, orcid.org/0000-0003-3600-8840; Asset Kaliyev, orcid.org/0000-0003-4594-4104; Farhad Rahmanifar, orcid.org/0000-0001-9585-7266