- 1Department of Pediatrics, The Third Xiangya Hospital, Central South University, Changsha, China
- 2Department of Ophthalmology, The Second Xiangya Hospital, Central South University, Changsha, China
- 3Department of General Surgery, Xiangya Hospital, Central South University, Changsha, China
Background: In recent years, there has been an increasing interest in using extracellular vesicles (EVs) as potential therapeutic agents or natural drug delivery systems in kidney-related diseases. However, a detailed and targeted report on the current condition of extracellular vesicle research in kidney-related diseases is lacking. Therefore, this prospective study was designed to investigate the use of bibliometric analysis to comprehensively overview the current state of research and frontier trends on extracellular vesicle research in kidney-related diseases using visualization tools.
Methods: The Web of Science Core Collection (WoSCC) database was searched to identify publications related to extracellular vesicle research in kidney-related diseases since 1999. Citespace, Microsoft Excel 2019, VOSviewer software, the R Bibliometrix Package, and an online platform were used to analyze related research trends to stratify the publication data and collaborations.
Results: From 1 January 1999 to 26 June 2022, a total of 1,122 EV-related articles and reviews were published, and 6,486 authors from 1,432 institutions in 63 countries or regions investigated the role of extracellular vesicles in kidney-related diseases. We found that the number of articles on extracellular vesicles in kidney-related diseases increased every year. Dozens of publications were from China and the United States. China had the most number of related publications, in which the Southeast University (China) was the most active institution in all EV-related fields. Liu Bi-cheng published the most papers on extracellular vesicles, while Clotilde Théry had the most number of co-citations. Most papers were published by The International Journal of Molecular Sciences, while Kidney International was the most co-cited journal for extracellular vesicles. We found that exosome-related keywords included exosome, exosm, expression, extracellular vesicle, microRNA, microvesicle, and liquid biopsy, while disease- and pathological-related keywords included biomarker, microRNA, apoptosis, mechanism, systemic lupus erythematosus, EGFR, acute kidney injury, and chronic kidney disease. Acute kidney disease (AKI), CKD, SLE, exosome, liquid biopsy, and extracellular vesicle were the hotspot in extracellular vesicle and kidney-related diseases research.
Conclusion: The field of extracellular vesicles in kidney-related disease research is rapidly growing, and its domain is likely to expand in the next decade. The findings from this comprehensive analysis of extracellular vesicles in kidney-related disease research could help investigators to set new diagnostic, therapeutic, and prognostic ideas or methods in kidney-related diseases.
Introduction
Kidney-related diseases, i.e., acute or chronic illnesses, have a significant influence on global health as a direct cause of morbidity and mortality (GBD Chronic Kidney Disease Collaboration, 2020; Kellum et al., 2021). To our knowledge, acute kidney disease (AKI) is generally acknowledged as the main risk factor for the incidence and mortality of chronic kidney disease (CKD). Short- and long-term status significantly affects functional conditions and may exacerbate resource utilization (Bellomo et al., 2012; Levey et al., 2015; Kellum et al., 2021). AKI-related mortality by far surpasses the mortality of other diseases, such as diabetes, breast cancer, and heart failure (Lewington et al., 2013; GBD Chronic Kidney Disease Collaboration, 2020; Kellum et al., 2021; Murray et al., 2021). According to the Global Burden of Diseases (GBD) Chronic Kidney Disease Collaboration statistics, ∼1.2 million people died from CKD in 2017, and the global all-age mortality rate from CKD increased by 41.5% between 1990 and 2017. The report from the Chronic Kidney Disease surveillance system in the United States in 2018 suggested that ∼37 million adult Americans, i.e., 15% of the US population, were affected by CKD, and 1 in every 3 US adults could develop CKD (Murray et al., 2021). Moreover, CKD-related cardiovascular disease was reported in 2.6 million deaths and 35.8 million disability-adjusted life-years (DALYs), with most DALYs attributable to CKD occurring in middle and low-middle socio-demographic index (SDI) quintiles. However, since CKD is preventable and treatable, it is worth paying more attention to its global health policy decision-making, especially in locations with low and middle SDI (GBD Chronic Kidney Disease Collaboration, 2020; Vanholder et al., 2022). According to these reports, we found a lack of improvement in the diagnosis and treatment of kidney-related diseases, leading to unmet clinical or medical needs. Therefore, there is an imperative requirement for new perspectives to treat kidney disease and its related complications or other burdens.
Due to the global burden and significant influence of an ever-increasing risk of kidney diseases (Bellomo et al., 2012; GBD Chronic Kidney Disease Collaboration, 2020; Kellum et al., 2021), there has been an increasing need for improving new therapeutics to control these diseases. In a few previous studies about EVs in kidney-related disease (Pisitkun et al., 2004; Zhou et al., 2006a; Bruno et al., 2009; Gonzales et al., 2009; Miranda et al., 2010; van Balkom et al., 2011; Alvarez et al., 2012; Merino et al., 2014; Fais et al., 2016), it was shown that EVs are endogenous or natural membranous nanoparticles that play important roles in essentially all organisms; were used as drug carriers in nanomedicine; and were found to play important roles in the diagnosis, treatment, and prognosis of kidney-related diseases (Kalluri and LeBleu, 2020; Lu and Huang, 2020; Fan et al., 2022; Tang et al., 2022; Yang et al., 2022). Recently, there have been increasing developments on EVs for the detection and treatment of more different types of kidney diseases, including CKD (Sun et al., 2019; Tang et al., 2020a; Grange et al., 2020; Jin et al., 2021; Grange and Bussolati, 2022; Tang et al., 2022; Wang et al., 2022), AKI (Sun et al., 2019; Tang et al., 2020a; Xie et al., 2020; Jin et al., 2021; Kraińska et al., 2021; Grange and Bussolati, 2022; Tang et al., 2022), lupus nephritis (Thongboonkerd, 2019; Garcia-Vives et al., 2020; Xie et al., 2020; van Zonneveld et al., 2021; Grange and Bussolati, 2022), kidney-related inflammation (Sun et al., 2019; Thongboonkerd, 2019; Tang et al., 2020a; Tang et al., 2020b; van Zonneveld et al., 2021), glomerular-related disorders (Thongboonkerd, 2019; Jin et al., 2021; Grange and Bussolati, 2022), transplantation (Thongboonkerd, 2019; Grange and Bussolati, 2022), diabetes (Thongboonkerd, 2019; Grange and Bussolati, 2022; Tang et al., 2022), cancer (Thongboonkerd, 2019; van Zonneveld et al., 2021), fibrosis (Thongboonkerd, 2019; Jin et al., 2020; Tang et al., 2022; Wang et al., 2022), drug resistance (Li et al., 2021; van Zonneveld et al., 2021), toxicity (Jiang et al., 2020), focal segmental glomerulosclerosis (Grange and Bussolati, 2022; Tang et al., 2022), and IgA nephropathy (Grange and Bussolati, 2022). However, despite the increased comprehension of the detection and treatment of EVs in kidney-related diseases (Lewington et al., 2013; Tang et al., 2020a; Jiang et al., 2020; van Zonneveld et al., 2021; Grange and Bussolati, 2022), with the rapid rise in the number of related publications, it is becoming increasingly difficult for scholars to summarize/catch up with the latest discoveries in this field. Thus, in this study, we conducted bibliometrics research on EVs in kidney-related diseases based on existing literature to provide a comprehensive overview of current hotspots for better decision-making in this domain.
Materials and methods
Data sources and search strategies
The Web of Science Core Collection (WoSCC) database was searched to obtain data on EVs in kidney-related diseases over the past 22 years (from 1 January 1999 to 26 June 2022). The search formula was set as [TS = (extracellular vesicle) AND TS = (kidney-related diseases)]. In this context, we selected a dataset derived from WoSCC as our target dataset for analytic purposes. Since the topic search of WoSCC can be interpreted as a model for keyword search based on words in the title, abstract, author keywords, and keyword plus, we chose the search topic to obtain a more precise topic. Moreover, we used MeSH to extract all search samples. A literature review was conducted on the following topics: the whole search formula of [TS = (extracellular vesicle) AND TS = (kidney-related diseases)] was set as [TS = (“extracellular vesicle” OR “vesicle, extracellular” OR “vesicles, extracellular” OR “exovesicles” OR “exovesicle” OR “exosomes” OR “apoptotic bodies” OR “apoptotic body” OR “bodies, apoptotic” OR “body, apoptotic”)] AND [TS = (“chronic kidney failure” OR “chronic renal insufficiency” OR “chronic kidney disease*” OR “renal failure*” OR “kidney failure” OR “renal impairment” OR “kidney impairment” OR “kidney dysfunction” OR “renal dysfunction” OR “reduced renal function” OR “CKD” OR “progressive kidney” OR “glomerular filtration rate” OR “GFR” OR “eGFR” OR proteinuri* OR “albuminuria” OR “microalbuminuria” OR “end-stage renal disease” OR “ESRD” OR “end-stage kidney disease” OR “ESKD” OR “dialysis” OR “renal replacement therapy” OR “kidney transplant” OR “lupus nephritis” OR “acute kidney injuries” OR “acute renal injury” OR “acute renal injuries” OR “acute renal insufficiencies” OR “acute kidney insufficiencies” OR “acute kidney insufficiency” OR “acute renal failure” OR “acute renal failures” OR “AKI” OR “systemic lupus erythematosus” OR “SLE” OR “nephrotic syndrome” OR “NS” OR “glomerulonephritides” OR “iga glomerulonephritis” OR “iga nephropathy” OR “nephritis”)]. The following information was gathered for the inclusion of related studies: the number of publications and citations, titles, publication year, countries, affiliations, authors, journals, keywords, and references of each publication (Figure 1; Supplementary Table S1).
Statistical analysis
For data analysis and visualization, it is necessary to have the appropriate software and online platform. Full records and cited references of all the documents in txt format were downloaded and assembled from WoSCC and imported to CiteSpace 6.1R2, 64 bits basic (Drexel University, Philadelphia, PA, United States), VOSviewer 1.6.17 (Leiden University, Netherlands), Microsoft Excel 2019, the R Bibliometrix Package, and one online platform (https://bibliometric.com) (Figure 1).
CiteSpace, a freely available Java application by Chen (2004), is widely used to analyze and visualize trends and designs in the scientific literature (Synnestvedt et al., 2005), with WoSCC as its main input data source. For this study, we performed clustering, timeline, and reference burst analysis of EVs in kidney-related diseases using CiteSpace.
VOSviewer software (https://www.vosviewer.com/) is an important and powerful tool for building and visualizing bibliometric networks (van Eck and Waltman, 2010). Co-authorship and citation-based and co-occurrence networks could be established based on data downloaded from the Web of Science. In this study, the co-citation networks examined were analyzed to identify countries, institutions, and authors that collaborated and journals that mutually cited one another. Co-occurrence networks were used to investigate keyword occurrences across publications. The size of the nodes represents publication numbers, and the thickness of the connecting lines provides a measurement of each node’s strength. Co-citation and co-occurrence networks were presented in separate parts for the threshold (the minimum number of documents and the maximum link strength) associated with each of these networks. An item’s circle size was proportional to its number of publications, and its line width was proportional to the magnitude of its links. An item’s total link strength reflects how closely the item was associated with others, while the same color indicated close association.
The R language-based Bibliometrix Package (4.1.0 Package), which can show publications of the 10 most productive authors over the past 22 years and identify research topic evolution, was used to analyze country scientific production, three-field plots (co-cited references, authors, and keywords), author production over time, author impact (H-index), and thematic evolution analysis.
In addition to the aforementioned software, assessments on cooperation relationships among countries were also performed on bibliometric analysis software (https://bibliometric.com/).
