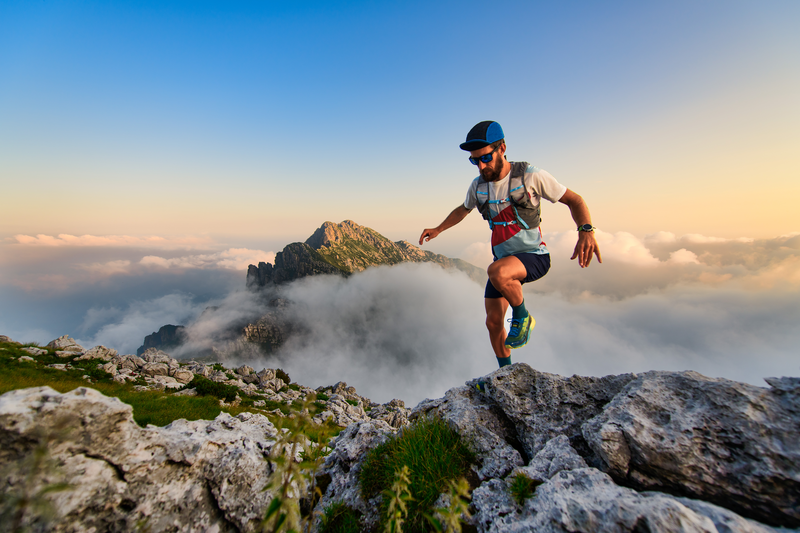
94% of researchers rate our articles as excellent or good
Learn more about the work of our research integrity team to safeguard the quality of each article we publish.
Find out more
OPINION article
Front. Cell Dev. Biol. , 13 December 2022
Sec. Cellular Biochemistry
Volume 10 - 2022 | https://doi.org/10.3389/fcell.2022.1081151
This article is part of the Research Topic Cholesterol Regulation in Health and Disease View all 5 articles
Many types of cancer are associated with aberrant cholesterol biosynthesis. There is increasing evidence that dysregulation of cholesterol synthesis within tumor cells promotes a milieu that is rich in cholesterol and is conducive to cellular proliferation, tumor progression and ultimately metastasis. The cholesterol metabolite 27-hydroxycholesterol has been specifically implicated in the progression of breast cancer (Nelson et al., 2013). Studies show that inhibiting the local production of cholesterol can hinder cancer development.
Cholesterol is an essential component of cellular membranes as well as being the precursor of a variety of steroid hormones. Its synthesis occurs through a sequence of complex reactions, beginning with the precursor acetyl-CoA, which is converted to hydroxyl methylglutaryl-CoA (HMG-CoA). The next step in the process, conversion of HMG-CoA to mevalonate, is catalyzed by the enzyme HMG-CoA reductase, which is targeted clinically by a variety of statin inhibitors to lower levels of circulating cholesterol and prevent the associated cardiovascular pathologies. Statins are anti-proliferative and consequently their effects against a variety of cancers have been studied, often with promising results. Commonly used drugs such as atorvastatin and simvastatin have been found to suppress cancers of the breast, lung, and ovary, among others (Barbalata et al., 2020). Statins, however, especially when given at high enough levels to be antitumorigenic, are linked to a number of undesirable side-effects due to their lowering of isoprenoid levels, causing defective posttranslational modification of membrane proteins and impaired membrane structure and function (McTaggart, 2006). Statins are also associated with an increase in type 2 diabetes (Ward et al., 2019). Because of the risks associated with statins, researchers have sought alternative means by which to disrupt cholesterol synthesis, that avoid the detrimental effects of statins.
A few steps downstream from HMG-CoA reductase in the biosynthesis of cholesterol, the enzyme 2,3-oxidosqualene cyclase (OSC) converts 2,3-monoepoxysqualene to lanosterol, from which cholesterol is ultimately derived. OSC inhibition is a potentially fruitful area of research with respect to lowering cholesterol levels, and studies using OSC inhibitors indeed show promise as a means of slowing or reversing a variety of cancers, without the harmful side-effects of statins. One such inhibitor is RO 48-8071 (4’-[6-(allylmethylamino) hexyloxy]-4-bromo-2′-fluorobenzophenone fumarate) (RO). RO has been shown to effectively suppress several different types of cancer, including cancers of the breast, pancreas, prostate, and ovary. Although a variety of different OSC inhibitors have been studied, RO appears to be particularly promising, hence our discussion will concentrate on this particular inhibitor.