Results
Analysis of the publication and citation trends
A total of 1,122 studies were obtained based on our search strategy. After excluding 60 articles, comprising meetings (n = 44), letters (n = 2), editorials (n = 11), corrections (n = 2), and duplicated records (n = 1), 1,062 articles in English were found eligible for this present study. We found a significant increase in global publications in the field, from four publications in 1999 to 189 publications in 2021. The specific search formula and sample are shown in Figure 1 and Supplementary Table S1A. Figure 2 and Supplementary Table S1B show that the number of EV-related publications on kidney diseases from 1999 to 2022 showed a rapid year-by-year increasing trend, with a slight decrease in 2015. The most significant increase was observed in the year 2020 and 2021, while the number of literature records steadily increased. The data for 2022 were incomplete at the time of writing this manuscript. The association between the number of publication years and publications was significantly correlated, with an R2 coefficient of 0.8235. Correspondingly, the total number of citations for the regained articles was EVs (n = 44,578). The annual citations displayed a similar upward trend, increasing steadily from two in 1999 to 9,748 in 2021, which showed a sudden and steep rise from 7,229 in 2020 to 9,748 in 2021, with a correlation coefficient R2 = 0.7754.
Source of EV-related publications (active countries, institutions, and journals)
Figure 3 and Tables 1, 2 show that many institutions, countries, and journals contributed to the publications of EVs in kidney-related diseases published worldwide from 1999 to 2022.
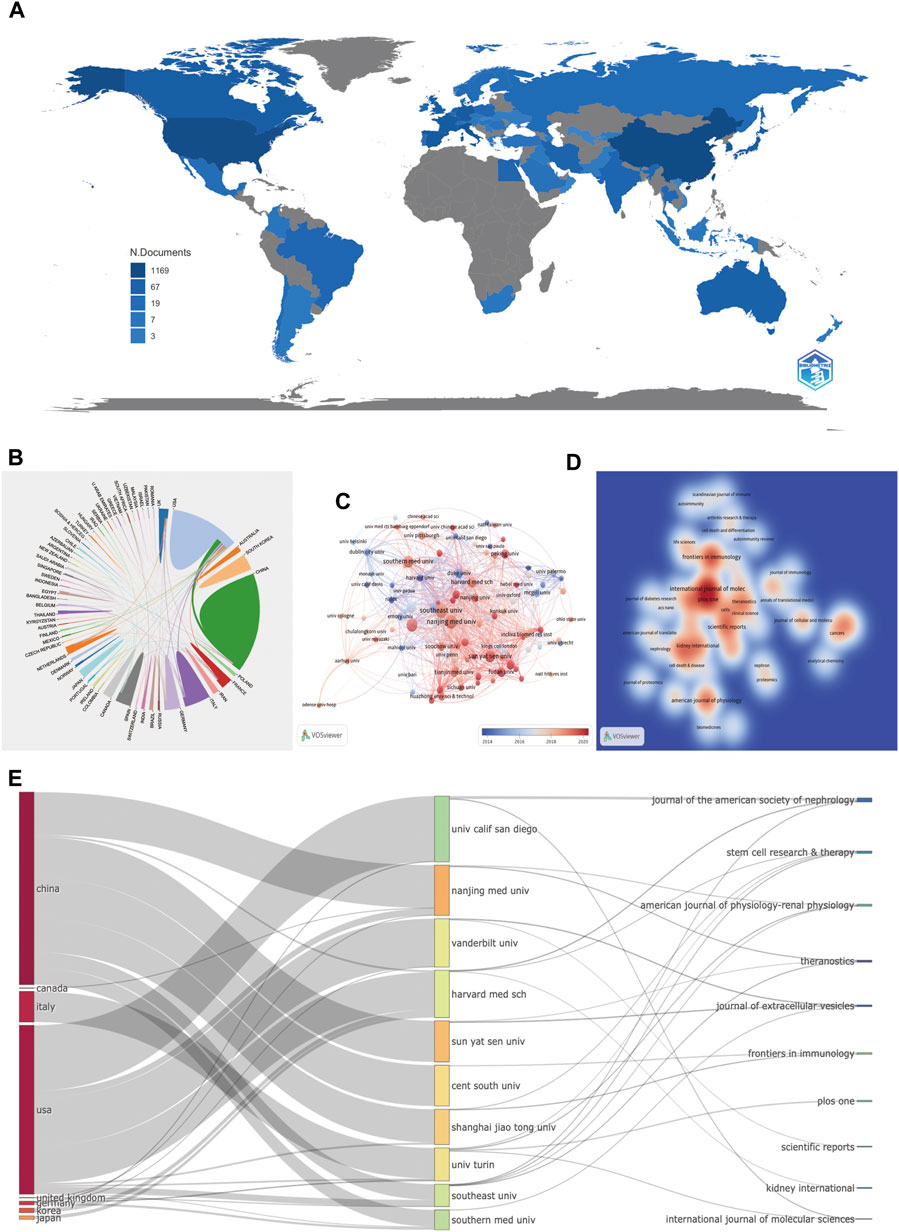
FIGURE 3. Countries, institutions, and journals related to EVs in kidney disease published worldwide. (A) Network map of the country distribution based on R for EVs in kidney disease. (B) Contributed countries cooperating based on an online bibliometric platform for EVs in kidney disease. (C) The chronological order of institutions produced the articles based on VOSviewer for EVs in kidney disease. (D) Journal density map based on VOSviewer for EVs in kidney disease. (E) The relationship of the countries, institutions, and journals produced articles based on an alluvial flow map based on R for EVs in kidney disease.
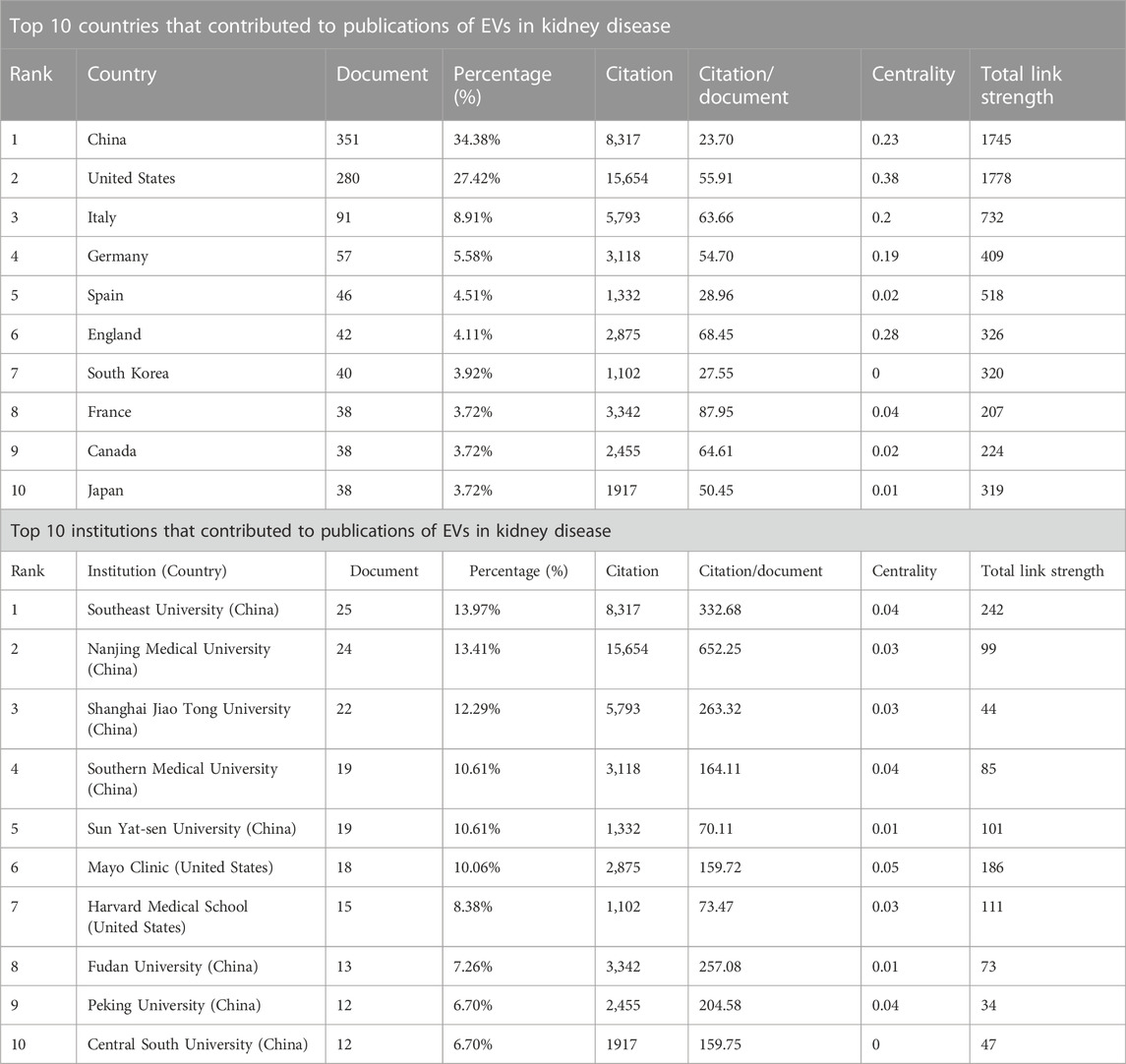
TABLE 1. Top 10 countries and institutions that contributed to publications of EVs in kidney disease.
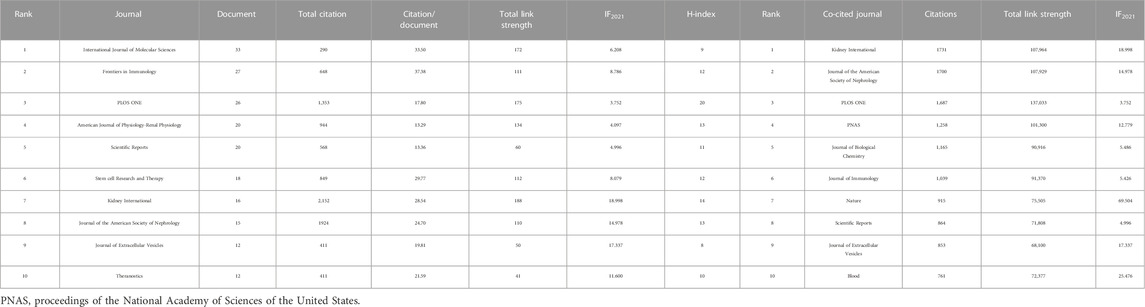
TABLE 2. Top 10 journals and co-cited journals by papers of extracellular vesicle in kidney disease.
Among the 63 countries and regions that contributed to the publications in this field, China had the greatest number of publications (351 articles, 34.38% of all the articles), followed by the United States (280, 27.42%), Italy (91, 8.91%), Germany (57, 5.58%), and Spain (46, 4.51%). Comparatively, the United States had the highest number of citations (15,654 citations), followed by China (8,317 citations), Italy (5,793 citations), France (3,342 citations), and Germany (3,118 citations), with the rest having < 3,000 citations. These findings showed that the United States (280 articles, 15,654 citations, 0.38 centralities, and 1778 total link strength) and China (351 articles, 8,317 citations, 0.23 centralities, and 1,745 total link strength) were the two leading countries in the number of citations and links among other countries (Table 1; Figures 3A, B).
It was reported that 1,423 institutions had published articles on EV use in kidney-related diseases in the past 22 years. Among 1,423 institutions that appeared at least five times, the remaining 107 institutions met the thresholds (Figure 3C). The 10 most productive institutions were almost entirely from China, except Harvard Medical School and Mayo Clinic from the United States. The Southeast University (25 articles) was the leading institution in publication, followed by the Nanjing Medical University (24 articles), Shanghai Jiao Tong University (22 articles), Southern Medical University (19 articles), Sun Yat-sen University (19 articles), and Mayo Clinic (18 articles). The Southeast University also showed the most frequent collaboration with other institutions (total link strength = 242 times), which was followed by the Mayo Clinic (186 times), Harvard Medical School (111 times), and Nanjing Medical University (99 times), suggesting their leading role in EVs in kidney-related diseases (Table 1; Figure 3C).
Among 462 productive journals that appeared at least five times, the remaining 48 productive journals met the thresholds (Figure 3D). The 10 most productive journals and co-cited journals on EVs in kidney diseases are displayed in Table 4, which shows that 20.4% of all publications were published in these journals. The top 5 in producing the number of published journals were the International Journal of Molecular Sciences (33 articles), Frontiers in Immunology (27 articles), PLOS ONE (26 articles), American Journal of Physiology-Renal Physiology (20 articles), and Scientific Reports (20 articles). According to the H-index of the top 10 productive journals, PLOS ONE had the largest number of impact measures (20 H-index), followed by Kidney International (14), American Journal of Physiology-Renal Physiology (13), Journal of the American Society of Nephrology (13), and Frontiers in Immunology (12). Interestingly that among these top H-index above were nephrology-related.
Among 4,358 co-cited journals that appeared at least 20 times, the remaining 531 co-cited journals met the thresholds (Figure 3D). Kidney International (1,731 citations and 107,964 total link strength), Journal of the American Society of Nephrology (1,700 citations and 107,929 total link strength), PLOS ONE (1,687 citations and 137,033 total link strength), PNAS (1,258 citations and 101,300 total link strength), and Journal of Biological Chemistry (1,165 citations and 90,916 total link strength) had the most co-citations with other journals.
The relationship between countries, institutions, and journals based on an alluvial flow map or three-field plot (every ten items were set) for EVs in kidney diseases is shown in Figure 3E. China contained or connected with seven targeted institutions (Nanjing Medical University, Sun Yat-sen University, Shanghai Jiao Tong University, Southern Medical University, Central South University, and Harvard Medical School). The United States contained or connected with eight targeted institutions (University of California San Diego, Vanderbilt University, Harvard Medical School, Nanjing Medical University, Southern Medical University, University of Turin, Shanghai Jiao Tong University, and Southern Medical University). The University of California San Diego connected with two targeted journals (Journal of the American Society of Nephrology and International Journal of Molecular Sciences); Nanjing Medical University connected with two targeted journals (American Journal of Physiology-Renal Physiology and Theranostics); Vanderbilt University connected with two targeted journals (Journal of Extracellular Vesicles and Scientific Reports); Harvard Medical School connected with three targeted journals (Kidney International, Journal of the American Society of Nephrology, and Stem Cell Research and Therapy); Sun Yat-sen University connected with two targeted journals (Journal of Extracellular Vesicles and Theranostics); Central South University connected with one targeted journal (Frontiers in Immunology); Shanghai Jiao Tong University connected with two targeted journals (Stem cell Research and Therapy and Frontiers in Immunology); University of Turin connected with four targeted journals (American Journal of Physiology-Renal Physiology, PLOS ONE, Stem Cell Research and Therapy, and International Journal of Molecular Sciences); Southern Medical University connects with four targeted journals (Theranostics, Stem Cell Research and Therapy, American Journal of Physiology-Renal Physiology, and Journal of the American Society of Nephrology); and Southern Medical University connected with one targeted journal (Journal of Extracellular Vesicles). Interestingly, most of the collaborations within the institutions in China or institutions in the United States took place within their respective institutions.
Analysis of authors, co-authorship, and co-cited references
The top 10 productive and co-cited authors on EVs in kidney diseases are listed in Table 3. Of the total of 6,486 authors, Liu Bi-cheng (15 articles, 745 citations, and 327 total link strength) and Lv Lin-li (13 articles, 678 citations, and 312 total link strength) were the most prolific authors among all 374 publication documents from all 56 productive authors. In contrast, Thery C (745 citations and 1,398 total link strength) and Bruno S (159 citations and 4,001 total link strength) were the most prolific co-cited authors among all 135,413 citations. Moreover, according to the publication timeline for the 10 most active authors shown in Figure 4A, Liu Bi-cheng, Lin-li Lv, Zhang Wei, Camussi Giovanni, Holthofer Harry, and Cortes Raquel had the longest period in publication on EVs in kidney-related diseases. Liu Bi-cheng and Wang Bing had the highest number of impact measures (H-index, 10), followed by Camussi Giovanni (H-index, 9), Lv Lin-li (H-index, 9), and Holthofer Harry (H-index, 9) (Figure 4B). The collaboration network of authors is shown in Figure 4C. Liu Bi-cheng, Wang Bing, Lv Lin-li, Li Zuo-lin, Tang Tao-tao, Feng Ye, Tang Ri-ning, Liu Hong, and Ni Hai-feng were identified as the biggest collaboration network and active teamwork in this field. Cortes Raquel, Forner MJ, Martinez-arroyo Olga, Ortega Ana, Redon Josep, and Perez-Hernandez Javier were the second biggest collaboration network and active teamwork in this field.
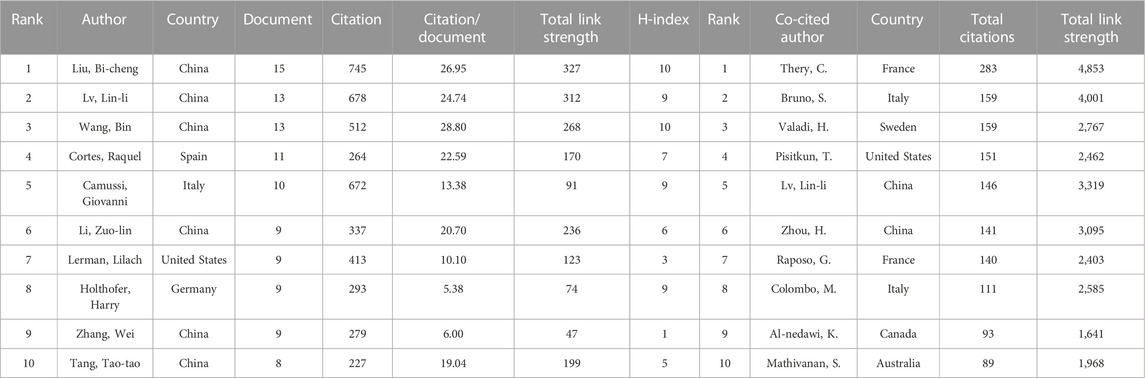
TABLE 3. Top 10 authors and co-cited authors in the field of extracellular vesicle in kidney disease.
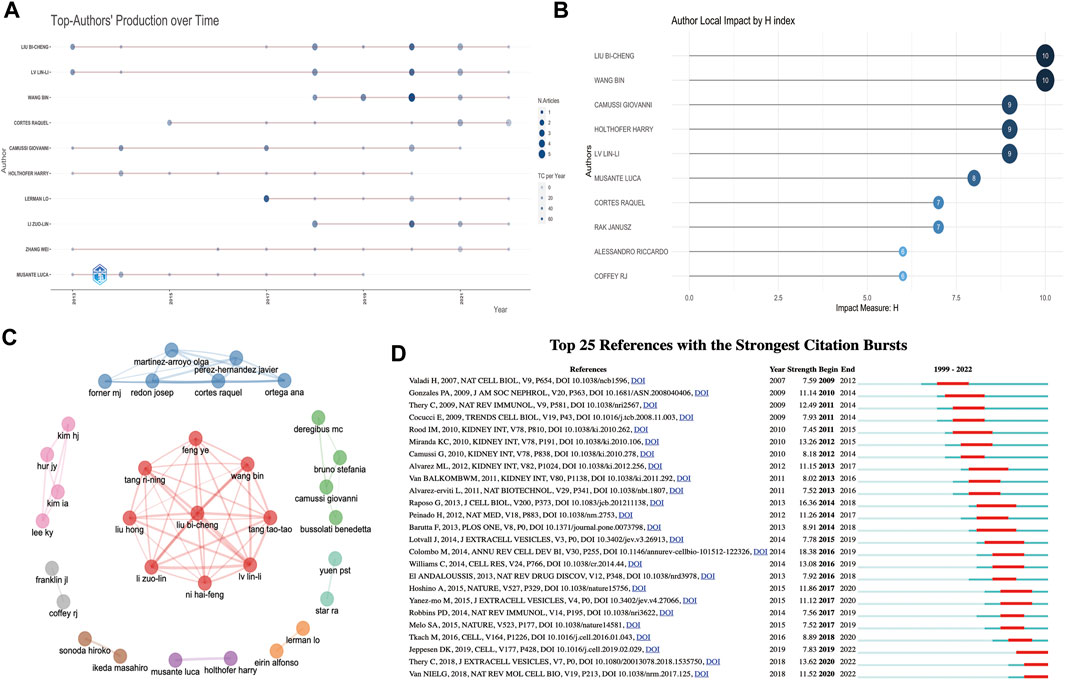
FIGURE 4. Analysis of authors and references involved in EVs in kidney disease. (A) The publication timeline for the 10 most active authors based on R. (B) Author local impact by H-index based on R. (C) Collaboration network of authors based on R. (D) Top 25 references with the strongest citation bursts based on CiteSpace involved in EVs in kidney disease.
The top 10 co-cited references related to EVs in kidney-related diseases are listed in Table 4. Of the 45,959 cited references, 130 co-cited references were cited at least 20 times in EVs (Supplementary Table S2). Valadi et al. (2007) (2007, 158 citations, and 1,273 total link strength), Pisitkun et al. (2004) (2004, 123 citations, and 1,028 total link strength), and Raposo and Stoorvogel (2013) (2013, 97 citations, and 643 total link strength) were the most selected prolific co-cited references among all 45,959 cited references. We also established or extracted highly influential or major achievement articles (Table 5) via co-cited references based on VOSviewer, Citespace, and the R bibliometrics package (Supplementary Tables S2, S4). Interestingly, most of the top 10 co-cited references and the top 8 publications were focused on basic biogenesis, secretion, function, identification, and proteomic profiling of exosomes or EVs. Four publications were reviews, and four others were articles, except two articles reported by Miranda et al. (2010), which concentrated on urinary exosomes as a novel and non-invasive source of nucleic acids for further investigation of renal disease biomarkers to find new potential treatments. In a publication by Bruno et al. (2009), the authors focused on AKI recovery and reported that AKI might be improved using microvesicles derived from mesenchymal stem cells (MSCs) by disrupting the vertical transfer of mRNA from mesenchymal stem cells to surviving tubular cells.
Additionally, “bursts” refer to references appearing over a period of time and reflect the popular topics during that period. The top 25 co-cited references with the strongest citation bursts are shown in Figure 4D. The findings indicated that the first instance of a citation burst occurred in 2009, and the most recent instance of a reference with a citation burst was recorded in 2020. The highest burst strength for EVs in kidney-related diseases was from Colombo et al. (2014) (18.38 strength), Raposo and Stoorvogel (2013) (16.36 strength), and Théry et al. (2018) (13.62 strength). Moreover, Tkach and Théry (2016), Jeppesen et al. (2019), Thery et al. (2018), and van Niel et al. (2018) received more attention in recent years. Interestingly, among the top 25 co-cited references, five co-cited references (20%) were from Kidney International, among which three were in the 2010 year strength (Camussi et al., 2010; Miranda et al., 2010; Rood et al., 2010), one was in 2011 (van Balkom et al., 2011), and one was in 2012 (Alvarez et al., 2012) (Figure 4D).
Analysis of keywords
The early stage (1999–2007) of inquiry on EVs in kidney-related disorders was mostly focused on “autoantibodies,” “biomarkers,” “systemic lupus erythematosus,” and “apoptosis,” as shown by the thematic evolution analysis of the author’s keywords based on publication year. These findings indicate that knowledge of EVs and kidney-related diseases was not well-understood in this stage, except for “systemic lupus erythematosus.” In the platform stage, the critical challenge of kidney-related diseases has steadily developed toward “chronic renal disease” and “kidney,” a breakthrough from 2008 to 2013. This occurred between the years 2008 and 2013. However, between the years 2014 and 2018, a shift was seen toward the concepts of “kidney transplantation,” “liquid biopsy,” “acute kidney injury,” “diabetic nephropathy,” “epigenetics,” and “urinary exosomes.” The terms “liquid biopsy” and “urinary exosomes” have captured the interest of academics throughout the course of the last three years (Figure 5A; Supplementary Table S3).