RO was developed by Hoffman-La Roche and its effectiveness at lowering plasma cholesterol levels studied in a variety of animal models (Morand et al., 1997). Our interest in the inhibitor occurred serendipitously following a study, using an inverse-docking approach, to identify novel small molecule anti-cancer drugs (Grinter et al., 2011). This led to an investigation at both the cellular level, and in vivo, using mouse xenograft models, into the effects of RO on breast cancer. RO induced apoptosis of tumor vessels in these mouse models, and quashed metastasis. Subsequent studies, using a spontaneous mouse model of pancreatic cancer (RIP-Tag2), and metastatic murine models of human colon carcinoma (HCT116) and pancreatic adenocarcinoma (HPAF-II), showed that RO suppressed angiogenesis, thereby reducing tumor vascularization (Maione et al., 2015).
In studies aimed at developing novel therapeutic techniques to combat hormone-dependent breast cancer, we found that RO decreased the expression of progesterone receptors in BT-474 and T-47D human breast cancer cells, and, furthermore, reduced levels of progestin-induced markers of cancer stem cells (CSCs), such as aldehyde dehydrogenase (ALDH), which are a hallmark of aggressive tumor growth, metastasis, and reappearance of cancer (Liang et al., 2017). RO also reduced expression of estrogen receptor α (ERα), which is known to promote proliferation of hormone-dependent breast cancer cells, while inducing ERβ expression (Liang et al., 2014). This latter observation is particularly exciting because ERβ is known to exert anti-proliferative effects in breast cancer cells. Studies conducted using a nude mouse model of BT-474 cell-derived xenografts grown in an estrogen-rich environment demonstrated that RO suppressed tumor growth in vivo. Immunohistochemical analysis of tumors collected at the end of the study showed that levels of ERα were also reduced in vivo, though in contrast to our observations using cultured BT-474 cells, up-regulation of ERβ was not as obvious. It is likely that this was due to tumors being collected at the experiment’s endpoint, by which time tumor cells expressing high levels of ERβ had already undergone apoptosis. By lowering the cellular ERα/ERβ ratio, RO reduces tumor cell proliferation.
In recent years there has been a search for naturally occurring plant compounds with anti-cancer properties. Our laboratory has studied several such agents and used them in combination with chemotherapeutic drugs that are often highly toxic, in order to develop novel, less toxic treatment methods. Recently, we investigated the combined effects of RO with one such compound, liquiritigenin (LQ), a flavonoid found in licorice that is also an ERβ agonist (Liang et al., 2022). A combination of RO and LQ significantly reduced the viability of cultured MCF-7 and BT-474 breast cancer cells compared with cells treated with either compound alone. Furthermore, growth of BT-474 derived tumor xenografts was inhibited in vivo and although both compounds reduced expression of ERα and markers of angiogenesis, while inducing ERβ expression and increasing apoptosis, a combination of the two enhanced these effects significantly over either agent alone.
A similar picture emerges when the effects of RO on prostate cancer are examined. In the early stages of prostate cancer, prostate cells express androgen receptors (AR) and their growth is hormone-dependent and treatable using anti-androgens. However, despite treatment, drug-resistant tumors often emerge that are classified as castration-resistant, despite AR still being involved in their growth (Shen et al., 2012). We conducted studies to investigate the effects of RO on both types of prostate cancer cells. When hormone-dependent (LNCaP) and castration-resistant (PC-3 and DU145) prostate cancer cells were exposed to RO, their viability was markedly reduced (Liang et al., 2016a). Normal prostate cells, grown in the presence of RO were, however, unaffected, suggesting little or no toxicity to normal cells. RO induced apoptosis in both hormone-dependent and castration-resistant prostate cancer cells and reduced levels of androgen receptors (AR), which are pro-proliferative and vital to the development of both types of prostate cancer cells. RO increased the levels of ERβ in both hormone-dependent LNCaP and castration-resistant PC-3 prostate cancer cells, demonstrating an anti-proliferative effect similar to that observed in breast cancer cells. Incubation of PC-3 cells with diarylpropionitrile (DPN), an ERβ agonist that stimulates ERβ activity, potentiated the effects of RO by reducing cell viability synergistically. The inhibitory effects of RO on prostate cancer in vivo were comparable with those observed in cultured cells. Castration-resistant PC-3 cells were injected into nude mice to create xenograft tumors which were allowed to develop for 6 weeks prior to administration of RO. Compared with control animals that did not receive the inhibitor, tumor growth was markedly suppressed, and, in some animals, tumors were completely eradicated by RO.
Ovarian cancer cells are known to express enzymes that are involved in cholesterol biosynthesis, including OSC (Zhao et al., 2021). Since we and others have shown RO to be effective in reducing the viability of a variety of cancer cells, and suppressing tumor growth, we conducted studies aimed at determining its effectiveness against epithelial ovarian cancer (EOC) (Liang et al., 2016b). OVCAR-3 (high grade serous) and SK-OV-3 (non-high grade serous) cells, exposed to RO in pharmacological and nanomolar levels, showed a significant loss of viability, whereas RO had no effect on normal ovarian cells. Furthermore, RO reduced the acquisition of stem-cell like properties by SK-OV-3 cells, as demonstrated by inhibition of ALDH activity. As in other types of cancer, RO effectively suppressed the growth of subcutaneous tumors derived from SK-OV-3 EOC cells in our well-developed nude mouse xenograft model. However, following an initial response to RO, tumors became resistant and started to grow again, suggesting that combination therapy using a combination of RO and additional chemotherapeutic agents might be necessary to fully control ovarian cancer growth in vivo.