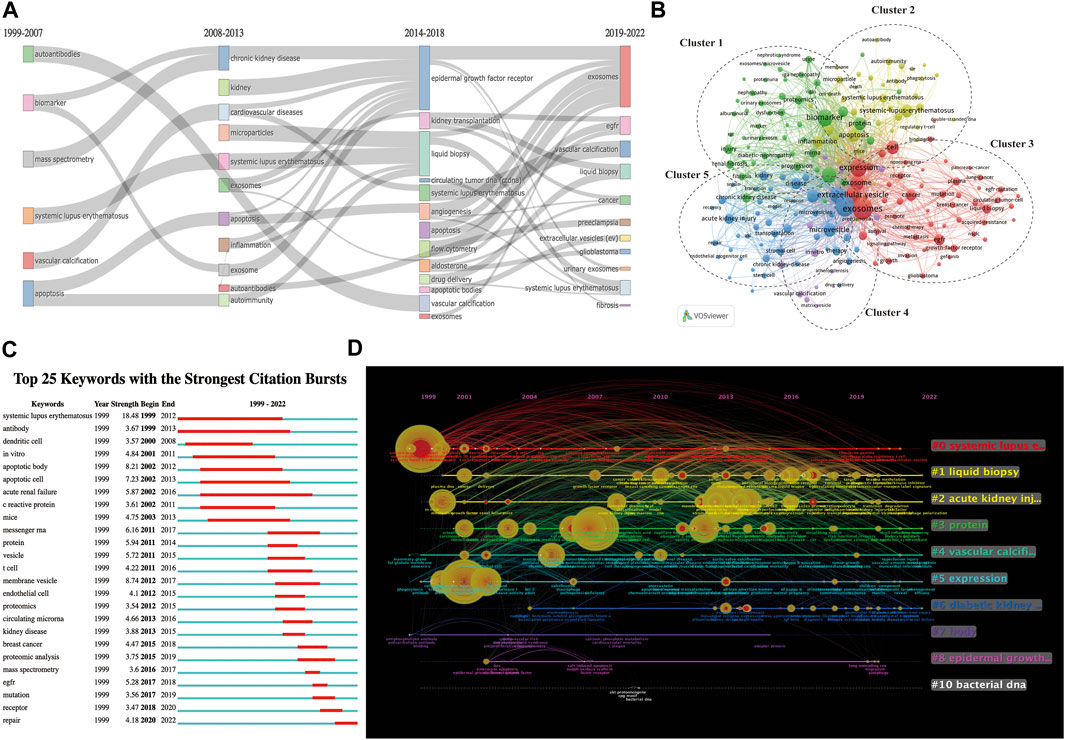
FIGURE 5. Keyword-related mapping in studies on EVs in kidney disease. (A) Major keywords evolution based on R for EVs in kidney disease research. (B) Visualization based on keyword co-occurrence relationship based on VOSviewer for EVs in kidney disease. In this network map, keywords with close relationships are assigned to one cluster with the same color. All the keywords could be divided into five clusters: cluster 1 (green nodes), cluster 2 (yellow nodes), cluster 3 (red nodes), cluster 4 (purple nodes), and cluster 5 (blue nodes). (C) Top 25 keywords with the strongest bursts by CiteSpace. (D) The timeline view of keywords based on CiteSpace related to EVs in kidney disease.
The top 25 co-occurrence keywords related to EVs in kidney-related diseases are shown in Table 6, suggesting that exosomes were the most frequent keywords (264 occurrences and 622 total link strength), followed by exosm (253 occurrences and 696 total link strength) and expression (193 occurrences and 530 total link strength). Among the top 25 keywords, it was seen that biomarker (167 occurrences and 501 total link strength), microRNA (138 occurrences and 415 total link strength), systemic lupus erythematosus (92 occurrences and 140 total link strength), EGFR (72 occurrences and 190 total link strength), acute kidney injury (62 occurrences and 171 total link strength), kidney (54 occurrences and 168 total link strength), liquid biopsy (53 occurrences and 139 total link strength), and chronic kidney disease (53 occurrences and 141 total link strength) were the most mentioned related to kidney diseases.
A total of 4,520 keywords were obtained, among which 424 keywords appeared at least 10 times (Figure 5B). As shown in Figure 5B, all of the keywords could be divided into the following five groups: cluster 1 (green nodes focus on the use of EVs as biomarkers for potential treatments or clinical applications in kidney-related diseases, including diabetic nephropathy, IgA nephropathy, nephrotic syndrome, and renal fibrosis), cluster 2 (yellow nodes focus on the use or functions of EVs as potential treatments of kidney-related diseases, including systemic lupus erythematosus and lupus nephritis), cluster 3 (red nodes focus on the mechanism of EVs in others diseases, including lung cancer and pancreatic cancer), cluster 4 (purple nodes focus on EV-related material, including drug delivery and serum), and cluster 5 (blue nodes focus on the mechanism or clinical applications from EVs in kidney-related diseases, including CKD and AKI).
The top 25 co-cited keywords with the strongest citation bursts based on Citespace are shown in Figure 5C. The highest burst strength relevant to kidney-related diseases was from systemic lupus erythematosus (1999), acute renal failure (2002), and kidney diseases (2013). Moreover, EGFR (2017), mutation (2017), receptor (2018), and repair (2020) received more attention in recent years (Figure 5C).
We applied the CiteSpace software to cluster the keywords and references to illustrate a timeline for keywords after clustering (Figure 5D; Supplementary Table S4). A total 10 clusters were formed: 1) systemic lupus erythematosus, 2) liquid biopsy, 3) acute kidney injury, 4) protein, 5) vascular calcification, 6) expression, 7) diabetic kidney disease, 8) body, 9) epidermal growth factor, and 10) bacterial DNA. According to the timeline view (Figure 5D), we found that the 1) systemic lupus erythematosus, 2) liquid biopsy, 3) acute kidney injury, 4) protein, 5) vascular calcification, 6) expression, and 7) diabetic kidney disease appeared to be significant and proceeded to develop between EVs and kidney diseases. 1) systemic lupus erythematosus, 2) liquid biopsy, 3) acute kidney injury, and 4) protein had the most prolonged period of attention and effects on EVs in kidney-related diseases.
Notably, we discovered numerous potentially unique characteristics of EVs in kidney-related diseases. Notably, we identified important keywords from relationships between the top 20 co-cited references, authors, and keywords evolution of EVs in kidney-related diseases (Figure 6), including “systemic lupus erythematosus,” “kidney,” “acute kidney injury,” “chronic kidney disease,” and “diabetic nephropathy.” They were almost connected with the top 10 authors and co-cited references (Tables 3, 4).
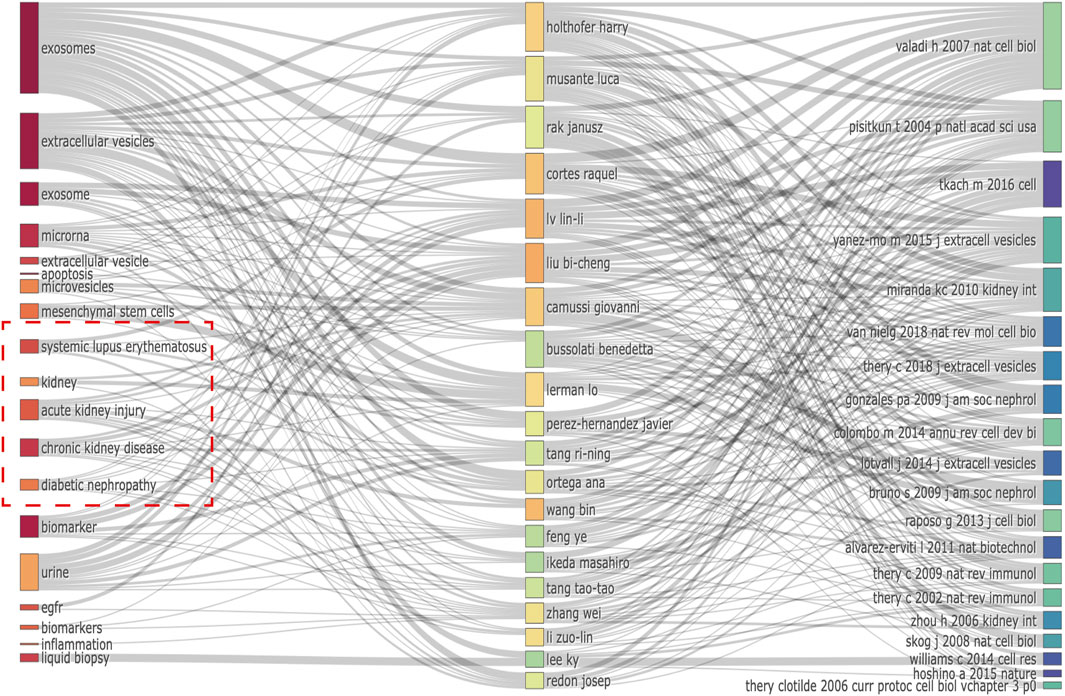
FIGURE 6. Relationship of the top 20 co-cited references, authors, and keywords evolution based on an alluvial flow map by R.
Discussion
Based on visual management software, we conducted a comprehensive bibliometric analysis of literature published on EVs and kidney-related diseases from the inception to 26 June 2020, most of which were published after 1999. A total of 1,122 EVs and kidney-related disease publications were obtained from the WoSCC. The number of EVs in kidney disease studies started in 2019 was >100. The interesting integrated evolution in the year 2019 demonstrated the forcefully increasing attention in the international arena on EVs for dealing with kidney-related diseases. A few recent bibliometric studies of EVs regarding improving the field of neuroscience (Long et al., 2022), mesenchymal stem cell-derived extracellular vesicles (MSC-EVs) (Zhang et al., 2022), liver diseases (Shao et al., 2022), and breast cancer (Teles et al., 2021) found a major evolution trend in annual publications around 2012–2016, suggesting that there have been rapid developments on EVs in the last decade.
According to the results in Table 1 and Figures 3A, B, China and the United States were the top 2 countries in publishing literature and linking to other countries. Comparatively, China was the top country in publishing literature and an active country in recent years. Conversely, the United States was the top country in the number of citations and total link strengths in this field. Moreover, China’s centrality and total link strengths were 0.23 and 1,745, while that of the United States were 0.38 and 1,778, respectively. In addition, of the top 10 institutions with the most studied items, eight were from China and two were from the United States. However, the top centrality and total link strength of Chinese institutions were 0.04 and 242 from the Southeast University, respectively, while those of the United States institutions were 0.05 and 186 from the Mayo Clinic, respectively. Therefore, the United States, China, and their corresponding institutions retained the leading status and engagement on EVs in kidney-related disease research, followed by Italy, Germany, Spain, and England, which had high centrality and total link strength, implying that they played important roles in the worldwide collaboration of EVs in kidney-related diseases research. Overall, the publication and citation number outcomes demonstrated that studies related to EVs in kidney-related diseases are still in a high-speed evolution stage, causing significant interest for future research.
Based on the number of citations and articles, total link strength, and H-index, PLOS ONE, Kidney International, American Journal of Physiology-Renal Physiology, Journal of the American Society of Nephrology, Frontiers in Immunology, Stem Cell Research and Therapy, International Journal of Molecular Sciences, and Theranostics were the most influential journals for researchers in EV-related kidney diseases. The most co-cited journals were also from Kidney International, the Journal of the American Society of Nephrology, and PLOS ONE (Table 2). Overall, these results also insinuate that journals in nephrology and on EVs might have a certain influence over a central role and balance on the publication of this study, suggesting the hotness in publishing high-impact papers in this field (Table 2).