The effects of RO on the viability of the pancreatic ductal adenocarcinoma cell line PANC-1 are in accordance with its effects in other types of cancer. RO was shown to reduce PANC-1 viability in vitro in a time-dependent manner, by inhibiting the cell cycle at the G1S phase (Ding et al., 2021). In studies to ascertain which cell cycle related genes might be affected by RO, it was found that expression of both cyclin B1 and cyclin E were reduced by RO, while p27 expression was increased. RO also affected the phosphorylation levels of ERK and JNK in PANC-1 cells, which suggests it may thereby exert certain anti-tumor effects by regulating apoptosis, angiogenesis, and metastasis. In xenograft studies in nude mice bearing PANC-1 derived tumors, it was shown that RO reduced tumor growth and size compared with controls. Immunohistochemical staining of tumor sections demonstrated lower levels of Ki67 staining in response to RO, confirming inhibition of PANC-1 cell proliferation in vivo.
Although statins are currently the drugs of choice for inhibiting the production of, and lowering circulating levels of cholesterol, to prevent the cardiovascular pathologies associated with high cholesterol, there is evidence in animal models that OSC inhibitors such as RO offer a viable, non-toxic alternative that avoids the negative consequences of statins (Morand et al., 1997). The anti-cancer effects of RO have yet to be fully realized; however, by inhibiting angiogenesis, reducing tumor vascularization, and suppressing metastasis, RO appears to exert its effects consistently against tumors of different origin, suggesting it might be beneficial against a wide variety of cancers. It remains to be investigated whether RO can exert anti-inflammatory effects and/or attract immune cells to destroy tumors. The anti-tumor effects of RO against various tumor types suggest the latter as possible mechanisms for anti-cancer effects of RO. Based on our observations of its potency against breast cancer, we believe that RO could be especially advantageous to postmenopausal women who are at risk for developing the disease, particularly those undergoing combination hormone replacement therapy (HRT) containing both estrogen and progestin, which is associated with an increased risk of breast disease. We propose that clinical studies to assess the effectiveness of RO in a variety of medical settings, including breast, prostate, and ovarian cancer, be initiated, with a view to providing physicians with a new, non-toxic agent with which to combat these diseases.
All authors listed have made a substantial, direct, and intellectual contribution to the work and approved it for publication.
The studies in the author’s laboratory were supported by grants and awards from the NIH, DOD, and the Susan Komen for Cure. Funds provided by the Zalk Missouri Professorship to SMH also supported these studies.
We would like to recognize Yayun Liang, and many graduate and undergraduate students and post-doctoral fellows for their dedication and hard work in successfully carrying out these investigations and want to express our thanks to our various collaborators for their invaluable expertise.
The authors declare that the research was conducted in the absence of any commercial or financial relationships that could be construed as a potential conflict of interest.
All claims expressed in this article are solely those of the authors and do not necessarily represent those of their affiliated organizations, or those of the publisher, the editors and the reviewers. Any product that may be evaluated in this article, or claim that may be made by its manufacturer, is not guaranteed or endorsed by the publisher.
Barbalata, C. I., Tefas, L. R., Achim, M., Tomuta, I., and Porfire, A. S. (2020). Statins in risk-reduction and treatment of cancer. World J. Clin. Oncol. 11 (8), 573–588. doi:10.5306/wjco.v11.i8.573
Ding, Z., Gu, Y., Huang, D., Zhou, H., Zhu, T., Luo, X., et al. (2021). Cholesterol biosynthesis inhibitor RO 48-8071 inhibits pancreatic ductal adenocarcinoma cell viability by deactivating the JNK and ERK/MAPK signaling pathway. Mol. Med. Rep. 24 (828), 828–8. doi:10.3892/mmr.2021.12468
Grinter, S. Z., Liang, Y., Huang, S-Y., Hyder, S. M., and Zou, X. (2011). An inverse docking approach for identifying new potential anti-cancer targets. J. Mol. Graph. Model. 29 (6), 795–799. doi:10.1016/j.jmgm.2011.01.002
Liang, Y., Aebi, J., Nephew, K. P., and Hyder, S. M. (2016b). Targeting cholesterol biosynthesis pathway to inhibit growth of drug resistant ovarian cancer cells. New Orleans, USA: 107th Annual American Association of Cancer Research Meeting. Abstract #3789.