Our results on authors’ analysis (Table 3; Figures 4A–C) showed that Liu Bi-cheng (corresponding author) from the Institute of Nephrology, Southeast University School of Medicine, was the most prolific author. He mainly focused on the association of EVs with kidney-related diseases (AKI, CKD, renal inflammation and fibrosis, diabetic nephropathy, etc.). Moreover, he and his colleagues (Lv Lin-li, top 2; Wang Bin, top 3; Tang Tao-tao, top 10; Li Zuo-lin, top 9) also contributed to the correlation between EV and kidney-related disease research (Lv et al., 2018a; Tang et al., 2019a; Wang et al., 2019a; Tang et al., 2019b; Lv et al., 2019; Tang and Liu, 2019; Tang et al., 2020a; Tang et al., 2020c; Wang et al., 2020; Cao et al., 2021a; Feng et al., 2021a; Feng et al., 2021b; Tang et al., 2021; Ding et al., 2022; Tang et al., 2022). Interestingly, these researchers not only had the biggest collaboration network and active teamwork in this field but also the longest period in publication and the highest number of impact measures. Camussi Giovanni (corresponding author) from the Department of Medical Sciences, University of Turin, was the top 5 prolific author for EVs in kidney disease. He mainly focused on the association of EVs with kidney-related diseases (AKI, CKD, systemic lupus erythematosus, ANCA-vasculitis, kidney fibrosis, and diabetic nephropathy) (Biancone and Camussi, 2014; Collino et al., 2015; Lener et al., 2015; Bussolati and Camussi, 2017; Dimuccio et al., 2020; Grange et al., 2020; Kholia et al., 2020; Ramírez-Bajo et al., 2020; Tetta et al., 2020; Kholia et al., 2021; Mazzariol et al., 2021; Bruno et al., 2022; Franzin et al., 2022). Additionally, Cortes Raquel (corresponding author) from the Cardiometabolic and Renal Risk Research Group, INCLIVA Biomedical Research Institute, was the top 4 prolific author for EVs in kidney-related diseases. Notably, he and his team mainly focused on the association of EVs with kidney-related diseases (CKD, kidney-related cardiovascular risk, hypertension, diabetes mellitus, lupus nephritis, and systemic lupus erythematosus) (Perez-Hernandez and Cortes, 2015; Perez-Hernandez et al., 2015; Perez-Hernandez et al., 2016; Perez-Hernandez et al., 2017; Perez-Hernandez et al., 2018a; Perez-Hernandez et al., 2018b; Olivares et al., 2018; Olivares et al., 2020; Ortega et al., 2020; Perez-Hernandez et al., 2021a; Perez-Hernandez et al., 2021b; Redon et al., 2021; Martinez-Arroyo et al., 2022). Overall, research on EVs in kidney-related diseases showed a good link for co-partnership among authors. Furthermore, most scholars were from different countries, and the cooperation was mostly confined to the research team. Researchers from different countries should reinforce collaboration and partake in beneficial terraces to achieve more significant progress and improve the clinical translation of research and the exchange of technological innovation among scientists working on different aspects between EVs and kidney-related diseases.
As shown in Table 4, of the 45,959 cited references, 130 co-cited references were cited at least 20 times in EVs (Table 4; Supplementary Table S2). We found that the co-cited references reported by Valadi et al. (2007) from Nature Cell Biology (IF = 28.213) titled “Exosome-mediated transfer of mRNAs and microRNAs are a novel mechanism of genetic exchange between cells” was the top in the number of citations and total link strengths in EVs in kidney-related diseases. All of them mainly focused on the significant use, formation, targeting, and function of EVs in kidney-related diseases, which included cancer and other diseases (Théry et al., 2002; Pisitkun et al., 2004; Théry et al., 2006; Valadi et al., 2007; Bruno et al., 2009; Théry et al., 2009; Miranda et al., 2010; Colombo et al., 2014; Yáñez-Mó et al., 2015). Additionally, Figure 4D and Table 5 results indicate that the most influential papers were published in basic and clinical journals. As a relatively rapid translation of basic research to clinical research on EVs and kidney-related diseases, the research on these topics forms a good connection between basic and clinical studies, suggesting a favorable development pattern on EVs in kidney disease research.
Keywords are the investigation focus and core objects of a study. Moreover the analysis of keywords suggested that the significant benefit of EVs in kidney-related diseases was research hotspots and keyword co-occurrence analysis. According to the keywords reported, the major keywords included evolution, network map, and top 25 co-occurrence keywords of EVs in kidney disease (Figures 5A, B; Table 6). The top 25 keywords with the strongest bursts and the timeline view of keywords related to EVs in kidney disease are shown in Figures 5C, D. It is possible to realize the allocation and growth of distinct research hotspots in this field. Subsequently, based on the results in the timeline viewer analysis of clustering, we defined the research hotspots and growth frontiers in the field of EVs in kidney-related diseases.
All 13 largest clusters are the results conducted by Citespace and those results were input intoSupplementary Table S4. This review described that urinary extracellular vesicles might have potential pathophysiologic, diagnostic, and therapeutic roles in renal diseases (Erdbrügger and Le, 2016). Cluster #1 was “acute kidney injury, extracellular vesicle, and stem cell-derived”. A review by Jin et al. (2021) entitled “Exosomes: Emerging therapy delivery tools and biomarkers for kidney diseases” mainly focused on the application of nanometer-sized small EVs coated with bilayer structure in diagnosis and their positive effects on the repair of kidney dysfunction and the designated mechanisms. Cluster #6 was “mesenchymal stem, extracellular vesicle, and stem cell-derived” and showed that mesenchymal stem cells-derived EVs could be a promising concept for the repair of damaged kidneys (Nargesi et al., 2017). Cluster #7 was “systemic lupus erythematosus and exosome-based drug delivery system.” This review focused on the crucial traits of nanometer-sized lipid-bilayer-enclosed EVs or exosome-based drug delivery systems in systemic lupus erythematosus (SLE) (Ortega et al., 2020). Cluster #13 was “human islet, extracellular vesicle, and extracellular vesicle.” This review focused on therapeutic options for autoimmune diseases (rheumatoid arthritis, autoimmune type 1 diabetes mellitus, and systemic lupus erythematosus) based on MSCs and MSC-EVs (Martinez-Arroyo et al., 2022). Cluster #18 was “novel biomarker source, peritoneal dialysis, and extracellular vesicle.” The review reported by Brahmadhi et al. entitled “Exosomal proteomics in kidney disease: From technical approaches to clinical applications” explains the proteomics-based studies on exosomes and their clinical applications in kidney-related diseases (AKI, CKD, renal transplantation, congenital kidney disease, and malignant kidney disorder) (Brahmadhi et al., 2022). Cluster #34 was “human islet and extracellular vesicle.” This article used the potential of human islet-derived EVs in modulating T- and B-cell response activation by EVs in the peripheral blood mononuclear cells (PBMCs) of patients with type 1 diabetes (T1D) (Rutman et al., 2018) (Supplementary Table S4).
Limitations
Although our study used a comprehensive retrieval strategy to search for data from WoSCC, several limitations should be considered. First, despite conducting the search strategy to the greatest extent by separating “EV-related kidney diseases,” all search samples were extracted by MeSH as search terms. However, fewer studies about EV-related cancer were included in the analysis, which might have caused some inconsistencies in our results. Second, machine algorithms (VOSviewer, CiteSpace, the R Bibliometrix Package, and Excel) were used, and most results might have a few deviations. Lastly, some recent power-published and potentially high-impact studies might not have been included in our research because of the low citation frequency. As a result, more bibliometric data updates would be required to further clarify the scientific trends and hotspots in EV-related kidney disease research.
Conclusion
To the best of our knowledge, our research is the first to provide a recent assessment of the emerging global trends on EVs in research on kidney-related diseases using a bibliometric approach. Research on EVs in kidney-related diseases is rapidly growing and likely to increase further in the next decade. In conclusion, this study disseminates a comprehensive analysis of EVs in research on kidney-related diseases, providing important information for investigators to formulate new diagnostic, therapeutic, and prognostic ideas or methods in kidney-related diseases.
Data availability statement
The datasets presented in this study can be found in online repositories. The names of the repository/repositories and accession number(s) can be found in the article/Supplementary Material.
Ethics statement
Ethical review and approval was not required for the study on human participants in accordance with the local legislation and institutional requirements. Written informed consent to participate in this study was provided by the participants’ legal guardian/next of kin. Written informed consent was obtained from the individual(s) and minor(s)’ legal guardian/next of kin for the publication of any potentially identifiable images or data included in this article.
Author contributions
MH had the idea for the study. HW, MH, and TV selected studies for inclusion and abstracted data. MH and PH did the statistical analyses. MH and HW interpreted the data. MH wrote the first draft. MZ and QH critically revised the paper for important intellectual content. All authors have read and approved the content of the manuscript.
Funding
This research was funded by Hunan innovative province construction project (Grant No. 2019SK2211), Key research and development project of Hunan Province (Grant No. 2020SK2089), The Natural Science Foundation of Hunan province (Grant Nos 2020JJ4833, 2019SK2211, and XY040019), Hunan Province Key Field R&D Program (Grant No. 2020SK2097), and Horizontal Project (Grant Nos KY080269, KY080262, XY080323, and XY080324).
Conflict of interest
The authors declare that the research was conducted in the absence of any commercial or financial relationships that could be construed as a potential conflict of interest.
Publisher’s note
All claims expressed in this article are solely those of the authors and do not necessarily represent those of their affiliated organizations, or those of the publisher, the editors and the reviewers. Any product that may be evaluated in this article, or claim that may be made by its manufacturer, is not guaranteed or endorsed by the publisher.
Supplementary material
The Supplementary Material for this article can be found online at: https://www.frontiersin.org/articles/10.3389/fcell.2022.1070516/full#supplementary-material
References
Alasmari, W. A., Abdelfattah-Hassan, A., El-Ghazali, H. M., Abdo, S. A., Ibrahim, D., ElSawy, N. A., et al. (2022). Exosomes derived from BM-MSCs mitigate the development of chronic kidney damage post-menopause via interfering with fibrosis and apoptosis. Biomolecules 12 (5), 663. doi:10.3390/biom12050663
Alvarez, M., Khosroheidari, M., Kanchi Ravi, R., and DiStefano, J. (2012). Comparison of protein, microRNA, and mRNA yields using different methods of urinary exosome isolation for the discovery of kidney disease biomarkers. Kidney Int. 82 (9), 1024–1032. doi:10.1038/ki.2012.256
Barutta, F., Tricarico, M., Corbelli, A., Annaratone, L., Pinach, S., Grimaldi, S., et al. (2013). Urinary exosomal microRNAs in incipient diabetic nephropathy. PLoS One 8 (11), e73798. doi:10.1371/journal.pone.0073798
Bellomo, R., Kellum, J., and Ronco, C. (2012). Acute kidney injury. Lancet 380 (9843), 756–766. doi:10.1016/s0140-6736(11)61454-2
Biancone, L., and Camussi, G. (2014). Stem cells in 2013: Potential use of stem or progenitor cells for kidney regeneration. Nat. Rev. Nephrol. 10 (2), 67–68. doi:10.1038/nrneph.2013.257
Brahmadhi, A., Chuang, Y. K., Wang, S. Y., Kao, C. C., and Tsai, I. L. (2022). Exosomal proteomics in kidney disease: From technical approaches to clinical applications. J. Food Drug Analysis 30 (2), 202–222. doi:10.38212/2224-6614.3409
Bruno, S., Chiabotto, G., Cedrino, M., Ceccotti, E., Pasquino, C., De Rosa, S., et al. (2022). Extracellular vesicles derived from human liver stem cells attenuate chronic kidney disease development in an in vivo experimental model of renal ischemia and reperfusion injury. Int. J. Mol. Sci. 23 (3), 1485. doi:10.3390/ijms23031485
Bruno, S., Grange, C., Collino, F., Deregibus, M. C., Cantaluppi, V., Biancone, L., et al. (2012). Microvesicles derived from mesenchymal stem cells enhance survival in a lethal model of acute kidney injury. PLoS One 7 (3), e33115. doi:10.1371/journal.pone.0033115
Bruno, S., Grange, C., Deregibus, M., Calogero, R., Saviozzi, S., Collino, F., et al. (2009). Mesenchymal stem cell-derived microvesicles protect against acute tubular injury. J. Am. Soc. Nephrol. JASN 20 (5), 1053–1067. doi:10.1681/asn.2008070798
Bussolati, B., and Camussi, G. (2017). Renal injury: Early apoptotic extracellular vesicles in injury and repair. Nat. Rev. Nephrol. 13 (9), 523–524. doi:10.1038/nrneph.2017.117
Camussi, G., Deregibus, M., Bruno, S., Cantaluppi, V., and Biancone, L. (2010). Exosomes/microvesicles as a mechanism of cell-to-cell communication. Kidney Int. 78 (9), 838–848. doi:10.1038/ki.2010.278
Cao, H., Cheng, Y., Gao, H., Zhuang, J., Zhang, W., Bian, Q., et al. (2020). In vivo tracking of mesenchymal stem cell-derived extracellular vesicles improving mitochondrial function in renal ischemia-reperfusion injury. ACS Nano 14 (4), 4014–4026. doi:10.1021/acsnano.9b08207
Cao, J., Wang, B., Tang, T., Lv, L., Ding, Z., Li, Z., et al. (2020). Three-dimensional culture of MSCs produces exosomes with improved yield and enhanced therapeutic efficacy for cisplatin-induced acute kidney injury. Stem cell Res. Ther. 11 (1), 206. doi:10.1186/s13287-020-01719-2
Cao, J., Wang, B., Tang, T., Wen, Y., Li, Z., Feng, S., et al. (2021). Exosomal miR-125b-5p deriving from mesenchymal stem cells promotes tubular repair by suppression of p53 in ischemic acute kidney injury. Theranostics 11 (11), 5248–5266. doi:10.7150/thno.54550
Cao, J. Y., Wang, B., Tang, T. T., Wen, Y., Li, Z. L., Feng, S. T., et al. (2021). Exosomal miR-125b-5p deriving from mesenchymal stem cells promotes tubular repair by suppression of p53 in ischemic acute kidney injury. Theranostics 11 (11), 5248–5266. doi:10.7150/thno.54550
Chen, C. (2004). Searching for intellectual turning points: Progressive knowledge domain visualization. Proc. Natl. Acad. Sci. U. S. A. 101 (1), 5303–5310. doi:10.1073/pnas.0307513100
Chen, L., Wang, Y., Li, S., Zuo, B., Zhang, X., Wang, F., et al. (2020). Exosomes derived from GDNF-modified human adipose mesenchymal stem cells ameliorate peritubular capillary loss in tubulointerstitial fibrosis by activating the SIRT1/eNOS signaling pathway. Theranostics 10 (20), 9425–9442. doi:10.7150/thno.43315
Cheng, L., Sun, X., Scicluna, B. J., Coleman, B. M., and Hill, A. F. (2014). Characterization and deep sequencing analysis of exosomal and non-exosomal miRNA in human urine. Kidney Int. 86 (2), 433–444. doi:10.1038/ki.2013.502
Collino, F., Bruno, S., Incarnato, D., Dettori, D., Neri, F., Provero, P., et al. (2015). AKI recovery induced by mesenchymal stromal cell-derived extracellular vesicles carrying MicroRNAs. J. Am. Soc. Nephrol. JASN 26 (10), 2349–2360. doi:10.1681/asn.2014070710
Colombo, M., Raposo, G., and Théry, C. (2014). Biogenesis, secretion, and intercellular interactions of exosomes and other extracellular vesicles. Annu. Rev. Cell Dev. Biol. 30, 255–289. doi:10.1146/annurev-cellbio-101512-122326
Delić, D., Eisele, C., Schmid, R., Baum, P., Wiech, F., Gerl, M., et al. (2016). Urinary exosomal miRNA signature in type II diabetic nephropathy patients. PLoS One 11 (3), e0150154. doi:10.1371/journal.pone.0150154
Dimuccio, V., Peruzzi, L., Brizzi, M., Cocchi, E., Fop, F., Boido, A., et al. (2020). Acute and chronic glomerular damage is associated with reduced CD133 expression in urinary extracellular vesicles. Am. J. physiology. Ren. physiology 318 (2), F486–F95. doi:10.1152/ajprenal.00404.2019
Ding, Z., Tang, T., Li, Z., Cao, J., Lv, L., Wen, Y., et al. (2022). Therapeutic effect of extracellular vesicles derived from HIF prolyl hydroxylase domain enzyme inhibitor-treated cells on renal ischemia/reperfusion injury. Kidney Dis. (Basel, Switz. 8 (3), 206–216. doi:10.1159/000522584
Dominguez, J., Liu, Y., Gao, H., Dominguez, J., Xie, D., and Kelly, K. (2017). Renal tubular cell-derived extracellular vesicles accelerate the recovery of established renal ischemia reperfusion injury. J. Am. Soc. Nephrol. JASN 28 (12), 3533–3544. doi:10.1681/asn.2016121278
Ebrahim, N., Ahmed, I., Hussien, N., Dessouky, A., Farid, A., Elshazly, A., et al. (2018). Mesenchymal stem cell-derived exosomes ameliorated diabetic nephropathy by autophagy induction through the mTOR signaling pathway. Cells 7 (12), 226. doi:10.3390/cells7120226
Eirin, A., Zhu, X., Puranik, A., Tang, H., McGurren, K., van Wijnen, A., et al. (2017). Mesenchymal stem cell-derived extracellular vesicles attenuate kidney inflammation. Kidney Int. 92 (1), 114–124. doi:10.1016/j.kint.2016.12.023
Erdbrügger, U., and Le, T. H. (2016). Extracellular vesicles in renal diseases: More than novel biomarkers? J. Am. Soc. Nephrol. 27 (1), 12–26. doi:10.1681/asn.2015010074
Fais, S., O'Driscoll, L., Borras, F., Buzas, E., Camussi, G., Cappello, F., et al. (2016). Evidence-based clinical use of nanoscale extracellular vesicles in nanomedicine. ACS Nano 10 (4), 3886–3899. doi:10.1021/acsnano.5b08015
Fan, Z., Jiang, C., Wang, Y., Wang, K., Marsh, J., Zhang, D., et al. (2022). Engineered extracellular vesicles as intelligent nanosystems for next-generation nanomedicine. Nanoscale horizons 7 (7), 682–714. doi:10.1039/d2nh00070a
Feng, S., Gao, Y., Yin, D., Lv, L., Wen, Y., Li, Z., et al. (2021). Identification of lumican and fibromodulin as hub genes associated with accumulation of extracellular matrix in diabetic nephropathy. Kidney & blood Press. Res. 46 (3), 275–285. doi:10.1159/000514013
Feng, Y., Zhong, X., Ni, H. F., Wang, C., Tang, T. T., Wang, L. T., et al. (2021). Urinary small extracellular vesicles derived CCL21 mRNA as biomarker linked with pathogenesis for diabetic nephropathy. J. Transl. Med. 19 (1), 355. doi:10.1186/s12967-021-03030-x
Franzin, R., Stasi, A., Sallustio, F., Bruno, S., Merlotti, G., Quaglia, M., et al. (2022). Extracellular vesicles derived from patients with antibody-mediated rejection induce tubular senescence and endothelial to mesenchymal transition in renal cells. Am. J. Transplant. doi:10.1111/ajt.17097
Garcia-Vives, E., Solé, C., Moliné, T., Vidal, M., Agraz, I., Ordi-Ros, J., et al. (2020). The urinary exosomal miRNA expression profile is predictive of clinical response in lupus nephritis. Int. J. Mol. Sci. 21 (4), 1372. doi:10.3390/ijms21041372
Gatti, S., Bruno, S., Deregibus, M. C., Sordi, A., Cantaluppi, V., Tetta, C., et al. (2011). Microvesicles derived from human adult mesenchymal stem cells protect against ischaemia-reperfusion-induced acute and chronic kidney injury. Nephrol. Dial. Transplant. 26 (5), 1474–1483. doi:10.1093/ndt/gfr015
GBD Chronic Kidney Disease Collaboration (2020). Global, regional, and national burden of chronic kidney disease 1990-2017a systematic analysis for the Global Burden of Disease Study 2017. Lancet 395 (10225), 709–733. doi:10.1016/s0140-6736(20)30045-3
Gonzales, P., Pisitkun, T., Hoffert, J., Tchapyjnikov, D., Star, R., Kleta, R., et al. (2009). Large-scale proteomics and phosphoproteomics of urinary exosomes. J. Am. Soc. Nephrol. JASN 20 (2), 363–379. doi:10.1681/asn.2008040406
Grange, C., and Bussolati, B. (2022). Extracellular vesicles in kidney disease. Nat. Rev. Nephrol. 18, 499–513. doi:10.1038/s41581-022-00586-9
Grange, C., Papadimitriou, E., Dimuccio, V., Pastorino, C., Molina, J., O'Kelly, R., et al. (2020). Urinary extracellular vesicles carrying klotho improve the recovery of renal function in an acute tubular injury model. Mol. Ther. J. Am. Soc. Gene Ther. 28 (2), 490–502. doi:10.1016/j.ymthe.2019.11.013
Gu, D., Zou, X., Ju, G., Zhang, G., Bao, E., and Zhu, Y. (2016). Mesenchymal stromal cells derived extracellular vesicles ameliorate acute renal ischemia reperfusion injury by inhibition of mitochondrial fission through miR-30. Stem cells Int. 2016, 2093940. doi:10.1155/2016/2093940
Hogan, M., Johnson, K., Zenka, R., Charlesworth, M., Madden, B., Mahoney, D., et al. (2014). Subfractionation, characterization, and in-depth proteomic analysis of glomerular membrane vesicles in human urine. Kidney Int. 85 (5), 1225–1237. doi:10.1038/ki.2013.422
Jeppesen, D., Fenix, A., Franklin, J., Higginbotham, J., Zhang, Q., Zimmerman, L., et al. (2019). Reassessment of exosome composition. Cell 177 (2), 428–445. doi:10.1016/j.cell.2019.02.029
Ji, C., Zhang, J., Zhu, Y., Shi, H., Yin, S., Sun, F., et al. (2020). Exosomes derived from hucMSC attenuate renal fibrosis through CK1δ/β-TRCP-mediated YAP degradation. Cell death Dis. 11 (5), 327. doi:10.1038/s41419-020-2510-4
Jiang, D., Rosenkrans, Z., Ni, D., Lin, J., Huang, P., and Cai, W. (2020). Nanomedicines for renal management: From imaging to treatment. Accounts Chem. Res. 53 (9), 1869–1880. doi:10.1021/acs.accounts.0c00323
Jiang, Z., Liu, Y., Niu, X., Yin, J., Hu, B., Guo, S., et al. (2016). Exosomes secreted by human urine-derived stem cells could prevent kidney complications from type I diabetes in rats. Stem cell Res. Ther. 7, 24. doi:10.1186/s13287-016-0287-2
Jin, C., Wu, P., Li, L., Xu, W., and Qian, H. (2021). Exosomes: Emerging therapy delivery tools and biomarkers for kidney diseases. Stem Cells Int. 2021, 7844455. doi:10.1155/2021/7844455
Jin, J., Shi, Y., Gong, J., Zhao, L., Li, Y., He, Q., et al. (2019). Exosome secreted from adipose-derived stem cells attenuates diabetic nephropathy by promoting autophagy flux and inhibiting apoptosis in podocyte. Stem cell Res. Ther. 10 (1), 95. doi:10.1186/s13287-019-1177-1
Jin, J., Sun, H., Shi, C., Yang, H., Wu, Y., Li, W., et al. (2020). Circular RNA in renal diseases. J. Cell. Mol. Med. 24 (12), 6523–6533. doi:10.1111/jcmm.15295
Kalluri, R., and LeBleu, V. S. (2020). The biology, function, and biomedical applications of exosomes. Science 367 (6478), eaau6977. doi:10.1126/science.aau6977