Liang, Y., Besch-Williford, C., Aebi, J. D., Mafuvadze, B., Cook, M. T., Zou, X., et al. (2014). Cholesterol biosynthesis inhibitors as potent novel anti-cancer agents: Suppression of hormone-dependent breast cancer by the oxidosqualene cyclase inhibitor RO 48-8071. Breast cancer Res. Treat. 146, 51–62. doi:10.1007/s10549-014-2996-5
Liang, Y., Besch-Williford, C., and Hyder, S. M. (2022). The estrogen receptor beta agonist liquiritigenin enhances the inhibitory effects of the cholesterol biosynthesis inhibitor RO 48-8071 on hormone-dependent breast cancer growth. Breast Cancer Res. Treat. 192, 53–63. doi:10.1007/s10549-021-06487-y
Liang, Y., Goyette, S., and Hyder, S. M. (2017). Cholesterol biosynthesis inhibitor RO 48-8071 reduces progesterone receptor expression and inhibits progestin-dependent stem cell-like cell growth in hormone-dependent human breast cancer cells. Breast Cancer 9, 487–494. doi:10.2147/BCTT.S140265
Liang, Y., Mafuvadze, B., Aebi, J. D., and Hyder, S. M. (2016a). Cholesterol biosynthesis inhibitor RO 48-8071 suppresses growth of hormone-dependent and castration-resistant prostate cancer cells. Onco. Targets. Ther. 9, 3223–3232. doi:10.2147/OTT.S105725
Maione, F., Oliaro-Bosso, S., Meda, C., Nicolantonio, F. D., Bussolio, F., Balliano, G., et al. (2015). The cholesterol biosynthesis enzyme oxidosqualene cyclase is a new target to impair tumour angiogenesis and metastasis dissemination. Sci. Rep. 5, 90541–90612.
McTaggart, S. J. (2006). Isoprenylated proteins. Cell. Mol. Life Sci. 63, 255–267. doi:10.1007/s00018-005-5298-6
Morand, O. H., Aebi, J. D., Dehmlow, H., Ji, Y-H., Ganus, N., Lengsfeld, H., et al. (1997). Ro 48-8071, a new 2, 3-oxidosqualene:lanosterol cyclase inhibitor lowering plasma cholesterol in hamsters, squirrel monkeys, and minipigs: Comparison to simvastatin. J. Lipid Res. 38, 373–390. doi:10.1016/s0022-2275(20)37449-6
Nelson, E. R., Wardell, S. E., Jasper, J. S., Park, S., Suchindran, S., Howe, M. K., et al. (2013). 27-hydroxycholesterol links hypercholesterolemia and breast cancer pathophysiology. Science 342 (6162), 1094–1098. doi:10.1126/science.1241908
Shen, H. C., Shanmugasundaram, K., Simon, N. I., Cai, C., Wang, H., Chen, S., et al. (2012). In silico discovery of androgen receptor antagonists with activity in castration resistant prostate cancer. Mol. Endocrinol. 26, 1836–1846. doi:10.1210/me.2012-1222
Ward, N. C., Watts, G. F., and Eckel, R. H. (2019). Statin toxicity: Mechanistic insights and clinical implications. Circ. Res. 124, 328–350. doi:10.1161/CIRCRESAHA.118.312782
Keywords: cholesterol biosynthesis, cancer, estrogen receptor alpha, estrogen receptor beta, oxidosqualene cyclase
Citation: SH S and SM H (2022) Should oxidosqualene cyclase in the cholesterol biosynthetic pathway be considered an anti-cancer target?. Front. Cell Dev. Biol. 10:1081151. doi: 10.3389/fcell.2022.1081151
Received: 26 October 2022; Accepted: 30 November 2022;
Published: 13 December 2022.
Edited by:
Marcus Tindall, University of Reading, United KingdomReviewed by:
Elena Ciaglia, University of Salerno, ItalyCopyright © 2022 SH and SM. This is an open-access article distributed under the terms of the Creative Commons Attribution License (CC BY). The use, distribution or reproduction in other forums is permitted, provided the original author(s) and the copyright owner(s) are credited and that the original publication in this journal is cited, in accordance with accepted academic practice. No use, distribution or reproduction is permitted which does not comply with these terms.
*Correspondence: Hyder SM, aHlkZXJzQG1pc3NvdXJpLmVkdQ==
Disclaimer: All claims expressed in this article are solely those of the authors and do not necessarily represent those of their affiliated organizations, or those of the publisher, the editors and the reviewers. Any product that may be evaluated in this article or claim that may be made by its manufacturer is not guaranteed or endorsed by the publisher.
Research integrity at Frontiers
Learn more about the work of our research integrity team to safeguard the quality of each article we publish.