Kellum, J., Romagnani, P., Ashuntantang, G., Ronco, C., Zarbock, A., and Anders, H. (2021). Acute kidney injury. Nat. Rev. Dis. Prim. 7 (1), 52. doi:10.1038/s41572-021-00284-z
Kholia, S., Herrera Sanchez, M., Cedrino, M., Papadimitriou, E., Tapparo, M., Deregibus, M., et al. (2020). Mesenchymal stem cell derived extracellular vesicles ameliorate kidney injury in aristolochic acid nephropathy. Front. cell Dev. Biol. 8, 188. doi:10.3389/fcell.2020.00188
Kholia, S., Herrera Sanchez, M., Deregibus, M., Sassoè-Pognetto, M., Camussi, G., and Brizzi, M. (2021). Human liver stem cell derived extracellular vesicles alleviate kidney fibrosis by interfering with the β-catenin pathway through miR29b. Int. J. Mol. Sci. 22 (19), 10780. doi:10.3390/ijms221910780
Khurana, R., Ranches, G., Schafferer, S., Lukasser, M., Rudnicki, M., Mayer, G., et al. (2017). Identification of urinary exosomal noncoding RNAs as novel biomarkers in chronic kidney disease. RNA (New York, N.Y.) 23 (2), 142–152. doi:10.1261/rna.058834.116
Kraińska, M., Pietrzkowska, N., Turlej, E., Zongjin, L., and Marycz, K. (2021). Extracellular vesicles derived from mesenchymal stem cells as a potential therapeutic agent in acute kidney injury (AKI) in felines: Review and perspectives. Stem cell Res. Ther. 12 (1), 504. doi:10.1186/s13287-021-02573-6
Lener, T., Gimona, M., Aigner, L., Börger, V., Buzas, E., Camussi, G., et al. (2015). Applying extracellular vesicles based therapeutics in clinical trials - an ISEV position paper. J. Extracell. vesicles 4, 30087. doi:10.3402/jev.v4.30087
Levey, A., Becker, C., and Inker, L. (2015). Glomerular filtration rate and albuminuria for detection and staging of acute and chronic kidney disease in adults: A systematic review. JAMA 313 (8), 837–846. doi:10.1001/jama.2015.0602
Lewington, A., Cerdá, J., and Mehta, R. (2013). Raising awareness of acute kidney injury: A global perspective of a silent killer. Kidney Int. 84 (3), 457–467. doi:10.1038/ki.2013.153
Li, J., Zhao, M., Xiang, X., He, Q., and Gui, R. (2021). A novel biomimetic nanomedicine system with anti-inflammatory and anti-osteoporosis effects improves the therapy efficacy of steroid-resistant nephrotic syndrome. J. nanobiotechnology 19 (1), 417. doi:10.1186/s12951-021-01165-z
Li, Z., Lv, L., Tang, T., Wang, B., Feng, Y., Zhou, L., et al. (2019). HIF-1α inducing exosomal microRNA-23a expression mediates the cross-talk between tubular epithelial cells and macrophages in tubulointerstitial inflammation. Kidney Int. 95 (2), 388–404. doi:10.1016/j.kint.2018.09.013
Lin, K. C., Yip, H. K., Shao, P. L., Wu, S. C., Chen, K. H., Chen, Y. T., et al. (2016). Combination of adipose-derived mesenchymal stem cells (ADMSC) and ADMSC-derived exosomes for protecting kidney from acute ischemia-reperfusion injury. Int. J. Cardiol. 216, 173–185. doi:10.1016/j.ijcard.2016.04.061
Liu, X., Miao, J., Wang, C., Zhou, S., Chen, S., Ren, Q., et al. (2020). Tubule-derived exosomes play a central role in fibroblast activation and kidney fibrosis. Kidney Int. 97 (6), 1181–1195. doi:10.1016/j.kint.2019.11.026
Liu, Y., Cui, J., Wang, H., Hezam, K., Zhao, X., Huang, H., et al. (2020). Enhanced therapeutic effects of MSC-derived extracellular vesicles with an injectable collagen matrix for experimental acute kidney injury treatment. Stem cell Res. Ther. 11 (1), 161. doi:10.1186/s13287-020-01668-w
Long, J., Zhang, Y., Liu, X., Pan, M., and Gao, Q. (2022). Exosomes in the field of neuroscience: A scientometric study and visualization analysis. Front. neurology 13, 871491. doi:10.3389/fneur.2022.871491
Lu, M., and Huang, Y. (2020). Bioinspired exosome-like therapeutics and delivery nanoplatforms. Biomaterials 242, 119925. doi:10.1016/j.biomaterials.2020.119925
Lv, L., Cao, Y., Ni, H., Xu, M., Liu, D., Liu, H., et al. (2013). MicroRNA-29c in urinary exosome/microvesicle as a biomarker of renal fibrosis. Am. J. physiology 305 (8), F1220–F1227. doi:10.1152/ajprenal.00148.2013
Lv, L., Feng, Y., Wen, Y., Wu, W., Ni, H., Li, Z., et al. (2018). Exosomal CCL2 from tubular epithelial cells is critical for albumin-induced tubulointerstitial inflammation. J. Am. Soc. Nephrol. JASN 29 (3), 919–935. doi:10.1681/asn.2017050523
Lv, L. L., Feng, Y., Tang, T. T., and Liu, B. C. (2019). New insight into the role of extracellular vesicles in kidney disease. J. Cell Mol. Med. 23 (2), 731–739. doi:10.1111/jcmm.14101
Lv, L. L., Wu, W. J., Feng, Y., Li, Z. L., Tang, T. T., and Liu, B. C. (2018). Therapeutic application of extracellular vesicles in kidney disease: Promises and challenges. J. Cell Mol. Med. 22 (2), 728–737. doi:10.1111/jcmm.13407
Martinez-Arroyo, O., Ortega, A., Forner, M., and Cortes, R. (2022). Mesenchymal stem cell-derived extracellular vesicles as non-coding RNA therapeutic vehicles in autoimmune diseases. Pharmaceutics 14 (4), 733. doi:10.3390/pharmaceutics14040733
Mazzariol, M., Camussi, G., and Brizzi, M. (2021). Extracellular vesicles tune the immune system in renal disease: A focus on systemic lupus erythematosus, antiphospholipid syndrome, thrombotic microangiopathy and ANCA-vasculitis. Int. J. Mol. Sci. 22 (8), 4194. doi:10.3390/ijms22084194
Merino, A., Hoogduijn, M., Borras, F., and Franquesa, M. (2014). Therapeutic potential of extracellular vesicles. Front. Immunol. 5, 658. doi:10.3389/fimmu.2014.00658
Miranda, K., Bond, D., McKee, M., Skog, J., Păunescu, T., Da Silva, N., et al. (2010). Nucleic acids within urinary exosomes/microvesicles are potential biomarkers for renal disease. Kidney Int. 78 (2), 191–199. doi:10.1038/ki.2010.106
Moon, P. G., Lee, J. E., You, S., Kim, T. K., Cho, J. H., Kim, I. S., et al. (2011). Proteomic analysis of urinary exosomes from patients of early IgA nephropathy and thin basement membrane nephropathy. Proteomics 11 (12), 2459–2475. doi:10.1002/pmic.201000443
Murray, R., Zimmerman, T., Agarwal, A., Palevsky, P., Quaggin, S., Rosas, S., et al. (2021). Kidney-related research in the United States: A position statement from the national kidney foundation and the American society of nephrology. Am. J. Kidney Dis. 78 (2), 161–167. doi:10.1053/j.ajkd.2021.04.006
Nargesi, A. A., Lerman, L. O., and Eirin, A. (2017). Mesenchymal stem cell-derived extracellular vesicles for renal repair. Curr. Gene Ther. 17 (1), 29–42. doi:10.2174/1566523217666170412110724
Olivares, D., Perez-Hernandez, J., Forner, M., Perez-Soriano, C., Tormos, M., Saez, G., et al. (2018). Urinary levels of sirtuin-1 associated with disease activity in lupus nephritis. Clin. Sci. 132 (5), 569–579. doi:10.1042/cs20171410
Olivares, D., Perez-Hernandez, J., Perez-Gil, D., Chaves, F., Redon, J., and Cortes, R. (2020). Optimization of small RNA library preparation protocol from human urinary exosomes. J. Transl. Med. 18 (1), 132. doi:10.1186/s12967-020-02298-9
Ortega, A., Martinez-Arroyo, O., Forner, M., and Cortes, R. (2020). Exosomes as drug delivery systems: Endogenous nanovehicles for treatment of systemic lupus erythematosus. Pharmaceutics 13 (1), 3. doi:10.3390/pharmaceutics13010003
Perez-Hernandez, J., and Cortes, R. (2015). Extracellular vesicles as biomarkers of systemic lupus erythematosus. Dis. markers 2015, 613536. doi:10.1155/2015/613536
Perez-Hernandez, J., Forner, M., Pinto, C., Chaves, F., Cortes, R., and Redon, J. (2015). Increased urinary exosomal MicroRNAs in patients with systemic lupus erythematosus. PloS one 10 (9), e0138618. doi:10.1371/journal.pone.0138618
Perez-Hernandez, J., Martinez-Arroyo, O., Ortega, A., Galera, M., Solis-Salguero, M., Chaves, F., et al. (2021). Urinary exosomal miR-146a as a marker of albuminuria, activity changes and disease flares in lupus nephritis. J. Nephrol. 34 (4), 1157–1167. doi:10.1007/s40620-020-00832-y
Perez-Hernandez, J., Olivares, D., Forner, M., Ortega, A., Solaz, E., Martinez, F., et al. (2018). Urinary exosome miR-146a is a potential marker of albuminuria in essential hypertension. J. Transl. Med. 16 (1), 228. doi:10.1186/s12967-018-1604-6
Perez-Hernandez, J., Olivares, M., Forner, M., Chaves, F., Cortes, R., and Redon, J. (2016). Urinary dedifferentiated podocytes as a non-invasive biomarker of lupus nephritis. Nephrol. Dial. Transplant. 31 (5), 780–789. doi:10.1093/ndt/gfw002
Perez-Hernandez, J., Olivares, M., Solaz, E., Martinez, F., Martínez-Hervas, S., Pichler, G., et al. (2018). Urinary podocyte-associated molecules and albuminuria in hypertension. J. Hypertens. 36 (8), 1712–1718. doi:10.1097/hjh.0000000000001747
Perez-Hernandez, J., Redon, J., and Cortes, R. (2017). Extracellular vesicles as therapeutic agents in systemic lupus erythematosus. Int. J. Mol. Sci. 18 (4), 717. doi:10.3390/ijms18040717
Perez-Hernandez, J., Riffo-Campos, A., Ortega, A., Martinez-Arroyo, O., Perez-Gil, D., Olivares, D., et al. (2021). Urinary- and plasma-derived exosomes reveal a distinct MicroRNA signature associated with albuminuria in hypertension. Hypertension 77 (3), 960–971. doi:10.1161/hypertensionaha.120.16598
Pisitkun, T., Shen, R., and Knepper, M. (2004). Identification and proteomic profiling of exosomes in human urine. Proc. Natl. Acad. Sci. U. S. A. 101 (36), 13368–13373. doi:10.1073/pnas.0403453101
Ramírez-Bajo, M., Martín-Ramírez, J., Bruno, S., Pasquino, C., Banon-Maneus, E., Rovira, J., et al. (2020). Nephroprotective potential of mesenchymal stromal cells and their extracellular vesicles in a murine model of chronic cyclosporine nephrotoxicity. Front. cell Dev. Biol. 8, 296. doi:10.3389/fcell.2020.00296
Ranghino, A., Bruno, S., Bussolati, B., Moggio, A., Dimuccio, V., Tapparo, M., et al. (2017). The effects of glomerular and tubular renal progenitors and derived extracellular vesicles on recovery from acute kidney injury. Stem Cell Res. Ther. 8 (1), 24. doi:10.1186/s13287-017-0478-5
Raposo, G., and Stoorvogel, W. (2013). Extracellular vesicles: Exosomes, microvesicles, and friends. J. cell Biol. 200 (4), 373–383. doi:10.1083/jcb.201211138
Redon, J., Selma-Soriano, E., and Cortes, R. (2021). Insights into glomerular filtration and albuminuria. Reply. N. Engl. J. Med. 385 (5), 478–8. doi:10.1056/NEJMc2108129
Rood, I., Deegens, J., Merchant, M., Tamboer, W., Wilkey, D., Wetzels, J., et al. (2010). Comparison of three methods for isolation of urinary microvesicles to identify biomarkers of nephrotic syndrome. Kidney Int. 78 (8), 810–816. doi:10.1038/ki.2010.262
Rutman, A. K., Negi, S., Gasparrini, M., Hasilo, C. P., Tchervenkov, J., and Paraskevas, S. (2018). Immune response to extracellular vesicles from human islets of langerhans in patients with type 1 diabetes. Endocrinology 159 (11), 3834–3847. doi:10.1210/en.2018-00649
Shao, B., Qin, Y. F., Ren, S. H., Peng, Q. F., Qin, H., Wang, Z. B., et al. (2022). Structural and temporal dynamics of mesenchymal stem cells in liver diseases from 2001 to 2021: A bibliometric analysis. Front. Immunol. 13, 859972. doi:10.3389/fimmu.2022.859972
Solé, C., Cortés-Hernández, J., Felip, M. L., Vidal, M., and Ordi-Ros, J. (2015). miR-29c in urinary exosomes as predictor of early renal fibrosis in lupus nephritis. Nephrol. Dial. Transpl. 30 (9), 1488–1496. doi:10.1093/ndt/gfv128
Sun, X., Meng, H., Wan, W., Xie, M., and Wen, C. (2019). Application potential of stem/progenitor cell-derived extracellular vesicles in renal diseases. Stem cell Res. Ther. 10 (1), 8. doi:10.1186/s13287-018-1097-5
Synnestvedt, M. B., Chen, C., and Holmes, J. H. (2005). CiteSpace II: Visualization and knowledge discovery in bibliographic databases. AMIA Annu. Symp. Proc. 2005, 724–728.
Tang, T., Lv, L., Wang, B., Cao, J., Feng, Y., Li, Z., et al. (2019). Employing macrophage-derived microvesicle for kidney-targeted delivery of dexamethasone: An efficient therapeutic strategy against renal inflammation and fibrosis. Theranostics 9 (16), 4740–4755. doi:10.7150/thno.33520
Tang, T., Wang, B., Li, Z., Wen, Y., Feng, S., Wu, M., et al. (2021). Kim-1 targeted extracellular vesicles: A new therapeutic platform for RNAi to treat AKI. J. Am. Soc. Nephrol. JASN 32 (10), 2467–2483. doi:10.1681/asn.2020111561
Tang, T., Wang, B., Lv, L., Dong, Z., and Liu, B. (2022). Extracellular vesicles for renal therapeutics: State of the art and future perspective. J. Control. release official J. Control. Release Soc. 349, 32–50. doi:10.1016/j.jconrel.2022.06.049
Tang, T., Wang, B., Lv, L., and Liu, B. (2020). Extracellular vesicle-based Nanotherapeutics: Emerging frontiers in anti-inflammatory therapy. Theranostics 10 (18), 8111–8129. doi:10.7150/thno.47865
Tang, T., Wang, B., Wu, M., Li, Z., Feng, Y., Cao, J., et al. (2020). Extracellular vesicle-encapsulated IL-10 as novel nanotherapeutics against ischemic AKI. Sci. Adv. 6 (33), eaaz0748. doi:10.1126/sciadv.aaz0748
Tang, T. T., and Liu, B. C. (2019). Extracellular vesicles: Opportunities and challenges for the treatment of renal fibrosis. Adv. Exp. Med. Biol. 1165, 693–709. doi:10.1007/978-981-13-8871-2_34
Tang, T. T., Lv, L. L., Lan, H. Y., and Liu, B. C. (2019). Extracellular vesicles: Opportunities and challenges for the treatment of renal diseases. Front. Physiol. 10, 226. doi:10.3389/fphys.2019.00226
Tang, T. T., Lv, L. L., Wang, B., Cao, J. Y., Feng, Y., Li, Z. L., et al. (2019). Employing macrophage-derived microvesicle for kidney-targeted delivery of dexamethasone: An efficient therapeutic strategy against renal inflammation and fibrosis. Theranostics 9 (16), 4740–4755. doi:10.7150/thno.33520
Tang, T. T., Wang, B., Lv, L. L., and Liu, B. C. (2020). Extracellular vesicle-based Nanotherapeutics: Emerging frontiers in anti-inflammatory therapy. Theranostics 10 (18), 8111–8129. doi:10.7150/thno.47865
Teles, R. H. G., Yano, R. S., Villarinho, N. J., Yamagata, A. S., Jaeger, R. G., Meybohm, P., et al. (2021). Advances in breast cancer management and extracellular vesicle research, a bibliometric analysis. Curr. Oncol. 28 (6), 4504–4520. doi:10.3390/curroncol28060382
Tetta, C., Deregibus, M., and Camussi, G. (2020). Stem cells and stem cell-derived extracellular vesicles in acute and chronic kidney diseases: Mechanisms of repair. Ann. Transl. Med. 8 (8), 570. doi:10.21037/atm.2020.03.19
Théry, C., Amigorena, S., Raposo, G., and Clayton, A. (2006). Isolation and characterization of exosomes from cell culture supernatants and biological fluids. Curr. Protoc. Cell Biol. 3, 22. doi:10.1002/0471143030.cb0322s30
Théry, C., Ostrowski, M., and Segura, E. (2009). Membrane vesicles as conveyors of immune responses. Nat. Rev. Immunol. 9 (8), 581–593. doi:10.1038/nri2567
Théry, C., Witwer, K., Aikawa, E., Alcaraz, M., Anderson, J., Andriantsitohaina, R., et al. (2018). Minimal information for studies of extracellular vesicles 2018 (MISEV2018): A position statement of the international society for extracellular vesicles and update of the MISEV2014 guidelines. J. Extracell. vesicles 7 (1), 1535750. doi:10.1080/20013078.2018.1535750
Théry, C., Zitvogel, L., and Amigorena, S. (2002). Exosomes: Composition, biogenesis and function. Nat. Rev. Immunol. 2 (8), 569–579. doi:10.1038/nri855
Thongboonkerd, V. (2019). Roles for exosome in various kidney diseases and disorders. Front. Pharmacol. 10, 1655. doi:10.3389/fphar.2019.01655
Tkach, M., and Théry, C. (2016). Communication by extracellular vesicles: Where we are and where we need to go. Cell 164 (6), 1226–1232. doi:10.1016/j.cell.2016.01.043
Valadi, H., Ekström, K., Bossios, A., Sjöstrand, M., Lee, J. J., and Lötvall, J. O. (2007). Exosome-mediated transfer of mRNAs and microRNAs is a novel mechanism of genetic exchange between cells. Nat. Cell Biol. 9 (6), 654–659. doi:10.1038/ncb1596
van Balkom, B., Pisitkun, T., Verhaar, M., and Knepper, M. (2011). Exosomes and the kidney: Prospects for diagnosis and therapy of renal diseases. Kidney Int. 80 (11), 1138–1145. doi:10.1038/ki.2011.292
van Eck, N. J., and Waltman, L. (2010). Software survey: VOSviewer, a computer program for bibliometric mapping. Scientometrics 84 (2), 523–538. doi:10.1007/s11192-009-0146-3
van Niel, G., D'Angelo, G., and Raposo, G. (2018). Shedding light on the cell biology of extracellular vesicles. Nat. Rev. Mol. cell Biol. 19 (4), 213–228. doi:10.1038/nrm.2017.125
van Zonneveld, A., Kölling, M., Bijkerk, R., and Lorenzen, J. (2021). Circular RNAs in kidney disease and cancer. Nat. Rev. Nephrol. 17 (12), 814–826. doi:10.1038/s41581-021-00465-9
Vanholder, R., Conway, P., Gallego, D., Scheres, E., and Wieringa, F. (2022). The European kidney health alliance (EKHA) and the decade of the KidneyTM. Nephrol. Dial. transplan 2022, gfac211. doi:10.1093/ndt/gfac211
Viñas, J., Burger, D., Zimpelmann, J., Haneef, R., Knoll, W., Campbell, P., et al. (2016). Transfer of microRNA-486-5p from human endothelial colony forming cell-derived exosomes reduces ischemic kidney injury. Kidney Int. 90 (6), 1238–1250. doi:10.1016/j.kint.2016.07.015
Wang, B., Wang, Z. M., Ji, J. L., Gan, W., Zhang, A., Shi, H. J., et al. (2020). Macrophage-derived exosomal mir-155 regulating cardiomyocyte pyroptosis and hypertrophy in uremic cardiomyopathy. JACC Basic Transl. Sci. 5 (2), 148–166. doi:10.1016/j.jacbts.2019.10.011
Wang, B., Yao, K., Huuskes, B. M., Shen, H. H., Zhuang, J., Godson, C., et al. (2016). Mesenchymal stem cells deliver exogenous MicroRNA-let7c via exosomes to attenuate renal fibrosis. Mol. Ther. 24 (7), 1290–1301. doi:10.1038/mt.2016.90
Wang, B., Zhang, A., Wang, H., Klein, J. D., Tan, L., Wang, Z. M., et al. (2019). miR-26a limits muscle wasting and cardiac fibrosis through exosome-mediated microRNA transfer in chronic kidney disease. Theranostics 9 (7), 1864–1877. doi:10.7150/thno.29579
Wang, H., Wang, B., Zhang, A., Hassounah, F., Seow, Y., Wood, M., et al. (2019). Exosome-mediated miR-29 transfer reduces muscle atrophy and kidney fibrosis in mice. Mol. Ther. J. Am. Soc. Gene Ther. 27 (3), 571–583. doi:10.1016/j.ymthe.2019.01.008
Wang, S., Qiu, Z., Chen, F., Mao, A., Bai, J., Hong, Y., et al. (2022). Bone marrow mesenchymal stem cell-derived extracellular vesicles containing miR-181d protect rats against renal fibrosis by inhibiting KLF6 and the NF-κB signaling pathway. Cell death Dis. 13 (6), 535. doi:10.1038/s41419-022-04875-w
Xie, M., Xiong, W., She, Z., Wen, Z., Abdirahman, A., Wan, W., et al. (2020). Immunoregulatory effects of stem cell-derived extracellular vesicles on immune cells. Front. Immunol. 11, 13. doi:10.3389/fimmu.2020.00013
Yáñez-Mó, M., Siljander, P. R., Andreu, Z., Zavec, A. B., Borràs, F. E., Buzas, E. I., et al. (2015). Biological properties of extracellular vesicles and their physiological functions. J. Extracell. Vesicles 4, 27066. doi:10.3402/jev.v4.27066
Yang, L., Patel, K., Rathnam, C., Thangam, R., Hou, Y., Kang, H., et al. (2022). Harnessing the therapeutic potential of extracellular vesicles for biomedical applications using multifunctional magnetic nanomaterials. Small, e2104783. doi:10.1002/smll.202104783
Zhang, C., Shang, Y., Chen, X., Midgley, A., Wang, Z., Zhu, D., et al. (2020). Supramolecular nanofibers containing arginine-glycine-aspartate (RGD) peptides boost therapeutic efficacy of extracellular vesicles in kidney repair. ACS Nano 14 (9), 12133–12147. doi:10.1021/acsnano.0c05681
Zhang, X., Lu, Y., Wu, S., Zhang, S., Li, S., and Tan, J. (2022). An overview of current research on mesenchymal stem cell-derived extracellular vesicles: A bibliometric analysis from 2009 to 2021. Front. Bioeng. Biotechnol. 10, 910812. doi:10.3389/fbioe.2022.910812
Zhou, H., Cheruvanky, A., Hu, X., Matsumoto, T., Hiramatsu, N., Cho, M. E., et al. (2008). Urinary exosomal transcription factors, a new class of biomarkers for renal disease. Kidney Int. 74 (5), 613–621. doi:10.1038/ki.2008.206
Zhou, H., Pisitkun, T., Aponte, A., Yuen, P., Hoffert, J., Yasuda, H., et al. (2006). Exosomal fetuin-A identified by proteomics: A novel urinary biomarker for detecting acute kidney injury. Kidney Int. 70 (10), 1847–1857. doi:10.1038/sj.ki.5001874
Zhou, H., Yuen, P., Pisitkun, T., Gonzales, P., Yasuda, H., Dear, J., et al. (2006). Collection, storage, preservation, and normalization of human urinary exosomes for biomarker discovery. Kidney Int. 69 (8), 1471–1476. doi:10.1038/sj.ki.5000273
Keywords: kidney disease, EVs, exosomes, acute kidney disease, chronic kidney disease, bibliometric analysis
Citation: Hun M, Wen H, Han P, Vun T, Zhao M and He Q (2023) Bibliometric analysis of scientific papers on extracellular vesicles in kidney disease published between 1999 and 2022. Front. Cell Dev. Biol. 10:1070516. doi: 10.3389/fcell.2022.1070516
Received: 14 October 2022; Accepted: 09 December 2022;
Published: 05 January 2023.
Edited by:
Yang-Mooi Lim, Universiti Tunku Abdul Rahman, MalaysiaReviewed by:
Giulia Cricrì, University of Milan, ItalyAlexander N. Kapustin, AstraZeneca, United Kingdom
Copyright © 2023 Hun, Wen, Han, Vun, Zhao and He. This is an open-access article distributed under the terms of the Creative Commons Attribution License (CC BY). The use, distribution or reproduction in other forums is permitted, provided the original author(s) and the copyright owner(s) are credited and that the original publication in this journal is cited, in accordance with accepted academic practice. No use, distribution or reproduction is permitted which does not comply with these terms.
*Correspondence: Mingyi Zhao, emhhb19taW5neWlAY3N1LmVkdS5jbg==; Qingnan He, aGVxbjI2MjlAY3N1LmVkdS5jbg==